- 1Department of Neurology, The First Affiliated Hospital of Guangxi Medical University, Nanning, China
- 2Department of Comprehensive Internal Medicine, Guangxi Medical University Affiliated Tumor Hospital, Nanning, China
- 3Department of Radiology, The First Affiliated Hospital of Guangxi Medical University, Nanning, China
- 4Department of Psychiatry, National Clinical Research Center for Mental Disorders, The Second Xiangya Hospital of Central South University, Changsha, China
Background: Blepharospasm (BSP) and dry eye disease (DED) are clinically common diseases characterized by an increased blinking rate. A sustained eyelid muscle activity may alter the cortical sensorimotor concordance and lead to secondary functional changes. This study aimed to explore the central mechanism of BSP by assessing brain functional differences between the two groups and comparing them with healthy controls.
Methods: In this study, 25 patients with BSP, 22 patients with DED, and 23 healthy controls underwent resting-state functional magnetic resonance imaging (fMRI) scan. The amplitude of low-frequency fluctuations (ALFF) was applied to analyze the imaging data.
Results: Analysis of covariance (ANCOVA) revealed widespread differences in ALFF across the three groups. In comparison with healthy controls, patients with BSP showed abnormal ALFF in the sensorimotor integration related-brain regions, including the bilateral supplementary motor area (SMA), left cerebellar Crus I, left fusiform gyrus, bilateral superior medial prefrontal cortex (MPFC), and right superior frontal gyrus (SFG). In comparison with patients with DED, patients with BSP exhibited a significantly increased ALFF in the left cerebellar Crus I and left SMA. ALFF in the left fusiform gyrus/cerebellar Crus I was positively correlated with symptomatic severity of BSP.
Conclusions: Our results reveal that the distinctive changes in the brain function in patients with BSP are different from those in patients with DED and healthy controls. The results further emphasize the primary role of sensorimotor integration in the pathophysiology of BSP.
Introduction
Characterized by excessive involuntary spasms of the orbicularis oculi, blepharospasm (BSP) is now recognized as a common form of adult-onset focal dystonia (1, 2). As disease progresses, it may result in difficulty in opening the eyes or even functional blindness, which causes functional disability in work and daily life and decreases quality of life (3–5). Though the symptomatology of this disease is well-defined, its pathophysiology remains unclear. In addition, the onset of BSP is insidious and mainly characterized by atypical symptoms such as increasing blinking and photophobia in early stages (6). These early symptoms of patients with BSP are very similar to the manifestations of dry eye disease (DED), which is considered a peripheral disease associated with tear hyperosmolarity and ocular surface inflammation (7). Typical manifestations of DED include photophobia, burning sensation, dryness of the eyes, and visual impairment. These unpleasant sensations in DED often initiated secondary increased blink rate. This might be a result of increased reflex non-spasmodic closure of the eyelids to the sensory symptoms other than the involuntary orbicularis oculi spasms in BSP. It is usually difficult to differentiate BSP in early stages from DED merely according to clinical symptoms. Differences in neural activity between the two diseases remain unclear, and sustained muscular activity might alter the cortical sensory-motor concordance directly or indirectly, leading to secondary structural or functional changes that are almost indistinguishable from the underlying pathophysiological characteristics of BSP. It is necessary to identify the potential central effects of peripheral sensory feedback.
Although BSP was initially believed to be a disease of the basal ganglia, neuroimaging evidence suggested that it involved the anatomy and function of several brain regions (8, 9). Promising neuroimaging techniques have provided new methods for analyzing changes in brain function and structure, thereby expanding the focus of traditional research. Structurally, voxel-based morphometry analysis has shown an increased gray matter density in the cingulate gyrus, primary sensorimotor cortex, and right middle frontal gyrus, indicating that multiple regions contribute to the development of BSP (10, 11). Functionally, many fMRI trials on BSP have revealed abnormal regional spontaneous brain activities and altered functional connectivity in sensorimotor structures, including the basal ganglia, SMA, cerebellum, primary sensorimotor cortex, and visual areas (12–16). These studies have demonstrated that local microstructural and functional abnormalities in multiple regions may be associated with BSP, thus bringing forward the viewpoint that BSP might be a network disorder (17). However, these functional and structural changes in BSP are unable to definitely divide into primary or secondary. Whether imaging abnormalities in these areas are characteristic spontaneous changes in BSP or secondary central effects caused by an increased blinking rate should be further investigated. Moreover, little is known about brain activity alterations in DED with commonly recognized peripheral mechanisms. Both disease situations present with similar clinical manifestations, especially increased blinking. To improve the understanding of spasmodic conditions, specific functional changes of BSP should be distinguished from possible confounding effects through dialectical comparative analysis. Therefore, DED may be used as controls to investigate the neural mechanisms of BSP.
In early studies about BSP, an activation paradigm evoked by simple motor tasks was used. However, different abnormalities have shown in the task-based fMRI studies during different task performance (18–20). The lack of a unified stimulus paradigm may make it difficult to support each other across the existent task-based studies. Besides, individual differences may activate the unrelated brain regions during performing the motor task. In contrast to task-based fMRI, increasing attention has been paid to resting-state fMRI (rs-fMRI), a promising neuroimaging technique that can examine brain neurophysiological processes without the requirement of specific task execution based on the fluctuation of the blood-oxygen-level dependent signal. The technique can avoid the potential limitations of task-based fMRI and contributes to further understanding of abnormalities of neural activity. As an effective analytical method of rs-fMRI technique, amplitude of low-frequency fluctuations (ALFF) is widely utilized to identify functional changes in brain regions at rest (21–24). In the present study, we used the ALFF method to explore the different types of alterations in the spontaneous regional brain activity among patients with BSP, patients with DED, and healthy controls. We also examined correlations between abnormal ALFF and clinical variables in patients with BSP. We hypothesized that ALFF alterations in BSP would be distinguishable from those in patients with DED and healthy subjects.
Methods and Materials
Participants
All participants in the present study volunteered to take part in the study and signed a written consent after they were informed of the research process. The study received ethics approval by the Ethics Committee of the First Affiliated Hospital of Guangxi Medical University. Patients with BSP and DED were treated at the Department of Neurology in the First Affiliated Hospital of Guangxi Medical University from October 2017 to October 2019.
Patients With BSP
Inclusion criteria: (1) blepharospasm in accordance with clinical diagnostic criteria (25); (2) no organic changes in conventional head MRI; (3) no other serious neurological or mental diseases; (4) not received botulinum toxin treatment or any medication for dystonia or mental illness within 3 months before enrollment; and (5) right-handedness. Exclusion criteria: (1) abnormal intracranial lesions found via MRI; (2) dry eye, eyelid aphasia, or severe systemic diseases, such as hepatolenticular degeneration; (3) previous history of neurological and mental diseases; and (4) inability to cooperate with or contraindicate MRI examination.
Patients With DED
Inclusion criteria: (1) conform to the diagnostic criteria in accordance with the TFOS DEWS II Definition and Classification Report (26); (2) no accompany blepharospasm; (3) not received botulinum toxin, and without any drugs for dystonia or mental illness within 3 months before enrollment; and (4) right-handedness. Exclusion criteria: (1) orbital organic lesions confirmed by orbital CT examination; (2) head and facial trauma or operation history and neuropsychiatric system diseases; (3) abnormal intracranial lesions found through imaging examination; and (4) inability to cooperate with or unsuitable for MRI examination.
Healthy Controls
Healthy adults from the local community were recruited as healthy controls and matched with age and education with subjects with BSP and patients with DED. Inclusion criteria: (1) no neurological and mental system diseases; (2) no abnormality in ophthalmology and nervous system examination; (3) no abnormality in head imaging examination; and (4) right-handedness.
Resting-State fMRI Images Acquisition
Images were collected by the same skilled technician using a Siemens 3T MR scanner in the First Affiliated Hospital of Guangxi Medical University. All subjects were given head retainers and earplugs to minimize head movement and machine noise. During scanning, all participants were required to relax and minimize head and body movement as much as possible, while staying awake with eyes closed. An echo-planar imaging sequence was used to acquire resting-state fMRI images with the following parameters: repetition time/echo time = 2,000 ms/30 ms, matrix size = 64 × 64, slices number = 30, flip angle = 90°, field of view = 24 cm, gap = 0.4 mm, slice thickness = 4 mm, and 250 volumes (500 s).
Clinical information, including age, gender, illness duration (except healthy control groups), education level, and BSP symptomatic severity, were collected. The symptom severity of patients with BSP was assessed by professional neurologists utilizing the Jankovic Rating Scale (JRS) (27). In addition, patients with BSP were assessed in terms of their depression and anxiety symptoms by using, respectively, the self-rating depression scale (SDS) and self-rating anxiety scale (SAS).
Data Preprocessing
Data Processing Assistant for Resting-State fMRI (DPARSF) software package based on MatlabR2012b platform was used to perform data preprocessing. First, images of the first 10 time points were eliminated, and the remaining 240 images were retained for analysis. Time layer and head motion were corrected to eliminate data whose head movement horizontal displacement was >2 mm and rotation was >2°. Next, the images were spatially normalized to the Montreal Neurological Institute (MNI) EPI template and resampled to 3 mm × 3 mm × 3 mm in the statistical parameter map software (SPM12, http://www.fil.ion.ucl.ac.uk/spm). Afterward, the processed image was spatially smoothened with a 4 mm full width at half maximum Gaussian kernel. Finally, linear drifts removal and temporal band-pass filtering (0.01–0.08 Hz) were conducted on all smoothed images to remove the influence of low-frequency drift and high-frequency respiratory and cardiac noise.
ALFF Analysis
The REST (http://resting-fmri.sourceforge.net) software was used to analyze ALFF (28). ALFF is the sum of the low-frequency spectral amplitudes across 0.01–0.08 Hz. First, the time series of each voxel was transformed to the frequency domain by utilizing a fast Fourier transform and the power spectrum was obtained. Then, the square root of each frequency on the power spectrum was calculated, and the mean square root across 0.01–0.08 Hz at each voxel was taken as the ALFF (29). For data standardization, the ALFF of each voxel was divided by the global mean ALFF value.
Statistical Analysis
Demographic data, such as gender, age, and education level, were statistically analyzed using IBM SPSS 23.0 software. Analyses of variance (ANOVA) were conducted to compare age and education level across the three groups, and gender differences were compared via a chi-square test. A two-sample t-test was used to compare illness duration between the BSP group and the DED group. The threshold of above tests was set at p < 0.05 that indicated statistically significant differences.
ANCOVA was performed to compare the ALFF images across three groups with the REST software. Subsequently, post-hoc t-tests were carried out to examine ALFF differences between each pair of groups. Age, gender, and education were used as covariates to reduce their potential impact. The significance threshold was corrected to p < 0.05 for multiple comparisons using Gaussian random field (GRF) theory (voxel significance: p < 0.001, cluster significance: p < 0.05).
The mean ALFF values were extracted from abnormal brain regions showing significant differences in BSP group and DED group by between-group comparisons for further correlation analysis.
Pearson correlation or Spearman correlation analyses were performed to examine the association of ALFF values and clinical variables in patients with BSP and patients with DED when apposite. Significance level was set at p < 0.05 (corrected for Bonferroni correction).
Results
Demographics and Clinical Information
A total of 70 participants were included in the study: 25 patients with BSP, 22 patients with DED, and 23 healthy controls. Demographics and clinical characteristics of these participants are shown in Table 1. There was no significant difference in age and education level across the three groups. Moreover, we observed that 20 patients of BSP (80.00%) exhibited sensory tricks, a characteristic feature in primary dystonia. That is, stimulus such as touching the cheeks and wearing glasses could temporarily alleviate the symptom of eyelid spasms.
Group Differences in ALFF
As shown in Figure 1 and Supplementary Figure 1, the ALFF values were significantly different in the frontal and cerebellar regions across the three groups by ANCOVA.
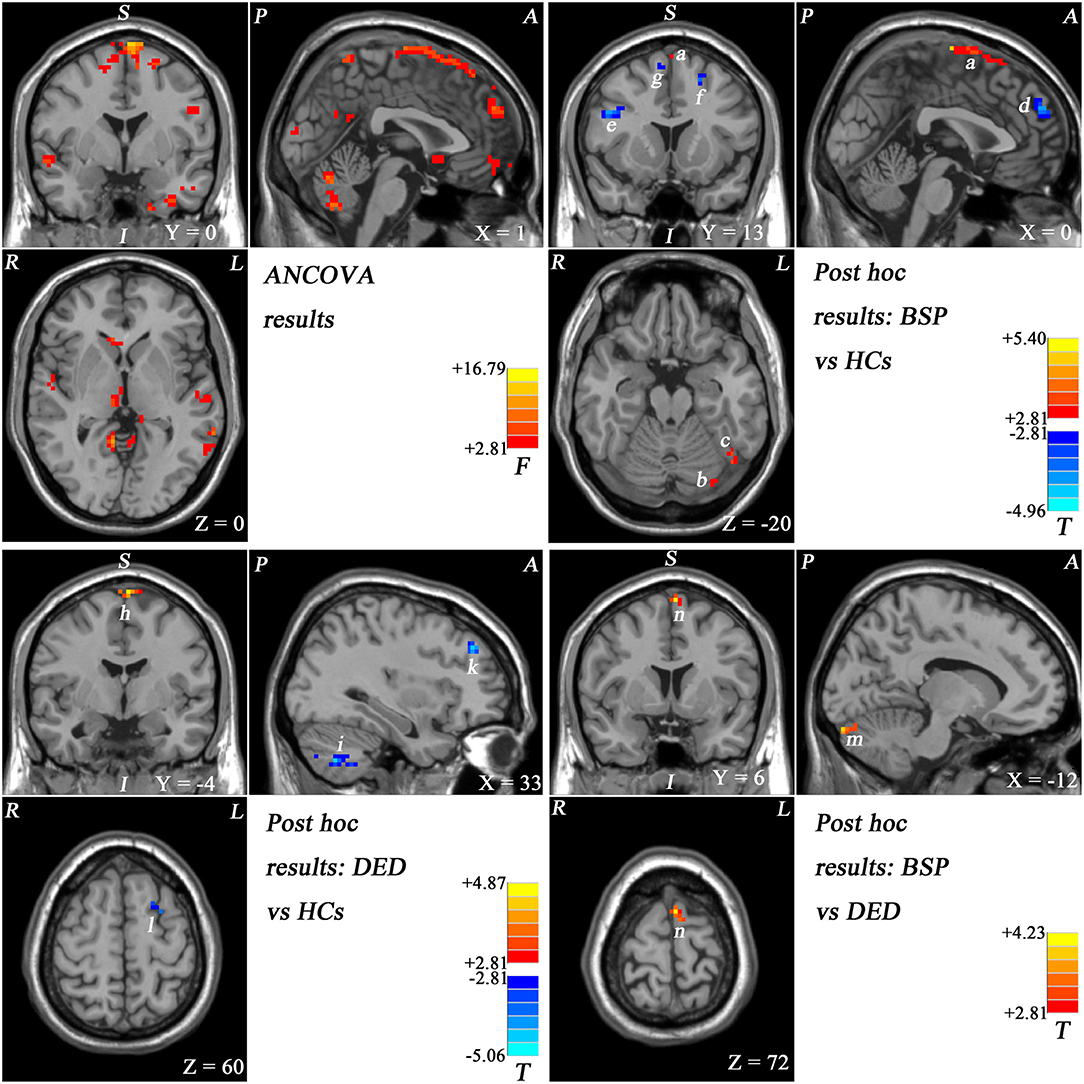
Figure 1. Statistical maps showing ALFF values differences in different brain regions across studied groups. Red and blue represent higher and lower ALFF, respectively. The color bars indicate the F-values from the ANOVA analyses and the T-values from post-hoc analysis between each pair of groups. (a) Bilateral SMA, (b) left cerebellar crus I, (c) left fusiform gyrus/cerebellar crus I, (d) bilateral superior MPFC, (e) right inferior frontal gyrus, (f) left middle frontal gyrus, (g) right superior frontal gyrus, (h) bilateral SMA, (i) right cerebellar VIII/VIIb, (k) right middle frontal gyrus, (l) left middle frontal gyrus, (m) left cerebellar crus I, and (n) left SMA.
Patients with BSP showed increased ALFF in the bilateral SMA, left cerebellar Crus I, and left fusiform gyrus/cerebellar Crus I and decreased ALFF in the superior part of the bilateral MPFC, right SFG, left middle frontal gyrus, and right inferior frontal gyrus compared with healthy controls (Figure 1; Supplementary Figure 2; Table 2).
Patients with DED exhibited increased ALFF in the bilateral SMA and decreased ALFF in the bilateral cerebellar VIII/VIIb, left middle frontal gyrus, and right middle frontal gyrus compared with that of healthy controls (Figure 1; Supplementary Figure 3; Table 2).
Compared with patients with DED, patients with BSP showed significantly increased ALFF in the left SMA and left cerebellar Crus I (Figure 1; Supplementary Figure 4; Table 2).
Correlation Analysis
A significantly positive correlation was observed between ALFF values in the left fusiform gyrus/cerebellar Crus I and symptomatic severity (r = 0.444, p = 0.026) in patients with BSP by using a Spearman correlation analysis (Figure 2). No significant correlation was revealed between abnormal ALFF and other clinical variables of patients with BSP. No correlation between abnormal ALFF and clinical variables was observed in the subjects with DED.
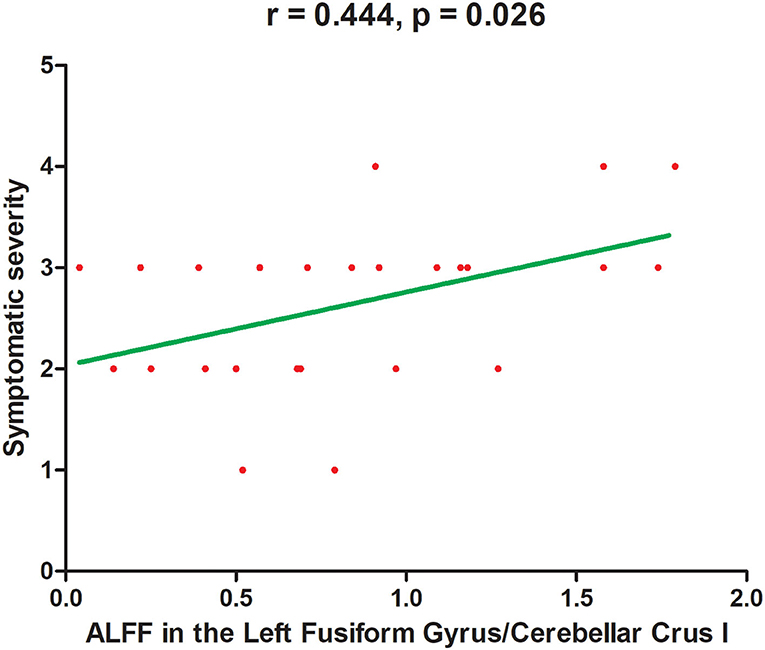
Figure 2. A significantly positive correlation between symptomatic severity and ALFF values in the left fusiform gyrus/cerebellar crus I in patients with BSP. ALFF, amplitude of low-frequency fluctuations. X-axis represents ALFF z-score. Y-axis represents symptomatic severity.
Discussion
The present study was the first to explore functional changes in brain regions of patients with BSP, patients with DED, and healthy controls by measuring ALFF of fMRI signals at rest. Abnormal ALFF values were located in the sensorimotor integration related brain regions in patients with BSP, including the bilateral SMA, left cerebellar Crus I, left fusiform gyrus, bilateral superior MPFC, and right SFG compared with healthy controls. Patients with BSP exhibited significantly increased ALFF in the left cerebellar Crus I and the left SMA compared with patients with DED. In addition, ALFF in the left fusiform gyrus/cerebellar Crus I was positively correlated with the symptomatic severity of BSP.
Dystonia was previously described as a movement disorder resulting from basal ganglion dysfunction, but this description has been remarkably revised, and the role of sensorimotor integration in the pathophysiology of dystonia has been gradually recognized (30–33). In general, sensorimotor integration refers to all processes that sensory information is used to plan and carry out volitional movements, and the sensory counterparts of each implemented movement (34). Indeed, patients with dystonia exhibit not only motor symptoms but also some sensory field disorders, including abnormalities in sensory and perceptual functions (35–38). For example, patients with BSP often complain to have photophobia and other ocular discomforts, and neck pain is frequently associated with cervical dystonia (39, 40). In addition, a well-known clinical feature of dystonia is the sensory trick that can alleviate dystonic symptoms in some ways (41–44). Similarly, touching certain areas of the face, wearing colored glasses, or singing can relieve the spasm symptom in patients with BSP (45, 46). In our study, 20 patients of BSP (80.00%) presented with sensory tricks, which might be the adjustment of motor output through the modification of sensory input. Sensorimotor integration is a complex neural activity involving multiple brain regions and circuits, most of which are component parts of the sensorimotor network (SMN) (47). This network mainly involves action execution and sensory processing, and the SMA is one of the key nodes (48). The SMA receives signals from the basal ganglia and cerebellum and then delivers them to primary motor cortex to coordinate motion execution instructions and participate in sensorimotor integration (36, 49, 50). Many structural and functional MRI studies on dystonia, including BSP, have usually revealed abnormalities in the SMA, and animal models of dystonia have shown overexcitability and increased proprioceptive input in the SMA (51–54). In our study, high ALFF was observed in the bilateral SMA in patients with BSP and patients with DED, and this result might be caused by some interference from peripheral paresthesia components. However, patients with BSP exhibited increased ALFF in the left SMA compared with patients with DED, indicating that this region might be a primary change in BSP. Speculatively, the present observation of increased ALFF in the left SMA may reflect the possibility that abnormal motor information from SMA to primary motor cortex results in improper motor outflow, creating involuntary closure of the orbicularis oculi that characterizes BSP.
The function of the cerebellum in motion control and coordination has been traditionally accepted, and further research evidence has emphasized the critical role of the cerebellum in the pathophysiology of dystonia (55, 56). For instance, diffusion tensor imaging studies have demonstrated abnormal integrity of cerebellar-thalamo-cortical fiber tracts in isolated focal dystonia phenotypes and decreased fractional anisotropy in the right cerebellum of patients with cervical dystonia (57, 58). Similarly, we found increased ALFF in the left cerebellar Crus I in patients with BSP compared with healthy controls and patients with DED. In addition, no correlation was seen between the increased ALFF in the left cerebellar crus I and JRS scores or symptomatic severity in BSP patients, suggesting that the abnormal ALFF values in the left cerebellar crus I might be independent of the illness duration and disease severity. The cerebellum receives fibers from the brainstem cerebellar pathway and projects them to the sensorimotor cortex through the cerebellar Crus I/II to modulate muscular tone and coordinate muscle groups (59, 60). In addition, separate cortico–cerebellar circuits can engage visual–spatial processing and response to the visual stimulus, supporting the assumption that the cerebellum is also involved in vision modulation (61). There is increased ALFF in the left cerebellar Crus I of patients with BSP, suggesting that the cerebellum might be another key node that received abnormal visual sensation and muscle tension caused by persistent eye spasm. As a consequence, it becomes hyperactive to regulate abnormal movement through sensorimotor integration process. Therefore, alterations in the left cerebellar Crus I might be distinctive in BSP, and an increased cerebellar neuronal activity might induce subsequent adaptive activities in motor systems. Traditional deep brain stimulation (DBS) and TMS therapeutic targets such as the globus pallidus interma and subthalamic nucleus of dystonia have expanded to the cerebellum in certain types of dystonia (62, 63). Cerebellum could be a potential target for in-depth exploration of intervention strategies in BSP.
Interestingly, our results showed an increased ALFF in the left fusiform gyrus and a positive correlation between ALFF in this region and symptomatic severity in patients with BSP. As part of the primary visual network, the fusiform gyrus is an important link in the human ventral visual information processing pathway that processes color information and facial expression perception (64, 65). A wide range of cognitive deficits are found in patients with BSP; among them, visuospatial function is the most frequently affected area (66). The characteristics of this visuospatial function abnormality was mutually supported by our results, which might be distinctive visual network changes secondary to aberrant visual input caused by eyelid spasms. Moreover, visual areas are widely connected to the basal ganglia and other motor areas. Thus, ALFF alterations in the left fusiform gyrus might result in visual-motor integration dysfunction, a form of sensorimotor integration. The positive correlation between ALFF in the left fusiform gyrus and symptomatic severity of BSP might represent an adaptive role or reaction to abnormal visual information.
We also found decreased ALFF in the bilateral superior MPFC and right SFG in patients with BSP. The MPFC and SFG are core components of the brain's default mode network (DMN), which is commonly recognized to be involved in cognitive functions and emotional processes (67). Our previous study in patients with BSP revealed an enhanced homotopic coordination in the inferior temporal gyrus and posterior cingulate cortex within the DMN (15). These abnormalities indicated distinct differences within the DMN in patients with BSP. One possibility is that these changes within the DMN might be related to nonmotor symptoms, such as anxiety, depression, and cognitive impairments, in patients with BSP; and these changes occur more frequently in patients with BSP than that in healthy controls (9). Although our results showed no significant difference in SAS/SDS scores between patients with BSP and healthy controls, it still raises a possibility that our patients might have subclinical emotional and cognitive impairments. A widely accepted viewpoint proposed that the MPFC is a high-level nervous center located at the top of the information processing stream, which flexibly map sensory signals to motor actions and achieve an adaptive choice behavior (68–70). It can be seen that the MPFC plays a role in sensorimotor integration. Moreover, MPFC has been thought to involve higher-level cognitive functions related to voluntary action, including formation of movement plans and choice of executive actions (71, 72). Likewise, the SFG is connected to the critical nodes of the motor-related network, including the precentral gyrus, caudate nucleus and thalamus (73). In this regard, the DMN might partly contribute to motor regulation apart from non-motor symptoms in BSP through functional connectivity.
In addition to small sample size, some limitations should be noted in this study. First, the potential dystonic activity of the eyelids during fMRI scanning should be considered although all subjects denied this phenomenon. Second, we were unable to detect the potential dysfunction of connections across different brain regions of patients with BSP because the ALFF measurements do not reflect the integration of the brain function activity at a network level. Furthermore, it should be noted that the application of head retainers and earplugs during images acquisition might induce sensory tricks, thus disturbing the “resting-state” to some extent.
Conclusion
Despite the limitations, the design of combining patients with BSP and patients with DED may be a feasible option to distinguish the secondary central effects of the sustained muscle activity in neuroimaging studies on BSP. In conclusion, our results reveal that the distinctive changes in the brain function of patients with BSP are different from those in patients with DED and healthy controls. The results further emphasize the primary role of sensorimotor integration in the pathophysiology of BSP.
Data Availability Statement
The raw data supporting the conclusions of this article will be made available by the authors, without undue reservation.
Ethics Statement
The studies involving human participants were reviewed and approved by the Ethics Committee of the First Affiliated Hospital of Guangxi Medical University. The patients/participants provided their written informed consent to participate in this study.
Author Contributions
SL, WG, CF, and WJ made substantial contributions to the conception of the work. YiL and WL did the acquisition of data. YX, YaL, YuL, JT, JW, LP, and ML analyzed the data. CF and WJ wrote the main manuscript text. All authors reviewed and approved the manuscript.
Funding
This study was supported by grants from the National Natural Science Foundation of China (Grant No. 81771447), National Key R&D Program of China (Grant No. 2016YFC1307100), Guangxi Appropriate Technology for Medical and Health Research Development Project (Grant No. S2020028), and Incubation Project of Research Team (Grant No. MINKEFY202108).
Conflict of Interest
The authors declare that the research was conducted in the absence of any commercial or financial relationships that could be construed as a potential conflict of interest.
Publisher's Note
All claims expressed in this article are solely those of the authors and do not necessarily represent those of their affiliated organizations, or those of the publisher, the editors and the reviewers. Any product that may be evaluated in this article, or claim that may be made by its manufacturer, is not guaranteed or endorsed by the publisher.
Acknowledgments
We are grateful to all the foundations mentioned above and all the participants in the study.
Supplementary Material
The Supplementary Material for this article can be found online at: https://www.frontiersin.org/articles/10.3389/fneur.2021.607476/full#supplementary-material
References
1. Hallett M. Blepharospasm: recent advances. Neurology. (2002) 59:1306–12. doi: 10.1212/01.WNL.0000027361.73814.0E
2. Peckham EL, Lopez G, Shamim EA, Richardson SP, Sanku S, Malkani R, et al. Clinical features of patients with blepharospasm: a report of 240 patients. Eur J Neurol. (2011) 18:382–6. doi: 10.1111/j.1468-1331.2010.03161.x
3. Jinnah HA, Berardelli A, Comella C, Defazio G, Delong MR, Factor S, et al. The focal dystonias: current views and challenges for future research. Mov Disord. (2013) 28:926–43. doi: 10.1002/mds.25567
4. Defazio G, Conte A, Gigante AF, Ferrazzano G, Pellicciari R, Dagostino S, et al. Clinical heterogeneity in patients with idiopathic blepharospasm: a cluster analysis. Parkinsonism Relat Disord. (2017) 40:64–8. doi: 10.1016/j.parkreldis.2017.04.014
5. Ferrazzano G, Conte A, Gigante A, Defazio G, Berardelli A, Fabbrini G. Disease progression in blepharospasm: a 5-year longitudinal study. Eur J Neurol. (2019) 26:268–73. doi: 10.1111/ene.13832
6. Defazio G, Abbruzzese G, Aniello MS, Di Fede R, Esposito M, Fabbrini G, et al. Eye symptoms in relatives of patients with primary adult-onset dystonia. Mov Disord. (2012) 27:305–7. doi: 10.1002/mds.24026
7. Shimazaki J. Definition and diagnostic criteria of dry eye disease: historical overview and future directions. Invest Ophthalmol Vis Sci. (2018) 59:DES7–DES12. doi: 10.1167/iovs.17-23475
8. Kranz G, Shamim EA, Lin PT, Kranz GS, Voller B, Hallett M. Blepharospasm and the modulation of cortical excitability in primary and secondary motor areas. Neurology. (2009) 73:2031–6. doi: 10.1212/WNL.0b013e3181c5b42d
9. Valls-Sole J, Defazio G. Blepharospasm: update on epidemiology, clinical aspects, and pathophysiology. Front Neurol. (2016) 7:45. doi: 10.3389/fneur.2016.00045
10. Martino D, Di Giorgio A, D'Ambrosio E, Popolizio T, Macerollo A, Livrea P, et al. Cortical gray matter changes in primary blepharospasm: a voxel-based morphometry study. Mov Disord. (2011) 26:1907–12. doi: 10.1002/mds.23724
11. Suzuki Y, Kiyosawa M, Wakakura M, Mochizuki M, Ishii K. Gray matter density increase in the primary sensorimotor cortex in long-term essential blepharospasm. Neuroimage. (2011) 56:1–7. doi: 10.1016/j.neuroimage.2011.01.081
12. Battistella G, Termsarasab P, Ramdhani RA, Fuertinger S, Simonyan KJCC. Isolated focal dystonia as a disorder of large-scale functional networks. Cereb Cortex. (2015) 27:1203–15. doi: 10.1093/cercor/bhv313
13. Huang XF, Zhu MR, Shan P, Pei CH, Liang ZH, Zhou HL, et al. Multiple neural networks malfunction in primary blepharospasm: an independent components analysis. Front Hum Neurosci. (2017) 11:235. doi: 10.3389/fnhum.2017.00235
14. Jochim A, Li Y, Gora-Stahlberg G, Mantel T, Berndt M, Castrop F, et al. Altered functional connectivity in blepharospasm/orofacial dystonia. Brain Behav. (2018) 8:e00894. doi: 10.1002/brb3.894
15. Wei J, Wei S, Yang R, Yang L, Yin Q, Li H, et al. Voxel-mirrored homotopic connectivity of resting-state functional magnetic resonance imaging in blepharospasm. Front Psychol. (2018) 9:1620. doi: 10.3389/fpsyg.2018.01620
16. Jiang WY, Lan Y, Cen CY, Liu Y, Feng CQ, Lei YW, et al. Abnormal spontaneous neural activity of brain regions in patients with primary blepharospasm at rest. J Neurol Sci. (2019) 403:44–9. doi: 10.1016/j.jns.2019.06.002
17. Defazio G, Hallett M, Jinnah HA, Conte A, Berardelli A. Blepharospasm 40 years later. Mov Disord. (2017) 32:498–509. doi: 10.1002/mds.26934
18. Baker RS, Andersen AH, Morecraft RJ, Smith CD. A functional magnetic resonance imaging study in patients with benign essential blepharospasm. J Neuroophthalmol. (2003) 23:11–5. doi: 10.1097/00041327-200303000-00003
19. Schmidt KE, Linden DE, Goebel R, Zanella FE, Lanfermann H, Zubcov AA. Striatal activation during blepharospasm revealed by fMRI. Neurology. (2003) 60:1738–43. doi: 10.1212/01.WNL.0000063306.67984.8C
20. Biswal BB. Resting state fMRI: a personal history. Neuroimage. (2012) 62:938–44. doi: 10.1016/j.neuroimage.2012.01.090
21. Hoptman MJ, Zuo XN, Butler PD, Javitt DC, D'Angelo D, Mauro CJ, et al. Amplitude of low-frequency oscillations in schizophrenia: a resting state fMRI study. Schizophr Res. (2010) 117:13–20. doi: 10.1016/j.schres.2009.09.030
22. Zhang Y, Zhu C, Chen H, Duan X, Lu F, Li M, et al. Frequency-dependent alterations in the amplitude of low-frequency fluctuations in social anxiety disorder. J Affect Disord. (2015) 174:329–35. doi: 10.1016/j.jad.2014.12.001
23. Li H, Guo W, Liu F, Chen J, Su Q, Zhang Z, et al. Enhanced baseline activity in the left ventromedial putamen predicts individual treatment response in drug-naive, first-episode schizophrenia: results from two independent study samples. EBioMedicine. (2019) 46:248–55. doi: 10.1016/j.ebiom.2019.07.022
24. Su Q, Yu M, Liu F, Zhang Z, Lei M, Jiang Y, et al. Frequency-specific alterations of the frontal-cerebellar circuit in first-episode, drug-naive somatization disorder. J Affect Disord. (2021) 280(Pt A):319–25. doi: 10.1016/j.jad.2020.11.090
25. Defazio G, Hallett M, Jinnah HA, Berardelli A. Development and validation of a clinical guideline for diagnosing blepharospasm. Neurology. (2013) 81:236–40. doi: 10.1212/WNL.0b013e31829bfdf6
26. Craig JP, Nichols KK, Akpek EK, Caffery B, Dua HS, Joo CK, et al. TFOS DEWS II definition and classification report. Ocul Surf. (2017) 15:276–83. doi: 10.1016/j.jtos.2017.05.008
27. Joseph J, Christopher K, Susanne G, Roman G, George CJMD. Relationship between various clinical outcome assessments in patients with blepharospasm. Mov Disord. (2009) 24:407–13. doi: 10.1002/mds.22368
28. Song XW, Dong ZY, Long XY, Li SF, Zuo XN, Zhu CZ, et al. REST: a toolkit for resting-state functional magnetic resonance imaging data processing. PLoS One. (2011) 6:e25031. doi: 10.1371/journal.pone.0025031
29. Zang YF, He Y, Zhu CZ, Cao QJ, Sui MQ, Liang M, et al. Altered baseline brain activity in children with ADHD revealed by resting-state functional MRI. Brain Dev. (2007) 29:83–91. doi: 10.1016/j.braindev.2006.07.002
30. Obermann M, Yaldizli O, De Greiff A, Lachenmayer ML, Buhl AR, Tumczak F, et al. Morphometric changes of sensorimotor structures in focal dystonia. Mov Disord. (2007) 22:1117–23. doi: 10.1002/mds.21495
31. Tinazzi M, Fiorio M, Fiaschi A, Rothwell JC, Bhatia KP. Sensory functions in dystonia: insights from behavioral studies. Mov Disord. (2009) 24:1427–36. doi: 10.1002/mds.22490
32. Patel N, Jankovic J, Hallett M. Sensory aspects of movement disorders. Lancet Neurol. (2014) 13:100–12. doi: 10.1016/S1474-4422(13)70213-8
33. Zittel S, Helmich RC, Demiralay C, Munchau A, Baumer T. Normalization of sensorimotor integration by repetitive transcranial magnetic stimulation in cervical dystonia. J Neurol. (2015) 262:1883–9. doi: 10.1007/s00415-015-7789-1
34. Avanzino L, Tinazzi M, Ionta S, Fiorio M. Sensory-motor integration in focal dystonia. Neuropsychologia. (2015) 79(Pt B):288–300. doi: 10.1016/j.neuropsychologia.2015.07.008
35. Stamelou M, Edwards MJ, Hallett M, Bhatia KP. The non-motor syndrome of primary dystonia: clinical and pathophysiological implications. Brain. (2012) 135(Pt 6):1668–81. doi: 10.1093/brain/awr224
36. Perruchoud D, Murray MM, Lefebvre J, Ionta S. Focal dystonia and the Sensory-Motor Integrative Loop for Enacting (SMILE). Front Hum Neurosci. (2014) 8:458. doi: 10.3389/fnhum.2014.00458
37. Yang J, Shao N, Song W, Wei Q, Ou R, Wu Y, et al. Nonmotor symptoms in primary adult-onset cervical dystonia and blepharospasm. Brain Behav. (2017) 7:e00592. doi: 10.1002/brb3.592
38. Ferrazzano G, Berardelli I, Conte A, Baione V, Concolato C, Belvisi D, et al. Motor and non-motor symptoms in blepharospasm: clinical and pathophysiological implications. J Neurol. (2019) 266:2780–5. doi: 10.1007/s00415-019-09484-w
39. Conte A, Berardelli I, Ferrazzano G, Pasquini M, Berardelli A, Fabbrini G. Non-motor symptoms in patients with adult-onset focal dystonia: sensory and psychiatric disturbances. Parkinsonism Relat Disord. (2016) 22(Suppl. 1):S111–S4. doi: 10.1016/j.parkreldis.2015.09.001
40. Byun YS, Kim SE, Paik JS, Yang SW. Increased pupillary constriction velocity in benign essential blepharospasm associated with photophobia. PLoS One. (2019) 14:e0217924. doi: 10.1371/journal.pone.0217924
41. Naumann M, Magyar-Lehmann S, Reiners K, Erbguth F, Leenders KL. Sensory tricks in cervical dystonia: perceptual dysbalance of parietal cortex modulates frontal motor programming. Ann Neurol. (2000) 47:322–8. doi: 10.1002/1531-8249(200003)47:3 <322::AID-ANA7>3.0.CO;2-E
42. Amadio S, Houdayer E, Bianchi F, Tesfaghebriel Tekle H, Urban IP, Butera C, et al. Sensory tricks and brain excitability in cervical dystonia: a transcranial magnetic stimulation study. Mov Disord. (2014) 29:1185–8. doi: 10.1002/mds.25888
43. Patel N, Hanfelt J, Marsh L, Jankovic J, members of the Dystonia Coalition. Alleviating manoeuvres (sensory tricks) in cervical dystonia. J Neurol Neurosurg Psychiatry. (2014) 85:882–4. doi: 10.1136/jnnp-2013-307316
44. Ramos VF, Karp BI, Hallett M. Tricks in dystonia: ordering the complexity. J Neurol Neurosurg Psychiatry. (2014) 85:987–93. doi: 10.1136/jnnp-2013-306971
45. Martino D, Liuzzi D, Macerollo A, Aniello MS, Livrea P, Defazio G. The phenomenology of the geste antagoniste in primary blepharospasm and cervical dystonia. Mov Disord. (2010) 25:407–12. doi: 10.1002/mds.23011
46. Ferrazzano G, Conte A, Belvisi D, Fabbrini A, Baione V, Berardelli A, et al. Writing, reading, and speaking in blepharospasm. J Neurol. (2019) 266:1136–40. doi: 10.1007/s00415-019-09243-x
47. Delnooz CC, Pasman JW, Beckmann CF, van de Warrenburg BP. Altered striatal and pallidal connectivity in cervical dystonia. Brain Struct Funct. (2015) 220:513–23. doi: 10.1007/s00429-013-0671-y
48. Smith SM, Fox PT, Miller KL, Glahn DC, Fox PM, Mackay CE, et al. Correspondence of the brain's functional architecture during activation and rest. Proc Natl Acad Sci U S A. (2009) 106:13040–5. doi: 10.1073/pnas.0905267106
49. Carbonnell L, Hasbroucq T, Grapperon J, Vidal F. Response selection and motor areas: a behavioural and electrophysiological study. Clin Neurophysiol. (2004) 115:2164–74. doi: 10.1016/j.clinph.2004.04.012
50. Gross J, Pollok B, Dirks M, Timmermann L, Butz M, Schnitzler A. Task-dependent oscillations during unimanual and bimanual movements in the human primary motor cortex and SMA studied with magnetoencephalography. Neuroimage. (2005) 26:91–8. doi: 10.1016/j.neuroimage.2005.01.025
51. Cuny E, Ghorayeb I, Guehl D, Escola L, Bioulac B, Burbaud PJN, et al. Sensory motor mismatch within the supplementary motor area in the dystonic monkey. Neurobiol Dis. (2008) 30:151–61. doi: 10.1016/j.nbd.2007.12.011
52. Opavsky R, Hlustik P, Otruba P, Kanovsky P. Sensorimotor network in cervical dystonia and the effect of botulinum toxin treatment: a functional MRI study. J Neurol Sci. (2011) 306:71–5. doi: 10.1016/j.jns.2011.03.040
53. Mantel T, Meindl T, Li Y, Jochim A, Gora-Stahlberg G, Kraenbring J, et al. Network-specific resting-state connectivity changes in the premotor-parietal axis in writer's cramp. Neuroimage Clin. (2018) 17:137–44. doi: 10.1016/j.nicl.2017.10.001
54. Jiang W, Lei Y, Wei J, Yang L, Wei S, Yin Q, et al. Alterations of interhemispheric functional connectivity and degree centrality in cervical dystonia: a resting-state fMRI study. Neural Plast. (2019) 2019:7349894. doi: 10.1155/2019/7349894
55. Filip P, Gallea C, Lehericy S, Bertasi E, Popa T, Marecek R, et al. Disruption in cerebellar and basal ganglia networks during a visuospatial task in cervical dystonia. Mov Disord. (2017) 32:757–68. doi: 10.1002/mds.26930
56. Shakkottai VG, Batla A, Bhatia K, Dauer WT, Dresel C, Niethammer M, et al. Current opinions and areas of consensus on the role of the cerebellum in dystonia. Cerebellum. (2017) 16:577–94. doi: 10.1007/s12311-016-0825-6
57. Teo JT, van de Warrenburg BP, Schneider SA, Rothwell JC, Bhatia KP. Neurophysiological evidence for cerebellar dysfunction in primary focal dystonia. J Neurol Neurosurg Psychiatry. (2009) 80:80–3. doi: 10.1136/jnnp.2008.144626
58. Berman BD, Honce JM, Shelton E, Sillau SH, Nagae LM. Isolated focal dystonia phenotypes are associated with distinct patterns of altered microstructure. Neuroimage Clin. (2018) 19:805–12. doi: 10.1016/j.nicl.2018.06.004
59. Buckner RL. The cerebellum and cognitive function: 25 years of insight from anatomy and neuroimaging. Neuron. (2013) 80:807–15. doi: 10.1016/j.neuron.2013.10.044
60. Witter L, De Zeeuw CI. Regional functionality of the cerebellum. Curr Opin Neurobiol. (2015) 33:150–5. doi: 10.1016/j.conb.2015.03.017
61. Brissenden JA, Tobyne SM, Osher DE, Levin EJ, Halko MA, Somers DC. Topographic cortico-cerebellar networks revealed by visual attention and working memory. Curr Biol. (2018) 28:3364–72.e3365. doi: 10.1016/j.cub.2018.08.059
62. Koch G, Porcacchia P, Ponzo V, Carrillo F, Caceres-Redondo MT, Brusa L, et al. Effects of two weeks of cerebellar theta burst stimulation in cervical dystonia patients. Brain Stimul. (2014) 7:564–72. doi: 10.1016/j.brs.2014.05.002
63. Bradnam LV, Graetz LJ, McDonnell MN, Ridding MC. Anodal transcranial direct current stimulation to the cerebellum improves handwriting and cyclic drawing kinematics in focal hand dystonia. Front Hum Neurosci. (2015) 9:286. doi: 10.3389/fnhum.2015.00286
64. Radua J, Phillips ML, Russell T, Lawrence N, Marshall N, Kalidindi S, et al. Neural response to specific components of fearful faces in healthy and schizophrenic adults. Neuroimage. (2010) 49:939–46. doi: 10.1016/j.neuroimage.2009.08.030
65. Muller VI, Hohner Y, Eickhoff SB. Influence of task instructions and stimuli on the neural network of face processing: an ALE meta-analysis. Cortex. (2018) 103:240–55. doi: 10.1016/j.cortex.2018.03.011
66. Yang J, Song W, Wei Q, Ou R, Cao B, Liu W, et al. Screening for cognitive impairments in primary blepharospasm. PLoS One. (2016) 11:e0160867. doi: 10.1371/journal.pone.0160867
67. Marsland AL, Kuan DC, Sheu LK, Krajina K, Kraynak TE, Manuck SB, et al. Systemic inflammation and resting state connectivity of the default mode network. Brain Behav Immun. (2017) 62:162–70. doi: 10.1016/j.bbi.2017.01.013
68. Gusnard DA, Akbudak E, Shulman GL, Raichle ME. Medial prefrontal cortex and self-referential mental activity: relation to a default mode of brain function. Proc Natl Acad Sci U S A. (2001) 98:4259–64. doi: 10.1073/pnas.071043098
69. Euston DR, Gruber AJ, McNaughton BL. The role of medial prefrontal cortex in memory and decision making. Neuron. (2012) 76:1057–70. doi: 10.1016/j.neuron.2012.12.002
70. Duncan NW, Hayes DJ, Wiebking C, Tiret B, Pietruska K, Chen DQ, et al. Negative childhood experiences alter a prefrontal-insular-motor cortical network in healthy adults: a preliminary multimodal rsfMRI-fMRI-MRS-dMRI study. Hum Brain Mapp. (2015) 36:4622–37. doi: 10.1002/hbm.22941
71. Rushworth MF. Intention, choice, and the medial frontal cortex. Ann N Y Acad Sci. (2008) 1124:181–207. doi: 10.1196/annals.1440.014
72. Phillips HN, Cope TE, Hughes LE, Zhang J, Rowe JB. Monitoring the past and choosing the future: the prefrontal cortical influences on voluntary action. Sci Rep. (2018) 8:7247. doi: 10.1038/s41598-018-25127-y
Keywords: blepharospasm, dry eye disease, functional magnetic resonance imaging, amplitude of low-frequency fluctuations, sensorimotor integration
Citation: Feng C, Jiang W, Xiao Y, Liu Y, Pang L, Liang M, Tang J, Lu Y, Wei J, Li W, Lei Y, Guo W and Luo S (2021) Comparing Brain Functional Activities in Patients With Blepharospasm and Dry Eye Disease Measured With Resting-State fMRI. Front. Neurol. 12:607476. doi: 10.3389/fneur.2021.607476
Received: 26 September 2020; Accepted: 24 September 2021;
Published: 27 October 2021.
Edited by:
Boldizsar Czeh, University of Pécs, HungaryReviewed by:
Manxiu Ma, Virginia Tech Carilion, United StatesGina Ferrazzano, Sapienza University of Rome, Italy
Copyright © 2021 Feng, Jiang, Xiao, Liu, Pang, Liang, Tang, Lu, Wei, Li, Lei, Guo and Luo. This is an open-access article distributed under the terms of the Creative Commons Attribution License (CC BY). The use, distribution or reproduction in other forums is permitted, provided the original author(s) and the copyright owner(s) are credited and that the original publication in this journal is cited, in accordance with accepted academic practice. No use, distribution or reproduction is permitted which does not comply with these terms.
*Correspondence: Wenbin Guo, guowenbin76@csu.edu.cn; Shuguang Luo, robert58243@sohu.com
†These authors have contributed equally to this work