- 1Cancer Center, Beijing Ditan Hospital, Capital Medical University, Beijing, China
- 2Neurosurgery Department, Beijing Ditan Hospital, Capital Medical University, Beijing, China
- 3Emergency Department, Beijing Youan Hospital, Capital Medical University, Beijing, China
Background: Immunotherapy has shown promising therapeutic efficacy in various cancers but not gliomas. Circulating lymphocytes play critical roles in cancer control and responses to immune checkpoint inhibitors. Treatment-related lymphopenia has been associated with poor survival in patients with various tumors. This meta-analysis evaluated the risk and impact of lymphopenia in patients with glioma.
Methods: The PubMed, Embase, Web of Science, and Cochrane Library databases were comprehensively searched. Eligible studies were included if they reported the incidence and risk factors of lymphopenia and the impact of lymphopenia on survival. Stata 16.0 was used for this meta-analysis.
Results: A total of 21 studies were included in the final systematic review and 20 were included in the quantitative analysis. The overall incidence of grade III/IV lymphopenia was 31.6% [95% confidence interval (CI), 22.3–40.8%]. Pooled results based on pathology of glioma revealed that the incidence in astrocytoma and astrocytoma oligodendroglioma patients was 20.2% (95% CI:5.9–34.4%), and the incidence in glioblastoma patients was 27.6% (95% CI:16.2–38.9%). Lymphopenia was associated with poor overall survival (hazard ratio, 1.99; 95% CI, 1.74–2.27; P < 0.001) compared to no lymphopenia. Brain receiving radiation dose of 20 or 25 Gy, female sex, older age, lower baseline lymphocyte count, and dexamethasone dose > 2 mg instead of baseline use were risk factors for lymphopenia.
Conclusions: Treatment-related lymphopenia was associated with decreased survival in patients with glioma. Optimization of chemoradiation regimens, particularly in patients with concurrent risk factors, can reduce lymphopenia and potentially improve survival in the era of immunotherapy.
Introduction
Gliomas are the most common type of central nervous system tumors among adults (1). Although glioma patients receive a combination of radiation therapy (RT) and chemotherapy as part of their treatment paradigm, either concomitantly and/or sequentially, the overall survival (OS) in cases of high-grade glioma, such as glioblastoma (GBM) remains poor (2). In recent years, immune checkpoint inhibitors have been effective for the treatment of various tumors, especially melanoma and non-small cell lung cancer (3, 4). However, immunotherapy presents a major challenge in glioma treatment because the brain is an immune-privileged organ. Increased understanding of immune characteristics during and after glioma treatment may provide insight into the optimal application of immunotherapeutic modalities.
Circulating lymphocytes have long been considered primary effector cells in the anti-tumor response, and a lack of lymphocytes could weaken the immune system's ability to eliminate tumor cells (5, 6). RT, temozolomide (TMZ), and glucocorticoids are routinely used to treat patients with high-grade gliomas. Each treatment causes lymphocyte toxicity. However, the concomitant use of these treatments is inevitable in clinical practice, thereby further inducing substantial lymphopenia, intensive immunosuppression, and opportunistic infections.
Lymphocytes are particularly sensitive to RT. Previous studies have demonstrated that lethal doses that decrease the surviving fraction by 50 and 90% are 2 and 3 Gy, respectively (7). A mathematical model revealed that the mean dose to circulating lymphocytes was 2.2 Gy, and 99% of lymphocytes received ≥ 0.5 Gy after a routine course of 30 fractions of 2 Gy RT (8). An observational study reported that a reduction of CD4 counts to <200/mm3 was observed in 17 (24%) of 70 patients following RT and glucocorticoids before the TMZ era (9). Indeed, recent studies and meta-analyses have reported that RT-induced lymphopenia is associated with a decreased survival in various cancers, including lung cancer, esophageal squamous cell carcinoma, and pancreatic cancer (10, 11). Moreover, severe and persistent lymphopenia has also been observed in patients with high-grade glioma after RT and TMZ (12, 13). Ongoing clinical trials are aiming to add nivolumab, an immune checkpoint inhibitor against programmed cell death 1 (PD-1), a pathway that downregulates the immune system, to RT [Checkmate 498, (NCT02617589)] or RT/TMZ [Checkmate 548 (NCT02667587)]. However, the latest results have suggested that nivolumab + RT or nivolumab + RT + TMZ did not show better efficacy than standard treatment, possibly due to lymphopenia (14).
A few recent studies have reported a relationship between iatrogenic lymphopenia and clinical outcomes in glioma patients (15, 16). Given the variability in lymphocyte counts among the general population and the small sample sizes, we performed this meta-analysis to list and assess studies of treatment-related lymphopenia in patients with newly diagnosed glioma and identify dosimetric and other risk factors for lymphopenia.
Materials and Methods
Search Strategies
The meta-analysis followed the guidelines of PRISMA (Preferred Reporting Items for Systematic Reviews and Meta-Analyses). A comprehensive electronic search was conducted using PubMed, EMBASE, Cochrane Library, and the Web of Science up to April 18, 2021. The search strategy was based on the following key words: “glioma,” “radiation,” “temozolomide,” “lymphopenia.” Details of the retrieval strategy are provided in Supplementary Table 1. The references in the identified studies were also traced to other relevant studies.
Study Selection Criteria
The inclusion criteria for the study were as follows:
(1) Patients newly diagnosed with glioma
(2) Patients treated with concomitant chemoradiotherapy with TMZ followed by adjuvant TMZ
(3) Having data on treatment-related lymphopenia
(4) Sufficient data were provided to calculate hazard ratios (HRs) and 95% confidence intervals (CIs)
(5) Prospective or retrospective studies
(6) Articles were published in full texts, excluding the following:
a. Case reports, animal experiments, conference abstracts, editorials, and reviews
b. Studies with insufficient information to evaluate HRs and 95% CIs
c. Patients who were included in identified articles in immunodeficiency states or using immune checkpoint inhibitors.
d. Studies that were not communicated in English
Data Extraction and Quality Assessment
Two investigators independently screened the studies that met our inclusion criteria and extracted the relevant information. Disagreements were resolved through discussions with an independent expert. The following information was extracted: first author's name, publication year, study design, country, sample size, age, sex, pathological type, treatment regimen, the cut-off to categorize high and low lymphocyte level, steroid use, HRs for OS, and 95% CIs. The incidence of severe lymphopenia was characterized based on and grade 3 or higher as reported by each studies using National Cancer Institute's Common Terminology Criteria for Adverse Events (CTCAE) definitions. The Quality Assessment of the Newcastle-Ottawa Scale (NOS) was used to evaluate the quality of eligible studies.
Statistical Analysis
For each study, the proportion of patients with severe lymphopenia was calculated, and a 95% exact confidence interval was derived. HRs with their 95% CIs from the included studies were used to calculate the pooled HR. Heterogeneity was evaluated using the Higgins I2 statistic, and I2 > 50% was defined as significant heterogeneity. A fixed effect model or random effect model was used according to the heterogeneity of the pooled results. The data were synthesized using a fixed-effects model with I2 <50%. Otherwise, a random-effect model was used. All statistical tests were two-sided, and statistical significance was defined as p < 0.05. Pooled data were analyzed using STATA 16.0 (Stata Corp, College Station, TX).
Results
Study Selection and Characteristics
A flow diagram of the literature selection process is shown in Figure 1. Our search yielded 2,374 relevant hits from the selected databases. A total of 1,616 records were included after duplicate removal. Among them, 1,552 were excluded after the titles and abstracts screening, leaving 64 relevant articles. The full-text articles of these 64 studies were reviewed; of them, 21 met the inclusion criteria. A total of 15 studies (12, 17–30) that evaluated the incidence of severe lymphopenia and six (12, 13, 15, 16, 31, 32) that evaluated the prognostic impact of lymphopenia on OS were selected. Furthermore, five studies (21, 30–33) that reported risk factors for lymphopenia on multivariate analysis were also included. As Mohan et al. (30) reported the incidence of lymphopenia in two cohorts in which patients were treated with proton therapy or X-ray therapy, we termed them “Mohan 2021 cohort 1” and “Mohan 2021 cohort 2,” respectively. The characteristics of the included studies are summarized in Tables 1, 2.
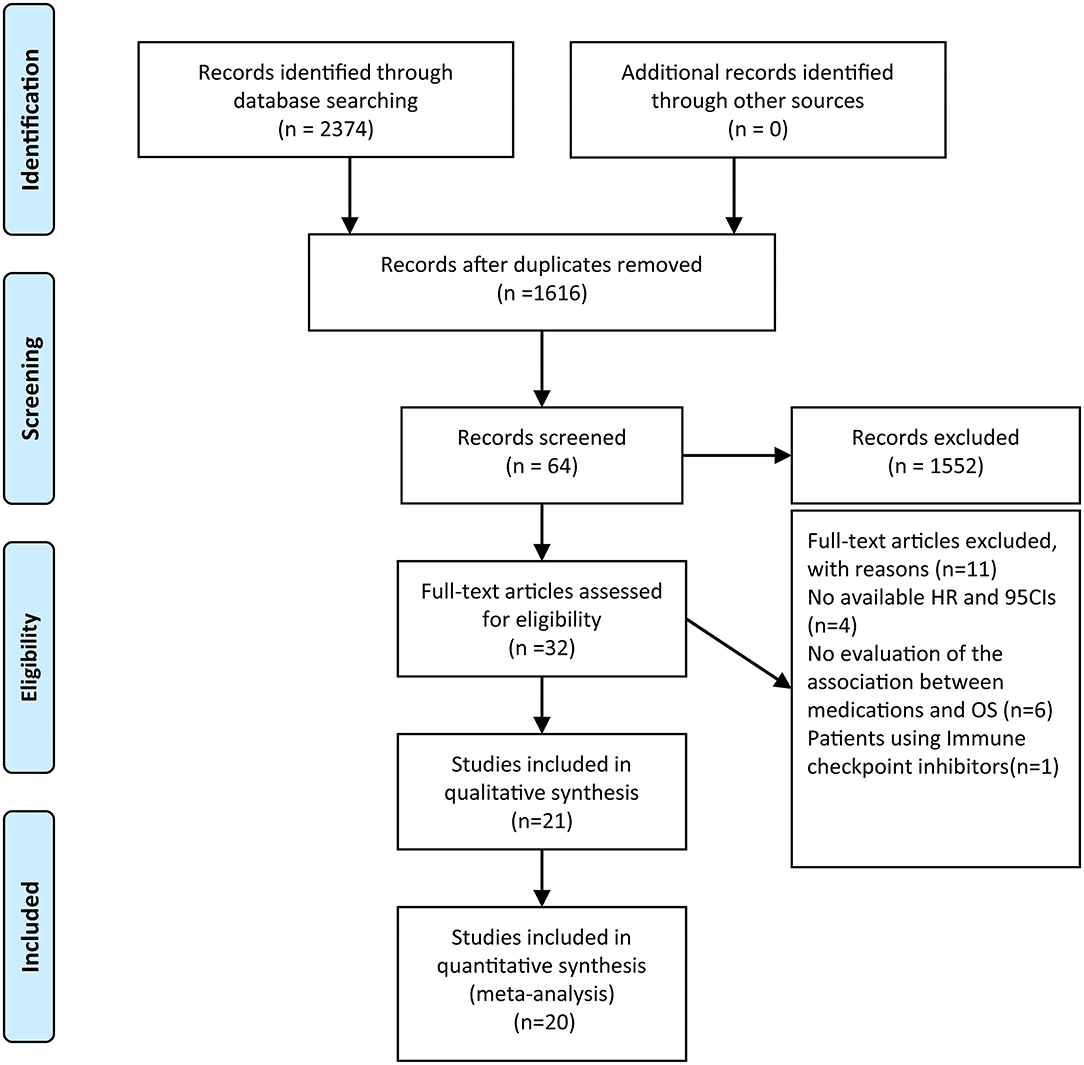
Figure 1. Flow chart of literature search and study selection. After carefully reviewed 21 studies were included in the final systematic review and 20 in the quantitative analysis.
Incidence of Severe Lymphopenia
A total of 1,944 patients with newly diagnosed glioma who were treated with concurrent RT/TMZ and adjuvant monthly TMZ were included in this analysis. The incidence of high-grade (i.e., III/IV) lymphopenia in these studies was 31.6% (95%CI: 22.3−40.8%) (Figure 2). Moreover, as several studies including multiple types of glioma (e.g., astrocytoma, oligodendroglioma, and GBM), pooled results based on the pathology of glioma revealed that the incidence in astrocytoma and astrocytoma oligodendroglioma patients was 20.2% (95% CI:5.9–34.4%) (Figure 3), while that in GBM patients was 27.6% (95% CI:16.2–38.9%) (Figure 4).
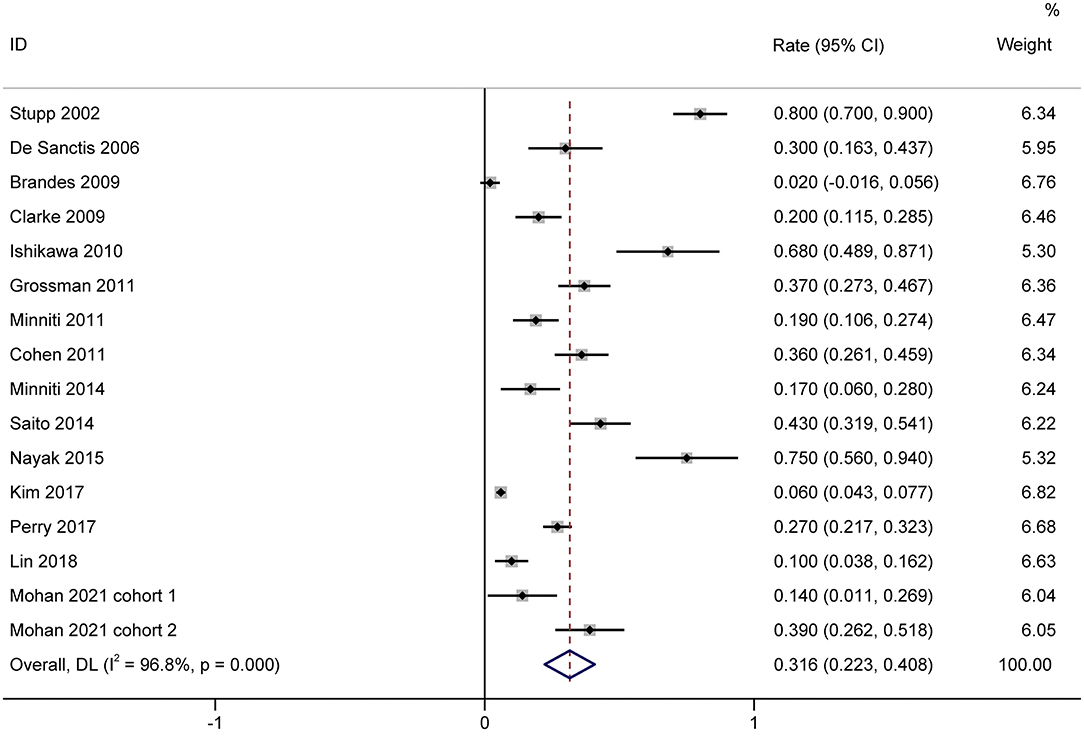
Figure 2. Forest plots of the incidence rate of severe lymphopenia and 95% CIs in glioma patients. Weights are from random-effects model.
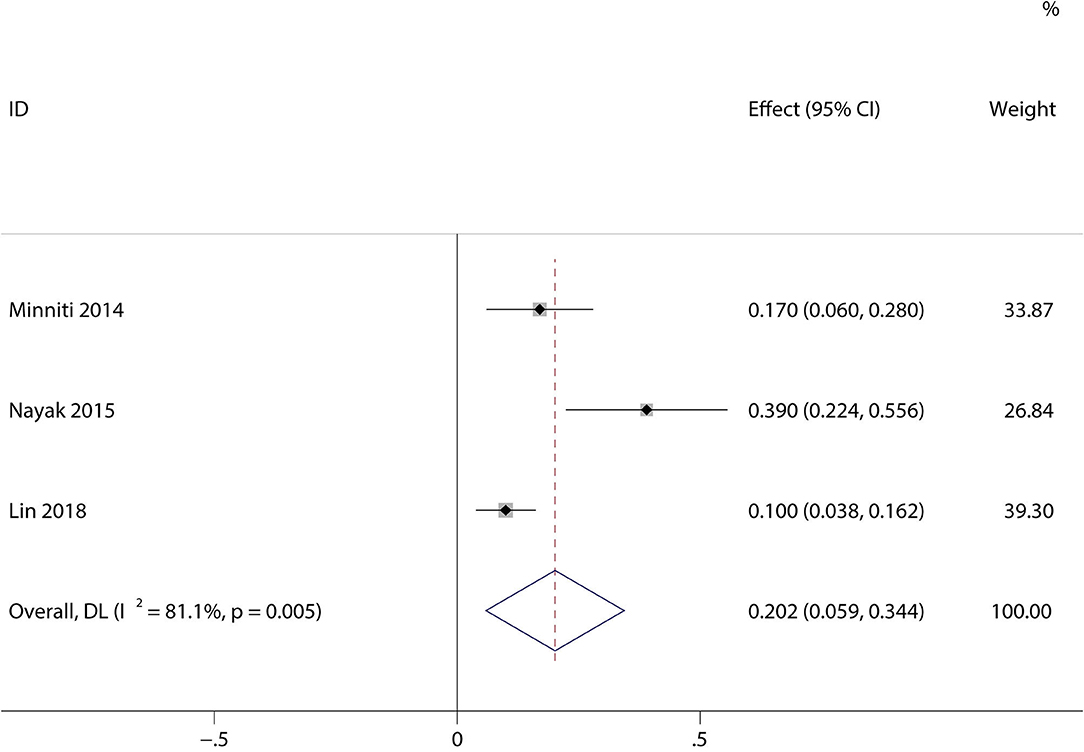
Figure 3. Forest plots of the incidence rate of severe lymphopenia and 95% CIs in astrocytoma and astrocytoma oligodendroglioma patients. Weights are from random-effects model.
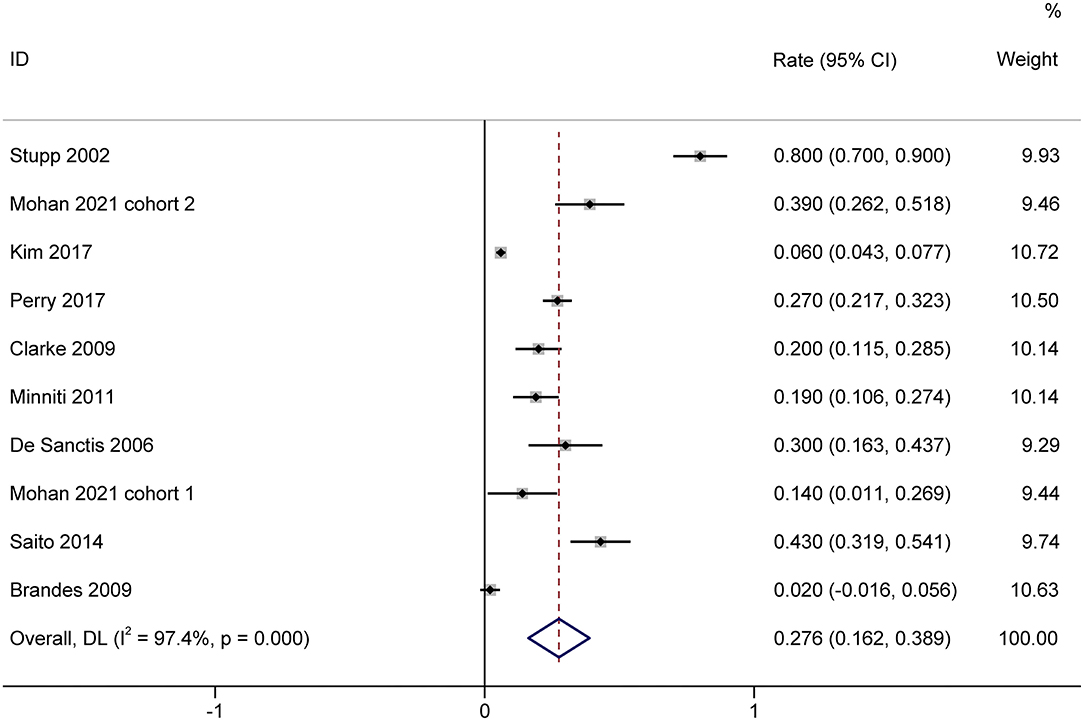
Figure 4. Forest plots of the incidence rate of severe lymphopenia and 95% CIs in GBM glioma patients. HR, hazard ratio; CI, confidence Interval; GBM, Glioblastoma. Weights are from random-effects model.
Impact of Lymphopenia on OS
The effect of treatment-related lymphopenia on OS was evaluated in 819 patients with newly diagnosed gliomas. Among them, one study of patients with various malignant gliomas was prospective, while the others that included GBM were retrospective. Most studies included patients with a median age of 54.2–59 years, while only Mendez et al. (13) included elderly GBM patients (age ≥ 65 years). The pooled results showed that patients with treatment-related lymphopenia had decreased survival (HR, 1.99; 95% CI, 1.74–2.27; P<0.001) compared to patients without lymphopenia (Figure 5). Similarly, lymphopenia was associated with inferior survival in patients with GBM after the exclusion of studies of patients with various malignant gliomas (HR, 2.00; 95% CI, 1.74–2.31; P< 0.001). We further conducted subgroup analyses of patients with GBM. Subgroup analyses by sex (female <50 vs. female ≥ 50%) showed that lymphopenia led to poor OS for patients in both subgroups (female <50%: HR = 2.01 95% CI = 1.53–2.66, P< 0.001; female ≥ 50%: HR = 2.00, 95% CI = 1.74–2.31, P< 0.001). The results of subgroups based on steroid use (<50 vs. ≥50%) demonstrated that lymphopenia led to poor OS in both subgroups (steroid use <50%: HR = 1.98, 95% CI = 1.70–2.32, P< 0.001; steroid use ≥ 50%: HR = 2.03, 95% CI = 1.75–2.35, P< 0.001).
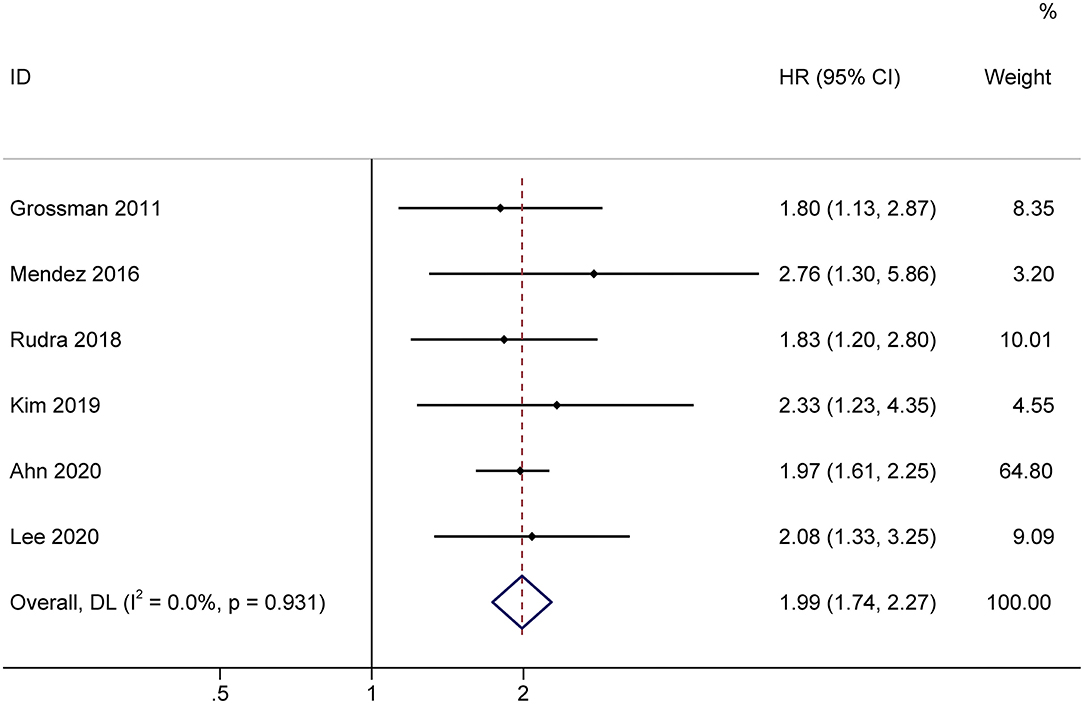
Figure 5. Forest plots of the prognostic impact of treatment-related lymphopenia on overall survival in glioma patients. HR, hazard ratio; CI, confidence Interval. Weights are from random-effects model.
Risk Factors for Lymphopenia
Five studies evaluated dosimetric parameters and other risk factors for lymphopenia (Table 3). Three studies reported that brain volume (receiving 25 or 20 Gy) was associated with an increased risk of lymphopenia. Moreover, female sex (four studies), older age (two studies) and lower baseline lymphocyte count (three studies) were also associated with increased risk of lymphopenia. Notably, several studies reported that baseline steroid use was not significantly associated with lymphopenia development, while a dexamethasone dose > 2 mg during RT was associated with an increased risk of lymphopenia.
Discussion
This meta-analysis pooled 1,944 glioma patients treated with concurrent RT/TMZ and adjuvant monthly TMZ to calculate the incidence of high-grade lymphopenia. Moreover, a total of 819 malignant glioma patients from six studies were pooled to examine the impact of treatment-induced lymphopenia on OS. We found that patients with post-treatment lymphopenia had poor OS compared to those with normal lymphocyte counts.
Lymphocytes, an essential component of the tumor microenvironment, have attracted interest in the era of immunotherapy. A reduced lymphocyte count is reportedly associated with inferior response rates, an increased risk of recurrence, and worse OS across various cancers, before, during or after treatment (6). Our study further confirmed that a decreased total lymphocyte count is associated with poor clinical outcomes in case of glioma, although the underlying mechanism has not been fully elucidated. One potential explanation for this finding is that the decline in lymphocytes after cytotoxic antineoplastic therapy may enhance the ability of surviving tumors to evade the immune system. Lymphocytes are considered essential for the suppression of tumor development within the immune microenvironment and as regulators of the immune surveillance process, thereby leading to poor prognosis in high-grade gliomas (2, 14).
RT, TMZ, and steroids are known to be lymphotoxic treatments in the routine management of patients with glioma. RT has long been considered an essential contributor to treatment-related lymphopenia in the management of various patients. RT-induced lymphopenia was first described in a large study that estimated long-term lymphocytes in women with breast cancer in 1971 (34), and it has since been observed in various cancers, such as glioma, pancreatic, lung, head and neck, esophageal, cervical, and bladder cancers (11, 35–37). Various circulating lymphocyte subpopulations that are indispensable parts of the immune response to cancer cells are influenced by RT, although the CD4 count was initially noted in a prospective study. Campian et al. (38) demonstrated that lymphocyte subpopulations, including immune cells of innate and adaptive immunity, were reduced in GBM patients after RT. Notably, regulatory T cells), a lymphocyte subpopulation that suppresses and controls the immune response, increased in patients with medulloblastoma during chemoradiotherapy, but decreased afterward (39). A possible mechanism behind lymphopenia in the brain is that a 6-week course of RT delivers a lymphotoxic dose to 99% of circulating blood cells due to limited bone marrow or lymphoid tissue exposure during RT (8). Hypofractionated radiation have been proven to be lymphoprotective because they have less of an effect on circulating lymphocytes (40). Furthermore, a higher brain volume receiving either 25 or 20 Gy was associated with a higher risk of lymphopenia. Huang et al. (33) first identified the optimal cutoff value for brain V25 Gy as 56% based on an outcome-oriented cut point determination method. Rudra et al. (31) further confirmed brain V25 Gy as the most significant dosimetric predictor of lymphopenia in GBM. Mohan et al. (30) reported brain V20 Gy was the most significant dosimetric factor associated with lymphopenia and that proton therapy yielded a lower Brain V20 Gy than photon therapy. The variation between BrainV25Gy vs. Brain V20 Gy might be partly explained by the fact that 33% percent of the patients received proton therapy. A recent study reported that GBM patients treated with proton therapy had reduced irradiated brain volumes and thus severe lymphopenia compared to patients treated with photon therapy (30). Hence, it is plausible to modify RT treatment strategies to alleviate lymphopenia.
Interestingly, Mendez et al. (13) compared total lymphocyte counts over time in older patients with GBM who received RT doses > 45 Gy vs. RT doses ≤ 45 Gy. The results showed that patients developed similar lymphopenia regardless of the RT dose they received. Lymphocyte counts reduced significantly in both short course (≤ 45 Gy) and longer course (>45 Gy) RT groups. Baseline median lymphocyte counts were 800 cells/mm3 which decreased to 600 cells/mm3 in patients who received RT doses ≤ 45 Gy, while these lymphocyte counts were 1,200 cells/mm3 which fell to 750 cells/mm3 in patients who received RT doses >45 Gy. The lower level of baseline lymphocytes in patients who received short course of RT may be a poor prognostic factor responsible for the shorter survival in these patients. These results are likely due to the small sample size, and an older patient population may have a higher risk of lymphopenia even with a short course of RT.
TMZ, which is associated with myelosuppressive toxicity, also causes lymphopenia. Previous studies reported that lymphopenia occurred in melanoma patients who were administered TMZ alone with a similar dose regimen to GBM patients who received RT/TMZ. A total of 29 (33%) patients developed grade III/IV lymphopenia, while 17 who discontinued TMZ after developing lymphopenia recovered normal lymphocyte counts in the range of 9 to 245+ days (41). The concurrent use of TMZ with RT increased the incidence of lymphopenia compared with RT alone. Perry et al. (28) reported grade III/IV lymphopenia in 10.3% of GBM patients given a shortened 3-week course of RT alone vs. 27.3% with the combination. Notably, Lin et al. (29) reported that concurrent TMZ during RT was the dominant factor resulting in lymphopenia within 3 months in younger patients with less aggressive grade II–III glioma. No cases of acute grade 3 lymphopenia after RT were observed among patients who did not received concurrent TMZ, and lymphopenia gradually mitigated over time in patients who received concurrent TMZ but no additional adjuvant TMZ. The role of concurrent or adjuvant TMZ in the development of lymphopenia in patients with GBM are requires further investigation.
Glucocorticoids are another commonly used lymphotoxic drug to mitigate edema in GBM patients before and after neurosurgery. Although glucocorticoids are considered immunosuppressive, their effects may be modest and dose-dependent. Several studies previously reported that baseline corticosteroid use was not significantly associated with an increased risk of lymphopenia after considering other confounding factors (e.g., tumor burden and radiation dose) (29, 31, 33). However, recent studies reported that cumulative dexamethasone dose is an independent risk factor for the development of lymphopenia during chemoradiation (32). In addition, the association between steroid use and clinical outcomes is controversial and may be affected by lymphopenia. In a recent meta-analysis evaluating the effects of steroids on outcomes in GBM patients treated with RT and/or TMZ, steroids significantly reduced OS and PFS (42). However, steroid use was not significantly associated with survival in our included studies (12, 13, 15, 16, 31, 32) when lymphopenia was included in multivariate models, suggesting that lymphopenia may be a significant adverse prognostic factor for OS.
In addition to the above factors, several baseline factors associated with an increased risk of lymphopenia were identified in various studies. As expected, patients who developed grade III–IV lymphopenia tended to have lower total lymphocyte counts before chemoradiotherapy. Lower lymphocyte counts or even lymphopenia might be a predisposing factor for tumor development and have been observed at the time of GBM diagnosis. Kim et al. (15) showed decreased levels of lymphocytes without steroid use at baseline, implying that lymphopenia is a frequent event in the natural progression of GBM. In addition to lower baseline lymphocyte counts, three studies reported that female sex is strongly associated with an increased risk of lymphopenia. In part, women have greater exposure of circulating lymphocytes to radiation as a higher rate of regional cerebral perfusion than man. Moreover, this sex-based phenomenon plays an important role in response to chemotherapy likely due to discrepancies in pharmacokinetics and pharmacodynamics between the sexes. Older age may reduce lymphocyte count due to telomere shortening and result in poorer physical condition. Currently, only one study included elderly patients with GBM (age ≥ 65) (13). This study showed that lymphopenia timing, severity, and duration were similar to those younger patients.
Our study has several limitations. First, most of the eligible studies were retrospective; thus, it is difficult to determine causation of lymphocytopenia among the volume of radiation, fractionations involved, TMZ, steroid use, and other possible confounding factors. Second, the heterogeneity of glial histological subtypes, the definition of lymphopenia and time of evaluation varied across different studies might have affected the stability and reliability of our analytical results. Third, most studies were unable to investigate the association between molecular markers and lymphopenia in the currently molecularly driven management paradigms, and only one study (13) reported there no association between MGMT and lymphopenia. Future prospective studies are required to incorporate molecular analysis to confirm our findings. Given the heterogeneity among studies, it should be considered hypothesis-generating. This review evaluates the impact of treatment-induced lymphopenia in HGG, and establishes the need for strategies to mitigate lymphopenia in these patients.
In conclusion, our study showed that post-treatment lymphopenia was associated with poor prognosis in high-grade glioma patients treated with RT/TMZ. Female sex, older age, brain receiving radiation dose of 20 or 25 Gy, and pretreatment lymphocyte levels are surrogate markers that predict lymphopenia, and minimizing them can reduce the lymphopenia and potentially improve the treatment outcomes of glioma patients, particularly in the current era of immunotherapy.
Data Availability Statement
The raw data supporting the conclusions of this article will be made available by the authors, without undue reservation.
Author Contributions
WL and EF: conception or design. HC, ShaC, and ZL: collection and/or assembly of data. YZ and ShiC: data analysis, interpretation, and manuscript writing. WL and EF: revision. YZ, ShiC, HC, ShaC, ZL, WL, and EF: final approval of manuscript.
Funding
This study was supported by Capital's Funds for Health Improvement and Research (Grant Number 2020-2475 2175) and Beijing Talents Project.
Conflict of Interest
The authors declare that the research was conducted in the absence of any commercial or financial relationships that could be construed as a potential conflict of interest.
Publisher's Note
All claims expressed in this article are solely those of the authors and do not necessarily represent those of their affiliated organizations, or those of the publisher, the editors and the reviewers. Any product that may be evaluated in this article, or claim that may be made by its manufacturer, is not guaranteed or endorsed by the publisher.
Supplementary Material
The Supplementary Material for this article can be found online at: https://www.frontiersin.org/articles/10.3389/fneur.2021.726561/full#supplementary-material
References
1. Davis ME. Epidemiology and overview of gliomas. Semin Oncol Nurs. (2018) 34:420–9. doi: 10.1016/j.soncn.2018.10.001
2. Liu S, Zhao Q, Shi W, Zheng Z, Liu Z, Meng L, et al. Advances in radiotherapy and comprehensive treatment of high-grade glioma: immunotherapy and tumor-treating fields. J Cancer. (2021) 12:1094–104. doi: 10.7150/jca.51107
3. Qu J, Mei Q, Liu L, Cheng T, Wang P, Chen L, et al. The progress and challenge of anti-PD-1/PD-L1 immunotherapy in treating non-small cell lung cancer. Ther Adv Med Oncol. (2021) 13:1758835921992968. doi: 10.1177/1758835921992968
4. Dimitriou F, Long GV, Menzies AM. Novel adjuvant options for cutaneous melanoma. Ann Oncol. (2021). doi: 10.1016/j.annonc.2021.03.198
5. Menetrier-Caux C, Ray-Coquard I, Blay JY, Caux C. Lymphopenia in cancer patients and its effects on response to immunotherapy: an opportunity for combination with cytokines? J Immunother Cancer. (2019) 7:85. doi: 10.1186/s40425-019-0549-5
6. Waldman AD, Fritz JM, Lenardo MJ. A guide to cancer immunotherapy: from T cell basic science to clinical practice. Nat Rev Immunol. (2020) 20:651–68. doi: 10.1038/s41577-020-0306-5
7. Nakamura N, Kusunoki Y, Akiyama M. Radiosensitivity of CD4 or CD8 positive human T-lymphocytes by an in vitro colony formation assay. Radiat Res. (1990) 123:224–7. doi: 10.2307/3577549
8. Yovino S, Kleinberg L, Grossman SA, Narayanan M, Ford E. The etiology of treatment-related lymphopenia in patients with malignant gliomas: modeling radiation dose to circulating lymphocytes explains clinical observations and suggests methods of modifying the impact of radiation on immune cells. Cancer Invest. (2013) 31:140–4. doi: 10.3109/07357907.2012.762780
9. Hughes MA, Parisi M, Grossman S, Kleinberg L. Primary brain tumors treated with steroids and radiotherapy: low CD4 counts and risk of infection. Int J Radiat Oncol Biol Phys. (2005) 62:1423–6. doi: 10.1016/j.ijrobp.2004.12.085
10. Upadhyay R, Venkatesulu BP, Giridhar P, Kim BK, Sharma A, Elghazawy H, et al. Risk and impact of radiation related lymphopenia in lung cancer: a systematic review and meta-analysis. Radiother Oncol. (2021) 157:225–33. doi: 10.1016/j.radonc.2021.01.034
11. Venkatesulu BP, Mallick S, Lin SH, Krishnan S. A systematic review of the influence of radiation-induced lymphopenia on survival outcomes in solid tumors. Crit Rev Oncol Hematol. (2018) 123:42–51. doi: 10.1016/j.critrevonc.2018.01.003
12. Grossman SA, Ye XB, Lesser G, Sloan A, Carraway H, Desideri S, et al. Immunosuppression in patients with high-grade gliomas treated with radiation and temozolomide. Clin Cancer Res. (2011) 17:5473–80. doi: 10.1158/1078-0432.CCR-11-0774
13. Mendez JS, Govindan A, Leong J, Gao F, Huang J, Campian JL. Association between treatment-related lymphopenia and overall survival in elderly patients with newly diagnosed glioblastoma. J Neurooncol. (2016) 127:329–35. doi: 10.1007/s11060-015-2037-1
14. Persico P, Lorenzi E, Dipasquale A, Pessina F, Navarria P, Politi LS, et al. Checkpoint inhibitors as high-grade gliomas treatment: state of the art and future perspectives. J Clin Med. (2021) 10:1367. doi: 10.3390/jcm10071367
15. Kim WJ, Dho YS, Ock CY, Kim JW, Choi SH, Lee ST, et al. Clinical observation of lymphopenia in patients with newly diagnosed glioblastoma. J Neurooncol. (2019) 143:321–8. doi: 10.1007/s11060-019-03167-2
16. Ahn S, Park JS, Jang J, Ahn KJ, Hong YK, Yang SH, et al. The association between total lymphocyte count after concomitant chemoradiation and overall survival in patients with newly diagnosed glioblastoma. J Clin Neurosc. (2020) 71:21–5. doi: 10.1016/j.jocn.2019.11.017
17. Stupp R, Dietrich PY, Kraljevic SO, Pica A, Maillard I, Maeder P, et al. Promising survival for patients with newly diagnosed glioblastoma multiforme treated with concomitant radiation plus temozolomide followed by adjuvant temozolomide. J Clin Oncol. (2002) 20:1375–82. doi: 10.1200/JCO.2002.20.5.1375
18. De Sanctis V, Mazzarella G, Osti MF, Valeriani M, Alfo M, Salvati M, et al. Radiotherapy and sequential temozolomide compared with radiotherapy with concomitant and sequential temozolomide in the treatment of newly diagnosed glioblastoma multiforme. Anticancer Drugs. (2006) 17:969–75. doi: 10.1097/01.cad.0000224446.31577.df
19. Brandes AA, Franceschi E, Tosoni A, Benevento F, Scopece L, Mazzocchi V, et al. Temozolomide concomitant and adjuvant to radiotherapy in elderly patients with glioblastoma: correlation with MGMT promoter methylation status. Cancer. (2009) 115:3512–8. doi: 10.1002/cncr.24406
20. Clarke JL, Iwamoto FM, Sul J, Panageas K, Lassman AB, DeAngelis LM, et al. Randomized phase II trial of chemoradiotherapy followed by either dose-dense or metronomic temozolomide for newly diagnosed glioblastoma. J Clin Oncol. (2009) 27:3861–7. doi: 10.1200/JCO.2008.20.7944
21. Ishikawa E, Yamamoto T, Sakamoto N, Nakai K, Akutsu H, Tsuboi K, et al. Low peripheral lymphocyte count before focal radiotherapy plus concomitant temozolomide predicts severe lymphopenia during malignant glioma treatment. Neurol Med Chir. (2010) 50:638–44. doi: 10.2176/nmc.50.638
22. Minniti G, Salvati M, Arcella A, Buttarelli F, D'Elia A, Lanzetta G, et al. Correlation between O6-methylguanine-DNA methyltransferase and survival in elderly patients with glioblastoma treated with radiotherapy plus concomitant and adjuvant temozolomide. J Neurooncol. (2011) 102:311–6. doi: 10.1007/s11060-010-0324-4
23. Cohen KJ, Pollack IF, Zhou T, Buxton A, Holmes EJ, Burger PC, et al. Temozolomide in the treatment of high-grade gliomas in children: a report from the children's oncology group. Neuro Oncol. (2011) 13:317–23. doi: 10.1093/neuonc/noq191
24. Minniti G, Scaringi C, Arcella A, Lanzetta G, Di Stefano D, Scarpino S, et al. IDH1 mutation and MGMT methylation status predict survival in patients with anaplastic astrocytoma treated with temozolomide-based chemoradiotherapy. J Neurooncol. (2014) 118:377–83. doi: 10.1007/s11060-014-1443-0
25. Saito K, Mukasa A, Narita Y, Tabei Y, Shinoura N, Shibui S, et al. Toxicity and outcome of radiotherapy with concomitant and adjuvant temozolomide in elderly patients with glioblastoma: a retrospective study. Neurol Med Chir. (2014) 54:272–9. doi: 10.2176/nmc.oa2012-0441
26. Nayak L, Panageas KS, Reiner AS, Huse JT, Pentsova E, Braunthal SG, et al. Radiotherapy and temozolomide for anaplastic astrocytic gliomas. J Neurooncol. (2015) 123:129–34. doi: 10.1007/s11060-015-1771-8
27. Kim BS, Nam DH, Kim IH, Kim JH, Yoon SM, Kang SG, et al. Concurrent chemoradiotherapy with temozolomide followed by adjuvant temozolomide for newly diagnosed glioblastoma patients: a retrospective multicenter observation study in Korea. Cancer Res Treat. (2017) 49:193–203. doi: 10.4143/crt.2015.473
28. Perry JR, Laperriere N, O'Callaghan CJ, Brandes AA, Menten J, Phillips C, et al. Short-course radiation plus temozolomide in elderly patients with glioblastoma. N Eng J Med. (2017) 376:1027–37. doi: 10.1056/NEJMoa1611977
29. Lin AJ, Campian JL, Hui C, Rudra S, Rao YJ, Thotala D, et al. Impact of concurrent versus adjuvant chemotherapy on the severity and duration of lymphopenia in glioma patients treated with radiation therapy. J Neurooncol. (2018) 136:403–11. doi: 10.1007/s11060-017-2668-5
30. Mohan R, Liu AY, Brown PD, Mahajan A, Dinh J, Chung C, et al. Proton therapy reduces the likelihood of high-grade radiation-induced lymphopenia in glioblastoma patients: phase II randomized study of protons vs photons. Neuro Oncol. (2021) 23:284–94. doi: 10.1093/neuonc/noaa182
31. Rudra S, Hui C, Rao YJ, Samson P, Lin AJ, Chang X, et al. Effect of radiation treatment volume reduction on lymphopenia in patients receiving chemoradiotherapy for glioblastoma. Int J Radiat Oncol Biol Phys. (2018) 101:217–25. doi: 10.1016/j.ijrobp.2018.01.069
32. Lee C, Ahn S, Park JS, Song JH, Hong YK, Jeun SS. Effect of cumulative dexamethasone dose during concomitant chemoradiation on lymphopenia in patients with newly diagnosed glioblastoma. Brain Tumor Res Treat. (2020) 8:71–6. doi: 10.14791/btrt.2020.8.e12
33. Huang J, Dewees TA, Badiyan SN, Speirs CK, Mullen DF, Fergus S, et al. Clinical and dosimetric predictors of acute severe lymphopenia during radiation therapy and concurrent temozolomide for high-grade glioma. Int J Radiat Oncol Biol Phys. (2015) 92:1000–7. doi: 10.1016/j.ijrobp.2015.04.005
34. McCredie JA, Inch WR, Sutherland RM. Effect of radiotherapy on peripheral blood lymphocytes in patients with carcinoma of the breast. Br J Surg. (1971) 58:862.
35. Wild AT, Herman JM, Dholakia AS, Moningi S, Lu Y, Rosati LM, et al. Lymphocyte-sparing effect of stereotactic body radiation therapy in patients with unresectable pancreatic cancer. Int J Radiat Oncol Biol Phys. (2016) 94:571–9. doi: 10.1016/j.ijrobp.2015.11.026
36. Davuluri R, Jiang W, Fang P, Xu C, Komaki R, Gomez DR, et al. Lymphocyte nadir and esophageal cancer survival outcomes after chemoradiation therapy. Int J Radiat Oncol Biol Phys. (2017) 99:128–35. doi: 10.1016/j.ijrobp.2017.05.037
37. Sage EK, Schmid TE, Geinitz H, Gehrmann M, Sedelmayr M, Duma MN, et al. Effects of definitive and salvage radiotherapy on the distribution of lymphocyte subpopulations in prostate cancer patients. Strahlenther Onkol. (2017) 193:648–55. doi: 10.1007/s00066-017-1144-7
38. Campian JL, Piotrowski AF, Ye X, Hakim FT, Rose J, Yan XY, et al. Serial changes in lymphocyte subsets in patients with newly diagnosed high grade astrocytomas treated with standard radiation and temozolomide. J Neurooncol. (2017) 135:343–51. doi: 10.1007/s11060-017-2580-z
39. Gururangan S, Reap E, Schmittling R, Kocak M, Reynolds R, Grant G, et al. Regulatory T cell subsets in patients with medulloblastoma at diagnosis and during standard irradiation and chemotherapy (PBTC N-11). Cancer Immunol Immunother. (2017) 66:1589–95. doi: 10.1007/s00262-017-2051-6
40. Kleinberg L, Sloan L, Grossman S, Lim M. Radiotherapy, lymphopenia, and host immune capacity in glioblastoma: a potentially actionable toxicity associated with reduced efficacy of radiotherapy. Neurosurgery. (2019) 85:441–53. doi: 10.1093/neuros/nyz198
41. Su YB, Sohn S, Krown SE, Livingston PO, Wolchok JD, Quinn C, et al. Selective CD4+ lymphopenia in melanoma patients treated with temozolomide: a toxicity with therapeutic implications. J Clin Oncol. (2004) 22:610–6. doi: 10.1200/JCO.2004.07.060
Keywords: glioma, lymphopenia, chemoradiation, meta-analysis, temozolomide
Citation: Zhang Y, Chen S, Chen H, Chen S, Li Z, Feng E and Li W (2022) Prognostic Value and Risk Factors of Treatment-Related Lymphopenia in Malignant Glioma Patients Treated With Chemoradiotherapy: A Systematic Review and Meta-Analysis. Front. Neurol. 12:726561. doi: 10.3389/fneur.2021.726561
Received: 17 June 2021; Accepted: 09 December 2021;
Published: 04 January 2022.
Edited by:
Khan Iftekharuddin, Old Dominion University, United StatesReviewed by:
Rakesh Jalali, Apollo Proton Cancer Centre, IndiaYasmin Lassen, Aarhus University Hospital, Denmark
Copyright © 2022 Zhang, Chen, Chen, Chen, Li, Feng and Li. This is an open-access article distributed under the terms of the Creative Commons Attribution License (CC BY). The use, distribution or reproduction in other forums is permitted, provided the original author(s) and the copyright owner(s) are credited and that the original publication in this journal is cited, in accordance with accepted academic practice. No use, distribution or reproduction is permitted which does not comply with these terms.
*Correspondence: Wei Li, d2VpbGk4OTg5QGNjbXUuZWR1LmNu; Enshan Feng, ZW5zaGFuZmVuZ0AxMjYuY29t
†These authors have contributed equally to this work