- 1Department of Neurology, Ajou University School of Medicine, Ajou University Medical Center, Suwon-si, South Korea
- 2Department of Biomedical Science, Ajou University School of Medicine, Ajou University Medical Center, Suwon-si, South Korea
Symptomatic hemorrhagic transformation (HT) is one of the complications most likely to lead to death in patients with acute ischemic stroke. HT after acute ischemic stroke is diagnosed when certain areas of cerebral infarction appear as cerebral hemorrhage on radiological images. Its mechanisms are usually explained by disruption of the blood-brain barrier and reperfusion injury that causes leakage of peripheral blood cells. In ischemic infarction, HT may be a natural progression of acute ischemic stroke and can be facilitated or enhanced by reperfusion therapy. Therefore, to balance risks and benefits, HT occurrence in acute stroke settings is an important factor to be considered by physicians to determine whether recanalization therapy should be performed. This review aims to illustrate the pathophysiological mechanisms of HT, outline most HT-related factors after reperfusion therapy, and describe prevention strategies for the occurrence and enlargement of HT, such as blood pressure control. Finally, we propose a promising therapeutic approach based on biological research studies that would help clinicians treat such catastrophic complications.
Introduction
Hemorrhagic transformation refers to hemorrhagic infarction that occurs after venous thrombosis or arterial thrombosis and embolism (1, 2). Autopsy studies have reported an HT rate of 18–42% in acute ischemic stroke due to arterial occlusion (1, 3). The frequency of HT has been reported mainly in clinical studies using brain imaging modalities, such as computed tomography (CT) or magnetic resonance imaging (MRI), rather than pathological studies (4). Therefore, prior to considering the frequency of occurrence of HT, we need to understand the imaging and clinical definitions of HT. Although rates of HT in ischemic stroke have been reported, more than half of all cerebral infarctions demonstrate certain stages of HT (5).
The radiographic definition of HT is generally classified by the European Cooperative Acute Stroke Study (ECASS) (6). On CT scans, the severity of HT is divided into two stages: hemorrhagic infarction (HI) and parenchymal hemorrhage (PH) with or without mass effect. Each stage is divided into two subtypes (7). Each characteristic is presented in Table 1 (8).
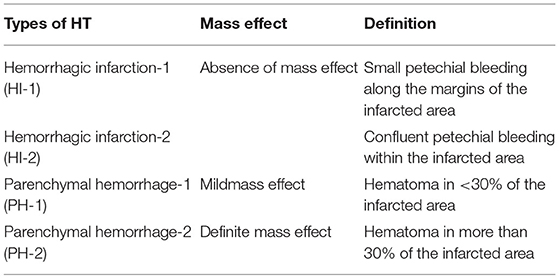
Table 1. Characteristics of hemorrhagic transformation (HT) according to European Cooperative Acute Stroke Study (ECASS) 2 (8).
With recent advances in intravenous (9) or endovascular (10) reperfusion therapies for acute ischemic stroke (11), stroke physicians need to deepen their understanding of cerebral hemorrhagic complications. Although the overall risks of complications have been well-documented in various randomized controlled trials (RCTs) of reperfusion therapies (12), the mechanisms underlying cerebral hemorrhage or hematoma after stroke in individual patients remain poorly understood. Intracranial bleeding after acute ischemic stroke has a significant impact on patient outcomes (13, 14), and controlling the risk of bleeding plays an important role in determining whether to proceed with recanalization (15). Large parenchymal hematomas and symptomatic intracerebral hemorrhage (sICH) are the most feared, tend to have a high mortality rate, and appear in up to 6% of patients after intravenous thrombolysis (16). In addition, infarction evolution with HT can lead to significant neurological deterioration (17–19). The frequency of HT is associated with different factors, such as epidemiological factors (e.g., age, pre-stroke treatment, and conditions), characteristics of the infarct (size of ischemic core and timing of follow-up), reperfusion techniques in the acute phase (intravenous thrombolysis, mechanical thrombectomy, or combined), radiological diagnosis (CT or MRI techniques), and use of antithrombotics after the acute phase (20–22).
Clinical Presentations, Histopathology, and Radiologic Features
Various criteria have been applied to define whether a hemorrhagic infarction is symptomatic; however, only parenchymal hematomas have been reported to be consistently linked to worsening and long-term deterioration (23). Many cases of HT, including most petechial hemorrhages, are asymptomatic (24). Only sICH (parenchymal hematoma) appears to be clinically evident and often exhibits rapid neurological deterioration (25). In untreated patients, HT rarely occurs during the first 6 h. It usually appears in the first few days, most within 4 days of infarction (26, 27). Patients who have undergone acute treatment with thrombolysis or thrombectomy usually experience bleeding 24 h after stroke onset (early HT) (28).
Pathologists have traditionally called petechial HT “red softening.” Petechial HT is considered to be due to (a) insufficient perfusion from adjacent collateral vessels or (b) reperfusion of infarcted tissues with weakened vessels (extravasation) (29). The former explains why HT occurs in patients with permanently occluded vessels (30), while the latter explains why the proportion of patients with HT is higher in those who receive reperfusion therapy than in those who do not receive reperfusion therapy (31).
Figure 1 shows the relationship between HT probability and reperfusion (R) after ischemic stroke. The radiologic features differ from those of petechial hemorrhagic infarction and parenchymal hemorrhage (19). Petechial hemorrhagic infarction usually appears as tiny punctate regions in the hemorrhage and is often not individually resolved (32). In parenchymal hematomas or hemorrhage, radiological features on both CT and MRI, which combine the features of ischemic infarction and cerebral hemorrhage, overlap (33).
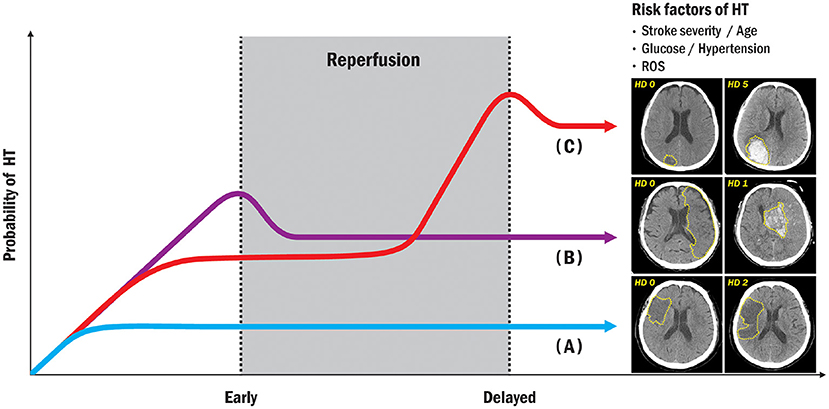
Figure 1. Illustration showing correlation between HT and reperfusion time after ischemic stroke. Under the risk factors commonly associated with HT; (A) no HT (no bleeding regardless of reperfusion); (B) early HT (definite bleeding usually 6–24 h after stroke); (C) delayed HT (definite bleeding usually more than 24 h after ischemic stroke). HT, hemorrhagic transformation; ROS, reactive oxygen species.
Mechanisms of HT After Ischemic Stroke
The blood–brain barrier (BBB) is a physiological barrier between the brain parenchyma and brain circulation that nourishes brain tissue, filters various substances from the brain to the blood, and protects the brain (34, 35). The BBB is composed of endothelial cells, basement membrane, pericytes, and astrocytes, collectively referred to as the neurovascular unit and linked to circulating peripheral blood cells (36, 37). Early disruption of the BBB plays a pivotal role in HT formation during acute ischemic stroke (38). Leukocyte types and various molecules are associated with HT after ischemic stroke (39). Neutrophils and brain tissue are major sources of matrix-metalloproteinase-9 (MMP-9) within the first 18–24 h after stroke (28, 40). Intravenous infusion of exogenous tissue plasminogen activator (tPA) can increase MMP-9 levels by activating neutrophils (41), and endogenous tPA can increase MMP-3 levels by acting on endothelial cell lipoprotein receptor protein (LRP) (42), and can increase MMP-2 levels by activating platelet-derived growth factor-CC as a trigger via astrocyte platelet-derived growth factor receptor A (43). Figure 2 shows a possible mechanism for early vs. delayed HT.
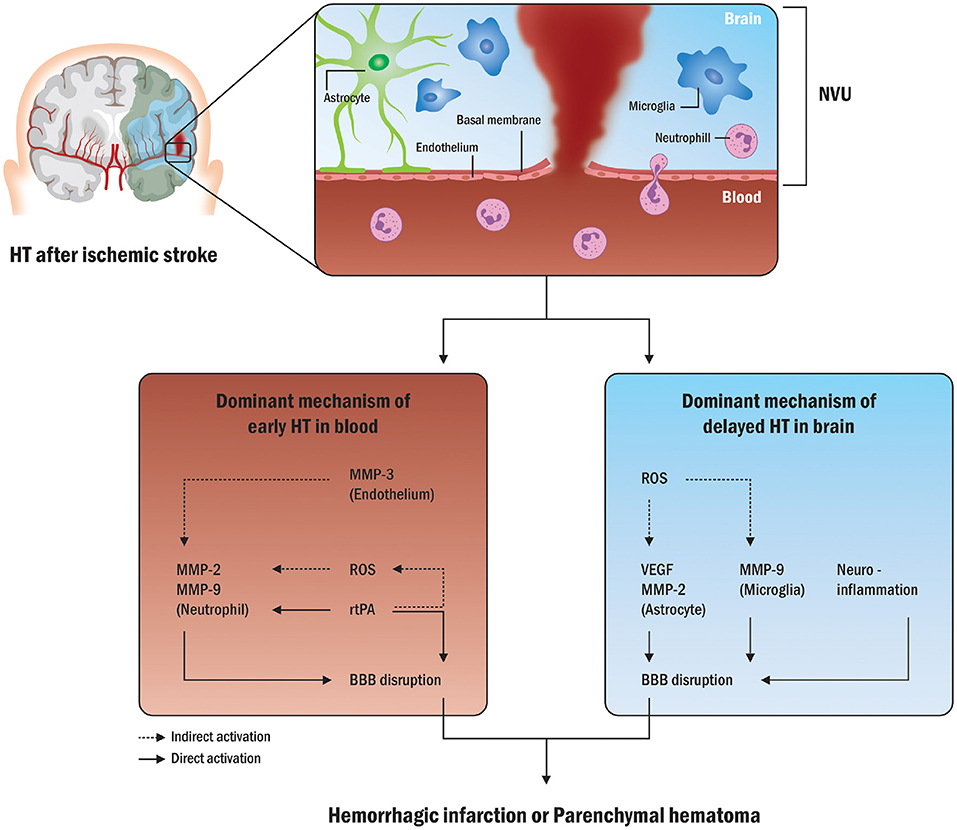
Figure 2. Possible mechanisms in early and delayed HT. The disruption of the BBB is a common pathway in HT formation following acute ischemic stroke. Various molecules from neutrophils and peripheral blood in possible processes in early HT are mainly associated with HT after ischemic stroke. Exogenous tPA can also increase MMP-9 levels by activating neutrophils and increasing MMP-2 levels. Conversely, in possible processes for delayed HT, the brain tissue is a major source of MMP-9 within the first 18–24 h following stroke, and endogenous tPA can act on endothelial cells to increase MMP-2 release from astrocytes as well as MMP-9 release from microglia. HT, hemorrhagic transformation; NVU, neurovascular unit; MMP, matrix-metalloproteinase; ROS, reactive oxygen species; tPA, tissue plasminogen activator; BBB, blood-brain barrier; VEGF, vascular endothelial growth factor.
Theoretically, cerebral infarction does not occur until the cerebral blood flow reaches a minimum threshold, where oxygen and glucose cannot be sufficiently guaranteed (44). As in other organs, infarcted cerebral tissue tends to bleed, and cerebral hemorrhage can lead to severe neurological deterioration (22). Mechanisms related to HT can be considered from various perspectives, such as histological changes, vascular occlusion, collateral circulation, BBB disruption, and infarct size (45, 46).
Acute cerebral ischemia leads to considerable damage to capillary cells, which causes an increase in vascular permeability and extravasation of blood in the brain parenchyma (47, 48). The two main factors described in this process are oxidative stress and reperfusion injury, which cause damage to blood vessels through various injury mechanisms, such as inflammation, leukocyte infiltration, vascular activation, and extracellular proteolysis (49, 50). The consequences are destruction of the basal lamina and endothelial tight junctions (51). Among the molecular processes involved, MMP-9 has been shown to play an important role in the destruction of basal lamina type IV collagen (52, 53). Destruction of the basal lamina leads to leakage of macromolecules into interstitial fluids in the central nervous system (54). In contrast to cytotoxic edema (cell death from ionic pump failure), the resulting ionic gradient causes interstitial edema, known as “vasogenic edema” (55). Vasogenic edema can lead to lesions in adjacent tissues. Therefore, this mechanism can worsen, causing malignant infarction, resulting in fatal consequences and high risk of HT (56).
Reperfusion can trigger harmful cascades, such as oxidative stress, suppression of protein synthesis, platelet activation, activation of the complement system, leukocyte infiltration, basal lamina disruption, and eventual cerebral cell death in the central nervous system (57–60). Reperfusion injury alone seems to be enough to cause fatal hematoma, but all ischemic strokes with tissue reperfusion do not cause hematoma (29). Fragments of thrombus with a large thrombotic burden can contribute to bleeding complications in the delayed phase (> 24 h) after acute stroke. Fragmentation of a large thrombus can lead to distal migration and damage to the vascular bed (61).
In summary, the development of HT after stroke involves multiple interconnected pathological processes from peripheral blood cells to neurovascular units, such as hyperactive ischemic cascades with increased MMP levels, excessive levels of ROS, coagulopathy, BBB breakdown, and reperfusion injury.
Factors Associated With HT After Ischemic Stroke
Although the usefulness of these HT-related factors may be limited in clinical practice, some factors predict HT. Given the fibrinolytic or antithrombotic therapy in acute ischemic stroke settings, imaging techniques, and predictive biomarkers can help screen specific patients at increased risk of HT as a group of particular interest (62–64). Advances in the use of neuroimaging and composite scores can lead to more personalized approaches for HT prediction, but various factors should be considered when drawing conclusions that may affect the timing of HT detection (16, 28). This point can be influenced by the accuracy of imaging modalities used, such as CT or MRI with or without gradient-echo or susceptibility-weighted sequences (65), which are more sensitive to the detection of blood products (64). Factors associated with HT after ischemic stroke are shown in detail in Table 2.
Reversal of Coagulopathy With Various Agents
Although coagulopathy correction remains the mainstay of treatment after tPA infusion, no specific agent has been found to be most effective in dealing with fatal HT expansion, which includes sICH (86). In patients with sICH, which occurs within 36 h after tPA infusion, there are several suggestions that can be considered depending on the mechanisms of action of reversal agents (87). The details are listed in Table 3.
Prevention of HT Expansion
Hematoma expansion or sICH is a major predictor of death and disability in patients with acute stroke with HT (88). Therefore, in addition to aggressive reversal of coagulopathy, other strategies to prevent hematoma expansion may be needed as therapeutic targets in sICH.
Elevation and variability in blood pressure have been linked to the risk of hematoma enlargement in patients with spontaneous ICH in observational studies (89). In patients with spontaneous intracerebral hemorrhage, studies have shown that intensive control of systolic blood pressure is relatively safe to lower to 140 mmHg, but this that measure had no apparent effect compared with the systolic blood pressure target of 180 mmHg (90). Although the optimal target for blood pressure control in sICH is still unclear, the treatment goal is to supply adequate blood flow to the ischemic area, reduce the pressure on the brain with autoregulation impairment, and eventually reduce the risk of hematoma expansion (91). However, the effects of BP on hematoma enlargement are for primary intracranial hemorrhage and are independent of those in patients with HT after ischemic stroke. Nonetheless, the recent Enhanced Control of Hypertension and Thrombolysis Stroke Study (ENCHANTED) trial has shown that intensive blood pressure control potentially reduces the risk of major intracranial hemorrhage in patients with acute ischemic stroke receiving intravenous thrombolytic therapy (92).
Patients with acute ischemic stroke may be at risk of additional ischemia, especially in a low blood pressure environment, if occluded vessels are not reopened following thrombolytic therapy or mechanical thrombectomy. Although several studies have linked poor neurological outcomes to decreased mean arterial pressure (93), Rasmussen et al. only included patients whose blood pressure was measured during endovascular procedures or intravenous alteplase infusion procedures, and the results were stratified according to the presence of sICH (94).
One study related to thrombolysis and blood pressure showed that decreased systolic blood pressure was associated with improved neurological outcomes and lower rates of sICH (86, 95). In the European Cooperative Acute Stroke Study II (ECASS II) clinical trial, higher systolic blood pressure was associated with worse functional outcomes and sICH (93). However, there was no clear evidence that lower blood pressure led to worse functional outcomes (87). In the presence of lethal HT after tPA infusion (especially parenchymal hemorrhage type 2), few data on blood pressure treatment are available, especially when compared with other types of HT. In tPA-related HT, healthcare providers should determine the target blood pressure and consider the severity of sICH, risk of bleeding enlargement, and risk of impending ischemia (96). Theoretically, with incomplete recanalization, higher blood pressure targets may be needed to maintain adequate collateral blood flow to the ischemic bed and reduce the risk of infarct growth among patients with HI-1 and HI-2 (97). Under complete recanalization, strict blood pressure control measures may be reasonable (98).
Stricter blood pressure control may be more beneficial and less harmful for patients with parenchymal hematoma at higher risk of hematoma enlargement. Hematomas with smaller volumes are generally left untreated (99), and deep-seated (thalamus or brainstem) hemorrhages are usually not evacuated.
In summary, healthcare providers should determine blood pressure targets by weighing the risk of worsening ischemia based on the severity of hemorrhage and its risk of expansion. Patients with incomplete recanalization may need higher blood pressure targets to maintain sufficient blood flow to the ischemic bed and reduce the risk of infarct growth. Conversely, patients with complete recanalization may need strict blood pressure control to avoid impending HT.
Blood Pressure Management After Thrombectomy for Preventing HT
Observational studies have shown an increased risk of HT in patients with high blood pressure and high variability in blood pressure, suggesting a close relationship between hemodynamics and HT (69). High variability in blood pressure has been considered a strong risk factor for cerebral edema and post-stroke HT, as rapid changes in blood pressure can easily rupture already damaged blood vessels due to ischemic insult (100, 101). Current guidelines recommend maintaining blood pressure below a fixed threshold of 180/105 mmHg for at least 24 h, regardless of thrombolytic or endovascular intervention (96, 102). A recent US study reported that a peak systolic BP of 158 mmHg in the first 24 h after endovascular therapy best dichotomized good and bad outcomes (103). A prospective randomized trial reported neutral results when determining whether a target systolic blood pressure (SBP) <130 mmHg after endovascular reperfusion can reduce the risk of intracranial hemorrhage (104). Therefore, lowering the post-reperfusion BP target can be considered to prevent reperfusion injury and promote tissue restoration in ischemic penumbra (98).
Cerebral autoregulation is the intrinsic dilative-constrictive capacity of the cerebral vasculature that preserves stable blood flow in the face of systemic blood pressure changes (105, 106). Autoregulatory capacity in acute stroke is crucial for maintaining stable blood flow to the ischemic penumbra and avoiding excessive hyperperfusion (107).
Petersen et al. reported more longitudinal autoregulation modes, indicating dynamic autoregulatory failures up to 1 week after emergent large vessel occlusion (ELVO) strokes (108). Figure 3 shows the presence of HT within or above the autoregulatory limits due to fluctuations in blood pressure. This investigation showed that the autoregulatory parameter in the ipsilateral cerebral hemisphere was lower than that in the opposite hemisphere, indicating a decrease in the ability to buffer blood pressure fluctuations (91). In patients with stroke with cerebral autoregulation impairment, restoration tends to be delayed for up to 3 months, emphasizing the clinical relevance of autoregulation in stroke research (109, 110).
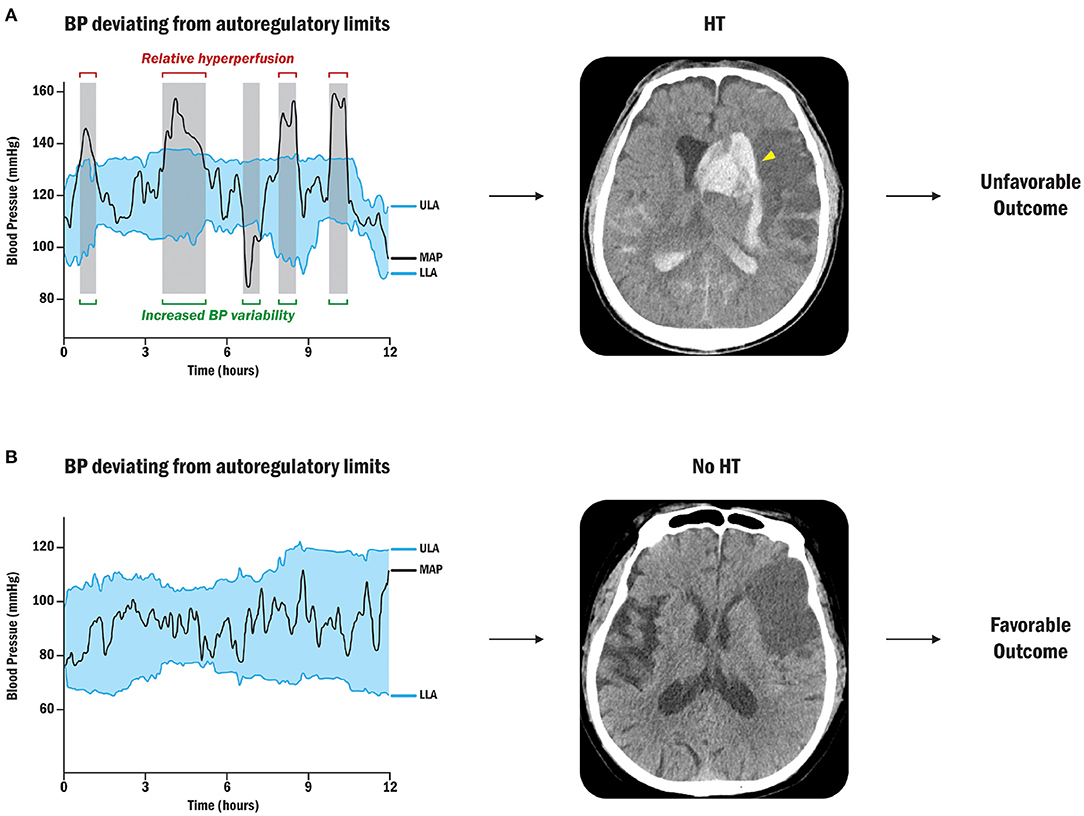
Figure 3. Sequential BP changes, cerebral autoregulation, and HT (91). (A) Patient with BP deviation from autoregulatory limits: Relative hyperperfusion above the upper limit of autoregulation may lead to HT and unfavorable outcomes. The yellow arrowhead points out the radiological HT after cerebral ischemic stroke. (B) Patient with acceptable BP fluctuations: Strictly controlled blood pressure within personalized limits of autoregulation can prevent secondary brain injury by protecting against HT after stroke. BP, blood pressure; ULA, upper limit of autoregulation; MAP, mean arterial pressure; LLA, lower limit of autoregulation.
In summary, continuous optimization of blood pressure would be a good method for patients tailored to their own physiology, where hemodynamic management represents an appropriate and neuroprotective avenue for critically ill patients. Exceeding the upper limits of autoregulation may predispose patients to reperfusion injury, and maintaining blood pressure within autoregulatory limits may avoid bleeding complications while achieving favorable outcomes. Furthermore, trajectory analysis has the potential to provide more individualized hemodynamic management during and after thrombectomy procedures in intensive care settings.
Medical Treatment and Neurosurgical Considerations
Hemorrhagic transformation after ischemic stroke can be suspected based on clinical presentation (neurological worsening in National Institutes of Health Stroke Scale, NIHSS, score) and radiological findings within 48 h on CT or MRI (111, 112). First, hemodynamic stabilization should be performed, followed by transfer to a neuro-intensive care unit if available (113). To evaluate the mechanisms of HT, HT-associated factors, such as clinical features, biochemical factors, genetics, imaging findings, and composite rating scores, should be analyzed. Temperature and glycemic controls should then be performed, such as mechanical prophylaxis for deep vein thrombosis (86). Second, blood pressure control and correction of coagulopathies should be mainstays of HT treatment (114). Finally, neurosurgical considerations are needed as soon as possible if hazardous HT is suspected (115). A plausible algorithm for appropriate clinical approaches to HT after ischemic stroke is depicted in Figure 4.
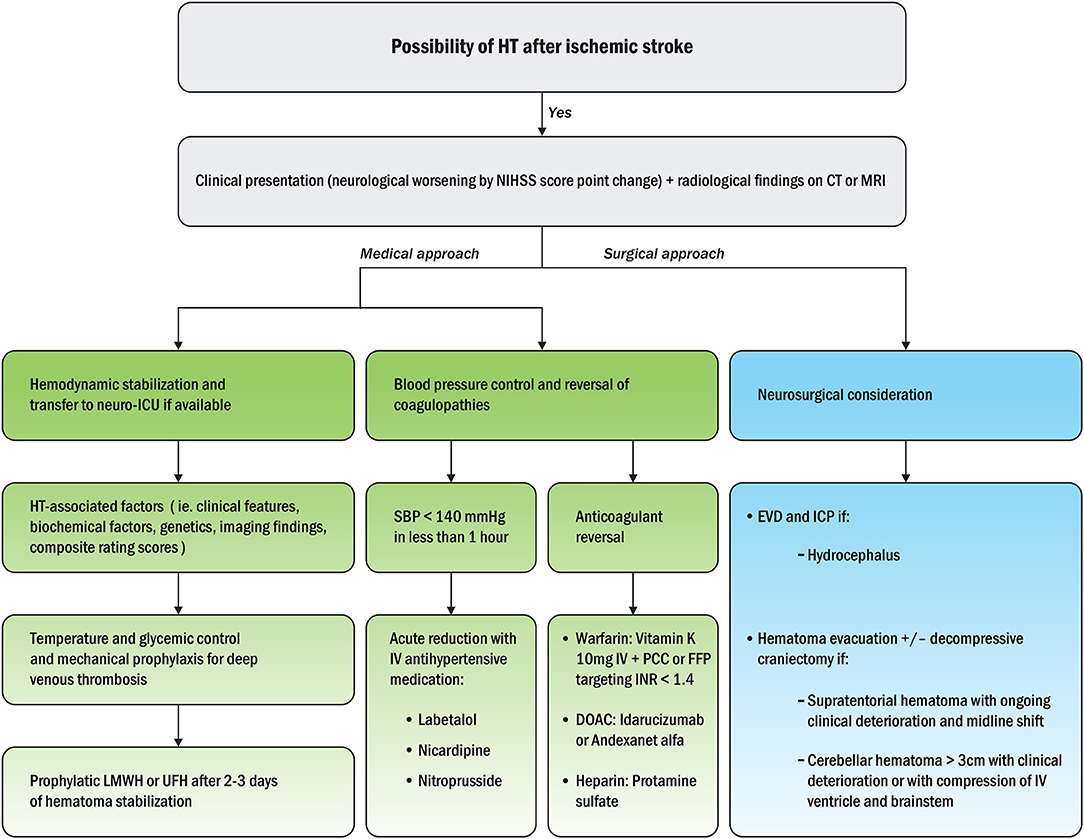
Figure 4. Treatment algorithm for appropriate medical and surgical approaches to HT after ischemic stroke (115). NIHSS, National Institutes of Health Stroke Scale; CT, computed tomography; MRI, magnetic resonance imaging; ICU, intensive care unit; LMWH, low molecular weight heparin; UFH, unfractionated heparin; SBP, systolic blood pressure; IV, intravenous; PCC, prothrombin complex concentrates; FFP, fresh frozen plasma; INR, international normalized ratio; DOAC, direct oral anticoagulants; EVD, external ventricular drainage; ICP, intracranial pressure.
It is challenging to construct evidence-based treatment algorithms for surgical interventions because of the lack of expected data to guide the therapeutic timing and surgical techniques to be implemented (116). Nonetheless, neurosurgical treatment can be considered in patients with sICH where outcomes can be improved despite ischemic injury. Indeed, the risks and benefits of rapid surgical decompression vs. iatrogenic injury must be carefully weighed in the setting of possible tPA-associated coagulopathy.
Neurosurgical treatment can also be considered in patients with supratentorial ICH who exhibit neurological deterioration, coma, significant midline shift, or elevated intracranial pressure refractory to medical treatment. The goal is to decompress the brain and reduce the impact of mass effect, malignant edema, and toxic blood byproducts (117). Open craniotomy can eliminate the compressive effect of a hematoma higher than 30 cm3 in volume from lobar, cerebellar, or surgically accessible basal ganglia hematomas (118, 119). However, this requires an incision through the cortex and white matter tracts along the path to the lesion. The clinical effectiveness of these interventions remains controversial (120, 121). Minimally invasive craniotomy and stereotactic hematoma evacuation are currently under investigation for spontaneous ICH and post-thrombolytic hemorrhage.
Potential Therapeutic Interventions Along With Biological Research on HT
Since HT in acute ischemic stroke is radiologically diagnosed, the timing of HT detection is affected by the accuracy of imaging modalities, such as CT or MRI, with or without gradient-echo or susceptibility-weighted imaging sequences that are more sensitive to blood products (122).
Strong evidence exists that matrix metalloproteinases, especially MMP-9, play a pivotal role in the pathogenesis of the abnormal permeability of the BBB, an important culprit of HT (42, 52, 53). The use of drugs to block the release of MMP-9 is challenging. The phosphodiesterase-III inhibitor cilostazol prevented the development of HT, reduced brain edema, prevented endothelial injury via reduction of MMP-9 activity, and prevented the BBB from opening in an experimental model (123–125). Another drug, the broad-spectrum MMP inhibitor BB-94, reduced the risk and severity of HT in rats with homologous clot-induced middle cerebral artery occlusion compared with rats treated with intravenous tPA alone (41). The MMP-9 inhibitor minocycline reduced the risk of HT after tPA in animal models (126, 127), and the Minocycline to Improve Neurologic Outcome in Stroke (MINOS) human trials showed a decrease in plasma MMP-9 levels in patients treated with intravenous tPA (128, 129). Targeted temperature management may be an important step to mitigate HT after recanalization in patients with clinically malignant ELVO, as it reduces the metabolic rate and excessive free radical levels, protects the BBB by reducing MMP-2 or MMP-9 expression, and inhibits immune system responses (130, 131). Nevertheless, effective management of lethal HT requires further experimental studies and trials based on core molecular mechanisms.
Conclusion
Symptomatic intracerebral hemorrhage is a life-threatening complication requiring emergent medical and surgical treatment in patients with acute ischemic stroke. Therefore, we need to understand possible mechanisms and treat this potentially serious complication with systematic algorithms in future stroke therapy.
Author Contributions
JH performed roles of the conception of this review and wrote the manuscript, interpretation of data, and revising it critically for important intellectual content. DK performed roles of interpretation of data and drafting the article. MK performed roles of the acquisition of data. All authors contributed to the article and approved the submitted version.
Conflict of Interest
The authors declare that the research was conducted in the absence of any commercial or financial relationships that could be construed as a potential conflict of interest.
Publisher's Note
All claims expressed in this article are solely those of the authors and do not necessarily represent those of their affiliated organizations, or those of the publisher, the editors and the reviewers. Any product that may be evaluated in this article, or claim that may be made by its manufacturer, is not guaranteed or endorsed by the publisher.
References
1. Lodder J, Krijne-Kubat B, Broekman J. Cerebral Hemorrhagic Infarction at Autopsy; Cardiac embolic cause and the relationship to the cause of death. Stroke. (1986) 17:626–9. doi: 10.1161/01.STR.17.4.626
2. Okada Y, Yamaguchi T, Minematsu K, Miyashita T, Sawada T, Sadoshima S, et al. Hemorrhagic transformation in cerebral embolism. Stroke. (1989) 20:598–603. doi: 10.1161/01.STR.20.5.598
3. England TJ, Bath PM, Sare GM, Geeganage C, Moulin T, O'Neill D, et al. Asymptomatic hemorrhagic transformation of infarction and its relationship with functional outcome and stroke subtype: assessment from the Tinzaparin in Acute Ischaemic Stroke Trial. Stroke. (2010) 41:2834–9. doi: 10.1161/STROKEAHA.109.573063
4. Lee J-H. Hemorrhagic transformation after acute ischemic stroke;prevalence predictive factors, and mechanisms. J Neurocrit Care. (2013) 6:59–66.
5. van Kranendonk KR, Treurniet KM, Boers AMM, Berkhemer OA, Coutinho JM, Lingsma HF, et al. Added prognostic value of hemorrhagic transformation quantification in patients with acute ischemic stroke. Front Neurol. (2020) 11:582767. doi: 10.3389/fneur.2020.582767
6. Fiorelli MBS, von Kummer R, del Zoppo GJ, Larrue V, Lesaffre E, Ringleb AP, et al. Hemorrhagic transformation within 36 hours of a cerebral infarct relationships with early clinical deterioration and 3-month outcome in the European Cooperative Acute Stroke Study I (ECASS I) cohort. Stroke. (1999) 30:2280–4. doi: 10.1161/01.STR.30.11.2280
7. Valentino F, Gentile L, Terruso V, Mastrilli S, Aridon P, Ragonese P, et al. Frequency and determinants for hemorrhagic transformation of posterior cerebral stroke : posterior ischemic stroke and hemorrhagic transformation. BMC Res Notes. (2017) 10:592. doi: 10.1186/s13104-017-2889-x
8. Marcell László J, Hortobágyi T. Hemorrhagic transformation of ischemic stroke. Vasc Dis Therap. (2017) 2:1–25. doi: 10.15761/VDT.1000130
9. Berge E, Whiteley W, Audebert H, De Marchis GM, Fonseca AC, Padiglioni C, et al. European Stroke Organisation (ESO) guidelines on intravenous thrombolysis for acute ischaemic stroke. Eur Stroke J. (2021) 6:I-LXII. doi: 10.1177/2396987321989865
10. Settecase F. Acute ischemic stroke endovascular therapy. Handb Clin Neurol. (2021) 176:199–227. doi: 10.1016/B978-0-444-64034-5.00008-0
11. Bhaskar S, Stanwell P, Cordato D, Attia J, Levi C. Reperfusion therapy in acute ischemic stroke: dawn of a new era? BMC Neurol. (2018) 18:8. doi: 10.1186/s12883-017-1007-y
12. Maier B, Desilles JP, Mazighi M. Intracranial hemorrhage after reperfusion therapies in acute ischemic stroke patients. Front Neurol. (2020) 11:599908. doi: 10.3389/fneur.2020.599908
13. van Kranendonk KR, Treurniet KM, Boers AMM, Berkhemer OA, van den Berg LA, Chalos V, et al. Hemorrhagic transformation is associated with poor functional outcome in patients with acute ischemic stroke due to a large vessel occlusion. J Neurointerv Surg. (2019) 11:464–8. doi: 10.1136/neurintsurg-2018-014141
14. Chiu D, Peterson L, Elkind MSV, Rosand J, Gerber LM, Silverstein MD, et al. Comparison of outcomes after intracerebral hemorrhage and ischemic stroke. J Stroke Cerebrovasc Dis. (2010) 19:225–9. doi: 10.1016/j.jstrokecerebrovasdis.2009.06.002
15. An SJ, Kim TJ, Yoon BW. Epidemiology, risk factors, and clinical features of intracerebral hemorrhage: an update. J Stroke. (2017) 19:3–10. doi: 10.5853/jos.2016.00864
16. Charbonnier G, Bonnet L, Biondi A, Moulin T. Intracranial bleeding after reperfusion therapy in acute ischemic stroke. Front Neurol. (2020) 11:629920. doi: 10.3389/fneur.2020.629920
17. Paciaroni M, Agnelli G, Corea F, Ageno W, Alberti A, Lanari A, et al. Early hemorrhagic transformation of brain infarction: rate, predictive factors, and influence on clinical outcome: results of a prospective multicenter study. Stroke. (2008) 39:2249–56. doi: 10.1161/STROKEAHA.107.510321
18. Lee JH, Park KY, Shin JH, Cha JK, Kim HY, Kwon JH, et al. Symptomatic hemorrhagic transformation and its predictors in acute ischemic stroke with atrial fibrillation. Eur Neurol. (2010) 64:193–200. doi: 10.1159/000319048
19. Stone JA, Willey JZ, Keyrouz S, Butera J, McTaggart RA, Cutting S, et al. Therapies for hemorrhagic transformation in acute ischemic stroke. Curr Treat Options Neurol. (2017) 19:1. doi: 10.1007/s11940-017-0438-5
20. Elsaid N, Mustafa W, Saied A. Radiological predictors of hemorrhagic transformation after acute ischemic stroke: an evidence-based analysis. Neuroradiol J. (2020) 33:118–33. doi: 10.1177/1971400919900275
21. Wen L, Zhang S, Wan K, Zhang H, Zhang X. Risk factors of haemorrhagic transformation for acute ischaemic stroke in Chinese patients receiving intravenous thrombolysis: a meta-analysis. Medicine. (2020) 99:e18995. doi: 10.1097/MD.0000000000018995
22. Paciaroni M, Bandini F, Agnelli G, Tsivgoulis G, Yaghi S, Furie KL, et al. Hemorrhagic transformation in patients with acute ischemic stroke and atrial fibrillation: time to initiation of oral anticoagulant therapy and outcomes. J Am Heart Assoc. (2018) 7:e010133. doi: 10.1161/JAHA.118.010133
23. Molina CA, Montaner J, Abilleira S, Ibarra B, Romero F, Arenillas JF, et al. Timing of spontaneous recanalization and risk of hemorrhagic transformation in acute cardioembolic stroke. Stroke. (2001) 32:1079–84. doi: 10.1161/01.STR.32.5.1079
24. Berger C, Fiorelli M, Steiner T, Schäbitz WR, Bozzao L, Bluhmki E, et al. HT of ischemic brain tissue asymptomatic or symptomatic? Stroke. (2001) 32:1330–5. doi: 10.1161/01.STR.32.6.1330
25. Jensen M, Schlemm E, Cheng B, Lettow I, Quandt F, Boutitie F, et al. Clinical characteristics and outcome of patients with hemorrhagic transformation after intravenous thrombolysis in the WAKE-UP trial. Front Neurol. (2020) 11:957. doi: 10.3389/fneur.2020.00957
26. Brott T, Lyden P, Grotta JC, Levine SR, Frankel M, Meyer M, et al. Intracerebral hemorrhage after intravenous t-PA therapy for ischemic stroke. the NINDS t-PA stroke study group. Stroke. (1997) 28:2109–18. doi: 10.1161/01.STR.28.11.2109
27. Lu A, Clark JF, Broderick JP, Pyne-Geithman GJ, Wagner KR, Khatri P, et al. Mechanical reperfusion is associated with post-ischemic hemorrhage in rat brain. Exp Neurol. (2009) 216:407–12. doi: 10.1016/j.expneurol.2008.12.020
28. Jickling GC, Liu D, Stamova B, Ander BP, Zhan X, Lu A, et al. Hemorrhagic transformation after ischemic stroke in animals and humans. J Cereb Blood Flow Metab. (2014) 34:185–99. doi: 10.1038/jcbfm.2013.203
29. Bang OY, Saver JL, Kim SJ, Kim GM, Chung CS, Ovbiagele B, et al. Collateral flow averts hemorrhagic transformation after endovascular therapy for acute ischemic stroke. Stroke. (2011) 42:2235–9. doi: 10.1161/STROKEAHA.110.604603
30. Campos F, Qin T, Castillo J, Seo JH, Arai K, Lo EH, et al. Fingolimod reduces hemorrhagic transformation associated with delayed tissue plasminogen activator treatment in a mouse thromboembolic model. Stroke. (2013) 44:505–11. doi: 10.1161/STROKEAHA.112.679043
31. Hacke W, Kaste M, Bluhmki E, Brozman M, Davalos A, Guidetti D, et al. Thrombolysis with alteplase 3 to 4.5 hours after acute ischemic stroke. N Engl J Med. (2008) 359:1317–29. doi: 10.1056/NEJMoa0804656
32. Bevers MB, Kimberly WT. Critical care management of acute ischemic stroke. Curr Treat Options Cardiovasc Med. (2017) 19:41. doi: 10.1007/s11936-017-0542-6
33. Heit JJ, Iv M, Wintermark M. Imaging of intracranial hemorrhage. J Stroke. (2017) 19:11–27. doi: 10.5853/jos.2016.00563
34. Kadry H, Noorani B, Cucullo L. A blood-brain barrier overview on structure, function, impairment, and biomarkers of integrity. Fluids Barriers CNS. (2020) 17:69. doi: 10.1186/s12987-020-00230-3
35. Kaplan L, Chow BW, Gu C. Neuronal regulation of the blood-brain barrier and neurovascular coupling. Nat Rev Neurosci. (2020) 21:416–32. doi: 10.1038/s41583-020-0322-2
36. Lochhead JJ, Yang J, Ronaldson PT, Davis TP. Structure, function, and regulation of the blood-brain barrier tight junction in central nervous system disorders. Front Physiol. (2020) 11:914. doi: 10.3389/fphys.2020.00914
37. Bell AH, Miller SL, Castillo-Melendez M, Malhotra A. The neurovascular unit: effects of brain insults during the perinatal period. Front Neurosci. (2019) 13:1452. doi: 10.3389/fnins.2019.01452
38. Arba F, Rinaldi C, Caimano D, Vit F, Busto G, Fainardi E. Blood-brain barrier disruption and hemorrhagic transformation in acute ischemic stroke: systematic review and meta-analysis. Front Neurol. (2020) 11:594613. doi: 10.3389/fneur.2020.594613
39. Song Q, Li Y, Wang Y, Wei C, Liu J, Liu M. Increased neutrophil-to-lymphocyte ratios are associated with greater risk of hemorrhagic transformation in patients with acute ischemic stroke. Curr Neurovasc Res. (2018) 15:326–35. doi: 10.2174/1567202616666181204122457
40. Wang X, Tsuji K, Lee SR, Ning M, Furie KL, Buchan AM, et al. Mechanisms of hemorrhagic transformation after tissue plasminogen activator reperfusion therapy for ischemic stroke. Stroke. (2004) 35(11 Suppl. 1):2726–30. doi: 10.1161/01.STR.0000143219.16695.af
41. Toshihisa Sumii EHL. Involvement of MMPs in thrombolysis-associated HT after embolic focal ischemia in rat. Stroke. (2002) 33:831–6. doi: 10.1161/hs0302.104542
42. Lakhan SE, Kirchgessner A, Tepper D, Leonard A. Matrix metalloproteinases and blood-brain barrier disruption in acute ischemic stroke. Front Neurol. (2013) 4:32. doi: 10.3389/fneur.2013.00032
43. Jin R, Yang G, Li G. Molecular insights and therapeutic targets for blood-brain barrier disruption in ischemic stroke: critical role of matrix metalloproteinases and tissue-type plasminogen activator. Neurobiol Dis. (2010) 38:376–85. doi: 10.1016/j.nbd.2010.03.008
44. Markus HS. Cerebral perfusion and stroke. J Neurol Neurosurg Psychiatry. (2004) 75:353–61. doi: 10.1136/jnnp.2003.025825
45. Khatri R, McKinney AM, Swenson B, Janardhan V. Blood– brain barrier, reperfusion injury, and hemorrhagic transformation in acute ischemic stroke. Neurology. (2012) 79:52–7. doi: 10.1212/WNL.0b013e3182697e70
46. Kalinin MN, Khasanova DR, Ibatullin MM. The hemorrhagic transformation index score: a prediction tool in middle cerebral artery ischemic stroke. BMC Neurol. (2017) 17:177. doi: 10.1186/s12883-017-0958-3
47. Gourdin MJ, Bree B, De Kock M. The impact of ischaemia-reperfusion on the blood vessel. Eur J Anaesthesiol. (2009) 26:537–47. doi: 10.1097/EJA.0b013e328324b7c2
48. Simard JM, Kent TA, Chen M, Tarasov KV, Gerzanich V. Brain oedema in focal ischaemia: molecular pathophysiology and theoretical implications. Lancet Neurol. (2007) 6:258–68. doi: 10.1016/S1474-4422(07)70055-8
49. Bernardo-Castro S, Sousa JA, Bras A, Cecilia C, Rodrigues B, Almendra L, et al. Pathophysiology of blood-brain barrier permeability throughout the different stages of ischemic stroke and its implication on hemorrhagic transformation and recovery. Front Neurol. (2020) 11:594672. doi: 10.3389/fneur.2020.594672
50. Granger DN, Kvietys PR. Reperfusion injury and reactive oxygen species: the evolution of a concept. Redox Biol. (2015) 6:524–51. doi: 10.1016/j.redox.2015.08.020
51. Song K, Li Y, Zhang H, An N, Wei Y, Wang L, et al. Oxidative stress-mediated Blood-Brain Barrier (BBB) disruption in neurological diseases. Oxidative Med Cell Longevity. (2020) 2020:1–27. doi: 10.1155/2020/4356386
52. Kelly MA, Shuaib A, Todd KG. Matrix metalloproteinase activation and blood-brain barrier breakdown following thrombolysis. Exp Neurol. (2006) 200:38–49. doi: 10.1016/j.expneurol.2006.01.032
53. Rosell A, Cuadrado E, Ortega-Aznar A, Hernandez-Guillamon M, Lo EH, Montaner J. MMP-9-positive neutrophil infiltration is associated to blood-brain barrier breakdown and basal lamina type IV collagen degradation during hemorrhagic transformation after human ischemic stroke. Stroke. (2008) 39:1121–6. doi: 10.1161/STROKEAHA.107.500868
54. Zhang W, Zhu L, An C, Wang R, Yang L, Yu W, et al. The blood brain barrier in cerebral ischemic injury – disruption and repair. Brain Hemorrhages. (2020) 1:34–53. doi: 10.1016/j.hest.2019.12.004
55. Michinaga S, Koyama Y. Pathogenesis of brain edema and investigation into anti-edema drugs. Int J Mol Sci. (2015) 16:9949–75. doi: 10.3390/ijms16059949
56. El Nawar R, Yeung J, Labreuche J, Chadenat ML, Duong DL, De Malherbe M, et al. MRI-based predictors of hemorrhagic transformation in patients with stroke treated by intravenous thrombolysis. Front Neurol. (2019) 10:897. doi: 10.3389/fneur.2019.00897
57. Kalogeris T, Baines CP, Krenz M, Korthuis RJ. Cell biology of ischemia/reperfusion injury. Int Rev Cell Mol Biol. (2012) 298:229–317. doi: 10.1016/B978-0-12-394309-5.00006-7
58. Gary S. Krause aBRT. suppression of protein synthesis in the reperfused brain. Stroke. (1993) 24:747–56. doi: 10.1161/01.STR.24.5.747
59. Hamann GF, Liebetrau M, Martens H, Burggraf D, Kloss CU, Bültemeier G, et al. Microvascular Basal Lamina Injury After Experimental Focal cerebral ischemia and reperfusion in the rat. J Cerebral Blood Folw Metab. (2002) 22:526–33. doi: 10.1097/00004647-200205000-00004
60. Xing C, Arai K, Lo EH, Hommel M. Pathophysiologic cascades in ischemic stroke. Int J Stroke. (2012) 7:378–85. doi: 10.1111/j.1747-4949.2012.00839.x
61. Kaesmacher J, Boeckh-Behrens T, Simon S, Maegerlein C, Kleine JF, Zimmer C, et al. Risk of thrombus fragmentation during endovascular stroke treatment. AJNR Am J Neuroradiol. (2017) 38:991–8. doi: 10.3174/ajnr.A5105
62. van Kranendonk KR, Treurniet KM, Boers AMM, Berkhemer OA, van den Berg LA, Chalos V, et al. Clinical and imaging markers associated with hemorrhagic transformation in patients with acute ischemic stroke. Stroke. (2019) 50:2037–43. doi: 10.1161/STROKEAHA.118.024255
63. Vo KD, Santiago F, Lin W, Hsu CY, Lee Y, Lee JM. MR imaging enhancement patterns as predictors of HT in AIS. AJNR Am J Neuroradiol. (2003) 24:674–9.
64. Lu G, He Q, Shen Y, Cao F. Potential biomarkers for predicting hemorrhagic transformation of ischemic stroke. Int J Neurosci. (2018) 128:79–89. doi: 10.1080/00207454.2017.1349766
65. Neeb L, Villringer K, Galinovic I, Grosse-Dresselhaus F, Ganeshan R, Gierhake D, et al. Adapting the computed tomography criteria of hemorrhagic transformation to stroke magnetic resonance imaging. Cerebrovasc Dis Extra. (2013) 3:103–10. doi: 10.1159/000354371
66. Mazya M, Egido JA, Ford GA, Lees KR, Mikulik R, Toni D, et al. Predicting the risk of symptomatic intracerebral hemorrhage in ischemic stroke treated with intravenous alteplase: safe Implementation of Treatments in Stroke (SITS) symptomatic intracerebral hemorrhage risk score. Stroke. (2012) 43:1524–31. doi: 10.1161/STROKEAHA.111.644815
67. Leira R, Sobrino T, Blanco M, Campos F, Rodriguez-Yanez M, Castellanos M, et al. A higher body temperature is associated with haemorrhagic transformation in patients with acute stroke untreated with recombinant tissue-type plasminogen activator (rtPA). Clin Sci. (2012) 122:113–9. doi: 10.1042/CS20110143
68. Guo Y, Yang Y, Zhou M, He L. Risk factors of haemorrhagic transformation for acute ischaemic stroke in Chinese patients receiving intravenous recombinant tissue plasminogen activator: a systematic review and meta-analysis. Stroke Vasc Neurol. (2018) 3:203–8. doi: 10.1136/svn-2018-000141
69. Kim TJ, Park HK, Kim JM, Lee JS, Park SH, Jeong HB, et al. Blood pressure variability and hemorrhagic transformation in patients with successful recanalization after endovascular recanalization therapy: a retrospective observational study. Ann Neurol. (2019) 85:574–81. doi: 10.1002/ana.25434
70. Whiteley WN, Slot KB, Fernandes P, Sandercock P, Wardlaw J. Risk factors for intracranial hemorrhage in acute ischemic stroke patients treated with recombinant tissue plasminogen activator: a systematic review and meta-analysis of 55 studies. Stroke. (2012) 43:2904–9. doi: 10.1161/STROKEAHA.112.665331
71. Ko S-B, Park H-K, Kim BM, Heo JH, Rha J-H, Kwon SU, et al. 2019 update of the Korean clinical practice guidelines of stroke for endovascular recanalization therapy in patients with acute ischemic stroke. J Korean Neurol Assoc. (2020) 38:77–87. doi: 10.17340/jkna.2020.2.1
72. Foerch C, Wunderlich MT, Dvorak F, Humpich M, Kahles T, Goertler M, et al. Elevated serum S100B levels indicate a higher risk of hemorrhagic transformation after thrombolytic therapy in acute stroke. Stroke. (2007) 38:2491–5. doi: 10.1161/STROKEAHA.106.480111
73. Ribo M, Montaner J, Molina CA, Arenillas JF, Santamarina E, Quintana M, et al. Admission fibrinolytic profile is associated with symptomatic hemorrhagic transformation in stroke patients treated with tissue plasminogen activator. Stroke. (2004) 35:2123–7. doi: 10.1161/01.STR.0000137608.73660.4c
74. Rodriguez-Gonzalez R, Blanco M, Rodriguez-Yanez M, Moldes O, Castillo J, Sobrino T. Platelet derived growth factor-CC isoform is associated with hemorrhagic transformation in ischemic stroke patients treated with tissue plasminogen activator. Atherosclerosis. (2013) 226:165–71. doi: 10.1016/j.atherosclerosis.2012.10.072
75. Jickling GC, Ander BP, Stamova B, Zhan X, Liu D, Rothstein L, et al. RNA in blood is altered prior to hemorrhagic transformation in ischemic stroke. Ann Neurol. (2013) 74:232–40. doi: 10.1002/ana.23883
76. del Rio-Espinola A, Fernandez-Cadenas I, Giralt D, Quiroga A, Gutierrez-Agullo M, Quintana M, et al. A predictive clinical-genetic model of tissue plasminogen activator response in acute ischemic stroke. Ann Neurol. (2012) 72:716–29. doi: 10.1002/ana.23664
77. Gonzalez-Conejero R, Fernandez-Cadenas I, Iniesta JA, Marti-Fabregas J, Obach V, Alvarez-Sabin J, et al. Role of fibrinogen levels and factor XIII V34L polymorphism in thrombolytic therapy in stroke patients. Stroke. (2006) 37:2288–93. doi: 10.1161/01.STR.0000236636.39235.4f
78. Bivard A, Kleinig T, Churilov L, Levi C, Lin L, Cheng X, et al. Permeability measures predict hemorrhagic transformation after ischemic stroke. Ann Neurol. (2020) 88:466–76. doi: 10.1002/ana.25785
79. Kidwell CS, Latour L, Saver JL, Alger JR, Starkman S, Duckwiler G, et al. Thrombolytic toxicity: blood brain barrier disruption in human ischemic stroke. Cerebrovasc Dis. (2008) 25:338–43. doi: 10.1159/000118379
80. Tong DC, Adami A, Moseley ME, Marks MP. Relationship between apparent diffusion coefficient and subsequent hemorrhagic transformation following acute ischemic stroke. Stroke. (2000) 31:2378–84. doi: 10.1161/01.STR.31.10.2378
81. Nisar T, Hanumanthu R, Khandelwal P. Symptomatic intracerebral hemorrhage after intravenous thrombolysis: predictive factors and validation of prediction models. J Stroke Cerebrovasc Dis. (2019) 28:104360. doi: 10.1016/j.jstrokecerebrovasdis.2019.104360
82. Cucchiara B, Tanne D, Levine SR, Demchuk AM, Kasner S. A risk score to predict intracranial hemorrhage after recombinant tissue plasminogen activator for acute ischemic stroke. J Stroke Cerebrovasc Dis. (2008) 17:331–3. doi: 10.1016/j.jstrokecerebrovasdis.2008.03.012
83. Strbian D, Engelter S, Michel P, Meretoja A, Sekoranja L, Ahlhelm FJ, et al. Symptomatic intracranial hemorrhage after stroke thrombolysis: the SEDAN score. Ann Neurol. (2012) 71:634–41. doi: 10.1002/ana.23546
84. Saposnik G, Guzik AK, Reeves M, Ovbiagele B, Johnston SC. Stroke prognostication using age and NIH stroke scale; SPAN-100. Neurology. (2013) 80:21–8. doi: 10.1212/WNL.0b013e31827b1ace
85. Flint AC, Faigeles BS, Cullen SP, Kamel H, Rao VA, Gupta R, et al. THRIVE score predicts ischemic stroke outcomes and thrombolytic hemorrhage risk in VISTA. Stroke. (2013) 44:3365–9. doi: 10.1161/STROKEAHA.113.002794
86. Hemphill JC III, Greenberg SM, Anderson CS, Becker K, Bendok BR, Cushman M, et al. Guidelines for the management of spontaneous intracerebral hemorrhage: a guideline for healthcare professionals from the American Heart Association/American Stroke Association. Stroke. (2015) 46:2032–60. doi: 10.1161/STR.0000000000000069
87. Yaghi S, Willey JZ, Cucchiara B, Goldstein JN, Gonzales NR, Khatri P, et al. Treatment and outcome of hemorrhagic transformation after intravenous alteplase in acute ischemic stroke: a scientific statement for healthcare professionals from the American Heart Association/American Stroke Association. Stroke. (2017) 48:e343–61. doi: 10.1161/STR.0000000000000152
88. Qureshi AI, Malik AA, Adil MM, Defillo A, Sherr GT, Suri MF. Hematoma enlargement among patients with traumatic brain injury; analysis of a prospective multicenter clinical trial. J Vasc Interv Neurol. (2015) 8:42–9.
89. Rodriguez-Luna D, Pineiro S, Rubiera M, Ribo M, Coscojuela P, Pagola J, et al. Impact of blood pressure changes and course on hematoma growth in acute intracerebral hemorrhage. Eur J Neurol. (2013) 20:1277–83. doi: 10.1111/ene.12180
90. Butcher KS, Jeerakathil T, Hill M, Demchuk AM, Dowlatshahi D, Coutts SB, et al. The intracerebral hemorrhage acutely decreasing arterial pressure trial. Stroke. (2013) 44:620–6. doi: 10.1161/STROKEAHA.111.000188
91. Silverman A, Kodali S, Sheth KN, Petersen NH. Hemodynamics and hemorrhagic transformation after endovascular therapy for ischemic stroke. Front Neurol. (2020) 11:728. doi: 10.3389/fneur.2020.00728
92. Anderson CS, Huang Y, Lindley RI, Chen X, Arima H, Chen G, et al. Intensive blood pressure reduction with intravenous thrombolysis therapy for acute ischaemic stroke (ENCHANTED): an international, randomised, open-label, blinded-endpoint, phase 3 trial. Lancet. (2019) 393:877–88. doi: 10.1016/S0140-6736(19)30038-8
93. McManus M, Liebeskind DS. Blood pressure in acute ischemic stroke. J Clin Neurol. (2016) 12:137–46. doi: 10.3988/jcn.2016.12.2.137
94. Rasmussen M, Schonenberger S, Henden PL, Valentin JB, Espelund US, Sorensen LH, et al. Blood pressure thresholds and neurologic outcomes after endovascular therapy for acute ischemic stroke: an analysis of individual patient data from 3 randomized clinical trials. JAMA Neurol. (2020) 77:622–31. doi: 10.1001/jamaneurol.2019.4838
95. Wajngarten M, Silva GS. Hypertension and stroke: update on treatment. Eur Cardiol. (2019) 14:111–5. doi: 10.15420/ecr.2019.11.1
96. Kim SM, Woo HG, Kim YJ, Kim BJ. Blood pressure management in stroke patients. J Neurocritic Care. (2020) 13:69–79. doi: 10.18700/jnc.200028
97. Mattle HP, Kappeler L, Arnold M, Fischer U, Nedeltchev K, Remonda L, et al. Blood pressure and vessel recanalization in the first hours after ischemic stroke. Stroke. (2005) 36:264–8. doi: 10.1161/01.STR.0000153052.59113.89
98. Vitt JR, Trillanes M, Hemphill JC III. Management of blood pressure during and after recanalization therapy for acute ischemic stroke. Front Neurol. (2019) 10:138. doi: 10.3389/fneur.2019.00138
99. Lee KS. How to treat chronic subdural hematoma? Past and now. J Korean Neurosurg Soc. (2019) 62:144–52. doi: 10.3340/jkns.2018.0156
100. Yong M, Kaste M. Association of characteristics of blood pressure profiles and stroke outcomes in the ECASS-II trial. Stroke. (2008) 39:366–72. doi: 10.1161/STROKEAHA.107.492330
101. Stead LG, Gilmore RM, Vedula KC, Weaver AL, Decker WW, Brown RD Jr. Impact of acute blood pressure variability on ischemic stroke outcome. Neurology. (2006) 66:1878–81. doi: 10.1212/01.wnl.0000219628.78513.b5
102. Powers WJ, Rabinstein AA, Ackerson T, Adeoye OM, Bambakidis NC, Becker K, et al. 2018 Guidelines for the early management of patients with acute ischemic stroke: a guideline for healthcare professionals from the American Heart Association/American Stroke Association. Stroke. (2018) 49:e46–110. doi: 10.1161/STR.0000000000000158
103. Mistry EA, Sucharew H, Mistry AM, Mehta T, Arora N, Starosciak AK, et al. Blood Pressure after Endovascular Therapy for Ischemic Stroke (BEST): a multicenter prospective cohort study. Stroke. (2019) 50:3449–55. doi: 10.1161/STROKEAHA.119.026889
104. Mazighi M, Labreuche J, Richard S, Gory B, Lapergue B, Sibon I, et al. Blood pressure target in acute stroke to reduce hemorrhage after endovascular therapy: the randomized BP TARGET study protocol. Front Neurol. (2020) 11:480. doi: 10.3389/fneur.2020.00480
105. Grune F, Klimek M. Cerebral blood flow and its autoregulation - when will there be some light in the black box? Br J Anaesth. (2017) 119:1077–9. doi: 10.1093/bja/aex355
106. Jordan JD, Powers WJ. Cerebral autoregulation and acute ischemic stroke. Am J Hypertens. (2012) 25:946–50. doi: 10.1038/ajh.2012.53
107. Wang A, Ortega-Gutierrez S, Petersen NH. Autoregulation in the Neuro ICU. Curr Treat Options Neurol. (2018) 20:20. doi: 10.1007/s11940-018-0501-x
108. Petersen NH, Ortega-Gutierrez S, Reccius A, Masurkar A, Huang A, Marshall RS. Dynamic cerebral autoregulation is transiently impaired for one week after large-vessel acute ischemic stroke. Cerebrovasc Dis. (2015) 39:144–50. doi: 10.1159/000368595
109. Castro P, Azevedo E, Sorond F. Cerebral autoregulation in stroke. Curr Atheroscler Rep. (2018) 20:37. doi: 10.1007/s11883-018-0739-5
110. Castro P, Serrador JM, Rocha I, Sorond F, Azevedo E. Efficacy of cerebral autoregulation in early ischemic stroke predicts smaller infarcts and better outcome. Front Neurol. (2017) 8:113. doi: 10.3389/fneur.2017.00113
111. Allen LM, Hasso AN, Handwerker J, Farid H. Sequence-specific MR imaging findings that are useful in dating ischemic stroke. Radiographics. (2012) 32:1285–97; discussion 97–9. doi: 10.1148/rg.325115760
112. von Kummer R, Broderick JP, Campbell BC, Demchuk A, Goyal M, Hill MD, et al. The heidelberg bleeding classification: classification of bleeding events after ischemic stroke and reperfusion therapy. Stroke. (2015) 46:2981–6. doi: 10.1161/STROKEAHA.115.010049
113. Claude Hemphill J III, Lam A. Emergency neurological life support: intracerebral hemorrhage. Neurocrit Care. (2017) 27(Suppl. 1):89–101. doi: 10.1007/s12028-017-0453-0
114. Carcel C, Wang X, Sato S, Stapf C, Sandset EC, Delcourt C, et al. Degree and timing of intensive blood pressure lowering on hematoma growth in intracerebral hemorrhage: intensive blood pressure reduction in acute cerebral hemorrhage trial-2 results. Stroke. (2016) 47:1651–3. doi: 10.1161/STROKEAHA.116.013326
115. Rocha E, Rouanet C, Reges D, Gagliardi V, Singhal AB, Silva GS. Intracerebral hemorrhage: update and future directions. Arq Neuropsiquiatr. (2020) 78:651–9. doi: 10.1590/0004-282x20200088
116. Weiser RE, Sheth KN. Clinical predictors and management of hemorrhagic transformation. Curr Treat Options Neurol. (2013) 15:125–49. doi: 10.1007/s11940-012-0217-2
117. Wang J, Wu QY, Du CP, Liu J, Zhang H, Wang JY, et al. Spontaneous cerebellar hemorrhage with severe brainstem dysfunction through minimally invasive puncture treatment by locating the simple bedside. Medicine. (2019) 98:e17211. doi: 10.1097/MD.0000000000017211
118. Manno EM, Atkinson JL, Fulgham JR, Wijdicks EF. Emerging medical and surgical management strategies in the evaluation and treatment of intracerebral hemorrhage. Mayo Clin Proc. (2005) 80:420–33. doi: 10.4065/80.3.420
119. Goldstein JN, Marrero M, Masrur S, Pervez M, Barrocas AM, Abdullah A, et al. Management of thrombolysis-associated symptomatic intracerebral hemorrhage. Arch Neurol. (2010) 67:965–9. doi: 10.1001/archneurol.2010.175
120. Mendelow AD, Gregson BA, Rowan EN, Murray GD, Gholkar A, Mitchell PM. Early surgery versus initial conservative treatment in patients with spontaneous supratentorial lobar intracerebral haematomas (STICH II): a randomised trial. Lancet. (2013) 382:397–408. doi: 10.1016/S0140-6736(13)60986-1
121. Creutzfeldt CJ, Tirschwell DL, Kim LJ, Schubert GB, Longstreth WT Jr, Becker KJ. Seizures after decompressive hemicraniectomy for ischaemic stroke. J Neurol Neurosurg Psychiatry. (2014) 85:721–5. doi: 10.1136/jnnp-2013-305678
122. Arnould MC, Grandin CB, Peeters A, Cosnard G, Duprez TP. Comparison of CT and three MR sequences for detecting and categorizing early (48 Hours) hemorrhagic transformation in hyperacute ischemic stroke. AJNR Am J Neuroradiol. (2004) 25:939–44.
123. Ishiguro M, Mishiro K, Fujiwara Y, Chen H, Izuta H, Tsuruma K, et al. Phosphodiesterase-III inhibitor prevents hemorrhagic transformation induced by focal cerebral ischemia in mice treated with tPA. PLoS One. (2010) 5:e15178. doi: 10.1371/journal.pone.0015178
124. Nonaka Y, Tsuruma K, Shimazawa M, Yoshimura S, Iwama T, Hara H. Cilostazol protects against hemorrhagic transformation in mice transient focal cerebral ischemia-induced brain damage. Neurosci Lett. (2009) 452:156–61. doi: 10.1016/j.neulet.2009.01.039
125. Takagi T, Hara H. Protective effects of cilostazol against hemorrhagic stroke: current and future perspectives. J Pharmacol Sci. (2016) 131:155–61. doi: 10.1016/j.jphs.2016.04.023
126. Turner RJ, Sharp FR. Implications of MMP9 for blood brain barrier disruption and hemorrhagic transformation following ischemic stroke. Front Cell Neurosci. (2016) 10:56. doi: 10.3389/fncel.2016.00056
127. Murata Y, Rosell A, Scannevin RH, Rhodes KJ, Wang X, Lo EH. Extension of the thrombolytic time window with minocycline in experimental stroke. Stroke. (2008) 39:3372–7. doi: 10.1161/STROKEAHA.108.514026
128. Switzer JA, Hess DC, Ergul A, Waller JL, Machado LS, Portik-Dobos V, et al. Matrix metalloproteinase-9 in an exploratory trial of intravenous minocycline for acute ischemic stroke. Stroke. (2011) 42:2633–5. doi: 10.1161/STROKEAHA.111.618215
129. Blacker DJ, Prentice D, Alvaro A, Bates TR, Bynevelt M, Kelly A, et al. Reducing haemorrhagic transformation after thrombolysis for stroke: a strategy utilising minocycline. Stroke Res Treat. (2013) 2013:362961. doi: 10.1155/2013/362961
130. Choi MH, Gil YE, Lee SJ, Lee JS, Hong JH, Sohn SI, et al. The clinical usefulness of targeted temperature management in acute ischemic stroke with malignant trait after endovascular thrombectomy. Neurocrit Care. (2020) 34:990–9. doi: 10.1007/s12028-020-01069-0
Keywords: cerebral hemorrhage, stroke, acute, hemorrhagic transformation (HT), risk factors, reperfusion
Citation: Hong JM, Kim DS and Kim M (2021) Hemorrhagic Transformation After Ischemic Stroke: Mechanisms and Management. Front. Neurol. 12:703258. doi: 10.3389/fneur.2021.703258
Received: 30 April 2021; Accepted: 21 October 2021;
Published: 30 November 2021.
Edited by:
Sang-Bae Ko, Seoul National University Hospital, South KoreaReviewed by:
Tae Jung Kim, Seoul National University Hospital, South KoreaJesse Claude Hemphill, University of California, San Francisco, United States
Copyright © 2021 Hong, Kim and Kim. This is an open-access article distributed under the terms of the Creative Commons Attribution License (CC BY). The use, distribution or reproduction in other forums is permitted, provided the original author(s) and the copyright owner(s) are credited and that the original publication in this journal is cited, in accordance with accepted academic practice. No use, distribution or reproduction is permitted which does not comply with these terms.
*Correspondence: Ji Man Hong, ZGFjZGFAaGFubWFpbC5uZXQ=