- 1School of First Clinical Medical, Hunan University of Chinese Medicine, Changsha, Hunan, China
- 2School of Acupuncture-Moxibustion and Tuina, Hunan University of Chinese Medicine, Changsha, Hunan, China
- 3Acupuncture and Rehabilitation Center, The First Hospital of Hunan University of Chinese Medicine, Changsha, Hunan, China
Objectives: With the advent of an aging population society, the morbidity and mortality rates of stroke are on the rise. Most surviving patients are often accompanied by a series of sequelae, which seriously affect patients’ social function and physical and mental health. The application of non-invasive brain stimulation (NIBS) in neurorehabilitation has attracted widespread attention. This study aims to explore the key theme and future direction of the research in this field.
Methods: Articles and reviews related to NIBS for stroke from January 1985 to September 2024 were identified from the Web of Science Core Collection database. The CiteSpace, VOSviewer software, and Charticulator website were used to visualize and analyze the publications, countries, institutions, authors, journals, keywords, cited references, subject categories, and funding agencies from various angles.
Results: A total of 4,453 papers were included in this study, with the United States publishing the most, followed by China. The most outstanding author was Fregni F from Harvard Medical School. Frontiers in Neurology had the highest number of publications. Plasticity and excitability represent two particularly major themes, and connectivity is the keyword of the research frontier in recent years.
Conclusion: NIBS shows considerable potential and broad development space in stroke rehabilitation. This study analyses the research hotspots and emerging trends in this field, thereby providing a framework for deeper research and contributing to the vigorous development of NIBS for stroke.
Highlights
• Most patients of stroke are often accompanied by a series of sequelae, which seriously affect patients' social function and physical and mental health.
• NIBS has significant therapeutic value and potential in the field of stroke rehabilitation.
• The research in this field is gradually shifting from clinical medicine to fundamental disciplines and social sciences.
• Functional connectivity of brain networks may be the future direction of mechanism research.
1 Introduction
Stroke is an acute cerebrovascular disease that causes damage to brain tissue, primarily due to the interruption of blood circulation within the brain. There are two main types of stroke: ischemic stroke (IS) and hemorrhagic stroke (HS) (1). The 2019 Global Burden of Disease (GBD) database indicates that stroke still has high rates of disability and remains the second leading cause of death globally, second only to heart disease (2). In recent years, the incidence of stroke has shown an alarming increase and tends to be younger due to unhealthy lifestyles and food safety issues, making it one of the major challenges to global health (3). In China, the prevalence of stroke in 2021 has increased by 104.26% since 1990, stroke is still the leading cause of health loss (4). The long-term prognosis of stroke patients is dependent on several factors, including the extent of brain damage and the duration of treatment. Individuals with severe brain injuries may suffer long-term disability or even mortality (5). With the advancement of medical care, the survival rate of stroke patients has been greatly improved. However, many patients suffer from severe neurological dysfunction due to missing the optimal time window. This not only affects the individual but also places a considerable economic burden on families and society at large (6). Therefore, it is imperative to improve post-stroke dysfunction and enhance patients’ ability to live independently and quality of life.
With the development of neurological disciplines and the advancement of technical means, brain stimulation has received more and more attention in the field of neurorehabilitation, and non-invasive brain stimulation (NIBS) is one of them (7). NIBS is a technique for modulating brain function that involves applying energy from physical factors, such as electricity, magnetism, or ultrasound, to the brain without damaging the scalp and skull. This approach is used to alter specific neural activities and behaviors. Unlike physical therapy, occupational therapy, and other forms of stroke rehabilitation, NIBS can directly modulate neural activity in specific brain regions and networks, thereby promoting neuronal plasticity and reconstruction of neural circuits (8, 9). In recent years, NIBS has been widely used in stroke rehabilitation due to its advantages of convenience, feasibility, non-invasiveness, cost-effectiveness, and efficacy. Numerous studies have confirmed that NIBS can significantly improve dysfunctions after stroke, including dysphagia, aphasia, dyskinesia, cognitive impairment, and depression. Additionally, NIBS has been shown to regulate the content and distribution of neurotransmitters in the brain, enhance the microenvironment of neurons, and influence the remodeling of synaptic function (10–14). NIBS has significant therapeutic value and potential in the field of stroke rehabilitation and has become one of the research hotspots in related academic fields both domestically and internationally.
Bibliometrics is a discipline that takes literature as the research object and explores the internal structural characteristics and patterns within a specific field through mathematical, statistical, and other measurement methods. This discipline plays a crucial role in the evaluation of scientific research and the planning of disciplinary development, characterized by its highly quantitative nature and strong practical applicability. At present, some researchers have conducted bibliometric analysis on transcranial magnetic stimulation (TMS) or transcranial direct current stimulation (tDCS) for stroke (15–19). The scope of this study is much broader, involving all NIBS techniques for stroke. This approach seeks to provide a systematic, objective, and comprehensive understanding of the current status, research hotspots, and emerging trends in the application of NIBS within the field of stroke rehabilitation. Ultimately, the findings aim to offer research directions for further inquiry and practice by rehabilitation clinicians and researchers.
2 Materials and methods
2.1 Data source and search strategy
The data for this study were sourced from the Web of Science Core Collection (WoSCC), which includes the Science Citation Index Expanded (SCI-Expanded), Social Sciences Citation Index (SSCI), Arts & Humanities Citation Index (AHCI), Conference Proceedings Citation Index-Science (CPCI-S), Conference Proceedings Citation Index-Social Science & Humanities (CPCI-SSH), Emerging Sources Citation Index (ESCI), Current Chemical Reactions (CCR-Expanded), and Index Chemicus (IC). Web of Science contains a wide range of international academic journals and stands as one of the most comprehensive and authoritative database platforms for obtaining global academic information, which highly represents academic development in a particular field (20). To avoid potential bias caused by database updates, literature retrieval and data extraction were performed on the same day.
To ensure a comprehensive search, subject terms were derived from the Medical Subject Headings (MeSH) database1, and a combination of these MeSH terms and related terms was employed, incorporating truncations (*) to increase the search rate. The search strategy is detailed in Supplementary material 1. The search time range was from January 1, 1985, to September 6, 2024. The search document type was set to “Article” and “Review,” and the document language was set to English. To ensure data accuracy, two researchers manually reviewed publications following predefined criteria. Any discrepancies encountered would be resolved by a third professional (Figure 1).
2.2 Exclusion criteria
(1) Literature related to book chapters, proceeding papers, meeting abstracts, editorial materials, letters, retracted publications, notes, news items, and corrections; (2) Irrelevant, duplicated, or unpublished literature; and (3) Literature not in English.
2.3 Bibliometric analysis
Literature that met the inclusion and exclusion criteria was exported to plain text named “download_xxx.txt” with “full record and cited references.” The exported documents were subsequently imported into the Charticulator online website, VOSviewer 1.6.20, and CiteSpace 6.3.R1 software for the construction of knowledge graphs and statistical analyses. The Charticulator online website, VOSviewer, and CiteSpace software are powerful tools for the analysis of scientific research literature, each with its unique features and advantages.
The Charticulator2 is a powerful and free online visualization platform developed by Microsoft Research. This website was used to conduct the collaborative analysis of countries (21).
VOSviewer is a free, Java-based bibliometric software tool that facilitates the construction and visualization of scientific literature networks. It generates detailed and comprehensible network maps based on various relationships (22). In this study, VOSviewer software was used for co-occurrence analysis of countries, institutions, authors, journals, cited references, and keywords. The count mode is full count, the minimum citation frequency is set appropriately, and the rest of the parameters are the default settings of the software. The node represents an element, node size indicates the number of literature, the connecting lines between nodes illustrate the collaborative relationships, and its thickness reflects the degree of closeness and extent of these connections. A thicker line signifies a closer relationship, while a greater number of lines indicates more extensive cooperation with other nodes, positioning it in a relatively core role and the higher its influence. Additionally, the color of the nodes represents the clusters formed by their cooperation.
CiteSpace is another free Java-based bibliometric software developed by Chen and Song, primarily designed to assist researchers in identifying and analyzing research hotspots and emerging trends within specific research areas (23). In this study, CiteSpace was used to generate the burst maps of countries, institutions, authors, and references, as well as the clustering and burst maps of keywords. Parameter settings: the period from January 1990 to September 2024, time slice is 1 year, TopN = 50, TopN% = 10.0%, the pruning methods are pathfinder and pruning sliced networks, while the rest are maintained at the system default settings.
2.4 Research ethics
The data sources for this study were obtained from public databases and no human subjects were involved in the study, therefore ethical approval was not required.
3 Results
3.1 Annual publications and citation trend
From 1985 to 2024, a total of 5,030 relevant publications were published in the WoSCC database concerning NIBS for stroke. After eliminating irrelevant literature types, duplicates, and non-English literature, 3,502 articles and 951 reviews were extracted. Figure 2 illustrates the annual publications and citation trend of the literature related to NIBS for stroke. Over the past 30 years, the annual publications in this field showed an upward trend, while the peak occurred in 2022. This growth can be roughly categorized into three stages: The first stage, from 1990 to 2010, was characterized by a slow annual growth rate, with the number of publications and citations remaining below 150 per year. The second stage is from 2011 to 2018, with a flat and gradual growth in related studies. The third stage, from 2019 to 2024, demonstrated a rapid growth trend and reached a peak in 2022, which indicated that the NIBS has been widely used in stroke rehabilitation with the development of neurological discipline and the advancement of technical means. The decline in the number of publications and citations in 2024 may be attributed to the inclusion of only 9 months of literature, but the overall trend remained stable and at a high level, reflecting the continuity and significance of NIBS in stroke rehabilitation. All publications were cited a total of 184,478 times, with an average of 41.43 citations per publication and an H-Index of 180.
3.2 Analysis of countries/regions
Analyzing the countries/regions of corresponding authors (Figure 3A), a total of 82 countries/regions have contributed to research in this field. The United States, China, Germany, and the United Kingdom emerged as the most active contributors, followed by Italy and Japan. In the map of the country/region cooperation network (Figure 3B), the thickness of the connecting lines is proportional to the degree of cooperation. Thus, thicker lines indicate closer collaborations between countries. Research on NIBS for stroke involved a wide range of countries, with the United States and Germany establishing the most collaborations with other nations, highlighting the importance of international cooperation for comprehensive research in this field. Table 1 illustrates the top 10 countries/regions with the most publications regarding NIBS for stroke. The United States ranked first (1,184, 26.60%), followed by China (697, 15.65%) and Germany (508, 11.41%). Collectively, these three countries accounted for 53.66% of the total publications, underscoring their significant contributions to the field. Additionally, the United States has the highest total citations (67,327) and total link strength (839), while the United Kingdom has the highest average citations (85.03).
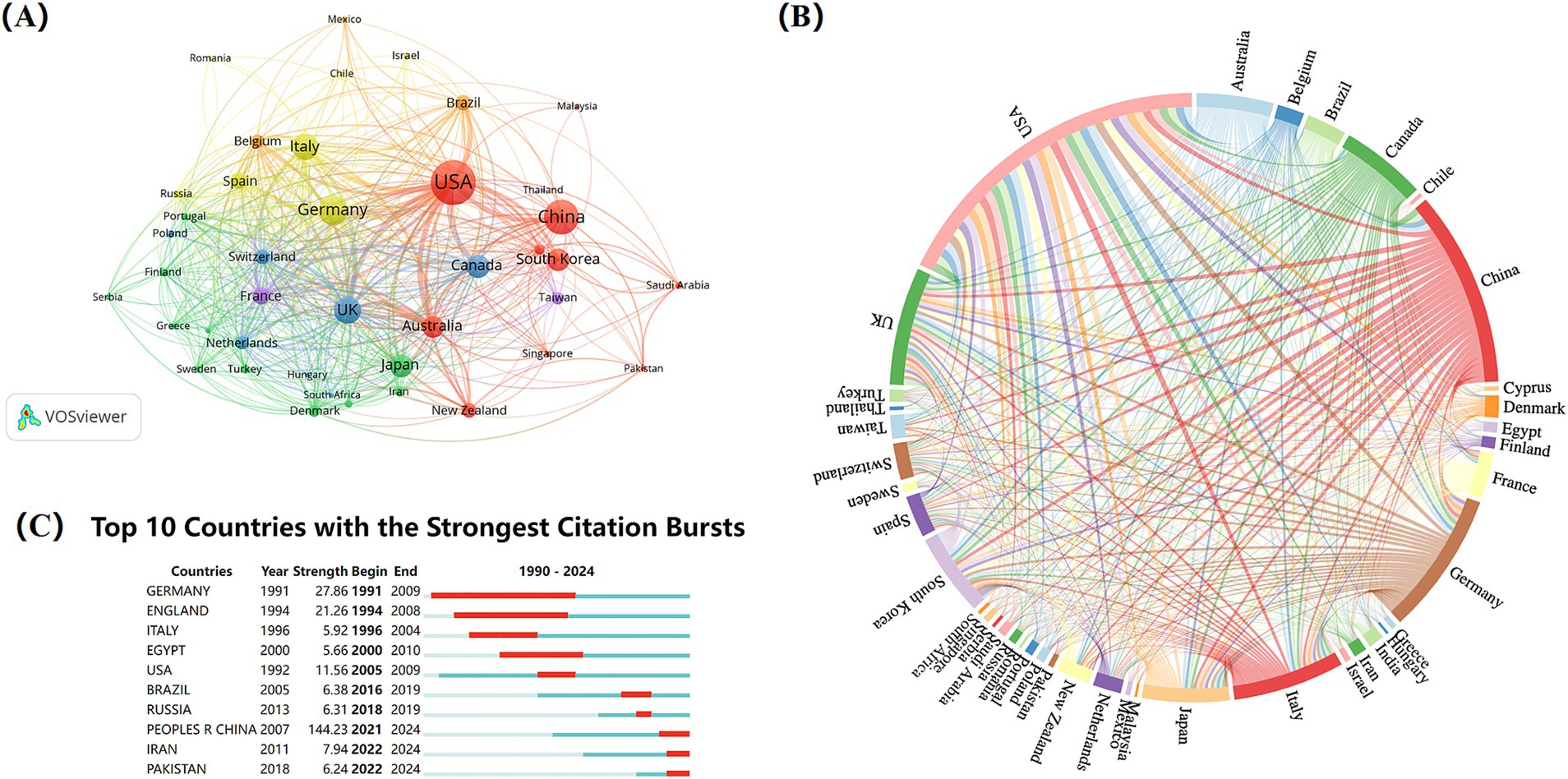
Figure 3. Analysis of countries/regions on NIBS for stroke. (A) Co-authorship analysis of countries/regions. The analysis was performed using VOSviewer with the method linlog/modularity, Eight occurrences were included only. (B) International collaboration analysis among different countries/regions. (C) Top 10 countries with the strongest citation bursts. Light blue indicates that the keyword has not yet appeared. Dark blue indicates that keywords have started to appear, but there have been no significant changes during the period. Red indicates the duration of the strongest citation.
Burst terms are keywords that exhibit the highest change rates over a specific period, reflecting historical changes, emerging trends, and future hotspots of research within the field. Among the top ten countries/regions with the strongest citation bursts (Figure 3C), China demonstrated the highest burst strength (144.23), with the burst years primarily concentrated in 2021–2024. This indicates that many Chinese researchers have recently engaged in NIBS techniques for stroke rehabilitation. Germany and the United Kingdom followed closely, with burst strength of 27.86 and 21.26, respectively.
3.3 Analysis of institutions
A total of 4,015 institutions published literature on NIBS for stroke. Table 2 lists the top ten relevant institutions with the highest number of publications, half of which are from the United States. Harvard University published the most literature, followed by University College London and the University of Auckland. The top three institutions with the most citations are Harvard University (12,355), University College London (9907) and Neurological Disease and Stroke (8052). It is worth noting that Harvard University is at the top of the list in terms of number of publications, number of citations and Total link strength, which to some extent reflects its high academic impact and capability to produce influential research outcomes.
Figure 4A is the overlay visualization map of institutions co-authorship analysis and institutions’ citation analysis. Nodes represent institutions, while the color of the nodes represents the year of publication, referring to the color gradient in the bottom right corner of the figure. Harvard University, the National Institute of Neurological Disease and Stroke, and Johns Hopkins University are depicted in purple, indicating that these institutions are early researchers and have been more far-reaching research in the field. The yellow nodes likely represent emerging institutions in this area, with Fudan University noted as the institution with the highest number of publications in recent years. Meanwhile, it can be found that Harvard University has more collaborations with other institutions, primarily within the United States, which facilitates in-depth research in this field through close cooperation domestically. Figure 4B is the overlay visualization map of institutions’ citation analysis. Purple represents institutions with high citations in the early years, larger nodes correspond to greater influence. Yellow represents institutions with high citations in recent years, indicating that the research of these institutions may be more in line with the current trends and hotspots. Overall, the top three in terms of total link strength are Harvard University (9515), University College London (6856), and University of Auckland (5737), revealing the dominance of the United States and the United Kingdom in this academic field.
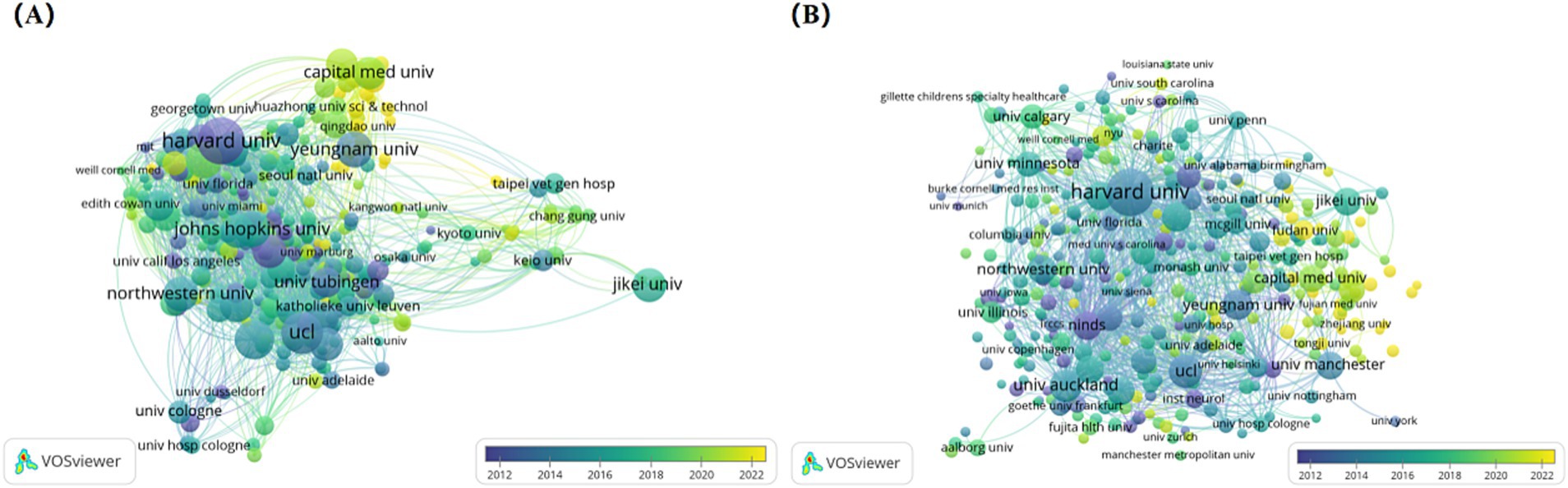
Figure 4. Analysis of institutions on NIBS for stroke. (A) Overlap visualization map of institutions co-authorship analysis generated by VOSviewer with the method linlog/modularity. Eight occurrences were included only. The purple and blue colors represented an early appearance, and the yellow color represented a late appearance. (B) Overlap visualization map of institutions’ citation analysis generated by VOSviewer with the method linlog/modularity. Eight occurrences were included only. The purple and blue colors represented an early appearance, and the yellow color represented a late appearance.
In the institutional burst detection (Figure 5), the top three in terms of burst strength are NINDS (21.88), NIH (18.42), and Sun Yat-sen University (14.52), with the years of bursts primarily concentrated on 1997–2011, 1997–2008, and 2020–202, respectively. Notably, from 2020 to 2024, many Chinese institutions began to engage in research within this field, and it is expected that a large number of research outputs will be produced subsequently.
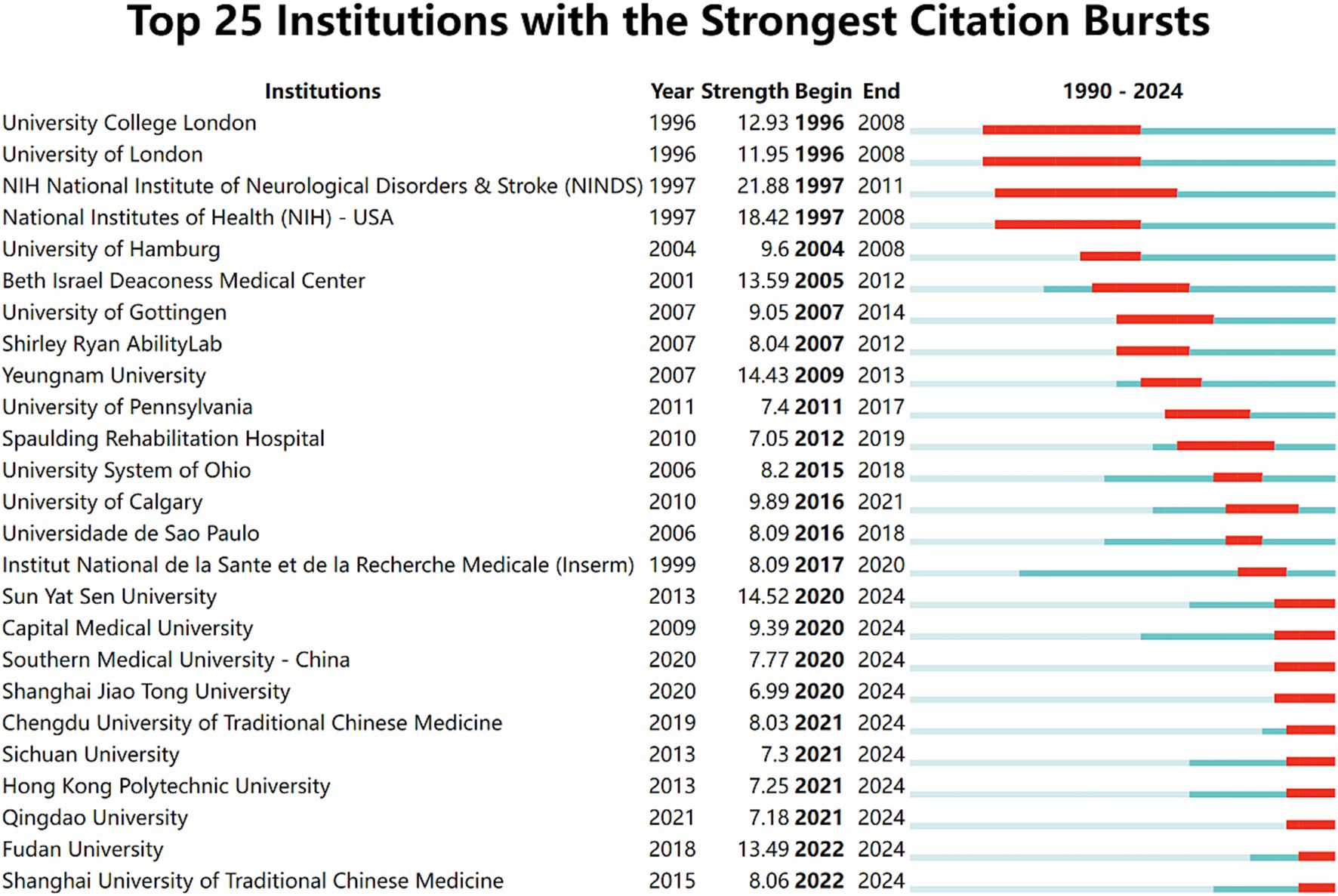
Figure 5. Top 25 institutions with the strongest citation bursts. Light blue indicates that the keyword has not yet appeared. Dark blue indicates that keywords have started to appear, but there have been no significant changes during the period. Red indicates the duration of the strongest citation.
3.4 Analysis of authors
A total of 15,886 researchers participated in this study, contributing to 4,453 publications. Table 3 lists the top 10 authors in the field with 499 publications. Among these authors, Fregni F (Harvard Medical School), Abo M (Jikei University), and Jang SH (Yeungnam University) were the outstanding contributors with the highest number of publications. Notably, Fregni F and Pascual-Leone A were both from Harvard Medical School. Fregni F topped the list with 74 publications and 6,169 citations, while Pascual-Leone A ranked first in terms of H-index and second in total citations (5,604). The H-index is one of the metrics for evaluating the scholarly impact of a researcher, organization, or journal, which was considered a more comprehensive and fair measure of research impact (24). A higher H-index implies that the author’s articles have been extensively cited. It is no doubt that the academic achievements of the Harvard Medical School team in this field have been fully recognized.
VOSviewer software was used to export network visualization and overlap visualization maps of authors’ collaboration. It reflects both the collaborative relationship between the authors and the timeliness of the research of the authors involved. The network visualization map of authors’ collaboration generated by VOSviewer (Figure 6A) indicated that NIBS for stroke rehabilitation has formed a range of core collaborations. Notably, Fregni F team (Harvard Medical School), Jang SH team (Jikei University), Cohen LG (University of Auckland), and Byblow WD (National Institute of Neurological Disease and Stroke) were four influential and relatively stable academic teams. The overlap visualization map of authors’ collaboration generated by VOSviewer (Figure 6B) shows that Luo J and Feng WW collaborated the most recently, with significant publications occurring between 2020 and 2022. It is reflected that this collaborative team may be a new star in the field, further indicating that the research of NIBS for stroke remains a prominent and important study hotspot.
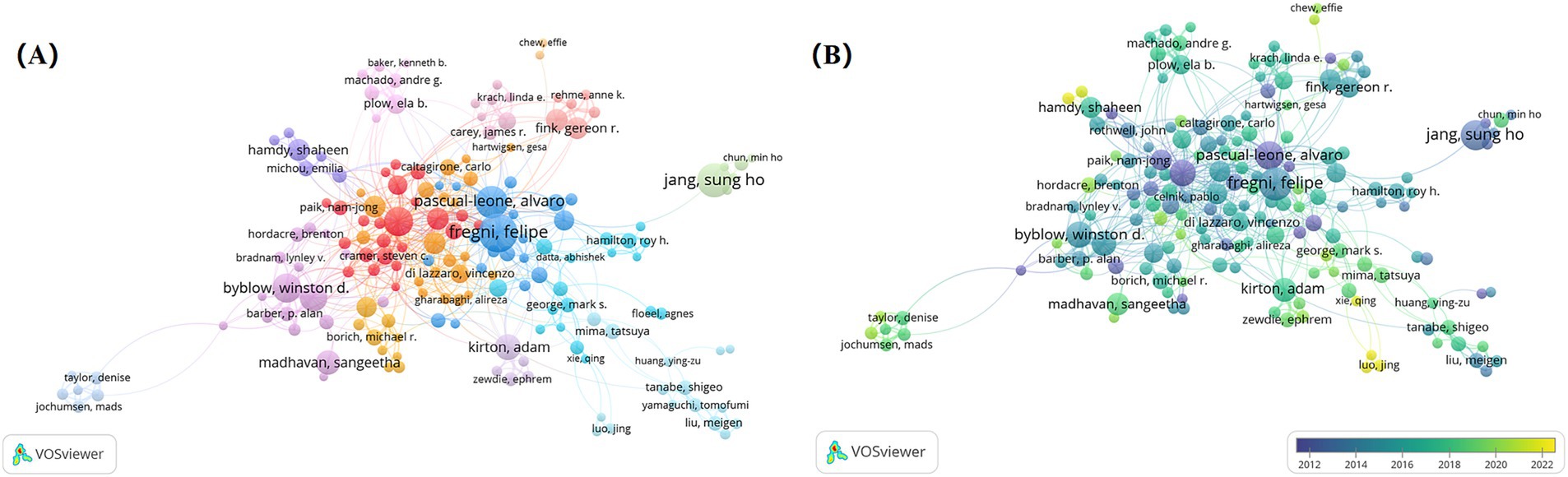
Figure 6. Analysis of authors on NIBS for stroke. (A) Network visualization map of authors’ collaboration analysis generated by VOSviewer with the method linlog/modularity. Eight occurrences were included only. (B) Overlap visualization map of authors’ collaboration analysis generated by VOSviewer with the method linlog/modularity. Eight occurrences were included only. The purple and blue colors represented an early appearance, and the yellow color represented a late appearance.
Figure 7 shows the top 25 authors with the strongest citation bursts. Jang SH, Cohen LG, and Pascual-leone A had the strongest bursts, indicating that they have created significant academic value for research related to NIBS in the field of stroke rehabilitation.
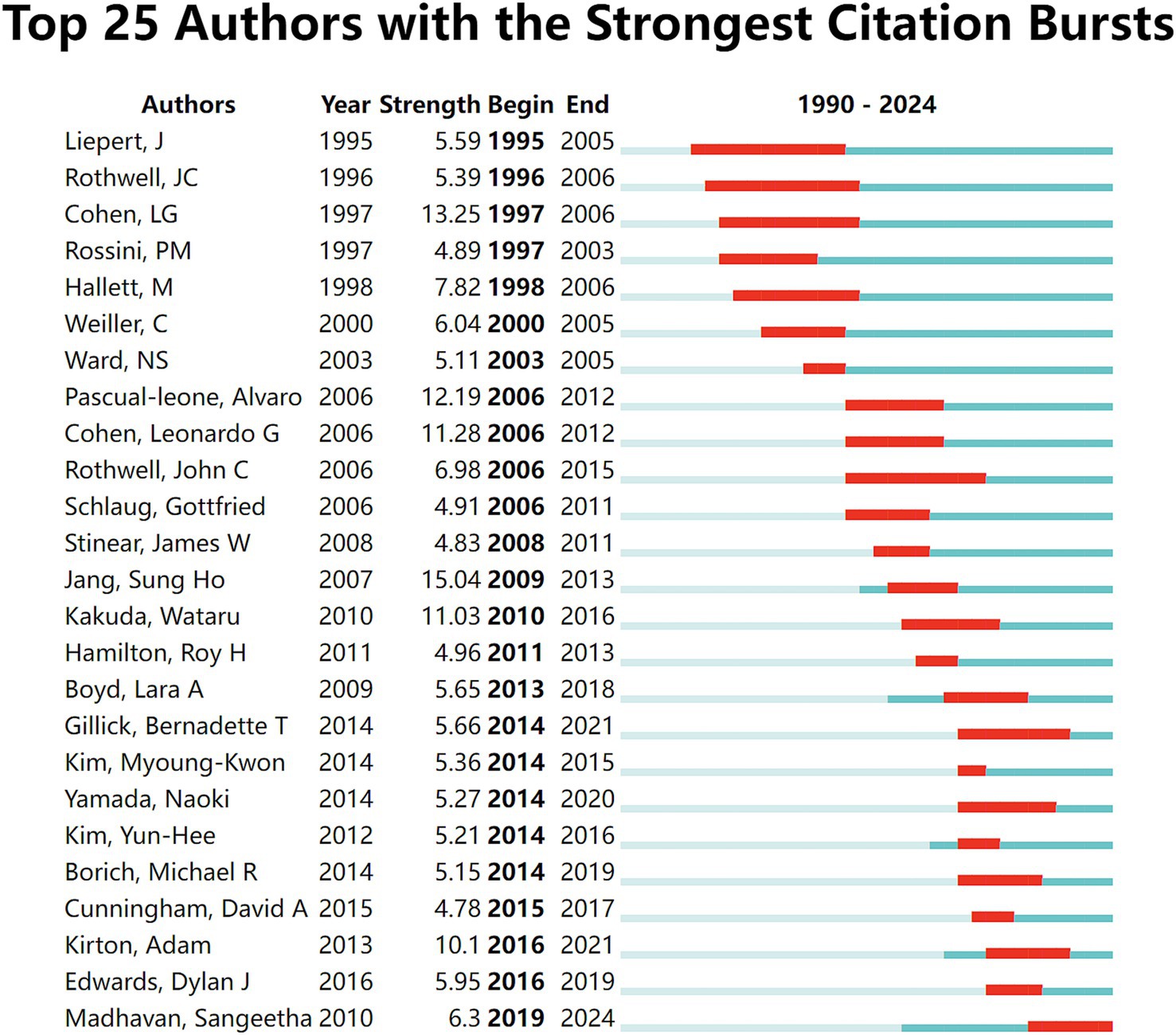
Figure 7. Top 25 authors with the strongest citation bursts. Light blue indicates that the keyword has not yet appeared. Dark blue indicates that keywords have started to appear, but there have been no significant changes during the period. Red indicates the duration of the strongest citation.
3.5 Analysis of journals
Journal publications are an important medium for the dissemination of scholarly knowledge and the exchange of learning, and this field of research has recently involved a total of 699 journals. Table 4 lists the top 10 journals with 1,111 articles on NIBS for stroke, accounting for 24.95% of the total literature. Among them, Frontiers in Neurology (160) had the highest number of publications, followed by Neurorehabilitation and Neural Repair (158), and Frontiers in Human Neuroscience (135). The top three in total citations were Clinical Neurophysiology (12,396), Stroke (10,896), and Neurorehabilitation and Neural Repair (8,074). In the Journal Citation Reports (JCR) partition, these journals accounted for 30% in Q1, 40% in Q2, 20%, and Q4 10%. The impact factor (IF) of the top ten journals in 2023 fluctuated from 1.9 to 7.8 points, with Stroke having the highest impact factor, as well as a significant number of total citations and Q1 partition, both of which confirmed the journal’s academic authority in the field. However, the overall impact factor remained low, with most journals below 4 points, which may be attributed to the relatively late emergence of modern rehabilitation, and related research is still in the slow development stage. Overall, the journals were closely linked to each other, with research covering a wide range of disciplines including neuroscience, physiology, and rehabilitation medicine. Frontiers in Neurology is probably the most popular journal in recent years (Figure 8).
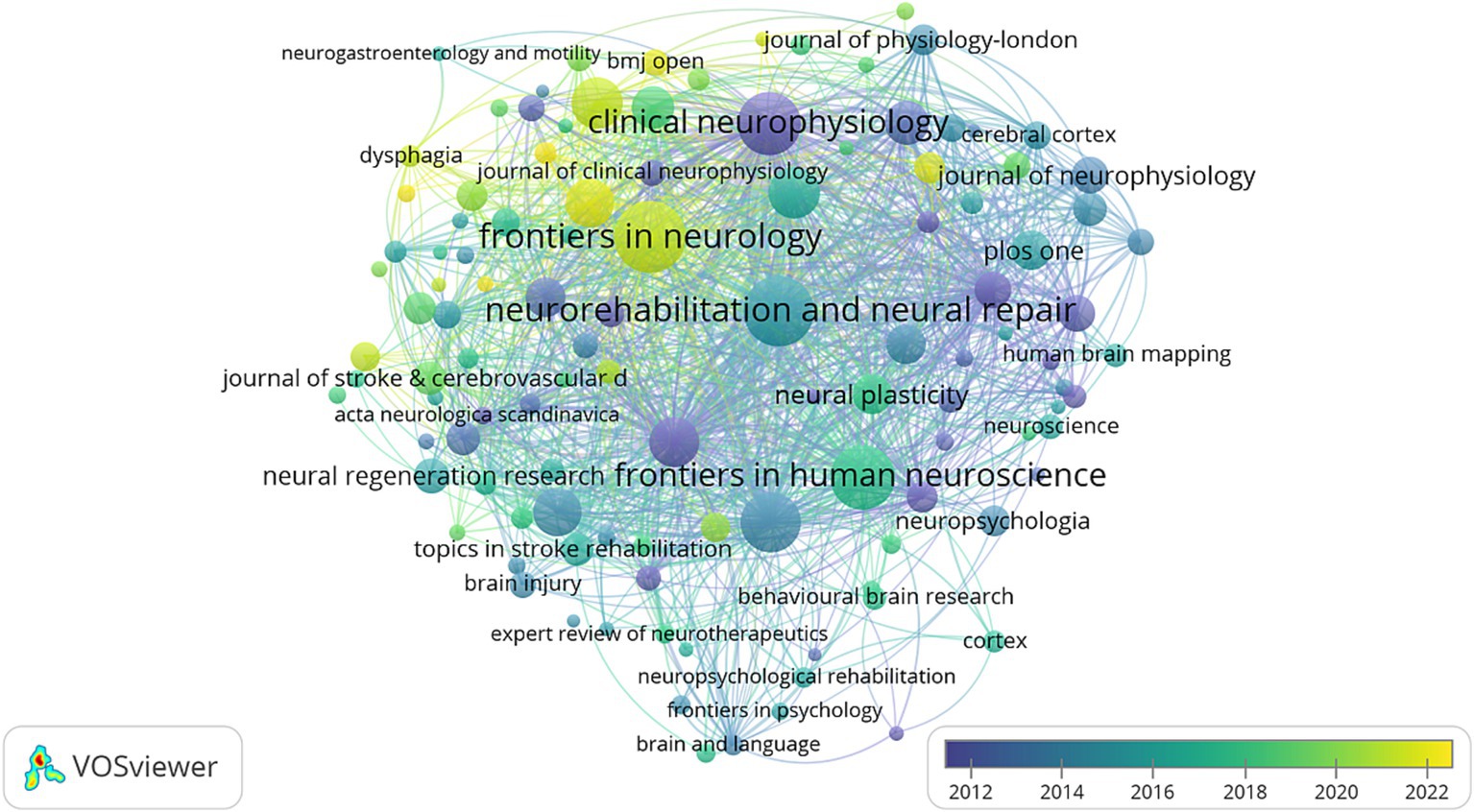
Figure 8. Overlap visualization map of journals analysis generated by VOSviewer with the method linlog/modularity. Eight occurrences were included only. The purple and blue colors represented an early appearance, and the yellow color represented a late appearance.
3.6 Analysis of keywords
The field of NIBS for stroke involved a total of 9,714 keywords, with 294 keywords appearing 30 times or more (Figure 9A). Among them, high-frequency keywords such as “stroke,” “transcranial magnetic stimulation,” “recovery,” “rehabilitation,” “plasticity,” and “excitability” highlighted their core position within the research. It can be seen that a series of new NIBS techniques for stroke rehabilitation have emerged in recent years, such as tDCS, rTMS, and theta-burst stimulation. Plasticity and excitability were the two major themes in mechanism research within this field, revealing the mechanisms of NIBS. Furthermore, aphasia and hemispatial neglect after stroke were more closely associated with TMS, providing a critical foundation for future in-depth research.
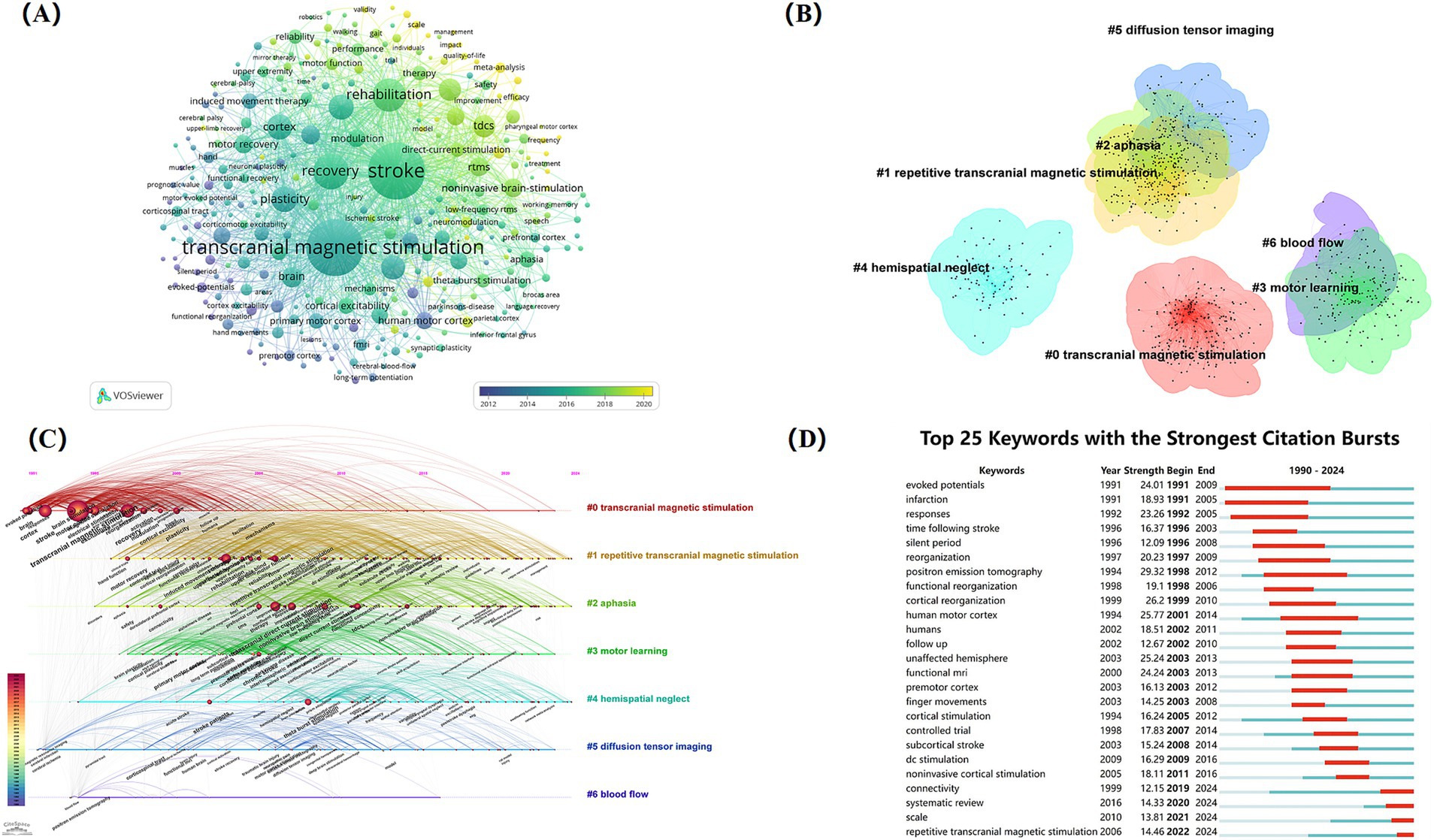
Figure 9. Analysis of keywords on NIBS for stroke. (A) Overlap visualization map of keywords co-occurrence analysis generated by VOSviewer with the method linlog/modularity. Thirty occurrences were included only. (B) Cluster map of keywords. (C) The timeline view of clustering analysis of keywords. (D) Top 25 keywords with the strongest citation bursts. Light blue indicates that the keyword has not yet appeared. Dark blue indicates that keywords have started to appear, but there have been no significant changes during the period. Red indicates the duration of the strongest citation.
The cluster analysis of keywords is the categorization and summary analysis of the clusters of keyword terms with similar meanings in the literature, assisting researchers in quickly grasping the main research themes within the field (25). In this study, keywords related to NIBS for stroke were analyzed by using CiteSpace. A total of six keyword clustering labels were formed (Figure 9B): #0 transcranial magnetic stimulation, #1 repetitive transcranial magnetic stimulation, #2 aphasia, #3 motor learning, #4 hemispatial neglect, #5 diffusion tensor imaging, and #6 blood flow.
The timeline of clustering analysis is analyzed based on the cluster analysis of keywords, focusing on the historical span of the clustered keywords to provide researchers with insights into stage hotspots and development trends within this research field. The timeline view of clustering of keywords (Figure 9C) indicates that cluster #0 (transcranial magnetic stimulation) has the earliest appearance and the longest duration, signifying that transcranial magnetic stimulation has been the topic theme. After 1995, cluster #1 (repetitive transcranial magnetic stimulation) became active, suggesting that with the development of modern technology, more innovative NIBS techniques were further developed and applied in clinical practice. Cluster #2 (aphasia) and cluster #4 (hemispatial neglect) are also noteworthy, highlighting the exploration of the efficacy of NIBS for treating specific post-stroke dysfunction, which had some evidence-based value. Cluster #5 and cluster #6 have received comparatively less attention in recent years.
Figure 9D illustrates the top 25 keywords with the strongest citation bursts from 1991 to 2016. “Positron emission tomography” and “human motor cortex” have the highest burst strength of 29.32 and 25.77, respectively. Notably, “evoked potentials” was the earliest keyword with long duration and high burst strength. Additionally, the keywords “repetitive transcranial magnetic stimulation,” “connectivity, “and” scale “exploded during 2019–2024, suggesting that these may represent the research hotspot of NIBS in stroke rehabilitation in recent years.
3.7 Analysis of cited references
The analysis of reference co-citation can reflect the status and hotspots of research to some extent, and the highly cited references are regarded as groundbreaking foundations in this research field (26). Over the past 30 years, a total of 100,965 papers have been cited in this field, and Table 5 lists the 10 most frequently cited papers, which mainly explore important scientific issues like clinical applications, treatment modalities, and mechanisms of NIBS in stroke. These highly cited references were published between 1994 and 2014, with citation counts ranging from 310 to 579. “Influence of Interhemispheric Interactions on Motor Function in Chronic Stroke” stood out with 579 citations and the highest total link strength.
This was followed by “Excitability changes induced in the human motor cortex by weak transcranial direct current stimulation,” as well as “Safety, ethical considerations, and application guidelines for the use of transcranial magnetic stimulation in clinical practice and research.” The first authors of two papers were Friedhelm Hummel, with one of them, “Non-invasive brain stimulation: a new strategy to improve neurorehabilitation after stroke?” published in one of the top journals in the field. This paper argued that TMS and tDCS can be developed into valuable treatments in neurorehabilitation, but must be further evaluated in multicentral clinical trials.
Network and density visualization map of references co-citation (Figures 10A,B) shows clustering into 7 categories with 666 nodes, 137,789 links, and 610,802 total link strength. Figure 10C shows the top 25 references with the strongest citation bursts, with the earliest citation burst occurring in 2002 and the most recent in 2022. Many references burst lasting about 4 or 5 years. The strongest burst was published in Clinical Neurophysiology, titled “Evidence-based guidelines on the therapeutic use of repetitive transcranial magnetic stimulation (rTMS): An update (2014–2018),” which introduces the latest guidelines for rTMS treatment, offering valuable insights for individualizing treatment and improving clinical efficacy.
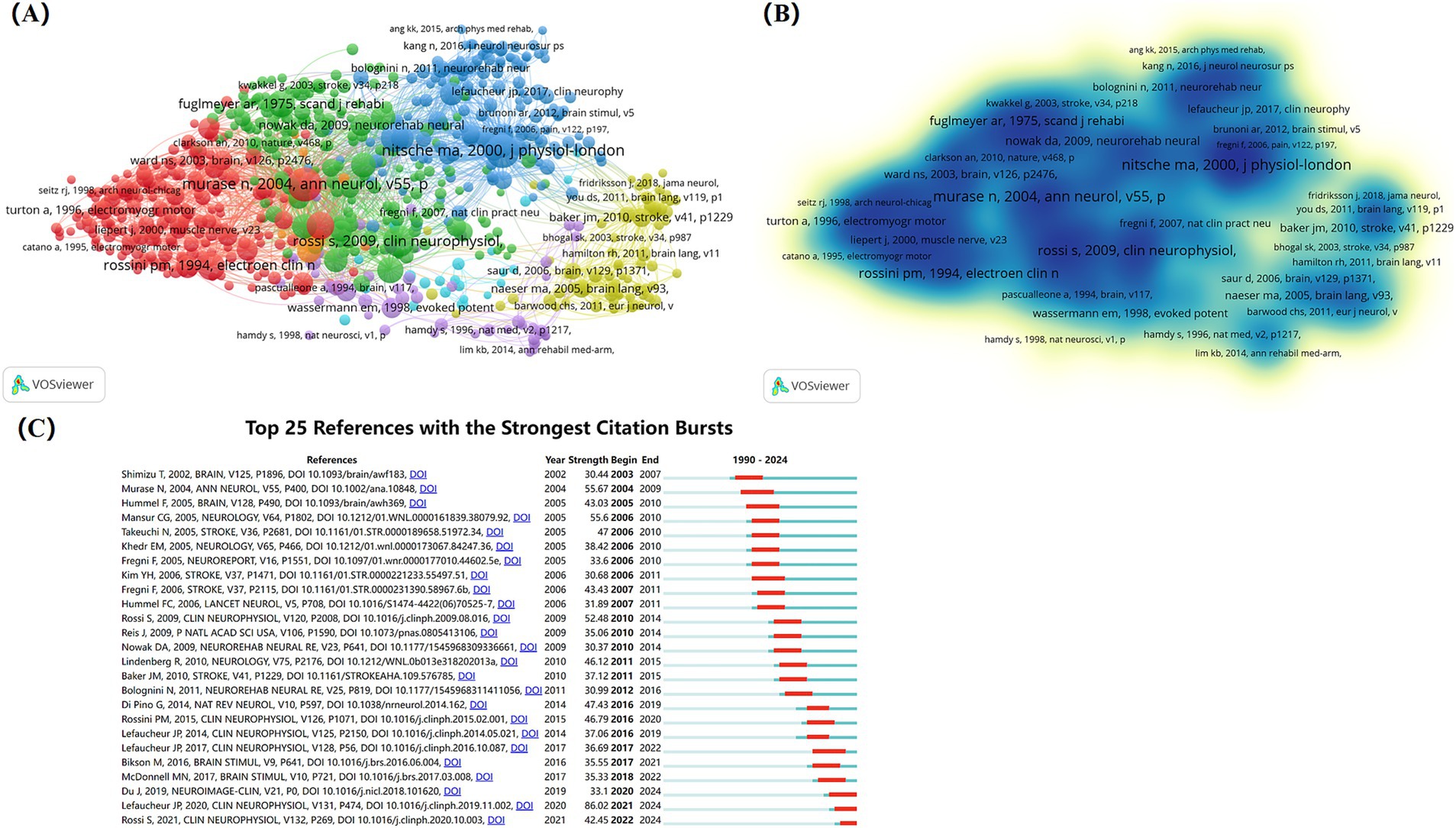
Figure 10. Analysis of cited references on NIBS for stroke. (A) Network visualization map of references co-citation analysis generated by VOSviewer with the method linlog/modularity. Forty occurrences were included only. (B) Density visualization map of references co-citation analysis generated by VOSviewer with the method linlog/modularity. Forty occurrences were included only. (C) Top 25 references with the strongest citation bursts. Light blue indicates that the keyword has not yet appeared. Dark blue indicates that keywords have started to appear, but there have been no significant changes during the period. Red indicates the duration of the strongest citation.
3.8 Analysis of subject categories
NIBS for stroke involved 121 subject categories, with its literature primarily concentrated in the fields of neurosciences, rehabilitation, psychology, and sport sciences. Table 6 lists the top 10 subject categories in this field, with neurosciences occupying the top one (244, 54.95%), followed by clinical neurology (1,579, 35.46%) and rehabilitation (17.22%). Figure 11 illustrates the top 25 subject categories with the strongest citation bursts. Notably, from 1994 to 2024, early research bursts in this field were predominantly concentrated in clinically oriented disciplines, such as peripheral vascular disease, clinical neurology, radiology, nuclear medicine & medical imaging. The research trend had a shift towards fundamental disciplines, including biochemistry & molecular biology, medicine, research & experimental, as well as social sciences like health care sciences & services, geriatrics & gerontology recently. It is suggested that the development of clinical, mechanistic, and social science research in NIBS for stroke is maturing with the progress of time.
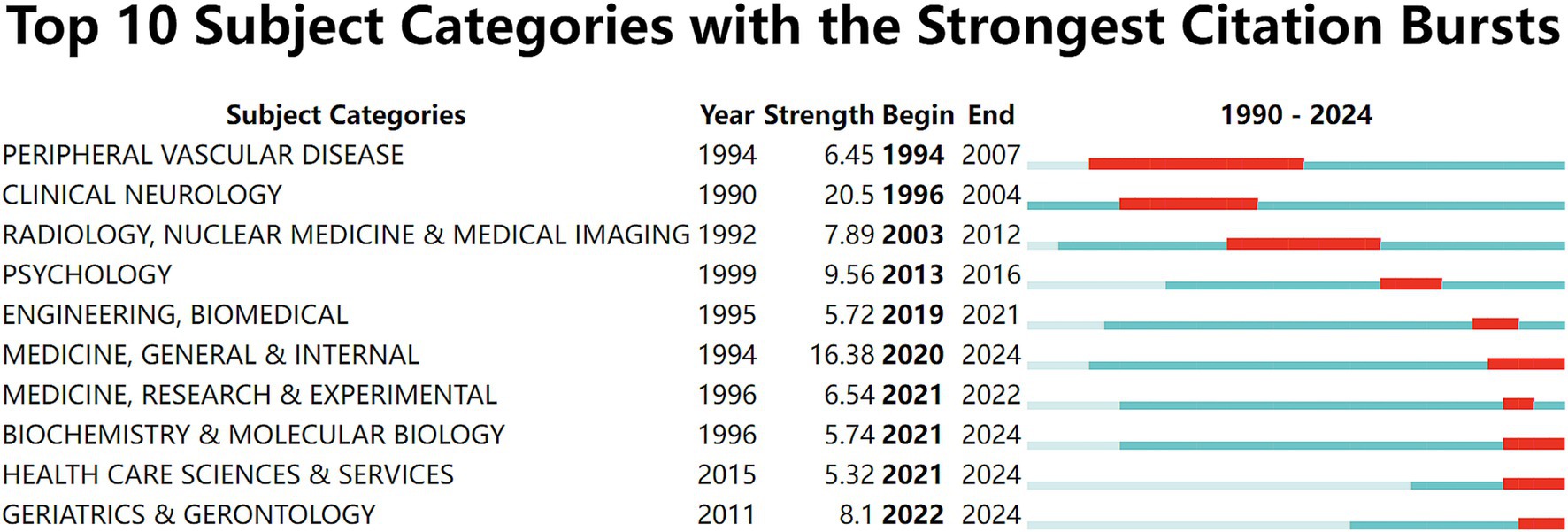
Figure 11. Top 25 subject categories with the strongest citation bursts. Light blue indicates that the keyword has not yet appeared. Dark blue indicates that keywords have started to appear, but there have been no significant changes during the period. Red indicates the duration of the strongest citation.
3.9 Analysis of funding agencies
A total of 3,068 organizations have provided funding for research in this area. Table 7 lists the top 10 organizations that provided the most funding for research in this field. The top three funding agencies were the United States Department of Health and Human Services (HHS), the National Institutes of Health (NIH), the National Natural Science Foundation of China (NSFC), with the United States being the major funding contributor to research in this field.
4 Discussion
4.1 Overview of results
This study analyzed 4,453 publications related to NIBS for stroke from 1985 to 2024, involving 82 countries/regions, 4,015 institutions, 15,886 researchers, and 699 journals. The publication trend can be broadly divided into three stages. From 1990 to 2010, the application of NIBS in stroke rehabilitation was in its infancy, and the related research was superficial. In the 1980s, Professor Anthony Barker of the University of Sheffield successfully applied non-invasive magnetic stimulation to elicit motor-evoked potentials, which opened the gate to a new world for research on transcranial magnetic stimulation in both fundamental and diagnostic fields (27). With the advancement of neuroimaging technology, researchers began to explore the higher brain functions of humans non-invasively (28). The most classic non-invasive brain stimulation technique, TMS, provided a new approach to scientific research by inducing “virtual lesions” (29). Arac’s team was the first to research TMS in stroke rehabilitation and explore its prognostic value (30). Although some studies have provided clinical evidence for the use of TMS in the treatment of stroke (31–34), the lack of standardization and convincing evidence regarding its effectiveness and the negative results from some studies have contributed to a relatively slow progression of research in this area (35). Fortunately, the interest of researchers remained high, probably due to the intuitive appeal of NIBS for their potential to promote neural plasticity (36). Between 2011 and 2018, the ever-evolving landscape of NIBS techniques led to an increase in clinical studies focused on stroke rehabilitation, broadening horizons for the development of the field. The number of publications in the field grew rapidly from 2019 to 2024, with a peak in 2022. This trend reflected a growing emphasis on NIBS for stroke rehabilitation by researchers, alongside the release of a series of expert consensus and guidelines (37, 38). Despite a slight decline in publications after 2022, the overall quantity is still considerable. It is not difficult to predict that future research in this area will continue its upward trajectory.
Currently, the United States, China, Germany, and the United Kingdom dominate this field, and the United States and Germany have the closest cooperation with other countries. Most of the active contributors were from developed countries, likely since NIBS techniques primarily originated in Europe and the United States. Moreover, developed countries have a high level of scientific research and abundant funding, providing important support for research in this field, which is an absolute advantage. Although China ranked second in the number of publications and has been increasingly active in more specific research in recent years, it lacked international cooperation, started late, and had less global influence. Surprisingly, half of the top 10 most productive institutions were from the United States, which may be attributed to the significant investment in fundamental research in the United States since 1950. The two major health agencies of the United States, HH and NIH, were key sources of funding for research in this area, further reaffirming the dominance and far-reaching influence of the United States. In recent years, China has strongly supported the development of public health and made rapid progress in this field, with Sun Yat-sen University as a typical representative. Over the past 10 years, Sun Yat-sen University has concentrated on NIBS for the rehabilitation of post-stroke dysphagia and aphasia, achieved a series of achievements and become a rising star in the academic landscape of this field (39–43).
Fregni F was a leader in terms of publications and citations, and his team’s main research interest was in exploring and developing NIBS techniques for the treatment of neurological disorders. Fregni F published a longitudinal cohort study protocol in 2021 that utilized assessment tools such as electroencephalography (EEG), functional near-infrared spectroscopy (fNIRS), TMS, and magnetic resonance imaging (MRI) to investigate biomarkers associated with dysfunction and to understand the impact LF-Rtms.
of rehabilitation on the neuroplasticity of the brain (44). Then with the help of EEG and TMS, the research further explored the relationship between the recovery of motor function of the upper limb and potential biomarkers, it is likely to provide significant value for accurate diagnosis, guidance of treatment, and disease prevention in stroke patients, making the study worthy of attention (45, 46). Fregni F and Pascual-leone A, both affiliated with Harvard University, have collaborated extensively and devoted to researching the efficacy and safety of the two classic NIBS techniques, tDCS and TMS, for stroke rehabilitation while exploring the optimal parameter or combination of each technique (47–49). In addition, Fregni F, as a leader in the field, has developed guidelines for the application of NIBS techniques in collaboration with researchers from Germany, Brazil, the United Kingdom, France, Italy, Denmark and other countries to assist clinicians in solving problems in clinical practice (50, 51). Broader international cooperation and high-quality outputs are expected in the future.
Of the top 10 highly productive journals, four were from Switzerland, three were in the United States, two were in Ireland, and one was in Netherlands, suggesting that these countries play a crucial role in advancing academic progress in the field. Three journals, Clinical Neurophysiology, Stroke, Neurorehabilitation and Neural Repair, ranked high in terms of total citations, all of which have a JCR partition of Q1 and primarily focus on clinical neurophysiology and neurorehabilitation research related to NIBS for the treatment of stroke, which are influential and noteworthy by researchers. In general, the impact factors of the journals typically ranged from 2 to 4 points, indicating that publishing high-quality studies in influential journals is still a challenge.
In the subject category, articles related to NIBS for stroke were mainly on neuroscience, clinical neurology and rehabilitation, which once again emphasized that stroke is a vascular disease that seriously affects human health and requires long-term neurorehabilitation management to improve dysfunction for alleviate the heavy burden. Among the top ten cited references, the one with the highest citation and total link strength was “Influence of Interhemispheric Interactions on Motor Function in Chronic Stroke” by Cohen LG, which describes the transcallosal effect in the lesioned hemisphere of patients with chronic stroke, laying the foundation for subsequent mechanistic research (52). It is worth noting that two articles published in Lancet and Nature (53, 54) found that NIBS can be used to monitor and regulate the excitability of neuronal circuits in the cortex, which partially validates the efficacy of NIBS in post-stroke rehabilitation. In recent years, the two publications with the strongest citation bursts are the expert guidelines on TMS and rTMS (37, 55). Meanwhile, we observed that a clinical practice guideline on stroke rehabilitation management, published in the Annals of Internal Medicine at the beginning of 2025, was cited 239 times within just 2 months. This indicates high attention among researchers in the standardized application of NIBS (56). But owing to the variability and complexity of the brain in different individuals, the efficacy of NIBS made a difference, which was a tough problem. Researchers should take individualized treatment protocols of NIBS for patients seriously.
4.2 Research hotspot analysis
Through co-occurrence, clustering, and burst analysis of keywords, it was found that the research hotspots and emerging trends in the field of NIBS for stroke were mainly concentrated in three aspects: the types, clinical indications, and assessment techniques of NIBS.
Currently, the mainstream technologies of NIBS mainly include two categories: TMS and Transcranial Electrical Stimulation (TES). TMS is primarily categorized into sTMS, pTMS, rTMS; TES is mainly classified into four categories: tDCS, transcranial alternating current stimulation (tACS), transcranial pulsed current stimulation (tPCS) and transcranial random noise stimulation (tRNS). In 2016, the American Heart Association/American Stroke Association issued specialized guidelines for stroke rehabilitation, recommending TMS and tDCS based on moderate-quality clinical trial evidence. This guideline emphasizes the adjunctive role of NIBS in the rehabilitation of chronic stroke. However, due to a lack of safety data and high-quality evidence, the use of NIBS was not recommended during the acute phase (57).
Clusters #0 and #1 constitute the core axis of the technical methodology. TMS serves as a fundamental tool for neuromodulation, concentrating its research on the mechanisms of cortical excitability regulation, whereas rTMS is primarily concerned with the optimization of therapeutic parameters. It is worth noting that rTMS was the most popular non-invasive brain stimulation technique in recent years. It may be attributed to the fact that rTMS can deliver repetitive, continuous and regular stimulation to effectively excite more horizontally oriented neurons. A recent meta-analysis demonstrated that rTMS significantly alleviated various dysfunctions after stroke and improved the quality of life for patients, and suggested that clinicians should incorporate it into rehabilitation protocol for post-stroke individuals (58). Some scholars have concentrated on the stimulation modes of rTMS. A qualitative systematic review indicated that rTMS applied to the injured hemisphere can ameliorate post-stroke motor deficits, but its parameters remain uncertain (59). Several clinical studies have found that both high-frequency repetitive transcranial magnetic stimulation (HF-rTMS) and low-frequency rTMS (LF-rTMS) can modulate the excitability of the cerebral motor cortex, thereby improving the motor function in the early stage of stroke, and HF-rTMS was more effective than LF- rTMS (60–62). In post-stroke aphasia, the LF-rTMS had a more immediate effect (63, 64). Thus, it is evident that the selection of rTMS modes is closely associated with the site of injury, the type of disorder and the stage of the disease. Furthermore, there is an urgent need for large-scale, high-quality and long-term studies to explore the optimal parameters of rTMS in stroke treatment. A Canadian multidisciplinary team established the CanStim platform and formulated expert consensus on rTMS for upper limb motor dysfunction after stroke. They proposed a standardized framework for clinical trials that emphasizes precise targeting, strict blinding, and multi-dimensional outcome assessments. This work has also laid the foundation for the standardized and normative clinical application and evidence-based transformation of rTMS (65).
While the development of TES has lagged behind that of rTMS, the studies of tDCS for stroke continue to rise. Compared to rTMS, tDCS is characterized by its cost-effectiveness, convenience, and ease of administration, which positions it as a promising adjunctive therapy for stroke rehabilitation. Guidelines have been established to provide technical implementation protocols for the remote application of tDCS in clinical research (66). tDCS may provide certain benefits in improving post-stroke dysfunction, however, the current evidence is insufficient to clearly delineate its therapeutic potential (19). Furthermore, the efficacy of tDCS may be influenced by various stimulation parameters, and the complex interactions among these parameters could result in inconsistent outcomes in post-stroke patients, a phenomenon akin to that observed with rTMS (67). Therefore, recent research hotspots have focused on the exploration of parameter standardization. A systematic review of parallel randomized clinical trials indicates that anodal transcranial direct current stimulation (a-tDCS) is a relatively safe intervention for patients with ischemic stroke, facilitating the recovery of upper limb function in these individuals (68). Another study has confirmed this finding and revealed that a-tDCS can improve cerebral hemodynamics in patients with cerebral ischemia (69). Furthermore, the combined application of two different NIBS techniques can enhance clinical efficacy. Preliminary findings suggested that the rTMS-tDCS may be more effective than rTMS alone (70, 71). The latest expert consensus suggests that, despite the increasing clinical practices and treatment guidelines indicating the potential therapeutic benefits of NIBS in improving post-stroke dysfunction, there are evidence-based translation barriers that limit their progress in the rehabilitation field. Simultaneously, this consensus provides a systematic framework for the application of TMS and tDCS in stroke rehabilitation, thereby accelerating the transition from laboratory to clinical practices and ultimately achieving precision and universalization in stroke rehabilitation (38).
From the clustering analysis of keywords, patients with post-stroke aphasia and hemispatial neglect were major subjects of NIBS. Post-stroke aphasia typically affects the language area of the dominant hemisphere. Commonly low-frequency rTMS in Broca’s area of the non-dominant hemisphere and tDCS of the bilateral hemispheres are applied to the treatment of post-stroke aphasia (72). Two meta-analyses reported consistently that the combination of NIBS techniques with speech training effectively enhances the speech function of patients with post-stroke aphasia (73, 74). Given that the recovery of the language network is dynamic and influenced by various factors, the underlying mechanisms of NIBS in post-stroke aphasia remain an unresolved issue. Shah P (75) proposed that a more comprehensive understanding of the potential of NIBS for post-stroke aphasia should integrate neuroimaging and electrophysiological measures.
Hemispatial neglect is a common cognitive dysfunction after stroke. Generally, the location of TMS and tDCS was mostly in the posterior parietal cortex, specifically the P3 and P4 regions of the EEG 10–20 localization system. The stimulation modes can be broadly categorized into two types: single stimulation of either the unaffected or affected cerebral hemisphere and simultaneous stimulation of both cerebral hemispheres. Some researchers have combined NIBS with feedback training or robot-assisted therapy to treat hemispatial neglect and found that it can effectively improve the relevant symptoms of patients (76–78). However, compared with post-stroke aphasia, there were relatively few studies on post-stroke hemispatial neglect, suggesting that hemispatial neglect may have the potential to become a major direction in the future.
Motor learning depends on a time-sensitive window of synaptic plasticity. NIBS directly influences the neural circuits involved in motor learning by modulating neural plasticity in specific brain regions, which holds significant value in rehabilitation, sports training, and neuroscience research. In patients of chronic stroke, the synergistic effect of NIBS combined with task-oriented training can effectively enhance clinical outcomes (79, 80). Clusters #5 and #6 indicated that current research highlights the predictive value of neuroimaging in assessing treatment response. Prior studies have utilized Arterial Spin Labeling (ASL) and Diffusion Tensor Imaging (DTI) to identify alterations in global cerebral blood flow (CBF) and fractional anisotropy (FA), investigating the effects of rTMS on motor impairment in stroke patients. The findings suggest that significant regions associated with the reorganization of motor function in the brains of stroke patients exhibit changes following rTMS intervention (81). In recent years, the development of neurophysiology and neuroimaging has become more mature. Many studies have combined NIBS with fMRI, EEG, fNIRS. It can not only monitor the neuronal activity of patients in real-time, enhance the accuracy of brain function assessment and provide precise treatment for stroke patients, but also further explore the mechanism of NIBS.
4.3 Future research trends
Future research trends in NIBS for stroke rehabilitation primarily focus on three aspects: deepening the understanding of underlying mechanisms, enhancing clinical efficacy through multimodal combined interventions, and overcoming technical challenges through multidisciplinary collaboration.
The therapeutic mechanisms of NIBS are complex and not yet fully understood, while cortical excitability and synaptic plasticity are two popular themes in NIBS mechanism research. Based on the stroke model of interactive interhemispheric inhibition, an appropriate increase in excitability of the affected side, coupled with inhibition of the healthy side, contributes to the functional recovery of the affected hemisphere (82, 83). NIBS can achieve a new balance in cortical excitability between the cerebral hemispheres and promote functional recovery after stroke (83, 84). Stroke is closely related to synaptic plasticity. Several studies have found that NIBS can induce long-term potentiation (LTP) and upregulate neuroplasticity-related proteins, such as brain-derived neurotrophic factor (BDNF) or N-methyl-d-aspartate receptor (NMDAR), which enhance plasticity of hippocampal protrusion and facilitate neurorepair (85–87). With the introduction of the brain network concept and the increasing number of related studies, researchers are beginning to recognize that stimulation generated by NIBS may exert therapeutic effects by affecting the functional (88) connectivity of brain networks (89, 90). This explains why the keyword “connectivity” was exploding from 2019 to 2024, and further exploration may bring surprising results. The ongoing development of brain networks in the field of NIBS is expected to inspire new research directions and bring promising prospects for stroke patients.
Multimodal combined interventions are poised to become a hotspot in future research. The essence of rehabilitation for neurological deficits after stroke lies in reactivating neuroplasticity and reconstructing functional networks. However, traditional single therapies often fall short of achieving comprehensive recovery due to their limited target effects and insufficient synergy. The introduction of multimodal combined interventions effectively solves the limitations of single therapies by integrating NIBS with synergistic approaches such as exercise training, virtual reality (VR), biofeedback, and acupuncture. For example, the multisensory input from VR can stimulate the cooperative functioning of multiple brain regions, including the default mode network and the dorsal attention network, while NIBS enhances task-related neural synchrony through targeted stimulation of key nodes, thereby improving motor dysfunction after stroke (91). As a method of complementary and alternative medicine, acupuncture has gained widespread acceptance in stroke rehabilitation in recent years (92). A meta-analysis revealed that the combination of acupuncture and rTMS significantly enhances motor function and daily living abilities in stroke patients (93). Although NIBS techniques are increasingly utilized in stroke rehabilitation research, their clinical effectiveness varies significantly due to several factors, including stimulation parameters, brain anatomy, lesion location, severity, genetics, and so on (94). The quantification of these factors can be enhanced through the integration of neuroimaging techniques. For example, the combination of EEG and fNIRS can provide a more accurate representation of the functional activities of brain neural networks, and new software developed from magnetic resonance technology can optimize the stimulation parameters of tDCS (95–97). The multimodal combined intervention of NIBS not only offers valuable insights into the mechanisms of stroke pathophysiology and the recovery of neurological function but also facilitates the possibility of personalized precision rehabilitation.
However, the clinical translation of multimodal combined interventions continues to face challenges. Firstly, the combined use of NIBS and neuroimaging instruments may present several issues, including magnetic field interference, radio frequency noise, current leakage, interference of patient movement, and so on (98). Secondly, the high cost of NIBS equipment and its dependence on professional operation limit its widespread adoption in primary healthcare. Therefore, interdisciplinary collaboration across neurology, rehabilitation, engineering, sociology, and geriatrics will be crucial for the development of NIBS in stroke rehabilitation, which aligns with the results of the disciplinary category analysis. Currently, researchers are continuously enhancing the development and design of technologies such as TMS-EEG, TMS-fMRI, and TMS-EEG-fMRI (99–101). However, this process still requires significant time and multidisciplinary knowledge. Furthermore, researchers strongly advocate for increased collaboration among scientists from diverse disciplinary backgrounds to provide scientific evidence supporting the efficacy of NIBS in improving clinical effects for stroke patients and reducing the burden on healthcare systems.
4.4 Limitations
This study has several limitations. Firstly, the search was confined to the WoSCC database. While the WoSCC database for high-quality bibliometric analyses is widely accepted among researchers, there are still some potentially relevant studies that were not included, which may have led to some selection bias. Secondly, the type of language was restricted to English, which may have resulted in a lack of comprehensiveness. Lastly, high-quality literature often requires time to achieve the expected citations, meaning that some recent high-quality studies may not yet have received sufficient attention. These limitations should be taken into account when interpreting the results of this study. It is anticipated that better analysis with updated and intact data will be conducted in the future to understand comprehensively the research dynamics in this field.
5 Conclusion
This study analyzed the relevant literature on NIBS for stroke from 1985 to 2024 by searching the WoSCC database and using bibliometric tools, which helps researchers understand the research dynamics in this field. NIBS technology holds great potential in stroke rehabilitation and has a wide landscape of development. Moving forward, it is essential to strengthen international cooperation between countries and conduct more high-quality and high-level studies, which will help the further advancement of this field.
Data availability statement
The original contributions presented in the study are included in the article/Supplementary material, further inquiries can be directed to the corresponding author.
Author contributions
ZL: Conceptualization, Writing – original draft. XZ: Data curation, Visualization, Writing – original draft. SX: Data curation, Software, Writing – original draft. WS: Supervision, Validation, Writing – original draft. WZ: Conceptualization, Funding acquisition, Writing – review & editing.
Funding
The author(s) declare that financial support was received for the research and/or publication of this article. This work was supported by the National Natural Science Foundation of China (Grant No. 82374596), the Hunan Provincial Key R&D program (Grant No. 2023SK2049), and the Stroke Research Center of China Traditional Chinese Medicine Inheritance and Innovation Project.
Conflict of interest
The authors declare that the research was conducted in the absence of any commercial or financial relationships that could be construed as a potential conflict of interest.
Generative AI statement
The authors declare that no Gen AI was used in the creation of this manuscript.
Publisher’s note
All claims expressed in this article are solely those of the authors and do not necessarily represent those of their affiliated organizations, or those of the publisher, the editors and the reviewers. Any product that may be evaluated in this article, or claim that may be made by its manufacturer, is not guaranteed or endorsed by the publisher.
Supplementary material
The Supplementary material for this article can be found online at: https://www.frontiersin.org/articles/10.3389/fneur.2025.1540405/full#supplementary-material
Footnotes
References
1. Stinear, CM, Lang, CE, Zeiler, S, and Byblow, WD. Advances and challenges in stroke rehabilitation. Lancet Neurol. (2020) 19:348–60. doi: 10.1016/S1474-4422(19)30415-6
2. GBD 2019 Stroke Collaborators. Global, regional, and national burden of stroke and its risk factors, 1990-2019: a systematic analysis for the global burden of disease study 2019. Lancet Neurol. (2021) 20:795–820. doi: 10.1016/S1474-4422(21)00252-0
3. Xie, X, Wang, L, Dong, S, Ge, S, and Zhu, T. Immune regulation of the gut-brain axis and lung-brain axis involved in ischemic stroke. Neural Regen Res. (2024) 19:519–28. doi: 10.4103/1673-5374.380869
4. Ji, C, Ge, X, Zhang, J, and Tong, H. The stroke burden in China and its Long-term trends: insights from the global burden of disease (GBD) study 1990-2021. Nutr Metab Cardiovasc Dis. (2025) 103848:103848. doi: 10.1016/j.numecd.2025.103848
5. Liu, L, Chen, W, Zhou, H, Duan, W, Li, S, Huo, X, et al. Chinese Stroke Association guidelines for clinical management of cerebrovascular disorders: executive summary and 2019 update of clinical management of ischaemic cerebrovascular diseases. Stroke Vasc Neurol. (2020) 5:159–76. doi: 10.1136/svn-2020-000378
6. Peng, B, Mohammed, FS, Tang, X, Liu, J, Sheth, KN, and Zhou, J. Nanotechnology approaches to drug delivery for the treatment of ischemic stroke. Bioact Mater. (2025) 43:145–61. doi: 10.1016/j.bioactmat.2024.09.016
7. Denison, T, and Morrell, MJ. Neuromodulation in 2035: the neurology future forecasting series. Neurology. (2022) 98:65–72. doi: 10.1212/WNL.0000000000013061
8. Camacho-Conde, JA, Gonzalez-Bermudez, MDR, Carretero-Rey, M, and Khan, ZU. Brain stimulation: a therapeutic approach for the treatment of neurological disorders. CNS Neurosci Ther. (2022) 28:5–18. doi: 10.1111/cns.13769
9. Yang, T, Liu, W, He, J, Gui, C, Meng, L, Xu, L, et al. The cognitive effect of non-invasive brain stimulation combined with cognitive training in Alzheimer's disease and mild cognitive impairment: a systematic review and meta-analysis. Alzheimers Res Ther. (2024) 16:140. doi: 10.1186/s13195-024-01505-9
10. Kumar, M, Kumar, A, Sindhu, RK, and Kushwah, AS. Arbutin attenuates monosodium L-glutamate induced neurotoxicity and cognitive dysfunction in rats. Neurochem Int. (2021) 151:105217. doi: 10.1016/j.neuint.2021.105217
11. Buetefisch, CM, Wei, L, Gu, X, Epstein, CM, and Yu, SP. Neuroprotection of low-frequency repetitive transcranial magnetic stimulation after ischemic stroke in rats. Ann Neurol. (2023) 93:336–47. doi: 10.1002/ana.26509
12. Zhao, Q, Wang, J, Li, Z, Song, L, and Li, X. Effect of anodic transcranial direct current stimulation combined with speech language therapy on nonfluent Poststroke aphasia. Neuromodulation. (2021) 24:923–9. doi: 10.1111/ner.13337
13. Zhang, K, Guo, L, Zhang, J, Rui, G, An, G, Zhou, Y, et al. tDCS accelerates the rehabilitation of MCAO-induced motor function deficits via neurogenesis modulated by the Notch1 signaling pathway. Neurorehabil Neural Repair. (2020) 34:640–51. doi: 10.1177/1545968320925474
14. Zong, X, Gu, J, Zhou, S, Ding, D, Hu, Y, Tucker, L, et al. Continuous theta-burst stimulation enhances and sustains neurogenesis following ischemic stroke. Theranostics. (2022) 12:5710–26. doi: 10.7150/thno.71832
15. Li, XY, Hu, R, Lou, TX, Liu, Y, and Ding, L. Global research trends in transcranial magnetic stimulation for stroke (1994-2023): promising, yet requiring further practice. Front Neurol. (2024) 15:1424545. doi: 10.3389/fneur.2024.1424545
16. Li, C, Tu, S, Xu, S, Zhang, Y, Yan, Z, Jia, J, et al. Research hotspots and Frontiers of transcranial direct current stimulation in stroke: a bibliometric analysis. Brain Sci. (2022) 13:15. doi: 10.3390/brainsci13010015
17. Devi, M. Exploring research trends and focal points in the application of transcranial magnetic stimulation for enhancing motor function in post-stroke patients: a bibliometric and content analytical approach. Injury. (2023) 54:111116. doi: 10.1016/j.injury.2023.111116
18. Zhou, K, Zhou, Y, Zeng, Y, Zhang, J, Cai, X, Qin, J, et al. Research hotspots and global trends of transcranial direct current stimulation in stroke: a bibliometric analysis. Neuropsychiatr Dis Treat. (2023) 19:601–13. doi: 10.2147/NDT.S400923
19. Juhi, A, Gayen, RK, Das, M, Goyal, C, Sharma, S, Khan, MA, et al. Exploring the research landscape of transcranial direct current stimulation in stroke: a bibliometric review. Cureus. (2024) 16:e76510. doi: 10.7759/cureus.76510
20. Wang, N, Kong, JQ, Bai, N, Zhang, HY, and Yin, M. Psychological interventions for depression in children and adolescents: a bibliometric analysis. World J Psychiatry. (2024) 14:467–83. doi: 10.5498/wjp.v14.i3.467
21. Ren, D, Lee, B, and Brehmer, M. Charticulator: interactive construction of bespoke chart layouts. IEEE Trans Vis Comput Graph. (2018) 25:789–99. doi: 10.1109/TVCG.2018.2865158
22. van Eck, NJ, and Waltman, L. Software survey: VOSviewer, a computer program for bibliometric mapping. Scientometrics. (2010) 84:523–38. doi: 10.1007/s11192-009-0146-3
23. Chen, C, and Song, M. Visualizing a field of research: a methodology of systematic scientometric reviews. PLoS One. (2019) 14:e0223994. doi: 10.1371/journal.pone.0223994
24. Hirsch, JE. An index to quantify an individual's scientific research output. Proc Natl Acad Sci USA. (2005) 102:16569–72. doi: 10.1073/pnas.0507655102
25. Zhao, ML, Lu, ZJ, Yang, L, Ding, S, Gao, F, Liu, YZ, et al. The cardiovascular system at high altitude: a bibliometric and visualization analysis. World J Cardiol. (2024) 16:199–214. doi: 10.4330/wjc.v16.i4.199
26. Wang, J, Cao, K, Chen, Z, Lyu, T, Xia, Q, Liu, L, et al. Research trends and hotspots of acupuncture therapy for obesity from 2004 to 2023: a bibliometric analysis. Complement Ther Med. (2024) 86:103092. doi: 10.1016/j.ctim.2024.103092
27. Barker, AT, Jalinous, R, and Freeston, IL. Non-invasive magnetic stimulation of human motor cortex. Lancet. (1985) 1:1106–7. doi: 10.1016/S0140-6736(85)92413-4
28. Shibasaki, H. Recent advances in non-invasive studies of higher brain functions. Brain Dev. (1993) 15:423–7. doi: 10.1016/0387-7604(93)90081-I
29. Pascual-Leone, A, Bartres-Faz, D, and Keenan, JP. Transcranial magnetic stimulation: studying the brain-behaviour relationship by induction of “virtual lesions”. Philos Trans R Soc Lond Ser B Biol Sci. (1999) 354:1229–38. doi: 10.1098/rstb.1999.0476
30. Araç, N, Sağduyu, A, Binai, S, and Ertekin, C. Prognostic value of transcranial magnetic stimulation in acute stroke. Stroke. (1994) 25:2183–6. doi: 10.1161/01.STR.25.11.2183
31. Boniface, SJ, Schubert, M, and Mills, KR. Suppression and long latency excitation of single spinal motoneurons by transcranial magnetic stimulation in health, multiple sclerosis, and stroke. Muscle Nerve. (1994) 17:642–6. doi: 10.1002/mus.880170612
32. Kukowski, B, and Haug, B. Quantitative evaluation of the silent period, evoked by transcranial magnetic stimulation during sustained muscle contraction, in normal man and in patients with stroke. Electromyogr Clin Neurophysiol. (1992) 32:373–8.
33. Kumar, KA, Murthy, J, and Ashok, KK. Motor evoked potentials by magnetic stimulation in acute ischaemic stroke: prognostic significance. Neurol India. (1995) 43:91–5.
34. Pascual-Leone, A, Valls-Solé, J, Wassermann, EM, and Hallett, M. Responses to rapid-rate transcranial magnetic stimulation of the human motor cortex. Brain. (1994) 117:847–58. doi: 10.1093/brain/117.4.847
35. Wassermann, EM, Grafman, J, Berry, C, Hollnagel, C, Wild, K, Clark, K, et al. Use and safety of a new repetitive transcranial magnetic stimulator. Electroencephalogr Clin Neurophysiol. (1996) 101:412–7. doi: 10.1016/0924-980X(96)96004-X
36. Nitsche, MA, and Paulus, W. Excitability changes induced in the human motor cortex by weak transcranial direct current stimulation. J Physiol. (2000) 527:633–9. doi: 10.1111/j.1469-7793.2000.t01-1-00633.x
37. Rossi, S, Antal, A, Bestmann, S, Bikson, M, Brewer, C, Brockmöller, J, et al. Safety and recommendations for TMS use in healthy subjects and patient populations, with updates on training, ethical and regulatory issues: expert guidelines. Clin Neurophysiol. (2021) 132:269–306. doi: 10.1016/j.clinph.2020.10.003
38. Edwards, JD, Dominguez-Vargas, AU, Rosso, C, Branscheidt, M, Sheehy, L, Quandt, F, et al. A translational roadmap for transcranial magnetic and direct current stimulation in stroke rehabilitation: consensus-based core recommendations from the third stroke recovery and rehabilitation roundtable. Int J Stroke. (2024) 19:145–57. doi: 10.1177/17474930231203982
39. Rao, J, Li, F, Zhong, L, Wang, J, Peng, Y, Liu, H, et al. Bilateral cerebellar intermittent Theta burst stimulation combined with swallowing speech therapy for dysphagia after stroke: a randomized, double-blind, sham-controlled, clinical trial. Neurorehabil Neural Repair. (2022) 36:437–48. doi: 10.1177/15459683221092995
40. Luo, J, Zheng, H, Zhang, L, Zhang, Q, Li, L, Pei, Z, et al. High-frequency repetitive transcranial magnetic stimulation (rTMS) improves functional recovery by enhancing neurogenesis and activating BDNF/TrkB signaling in ischemic rats. Int J Mol Sci. (2017) 18:455. doi: 10.3390/ijms18020455
41. Luo, J, Feng, Y, Li, M, Yin, M, Qin, F, and Hu, X. Repetitive transcranial magnetic stimulation improves neurological function and promotes the anti-inflammatory polarization of microglia in ischemic rats. Front Cell Neurosci. (2022) 16:878345. doi: 10.3389/fncel.2022.878345
42. Luo, J, Feng, Y, Hong, Z, Yin, M, Zheng, H, Zhang, L, et al. High-frequency repetitive transcranial magnetic stimulation promotes neural stem cell proliferation after ischemic stroke. Neural Regen Res. (2024) 19:1772–80. doi: 10.4103/1673-5374.389303
43. Chen, J, Zhao, F, Hong, J, Li, C, Zhang, J, Shan, Y, et al. Effect of high-frequency repetitive transcranial magnetic stimulation on swallowing function and pneumonia in poststroke dysphagia in rats. Brain Res. (2024) 1832:148846. doi: 10.1016/j.brainres.2024.148846
44. Simis, M, Imamura, M, Sampaio de Melo, P, Marduy, A, Battistella, L, and Fregni, F. Deficit of inhibition as a marker of neuroplasticity (DEFINE study) in rehabilitation: a longitudinal cohort study protocol. Front Neurol. (2021) 12:695406. doi: 10.3389/fneur.2021.695406
45. Lacerda, GJ, Pacheco-Barrios, K, Barbosa, SP, Marques, LM, Battistella, L, and Fregni, F. A neural signature for brain compensation in stroke with EEG and TMS: insights from the DEFINE cohort study. Neurophysiol Clin. (2024) 54:102985. doi: 10.1016/j.neucli.2024.102985
46. Simis, M, Thibaut, A, Imamura, M, Battistella, LR, and Fregni, F. Neurophysiological biomarkers of motor improvement from constraint-induced movement therapy and robot-assisted therapy in participants with stroke. Front Hum Neurosci. (2023) 17:1188806. doi: 10.3389/fnhum.2023.1188806
47. Franco, SB, Marques, LM, Battistella, LR, Fregni, F, and Simis, M. Seizure induced by single-pulse TMS delivered to lesioned hemisphere in stroke. J ECT. (2024) 41:e10–1. doi: 10.1097/YCT.0000000000001068
48. Corrêa, FI, Uehara, L, de Andrade, ML, da Silva, GO, De Angelis, K, Viana, A, et al. The impact of stimulation parameters on cardiovascular outcomes in chronic stroke patients following transcranial direct current stimulation-a pilot controlled, randomized, double-blind crossover trial. Biomedicines. (2024) 12:1998. doi: 10.3390/biomedicines12091998
49. Tangjade, A, Suputtitada, A, Pacheco-Barrios, K, and Fregni, F. Noninvasive neuromodulation combined with rehabilitation therapy improves balance and gait speed in patients with stroke: a systematic review and network Meta-analysis. Am J Phys Med Rehabil. (2024) 103:789–96. doi: 10.1097/PHM.0000000000002439
50. Woods, AJ, Antal, A, Bikson, M, Boggio, PS, Brunoni, AR, Celnik, P, et al. A technical guide to tDCS, and related non-invasive brain stimulation tools. Clin Neurophysiol. (2016) 127:1031–48. doi: 10.1016/j.clinph.2015.11.012
51. Antal, A, Alekseichuk, I, Bikson, M, Brockmöller, J, Brunoni, AR, Chen, R, et al. Low intensity transcranial electric stimulation: safety, ethical, legal regulatory and application guidelines. Clin Neurophysiol. (2017) 128:1774–809. doi: 10.1016/j.clinph.2017.06.001
52. Murase, N, Duque, J, Mazzocchio, R, and Cohen, LG. Influence of interhemispheric interactions on motor function in chronic stroke. Ann Neurol. (2004) 55:400–9. doi: 10.1002/ana.10848
53. Di Pino, G, Pellegrino, G, Assenza, G, Capone, F, Ferreri, F, Formica, D, et al. Modulation of brain plasticity in stroke: a novel model for neurorehabilitation. Nat Rev Neurol. (2014) 10:597–608. doi: 10.1038/nrneurol.2014.162
54. Hummel, FC, and Cohen, LG. Non-invasive brain stimulation: a new strategy to improve neurorehabilitation after stroke? Lancet Neurol. (2006) 5:708–12. doi: 10.1016/S1474-4422(06)70525-7
55. Lefaucheur, JP, Aleman, A, Baeken, C, Benninger, DH, Brunelin, J, Di Lazzaro, V, et al. Evidence-based guidelines on the therapeutic use of repetitive transcranial magnetic stimulation (rTMS): An update (2014-2018). Clin Neurophysiol. (2020) 131:474–528. doi: 10.1016/j.clinph.2019.11.002
56. Eapen, BC, Tran, J, Ballard-Hernandez, J, Buelt, A, Hoppes, CW, Matthews, C, et al. Stroke rehabilitation: synopsis of the 2024 U.S. Department of Veterans Affairs and U.S. Department of Defense clinical practice guidelines. Ann Intern Med. (2025) 178:249–68. doi: 10.7326/ANNALS-24-02205
57. Winstein, CJ, Stein, J, Arena, R, Bates, B, Cherney, LR, Cramer, SC, et al. Guidelines for adult stroke rehabilitation and recovery: a guideline for healthcare professionals from the American Heart Association/American Stroke Association. Stroke. (2016) 47:e98–e169. doi: 10.1161/STR.0000000000000098
58. Duan, X, Huang, D, Zhong, H, Wu, J, Xiao, Z, Yang, P, et al. Efficacy of rTMS in treating functional impairment in post-stroke patients: a systematic review and meta-analysis. Neurol Sci. (2024) 45:3887–99. doi: 10.1007/s10072-024-07455-2
59. Hiscock, A, Miller, S, Rothwell, J, Tallis, RC, and Pomeroy, VM. Informing dose-finding studies of repetitive transcranial magnetic stimulation to enhance motor function: a qualitative systematic review. Neurorehabil Neural Repair. (2008) 22:228–49. doi: 10.1177/1545968307307115
60. Sasaki, N, Mizutani, S, Kakuda, W, and Abo, M. Comparison of the effects of high- and low-frequency repetitive transcranial magnetic stimulation on upper limb hemiparesis in the early phase of stroke. J Stroke Cerebrovasc Dis. (2013) 22:413–8. doi: 10.1016/j.jstrokecerebrovasdis.2011.10.004
61. Sasaki, N, Kakuda, W, and Abo, M. Bilateral high- and low-frequency rTMS in acute stroke patients with hemiparesis: a comparative study with unilateral high-frequency rTMS. Brain Inj. (2014) 28:1682–6. doi: 10.3109/02699052.2014.947626
62. Du, J, Yang, F, Hu, J, Hu, J, Xu, Q, Cong, N, et al. Effects of high- and low-frequency repetitive transcranial magnetic stimulation on motor recovery in early stroke patients: evidence from a randomized controlled trial with clinical, neurophysiological and functional imaging assessments. Neuroimage Clin. (2019) 21:101620. doi: 10.1016/j.nicl.2018.101620
63. Li, T, Zeng, X, Lin, L, Xian, T, and Chen, Z. Effects of repetitive transcranial magnetic stimulation with different frequencies on post-stroke aphasia: a PRISMA-compliant meta-analysis. Medicine. (2020) 99:e20439. doi: 10.1097/MD.0000000000020439
64. Hu, XY, Zhang, T, Rajah, GB, Stone, C, Liu, LX, He, JJ, et al. Effects of different frequencies of repetitive transcranial magnetic stimulation in stroke patients with non-fluent aphasia: a randomized, sham-controlled study. Neurol Res. (2018) 40:459–65. doi: 10.1080/01616412.2018.1453980
65. Edwards, JD, Black, SE, Boe, S, Boyd, L, Chaves, A, Chen, R, et al. Canadian platform for trials in noninvasive brain stimulation (CanStim) consensus recommendations for repetitive transcranial magnetic stimulation in upper extremity motor stroke rehabilitation trials. Neurorehabil Neural Repair. (2021) 35:103–16. doi: 10.1177/1545968320981960
66. Charvet, LE, Kasschau, M, Datta, A, Knotkova, H, Stevens, MC, Alonzo, A, et al. Remotely-supervised transcranial direct current stimulation (tDCS) for clinical trials: guidelines for technology and protocols. Front Syst Neurosci. (2015) 9:26. doi: 10.3389/fnsys.2015.00026
67. Lee, SH, and Yoo, YJ. A literature review on optimal stimulation parameters of transcranial direct current stimulation for motor recovery after stroke. Brain Neurorehabil. (2024) 17:e24. doi: 10.12786/bn.2024.17.e24
68. Yu, L, Chen, H, Chen, C, Lin, Y, Huang, Z, Wang, J, et al. Efficacy of anodal transcranial direct current stimulation for upper extremity function after ischemic stroke: a systematic review of parallel randomized clinical trials. J Stroke Cerebrovasc Dis. (2025) 34:108112. doi: 10.1016/j.jstrokecerebrovasdis.2024.108112
69. Gunduz, ME, Kocahasan, M, and Keser, Z. Transcranial direct current stimulation to provide neuroprotection and enhance cerebral blood flow in stroke: a comprehensive review. Medicina (Kaunas). (2024) 60:2061. doi: 10.3390/medicina60122061
70. Hu, AM, Huang, CY, He, JG, and Wu, L. Effect of repetitive transcranial magnetic stimulation combined with transcranial direct current stimulation on post-stroke dysmnesia: a preliminary study. Clin Neurol Neurosurg. (2023) 231:107797. doi: 10.1016/j.clineuro.2023.107797
71. Gong, Y, Long, XM, Xu, Y, Cai, XY, and Ye, M. Effects of repetitive transcranial magnetic stimulation combined with transcranial direct current stimulation on motor function and cortex excitability in subacute stroke patients: a randomized controlled trial. Clin Rehabil. (2021) 35:718–27. doi: 10.1177/0269215520972940
72. Williams, EER, Sghirripa, S, Rogasch, NC, Hordacre, B, and Attrill, S. Non-invasive brain stimulation in the treatment of post-stroke aphasia: a scoping review. Disabil Rehabil. (2024) 46:3802–26. doi: 10.1080/09638288.2023.2259299
73. Chai, L, Huang, Y, Guo, X, Xiong, A, Lin, B, and Huang, J. Does SLT combined with NIBS enhance naming recovery in post-stroke aphasia? A meta-analysis and systematic review. NeuroRehabilitation. (2024) 54:543–61. doi: 10.3233/NRE-240065
74. Han, C, Tang, J, Tang, B, Han, T, Pan, J, and Wang, N. The effectiveness and safety of noninvasive brain stimulation technology combined with speech training on aphasia after stroke: a systematic review and meta-analysis. Medicine. (2024) 103:e36880. doi: 10.1097/MD.0000000000036880
75. Shah, PP, Szaflarski, JP, Allendorfer, J, and Hamilton, RH. Induction of neuroplasticity and recovery in post-stroke aphasia by non-invasive brain stimulation. Front Hum Neurosci. (2013) 7:888. doi: 10.3389/fnhum.2013.00888
76. Làdavas, E, Giulietti, S, Avenanti, A, Bertini, C, Lorenzini, E, Quinquinio, C, et al. A-tDCS on the ipsilesional parietal cortex boosts the effects of prism adaptation treatment in neglect. Restor Neurol Neurosci. (2015) 33:647–62. doi: 10.3233/RNN-140464
77. Bang, DH, and Bong, SY. Effect of combination of transcranial direct current stimulation and feedback training on visuospatial neglect in patients with subacute stroke: a pilot randomized controlled trial. J Phys Ther Sci. (2015) 27:2759–61. doi: 10.1589/jpts.27.2759
78. Kim, SB, Lee, KW, Lee, JH, Lee, SJ, Park, JG, and Lee, JB. Effect of combined therapy of robot and low-frequency repetitive transcranial magnetic stimulation on Hemispatial neglect in stroke patients. Ann Rehabil Med. (2018) 42:788–97. doi: 10.5535/arm.2018.42.6.788
79. Wang, RY, Wang, FY, Huang, SF, and Yang, YR. High-frequency repetitive transcranial magnetic stimulation enhanced treadmill training effects on gait performance in individuals with chronic stroke: a double-blinded randomized controlled pilot trial. Gait Posture. (2019) 68:382–7. doi: 10.1016/j.gaitpost.2018.12.023
80. Abdullahi, A, Wong, TW, Van Criekinge, T, and Ng, SS. Combination of noninvasive brain stimulation and constraint-induced movement therapy in patients with stroke: a systematic review and meta-analysis. Expert Rev Neurother. (2023) 23:187–203. doi: 10.1080/14737175.2023.2177154
81. Jin, Y, Bai, X, Jiang, B, Guo, Z, and Mu, Q. Repetitive transcranial magnetic stimulation induces quantified functional and structural changes in subcortical stroke: a combined arterial spin labeling perfusion and diffusion tensor imaging study. Front Hum Neurosci. (2022) 16:829688. doi: 10.3389/fnhum.2022.829688
82. Gerges, ANH, Hordacre, B, Pietro, FD, Moseley, GL, and Berryman, C. Do adults with stroke have altered interhemispheric inhibition? A systematic review with Meta-analysis. J Stroke Cerebrovasc Dis. (2022) 31:106494. doi: 10.1016/j.jstrokecerebrovasdis.2022.106494
83. Dionísio, A, Duarte, IC, Patrício, M, and Castelo-Branco, M. The use of repetitive transcranial magnetic stimulation for stroke rehabilitation: a systematic review. J Stroke Cerebrovasc Dis. (2018) 27:1–31. doi: 10.1016/j.jstrokecerebrovasdis.2017.09.008
84. Rodríguez, A, Amaya-Pascasio, L, Gutiérrez-Fernández, M, García-Pinteño, J, Moreno, M, and Martínez-Sánchez, P. Non-invasive brain stimulation for functional recovery in animal models of stroke: a systematic review. Neurosci Biobehav Rev. (2024) 156:105485. doi: 10.1016/j.neubiorev.2023.105485
85. Ranieri, F, Podda, MV, Riccardi, E, Frisullo, G, Dileone, M, Profice, P, et al. Modulation of LTP at rat hippocampal CA3-CA1 synapses by direct current stimulation. J Neurophysiol. (2012) 107:1868–80. doi: 10.1152/jn.00319.2011
86. Di Lazzaro, V, Pellegrino, G, Di Pino, G, Corbetto, M, Ranieri, F, Brunelli, N, et al. Val66Met BDNF gene polymorphism influences human motor cortex plasticity in acute stroke. Brain Stimul. (2015) 8:92–6. doi: 10.1016/j.brs.2014.08.006
87. Zhao, CG, Sun, W, Ju, F, Jiang, S, Wang, H, Sun, XL, et al. Analgesic effects of navigated repetitive transcranial magnetic stimulation in patients with acute central Poststroke pain. Pain Ther. (2021) 10:1085–100. doi: 10.1007/s40122-021-00261-0
88. Subramanian, SK, and Prasanna, SS. Virtual reality and noninvasive brain stimulation in stroke: how effective is their combination for upper limb motor improvement?-a Meta-analysis. PM R. (2018) 10:1261–70. doi: 10.1016/j.pmrj.2018.10.001
89. Han, Y, Jing, Y, Shi, Y, Mo, H, Wan, Y, Zhou, H, et al. The role of language-related functional brain regions and white matter tracts in network plasticity of post-stroke aphasia. J Neurol. (2024) 271:3095–115. doi: 10.1007/s00415-024-12358-5
90. Olgiati, E, Violante, IR, Xu, S, Sinclair, TG, Li, LM, Crow, JN, et al. Targeted non-invasive brain stimulation boosts attention and modulates contralesional brain networks following right hemisphere stroke. Neuroimage Clin. (2024) 42:103599. doi: 10.1016/j.nicl.2024.103599
91. Llorens, R, Fuentes, MA, Borrego, A, Latorre, J, Alcañiz, M, Colomer, C, et al. Effectiveness of a combined transcranial direct current stimulation and virtual reality-based intervention on upper limb function in chronic individuals post-stroke with persistent severe hemiparesis: a randomized controlled trial. J Neuroeng Rehabil. (2021) 18:108. doi: 10.1186/s12984-021-00896-2
92. Zhang, J, Ji, C, Zhai, X, Ren, S, and Tong, H. Global trends and hotspots in research on acupuncture for stroke: a bibliometric and visualization analysis. Eur J Med Res. (2023) 28:359. doi: 10.1186/s40001-023-01253-w
93. Xie, Y, Pan, J, Chen, J, Zhang, D, and Jin, S. Acupuncture combined with repeated transcranial magnetic stimulation for upper limb motor function after stroke: a systematic review and meta-analysis. NeuroRehabilitation. (2023) 53:423–38. doi: 10.3233/NRE-230144
94. Li, LM, Uehara, K, and Hanakawa, T. The contribution of interindividual factors to variability of response in transcranial direct current stimulation studies. Front Cell Neurosci. (2015) 9:181. doi: 10.3389/fncel.2015.00181
95. Berger, A, Horst, F, Müller, S, Steinberg, F, and Doppelmayr, M. Current state and future prospects of EEG and fNIRS in robot-assisted gait rehabilitation: a brief review. Front Hum Neurosci. (2019) 13:172. doi: 10.3389/fnhum.2019.00172
96. Yoo, YJ, Park, HJ, Kim, TY, Yoon, MJ, Oh, HM, Lee, YJ, et al. MRI-based personalized transcranial direct current stimulation to enhance the upper limb function in patients with stroke: study protocol for a double-blind randomized controlled trial. Brain Sci. (2022) 12:1673. doi: 10.3390/brainsci12121673
97. Auriat, AM, Neva, JL, Peters, S, Ferris, JK, and Boyd, LA. A review of transcranial magnetic stimulation and multimodal neuroimaging to characterize post-stroke neuroplasticity. Front Neurol. (2015) 6:226. doi: 10.3389/fneur.2015.00226
98. Bohning, DE, Shastri, A, Nahas, Z, Lorberbaum, JP, Andersen, SW, Dannels, WR, et al. Echoplanar BOLD fMRI of brain activation induced by concurrent transcranial magnetic stimulation. Investig Radiol. (1998) 33:336–40. doi: 10.1097/00004424-199806000-00004
99. Hernandez-Pavon, JC, Veniero, D, Bergmann, TO, Belardinelli, P, Bortoletto, M, Casarotto, S, et al. TMS combined with EEG: recommendations and open issues for data collection and analysis. Brain Stimul. (2023) 16:567–93. doi: 10.1016/j.brs.2023.02.009
100. Mizutani-Tiebel, Y, Tik, M, Chang, KY, Padberg, F, Soldini, A, Wilkinson, Z, et al. Concurrent TMS-fMRI: technical challenges, developments, and overview of previous studies. Front Psych. (2022) 13:825205. doi: 10.3389/fpsyt.2022.825205
101. Peters, JC, Reithler, J, Graaf, TA, Schuhmann, T, Goebel, R, and Sack, AT. Concurrent human TMS-EEG-fMRI enables monitoring of oscillatory brain state-dependent gating of cortico-subcortical network activity. Commun Biol. (2020) 3:40. doi: 10.1038/s42003-020-0764-0
102. Rossi, S, Hallett, M, Rossini, PM, and Pascual-Leone, A. Safety, ethical considerations, and application guidelines for the use of transcranial magnetic stimulation in clinical practice and research. Clinical neurophysiology: official journal of the International Federation of Clinical Neurophysiology. (2009) 120:2008–39. doi: 10.1016/j.clinph.2009.08.016
103. Hummel, F, Celnik, P, Giraux, P, Floel, A, Wu, WH, Gerloff, C, et al. Effects of non-invasive cortical stimulation on skilled motor function in chronic stroke. Brain: a journal of neurology. (2005) 128:490–9. doi: 10.1093/brain/awh369
104. Rossini, PM, Barker, AT, Berardelli, A, Caramia, MD, Caruso, G, Cracco, RQ, et al. Non-invasive electrical and magnetic stimulation of the brain, spinal cord and roots: basic principles and procedures for routine clinical application. Report of an Ifcn Committee. Electroencephalography and clinical neurophysiology. (1994) 91:79–92. doi: 10.1016/0013-4694(94)90029-9
105. Huang, YZ, Edwards, MJ, Rounis, E, Bhatia, KP, and Rothwell, JC. Theta burst stimulation of the human motor cortex. Neuron. (2005) 45:201–6. doi: 10.1016/j.neuron.2004.12.033
106. Nitsche, MA, and Paulus, W. Sustained excitability elevations induced by transcranial dc motor cortex stimulation in humans. Neurology. (2001) 57:1899–901. doi: 10.1212/wnl.57.10.1899
Keywords: stroke, non-invasive brain stimulation, rehabilitation, bibliometric analysis, visualization
Citation: Li Z, Zhao X, Xie S, Shi W and Zhang W (2025) Emerging trends and research hotspots of non-invasive brain stimulation for stroke: a bibliometric and visualization study. Front. Neurol. 16:1540405. doi: 10.3389/fneur.2025.1540405
Edited by:
Maria Concetta Pellicciari, Libera Università Maria SS. Assunta University, ItalyReviewed by:
Xiaolong Sun, Air Force Medical University, ChinaXu Zhai, China Academy of Chinese Medical Sciences, China
Copyright © 2025 Li, Zhao, Xie, Shi and Zhang. This is an open-access article distributed under the terms of the Creative Commons Attribution License (CC BY). The use, distribution or reproduction in other forums is permitted, provided the original author(s) and the copyright owner(s) are credited and that the original publication in this journal is cited, in accordance with accepted academic practice. No use, distribution or reproduction is permitted which does not comply with these terms.
*Correspondence: Wei Zhang, emhhbmd3ZWlAaG51Y20uZWR1LmNu
†ORCID: Wei Zhang, orcid.org/0009-0000-6900-7209