- 1Department of Psychiatry, Chang Gung Memorial Hospital at Linkou, Linkou, Taiwan
- 2Graduate Institute of Clinical Medicine, National Taiwan University College of Medicine, Taipei, Taiwan
- 3Department of Neurology, Neuroscience Research Center, Chang Gung Memorial Hospital at Linkou, Taipei, Taiwan
- 4Department of Medical Imaging and Intervention, Chang Gung Memorial Hospital at Linkou, Taipei, Taiwan
- 5Graduate Institute of Clinical Medical Science, Chang Gung University, Taoyuan, Taiwan
- 6Clinical Informatics and Medical Statistics Research Center, Chang Gung University, Taoyuan, Taiwan
- 7Research Services Center for Health Information, Chang Gung University, Taoyuan, Taiwan
- 8Medical School, Chang Gung University, Taoyuan, Taiwan
- 9Institute of Cognitive Neuroscience, National Central University, Taoyuan, Taiwan
Intermittent theta burst stimulation (iTBS), a patterned repetitive transcranial magnetic stimulation, was applied over the posterior superior temporal sulcus (pSTS) or dorsolateral prefrontal cortex (DLPFC) to explore its impact in adults with autism spectrum disorder (ASD). Among 25 adults with ASD, 19 (mean age: 20.8 years) completed the randomized, sham-controlled, crossover trial. Every participant received iTBS over the bilateral DLPFC, bilateral pSTS and inion (as a sham control stimulation) in a randomized order with a 1-week interval. Neuropsychological functions were assessed using the Conners' Continuous Performance Test (CCPT) and the Wisconsin Card Sorting Test (WCST). Behavioral outcomes were measured using the Yale-Brown Obsessive Compulsive Scale (Y-BOCS) and the Social Responsiveness Scale (SRS). In comparison to that in the sham stimulation, the reaction time in the CCPT significantly decreased following single DLPFC session (p = 0.04, effect size = 0.71) while there were no significant differences in the CCPT and WCST following single pSTS session. Besides, the results in behavioral outcomes were inconsistent and had discrepancy between reports of parents and patients. In conclusion, a single session of iTBS over the bilateral DLPFC may alter the neuropsychological function in adults with ASD. The impacts of multiple-sessions iTBS over the DLPFC or pSTS deserve further investigations.
Introduction
Autism spectrum disorder (ASD) is characterized by an early onset of difficulties with social-communication, and repetitive, restricted, stereotyped behaviors, and interests (Lai et al., 2014). Although the precise etiology of ASD is not conclusive, abnormalities in regional brain volumes (Nickl-Jockschat et al., 2012), patterns of brain perfusion (Ohnishi et al., 2000), neural biochemical characteristics of ASD (Baruth et al., 2013), and excitatory/inhibitory neurotransmission (LeBlanc and Fagiolini, 2011) have been reported. Unfortunately, there are limited biological interventions available to treat ASD.
Repetitive transcranial magnetic stimulation (rTMS), a non-invasive technique of repetitive stimulation of the neural circuits, is capable of producing long-lasting changes in cortical excitability beyond the period of stimulation (Fang et al., 2010; Pell et al., 2011). Although the precise mechanism of action of rTMS is still unclear, recent studies found that rTMS can induce changes similar to long-term potentiation (LTP) or long-term depression (LTD) via modulation of synaptic plasticity (Huang et al., 2007; Pell et al., 2011). Recently, rTMS has shown some potential clinical benefits in several psychiatric disorders such as major depressive disorder (Gaynes et al., 2014), schizophrenia (Rajji et al., 2013), post-traumatic stress disorder (Cohen et al., 2004; Watts et al., 2012), and ASD (Enticott et al., 2012, 2014).
The application of rTMS in ASD has been studied recently. Several neurobiological targets, including the dorsolateral prefrontal cortex (DLPFC), medial prefrontal cortex, dorso-medial prefrontal cortex and Broca's area, have been studied (Sokhadze et al., 2009, 2010, 2012; Baruth et al., 2010; Enticott et al., 2011, 2012, 2014; Fecteau et al., 2011). Among these, rTMS over the DLPFC has been investigated the most. Studies have investigated the therapeutic effect of rTMS over the DLPFC using several different designs, such as changes in the stimulation site (left, right, or bilateral), stimulation frequency (from once per week to twice per week), total number of stimulation sessions (1–18) and protocol frequency (0.5–5 Hz) (Oberman et al., 2016). These studies demonstrated that low-frequency rTMS over the DLPFC might restore the excitatory/inhibitory imbalance and improve the repetitive behaviors observed in ASD. However, the social impairments observed in ASD did not change with these interventions (Baruth et al., 2010; Sokhadze et al., 2010, 2014; Casanova et al., 2014).
Another potential target of rTMS for ASD is the posterior superior temporal sulcus (pSTS). The pSTS was first found to be involved in the process of biological motion (Puce and Perrett, 2003) and a further important role for it has been demonstrated in social perception (Zilbovicius et al., 2006; Redcay, 2008). Atypical activation patterns of the pSTS in ASD have been reported in several social experiments (Mason et al., 2008; Redcay et al., 2013). Moreover, studies on cerebral blood flow revealed hypoperfusion of the bilateral STS in sedated children with ASD (Ohnishi et al., 2000; Zilbovicius et al., 2000) and the level of hypoperfusion in the left STS was associated with the clinical severity of autism (Gendry Meresse et al., 2005).
The impact of rTMS over the pSTS has been tested in healthy adults. Grossman first found that low-frequency rTMS over the right pSTS temporarily impaired the perception of biological motion in healthy adults (Grossman et al., 2005). In addition, van Kemenade demonstrated that the sensitivity to detect biological motion marginally declined after continuous theta burst TMS over the left pSTS (van Kemenade et al., 2012). One recent fMRI study demonstrated that low-frequency rTMS over the bilateral pSTS produced remote hemodynamic effects in a network of specific brain areas, including the lateral occipital-temporal cortex, intraparietal sulcus, and ventral premotor cortex (Arfeller et al., 2013). Since these studies demonstrated that low-frequency rTMS (inhibitory protocol) over the pSTS might impair the perception of biological motion in healthy adults, it might be possible to apply an excitatory protocol over the pSTS to enhance the perception of biological motion. Therefore, it might be feasible to enhance the function of the pSTS to improve social cognition in patients with ASD (Saitovitch et al., 2016). However, to the best of our knowledge, there has so far been no study investigating the impact of rTMS over the pSTS in patients with ASD.
Theta burst stimulation (TBS), a modified protocol of rTMS, can very quickly produce an LTP- or LTD-like effect by using bursts at the same frequency (three pulses at 50 Hz, repeated five times per second) and intensity (Huang et al., 2005, 2007, 2011). For TBS, the direction of the after-effects depends on whether the bursts are delivered continuously (cTBS, producing LTD-like effects, inhibitory) or intermittently (iTBS, producing LTP-like effects, excitatory). In comparison to traditional rTMS, the stimulus duration is shorter in TBS (TBS: 20–240 s; rTMS: 5–30 min) (Pell et al., 2011). The shorter stimulus duration makes TBS more appropriate for ASD in clinical practice.
In this pilot study, we aimed to explore the impacts of single session TBS over the DLPFC and the pSTS in ASD. Based on the review mentioned above, the excitatory protocol (iTBS) was chosen for use in our study. In addition, bilateral cerebral hemispheres were stimulated to maximize the intervention effect. Using a randomized, crossover, and sham-controlled study design, we investigated the impact of iTBS over the bilateral DLPFC and bilateral pSTS compared to the sham-control condition. Neuropsychological function was measured using the Conner's Continuous Performance Test (CCPT) and the Wisconsin Card Sorting Test (WCST). In addition, the behavioral outcomes were measured using the Yale-Brown Obsessive Compulsive Scale (Y-BOCS) and the social responsiveness scale (SRS) in both the participants and their parents.
Materials and Methods
Subjects
We recruited participants, who were older than 18 years of age through advertisement at the outpatient clinic of the Department of Psychiatry, Chang Gung Memorial Hospital, Taiwan. People interested in our study were first interviewed by a board-certified child psychiatrist (First author). Once they and their parents agreed to join the study, several assessments were arranged. First, the diagnosis of ASD was evaluated according to the DSM-IV and ICD-10 criteria by the first author and this were further confirmed using the Chinese version of the Autism Diagnostic Interview-Revised (ADI-R) and Autism Diagnostic Observation Schedule (ADOS) by another senior board-certified child psychiatrist (Dr. Wu). Patients with a diagnosis of autistic disorder, Asperger syndrome, or pervasive developmental disorder, were included in our study. Patients with any history of systemic medical illness, seizures, severe head injury, suicide attempts, schizophrenia, bipolar affective disorder, substance abuse, pregnancy, or the presence of an implanted medical device such as a cardiac pacemaker, were excluded from the study. A total of 25 participants with ASD were enrolled in the current study. Six participants completed the baseline assessments but refused the iTBS interventions for personal reasons. The other 19 participants, aged 18–29 years old, completed the entire study. The demographic data are shown in Table 1. The participants were maintained on the same medication during the whole study period. Three of the participants (16%) took psychotropic medications (methylphenidate = 1, fluoxetine = 1, and sertraline = 1).
Study Design
This was a randomized, sham-controlled, crossover trial to investigate the impact of iTBS in ASD. The participants received iTBS over the bilateral DLPFC, bilateral pSTS and inion (as a sham control stimulation) in a randomized order, with a 1-week interval between each session. The order of randomization of the DLPFC, pSTS, and inion followed the rules of William design with an order of 3 (6 sequences). The social and repetitive behaviors were assessed immediately before, 8 h and 2 days after the interventions. Neuropsychological functions were assessed before and after (within 1 h) the intervention.
The Research Ethics Committee at the Chang Gung Memorial Hospital approved this study before its implementation. The procedures and purpose of our study were explained face-to-face to the participants and their parents, who then provided written informed consent.
Target Identification
The scans were collected on a 3T magnetic resonance imaging (MRI) scanner (Trio, Siemens Medical Solutions, Erlangen, Germany), using a 12-channel head coil. A high resolution 3D-MPRAGE sequence for anatomic localization was acquired using the following parameters: TR = 2,250 ms, TE = 2.6 ms, TI = 900 ms, FOV = 240 mm, Flip Angle = 9°, matrix = 240 × 256, voxel size = 1.0 × 1.0 × 1.0 mm. To minimize movement artifacts, the head of every subject was firmly fixed with pads. All images were examined to ensure that they were free of motion and artifacts at the time of image acquisition.
We derived the iTBS sites for each of our individual participants using the same normalized coordinates. The sites of the left and right pSTS were based on the study by Van Overwalle and Baetens (2009), who analyzed 200 functional MRI (fMRI) studies and reported the averaged pSTS coordinates on the Talairach atlas (±50, −55, 10). The final Montreal Neurological Institute (MNI) coordinates of the pSTS were ±50.5, −57.1, 7.9 (for conversion from the coordinates on the Talairach atlas to those on the MNI template, refer to http://imaging.mrc-cbu.cam.ac.uk/imaging/MniTalairach). The sites of the left and right DLPFC (MNI coordinates: −41.9, 35.1, 33.7; 39.9, 36.8, 33.9, respectively) were adapted from Mylius et al. (2013), where researchers first anatomically defined the DLPFC at the separating line between the anterior and middle thirds of the middle frontal gyrus, and then derived the MNI coordinates through structural normalization using 50 normal volunteers (24 men). We specifically used a subset of data from male participants here since our present experiment happened to recruit mainly male participants.
To target the normalized coordinates precisely, the T1-weighted structural image of each participant was first spatially normalized with SPM8 (http://www.fil.ion.ucl.ac.uk/spm/software/spm8) to find the transformation matrix. We then applied an inverse transformation matrix, which accepts the normalized coordinates as the input and gives the corresponding coordinates in the participant's native structural image as the output (Figure 1 shows the four stimulation sites of a representative participant and the coordinates rendered on a normalized skull-stripped brain). These output coordinates were marked on the native structural image in the Navigated Brain Stimulation (NBS) system (Nexstim®, Helsinki, Finland), which, with the aid of an infrared tracking device, can visualize the position of the TMS coil relative to the structure of the head and brain of each individual.
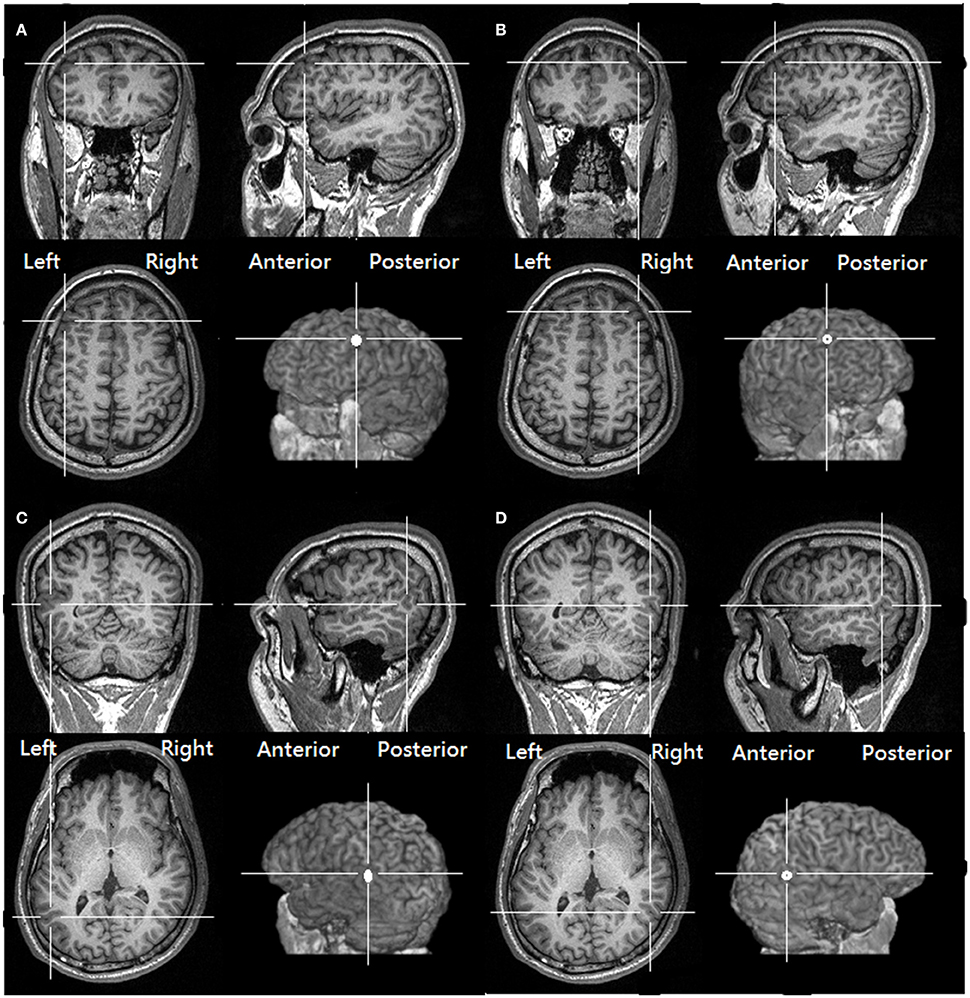
Figure 1. The targeted location over the (A) left DLPFC (B) right DLPFC (C) Left pSTS (D) right pSTS of one of our participants, as derived from transforming the same normalized MNI coordinates to the structural image of the participant (See Methods, Target identification).
Transcranial Magnetic Stimulation
Electromyography was recorded with surface electrodes from the right first dorsal interosseous muscle (FDI) using a belly-tendon montage. TMS was performed using a 70 mm figure-of-eight coil connected to a Magstim Super Rapid2 system (Magstim Company, Oxford, UK). The coil was placed over the contralateral motor cortex tangentially to the scalp with the handle pointing backward. The motor hot-spot was determined as the location on the scalp where TMS produced the largest MEP from the FDI at rest. The active motor threshold (AMT) was defined as the minimum stimulation intensity over the motor hot-spot that could elicit an MEP of no less than 200 mV in 5 out of 10 trials during voluntary contraction of the FDI.
Theta Burst Stimulation
The iTBS paradigm, which has been shown to produce long-lasting excitation of the cortex, was applied in the current study (Huang et al., 2005). Each TBS train comprised of a burst of 3 pulses at 50 Hz given 10 times every 200 ms. The TBS train was given 20 times every 10 s so that there were 600 pulses in total. In the study, two courses of iTBS separated by a 5-min break were given to the target in the left hemisphere first. Then, two other courses of iTBS were applied to the target in the right hemisphere. With regard to the inion, two courses of iTBS were given with a break of 5 min.
The stimulus intensity for the DLPFC and pSTS was set at 80% AMT of the right FDI. As for the stimulus intensity for the sham control stimulation over the inion, 60% of the AMT was applied with an 180-degree reversal of the coil (Huang et al., 2012; Chuang et al., 2014).
Neuropsychological Function Outcome Measures
The CCPT and WCST, which were used to evaluate the impact of iTBS on neuropsychological function, were completed before and within 1 h after the stimulation.
The CCPT is a non-X type CPT test, which is a Go/No-Go task lasting for 14 min (Conners et al., 2003). For the test, 360 trials, which took approximately 250 ms each, appeared on the computer screen. The participant was requested to respond by pressing the spacebar on the keyboard when a letter other than the target letter “X” appeared. The reaction times, omission errors, and commission errors were calculated to assess neuropsychological function before and after TBS.
The computerized version of the WCST: Computer Version 4 research edition (WCST: CV4) (Heaton, 2003) was used in our study. The participants were asked to choose the correct card from four categories of cards, in which the stimuli are multidimensional according to color, shape, and number and each dimension is defined by a sorting rule. By trial and error, the participant had to find the sorting rule given from the feedback (“Right” or “Wrong”) on the screen following each sort. The classification principle changed without warning following sequences of 10 consecutive correct matches. Testing continued until all 128 cards were sorted and irrespective of whether the participant completed all of the rule shifts. We measured the total errors, perseverative response (the number of incorrect responses that had been correct for the preceding category), perseveration errors (the number of errors where the participant used the same rule for their choice as the previous choice), conceptual level responses (the number of correct responses that occurred in runs of three or more divided by the number of trials and then multiplied by 100), and the number of categories completed (the number of runs of ten corrected responses).
Clinical Symptoms Outcome Measures
The Y-BOCS and SRS were used to evaluate the impact of iTBS on behavioral outcomes. The Y-BOCS and SRS were completed by participants and their parents three times, i.e., before, 8 h and 2 days after the iTBS conditioning, for every stimulation session.
The SRS is a self-report instrument that quantifies the severity of social communication deficits in ASD (Constantino, 2005). The SRS contains 65 items and can be completed by the participants or their parents in approximately 15 min. Items were rated on a 4-point Likert scale from “1” (not sure) to “4” (almost always true) and can be rated in five subscales: social awareness, social cognition, social communication, social motivation, and autistic mannerisms. Previous studies have demonstrated the good psychometric properties of the SRS in children, adolescents, and adults (Constantino, 2005; Constantino and Todd, 2005; Bolte, 2012). In addition, the psychometric properties of the Chinese version of the SRS were also investigated and they demonstrated good test–retest reliability (intra-class correlations: 0.751–0.852), internal consistency (Cronbach's alpha: 0.944–0.947), and convergent validity with the Chinese SCQ (Pearson correlations: 0.609–0.865) (Gau et al., 2013).
The Y-BOCS is a gold-standard measurement tool for symptoms severity in obsessive-compulsive disorder (Goodman et al., 1989a,b), and can be used to assess the repetitive behaviors observed in ASD (Hollander et al., 2000, 2006). The Y-BOCS can be assessed by clinicians or by self-report (Baer, 1991). The Y-BOCS consists of a comprehensive symptom checklist and a 10-item severity scale. The severity scale evaluates the recent degree of impairments in five clinical domains: time taken, functional impairment, psychological distress, efforts to resist, and perceived sense of control. Items are rated on a 5-point Likert scale (range: 0–4) and are used to generate a total Y-BOCS score and subscale scores for obsessions and compulsions. Previous studies have demonstrated the good psychometric properties of the Y-BOCS (Frost et al., 1995; Woody et al., 1995).
Statistical Analysis
The means (±standard deviation, SD) of the behavioral and neuropsychological outcomes were calculated for each iTBS intervention. The changes of outcomes were compared using a linear mixed model for repeated measures to analyze the group difference (DLPFC vs. sham, pSTS vs. sham) within one model. In the linear mixed model, we used the study periods and intervention group as fixed effects and the participants as a random effect. The t-type confidence limits were constructed for each of the fixed-effects parameter estimates. P-values were adjusted using Dunnett's test for the comparison of intervention groups and sham control. In addition, Cohen's d was used to compute the effect size on the changes from baseline for the comparison of intervention groups and sham control. A p < 0.05 was considered significant. SAS 9.3 software was used for our analysis.
Results
Neuropsychological Functions
The raw data of the neuropsychological outcomes before and after iTBS are presented in Table 2. In summary, omission errors and reaction time decreased after iTBS over the DLPFC, while commission errors increased after iTBS over the pSTS in the CCPT. As for the WCST, total errors, perseveration responses and errors decreased after the sham stimulation, but there was no obvious difference in the DLPFC and pSTS interventions.
When comparing the pSTS and DLPFC iTBS treatments to sham treatments (Table 3), the reaction time in the CCPT significantly decreased after iTBS over the DLPFC (p = 0.04, effect size = 0.71), while commission errors in the CCPT, and total errors in the WCST non-significantly increased after iTBS over the pSTS (p = 0.07, effect size = 0.79; p = 0.06, effect size = 0.65, respectively).
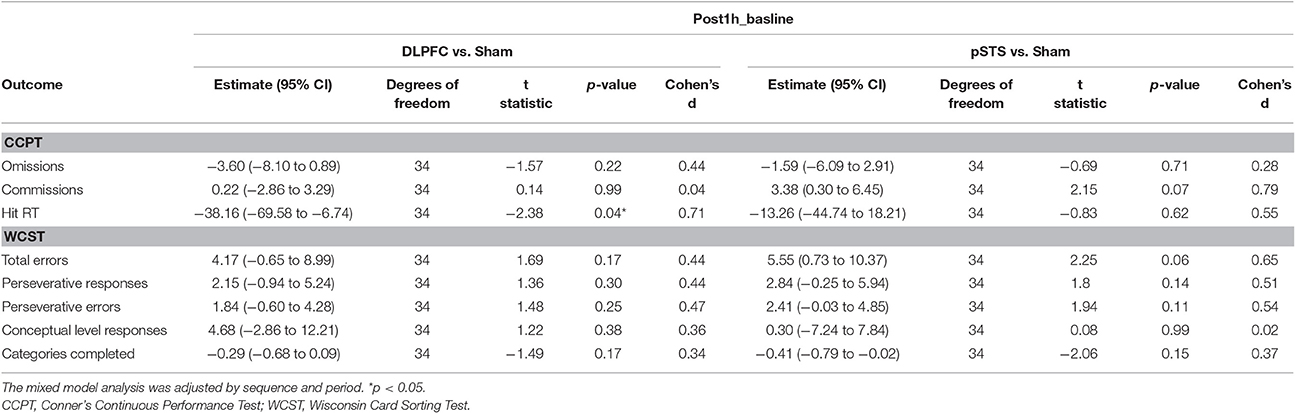
Table 3. Adjusted estimate of neuropsychological outcome difference from baseline based on the mixed model analysis.
Clinical Symptoms
The raw data of clinical outcomes including baseline, 8 h post-iTBS (post 8 h) and 2 days post-iTBS (post 2 days) are presented in Table 4. The outcome differences (before and after interventions) in the DLPFC and pSTS in comparison to the sham treatment are presented in Table 5 (post 8 h) and Table 6 (post 2 days). The differences were presented in Figure 2 (participants) and Figure 3 (parents).
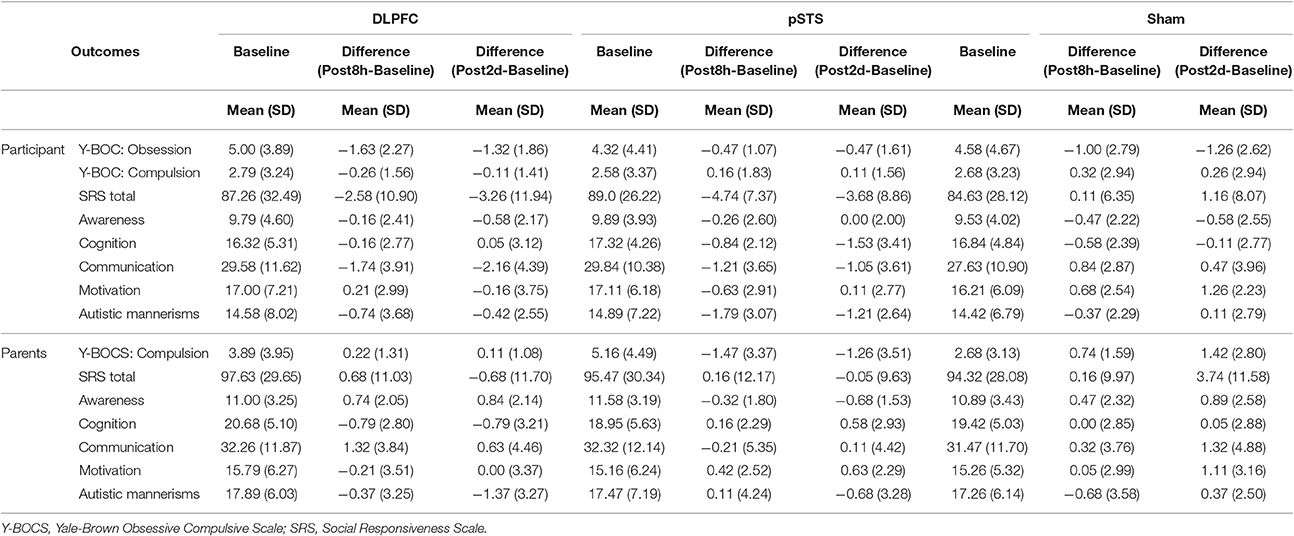
Table 4. Behavioral outcomes at baseline, post 8 h and post 2 days reported by participants and parents.
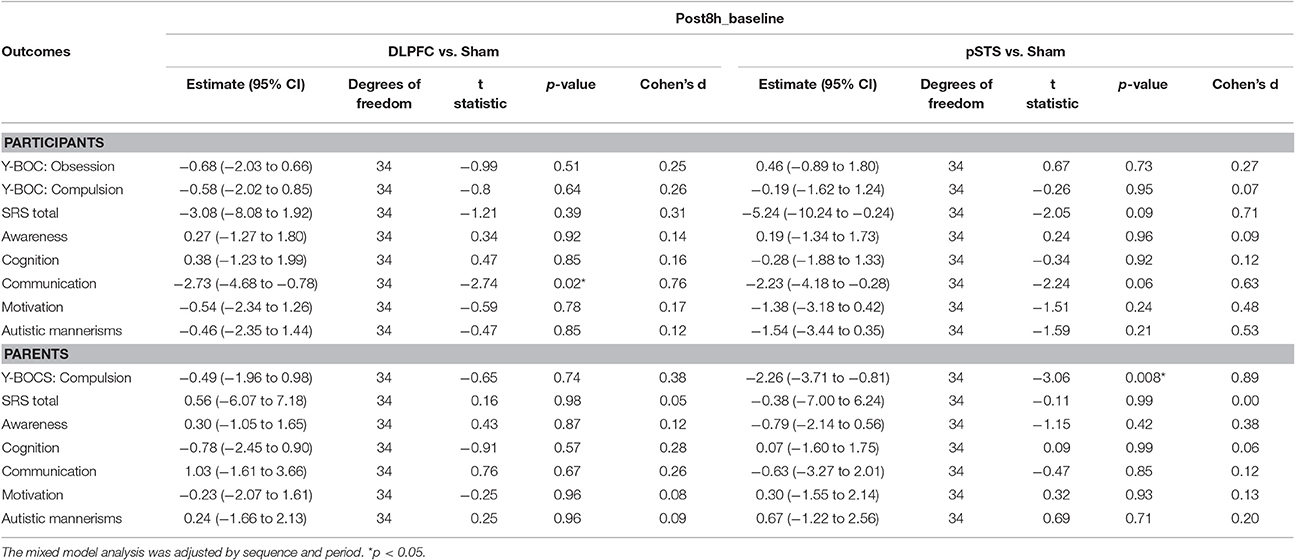
Table 5. Adjusted estimate of behavioral outcome difference between baseline and post 8 h based on the mixed model.
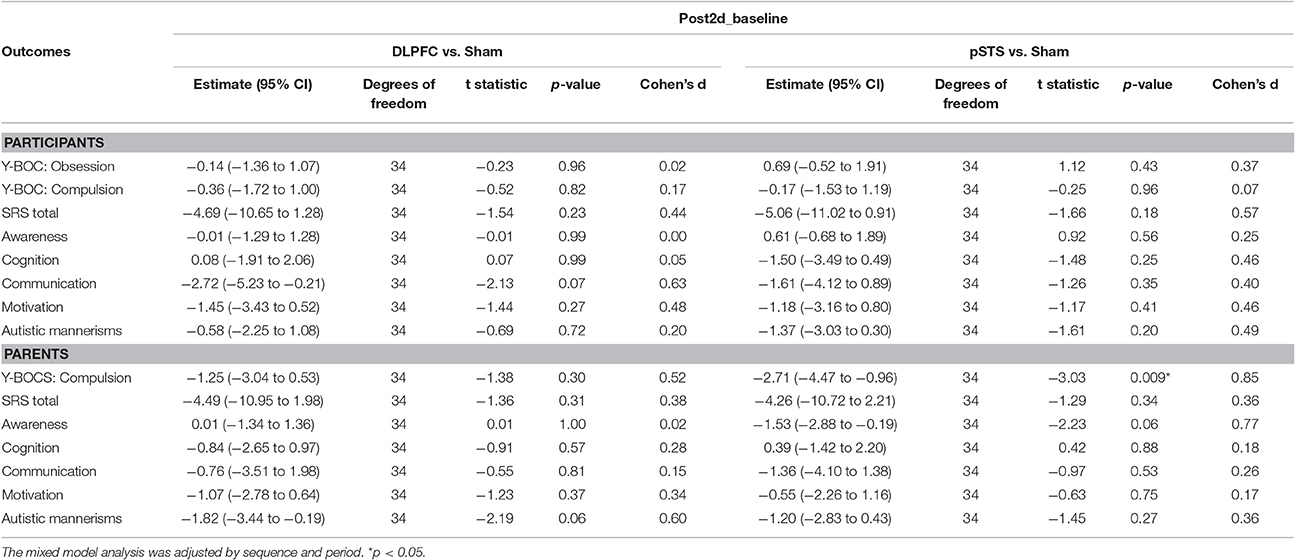
Table 6. Adjusted estimate of behavioral outcome difference between baseline and post 2 days based on the mixed model.
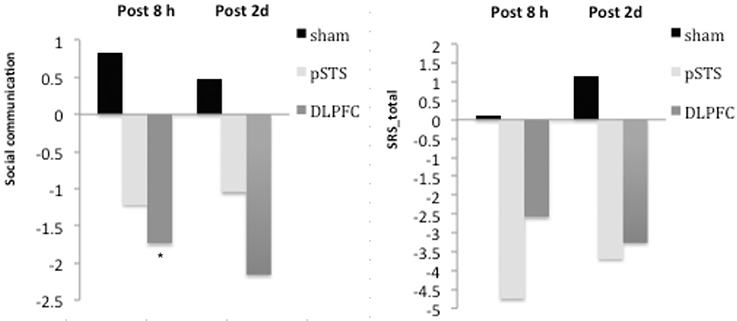
Figure 2. Changes of behavioral symptoms before and after iTBS using participants' reports. *p < 0.05.
For the repetitive behaviors measured by the Y-BOCS, scores of compulsive behaviors significantly decreased at 8 h (estimate = −2.26, p = 0.008, effect size = 0.89) and 2 days (estimate = −2.71, p = 0.009, effect size = 0.85) after the pSTS stimulation in comparison to the sham stimulation using the data from the reports of the parents. However, the results were not significant when the data from the reports of the participants were used. As for the comparison between the DLPFC and sham stimuli, there was no significant difference in either the self-reported or parent-reported Y-BOCS.
For the social outcomes measured by the SRS, self-reported scores of social communication significantly decreased at 8 h (estimate = −2.73, p = 0.02, effect size = 0.76), but not 2 days, after the iTBS over the DLPFC when compared to the sham treatment. However, no significant change was found when the data from the reports of parents were used. In addition, there was no significant improvement in both self-reported and parents' reported scores after iTBS over the pSTS when compared to the sham treatment.
Adverse Effects
Three participants felt transient discomfort during iTBS over the DLPFC because of muscle twitches around the eyes. No other adverse effect, such as a headache or seizure, was reported. Moreover, there was no obvious change in anxiety or mood symptoms observed using clinical assessment after iTBS.
Discussion
Using a randomized, sham-controlled and crossover design, our pilot study investigated the impacts of iTBS on the DLPFC and pSTS in adults with ASD. We found that one session of iTBS over the bilateral DLPFC may alter the neuropsychological function in adults with ASD. The hit reaction time in the CCPT significantly decreased following the DLPFC session. Although we found commission errors in the CCPT and total errors in the WCST increased following the pSTS session, the results were not statistically significant.
rTMS over the pSTS
The STS is important for social perception and social cognition (Zilbovicius et al., 2006). The anterior part of the STS is involved in voice processing (Kriegstein and Giraud, 2004), while the posterior part of the STS is important for analyzing biological motion (Pelphrey et al., 2003) and predicting the intention of others (Wyk et al., 2009). Previous studies have demonstrated the abnormal presentations of the pSTS in ASD (Boddaert et al., 2004; Redcay, 2008; Alaerts et al., 2014) and it has been proposed that the pSTS may be a therapeutic target in ASD (Saitovitch et al., 2016). However, the effect rTMS over pSTS on ASD has never been studied.
In the pilot study, we found a trend that iTBS over the pSTS may alter some neuropsychological functions in ASD, such as increasing commission errors in the CCPT and the total errors in the WCST. However, these findings were not statistically significant. Commission errors in the CCPT are known to relate to impulsivity, and the total errors in the WCST are related to cognitive flexibility. The findings may imply that, aside from the possible benefits on social behaviors, iTBS over the pSTS could impair some neuropsychological functions by increasing impulsivity and decreasing cognitive flexibility in ASD.
The results in behavioral outcomes were ambiguous. Although the parents reported significantly decreased compulsive behaviors in patients after iTBS (an excitatory protocol) over the pSTS, the patients did not report similar improvement. Besides, although the social communication and awareness improved after iTBS over the pSTS, the results were not statistically significant. Hence, the impacts of iTBS over the pSTS on behavior outcomes should be interpreted with caution, and a further study with a multi-sessional design may help for clarifying this point.
rTMS over the DLPFC
Previous studies have demonstrated the possible therapeutic effects of rTMS over the DLPFC in ASD, including alterations in the event-related potential component in several brain areas (Sokhadze et al., 2009; Baruth et al., 2010), decreases in the omission errors in a modified three category oddball task (Sokhadze et al., 2012) and decreases in repetitive behaviors (Baruth et al., 2010; Sokhadze et al., 2010; Casanova et al., 2014). However, the social impairments observed in ASD did not improve (Baruth et al., 2010; Sokhadze et al., 2010).
Previous studies have found that rTMS over the DLPFC may alter several neuropsychological functions in healthy adults. Wagner found that one session rTMS over the left DLPFC (20 Hz) could significantly alter the visual divided attention while the results in Stroop test and WCST were unaffected in healthy adults (Wagner et al., 2006). In addition, Vanderhasselt demonstrated that one session high-frequency (10 Hz) rTMS over the DLPFC could decrease the reaction time in the Stroop test and set-switching test in healthy female adults (Vanderhasselt et al., 2006a,b). Moreover, the following studies demonstrated that rTMS over the DLPFC could alter the neuropsychological functions including the performance in digit span test (Aleman and van't Wout, 2008), the Tower of London task (van den Heuvel et al., 2013), and set-shift trials (Gerrits et al., 2015) in healthy adults.
In addition to healthy adults, rTMS over the DLPFC can also alter the neuropsychological functions in ASD. Sokhadze found that low-frequency rTMS over the DLPFC could significantly decrease the omission errors, commission errors and increase the reaction time in the Kanizsa illusory figure visual oddball task in ASD (Sokhadze et al., 2012, 2014). In contrast to previous studies in ASD, we found that single session iTBS over the DLPFC significantly reduced the reaction time in the CCPT but had no effect on omission and commission errors in the CCPT or any subscales in the WCST. The opposing results for reaction time might be explained by the stimulation protocol: the effect of low-frequency rTMS is inhibitory, while the iTBS used in our study is excitatory. In consistent with previous studies in healthy adults (Vanderhasselt et al., 2006a,b), we found that excitatory rTMS over the DLPFC could significantly decrease the reaction time of the neuropsychological tests in ASD.
Mechanism of Action of rTMS in ASD
Most studies have demonstrated that low-frequency rTMS improves some deficits in ASD. Low-frequency rTMS is thought to induce long-term depression (Hoffman and Cavus, 2002). Event-related potential studies have indeed confirmed that the benefit of low-frequency rTMS over the DLPFC comes from increasing the activation of inhibitory circuits in ASD (Sokhadze et al., 2009, 2010; Baruth et al., 2010; Casanova et al., 2012).
Interestingly, we found that excitatory TBS over the DLPFC also altered the neuropsychological function in ASD. Previous studies have shown that the balance of cortical excitation and inhibition is abnormal in ASD (Casanova et al., 2002; Yizhar et al., 2011). This imbalance contributes to abnormalities in the cortical minicolumns, especially within the prefrontal cortex (Casanova, 2006). iTBS is known to enhance both the excitatory and inhibitory circuits beneath the coil (Huang et al., 2005, 2011). Therefore, we propose that iTBS enhances some inhibitory circuits that are required to improve social function in ASD. Moreover, the study of descending volleys of rTMS showed that iTBS enhanced mainly the later I waves, while 1 Hz rTMS suppressed mainly the I1 wave, indicating that iTBS and low-frequency rTMS may activate different circuits (Di Lazzaro et al., 2010). This may explain why the effect of iTBS is not simply opposite to the effect of low-frequency rTMS over the DLPFC.
This pilot study has several limitations. First, the sample size is relatively small, although it is comparable to that used in previous studies. Thus, the effect of iTBS over the pSTS or DLPFC may not be obvious. However, even with the small subject numbers, we still found the significant effect of iTBS over the pSTS or DLPFC on behavioral and neuropsychological outcomes compared to the sham treatment. The second limitation is the measurement of neuropsychological function. In the pilot study, we used only the CCPT and WCST, which are not directly related to the function of pSTS, to evaluate executive abilities because limited tools were available when the study was initiated. Other fields of neuropsychological function such as social cognition or biological motion detection should be considered as measures for the effect of pSTS stimulation in future studies. The third limitation is the measurement tool used to evaluate the behavioral symptoms. Consistent with previous studies, we used the SRS to measure the impact of rTMS (Sokhadze et al., 2009, 2010; Baruth et al., 2010; Casanova et al., 2012). However, the SRS was designed to evaluate the social ability of participants in the past 6 months, and may not be sensitive enough to capture changes over very short time periods. The development of better measurements to assess the impact of rTMS in ASD is important and will be necessary for the future (Oberman et al., 2016). The fourth limitation is the time window to evaluate the changes in behaviors and social symptoms in ASD. One early study demonstrated that the aftereffect of rTMS on compulsive behaviors could be found 8 h afterward (Greenberg et al., 1997). Following studies demonstrated that the effect of one session rTMS does not last for 1 week, and a week interval has been commonly applied to control the residual effect in a within-subject design (Simis et al., 2013; Kim and Shin, 2014; Tard et al., 2016). Based on these findings, we decided to evaluate the changes in behaviors and social symptoms in ASD 8 h and 2 days afterward. However, it is indeed arguable that the effect on behaviors and social symptoms may last longer than the period that we followed up. Longer time window to evaluate the changes in behavior and social symptoms should be considered in the future studies. To minimize the possible residual effect that may affect the effect of the subsequent stimulation, the order of the interventions was controlled and counterbalanced, and a mixed model analysis was used. The final limitation is the possibility of observational bias and placebo effects. Several steps were adopted to decrease these risks: the performance of iTBS and the data analysis were completed by different people; parents were not present during iTBS so that they would not know the stimulation location during each session; a crossover design and sham control treatment was applied to reduce the possibility of placebo effects.
This pilot study has several strengths. First, by adopting a crossover design, we were able to clearly compare the effect of iTBS over the DLPFC, the pSTS and the sham control in the same individuals. Second, the behavioral outcomes were measured using different resources, including both the participants themselves and their parents. Third, the localization of the DLPFC and pSTS via the process of coordinate transformation and the TMS-dedicated navigation system used is more precise than those in previous studies where no navigator was used.
In conclusion, this study revealed that the neuropsychological function in ASD was modified by the iTBS intervention. However, the effect of iTBS over either the DLPFC or the pSTS on behaviors in adults with ASD remains inconclusive and deserves further evaluation. To our knowledge, this is the first study to demonstrate the impacts of a facilitatory intervention over the pSTS in adults with ASD. Our findings indicate that the pSTS could be a new intervention target for ASD; thus, further long-term investigations should be performed in the future.
Author Contributions
HN, JH, CW, YW, RC, and YH participated in the study concept and design. HN and YW were involved in subjects recruitment and assessment. CW participated in acquisition of imaging data. HN did the iTBS intervention. HN and CC were involved in data analysis and interpretations. HN and JH were responsible for Table and figure production. HN, JH, and YH were involved in the writing of manuscript. All authors read and approved this manuscript.
Conflict of Interest Statement
The authors declare that the research was conducted in the absence of any commercial or financial relationships that could be construed as a potential conflict of interest.
The reviewer AS and handling Editor declared their shared affiliation, and the handling Editor states that the process nevertheless met the standards of a fair and objective review.
Acknowledgments
This work was supported by grants from the National Science Council of Taiwan (NSC 100-2314-B-182A-0175 and NSC 102-2314-B-182 -030 -MY3), National Health Research Institutes of Taiwan (NHRI-EX104-10343NI) and Chang Gung Memorial Hospital (CMRPG3D0781, BMRP844). We would like to thank Miss Hsiao-Ting Juang, who was supported by grants from the Center for Big Data Analytics and Statistics (Grant CLRPG3D0043) and Research Services Center For Health Information (Grant CIRPD1D0031) and Mr. Yi-Lung Chen, doctoral candidate of Graduate Institute of Epidemiology and Preventive Medicine, College of Public Health, National Taiwan University for their assistance in statistical analysis and Miss Su-Chuan Lin for her assistance in the experimental setups. We are grateful to all participants and their parents for taking part in the study.
References
Alaerts, K., Woolley, D. G., Steyaert, J., Di Martino, A., Swinnen, S. P., and Wenderoth, N. (2014). Underconnectivity of the superior temporal sulcus predicts emotion recognition deficits in autism. Soc. Cogn. Affect. Neurosci. 9, 1589–1600. doi: 10.1093/scan/nst156
Aleman, A., and van't Wout, M. (2008). Repetitive transcranial magnetic stimulation over the right dorsolateral prefrontal cortex disrupts digit span task performance. Neuropsychobiology 57, 44–48. doi: 10.1159/000129666
Arfeller, C., Schwarzbach, J., Ubaldi, S., Ferrari, P., Barchiesi, G., and Cattaneo, L. (2013). Whole-brain haemodynamic after-effects of 1-Hz magnetic stimulation of the posterior superior temporal cortex during action observation. Brain Topogr. 26, 278–291. doi: 10.1007/s10548-012-0239-9
Baruth, J. M., Casanova, M. F., El-Baz, A., Horrell, T., Mathai, G., Sears, L., et al. (2010). Low-frequency repetitive Transcranial Magnetic Stimulation (rTMS) modulates evoked-gamma frequency oscillations in Autism Spectrum Disorder (ASD). J. Neurother. 14, 179–194. doi: 10.1080/10874208.2010.501500
Baruth, J. M., Wall, C. A., Patterson, M. C., and Port, J. D. (2013). Proton magnetic resonance spectroscopy as a probe into the pathophysiology of autism spectrum disorders (ASD): a review. Autism Res. 6, 119–133. doi: 10.1002/aur.1273
Boddaert, N., Chabane, N., Gervais, H., Good, C. D., Bourgeois, M., Plumet, M. H., et al. (2004). Superior temporal sulcus anatomical abnormalities in childhood autism: a voxel-based morphometry MRI study. Neuroimage 23, 364–369. doi: 10.1016/j.neuroimage.2004.06.016
Bolte, S. (2012). Brief Report: the Social Responsiveness Scale for Adults (SRS-A): initial results in a German cohort. J. Autism Dev. Disord. 42, 1998–1999. doi: 10.1007/s10803-011-1424-5
Casanova, M. F. (2006). Neuropathological and genetic findings in autism: the significance of a putative minicolumnopathy. Neuroscientist 12, 435–441. doi: 10.1177/1073858406290375
Casanova, M. F., Baruth, J. M., El-Baz, A., Tasman, A., Sears, L., and Sokhadze, E. (2012). Repetitive Transcranial Magnetic Stimulation (rTMS) Modulates Event-Related Potential (ERP) indices of attention in autism. Transl. Neurosci. 3, 170–180. doi: 10.2478/s13380-012-0022-0
Casanova, M. F., Buxhoeveden, D. P., and Brown, C. (2002). Clinical and macroscopic correlates of minicolumnar pathology in autism. J. Child Neurol. 17, 692–695. doi: 10.1177/088307380201700908
Casanova, M. F., Hensley, M. K., Sokhadze, E. M., El-Baz, A. S., Wang, Y., Li, X., et al. (2014). Effects of weekly low-frequency rTMS on autonomic measures in children with autism spectrum disorder. Front. Hum. Neurosci. 8:851. doi: 10.3389/fnhum.2014.00851
Chuang, W. L., Huang, Y. Z., Lu, C. S., and Chen, R. S. (2014). Reduced cortical plasticity and GABAergic modulation in essential tremor. Mov. Disord. 29, 501–507. doi: 10.1002/mds.25809
Cohen, H., Kaplan, Z., Kotler, M., Kouperman, I., Moisa, R., and Grisaru, N. (2004). Repetitive transcranial magnetic stimulation of the right dorsolateral prefrontal cortex in posttraumatic stress disorder: a double-blind, placebo-controlled study. Am. J. Psychiatry 161, 515–524. doi: 10.1176/appi.ajp.161.3.515
Conners, C. K., Epstein, J. N., Angold, A., and Klaric, J. (2003). Continuous performance test performance in a normative epidemiological sample. J. Abnorm. Child Psychol. 31, 555–562. doi: 10.1023/A:1025457300409
Constantino, J. N. (2005). Social Responsiveness Scale (SRS). Los Angeles, CA: Western Psychological Services.
Constantino, J. N., and Todd, R. D. (2005). Intergenerational transmission of subthreshold autistic traits in the general population. Biol. Psychiatry 57, 655–660. doi: 10.1016/j.biopsych.2004.12.014
Di Lazzaro, V., Profice, P., Pilato, F., Dileone, M., Oliviero, A., and Ziemann, U. (2010). The effects of motor cortex rTMS on corticospinal descending activity. Clin. Neurophysiol. 121, 464–473. doi: 10.1016/j.clinph.2009.11.007
Enticott, P. G., Fitzgibbon, B. M., Kennedy, H. A., Arnold, S. L., Elliot, D., Peachey, A., et al. (2014). A double-blind, randomized trial of deep repetitive transcranial magnetic stimulation (rTMS) for autism spectrum disorder. Brain Stimul. 7, 206–211. doi: 10.1016/j.brs.2013.10.004
Enticott, P. G., Kennedy, H. A., Zangen, A., and Fitzgerald, P. B. (2011). Deep repetitive transcranial magnetic stimulation associated with improved social functioning in a young woman with an autism spectrum disorder. J. ECT 27, 41–43. doi: 10.1097/YCT.0b013e3181f07948
Enticott, P. G., Rinehart, N. J., Tonge, B. J., Bradshaw, J. L., and Fitzgerald, P. B. (2012). Repetitive transcranial magnetic stimulation (rTMS) improves movement-related cortical potentials in autism spectrum disorders. Brain Stimul. 5, 30–37. doi: 10.1016/j.brs.2011.02.001
Fang, J. H., Chen, J. J. J., Hwang, I. S., and Huang, Y. Z. (2010). Review: repetitive transcranial magnetic stimulation over the human primary motor cortex for modulating motor control and motor learning. J. Med. Biol. Eng. 30, 193–201. doi: 10.5405/jmbe.30.4.01
Fecteau, S., Agosta, S., Oberman, L., and Pascual-Leone, A. (2011). Brain stimulation over Broca's area differentially modulates naming skills in neurotypical adults and individuals with Asperger's syndrome. Eur. J. Neurosci. 34, 158–164. doi: 10.1111/j.1460-9568.2011.07726.x
Frost, R. O., Steketee, G., Krause, M. S., and Trepanier, K. L. (1995). The relationship of the Yale-Brown Obsessive Compulsive Scale (YBOCS) to other measures of obsessive compulsive symptoms in a nonclinical population. J. Pers. Assess. 65, 158–168. doi: 10.1207/s15327752jpa6501_12
Gau, S. S. F., Liu, L. T., Wu, Y. Y., Chiu, Y. N., and Tsai, W. C. (2013). Psychometric properties of the Chinese version of the social responsiveness scale. Res. Autism Spectr. Disord. 7, 349–360. doi: 10.1016/j.rasd.2012.10.004
Gaynes, B. N., Lloyd, S. W., Lux, L., Gartlehner, G., Hansen, R. A., Brode, S., et al. (2014). Repetitive transcranial magnetic stimulation for treatment-resistant depression: a systematic review and meta-analysis. J. Clin. Psychiatry 75, 477–489; quiz 489. doi: 10.4088/JCP.13r08815
Gendry Meresse, I., Zilbovicius, M., Boddaert, N., Robel, L., Philippe, A., Sfaello, I., et al. (2005). Autism severity and temporal lobe functional abnormalities. Ann. Neurol. 58, 466–469. doi: 10.1002/ana.20597
Gerrits, N. J., van den Heuvel, O. A., and van der Werf, Y. D. (2015). Decreased neural activity and neural connectivity while performing a set-shifting task after inhibiting repetitive transcranial magnetic stimulation on the left dorsal prefrontal cortex. BMC Neurosci. 16:45. doi: 10.1186/s12868-015-0181-3
Goodman, W. K., Price, L. H., Rasmussen, S. A., Mazure, C., Delgado, P., Heninger, G. R., et al. (1989a). The yale-brown obsessive compulsive scale. II. validity. Arch. Gen. Psychiatry 46, 1012–1016.
Goodman, W. K., Price, L. H., Rasmussen, S. A., Mazure, C., Fleischmann, R. L., Hill, C. L., et al. (1989b). The yale-brown obsessive compulsive scale. I. Development, use, and reliability. Arch. Gen. Psychiatry 46, 1006–1011.
Greenberg, B. D., George, M. S., Martin, J. D., Benjamin, J., Schlaepfer, T. E., Altemus, M., et al. (1997). Effect of prefrontal repetitive transcranial magnetic stimulation in obsessive-compulsive disorder: a preliminary study. Am. J. Psychiatry 154, 867–869. doi: 10.1176/ajp.154.6.867.
Grossman, E. D., Battelli, L., and Pascual-Leone, A. (2005). Repetitive TMS over posterior STS disrupts perception of biological motion. Vision Res. 45, 2847–2853. doi: 10.1016/j.visres.2005.05.027
Heaton, R. K. (2003). Wisconsin Card Sorting Test: Computer Version 4, Research Edition (WCST:CV4). Odessa, FL: Psychological Assessment Resources.
Hoffman, R. E., and Cavus, I. (2002). Slow transcranial magnetic stimulation, long-term depotentiation, and brain hyperexcitability disorders. Am. J. Psychiatry 159, 1093–1102. doi: 10.1176/appi.ajp.159.7.1093
Hollander, E., Novotny, S., Allen, A., Aronowitz, B., Cartwright, C., and DeCaria, C. (2000). The relationship between repetitive behaviors and growth hormone response to sumatriptan challenge in adult autistic disorder. Neuropsychopharmacology 22, 163–167. doi: 10.1016/S0893-133X(99)00121-9
Hollander, E., Soorya, L., Wasserman, S., Esposito, K., Chaplin, W., and Anagnostou, E. (2006). Divalproex sodium vs. placebo in the treatment of repetitive behaviours in autism spectrum disorder. Int. J. Neuropsychopharmacol. 9, 209–213. doi: 10.1017/S1461145705005791
Huang, Y. Z., Chen, R. S., Rothwell, J. C., and Wen, H. Y. (2007). The after-effect of human theta burst stimulation is NMDA receptor dependent. Clin. Neurophysiol. 118, 1028–1032. doi: 10.1016/j.clinph.2007.01.021
Huang, Y. Z., Edwards, M. J., Rounis, E., Bhatia, K. P., and Rothwell, J. C. (2005). Theta burst stimulation of the human motor cortex. Neuron 45, 201–206. doi: 10.1016/j.neuron.2004.12.033
Huang, Y. Z., Lu, C. S., Rothwell, J. C., Lo, C. C., Chuang, W. L., Weng, Y. H., et al. (2012). Modulation of the disturbed motor network in dystonia by multisession suppression of premotor cortex. PLoS ONE 7:e47574. doi: 10.1371/journal.pone.0047574
Huang, Y. Z., Rothwell, J. C., Chen, R. S., Lu, C. S., and Chuang, W. L. (2011). The theoretical model of theta burst form of repetitive transcranial magnetic stimulation. Clin. Neurophysiol. 122, 1011–1018. doi: 10.1016/j.clinph.2010.08.016
Kim, Y. K., and Shin, S. H. (2014). Comparison of effects of transcranial magnetic stimulation on primary motor cortex and supplementary motor area in motor skill learning (randomized, cross over study). Front. Hum. Neurosci. 8:937. doi: 10.3389/fnhum.2014.00937
Kriegstein, K. V., and Giraud, A. L. (2004). Distinct functional substrates along the right superior temporal sulcus for the processing of voices. Neuroimage 22, 948–955. doi: 10.1016/j.neuroimage.2004.02.020
Lai, M. C., Lombardo, M. V., and Baron-Cohen, S. (2014). Autism. Lancet 383, 896–910. doi: 10.1016/S0140-6736(13)61539-1
LeBlanc, J. J., and Fagiolini, M. (2011). Autism: a “critical period” disorder? Neural Plast. 2011:921680. doi: 10.1155/2011/921680
Mason, R. A., Williams, D. L., Kana, R. K., Minshew, N., and Just, M. A. (2008). Theory of Mind disruption and recruitment of the right hemisphere during narrative comprehension in autism. Neuropsychologia 46, 269–280. doi: 10.1016/j.neuropsychologia.2007.07.018
Mylius, V., Ayache, S. S., Ahdab, R., Farhat, W. H., Zouari, H. G., Belke, M., et al. (2013). Definition of DLPFC and M1 according to anatomical landmarks for navigated brain stimulation: inter-rater reliability, accuracy, and influence of gender and age. Neuroimage 78, 224–232. doi: 10.1016/j.neuroimage.2013.03.061
Nickl-Jockschat, T., Habel, U., Michel, T. M., Manning, J., Laird, A. R., Fox, P. T., et al. (2012). Brain structure anomalies in autism spectrum disorder–a meta-analysis of VBM studies using anatomic likelihood estimation. Hum. Brain Mapp. 33, 1470–1489. doi: 10.1002/hbm.21299
Oberman, L. M., Enticott, P. G., Casanova, M. F., Rotenberg, A., Pascual-Leone, A., McCracken, J. T., et al. (2016). Transcranial magnetic stimulation in autism spectrum disorder: challenges, promise, and roadmap for future research. Autism Res. 9, 184–203. doi: 10.1002/aur.1567
Ohnishi, T., Matsuda, H., Hashimoto, T., Kunihiro, T., Nishikawa, M., Uema, T., et al. (2000). Abnormal regional cerebral blood flow in childhood autism. Brain 123(Pt 9), 1838–1844. doi: 10.1093/brain/123.9.1838
Pell, G. S., Roth, Y., and Zangen, A. (2011). Modulation of cortical excitability induced by repetitive transcranial magnetic stimulation: influence of timing and geometrical parameters and underlying mechanisms. Prog. Neurobiol. 93, 59–98. doi: 10.1016/j.pneurobio.2010.10.003
Pelphrey, K. A., Mitchell, T. V., McKeown, M. J., Goldstein, J., Allison, T., and McCarthy, G. (2003). Brain activity evoked by the perception of human walking: controlling for meaningful coherent motion. J. Neurosci. 23, 6819–6825.
Puce, A., and Perrett, D. (2003). Electrophysiology and brain imaging of biological motion. Philos. Trans. R. Soc. Lond. B Biol. Sci. 358, 435–445. doi: 10.1098/rstb.2002.1221
Rajji, T. K., Rogasch, N. C., Daskalakis, Z. J., and Fitzgerald, P. B. (2013). Neuroplasticity-based brain stimulation interventions in the study and treatment of schizophrenia: a review. Can. J. Psychiatry 58, 93–98. doi: 10.1177/070674371305800206
Redcay, E. (2008). The superior temporal sulcus performs a common function for social and speech perception: implications for the emergence of autism. Neurosci. Biobehav. Rev. 32, 123–142. doi: 10.1016/j.neubiorev.2007.06.004
Redcay, E., Dodell-Feder, D., Mavros, P. L., Kleiner, M., Pearrow, M. J., Triantafyllou, C., et al. (2013). Atypical brain activation patterns during a face-to-face joint attention game in adults with autism spectrum disorder. Hum. Brain Mapp. 34, 2511–2523. doi: 10.1002/hbm.22086
Saitovitch, A., Popa, T., Lemaitre, H., Rechtman, E., Lamy, J. C., Grevent, D., et al. (2016). Tuning eye-gaze perception by transitory STS Inhibition. Cereb. Cortex 26, 2823–2831. doi: 10.1093/cercor/bhw045
Simis, M., Adeyemo, B. O., Medeiros, L. F., Miraval, F., Gagliardi, R. J., and Fregni, F. (2013). Motor cortex-induced plasticity by noninvasive brain stimulation: a comparison between transcranial direct current stimulation and transcranial magnetic stimulation. Neuroreport 24, 973–975. doi: 10.1097/WNR.0000000000000021
Sokhadze, E., Baruth, J., Tasman, A., Mansoor, M., Ramaswamy, R., Sears, L., et al. (2010). Low-frequency repetitive transcranial magnetic stimulation (rTMS) affects event-related potential measures of novelty processing in autism. Appl. Psychophysiol. Biofeedback 35, 147–161. doi: 10.1007/s10484-009-9121-2
Sokhadze, E. M., Baruth, J. M., Sears, L., Sokhadze, G. E., El-Baz, A. S., and Casanova, M. F. (2012). Prefrontal neuromodulation using rTMS improves error monitoring and correction function in autism. Appl. Psychophysiol. Biofeedback 37, 91–102. doi: 10.1007/s10484-012-9182-5
Sokhadze, E. M., El-Baz, A., Baruth, J., Mathai, G., Sears, L., and Casanova, M. F. (2009). Effects of low frequency repetitive transcranial magnetic stimulation (rTMS) on gamma frequency oscillations and event-related potentials during processing of illusory figures in autism. J. Autism Dev. Disord. 39, 619–634. doi: 10.1007/s10803-008-0662-7
Sokhadze, E. M., El-Baz, A. S., Tasman, A., Sears, L. L., Wang, Y., Lamina, E. V., et al. (2014). Neuromodulation integrating rTMS and neurofeedback for the treatment of autism spectrum disorder: an exploratory study. Appl. Psychophysiol. Biofeedback 39, 237–257. doi: 10.1007/s10484-014-9264-7
Tard, C., Devanne, H., Defebvre, L., and Delval, A. (2016). Single session intermittent theta-burst stimulation on the left premotor cortex does not alleviate freezing of gait in Parkinson's disease. Neurosci. Lett. 628, 1–9. doi: 10.1016/j.neulet.2016.05.061
van den Heuvel, O. A., Van Gorsel, H. C., Veltman, D. J., and Van Der Werf, Y. D. (2013). Impairment of executive performance after transcranial magnetic modulation of the left dorsal frontal-striatal circuit. Hum. Brain Mapp. 34, 347–355. doi: 10.1002/hbm.21443
Vanderhasselt, M. A., De Raedt, R., Baeken, C., Leyman, L., and D'Haenen, H. (2006a). The influence of rTMS over the left dorsolateral prefrontal cortex on Stroop task performance. Exp. Brain Res. 169, 279–282. doi: 10.1007/s00221-005-0344-z
Vanderhasselt, M. A., De Raedt, R., Baeken, C., Leyman, L., and D'Haenen, H. (2006b). The influence of rTMS over the right dorsolateral prefrontal cortex on intentional set switching. Exp. Brain Res. 172, 561–565. doi: 10.1007/s00221-006-0540-5
van Kemenade, B. M., Muggleton, N., Walsh, V., and Saygin, A. P. (2012). Effects of TMS over premotor and superior temporal cortices on biological motion perception. J. Cogn. Neurosci. 24, 896–904. doi: 10.1162/jocn_a_00194
Van Overwalle, F., and Baetens, K. (2009). Understanding others' actions and goals by mirror and mentalizing systems: a meta-analysis. Neuroimage 48, 564–584. doi: 10.1016/j.neuroimage.2009.06.009
Wagner, M., Rihs, T. A., Mosimann, U. P., Fisch, H. U., and Schlaepfer, T. E. (2006). Repetitive transcranial magnetic stimulation of the dorsolateral prefrontal cortex affects divided attention immediately after cessation of stimulation. J. Psychiatr. Res. 40, 315–321. doi: 10.1016/j.jpsychires.2005.06.001
Watts, B. V., Landon, B., Groft, A., and Young-Xu, Y. (2012). A sham controlled study of repetitive transcranial magnetic stimulation for posttraumatic stress disorder. Brain Stimul. 5, 38–43. doi: 10.1016/j.brs.2011.02.002
Woody, S. R., Steketee, G., and Chambless, D. L. (1995). Reliability and validity of the Yale-Brown Obsessive-Compulsive Scale. Behav. Res. Ther. 33, 597–605.
Wyk, B. C., Hudac, C. M., Carter, E. J., Sobel, D. M., and Pelphrey, K. A. (2009). Action understanding in the superior temporal sulcus region. Psychol. Sci. 20, 771–777. doi: 10.1111/j.1467-9280.2009.02359.x
Yizhar, O., Fenno, L. E., Prigge, M., Schneider, F., Davidson, T. J., O'Shea, D. J., et al. (2011). Neocortical excitation/inhibition balance in information processing and social dysfunction. Nature 477, 171–178. doi: 10.1038/nature10360
Zilbovicius, M., Boddaert, N., Belin, P., Poline, J. B., Remy, P., Mangin, J. F., et al. (2000). Temporal lobe dysfunction in childhood autism: a PET study. Positron emission tomography. Am. J. Psychiatry 157, 1988–1993. doi: 10.1176/appi.ajp.157.12.1988
Keywords: repetitive transcranial magnetic stimulation, theta burst stimulation, dorsolateral prefrontal cortex, posterior superior temporal sulcus, autism spectrum disorder
Citation: Ni H-C, Hung J, Wu C-T, Wu Y-Y, Chang C-J, Chen R-S and Huang Y-Z (2017) The Impact of Single Session Intermittent Theta-Burst Stimulation over the Dorsolateral Prefrontal Cortex and Posterior Superior Temporal Sulcus on Adults with Autism Spectrum Disorder. Front. Neurosci. 11:255. doi: 10.3389/fnins.2017.00255
Received: 10 January 2017; Accepted: 20 April 2017;
Published: 09 May 2017.
Edited by:
Stefano Ferraina, Sapienza University of Rome, ItalyReviewed by:
Antonio Suppa, Sapienza University of Rome, ItalyPeter G. Enticott, Deakin University, Australia
Copyright © 2017 Ni, Hung, Wu, Wu, Chang, Chen and Huang. This is an open-access article distributed under the terms of the Creative Commons Attribution License (CC BY). The use, distribution or reproduction in other forums is permitted, provided the original author(s) or licensor are credited and that the original publication in this journal is cited, in accordance with accepted academic practice. No use, distribution or reproduction is permitted which does not comply with these terms.
*Correspondence: Ying-Zu Huang, eXpodWFuZ0BjZ21oLm9yZy50dw==