- 1Department of Neurology, West China Hospital of Sichuan University, Chengdu, Sichuan, China
- 2Department of Radiology, West China Hospital, Sichuan University, Chengdu, China
- 3Cardiac Ultrasound Office, Department of Cardiology, West China Hospital, Sichuan University, Chengdu, China
Background and objectives: Atrial fibrillation (AF) has been linked to dementia risk, partly explained by cerebral small vessel disease (CSVD). Since AF and cardiovascular comorbidities were associated with cardiac dysfunction, we aimed to determine the association between echocardiographic parameters and neuroimaging markers of CSVD in patients with AF-related ischemic stroke.
Methods: This cross-sectional study enrolled patients with AF-related ischemic stroke from March 2013 to December 2019 who underwent transthoracic echocardiography and brain 3T MRI, including T1, T2, Flair, and SWI imaging sequences. We assessed the presence of lacunes and cerebellar microbleeds (CMBs), the severity of white matter hyperintensity (WMH) scored by the Fazekas scale (0-6), and the severity of enlarged perivascular spaces (EPVS) in basal ganglia (BG) and centrum semiovale (CSO) classified into three categories (0–10, 10–25, and >25). CSVD burden was rated on a 0-to-4 ordinal scale. Generalized linear regression analysis and post hoc comparisons with Bonferroni correction were performed to assess the association between various echocardiographic parameters and these lesions, adjusted for demographics and potential confounders.
Results: 119 patients (68.38 ± 12.692 years; male 45.4 %) were included for analysis, of whom 55 (46.2%) had lacunes, 40 (33.6%) had CMBs, and median severity for WMH, BG-EPVS, CSO-EPVS, and CSVD burden were 2 (IQR: 1–3), 1 (IQR: 1–2), 1 (IQR: 0–1), and 1 (IQR: 1–2) respectively. In multivariable, fully adjusted models, left ventricular posterior wall thickness (LVPW) was associated with a higher risk of lacunes (RR 1.899, 95% CI: 1.342–2.686) and CSVD burden (RR = 2.081, 95%CI: 1.562–2.070). Right atrial diameter (RAD) was associated with greater CSO-EPVS (RR = 2.243, 95%CI: 1.234–4.075). No echocardiographic parameters were revealed to be associated with CMBs and WMH.
Conclusion: In patients with AF-related ischemic stroke, LVPW is associated with a higher risk of lacunes and CSVD burden, while RAD was associated with greater CSO-EPVS. Larger studies are required to determine these associations and to elucidate if these associations can help facilitate cognitive evaluation and brain MRI screening.
Introduction
Increasing evidence suggests that atrial fibrillation (AF) appears to be correlated with cognitive decline independent of clinical stroke (1, 2). Cerebral small vessel disease (CSVD) is one of the pathological mechanisms through which AF might lead to cognitive impairment. A more recent large sample of study (3) has demonstrated that nearly 25% of patients with AF-related ischemic stroke or transient ischemic attack have preexisting cognitive impairment. They found imaging markers of CSVD were independently associated with cognitive impairment prior to ischemic events.
There is no complete explanation of the mechanism that links AF and CSVD, but chronic cerebral hypoperfusion, inflammation, and shared vascular risk factors, such as hypertension and diabetes mellitus, may all be involved. Patients with AF are more likely to develop cardiac dysfunction, for example, many patients with AF develop an enlarged left atrium (LA) and enlarged left ventricular (LV), and are also associated with an increased incidence of heart failure. Conversely, patients with cardiac dysfunction are known to contribute to AF development and maintenance (4–6). According to previous studies, some cardiac subclinical indicators, such as left ventricular structure and LV systolic dysfunction, may contribute to greater white matter hyperintensity (WMH) (6), and LA volume, may contribute to silent brain infarcts (7) even in the absence of AF. However, none of them investigated whether cardiac structural or functional abnormalities correlated with neuroimaging markers of CSVD in patients with AF-related ischemic stroke. Thus, we evaluated the cross-sectional association of the echocardiographic parameters of cardiac structure or function with the neuroimaging markers of CSVD on MRI in patients with AF-related ischemic stroke.
Materials and methods
Patients and evaluation
The data that support the findings of this cross-sectional study is available from the Chengdu stroke registry, an ongoing prospective hospital-based database (8). We defined AF-related ischemic stroke when the patients had a cardioembolic stroke with AF. Acute ischaemic stroke (AIS) patients who underwent transthoracic echocardiography and the brain 3T MRI, including T1, T2, Flair, and susceptibility-weighted imaging (SWI) sequences imaging between March 2013 and December 2019 were eligible if they were 18 years or older. Patients with non-cardioembolic stroke, no evidence of AF, bilateral stroke infarcts; time from admission to stroke onset over 14 days, and unavailable MRI imaging were excluded. The diagnosis of AIS was based on the World Health Organization (9). AF was recorded as present if there was any history of it in the past, or if it was present on the electrocardiogram at the time of admission. The trial of ORG 10,172 in acute stroke treatment (TOAST) classification was used to determine AIS subtypes on the basis of their etiology (10). The echocardiogram and brain MRI were completed within 4–14 days after admission.
The study was approved by the Ethics Committee of West China Hospital, Sichuan University Scientific Research (2014[69]), and followed the Declaration of Helsinki. All patients provided written consent.
Echocardiographic data
All patients were evaluated by 2-D transthoracic echocardiography during hospitalization, using a Philips iE33 (Philips Medical Systems, USA) echocardiography device. A single trained investigator with considerable echocardiographic experience performed all the echocardiographic evaluations blinded to other information. All measurements were performed according to the American Society of Echocardiography guidelines.
We collected echocardiographic parameters including left atrial anteroposterior diameter (LAD), left ventricular end-diastolic diameter (LVD), right anteroposterior atrial diameter (RAD), right ventricular end-diastolic diameter (RVD), interventricular septum diameter (IVS), left ventricular posterior wall diameter (LVPW), left ventricular end-diastolic diameter (LVEDD), left ventricular end-systolic diameter (LVESD), left ventricular end-diastolic volume (LVEDV), left ventricular end-systolic volume (LVESV), left ventricular cardiac and stroke volume (SV) and the left ventricular ejection fraction (LVEF) was calculated using two-plane Simpson method.
Brain magnetic resonance imaging
MRI was performed on a 3.0-T GE scanner Discovery or Architect scanner at West China Hospital of Sichuan University. The MRI protocol included: (1) axial T1 weighted: TE = 24 ms, TR = 1,750 ms, echo train length (ETL) = 10, bandwidth (BW) = 41.67KHz, matrix = 320 × 224, slice thickness = 5 mm, filed-of-view = 240 mm, spacing = 1, number of excitations (NEX) = 1; (2) axial T2 weighted: TE = 93 ms, TR = 5,727 ms, ETL = 32, BW = 83.3 KHz, matrix = 512 × 512, filed-of-view = 240 mm, slice thickness = 5 mm, spacing = 1, NEX = 1.5; (3) FLAIR weighted: TE = 145 ms, TR = 8,400 ms, inversion time (TI) = 2,100 ms, BW = 83.3 KHz, flip angle (FA) = 145°, matrix = 320 × 224, slice thickness = 5 mm, filed-of-view = 240 mm, spacing = 1, NEX = 1; (4) Between March 2013 and May 2018, SWI weighted: TE = 20 ms, TR = 27 ms, Flip angle = 15°, slices = 64, slice thickness = 2 mm, and field of view = 185 × 220 mm); between April 2018 and December 2019, the parameters were: SWI (TE: 22 ms, TR: 38 ms, Flip angle: 15°, slices: 64, slice thickness/gap: 2/2 mm, and field of view: 240 × 216 mm).
MRI measurements were made in accordance with the consensus criteria of the Standards for Reporting Vascular changes on neuroimaging (STRIVE) (11). Lacunes were defined as deep lesions (3–15 mm) exhibiting CSF-like signals on all sequences. CMBs appeared as small, rounded, or circular hypointense lesions within the brain parenchyma with clear margins, and had a size of 2–10 mm on the SWI image. In this study, we evaluated the presence of lacunes and cerebellar microbleeds (CMBs). We evaluated lacunes and cerebellar microbleeds (CMBs) according to the presence or absence of these structures. White matter hyperplasia (WMH) was defined by FLAIR sequencing as patches of white matter in periventricular areas or the centrum semiovale area. Periventricular and deep WMHs were graded on the Fazekas scale from 0 to 3; the sum of these two parts (0 to 6) was used to calculate the total Fazekas score. Enhanced perivascular spaces (EPVSs) are defined by small punctate (if perpendicular) or linear hyperintensities (if longitudinal) on T2 images of the basal ganglia (BG) or centrum semiovale (CSO), and classified into three categories (0–10, 10–25, and >25). An ordinal score ranging from 0 to 4 was constructed to reflect the total burden of CSVD. Two trained neurologists blind to all clinical information about the study patients reviewed and analyzed the MR images.
Visualization and evaluation of MRI images were performed by a single rater (K.L.Y) blinded to clinical data. For the purpose of assessing inter-rater agreement, a second rater (W.D.T.) evaluated 25 randomly selected patients for the presence of CMBs (kappa 0.85, p =0.002), lacunes (kappa 0.66, p = 0.031), the severity of WMH (kappa 0.75, p = 0.002), BG-EPVS (kappa 0.62, p < 0.001), and CSO-EPVS (kappa 0.62, p < 0.001).
Clinical measures
Information about demographics and clinical characteristics including age, gender, previous medical history (hypertension, diabetes mellitus, and hyperlipidemia), heart disease (coronary heart disease, cardiac valve disease, congestive heart failure), prior antithrombotic agents (antiplatelets, lipid-lowering, and anticoagulants) use, smoking and alcohol status. CHA2DS2-VASc score was calculated as previously described (12). Hypertension was defined by a physician's self-reported diagnosis, the use of hypertensive medications, or a measured systolic blood pressure >_140 mmHg systolic or diastolic blood pressure >_90 mmHg. Diabetes mellitus was defined by a self-reported physician's self-report, the use of hypoglycemic medications, and non-fasting serum glucose levels above 200 mg/dL, or fasting serum glucose levels above 60 mg/dL. Hyperlipidemia was defined as LDL-c >_160 mmol/dl, a history of hyperlipidemia or lipid-lowering medication. Heart disease was based on hospital discharge diagnosis codes. Coronary heart disease was defined as having a history of coronary heart disease or having been clinically diagnosed with coronary heart disease during hospitalization. Cardiac valve disease was defined as either a self-reported history of valvular disease recorded on the CRF or cardiac valve replacement, stenosis, regurgitation affecting one or more heart valves evaluated by echocardiography during hospitalization. Congestive heart failure was defined as having a history of chronic congestive heart failure.
Statistical analysis
Data are presented as means ± SD or medians with interquartile ranges, and as proportions for categorical variables as appropriate. Inter-rater reliability of the neuroimaging variable was tested using the kappa statistic.
Univariate analysis for the association of clinical characteristics and echocardiographic parameters with MRI markers of CSVD was assessed by using generalized linear models (GLM). The Spearman χ2 test was used for categorical variables comparison. Multivariate analysis was performed to determine the contribution of echocardiographic parameters to neuroimaging burden of CSVD using generalized linear models (GLM) adjusted for age, sex, and factors with statistical significance by univariate analysis. Due to colinearity issues, both the CHA2DS2-VASc score and its sub-variables were entered into separate multivariate models. We used a regression model with a logit link function for the association of echocardiographic parameters with the presence of lacunes and CMBs. Analysis for the association of echocardiographic parameters with severity for WMH, BG-EPVS, CSO-EPVS, and CSVD burden was performed using a regression model with a probit link function. Risk ratios (RR) per standard deviation change and 95% confidence intervals (CI) were presented.
All analyses were performed using SPSS 25.0 (IBM, Armonk, New York). A P-value < 0.05 was considered statistically significant. Again, a Bonferroni correction (Bonferroni correction = 0.05/number of subsamples = 0.004) was applied to 12 echocardiographic variables for multiple comparisons.
Results
Clinical characteristics and echocardiographic parameters
A total of 378 AIS patients who underwent transthoracic echocardiography and 3T MRI were registered in the present analysis. The following patients were excluded: 133 because they had a non-cardioembolic stroke, 25 because bilateral stroke infarcts affected the identification of CSVD on neuroimaging, 30 because of no evidence of AF, and 51 because of time from admission to stroke onset over 14 days. Furthermore, 20 patients had unavailable MRI imaging, leading to the final study sample of 119 as shown in Figure 1.
The mean age of eligible patients was 68.38 ± 12.692 years, and 45.4% were male. Median CHA2DS2-VASc score was 2 (IQR: 1–3). The median time interval between echocardiogram and diagnosis of AF was 1 month (IQR: 0–36 months). The baseline characteristics and echocardiographic parameters are shown in Table 1. Among them, 55 (46.2%) had lacunes, 40 (33.6%) had CMBs, and median severity for WMH, BG-EPVS, CSO-EPVS, and CSVD burden were 2 (IQR: 1–3), 1 (IQR: 1–2), 1 (IQR: 0–1), and 1 (IQR: 1–2) respectively.
Univariate analysis for clinical characteristics associated with MRI markers of CSVD
The univariate analysis of the clinical characteristics presented in Table 2 demonstrated that hypertension, diabetes, and CHA2DS2-VASc score were significantly associated with the risk of lacunes (all P < 0.05). Age, hypertension, cardiac valve disease, and CHA2DS2-VASc score were significantly associated with the risk of greater WMH (all P < 0.05). Age and CHA2DS2-VASc score were significantly associated with the risk of CMBs (P < 0.05). Besides, age, hypertension, diabetes, coronary artery disease, cardiac valve disease, CHA2DS2-VASc score, and prior anticoagulant therapy were significantly associated with BG-EPVS severity (all P < 0.05), while age, hypertension, cardiac valve disease, CHA2DS2-VASc score, and current drinkers were significantly associated with CSO-EPVS severity (all P < 0.05). Cardiac valve disease, congestive heart failure, and CHA2DS2-VASc score were significantly associated with CSVD burden (all P < 0.05).
Univariate analysis and correlation matrix of MRI markers of CSVD and echocardiographic parameters
Univariate analysis for the association of echocardiographic parameters with MRI markers of CSVD as shown in Table 3 demonstrated that LAD (RR, 0.958; 95% CI, 0.918–1.000; P = 0.049), IVS (RR, 1.230; 95% CI, 1.022–1.481; P = 0.029), and LVPW (RR, 1.738; 95% CI, 1.271–2.375; P = 0.001) were significantly associated with the risk of lacunes. With Bonferroni correction, the associations between LAD and IVS and lacunes were attenuated. LAD (RR, 0.958; 95% CI, 0.924–0.994; P = 0.021), IVS (RR, 1.195; 95% CI, 1.043–1.346; P = 0.012), and LVPW (RR, 1.392; 95% CI, 1.094–1.765; P = 0.006) were significantly associated with greater WMH, whereas the statistically significant difference in these associations was no longer significant after Bonferroni correction. LVPW (RR, 1.376; 95% CI, 1.095–1.658; P = 0.009) was significantly associated with BG-EPVS severity, and RAD (RR, 1.048; 95% CI, 1.011–1.087; P = 0.011) was significantly associated with CSO-EPVS severity, while a Bonferroni correction attenuated these associations. In addition, LAD (RR, 0.946; 95% CI, 0.907–0.985; P = 0.008) and LVPW (RR, 1.923; 95% CI, 1.473–2.512; P < 0.001) were significantly associated with CSVD burden. Bonferroni correction reduced the association between LAD and CSVD burden.
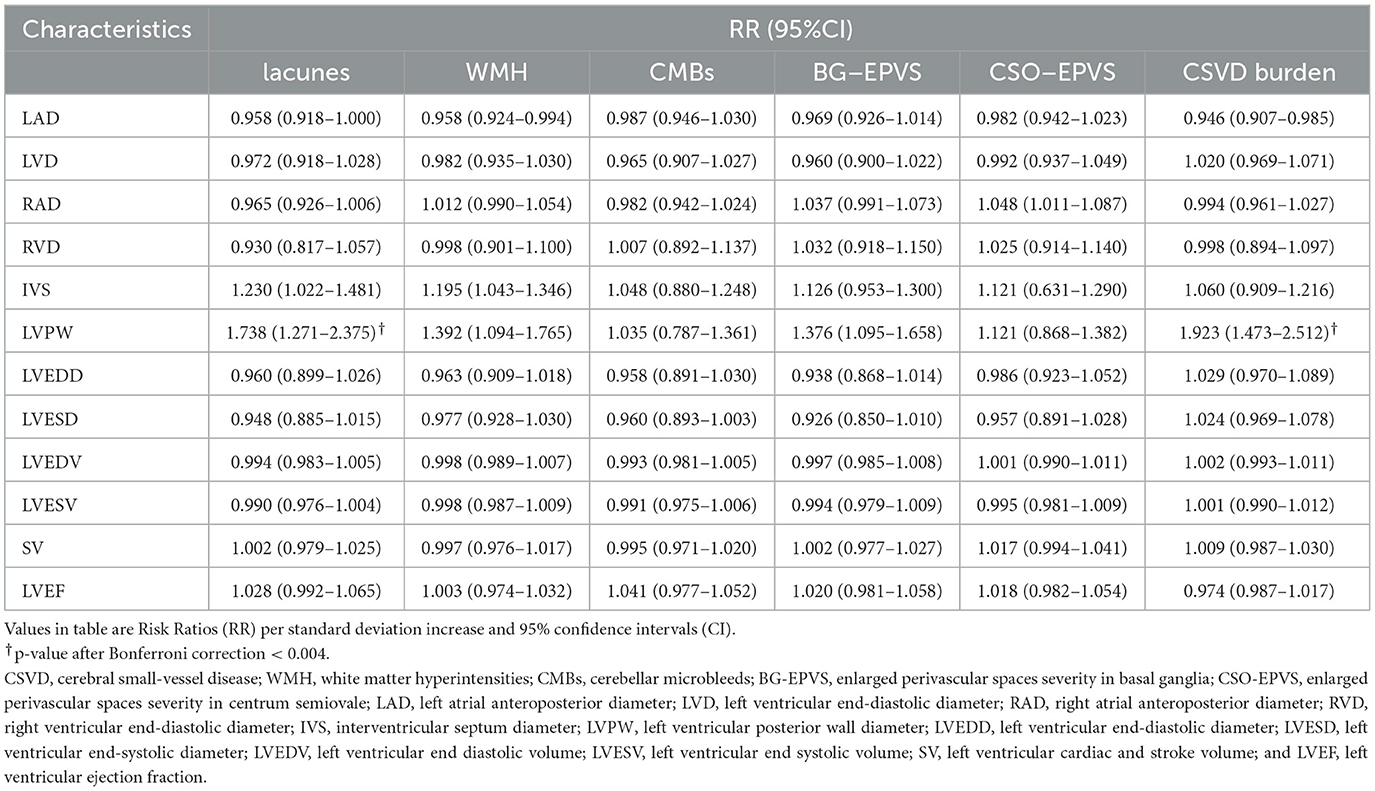
Table 3. Univariate analysis for association of echocardiographic parameters with MRI markers of CSVD.
Spearman correlation matrix as shown in Figure 2 revealed that LAD was negatively correlated with lacunes (rs = −0.184, P = 0.020) and WMH (rs = −0.204, P = 0.047), while both IVS and LVPW were positively correlated with lacunes (rs = 0.208, P = 0.007; rs = 0.334, P < 0.001 respectively) and WMH (rs = 0.213, P = 0.005; rs = 0.267, P = 0.004 respectively). LVPW was positively correlated with BG-EPVS severity (rs = 0.255, P = 0.007), while RAD was positively correlated with CSO-EPVS severity (rs = 0.258, P = 0.002). In addition, LAD and LVPW were positively correlated with CSVD burden (rs = −0.267, P = 0.003; rs = 0.434, P < 0.001 respectively).
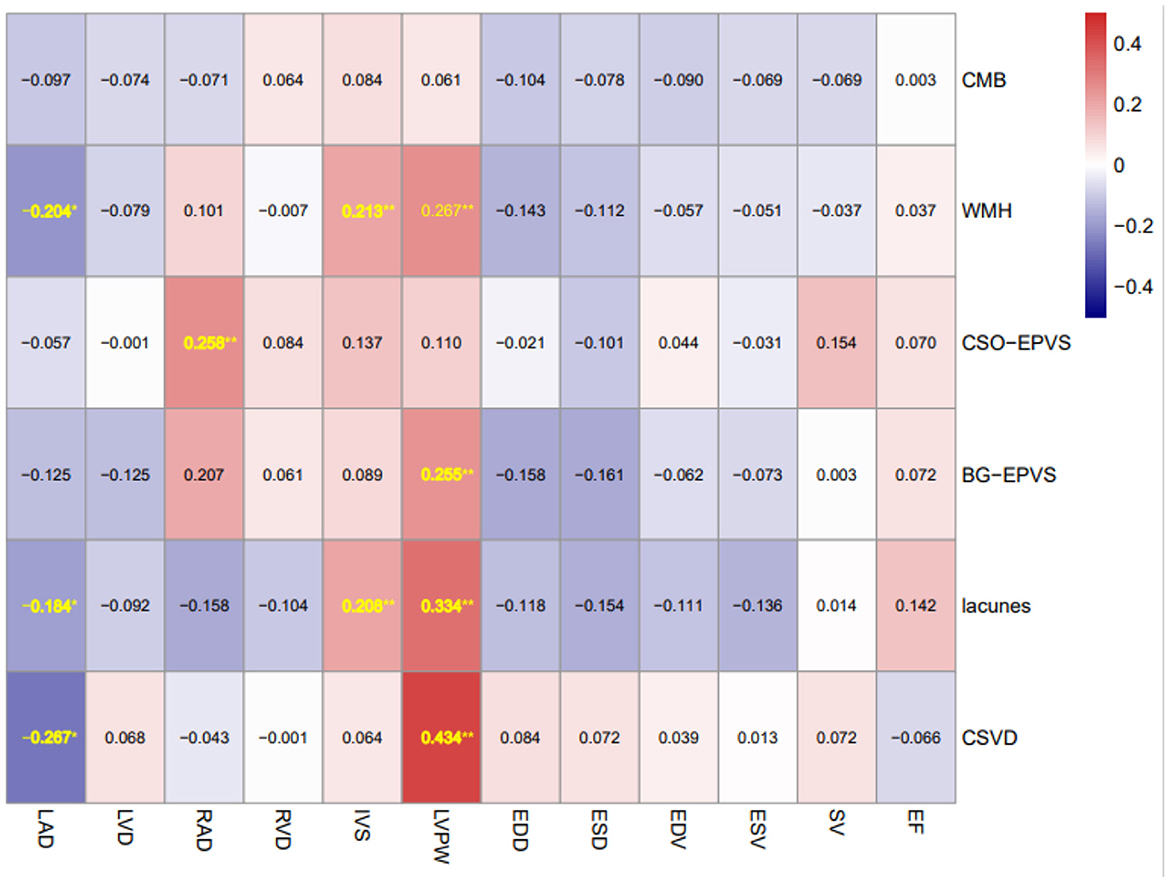
Figure 2. Spearman correlation matrix of MRI markers of CSVD and echocardiographic parameters. Spearman correlation matrix of MRI markers of CSVD and echocardiographic parameters (number shown in the matrix is r coefficient with statistical significance) *P < 0.05 and **P < 0.01. CSVD, cerebral small-vessel disease; WMH, white matter hyperintensities; CMBs, cerebellar microbleeds; BG-EPVS, enlarged perivascular spaces severity in basal ganglia; CSO-EPVS, enlarged perivascular spaces severity in centrum semiovale; LAD, left atrial anteroposterior diameter; LVD, left ventricular end-diastolic diameter; RAD, right atrial anteroposterior diameter; RVD, right ventricular end-diastolic diameter; IVS, interventricular septum diameter; LVPW, left ventricular posterior wall diameter; LVEDD, left ventricular end-diastolic diameter; LVESD, left ventricular end-systolic diameter; LVEDV, left ventricular end diastolic volume; LVESV, left ventricular end systolic volume; SV, left ventricular cardiac and stroke volume; and LVEF, left ventricular ejection fraction.
Multivariate analysis for association of echocardiographic parameters with MRI markers of CSVD
Multivariate analysis included age, gender, and the variables associated with MRI markers of CSVD identified by univariate analysis and found that LVPW remained significantly associated with a higher risk of lacunes (RR = 1.899, 95%CI: 1.342–2.686, P < 0.001), greater WMH (RR, 1.297; 95% CI, 1.016–1.504; P = 0.032), and CSVD burden (RR = 2.081, 95%CI: 1.562–2.070, P < 0.001). RAD is significantly associated with CSO-EPVS severity (RR = 2.243, 95%CI: 1.234–4.075, p = 0.004). After Bonferroni correction, there was no significant difference between LVPW and greater WMH. No significant associations were observed with any echocardiographic parameters and CMBs (Table 4). These associations remained consistent after accounting for CHA2DS2-VASc Score in multivariate analysis (Supplementary Table).
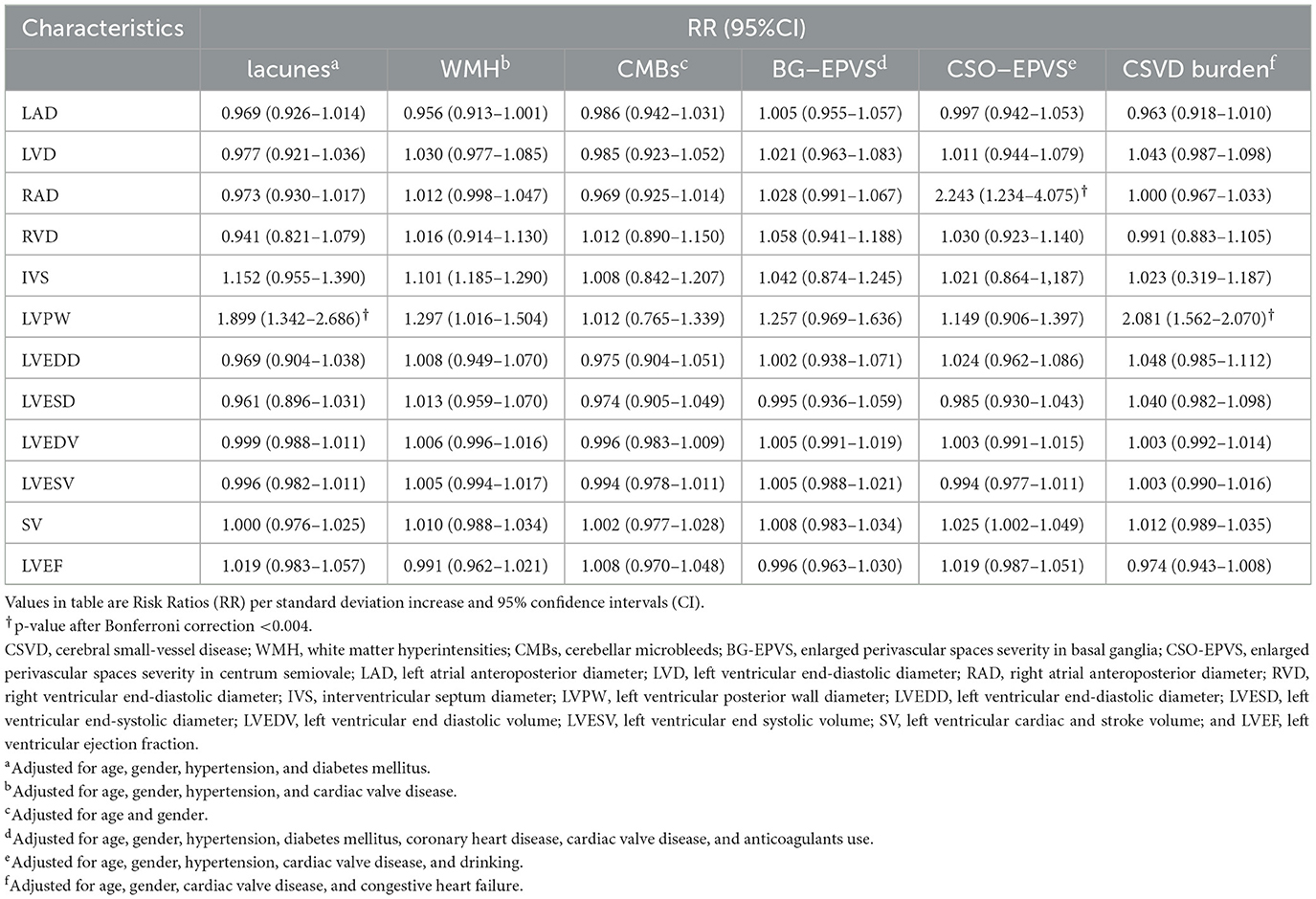
Table 4. Multivariate analysis for association of echocardiographic parameters with MRI markers of CSVD.
Discussion
To the best of my knowledge, this is the first study carried out on the association between various echocardiographic parameters and neuroimaging burden of CSVD in patients with AF-related ischemic stroke. The main findings of our study are summarized below: (1) LVPW was associated with a higher risk of lacunes and CSVD burden. (2) RAD was associated with greater CSO-EPVS. These associations remained significant after adjusting for age, sex, and potential confounders. We emphasized the importance of echocardiographic assessment in clinical practice to facilitate cognitive evaluation and brain MRI screening of AF-related patients.
Mechanisms linking the association between LVPW and prevalent lacunes and CSVD burden in patients with AF-related ischemic stroke may involve various aspects, as follows. First, this association may be partially mediated by abnormal perfusion of the brain microvasculature. Compared to sinus rhythm, AF could trigger a higher variability, which would lead to critical cerebral hemodynamic events (hypoperfusion or hypertensive events) at the capillary level during AF (13). Cardiac changes of LVPW can contribute to AF development and maintenance, which could in turn disrupt the perfusion of the brain microvasculature. Another potential explanation linking an increase in LVPW and cerebrovascular disease could be at least explained by shared risk factors, which is in line with Michelle et al. (14). Previous study indicated that greater LVPW is common in longstanding hypertension (15), and thus could be considered a potent marker of cardiovascular risk. The greater LVPW may be not directly associated with lacunes and CSVD burden but rather indirectly as an index of exposure to cardiovascular risk factors. In addition, these brain injuries may also be caused by microemboli resulting from this cardiac abnormality. Further study with sufficient data to speculate about the likelihood of these alternative mechanisms is needed.
Another interesting finding of the present study was that RAD was associated with greater CSO-EPVS. Fewer studies involved the association between right atrium diameter and neuroimaging markers of CSVD previously. In fact, AF can also result in enlargement and fibrosis of the right atrium, which may interfere with the function of the right atrium (16, 17). Right atrium enlargement is usually accompanied by elevated right atrial pressure, according to research (18). Under pathological conditions, the enlarged right atrium may contribute to an elevation in the volume and pressure of systemic circulation veins, and even result in cerebrovenous congestion and obstruction of glymphatic drainage from the perivascular spaces (19). Perivascular spaces are potential spaces filled with interstitial fluid that flow along cerebral arteries and veins, which have been shown to play an important role in the clearance of interstitial fluid and waste products from the brain into the meningeal and cervical lymphatics (20). Insufficient glymphatic clearance caused by an enlarged right atrium may ultimately lead to toxic metabolic accumulation and neurovascular inflammation (21). According to one study (22) of chronic valvular heart disease patients, right atrial pressure is independently associated with higher WMH volume. RAD has also been reported (23) to be negatively correlated with mean deep regional CBF. Collectively, there is therefore a need to continuously pay attention to pathogenesis involving RAD in CSVD in future research.
Our study should be considered in light of several limitations. Firstly, due to the current study's cross-sectional design, we cannot examine causal relationships between echocardiographic characteristics and neuroimaging markers of CSVD in AF-related patients, but only document associations. Secondly, echocardiography is known to have limited precision, while the cost and ease of echocardiography make it an ideal examination for admission to a hospital by individuals. Thirdly, patients with permanent or persistent AF may have very different echocardiographic parameters to those with paroxysmal and that has been shown in several prior papers, while the retrospective design represents a limitation of the current study to obtain information on the type of AF. Lastly, it was done in a single center with a relatively small number of patients which may cause selection bias.
Conclusion
In patients with AF-related ischemic stroke, LVPW is associated with a higher risk of lacunes and CSVD burden, while RAD was associated with greater CSO-EPVS. Further studies with a larger sample size and a longitudinal design were needed to continue to determine these associations and to elucidate if these associations can help facilitate cognitive evaluation and brain MRI screening.
Data availability statement
The original contributions presented in the study are included in the article/Supplementary material, further inquiries can be directed to the corresponding author.
Ethics statement
The study was approved by the Ethics Committee of West China Hospital, Sichuan University Scientific Research. The patients/participants provided their written informed consent to participate in this study.
Author contributions
KY: study concept, data analysis, statistics, and paper writing. WT: study concept, patient recruitment, data analysis, statistics, and study protocol development. ZW and DL: imaging data acquisition and interpretation of data. MX: study protocol design and data analysis. JL: data analysis and informed consent acquisition. ML: study concept and guidance. All authors contributed to the article and approved the submitted version.
Funding
The study was supported by the National Natural Science Foundation of China (Grant Nos. 81974181 and 81601022) and the 1.3.5 project for disciplines of excellence, West China Hospital, Sichuan University (ZYGD18009).
Conflict of interest
The authors declare that the research was conducted in the absence of any commercial or financial relationships that could be construed as a potential conflict of interest.
Publisher's note
All claims expressed in this article are solely those of the authors and do not necessarily represent those of their affiliated organizations, or those of the publisher, the editors and the reviewers. Any product that may be evaluated in this article, or claim that may be made by its manufacturer, is not guaranteed or endorsed by the publisher.
Supplementary material
The Supplementary Material for this article can be found online at: https://www.frontiersin.org/articles/10.3389/fneur.2023.1137488/full#supplementary-material
Abbreviations
AF, Atrial fibrillation; CSVD, cerebral small vessel disease; WMH, white matter hyperintensity; CMBs, cerebellar microbleeds; LA, left atrium; LV, left ventricular; EPVS, enlarged perivascular spaces; BG-EPVS, enlarged perivascular spaces severity in basal ganglia; CSO-EPVS, enlarged perivascular spaces severity in centrum semiovale; SWI, susceptibility weighted imaging; TOAST-criteria, Trial of Org 10,172 in Acute Stroke Treatment; LAD, left atrial anteroposterior diameter; LVD, left ventricular end-diastolic diameter; RAD, right atrial anteroposterior diameter; RVD, right ventricular end-diastolic diameter; IVS, interventricular septum diameter; LVPW, left ventricular posterior wall diameter; LVEDD, left ventricular end-diastolic diameter; LVESD, left ventricular end-systolic diameter; LVEDV, left ventricular end diastolic volume; LVESV, left ventricular end systolic volume; SV, left ventricular cardiac and stroke volume; LVEF, the left ventricular ejection fraction; RR, Risk Ratios; CI,: confidence intervals; ETL, echo train length; BW, bandwidth; NEX, number of excitations; GLM, generalized linear models.
References
1. Rivard L, Friberg L, Conen D, Healey JS, Berge T, Boriani G, et al. Atrial fibrillation and dementia: a report from the AF-SCREEN international collaboration. Circulation. (2022) 145:392–409. doi: 10.1161/CIR.0000000000001067
2. Diener H-C, Hart RG, Koudstaal PJ, Lane DA, Lip GYH. Atrial fibrillation and cognitive function: JACC review topic of the week. J Am Coll Cardiol. (2019) 73:612–9. doi: 10.1016/j.jacc.2018.10.077
3. Banerjee G, Chan E, Ambler G, Wilson D, Cipolotti L, Shakeshaft C, et al. Cognitive impairment before atrial fibrillation–related ischemic events: neuroimaging and prognostic associations. J Am Heart Assoc. (2020) 9:e014537. doi: 10.1161/JAHA.119.014537
4. Carlisle MA, Fudim M, DeVore AD, Piccini JP. Heart failure and atrial fibrillation, like fire and fury. JACC: Heart Failure. (2019) 7:447–56. doi: 10.1016/j.jchf.2019.03.005
5. Weil BR, Ozcan C. Cardiomyocyte remodeling in atrial fibrillation and hibernating myocardium: shared pathophysiologic traits identify novel treatment strategies? BioMed Res Int. (2015) 2015:587361. doi: 10.1155/2015/587361
6. Andrade J, Khairy P, Dobrev D, Nattel S. The clinical profile and pathophysiology of atrial fibrillation: relationships among clinical features, epidemiology, and mechanisms. Circ Res. (2014) 114:1453–68. doi: 10.1161/CIRCRESAHA.114.303211
7. Russo C, Jin Z, Liu R, Iwata S, Tugcu A, Yoshita M, et al. LA volumes and reservoir function are associated with subclinical cerebrovascular disease: the CABL (Cardiovascular Abnormalities and Brain Lesions) study. JACC Cardiovas Imag. (2013) 6:313–23. doi: 10.1016/j.jcmg.2012.10.019
8. Tao W, Wang Z, Liu J, Li J, Deng Y, Guo W, et al. Acute cerebral microinfarcts in acute ischemic stroke: imaging and clinical significance. Cerebrovasc Dis. (2022) 51:755–63. doi: 10.1159/000524021
9. Hatano S. Experience from a multicentre stroke register: a preliminary report. Bull World Health Organ. (1976) 54:541.
10. Adams HP Jr, Bendixen BH, Kappelle LJ, Biller J, Love BB, et al. Classification of subtype of acute ischemic stroke. Definitions for use in a multicenter clinical trial. TOAST. Trial of Org 10172 in acute stroke treatment. Stroke. (1993) 24:35–41. doi: 10.1161/01.STR.24.1.35
11. Wardlaw JM, Smith EE, Biessels GJ, Cordonnier C, Fazekas F, Frayne R, et al. Neuroimaging standards for research into small vessel disease and its contribution to ageing and neurodegeneration. Lancet Neurol. (2013) 12:822–38. doi: 10.1016/S1474-4422(13)70060-7
12. Lip GY, Nieuwlaat R, Pisters R, Lane DA, Crijns HJ. Refining clinical risk stratification for predicting stroke and thromboembolism in atrial fibrillation using a novel risk factor-based approach: the euro heart survey on atrial fibrillation. Chest. (2010) 137:263–72. doi: 10.1378/chest.09-1584
13. Anselmino M, Scarsoglio S, Saglietto A, Gaita F, Ridolfi L. Transient cerebral hypoperfusion and hypertensive events during atrial fibrillation: a plausible mechanism for cognitive impairment. Sci Rep. (2016) 6:1–8. doi: 10.1038/srep28635
14. Johansen MC, Shah AM, Lirette ST, Griswold M, Mosley TH, Solomon SD, et al. Associations of echocardiography markers and vascular brain lesions: the ARIC Study. J Am Heart Assoc. (2018) 7:e008992. doi: 10.1161/JAHA.118.008992
15. Cheng S, Fernandes VR, Bluemke DA, McClelland RL, Kronmal RA, Lima JA. Age-related left ventricular remodeling and associated risk for cardiovascular outcomes: the multi-ethnic study of atherosclerosis. Circ Cardiovasc Imag. (2009) 2:191–8. doi: 10.1161/CIRCIMAGING.108.819938
16. Aksu U, Kalkan K, Gulcu O, Aksakal E, Öztürk M, Topcu S. The role of the right atrium in development of postoperative atrial fibrillation: a speckle tracking echocardiography study. J Clin Ultrasound. (2019) 47:470–6. doi: 10.1002/jcu.22736
17. Guta AC, Badano LP, Tomaselli M, Mihalcea D, Bartos D, Parati G, et al. The pathophysiological link between right atrial remodeling and functional tricuspid regurgitation in patients with atrial fibrillation: a three-dimensional echocardiography study. J Am Soc Echocardiograp. (2021) 34:585–94.e1. doi: 10.1016/j.echo.2021.01.004
18. Austin C, Alassas K, Burger C, Safford R, Pagan R, Duello K, et al. Echocardiographic assessment of estimated right atrial pressure and size predicts mortality in pulmonary arterial hypertension. Chest. (2015) 147:198–208. doi: 10.1378/chest.13-3035
19. Lahiri S, Schlick KH, Padrick MM, Rinsky B, Gonzalez N, Jones H, et al. Cerebral Pulsatility index is elevated in patients with elevated right atrial pressure. J Neuroimaging. (2018) 28:95–8. doi: 10.1111/jon.12456
20. Wardlaw JM, Benveniste H, Nedergaard M, Zlokovic BV, Mestre H, Lee H, et al. Perivascular spaces in the brain: anatomy, physiology and pathology. Nat Rev Neurol. (2020) 16:137–53. doi: 10.1038/s41582-020-0312-z
21. Rasmussen MK, Mestre H, Nedergaard M. The glymphatic pathway in neurological disorders. Lancet Neurol. (2018) 17:1016–24. doi: 10.1016/S1474-4422(18)30318-1
22. Lee W-J, Jung K-H, Ryu YJ, Kim J-M, Lee S-T, Chu K, et al. Association of cardiac hemodynamic factors with Severity of white matter Hyperintensities in chronic Valvular heart disease. JAMA Neurol. (2018) 75:80–7. doi: 10.1001/jamaneurol.2017.2853
Keywords: atrial fibrillation, echocardiographic characteristics, cerebral small-vessel disease, ischemic stroke, cardiac dysfunction
Citation: Ye K, Tao W, Wang Z, Li D, Xu M, Liu J and Liu M (2023) Echocardiographic correlates of MRI imaging markers of cerebral small-vessel disease in patients with atrial-fibrillation-related ischemic stroke. Front. Neurol. 14:1137488. doi: 10.3389/fneur.2023.1137488
Received: 04 January 2023; Accepted: 20 February 2023;
Published: 23 March 2023.
Edited by:
Hsin-Hsi Cynthia Tsai, National Taiwan University, TaiwanReviewed by:
Marialuisa Zedde, IRCCS Local Health Authority of Reggio Emilia, ItalyEdward El-Am, Mayo Clinic, United States
Copyright © 2023 Ye, Tao, Wang, Li, Xu, Liu and Liu. This is an open-access article distributed under the terms of the Creative Commons Attribution License (CC BY). The use, distribution or reproduction in other forums is permitted, provided the original author(s) and the copyright owner(s) are credited and that the original publication in this journal is cited, in accordance with accepted academic practice. No use, distribution or reproduction is permitted which does not comply with these terms.
*Correspondence: Ming Liu, d3lwbG1oQGhvdG1haWwuY29t
†These authors have contributed equally to this work and share first authorship