- Department of Neurology, King Edward Medical University/Mayo Hospital, Lahore, Pakistan
Objective: Since its discovery as an antioxidant, melatonin has been increasingly recognized for its therapeutic potential beyond sleep disturbances in neurodegenerative disorders. This study aims to evaluate efficacy of various melatonin doses, treatment durations, and formulations, in alleviating motor symptoms and sleep disturbances in Parkinson’s disease, the second most common neurodegenerative disorder worldwide.
Methods: PubMed, Cochrane Library, ClinicalTrials.gov and other databases were systematically searched to retrieve randomized controlled trials (RCTs) administrating melatonin to Parkinson’s disease patients until June 10th, 2023. Outcomes including Unified Parkinson Disease Rating Scale (UPDRS) scores and Pittsburgh Sleep Quality Index (PSQI) scores, were pooled and reported as mean differences (MD) with 95% confidence intervals (CIs). Meta-analysis was performed using an inverse variance random-effects model in Review Manager 5.4 software. Trial Sequential Analysis was performed to avoid false-positive results from random errors.
Results: Five RCTs with a total of 155 patients were included. Statistically significant reductions in UPDRS total scores were observed in groups receiving Melatonin ≥10 mg/day (MD = −11.35, 95% CI: −22.35 to −0.35, I2 = 0%, p = 0.04) and immediate release formulations (MD = −11.35, 95% CI: −22.35 to −0.35, I2 = 0%, p = 0.04). No significant effects on individual UPDRS II, III, and IV scores were observed, regardless of melatonin dosage and treatment duration. Moreover, significant improvements in PSQI scores were observed with only immediate-release melatonin formulations (MD = −2.86, 95% CI: −4.74 to −0.97, I2 = 0%, p = 0.003).
Conclusion: Melatonin ≥10 mg/day for a minimum duration of ≥12 weeks in immediate-release formulations consistently demonstrated significant therapeutic potential in improving motor symptom and sleep disturbances in Parkinson disease. However, further trials are warranted to investigate its impact when initiated early in the disease course to fully explore its true therapeutic potential.
Systematic review registration: Unique identifier: CRD42023427491 (PROSPERO).
1. Introduction
N-Acetyl-5-methoxytryptamine, commonly known as melatonin, is a neurohormone synthesized and secreted by the pineal gland (1, 2). While renowned for its pivotal role in regulating circadian cycles (3) and sleep–wake harmony (4), its identification as a powerful direct free radical scavenger (5–7) and an indirect antioxidant (8–15) have emerged as an unexpected revelation. Since its discovery as an antioxidant, melatonin has been increasingly recognized for its therapeutic potential beyond sleep disturbances across a wide range of medical conditions, specifically age-related neurodegenerative conditions (16–20). These neurodegenerative disorders, often driven by free radical-mediated apoptosis (21), present a challenge to treat due to limited blood–brain barrier penetration by various antioxidants. Exogenous melatonin, known for its ability to readily cross the blood–brain barrier (22, 23) coupled with its free radical scavenging and antioxidant properties, positions itself as a promising therapeutic agent for these conditions (24). While melatonin holds value for managing various neurodegenerative conditions, this article focuses only on its role in Parkinson’s disease, the second most common neurodegenerative disease worldwide (25).
Parkinson’s disease is a movement disorder characterized by progressive loss of dopaminergic neurons in substantia nigra pars compacta (25). Recent developments emphasize that, in addition to α-synuclein-induced cytotoxicity (26), increased free radical production, oxidative stress and neuroinflammation also contribute to this neuronal degeneration (27–30). Melatonin’s ability to inhibit α-synuclein-induced cytotoxicity (31), along with its free radical-neutralizing and antioxidant properties (32–34) offer potential for attenuating this neuronal loss. Furthermore, sleep disturbances, the most disabling non-motor symptoms in Parkinson disease (35, 36), are associated with reduced expression of melatonin receptors in the substantia nigra (37). Additionally, a drop in endogenous melatonin concentrations is also observed following Antiparkinson therapy (38). Exogenous melatonin can effectively counteract these reductions, and its sleep-inducing effects (4, 39, 40), along with its safe profile, make it superior for addressing sleep disturbances compared to alternatives like Clonazepam, with undesirable hangover effects (41). Although, ample experimental evidence (18, 19, 31, 33, 42) supports melatonin use in Parkinson’s disease, further evaluation is necessary to determine its real-world clinical applications.
Randomized controlled trials (RCTs) exploring melatonin’s efficacy in Parkinson’s disease have yielded inconsistent results, warranting a meta-analysis. However, only existing meta-analysis (43) evaluates melatonin exclusively for sleep disorders in Parkinson’s disease. It also fails to stratify the analysis by doses, treatment durations, and melatonin formulations, such as immediate-release (half-life: 20–30 min) and prolonged-release (half-life: 3–4 h) (44). This limited approach emphasizes the need for a newer updated and a more thorough meta-analysis. Therefore, this analysis aims to evaluate efficacy of various melatonin doses, treatment durations, and formulations compared to a placebo, in alleviating both motor symptoms and sleep disturbances in Parkinson’s disease, utilizing the standard Unified Parkinson Disease Rating Scale (UPDRS) (45, 46) and the Pittsburgh Sleep Quality Index (PSQI) (47).
2. Methods
This meta-analysis adheres to Preferred Reporting Items for Systematic Reviews and Meta-Analyzes (PRISMA) guidelines (48) and is registered with International Prospective Register of Systematic Reviews, PROSPERO (CRD42023427491).
2.1. Literature search
A comprehensive literature search was conducted from 1 June 2023 to 10 June 2023. Following databases were searched: MEDLINE (via PubMed), Cochrane Controlled Register of Trials (CENTRAL) (via Cochrane Library), ResearchGate, Google Scholar, Europe PMC, ClinicalTrials.gov, WHO ICTRP, and SciELO, without restrictions on language or publication period. Search terms included “Melatonin,” “melatonin therapy,” “melatonin supplement,” “Parkinson’s disorder,” and ‘Parkinsonism.’ among others. The detailed search strategy can be found in Supplementary Table S1. Additionally, the reference lists of previous meta-analyzes and included trials were manually screened to minimize the possibility of overlooking relevant studies.
2.2. Eligibility criteria and study selection
Studies were deemed eligible if they confirmed the following criteria: (1) Population: Participants were adults (age > 18), diagnosed with Parkinson’s disease using a recognized criterion (49); (2) Intervention: Experimental group received melatonin; (3) Comparator: Control group received a placebo; (4) Outcomes: Motor symptoms were assessed using the Unified Parkinson Disease Rating Scale (UPDRS) (45), or sleep quality was assessed using the Pittsburgh Sleep Quality Index (PSQI) (47); and (5) Study design: Either parallel-group RCTs or cross-over RCTs that reported pre-cross-over results to ensure the absence of residual effects from previous interventions. Exclusion criteria comprised: (1) Animal studies, case reports, nonrandomized trials or observational studies; (2) Trials with control group receiving drugs other than a placebo; and (3) Trials lacking eligible outcome measurements.
2.3. Data extraction
Electronic search results were imported into Mendeley Desktop. Two investigators (H and Z), blinded to each other’s decision, screened records independently. Redundant and unrelated records were removed by reviewing titles and abstracts. Full texts of remaining records were assessed for eligibility. Investigators used a pre-designed data collection sheet to extract: (1) Baseline characteristics; (2) UPDRS subscale and total scores; and (3) PSQI scores. Continuous variables were recorded as means with standard deviations (SDs), and when medians and ranges were reported, approximations were made based on Wan and Luo’s methods (50, 51). Corresponding authors were contacted, if necessary, to obtain missing data or additional details.
2.4. Risk of bias assessment in individual studies
The revised Cochrane “Risk of Bias” tool for RCTs (RoB 2.0) (52) was used to assess bias in individual studies. Articles were evaluated across five domains: randomization process, deviations from intended interventions, missing outcome data, outcome measurement and selection of reported result. Each item was categorized as low, some concerns, or high risk. Disagreements were resolved through discussion and reasoning, with a final decision made by a third independent reviewer (DS) if needed.
2.5. Confidence in cumulative evidence
Quality of evidence was assessed according to GRADE (grading of recommendations assessment, development and evaluation) approach using GRADEpro GDT software (53). Evidence was graded as high, moderate, low, or very low based on the proximity of true effect to the estimated effect. High grade indicates close proximity, moderate indicates likelihood of proximity with some possibility of difference, low indicates likelihood of potential difference, and very low indicates substantial difference.
2.6. Statistical analyzes
Measurements (UPDRS and PQSI scores) were treated as continuous outcomes. Mean changes were expressed as mean difference (MD) at a 95% confidence interval. Meta-analysis was performed using an inverse variance random-effects model. Subgroup analyzes was based on treatment duration, melatonin dose, and formulation. Statistical analysis was performed using Review Manager (RevMan, Version 5.4). Heterogeneity and variability were assessed using Chi2 and I2 statistics, with an I2 value above 50% indicating significant heterogeneity. To explore the causes of heterogeneity, a leave-one-out sensitivity analysis was performed. Due to small number of eligible studies (<10), funnel plots to assess publication bias could not be constructed (54). Still, Trial Sequential Analysis was employed to eliminate false-positive results caused by random errors (55).
3. Results
3.1. Study selection
An in-depth database search yielded 1,602 records, while 2 articles were identified through additional sources. After removing duplicates (n = 36), primary screening excluded 1,557. Full-text screening of the remaining records, in accordance with inclusion criteria, resulted in the selection 5 RCTs for quantitative synthesis. The PRISMA flowchart is summarized in Figure 1.
3.2. Study and patient characteristics
Extensive literature review identified 5 RCTs spanning from 2007 to 2020 (56–60). Pooled sample included 155 patients, 75 (48.38%) in melatonin group and 80 (51.61%) in placebo group. Parkinson’s disease diagnosis was based on the UK Parkinson’s Disease Society Brain Bank criteria (49), except in one study (59) that used presence of at least 2 of the following: tremor, rigidity, and/or akinesia. In each trial different melatonin doses were used. Two trials (58,60) used prolonged-release melatonin (PRM), while, the rest employed immediate-release formulations. Trial durations also varied, two (59, 60) lasted 4 weeks, two (57, 58) lasted 12 weeks, and one (56) lasted 1 year.
UPDRS and PQSI scores were reported inconsistently. Across all RCTs, only 1 cohort (57) reported UPDRS I score, 2 cohorts (57, 59) reported UPDRS II scores, 4 cohorts (57–60) reported UPDRS III scores, 2 cohorts (57, 59) reported UPDRS IV scores, 3 cohorts (56–58) reported total UPDRS scores, and 4 cohorts (57–60) reported PQSI scores. Table 1 summarizes these characteristics of included RCTs.
3.3. Quality assessment of included studies
No trial was judged to have high risk of bias. Two trials (56, 59) raised concerns about overall bias due to insufficient explanation of randomization and allocation concealment methods, as well as inadequate blinding of outcome assessors. One trial (60) had concerns regarding insufficient blinding of carers and intervention providers (Figure 2). The remaining trials had low risk of bias in all domains.
3.4. Outcome
3.4.1. Parkinson disease severity
Among the 5 included trials, data on UPDRS total scores were available in 3 trials involving 93 patients (56–58). Significant reductions in UPDRS total scores were observed in groups receiving Melatonin ≥10 mg/day (MD = -11.35, 95% CI: −22.35 to −0.35, I2 = 0%, p = 0.04) and immediate release formulation (MD = -11.35, 95% CI: −22.35 to −0.35, I2 = 0%, p = 0.04). However, no significant reduction and high heterogeneity was seen with Melatonin ≥4 mg/day (MD = −3.27, 95% CI: −19.15 to 12.61, I2 = 63%, p = 0.69, Figure 3). Sensitivity analysis in the Melatonin ≥4 mg/day group (I2 = 87%) eliminated heterogeneity upon removing the Gilat et al. trial from the analysis with the pooled estimate favoring melatonin significantly (MD = -11.35, 95% CI: −22.35 to −0.35, I2 = 0%, p = 0.04) (Supplementary Table S2).
UPDRS subscale score were reported in 4 trials encompassing 132 patients (57–60) and analysis revealed that melatonin had no significant effect on individual UPDRS II, III, and IV scores, regardless of dosage and treatment duration, although a marginal reduction in UPDRS IV scores was observed favoring melatonin. Mean difference for each subscales were 0.43 (95% CI: −5.14 to 5.99, I2 = 0%, p = 0.88), 1.11 (95% CI: −3.08 to 5.53, I2 = 0%, p = 0.58) and − 0.74 (95% CI: −3.06 to 1.57, I2 = 0%, p = 0.53), respectively (Figure 4).
3.4.2. Sleep quality
Subjective sleep quality was assessed using the PQSI scale in four trials including a total of 132 patients (57–60). No significant improvement in PQSI scores was observed in groups receiving melatonin for ≥4 weeks (57–60) (MD = −1.47, 95% CI: −3.84 to 0.90, I2 = 72%, p = 0.22) and ≥ 12 weeks (57, 58) (MD = −0.97, 95% CI: −4.59 to 2.65, I2 = 89%, p = 0.60, Figure 5). Heterogeneity in the ≥4 weeks cohort (I2 = 72%) was mitigated by the exclusion of the Gilat et al. trial, significantly altering the results in favor of melatonin (MD = -2.72, 95% CI: −4.34 to −1.09, I2 = 0%, p = 0.001) (Supplementary Table S3). However. inclusion of only two studies in ≥12 weeks cohort (I2 = 89%) limited its sensitivity analysis.
Further analysis based on dosage revealed no significant improvements with Melatonin ≥3 mg/day (57–59) (MD = −1.28, 95% CI: −4.26 to 1.70, I2 = 79%, p = 0.60) and Melatonin ≥4 mg/day (57, 58) (MD = −0.97, 95% CI: −4.59 to 2.65, I2 = 89%, p = 0.60, Figure 5). Sensitivity analysis in Melatonin ≥3 mg/day cohort (I2 = 79%) eliminated heterogeneity upon removing Gilat et al. trial, and the results significantly favored melatonin (MD = -2.86, 95% CI: −4.74 to −0.97, I2 = 0%, p = 0.003). On the other hand, sensitivity analysis on Melatonin ≥4 mg/day cohort (I2 = 89%), which included only two studies, was not performed.
In an exclusive analysis of trials utilizing immediate-release formulations (57, 59) melatonin showed statistically significant improvement in PQSI scores (MD = −2.86, 95% CI: −4.74 to −0.97, I2 = 0%, p = 0.003, Figure 5). In contrast, analysis of trials using prolonged-release formulations (58, 60) demonstrated no statistically significant improvement and high heterogeneity (MD = −0.39, 95% CI: −3.34 to −2.57, I2 = 67%, p = 0.80), however, the inclusion of only two studies limited the scope of sensitivity analysis.
3.5. Trial sequence analysis
Trial Sequential Analysis on UPDRS total scores in Melatonin ≥10 mg/day group (Figure 6A) revealed that the cumulative z-score line did not cross any boundaries and remained within the “non-significant” zone, indicating insufficient statistical evidence. Additionally, Trial Sequential Analysis on PQSI scores in immediate release formulation group (Figure 6B) showed that cumulated z-score line did cross the benefit boundary, but the required information size was not reached. Therefore, despite observation of significant results, it is not yet appropriate to make direct clinical recommendations without further investigation.
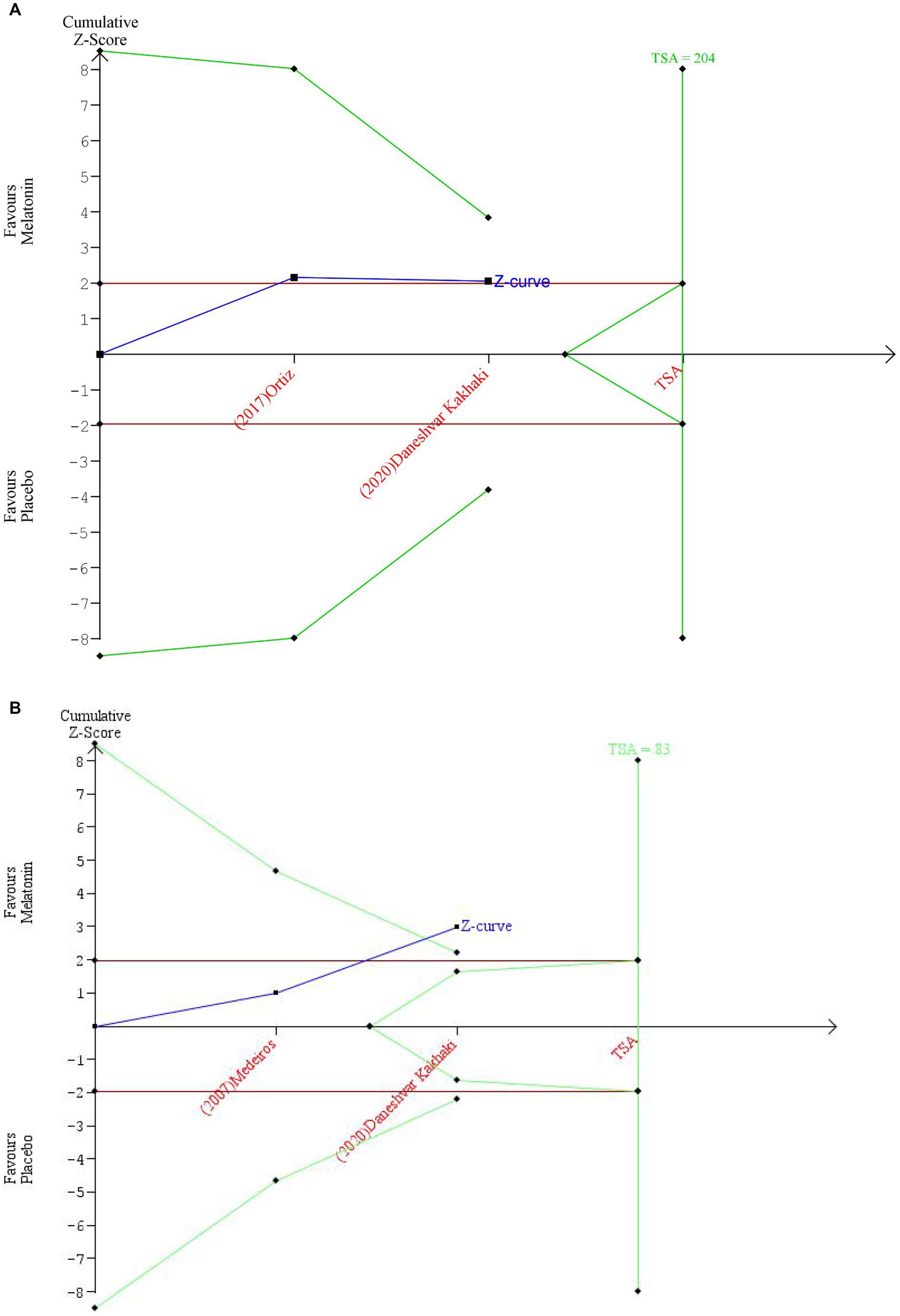
Figure 6. (A) Trial sequential analysis of the efficacy of melatonin vs. placebo on severity of Parkinson’s disease symptoms. (B) Trial sequential analysis of the efficacy of melatonin vs. placebo on sleep quality in Parkinson’s disease.
3.6. Assessment of certainty of evidence
The GRADE assessment revealed low quality of evidence for UPDRS total scores and moderate quality of evidence for UPDRS II scores. However, evidence for UPDRS III, UPDRS IV, and PQSI scores was of high quality. Factors contributing to downgrading of evidence included high heterogeneity, wide confidence intervals, imprecision due to unavailable SDs, and insufficient directionality. The detailed GRADE summary of findings is provided in Supplementary Table S4.
4. Discussion
Our comprehensive meta-analysis indicates that melatonin therapy merits serious consideration for Parkinson’s disease. Dosages of ≥10 mg/day for a minimum duration of ≥12 weeks in immediate-release formulations, have consistently demonstrated significant potential. These findings reinforce the rationale of our study, suggesting that melatonin, when used in specific treatment regimens, may alleviate symptom severity and reduce sleep disturbances in Parkinson’s disease.
To the best of our knowledge, this is the first meta-analysis to date that evaluates efficacy of melatonin on motor symptoms in Parkinson disease using the standard Unified Parkinson Disease Rating Scale (UPDRS) (45). Analysis of UPDRS total scores indicate that after at least 12 weeks of treatment, melatonin significantly impacts Parkinson’s disease progression when doses of ≥10 mg/day are used. This trend of enhanced melatonin efficacy with higher doses at longer treatment durations has been consistently reported in trails comparing 50 mg/day melatonin with 0.25 mg (61, 62) and 50 mg/day melatonin with 5 mg (63) for various outcomes. Furthermore, trials included in our analysis also reported significant results with 50 mg/day melatonin for 1 year (56) and non-significant results with 10 mg/day or 4 mg/day melatonin for 12 weeks (57, 58). These findings, supported further by melatonin’s ability to exhibit virtually no acute or chronic toxicity (64, 65), strongly advocate its long-term utilization at higher doses as a safe choice.
Furthermore, it is important to address the observed heterogeneity in treatment response at different melatonin doses. Analysis of UPDRS total scores in groups receiving melatonin ≥10 mg/day revealed significant results with no heterogeneity (I2 = 0%). However, including studies with <10 mg doses increased heterogeneity substantially (I2 = 63%). Potential contributors may include dose-dependent and formulation-dependent pharmacokinetics of melatonin, as low dose studies used prolonged release formulations and high dose studies used immediate release formulations (44). Moreover, variations in treatment duration could also play a role, as longer durations with higher doses consistently demonstrated enhanced efficacy in previous studies (56–58).
Apart from dosage and duration, a crucial difference among these trails was the timing of melatonin intervention. In the significant study (56), melatonin was initiated in newly diagnosed patients immediately after observing a satisfactory response to anti-Parkinson’s therapy. In contrast, patients in non-significant studies (57, 58) had mean disease duration of 5.7 ± 1.9 and 5.0 ± 3.9 years respectively, indicating significant pre-existing damage at the time of melatonin introduction. This selection of patients with longer disease duration and introduction of melatonin at a later stage reveal an inherent flaw, as starting melatonin before neuronal loss is crucial for its free radical scavenging and antioxidant properties (18, 19, 31, 33, 42) to effectively prevent degeneration and reduce symptom severity in Parkinson’s disease. In addition, a sub-analysis focusing on only immediate-release formulations, also yielded significant results, however, use of prolonged-release formulation in only one study (58) hinders appropriate comparisons.
Taking a step further, we analyzed melatonin’s impact on each UPDRS subscale. Unfortunately, only one trial (57) reported UPDRS I score, rendering further analysis unfeasible, nevertheless, the results were statistically significant. Subsequent analysis indicated that melatonin has no significant effect on individual UPDRS II, III, and IV subscale scores, but it is also important to consider that these trials (57–60), included patients with average disease duration of >5 years, indicating significant pre-existing neuronal loss. Despite this, a slight reduction in UPDRS IV scores favored melatonin, possibly due to its ability to enhance efficacy and reduce side effects of other drugs through its anti-oxidant properties (15). This finding suggests that melatonin’s influence on UPDRS total scores may stem from its ability to counteract adverse treatment effects of Anti-Parkinson therapy (dyskinesias, off duration, nausea, vomiting, anorexia, insomnia, hypersomnia, orthostasis) (66), thereby enhancing overall treatment tolerability and quality of life in patients with Parkinson’s disease.
Lastly, we analyzed melatonin’s efficacy in improving sleep quality using the Pittsburgh Sleep Quality Index (PSQI) (47). Analysis revealed that melatonin will significantly improve PQSI scores only if immediate release formulations were used. Treatment durations of 4 and 12 weeks, doses of ≥3 and ≥ 4 mg/day, and prolonged-release formulations showed no significant impact, however, a significant heterogeneity was observed in these sub-analyzes. Excluding the Gilat et al. trial eliminated this heterogeneity, and the pooled estimates than favored melatonin for ≥4 weeks cohort (p = 0.001) and Melatonin ≥3 mg/day cohort (p = 0.003). This heterogeneity and its elimination can be attributed to the use of prolonged-release melatonin (PRM) in the excluded trial. PRM has been favored for sleep disorders by numerous case reports and small open label trials (67–73) as it mimics a more physiologic release (74), but has consistently failed to demonstrate a significant potential. It is also worth mentioning here that in our study, UPDRS scores analysis favored higher melatonin doses for longer durations, while PQSI scores analysis did not show a similar trend. This disparity can be explained by notorious sleep-inducing effects of melatonin, which are observed even at doses as low as 3 mg/day (75), and suggest melatonin’s pleiotropy (bifunctional pharmacology) in Parkinson’s disease.
In our analysis, we observed that melatonin supplementation in Parkinson’s disease demonstrated positive effects on both motor symptoms and sleep disturbances. However, sleep alone can directly influence motor symptoms too. The “sleep benefit,” an improvement of motor functions upon awakening, is seen in more than 40% of Parkinson’s disease patients, and is attributed to improved dopaminergic function as a result of increased storage of dopamine in nigrostriatal terminals during sleep (76). Hence, melatonin can indirectly lead to an improvement in motor symptoms through sleep improvement. This effect appears to be unrelated to its antioxidant properties, indicating a multifaceted potential for melatonin in Parkinson’s disease treatment.
We believe that our findings open up new avenues for clinicians and researchers to debate and explore in this topic. As far as we know, a systematic categorization of melatonin into dose groups for motor symptoms and sleep disturbances in Parkinson’s disease has not been conducted before, and is a defining feature of this meta-analysis. Furthermore, it strongly recommends the use of long-term, high-dose immediate-release melatonin in future investigations and emphasizes the significance of selecting patients with shorter disease duration and initiating melatonin early to fully explore its true therapeutic potential.
4.1. Limitations
Limitations of this meta-analysis stem from scarcity of available data, primarily due to the novelty of this topic. Only five RCTs, each with a small sample size, met the inclusion criteria and data from crossover RCTs were excluded due to lack of pre-crossover result reporting. Out of the 5 included trials, only 3 trials reported UPDRS total scores, thus highlighting the need of standardized reporting in future trials. Inconsistencies in reporting UPDRS scores and use of estimations for missing means and SDs in some studies further contribute to its limitations. Moreover, included studies only reported UPDRS score for motor outcomes; data on DAT imaging and other motor outcome assessment scales like H-Y stage were not available. Lastly, considerable heterogeneity was observed, particularly due to study by Gilat et al. While the exclusion of this outlier effectively eliminated heterogeneity, it is important to consider these limitations in the interpretation of findings.
4.2. Conclusion
Melatonin at dose of ≥10 mg/day for a minimum duration of ≥12 weeks and immediate-release formulations consistently demonstrated significant potential. Given its extensive range of effects, melatonin holds promise in improving both motor symptom and sleep disturbances in Parkinson disease. However, further trials are warranted to investigate its impact when initiated early in the disease course.
Data availability statement
The original contributions presented in the study are included in the article/Supplementary material, further inquiries can be directed to the corresponding author.
Author contributions
SI: Conceptualization, Methodology, Supervision, Validation, Visualization, Writing – review & editing. HS: Data curation, Formal analysis, Investigation, Resources, Software, Writing – original draft. Zainab: Data curation, Formal analysis, Investigation, Resources, Software, Writing – original draft.
Funding
The author(s) declare that no financial support was received for the research, authorship, and/or publication of this article.
Conflict of interest
The authors declare that the research was conducted in the absence of any commercial or financial relationships that could be construed as a potential conflict of interest.
Publisher’s note
All claims expressed in this article are solely those of the authors and do not necessarily represent those of their affiliated organizations, or those of the publisher, the editors and the reviewers. Any product that may be evaluated in this article, or claim that may be made by its manufacturer, is not guaranteed or endorsed by the publisher.
Supplementary material
The Supplementary material for this article can be found online at: https://www.frontiersin.org/articles/10.3389/fneur.2023.1265789/full#supplementary-material
References
1. Hickman, AB, Klein, DC, and Dyda, F. Melatonin biosynthesis: the structure of serotonin N-acetyltransferase at 2.5 Å resolution suggests a catalytic mechanism. Mol Cell. (1999) 3:23–32. doi: 10.1016/S1097-2765(00)80171-9
2. Donohue, SJ, Roseboom, PH, Illnerova, H, Weller, JL, and Klein, DC. Human hydroxyindole-O-methyltransferase: presence of LINE-1 fragment in a cDNA clone and pineal mRNA. DNA Cell Biol. (1993) 12:715–27. doi: 10.1089/dna.1993.12.715
3. Kennaway, DJ, and Wright, H. Melatonin and circadian rhythms. Curr Top Med Chem. (2002) 2:199–209. doi: 10.2174/1568026023394380
4. Arendt, J, and Skene, DJ. Melatonin as a chronobiotic. Sleep Med Rev. (2005) 9:25–39. doi: 10.1016/j.smrv.2004.05.002
5. Tan, DX, Reiter, RJ, Manchester, LC, Yan, MT, El-Sawi, M, Sainz, RM, et al. Chemical and physical properties and potential mechanisms: melatonin as a broad spectrum antioxidant and free radical scavenger. Curr Top Med Chem. (2002) 2:181–97. doi: 10.2174/1568026023394443
6. Tan, DX, Hardeland, R, Manchester, LC, Poeggeler, B, Lopez-Burillo, S, Mayo, JC, et al. Mechanistic and comparative studies of melatonin and classic antioxidants in terms of their interactions with the ABTS cation radical. J Pineal Res. (2003) 34:249–59. doi: 10.1034/j.1600-079X.2003.00037.x
7. Castroviejo, D, Escames, G, Carazo, A, León, J, Khaldy, H, and Reiter, RJ. Melatonin, mitochondrial homeostasis and mitochondrial-related diseases. Curr Top Med Chem. (2002) 2:133–51. doi: 10.2174/1568026023394344
8. Hardeland, R, Reiter, RJ, Poeggeler, B, and Tan, DX. The significance of the metabolism of the neurohormone melatonin: antioxidative protection and formation of bioactive substances. Neurosci Biobehav Rev. (1993) 17:347–57. doi: 10.1016/S0149-7634(05)80016-8
9. Reiter, RJ, Tan, DX, Osuna, C, and Gitto, E. Actions of melatonin in the reduction of oxidative stress. A review. J Biomed Sci. (2000) 7:444–58. doi: 10.1007/BF02253360
10. Rodriguez, C, Mayo, JC, Sainz, RM, Antolín, I, Herrera, F, Martín, V, et al. Regulation of antioxidant enzymes: a significant role for melatonin. J Pineal Res. (2004) 36:1–9. doi: 10.1046/j.1600-079X.2003.00092.x
11. Urata, Y, Honma, S, Goto, S, Todoroki, S, Iida, T, Cho, S, et al. Melatonin induces γ-glutamylcysteine synthetase mediated by activator protein-1 in human vascular endothelial cells. Free Radic Biol Med. (1999) 27:838–47. doi: 10.1016/S0891-5849(99)00131-8
12. Gitto, E, Tan, DX, Reiter, RJ, Karbownik, M, Manchester, LC, Cuzzocrea, S, et al. Individual and synergistic antioxidative actions of melatonin: studies with vitamin E, vitamin C, glutathione and desferrrioxamine (desferoxamine) in rat liver homogenates. J Pharm Pharmacol. (2001) 53:1393–401. doi: 10.1211/0022357011777747
13. Mayo, JC, Sainz, RM, Antolin, I, Herrera, F, Martin, V, and Rodriguez, C. Melatonin regulation of antioxidant enzyme gene expression. Cell Mol Life Sci. (2002) 59:1706–13. doi: 10.1007/PL00012498
14. Mayo, JC, Tan, DX, Sainz, RM, Lopez-Burillo, S, and Reiter, RJ. Oxidative damage to catalase induced by peroxyl radicals: functional protection by melatonin and other antioxidants. Free Radic Res. (2003) 37:543–53. doi: 10.1080/1071576031000083206
15. Reiter, RJ, Tan, DX, Sainz, RM, Mayo, JC, and Lopez-Burillo, S. Melatonin: reducing the toxicity and increasing the efficacy of drugs. J Pharm Pharmacol. (2002) 54:1299–321. doi: 10.1211/002235702760345374
16. Pappolla, MA, Chyan, YJ, Poeggeler, B, Frangione, B, Wilson, G, Ghiso, J, et al. An assessment of the antioxidant and the antiamyloidogenic properties of melatonin: implications for Alzheimer's disease. J Neural Transm. (2000) 107:203–31. doi: 10.1007/s007020050018
17. Brusco, LI, Márquez, M, and Cardinali, DP. Monozygotic twins with Alzheimer's disease treated with melatonin: case report. J Pineal Res. (1998) 25:260–3. doi: 10.1111/j.1600-079X.1998.tb00396.x
18. Antolín, I, Mayo, JC, Sainz, RM, del Brío, MA, Herrera, F, Martín, V, et al. Protective effect of melatonin in a chronic experimental model of Parkinson’s disease. Brain Res. (2002) 943:163–73. doi: 10.1016/S0006-8993(02)02551-9
19. Wilkinson, RG. Health, hierarchy, and social anxiety. Ann N Y Acad Sci. (1999) 896:48–63. doi: 10.1111/j.1749-6632.1999.tb08104.x
20. Cohen-Mansfield, J, Garfinkel, D, and Lipson, S. Melatonin for treatment of sundowning in elderly persons with dementia–a preliminary study. Arch Gerontol Geriatr. (2000) 31:65–76. doi: 10.1016/S0167-4943(00)00068-6
21. Uttara, BAV, Singh, A, Zamboni, P, and Mahajan, R. Oxidative stress and neurodegenerative diseases: a review of upstream and downstream antioxidant therapeutic options. Curr Neuropharmacol. (2009) 7:65–74. doi: 10.2174/157015909787602823
22. Reiter, RJ, Tan, DX, Manchester, LC, and Qi, W. Chemical and biological aspects of melatonin as a direct free radical scavenger and as an indirect antioxidant. Biol Signals Recept. (2000) 9:160–71.
23. Reiter, RJ, Tan, DX, Manchester, LC, Terron, MP, Flores, LJ, and Koppisepi, S. Medical implications of melatonin: receptor-mediated and receptor-independent actions. Adv Med Sci. (2007) 52:11–28.
24. Reiter, RJ. Oxidative damage in the central nervous system: protection by melatonin. Prog Neurobiol. (1998) 56:359–84. doi: 10.1016/S0301-0082(98)00052-5
25. Dorsey, ER, and Bloem, BR. The Parkinson pandemic—a call to action. JAMA Neurol. (2018) 75:9–10. doi: 10.1001/jamaneurol.2017.3299
26. Bridi, JC, and Hirth, F. Mechanisms of α-synuclein induced synaptopathy in Parkinson's disease. Front Neurosci. (2018) 12:80. doi: 10.3389/fnins.2018.00080
27. Venkatesh Gobi, V, Rajasankar, S, Ramkumar, M, Dhanalakshmi, C, Manivasagam, T, Justin Thenmozhi, A, et al. Agaricus blazei extract attenuates rotenone-induced apoptosis through its mitochondrial protective and antioxidant properties in SH-SY5Y neuroblastoma cells. Nutr Neurosci. (2018) 21:97–107. doi: 10.1080/1028415X.2016.1222332
28. Zündorf, G, and Reiser, G. Calcium dysregulation and homeostasis of neural calcium in the molecular mechanisms of neurodegenerative diseases provide multiple targets for neuroprotection. Antioxid Redox Signal. (2011) 14:1275–88. doi: 10.1089/ars.2010.3359
29. Taylor, JM, Main, BS, and Crack, PJ. Neuroinflammation and oxidative stress: co-conspirators in the pathology of Parkinson’s disease. Neurochem Int. (2013) 62:803–19. doi: 10.1016/j.neuint.2012.12.016
30. Hritcu, L, and Ciobica, A. Intranigral lipopolysaccharide administration induced behavioral deficits and oxidative stress damage in laboratory rats: relevance for Parkinson's disease. Behav Brain Res. (2013) 253:25–31. doi: 10.1016/j.bbr.2013.07.006
31. Saravanan, KS, Sindhu, KM, and Mohanakumar, KP. Melatonin protects against rotenone-induced oxidative stress in a hemiparkinsonian rat model. J Pineal Res. (2007) 42:247–53. doi: 10.1111/j.1600-079X.2006.00412.x
32. Thomas, B, and Mohanakumar, KP. Melatonin protects against oxidative stress caused by 1-methyl-4-phenyl-1, 2, 3, 6-tetrahydropyridine in the mouse nigrostriatum. J Pineal Res. (2004) 36:25–32. doi: 10.1046/j.1600-079X.2003.00096.x
33. Rasheed, MZ, Andrabi, SS, Salman, M, Tabassum, H, Shaquiquzzaman, M, Parveen, S, et al. Melatonin improves behavioral and biochemical outcomes in a rotenone-induced rat model of Parkinson's disease. J Environ Pathol Toxicol Oncol. (2018) 37:139–50. doi: 10.1615/JEnvironPatholToxicolOncol.2018025666
34. Acuña-Castroviejo, D, Coto-Montes, A, Monti, MG, Ortiz, GG, and Reiter, RJ. Melatonin is protective against MPTP-induced striatal and hippocampal lesions. Life Sci. (1996) 60:PL23–9. doi: 10.1016/s0024-3205(96)00606-6
35. Clarenbach, P. Parkinson's disease and sleep. J Neurol. (2000) 247:IV20–3. doi: 10.1007/PL00007773
36. Oerlemans, WG, and de Weerd, AW. The prevalence of sleep disorders in patients with Parkinson's disease: a self-reported, community-based survey. Sleep Med. (2002) 3:147–9.
37. Adi, N, Mash, DC, Ali, Y, Singer, C, Shehadeh, L, and Papapetropoulos, S. Melatonin MT1 and MT2 receptor expression in Parkinson's disease. Med Sci Monit. (2010) 16:BR61–7.
38. Bordet, R, Devos, D, Brique, S, Touitou, Y, Guieu, JD, Libersa, C, et al. Study of circadian melatonin secretion pattern at different stages of Parkinson's disease. Clin Neuropharmacol. (2003) 26:65–72. doi: 10.1097/00002826-200303000-00005
39. Pandi-Perumal, SR, Zisapel, N, Srinivasan, V, and Cardinali, DP. Melatonin and sleep in aging population. Exp Gerontol. (2005) 40:911–25. doi: 10.1016/j.exger.2005.08.009
40. Campos, FL, da Silva-Júnior, FP, de Bruin, VM, and de Bruin, PF. Melatonin improves sleep in asthma: a randomized, double-blind, placebo-controlled study. Am J Respir Crit Care Med. (2004) 170:947–51. doi: 10.1164/rccm.200404-488OC
41. Shin, C, Park, H, Lee, WW, Kim, HJ, Kim, HJ, and Jeon, B. Clonazepam for probable REM sleep behavior disorder in Parkinson's disease: a randomized placebo-controlled trial. J Neurol Sci. (2019) 401:81–6. doi: 10.1016/j.jns.2019.04.029
42. Paul, R, Phukan, BC, Thenmozhi, AJ, Manivasagam, T, Bhattacharya, P, and Borah, A. Melatonin protects against behavioral deficits, dopamine loss and oxidative stress in homocysteine model of Parkinson's disease. Life Sci. (2018) 192:238–45. doi: 10.1016/j.lfs.2017.11.016
43. Ma, H, Yan, J, Sun, W, Jiang, M, and Zhang, Y. Melatonin treatment for sleep disorders in Parkinson's disease: a meta-analysis and systematic review. Front Aging Neurosci. (2022) 14:784314. doi: 10.3389/fnagi.2022.784314
44. Lyseng-Williamson, KA. Melatonin prolonged release: in the treatment of insomnia in patients aged≥ 55 years. Drugs Aging. (2012) 29:911–23. doi: 10.1007/s40266-012-0018-z
45. Goetz, CG, Tilley, BC, Shaftman, SR, Stebbins, GT, Fahn, S, Martinez-Martin, P, et al. Movement Disorder Society-sponsored revision of the unified Parkinson's disease rating scale (MDS-UPDRS): scale presentation and clinimetric testing results. Mov Disord. (2008) 23:2129–70. doi: 10.1002/mds.22340
46. Ramaker, C, Marinus, J, Stiggelbout, AM, and Van Hilten, BJ. Systematic evaluation of rating scales for impairment and disability in Parkinson's disease. Mov Disord. (2002) 17:867–76. doi: 10.1002/mds.10248
47. Buysse, DJ, Reynolds, CF, Monk, TH, Berman, SR, and Kupfer, DJ. The Pittsburgh sleep quality index: a new instrument for psychiatric practice and research. Psychiatry Res. (1989) 28:193–213. doi: 10.1016/0165-1781(89)90047-4
48. Iversen, HH, Bjertnæs, ØA, and Skudal, KE. Patient evaluation of hospital outcomes: an analysis of open-ended comments from extreme clusters in a national survey. BMJ Open. (2014) 4:e004848. doi: 10.1136/bmjopen-2014-004848
49. Hughes, A, Daniel, S, Kilford, L, and Ajjon, L. Accuracy of clinical diagnosis of idiopathic Parkinson’s disease: a clinico-pathological study of 100 cases. J Neurol Neurosurg Psychiatry. (1992) 55:181–4. doi: 10.1136/jnnp.55.3.181
50. Wan, X, Wang, W, Liu, J, and Tong, T. Estimating the sample mean and standard deviation from the sample size, median, range and/or interquartile range. BMC Med Res Methodol. (2014) 14:135. doi: 10.1186/1471-2288-14-135
51. Luo, D, Wan, X, Liu, J, and Tong, T. Optimally estimating the sample mean from the sample size, median, mid-range, and/or mid-quartile range. Stat Methods Med Res. (2018) 27:1785–805. doi: 10.1177/0962280216669183
52. Sterne, JA, Savović, J, Page, MJ, Elbers, RG, Blencowe, NS, Boutron, I, et al. RoB 2: a revised tool for assessing risk of bias in randomised trials. BMJ. 366:l4898. doi: 10.1136/bmj.l4898
53. GRADEpro GDT: GRADEpro Guideline Development Tool [Software]. McMaster University and evidence prime. (2022). Available at: gradepro.org (accessed June 10, 2020).
54. Vandenbroucke, JP. Bias in meta-analysis detected by a simple, graphical test. Experts' views are still needed. BMJ. (1998) 316:469.
55. Thorlund, K, Engstrøm, J, Wetterslev, J, Brok, J., Imberger, G., and Gluud, C. User manual for trial sequential analysis (TSA). Copenhagen trial unit, Centre for Clinical Intervention research, Copenhagen, Denmark. (2011):1–115. Available at: www.ctu.dk/tsa (accessed June 15, 2020).
56. Ortiz, GG, Moráles-Sánchez, EW, Pacheco-Moisés, FP, Jiménez-Gil, FJ, Macías-Islas, MA, Mireles-Ramírez, MA, et al. Effect of melatonin administration on cyclooxygenase-2 activity, serum levels of nitric oxide metabolites, lipoperoxides and glutathione peroxidase activity in patients with Parkinson’s disease. Gac Med Mex. (2017) 153:S72–81. doi: 10.24875/GMM.M000008
57. Kakhaki, RD, Ostadmohammadi, V, Kouchaki, E, Aghadavod, E, Bahmani, F, Tamtaji, OR, et al. Melatonin supplementation and the effects on clinical and metabolic status in Parkinson's disease: a randomized, double-blind, placebo-controlled trial. Clin Neurol Neurosurg. (2020) 1:105878. doi: 10.1016/j.clineuro.2020.105878
58. Gilat, M, Coeytaux Jackson, A, Marshall, NS, Hammond, D, Mullins, AE, Hall, JM, et al. Melatonin for rapid eye movement sleep behavior disorder in Parkinson's disease: a randomised controlled trial. Mov Disord. (2020) 35:344–9. doi: 10.1002/mds.27886
59. Medeiros, CA, Carvalhedo de Bruin, PF, Lopes, LA, Magalhães, MC, de Lourdes, SM, and de Bruin, VM. Effect of exogenous melatonin on sleep and motor dysfunction in Parkinson's disease. A randomized, double blind, placebo-controlled study. J Neurol. (2007) 254:459–64. doi: 10.1007/s00415-006-0390-x
60. Ahn, JH, Kim, M, Park, S, Jang, W, Park, J, Oh, E, et al. Prolonged-release melatonin in Parkinson's disease patients with a poor sleep quality: a randomized trial. Parkinsonism Relat Disord. (2020) 75:50–4. doi: 10.1016/j.parkreldis.2020.03.029
61. Singer, C, McArthur, A, Hughes, R, Sack, R, Kaye, J, and Lewy, A. High dose melatonin administration and sleep in the elderly. Sleep Res. (1995) 24:151.
62. Singer, C, McArthur, A, Hughes, R, Sack, R, Kaye, J, and Lewy, A. Physiologic melatonin administration and sleep in the elderly. Sleep Res. (1995) 24:152.
63. Dowling, GA, Mastick, J, Colling, E, Carter, JH, Singer, CM, and Aminoff, MJ. Melatonin for sleep disturbances in Parkinson's disease. Sleep Med. (2005) 6:459–66. doi: 10.1016/j.sleep.2005.04.004
64. Jahnke, G, Marr, M, Myers, C, Wilson, R, Travlos, G, and Price, C. Maternal and developmental toxicity evaluation of melatonin administered orally to pregnant Sprague-Dawley rats. Toxicol Sci. (1999) 50:271–9. doi: 10.1093/toxsci/50.2.271
65. Seabra, MD, Bignotto, M, Pinto, LR Jr, and Tufik, S. Randomized, double-blind clinical trial, controlled with placebo, of the toxicology of chronic melatonin treatment. J Pineal Res. (2000) 29:193–200. doi: 10.1034/j.1600-0633.2002.290401.x
66. You, H, Mariani, LL, Mangone, G, Febvre, L, de Nailly, D, Charbonnier-Beaupel, F, et al. Molecular basis of dopamine replacement therapy and its side effects in Parkinson’s disease. Cell Tissue Res. (2018) 373:111–35. doi: 10.1007/s00441-018-2813-2
67. Kunz, D, and Bes, F. Melatonin as a therapy in REM sleep behavior disorder patients: an open-labeled pilot study on the possible influence of melatonin on REM-sleep regulation. Mov Disord. (1999) 14:507–11. doi: 10.1002/1531-8257(199905)14:3<507::AID-MDS1021>3.0.CO;2-8
68. McGrane, IR, Leung, JG, Louis, EK, and Boeve, BF. Melatonin therapy for REM sleep behavior disorder: a critical review of evidence. Sleep Med. (2015) 16:19–26. doi: 10.1016/j.sleep.2014.09.011
69. de Almeida, CM, Pachito, DV, Sobreira-Neto, MA, Tumas, V, and Eckeli, AL. Pharmacological treatment for REM sleep behavior disorder in Parkinson disease and related conditions: a scoping review. J Neurol Sci. (2018) 393:63–8. doi: 10.1016/j.jns.2018.08.008
70. Takeuchi, N, Uchimura, N, Hashizume, Y, Mukai, M, Etoh, Y, Yamamoto, K, et al. Melatonin therapy for REM sleep behavior disorder. Psychiatry Clin Neurosci. (2001) 55:267–9. doi: 10.1046/j.1440-1819.2001.00854.x
71. Boeve, BF, Silber, MH, and Ferman, TJ. Melatonin for treatment of REM sleep behavior disorder in neurologic disorders: results in 14 patients. Sleep Med. (2003) 4:281–4. doi: 10.1016/S1389-9457(03)00072-8
72. McCarter, SJ, Boswell, CL, Louis, EK, Dueffert, LG, Slocumb, N, Boeve, BF, et al. Treatment outcomes in REM sleep behavior disorder. Sleep Med. (2013) 14:237–42. doi: 10.1016/j.sleep.2012.09.018
73. Schaefer, C, Kunz, D, and Bes, F. Melatonin effects in REM sleep behavior disorder associated with obstructive sleep apnea syndrome: a case series. Curr Alzheimer Res. (2017) 14:1084–9. doi: 10.2174/1567205014666170523094938
74. Lemoine, P, Garfinkel, D, Laudon, M, Nir, T, and Zisapel, N. Prolonged-release melatonin for insomnia–an open-label long-term study of efficacy, safety, and withdrawal. Ther Clin Risk Manag. (2011) 7:301–11. doi: 10.2147/TCRM.S23036
75. Seppi, K, Ray Chaudhuri, K, Coelho, M, Fox, SH, Katzenschlager, R, Perez Lloret, S, et al. Update on treatments for nonmotor symptoms of Parkinson's disease—an evidence-based medicine review. Mov Disord. (2019) 34:180–98. doi: 10.1002/mds.27602
Keywords: melatonin, Parkinson’s disease, movement disorders, motor symptoms, sleep disturbances
Citation: Iftikhar S, Sameer HM and Zainab (2023) Significant potential of melatonin therapy in Parkinson’s disease – a meta-analysis of randomized controlled trials. Front. Neurol. 14:1265789. doi: 10.3389/fneur.2023.1265789
Edited by:
Görsev Yener, İzmir University of Economics, TürkiyeReviewed by:
Nian Xiong, Huazhong University of Science and Technology, ChinaHéctor Alberto González-Usigli, Mexican Social Security Institute (IMSS), Mexico
Copyright © 2023 Iftikhar, Sameer and Zainab. This is an open-access article distributed under the terms of the Creative Commons Attribution License (CC BY). The use, distribution or reproduction in other forums is permitted, provided the original author(s) and the copyright owner(s) are credited and that the original publication in this journal is cited, in accordance with accepted academic practice. No use, distribution or reproduction is permitted which does not comply with these terms.
*Correspondence: Sadaf Iftikhar, c2FkYWZuZXVyb2xvZ3lAa2VtdS5lZHUucGs=