- 1BARLO MS Center, St. Michael's Hospital, Toronto, ON, Canada
- 2Keenan Research Centre for Biomedical Science, St. Michael's Hospital, Toronto, ON, Canada
- 3Institute of Infection & Immunity, University of Glasgow, Glasgow, Scotland, United Kingdom
- 4Institute of Medical Science, Temerty Faculty of Medicine, University of Toronto, Toronto, ON, Canada
Research suggests that physical exercise can promote an anti-inflammatory and neuroprotective state. If so, increasing or optimizing exercise could be considered a 'disease-modifying intervention' in neuroinflammatory diseases, such as multiple sclerosis (MS). Exercise intervention studies conducted in animal models of MS are promising. Various aerobic and strength training regimes have been shown to delay disease onset and to reduce both the clinical and pathological disease severity in mice. However, fundamental differences between the physiology of animals and humans, the disease states studied, and the timing of exercise intervention are significant. In animal models of MS, most exercise interventions begin before disease initiation and before any clinical sign of disease. In contrast, studies in humans recruit participants on average nearly a decade after diagnosis and often once disability is established. If, as is thought to be the case for disease-modifying treatments, the immunomodulatory effect of exercise decreases with advancing disease duration, current studies may therefore fail to detect the true disease-modifying potential. Clinical studies in early disease cohorts are needed to determine the role of exercise as a disease-modifying intervention for people with MS.
Introduction
Multiple sclerosis (MS) is an immune-mediated disorder of the central nervous system (CNS). The underlying pathological process is a triad of inflammation, demyelination, and neurodegeneration, with inflammation particularly dominant in early disease (1). Clinically, MS can be described as 'relapsing remitting MS' (RRMS), where acute bouts of neurological deficit are followed by variable recovery and stability, or as progressive MS [primary progressive (PPMS) or secondary progressive (SPMS)], where insidious accumulation of disability occurs with less pronounced relapses (2). Highly effective disease-modifying treatments (DMTs) that target immune cells have revolutionized the management of RRMS (3, 4). However, despite significantly reducing clinical and radiological evidence of focal disease activity, the success of DMTs in preventing disease progression has been relatively disappointing. Progression independent of relapse activity still occurs (5). Prescription of DMTs in progressive MS remains restricted and their use may also be limited over concerns of their risk of potentially serious adverse effects. This is particularly true early in the disease course when patients are often young with minimal disability. Unfortunately, it is at this point, early in the disease, when DMTs have the greatest potential to impact the long-term risk of disability (4).
Exercise as a disease-modifying intervention
A “disease-modifying intervention” (DMI) describes the act of altering a modifiable trait or state with the aim of delaying, slowing or lessening disease progression. In MS, DMIs might include smoking cessation (6), reducing obesity (7), or changes to diet (8). DMIs are a separate entity from disease-modifying treatments and should be considered in all patients with MS (pwMS), irrespective of DMT status.
Exercise is defined as a purposeful, effortful physical activity performed to promote or maintain health or fitness. Exercise can take numerous forms but can be broadly characterized as resistance training or aerobic activity. Resistance training strains the neuromuscular system, boosting anaerobic endurance, increasing muscle strength, and enhancing bone health (9). Aerobic exercise taxes the cardiovascular system, increasing aerobic capacity (9). Aerobic capacity is a strong health predictor and is associated with lower all-cause mortality (10).
Research conducted in animal models of MS suggests that exercise may modulate the systemic immune system to promote an anti-inflammatory and neuroprotective state within the CNS (11). If so, exercise might also be considered a DMI (12, 13). However, whether the immunomodulatory effects of exercise in rodents translate to humans, and, if so, how such effects might be maximized in the care of pwMS, is unknown.
Methods
To better understand whether the disease modifying effects of exercise seen in animal models of MS are potentially clinically translatable, we conducted a literature review comparing preclinical and clinical exercise intervention studies. As this was a literature review, no priori review protocol or search strategy were implemented. Based on our findings, we then conduced systematic reviews of the timing of exercise intervention in the most commonly employed animal model of MS (experimental autoimmune encephalomyelitis – EAE) and in people with MS. We developed illustrative figures based on these results. The search strategies are depicted in Table 1. All statistics were calculated using GraphPad Prism version 8.4.3. Data were not normally distributed and are depicted as median and interquartile range. Figures were created using GraphPad prism and BioRender.com.
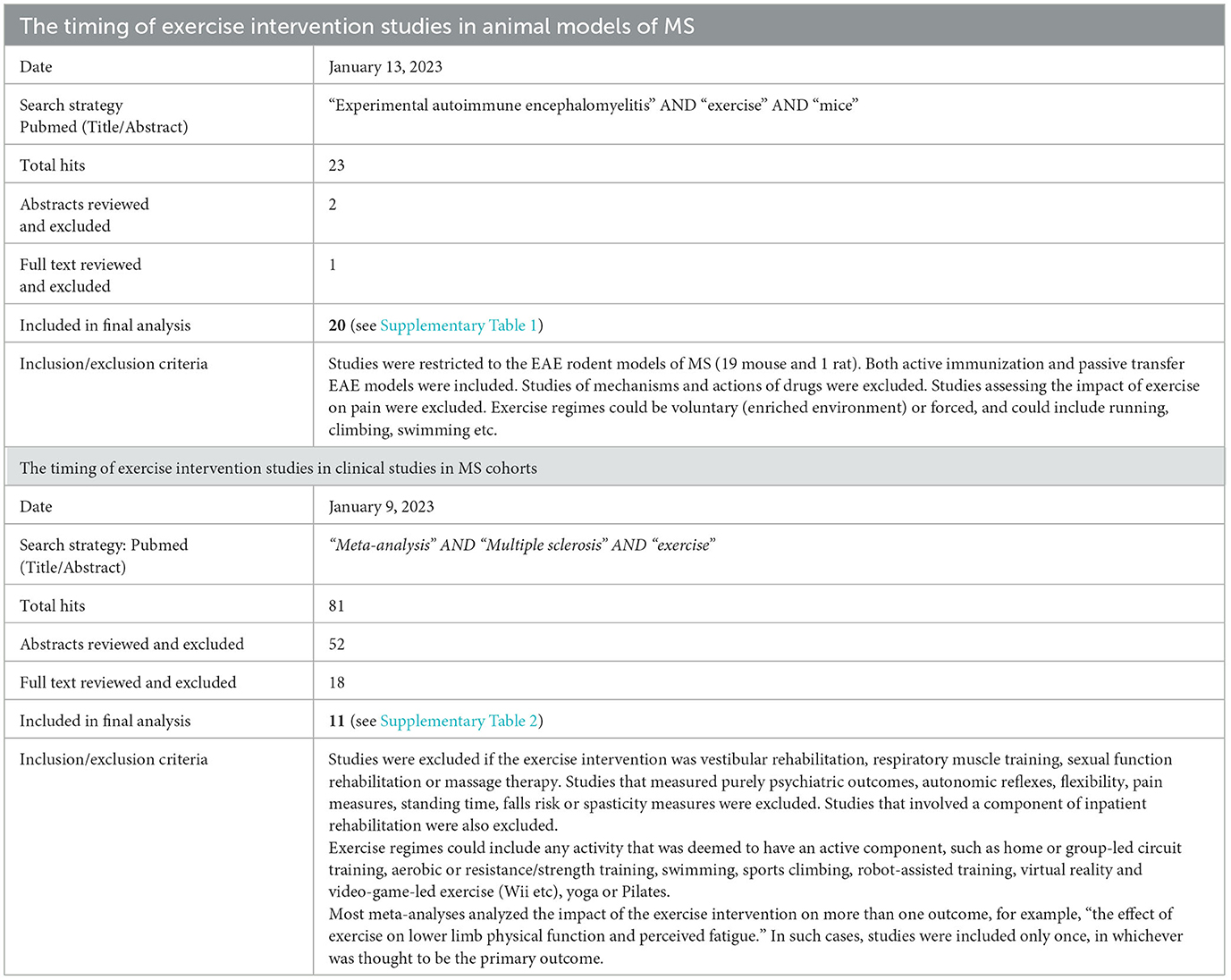
Table 1. Systematic search strategy to determine the timing of exercise interventions in animal models of MS compared with clinical studies in MS cohorts.
Exercise intervention studies are conducted too early in animal models of MS
Animal models of MS enable exercise intervention studies to be conducted over short periods of time, at clearly defined points in the disease, and to link clinical outcomes with pathological analysis; and results are promising. Both aerobic and resistance training regimes have been shown to delay disease onset and reduce both clinical and pathological disease severity (14–18). These outcomes are thought to primarily arise through modulation of the systemic immune system; however, the specific mechanism of modulation appears to differ depending on the type and intensity of exercise.
Experimental autoimmune encephalomyelitis (EAE) is the most employed animal model of MS. EAE can be induced by immunization with myelin proteins (usually myelin oligodendrocyte glycoprotein, MOG, or proteolipid protein, PLP) or through adoptive transfer of autoreactive T cells. Different models result in different EAE phenotypes. For example, PLP-induced EAE is reported to be milder, with a remitting-relapsing course, compared with the chronic disease course of MOG-induced EAE (19). Spontaneous EAE models also exist. In chronic EAE models (where mice develop a chronic motor deficit), strength training has been shown to upregulate peripherally circulating regulatory T cells (Tregs), whereas endurance training had a greater effect on restoration of blood-brain-barrier (BBB) integrity (15). High-intensity interval training has been shown to reduce populations of pro-inflammatory T helper (Th) cells (Th1 and Th17) specifically, whereas high-intensity continuous training led to a more general reduction in T cell populations (20). Studies in different animal models, such as the Cuprizone and lysolecithin-induced demyelination models, suggest that exercise may also exert effects directly within the CNS through limitation of microglial activation (18) and increase of local anti-oxidant responses (21).
There are of course limitations in drawing conclusions from animal models. Animal models only partly mimic the pathology of MS; rodent stamina, activity levels, and innate drive to exercise differ significantly from humans; and certain training regimes, such as forced exercise, cannot be recapitulated and the effect of stress may independently alter the disease course (22). In addition, we noted that most studies in animal models begin the training regime prior to initiation of the disease state, Figure 1 and Supplementary Table 1.
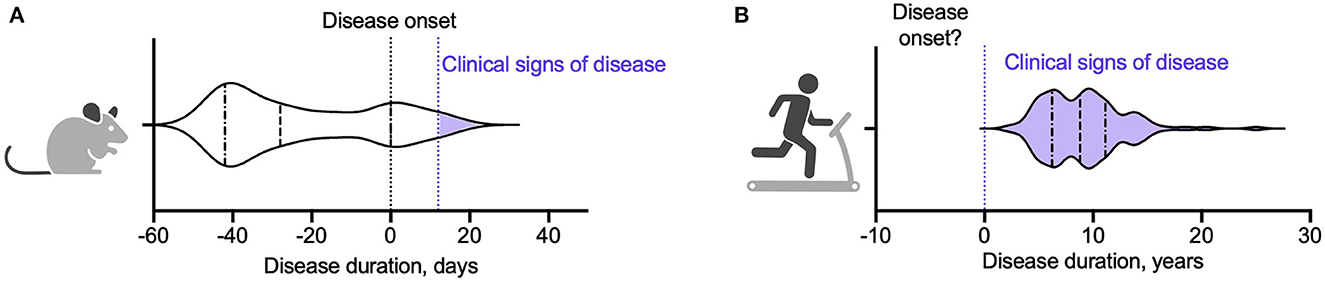
Figure 1. Violin plots showing point of initiation of exercise intervention in (A) an animal model of MS and (B) people with MS. (A) Summary of twenty studies assessing the impact of exercise on clinical, immunological, and pathological outcomes in EAE (15–17, 20, 23, 53–67). Animals began training a median of 28 days prior to EAE induction (denoted as day 0). The inter-quartile range was −42 to 0 days. Fourteen studies (70%) initiated and/or completed the exercise intervention prior to EAE induction. One study initiated the exercise intervention on day 0 (63). Of the five studies that started the exercise intervention after induction of EAE, three began the exercise programme on day 1 (prior to the animals developing clinical signs of disease) (53, 61, 62) and two had subgroups that began exercise at the point the animals developed clinical signs of disease (day 12 and day 18) (16, 23). (B) Summary of 117 studies from eleven meta-analyses assessing the impact of exercise interventions on clinical and immunological measures in pwMS (30, 31, 34, 35, 50, 51, 68–72). Study participants had a median disease duration of 8.8 years. The inter-quartile range was 6.2–11 years.
Few studies have examined the effects of an exercise intervention delivered after induction of EAE, and those that have report mixed results. Klaren et al. (23) found no significant effect of exercise intervention on clinical disability scores when animals exercised during remission after initial disease onset in a PLP-induced relapsing remitting EAE model. In contrast, Le Page et al. (14) found that 2 days of severe exercise after transfer of encephalitogenic T cells delayed disease onset (but not ultimate disease severity), whereas the same exercise regime prior to induction of adoptive EAE did not change the disease course (14). Finally, Shahidi et al. (16). showed that aerobic exercise reduced clinical and pathological severity of EAE equally, regardless of whether mice started training before EAE induction or after the clinical onset of disease. Variability in results from these studies may reflect the relatively short window (two-three weeks) between disease initiation and animal sacrifice. Beginning an exercise intervention only after initiation of disease reduces the duration of training (and potentially also the training intensity), and as such may underestimate potential beneficial effects (22).
Exercise intervention studies are conducted late in people with MS
In healthy humans, moderate-intensity exercise (in particular) has been shown to promote an anti-inflammatory and neuroprotective state through multiple pathways (24). Myocyte release of interleukin-6 (IL-6) upregulates the release of anti-inflammatory cytokines (such as IL-10 and IL-1 receptor alpha) and downregulates the release of pro-inflammatory cytokines (such as TNF-α and IFN-γ) (24–26). Proliferation of naive cell subsets alongside apoptosis of quiescent and exhausted cells alters circulating cell profiles (24, 26). Exercise has also been shown to enhance expression of neurotrophins, such as brain derived neurotrophic factor (BDNF), that regulate neurogenesis, neuronal function and survival (27). Finally, exercise may also modulate glia cell phenotype and function (28).
However, whether biological and physiological responses to exercise differ in the context of immune dysregulation is not clear. For example, one meta-analysis found that, in contrast with controls, neither acute nor regular exercise led to a significant change in peripherally circulating IL-6 in pwMS (29). Moreover, whether the immunomodulatory changes that occur in the context of exercise might have a clinically meaningful impact on the disease course in MS requires specific studies.
The literature on exercise intervention studies in pwMS is expansive, with huge heterogeneity in participant cohorts, training regimes, study durations and endpoints. It is notable however that the majority of studies are conducted in MS cohorts with long disease durations and established disability, Figure 2, Supplementary Table 2. This is perhaps unsurprising, given the traditional view of exercise as rehabilitation (13). For example, studies of upper limb strength training tend to recruit individuals with arm weakness or for whom upper limb function is particularly important, such as wheelchair users. Such cohorts are likely to have long disease durations and high disability scores [measured by the Expanded Disability Status Scale (EDSS)], as demonstrated in a systematic review by Neira et al. (30) in which participants across eight studies of upper limb strength training had average disease durations between 9 and 27 years, and EDSS scores between 3.5 and 9.
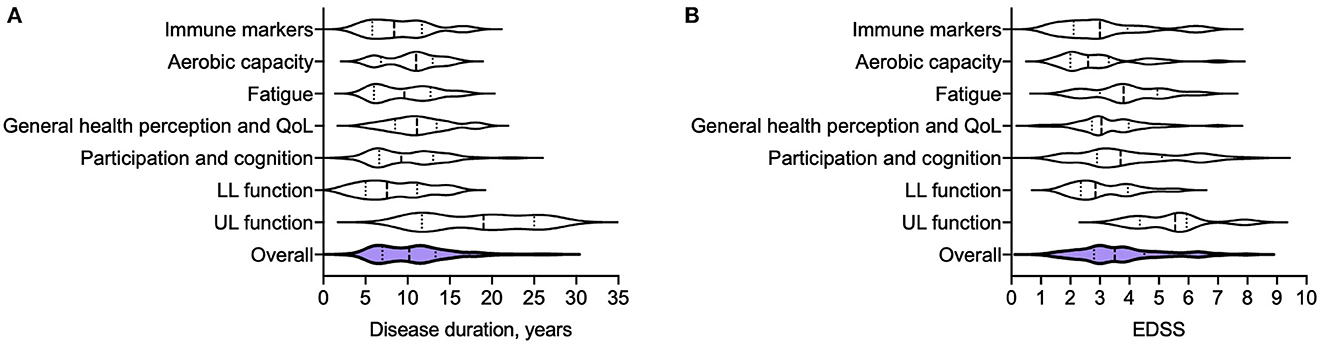
Figure 2. Disease duration (A) and EDSS (B) of MS participants from 166 different exercise intervention studies that were summarized in 11 meta-analyses (30, 31, 34, 35, 50, 51, 68–72). Disease duration and EDSS are reported as median (years), and interquartile range. Immune markers: Disease duration 8.4 (5.8–12), EDSS 3 (2.1–3.9) (35, 72); Aerobic capacity: Disease duration 11 (6.8–13), EDSS 2.6 (2–3.3) (31); Fatigue: Disease duration 9.6 (6–13), EDSS 3.8 (3–5) (50); General health perception and quality of life (QoL): Disease duration 11 (8.5–13), EDSS 3.1 (2.7–4) (34, 51, 70);Participation and cognition: Disease duration 19 (12–25), EDSS 5.6 (4.5–5.9) (68, 71); Lower limb function: Disease duration 8.1 (5–11), EDSS 2.9 (2.4–4) (69); Upper limb function: Disease duration 9.3 (6.6–13), EDSS, 3.7 (2.9–5.1) (30); Summary: Disease duration of overall cohort (from 117 separate studies) 8.8 (6.2–11), EDSS of overall cohort (from 166 separate studies) 2.7 (2–3.5).
Exercise intervention studies of lower limb function or aerobic capacity may have lower average EDSS scores, but are often heterogeneous. Langeskov-Christensen et al. (31) analyzed the effects of aerobic training on aerobic capacity across 17 randomized control trials of participants with an EDSS range of 0 to 8. A significant effect in favor of exercise intervention was found, but heterogeneity between results was thought to reflect variability between study cohorts. One potential explanation posed was that deconditioning in higher EDSS groups may limit the degree of improvement in aerobic capacity over a short study period (31).
Studies across the entire clinical spectrum of MS are needed to understand the specific effects of exercise at different disease stages. However, if, as is thought to be the case for DMTs, the immunomodulatory potential is greatest in early disease, current exercise studies may miss the “window of opportunity” in which to exert a clinically meaningful effect on the disease course (4, 32). To date, few clinical studies have been conducted specifically in early disease cohorts (32).
Can evidence from animal studies inform the design of exercise studies in pwMS?
Animal models provide important mechanistic insights. For example, results suggest that various types of exercise have the potential to induce immunomodulatory effects, and that future exercise intervention studies might not need to restrict participants to a specific modality or regime. Preclinical studies have provided valuable insights into the anti-inflammatory effects associated with exercise. Although few preclinical studies have investigated the effect of exercise intervention after disease onset, those that have suggest the role of exercise as DMI is worth pursuing in clinical studies (16).
Human studies, in “real-world” cohorts (and ideally in early disease) are required to learn how to optimize exercise regimes in order to maximize therapeutic effects. Real-world clinical studies are also required to identify measures or biomarkers that could be employed in the monitoring of an exercise activity or the response to exercise, and then linked with long-term clinical outcomes.
Wearable biosensor technologies could be employed to monitor exercise adherence and provide an estimate of exercise intensity (33). Depending on the exercise intervention, quantification of VO2max (31), “1 rep max” strength (34), grip strength (30) or timed walk test could be used as a measure of the overall physical effects of exercise. The identification of a specific fluid biomarker that might best reflect an individual's immune response to exercise is more difficult. Changes in circulating cell profiles are found pre-clinic studies, but results are heterogeneous, and analysis expensive to perform and labor intensive. Protein biomarkers may be most easily adapted to clinic studies (and thus clinical practice).
BDNF is a member of the neurotrophin family. Neurotrophins play an essential role in neuro-regeneration and neuroprotection (27, 35). In pwMS, blood BDNF levels have been reported to increase during relapse (potentially as a compensatory mechanism) and normalize during remission (35). A recent meta-analysis of 13 studies found that physical activity in pwMS significantly increased baseline serum BDNF levels (35), as has been previously described in healthy populations. However, when broken down into subgroups, no significant differences were identified related to exercise type or duration. Although the study was unable to identify a direct connection between exercise intensity or duration with BDNF alterations, it is possible that variations in study designs, the small size of study groups, diverse patient backgrounds, and different BDNF measurement techniques might obscure the potential effects of specific exercises and their duration on BDNF levels.
IL-6 is a pleotropic cytokine that has been shown to have both pro and anti-inflammatory effects, possibly depending on the source of the cytokine (29). IL6 secreted by B cells, T cells and macrophages, induces synthesis of acute phase proteins, and stimulates antibody production and effector T cell development (36). IL-6 is upregulated in myocytes during exercise in response to a fall in muscle glycogen content and in proportion to exercise intensity (24). IL-6 released from myocytes may upregulate the release of anti-inflammatory cytokines (such as IL-10 and IL-1 receptor alpha) and downregulate the release of pro-inflammatory cytokines (such as TNF and IFN-γ) (24–26, 37).
IL-6 has been studied as a biomarker of the immune effects of exercise in athletes, controls and disease populations (24). Studies in pwMS show inconsistent results. Some report an increase in IL-6 in both pwMS (RRMS) and controls (38), whereas, others reporting no significant acute or long-term change in IL-6 in pwMS (progressive MS) following training (39). One potential reason for discrepancy may be variability in the exercise regimes or intensity achieved between RRMS and progressive MS populations.
TNF is another pleiotropic cytokine that is thought to exert both pro- and inti-inflammatory effects and play a role in immune dysregulation, neuroinflammation and demyelination in MS (40). In post-mortem studies, high TNF levels are found in close proximity of MS lesions (40). Several studies have demonstrated elevated TNF levels in blood or CSF, with some showing a correlation with disease progression or disease activity (29, 38, 40).
In healthy controls, TNF concentrations have been shown to increase immediately following resistance training and recover within 24 h (41). Similar responses have been reported in pwMS (38). Interestingly, one study found that whilst resting levels of cytokines (including TNF) did not differ between sedentary and trained pwMS, sedentary pwMS show a blunted cytokine response to exercise compared with trained pwMS (42). However, in contrast, meta-analysis of 11 studies reported that baseline TNF levels in the blood of pwMS decreased after regular exercise (29), suggesting that regular exercise may have an anti-inflammatory effect on blood TNF levels in pwMS.
Protein biomarkers of axonal damage, such as neurofilament light chain (NfL), or astroglial activation, such as glial fibrillary acidic protein (GFAP) may serve as ‘endpoint' biomarkers. In one randomized control trial (RCT), 38 individuals with RRMS who participated in an 8-week aerobic training programme had significantly lower serum NfL and GFAP levels post-training compared with pre-training. No significant changes in either biomarker were observed in the control group (43). However, another RCT of 89 pwMS found no significant change in either NfL or GFAP over a 16-week aerobic training period (44).
Whilst there are many potential confounders (including age; sex; genetics; co-morbidities; concurrent infection; type, duration and effort of exercise; and timing of blood-draw in relation to exercise), the identification of biomarkers that provide insight into an individual's immunomodulatory responsiveness to exercise could enable “personalized prescription” of exercise as a component of precision medicine (45). This would be a significant shift from the current status quo where exercise is viewed as a tertiary therapy for rehabilitation, to exercise as a therapy for secondary (13, 32), or possibly even primary prevention (13, 46, 47).
Human studies cannot replicate preclinical models in initiating an exercise intervention prior to clinical disease onset. However, several retrospective analyses of physical activity and risk of MS have been conducted to study the potential primary preventative effects of exercise in humans.
A large case-control study across several European countries found that vigorous physical activity in adolescence was inversely associated with risk of MS, with an odds ratio of 0.74 after adjusting for confounders such as outdoor activity, body size, smoking and infective mononucleosis (48). Another study analyzed a historical cohort who undertook mandatory conscription at age 19 years and found a similar inverse relationship between aerobic fitness and MS risk, with an adjusted relative risk of 0.69 (46). Directionality of causality is always a consideration, however results remained significant when men who developed MS within 10 years of conscriptions were excluded from analysis (46). These studies raise the question whether greater physical fitness truly protects against developing MS, or, as is seen in animal models, postpones clinical presentation (13, 47); and if so, whether postponement occurs because the disease course has been modified, or simply because a greater physical reserve might better mask disability.
Promoting exercise as routine clinical care for all pwMS
Exercise has a low-risk profile and is safe for pwMS. In addition to the physical gains in endurance, balance and strength, positive effects on fatigue and mood can improve quality of life and work outcomes (49–52). This is significant as symptoms such as fatigue are common, intrusive, and difficult to treat; and DMTs have little impact. For these reasons, the general health benefits of exercise should be promoted to all pwMS, but further research is required before we can advocate the disease modifying effects.
Conclusion
Research conducted in animal models suggests that exercise has the potential to modify the disease course of MS, particularly if prescribed early and alongside DMTs (11, 17). However, the substantial discord between preclinical research and “real-world” clinical studies limits our ability to determine whether exercise regimes that produce immunomodulatory effects in animals are feasible, practical, and clinically translatable. Clinical studies, specifically in early disease cohorts, are needed to determine whether exercise could have a role as a disease modifying intervention for people with MS.
Author contributions
S-JM: data curation, analysis, and visualization. All authors contributed equally to conceptualization, literature review, manuscript reviewing, and editing.
Funding
This work was supported by the Branch Out Neurological Foundation.
Acknowledgments
We wish to thank Professor Nathalie Arbor for the insightful discussion about animal models of MS.
Conflict of interest
S-JM has received a grant from the MS Society of Canada and funding for international conference attendance from EMD-Serono. RS received grants from the MS Society of Canada and the J.P. Bickell Foundation, received consulting fees from Biogen-Idec and Novartis, received payment or honoraria for lectures, presentations, speaker's bureaus, manuscript writing or educational events from Biogen-Idec, Sanofi Genzyme, and EMD-Serono, has participated on advisory boards for Novartis and BMS, and received support to attend a scientific meeting from EMD-Serono.
Publisher's note
All claims expressed in this article are solely those of the authors and do not necessarily represent those of their affiliated organizations, or those of the publisher, the editors and the reviewers. Any product that may be evaluated in this article, or claim that may be made by its manufacturer, is not guaranteed or endorsed by the publisher.
Supplementary material
The Supplementary Material for this article can be found online at: https://www.frontiersin.org/articles/10.3389/fneur.2023.1190208/full#supplementary-material
References
1. Lassmann H. Pathogenic mechanisms associated with different clinical courses of multiple sclerosis. Front Immunol. (2019) 9:3116. doi: 10.3389/fimmu.2018.03116
2. Lublin FD, Reingold SC, Cohen JA, Cutter GR, Sørensen PS, Thompson AJ, et al. Defining the clinical course of multiple sclerosis: the 2013 revisions. Neurology. (2014) 83:278–86. doi: 10.1212/WNL.0000000000000560
3. Martin R, Sospedra M, Rosito M, Engelhardt B. Current multiple sclerosis treatments have improved our understanding of MS autoimmune pathogenesis. Eur J Immunol. (2016) 46:2078–90. doi: 10.1002/eji.201646485
4. Simonsen CS, Flemmen HØ, Broch L, Brunborg C, Berg-Hansen P, Moen SM, et al. Early high efficacy treatment in multiple sclerosis is the best predictor of future disease activity over 1 and 2 years in a Norwegian population-based registry. Front Neurol. (2021) 12:693017. doi: 10.3389/fneur.2021.693017
5. Kappos L, Wolinsky JS, Giovannoni G, Arnold DL, Wang Q, Bernasconi C, et al. Contribution of relapse-independent progression vs relapse-associated worsening to overall confirmed disability accumulation in typical relapsing multiple sclerosis in a pooled analysis of 2 randomized clinical trials. JAMA Neurol. (2020) 77:1132–40. doi: 10.1001/jamaneurol.2020.1568
6. Healy BC, Ali EN, Guttmann CRG, Chitnis T, Glanz BI, Buckle G, et al. Smoking and disease progression in multiple sclerosis. Arch Neurol. (2009) 66:858–64. doi: 10.1001/archneurol.2009.122
7. Lutfullin I, Eveslage M, Bittner S, Antony G, Flaskamp M, Luessi F, et al. Association of obesity with disease outcome in multiple sclerosis. J Neurol Neurosurg Psychiatr. (2023) 97:57–61. doi: 10.1136/jnnp-2022-329685
8. Katz Sand I. The role of diet in multiple sclerosis: mechanistic connections and current evidence. Curr Nutr Rep. (2018) 7:150–60. doi: 10.1007/s13668-018-0236-z
9. Garber CE, Blissmer B, Deschenes MR, Franklin BA, Lamonte MJ, Lee I-M, et al. Quantity and quality of exercise for developing and maintaining cardiorespiratory, musculoskeletal, and neuromotor fitness in apparently healthy adults guidance for prescribing exercise. Med Sci Sports Med. (2011) 43:1334–59. doi: 10.1249/MSS.0b013e318213fefb
10. Kodama S, Saito K, Tanaka S, Maki M, Yachi Y, Asumi M, et al. Cardiorespiratory fitness as a quantitative predictor of all-cause mortality and cardiovascular events in healthy men and women: a meta-analysis. JAMA. (2009) 301:2024–35. doi: 10.1001/jama.2009.681
11. Parnow A, Hafedh M, Tsunoda I, Patel DI, Baker JS, Saeidi A, et al. Effectiveness of exercise interventions in animal models of multiple sclerosis. Front Med. 10:1143766. doi: 10.3389/fmed.2023.1143766
12. Motl R, Pilutti L. The benefits of exercise training in multiple sclerosis. Nat Rev Neurol. (2012) 8:487–97. doi: 10.1038/nrneurol.2012.136
13. Dalgas U, Langeskov-Christensen M, Stenager E, Riemenschneider M, Hvid LG. Exercise as medicine in multiple sclerosis—time for a paradigm shift: preventive, symptomatic, and disease-modifying aspects and perspectives. Curr Neurol Neurosci Rep. (2010) 19, 1–13. doi: 10.1007/s11910-019-1002-3
14. Page CL, Bourdoulous S, Béraud E, Couraud PO, Rieu M, Ferry A. Effect of physical exercise on adoptive experimental auto-immune encephalomyelitis in rats. Eur J Appl Physiol Occup Physiol. (1996) 73:130–5. doi: 10.1007/BF00262821
15. Souza PS, Gonçalves ED, Pedroso GS, Farias HR, Junqueira SC, Marcon R, et al. Physical exercise attenuates experimental autoimmune encephalomyelitis by inhibiting peripheral immune response and blood-brain barrier disruption. Mol Neurobiol. (2017) 54:4723–37. doi: 10.1007/s12035-016-0014-0
16. Shahidi SH, Kordi MR, Rajabi H, Malm C, Shah F, Quchan ASK. Exercise modulates the levels of growth inhibitor genes before and after multiple sclerosis. J Neuroimmunol. (2020) 341:577172. doi: 10.1016/j.jneuroim.2020.577172
17. Bernardes D, De Oliveira ALR. Regular exercise modifies histopathological outcomes of pharmacological treatment in experimental autoimmune encephalomyelitis. Front Neurol. (2018) 9:950. doi: 10.3389/fneur.2018.00950
18. Gentile A, Musella A, De Vito F, Rizzo FR, Fresegna D, Bullitta S, et al. Immunomodulatory effects of exercise in experimental multiple sclerosis. Front Immunol. (2019) 10:21297. doi: 10.3389/fimmu.2019.02197
19. Badawi AH, Siahaan TJ. Suppression of MOG- and PLP-induced experimental autoimmune encephalomyelitis using a novel multivalent bifunctional peptide inhibitor. J Neuroimmunol. (2013) 263:20–7. doi: 10.1016/j.jneuroim.2013.07.009
20. Goldberg Y, Fainstein N, Zaychik Y, Hamdi L, Segal S, Nabat H, et al. Continuous and interval training attenuate encephalomyelitis by separate immunomodulatory mechanisms. Ann Clin Transl Neurol. (2021) 8:190–200. doi: 10.1002/acn3.51267
21. Lozinski BM, de Almeida LGN, Silva C, Dong Y, Brown D, Chopra S, et al. Exercise rapidly alters proteomes in mice following spinal cord demyelination. Sci Rep. (2021) 11:7239. doi: 10.1038/s41598-021-86593-5
22. Klaren RE, Motl RW, Woods JA, Miller SD. Effects of exercise in experimental autoimmune encephalomyelitis (an animal model of multiple sclerosis). J Neuroimmunol. (2014) 274:14–9. doi: 10.1016/j.jneuroim.2014.06.014
23. Klaren RE, Stasula U, Steelman AJ, Hernandez J, Pence BD, Woods JA, et al. Effects of exercise in a relapsing-remitting model of experimental autoimmune encephalomyelitis. J Neurosci Res. (2016) 94:907–14. doi: 10.1002/jnr.23783
24. Gleeson M, Bishop NC, Stensel DJ, Lindley MR, Mastana SS, Nimmo MA. The anti-inflammatory effects of exercise: Mechanisms and implications for the prevention and treatment of disease. Nat Rev Immunol. (2011) 11:607–10. doi: 10.1038/nri3041
25. Pedersen BK, Fischer CP. Beneficial health effects of exercise — the role of IL-6 as a myokine. Trends Pharacol Sci. (2007) 28:152–6. doi: 10.1016/j.tips.2007.02.002
26. Duggal NA, Niemiro G, Harridge SDR, Simpson RJ, Lord JM. Can physical activity ameliorate immunosenescence and thereby reduce age-related multi-morbidity? Nat Rev Immunol. (2019) 19:563–72. doi: 10.1038/s41577-019-0177-9
27. Miranda M, Morici JF, Zanoni MB, Bekinschtein P. Brain-derived neurotrophic factor: a key molecule for memory in the healthy and the pathological brain. Front Cell Neurosci. (2019) 13:1–25. doi: 10.3389/fncel.2019.00363
28. Barad Z, Augusto J, Kelly ÁM. Exercise-induced modulation of neuroinflammation in ageing. J Physiol. (2022) 45:1–15. doi: 10.1113/JP282894
29. Shobeiri P, Seyedmirzaei H, Karimi N, Rashidi F, Teixeira AL, Brand S, et al. IL-6 and TNF-α responses to acute and regular exercise in adult individuals with multiple sclerosis (MS): a systematic review and meta-analysis. Eur J Med Res. (2022) 27:185. doi: 10.1186/s40001-022-00814-9
30. Neira VE, Niemietz TD, Farrell III JW. The effects of exercise training on upper extremity function for persons with multiple sclerosis: a systematic review. J Rehabil Med Clin Commun. (2022) 5: jrmcc00087. doi: 10.2340/jrmcc.v5.2306
31. Langeskov-Christensen M, Heine M, Kwakkel G, Dalgas U. Aerobic capacity in persons with multiple sclerosis: a systematic review and meta-analysis. Sports Med. (2015) 45:905–23. doi: 10.1007/s40279-015-0307-x
32. Riemenschneider M, Hvid LG, Stenager E, Dalgas U. Is there an overlooked “window of opportunity” in MS exercise therapy? Perspectives for early MS rehabilitation. Multiple Sclerosis J. (2018) 24:886–94. doi: 10.1177/1352458518777377
33. Bradshaw MJ, Farrow S, Motl RW, Chitnis T. Wearable biosensors to monitor disability in multiple sclerosis. Neurol Clin Pract. (2017) 7: 354–62. doi: 10.1212/CPJ.0000000000000382
34. Andreu-Caravaca L, Ramos-Campo DJ, Chung LH, Martínez-Rodríguez A, Rubio-Arias JÁ. Effects and optimal dosage of resistance training on strength, functional capacity, balance, general health perception, and fatigue in people with multiple sclerosis: a systematic review and meta-analysis. Disabil Rehabil. (2022) 45:1595–1607.
35. Shobeiri P, Karimi A, Momtazmanesh S, Teixeira AL, Teunissen CE, van Wegen EEH, et al. Exercise-induced increase in blood-based brain-derived neurotrophic factor (BDNF) in people with multiple sclerosis: A systematic review and meta-analysis of exercise intervention trials. PLoS ONE. (2022) 17:1–20. doi: 10.1371/journal.pone.0264557
36. Tanaka T, Narazaki M, Kishimoto T. Il-6 in inflammation, immunity, and disease. Cold Spring Harb Perspect Biol. (2014) 6:16295. doi: 10.1101/cshperspect.a016295
37. Petersen A, Pedersen B. The role of IL-6 in mediating the anti-inflammatory effects of exercise. J Physiol Pharmacol. (2006) 10:43–51. doi: 10.1249/00005768-200605001-00226
38. Castellano V, Patel DI, White LJ. Cytokine response to acute and chronic exercise in multiple sclerosis. J Appl Physiol. (2008) 104:1697–702. doi: 10.1152/japplphysiol.00954.2007
39. Briken S, Rosenkranz SC, Keminer O, Patra S, Ketels G, Heesen C, et al. Effects of exercise on Irisin, BDNF and IL-6 serum levels in patients with progressive multiple sclerosis. J Neuroimmunol. (2016) 299:53–8. doi: 10.1016/j.jneuroim.2016.08.007
40. Fresegna D, Bullitta S, Musella A, Rizzo FR, De Vito F, Guadalupi L, et al. Re-examining the role of TNF in MS pathogenesis and therapy. Cells. (2020) 9:290. doi: 10.3390/cells9102290
41. Townsend JR, Hoffman JR, Fragala MS, Jajtner AR, Gonzalez AM, Wells AJ, et al. TNF-α and TNFR1 responses to recovery therapies following acute resistance exercise. Front Physiol. (2015) 6:48. doi: 10.3389/fphys.2015.00048
42. Heesen C, Gold SM, Hartmann S, Mladek M, Reer R, Braumann K-M, et al. Endocrine and cytokine responses to standardized physical stress in multiple sclerosis. Brain Behav Immun. (2003) 17:473–81. doi: 10.1016/S0889-1591(03)00077-1
43. Ercan Z, Bilek F, Demir CF. The effect of aerobic exercise on Neurofilament light chain and glial Fibrillary acidic protein level in patients with relapsing remitting type multiple sclerosis. Mult Scler Relat Disord. (2021) 9:2290. doi: 10.1016/j.msard.2021.103219
44. Gravesteijn A, Beckerman H, Willemse E, Hulst H, de Jong B, Teunissen C, et al. Brain-derived neurotrophic factor, neurofilament light and glial fibrillary acidic protein do not change in response to aerobic training in people with MS-related fatigue - a secondary analysis of a randomized controlled trial. Mult Scler Relat Disord. (2022) 70: 104489. doi: 10.1016/j.msard.2022.104489
45. Pedersen BK, Saltin B. Exercise as medicine - Evidence for prescribing exercise as therapy in 26 different chronic diseases. Scand J Med Sci Sports. (2015) 25:1–72. doi: 10.1111/sms.12581
46. Cortese M, Riise T, Bjørnevik K, Myhr K-M, Group MSCSDS. Body size and physical exercise, and the risk of multiple sclerosis. Multiple Sclerosis J. (2018) 24:270–8. doi: 10.1177/1352458517699289
47. Dorans KS, Massa J, Chitnis T, Ascherio A, Munger KL. Physical activity and the incidence of multiple sclerosis. Neurology. (2016) 87:1770–6. doi: 10.1212/WNL.0000000000003260
48. Wesnes K, Myhr K-M, Riise T, Cortese M, Pugliatti M, Boström I, et al. Physical activity is associated with a decreased multiple sclerosis risk: the EnvIMS study. Multiple Sclerosis. (2018) 24:150–7. doi: 10.1177/1352458517694088
49. Nourbakhsh B, Revirajan N, Morris B, Cordano C, Creasman J, Manguinao M, et al. Safety and efficacy of amantadine, modafinil, and methylphenidate for fatigue in multiple sclerosis: a randomised, placebo-controlled, crossover, double-blind trial. Lancet Neurol. (2021) 20:38–48. doi: 10.1016/S1474-4422(20)30354-9
50. Heine M, van de Port I, Rietberg MB, van Wegen EE, Kwakkel G. Exercise therapy for fatigue in multiple sclerosis. Cochrane Database Syst Rev. (2015) 2015:CD009956. doi: 10.1002/14651858.CD009956.pub2
51. Latimer-Cheung AE, Pilutti LA, Hicks AL, Ginis KAM, Fenuta AM, MacKibbon KA, et al. Effects of exercise training on fitness, mobility, fatigue, and health-related quality of life among adults with multiple sclerosis: a systematic review to inform guideline development. Arch Phys Med Rehabil. (2013) 94:1800–28. doi: 10.1016/j.apmr.2013.04.020
52. Motl RW, Gosney JL. Effects of exercise training on quality of life in multiple sclerosis: a meta-analysis. Multiple Sclerosis. (2008) 14:129–35. doi: 10.1177/1352458507080464
53. Gilio L, Fresegna D, Gentile A, Guadalupi L, Sanna K, De Vito F, et al. Preventive exercise attenuates IL-2-driven mood disorders in multiple sclerosis. Neurobiol Dis. (2022) 172:105817. doi: 10.1016/j.nbd.2022.105817
54. El-Emam MA, Achy SE, Abdallah DM, El-Abhar HS, Gowayed MA. Does physical exercise improve or deteriorate treatment of multiple sclerosis with mitoxantrone? Experimental autoimmune encephalomyelitis study in rats. BMC Neurosci. (2022) 23:1–17. doi: 10.1186/s12868-022-00692-1
55. Zaychik Y, Fainstein N, Touloumi O, Goldberg Y, Hamdi L, Segal S, et al. High-intensity exercise training protects the brain against autoimmune neuroinflammation: regulation of microglial redox and pro-inflammatory functions. Front Cell Neurosci. (2021) 15: 640724. doi: 10.3389/fncel.2021.640724
56. Torabimehr F, Kordi MR, Nouri R, Ai J, Shirian S. The role of forced and voluntary training on accumulation of neural cell adhesion molecule and polysialic acid in muscle of mice with experimental autoimmune encephalomyelitis. Evid Based Compl Alternat Med. (2020) 2020:5160958. doi: 10.1155/2020/5160958
57. Fainstein N, Tyk R, Touloumi O, Lagoudaki R, Goldberg Y, Agranyoni O, et al. Exercise intensity- dependent immunomodulatory effects on encephalomyelitis. Ann Clin Transl Neurol. (2019) 6:1647–58. doi: 10.1002/acn3.50859
58. Xie Y, Li Z, Wang Y, Xue X, Ma W, Zhang Y, et al. Effects of moderate- versus high- intensity swimming training on inflammatory and CD4+ T cell subset profiles in experimental autoimmune encephalomyelitis mice. J Neuroimmunol. (2019) 328:60–7. doi: 10.1016/j.jneuroim.2018.12.005
59. Bernardes D, Oliveira, ALR. Comprehensive catwalk gait analysis in a chronic model of multiple sclerosis subjected to treadmill exercise training. BMC Neurol. (2017) 17:160. doi: 10.1186/s12883-017-0941-z
60. Bernardes D, Brambilla R, Bracchi-Ricard V, Karmally S, Dellarole A, Carvalho-Tavares J, et al. Prior regular exercise improves clinical outcome and reduces demyelination and axonal injury in experimental autoimmune encephalomyelitis. J Neurochem. (2016) 136:63–73. doi: 10.1111/jnc.13354
61. Benson C, Paylor JW, Tenorio G, Winship I, Baker G, Kerr BJ. Voluntary wheel running delays disease onset and reduces pain hypersensitivity in early experimental autoimmune encephalomyelitis (EAE). Exp Neurol. (2015) 271:279–90. doi: 10.1016/j.expneurol.2015.05.017
62. Pryor W, Freeman K, Larson R, Edwards G, White LJ. Chronic exercise confers neuroprotection in experimental autoimmune encephalomyelitis. J Neurosci Res. (2015) 93:697–706. doi: 10.1002/jnr.23528
63. Rossi S, Furlan R, De Chiara V, Musella A, Lo Giudice T, Mataluni G, et al. Exercise attenuates the clinical, synaptic and dendritic abnormalities of experimental autoimmune encephalomyelitis. Neurobiol Dis. (2009) 36:51–9. doi: 10.1016/j.nbd.2009.06.013
64. Bernardes D, Oliveira-Lima OC, da Silva TV, Faraco CCF, Leite HR, Juliano MA, et al. Differential brain and spinal cord cytokine and BDNF levels in experimental autoimmune encephalomyelitis are modulated by prior and regular exercise. J Neuroimmunol. (2013) 264:24–34. doi: 10.1016/j.jneuroim.2013.08.014
65. Einstein O, Fainstein N, Touloumi O, Lagoudaki R, Hanya E, Grigoriadis N, et al. Exercise training attenuates experimental autoimmune encephalomyelitis by peripheral immunomodulation rather than direct neuroprotection. Exp Neurol. (2018) 299:56–64. doi: 10.1016/j.expneurol.2017.10.008
66. Hamdi L, Gabat HN, Goldberg Y, Fainstein N, Ben-Hur T, Einstein O. Exercise training alters autoimmune cell invasion into the brain in autoimmune encephalomyelitis. Ann Clin Transl Neurol. (2022) 9:1792–806. doi: 10.1002/acn3.51677
67. Nazari M, Kordi M, Minasian V, Saffar Kohneh Quchan A. Ameliorating effect of 6-week swimming exercise on mice with experimental autoimmune encephalomyelitis (EAE) by reducing fetuin-A and increasing AMPK & NAD levels in liver tissue. Iran J Basic Med Sci. (2022) 25:1016–20. doi: 10.22038/IJBMS.2022.65117.14335
68. Edwards T, Michelsen A, Fakolade A, Dalgas U, Pilutti LA. Exercise training improves participation in persons with multiple sclerosis: a systematic review and meta-analysis. J Sport Health Sci. (2022) 11:393–402. doi: 10.1016/j.jshs.2021.07.007
69. Taul-Madsen L, Connolly L, Dennett R, Freeman J, Dalgas U, Hvid LG. Is aerobic or resistance training the most effective exercise modality for improving lower extremity physical function and perceived fatigue in people with multiple sclerosis? A systematic review and meta-analysis. Arch Phys Med Rehabil. (2021) 102:2032–48. doi: 10.1016/j.apmr.2021.03.026
70. Reina-Gutiérrez S, Cavero-Redondo I, Martínez-Vizcaíno V, Núñez de Arenas-Arroyo S, López-Muñoz P, Álvarez-Bueno C, et al. The type of exercise most beneficial for quality of life in people with multiple sclerosis: a network meta-analysis. Ann Phys Rehabil Med. (2021) 65:1010578. doi: 10.1016/j.rehab.2021.101578
71. Gharakhanlou R, Wesselmann L, Rademacher A, Lampit A, Negaresh R, Kaviani M, et al. Exercise training and cognitive performance in persons with multiple sclerosis: a systematic review and multilevel meta-analysis of clinical trials. Multiple Sclerosis J. (2021) 27:1977–93. doi: 10.1177/1352458520917935
Keywords: multiple sclerosis, exercise, experimental autoimmune encephalomyelitis, translational research, biomarkers
Citation: Martin S-J and Schneider R (2023) Multiple sclerosis and exercise—A disease-modifying intervention of mice or men? Front. Neurol. 14:1190208. doi: 10.3389/fneur.2023.1190208
Received: 20 March 2023; Accepted: 19 September 2023;
Published: 10 October 2023.
Edited by:
Letizia Leocani, San Raffaele Hospital (IRCCS), ItalyReviewed by:
Mario Stampanoni Bassi, Mediterranean Neurological Institute Neuromed (IRCCS), ItalyCopyright © 2023 Martin and Schneider. This is an open-access article distributed under the terms of the Creative Commons Attribution License (CC BY). The use, distribution or reproduction in other forums is permitted, provided the original author(s) and the copyright owner(s) are credited and that the original publication in this journal is cited, in accordance with accepted academic practice. No use, distribution or reproduction is permitted which does not comply with these terms.
*Correspondence: Sarah-Jane Martin, sarahjane.martin@glasgow.ac.uk