- 1Department of Otolaryngology & Head and Neck Surgery, Ruijin Hospital, Shanghai Jiao Tong University School of Medicine, Shanghai, China
- 2Shanghai Key Laboratory of Translational Medicine on Ear and Nose Diseases, Shanghai, China
- 3Department of Otolaryngology & Head and Neck Surgery, Shanghai Ninth People’s Hospital, Shanghai Jiao Tong University School of Medicine, Shanghai, China
- 4Department of Otolaryngology & Head and Neck Surgery, EENT Hospital, Fudan University, Shanghai, China
- 5Key Laboratory of Hearing Medicine, National Health and Family Planning Commission, Shanghai, China
The incidences of presbycusis and dementia are high among geriatric diseases. Presbycusis is the general term applied to age-related hearing loss and can be caused by many risk factors, such as noise exposure, smoking, medication, hypertension, family history, and other factors. Mutation of mitochondrial DNA in hair cells, spiral ganglion cells, and stria vascularis cells of the cochlea is the basic mechanism of presbycusis. Dementia is a clinical syndrome that includes the decline of cognitive and conscious states and is caused by many neurodegenerative diseases, of which Alzheimer’s disease (AD) is the most common. The amyloid cascade hypothesis and tau hypothesis are the two major hypotheses that describe the AD pathogenic mechanism. Recent studies have shown that deposition of Aβ and hyperphosphorylation of the tau protein may cause mitochondrial dysfunction. An increasing number of papers have reported that, on one hand, the auditory system function in AD patients is damaged as their cognitive ability declines and that, on the other hand, hearing loss may be a risk factor for dementia and AD. However, the relationship between presbycusis and AD is still unknown. By reviewing the relevant literature, we found that the SIRT1-PGC1α pathway and LKB1 (or CaMKKβ)-AMPK pathway may play a role in the preservation of cerebral neuron function by taking part in the regulation of mitochondrial function. Then vascular endothelial growth factor signal pathway is activated to promote vascular angiogenesis and maintenance of the blood–brain barrier integrity. Recently, experiments have also shown that their expression levels are altered in both presbycusis and AD mouse models. Therefore, we propose that exploring the specific molecular link between presbycusis and AD may provide new ideas for their prevention and treatment.
Introduction
The elderly population worldwide is currently approximately 900 million (de Carvalho et al., 2015). There has been a dramatic shift in the distribution of deaths from younger to older ages and from maternal, perinatal, nutritional, and communicable causes to non-communicable disease causes (Mathers and Loncar, 2005). Chronic diseases, of which dementia and presbycusis account for a large part, become more prevalent with age. This trend is exacerbated by lifestyle and behavior changes that predispose individuals to these diseases. According to the World Alzheimer Report, there were an estimated 35.6 million people with AD and other dementias in the year 2010; this number will reach 66 million by 2030 and 115 million by 2050 (Wortmann, 2012). In addition to cognitive decline, AD is also associated with secondary diseases including cardiovascular disease, tumors and sensory system dysfunctions, such as vision and hearing loss (Masters et al., 2015), which impose a heavy burden on patients as well as on society. Therefore, exploring the connections between AD and other diseases is significant for early diagnosis and prevention. As early as 1964, hearing impairment was thought to lead to mental illness caused by isolation (Kay et al., 1964). Subsequently, hearing loss was thought to be independently associated with accelerated cognitive decline and dementia (Lin et al., 2011, 2013). Hearing loss is not only the result of auditory organ damage, but may also result from central nervous system dysfunction in auditory information processing. Therefore, an increasing number of researchers have begun to explore whether improving hearing function can improve cognitive disabilities or reduce the risk of dementia later in life. Although Lin et al. (2011) found that treating hearing loss with hearing aids did not significantly decrease the risk of dementia, the risk of incident dementia did increase in participants with a hearing loss of over 25 dB. Dawes et al. (2015) suggested that hearing aids might improve cognitive performance, but this positive effect may not be the result of reducing the adverse effects of hearing loss, such as social segregation or depressed emotion. It remains unclear how presbycusis correlates with cognitive decline, dementia or AD (through social isolation caused by hearing loss, through common neuropathological pathways, or through vascular factors) and whether the relationship between them is unidirectional or bidirectional. As a result, we first review the auditory pathological changes in AD patients and AD mouse models; then, we emphasize how hearing impairment affects the incidence of cognitive decline, dementia and AD on the basis of epidemiologic evidence. Importantly, we summarize the enzymes and proteins that may contribute to the pathogenesis of both presbycusis and AD, including factors such as VEGF, SIRT1-PGC1α, and LKB1 (or CaMKKβ)-AMPK, because AD is the most common type of dementia and the molecular mechanism of AD has been extensively explored. Abnormal expression of these enzymes and proteins may cause AD (Kalaria et al., 1998; Won et al., 2010; Kumar et al., 2013) and dysfunction of cochlear hair cells (Picciotti et al., 2006; Hill et al., 2016; Xue et al., 2016). However, the specific connections between presbycusis and dementia still require further study.
Presbycusis, Dementia and Alzheimer’s Disease
Presbycusis
Presbycusis is the general term applied to ARHL. The risk factors for presbycusis include noise exposure, smoking, medication, hypertension, family history and other factors (Mills et al., 2009). According to statistics from the World Health Organization (data from World health Organization [WHO], 2017), a person whose hearing thresholds are over 25 dB in both ears is said to have hearing loss. Hearing loss can be classified as mild, moderate, severe, or profound. Nearly one-third of people over 65 years of age suffer from disabling hearing loss. This disorder is characterized by hearing sensitivity reduction (particularly at high frequencies), reduced understanding of speech in noisy environments, delayed central processing of acoustic information, and impaired localization of sound sources (Tavanai and Mohammadkhani, 2017). Severe hearing loss will affect an individual’s psychosocial status and cause social segregation, depression or loss of self-confidence. Although at the early stage, the peripheral auditory dysfunction may be the fundamental pathological change of ARHL, impairment of CAP function becomes increasingly important in late ARHL (Gates and Mills, 2005).
Many molecular theories have been proposed for the development of ARHL. One theory posits that accumulation of ROS in the mitochondria of inner ear hair cells, spiral ganglions and epithelial cells of the stria vascularis can cause further oxidative stress and mtDNA mutations (Yamasoba, 2009; Yamasoba et al., 2013). Markaryan et al. (2009) quantified mtDNA in human cochlear tissue samples, and the deletion rate of mtDNA (4977 bp) was found to reach 32%. However, the deletion rate of mtDNA (4834 bp) was found by Kong et al. (2006) to reach over 90% in the inner ear of rats treated with D-galactose, and this type of mutation can enhance the sensitivity of the inner ear to an aminoglycoside antibiotic.
Dementia and Alzheimer’s Disease
Dementia is a chronic and progressive deterioration disease characterized by cognitive dysfunction and abnormal mental behavior. It has become the greatest global challenge for health and social care in the 21st century (Livingston et al., 2017). Two of more common types of dementia are AD, which is characterized as a progressive, unremitting and neurodegenerative disorder (Masters et al., 2015); and vascular dementia (VD), which is mainly caused by hypertension and arteriosclerosis. AD is the main type of dementia that has caught global attention. The population of people with AD and other dementias will reach 66 million by 2030 and 115 million by 2050 (Wortmann, 2012). Dementia is associated with age, and the incidence of dementia increase from 3.9/1000 person years (pyr) at an age of 60–64 to 104.8/1000 pyr at an age of over 90, which indicates that the incidence doubles every 6.3 years. Currently, Aβ, tau protein and ApoE are the three main elements that are thought to contribute to AD. The pathology of dementia, especially AD, which is focused on in the following section, includes: (1) loss of neurons in the temporal lobes and hippocampus; (2) NFTs; (3) SPs that consist of amyloid-β (Aβ); and (4) amyloidopathy of the cerebrovasculature (Hyman, 1998). NFTs and SPs are characteristic pathological changes. The amyloid cascade hypothesis and tau hypothesis are the two major hypotheses that describe the AD pathogenic mechanism. The average duration of illness is 8–10 years, but the clinically symptomatic phases are preceded by preclinical and prodromal stages that typically extend over two decades (Masters et al., 2015). Therefore, it is of great clinical significance to diagnose and prevent AD at an early stage.
Pathological Changes of the Auditory System in Patients with AD
Central auditory processing dysfunction is highly evident in persons with Alzheimer’s disease (Idrizbegovic et al., 2011), and pathological changes have also been found in the auditory system, as described in the following studies. The auditory nervous pathway originates from afferent neurons called spiral ganglion cells in cochlea. Spiral ganglion cells are a type of bipolar neurons located in the Rosenthal canals of the bony modiolus. Next, the axons of spiral ganglion cells project to the cochlear nucleus complex. Then, most of the axons from the cochlear nucleus complex cross the midline and ascend in the contralateral lateral lemniscus, terminating in the inferior colliculus and medial geniculate body. Finally, all ascending neurons form an auditory radiation and terminate in the auditory center of the transverse temporal gyrus (Lonsbury-Martin et al., 2009). Neurons in different parts of the medial geniculate body are represented with the low best frequencies arranged laterally and high best frequencies arranged medially (Aitkin and Webster, 1971). Volume and quality loss of the cerebrum (meaning the sulcus is wider and deeper) as well as atrophy of the gyri have been observed in the cerebrum of AD patients using imaging methods. Consequently, we hypothesize that patients with AD might have hearing loss at both low and high frequencies if the neurons in the medial geniculate body are widely degenerated. Therefore, every link in the auditory pathway may display pathological changes associated with AD, thus leading to hearing impairment (Figure 1).
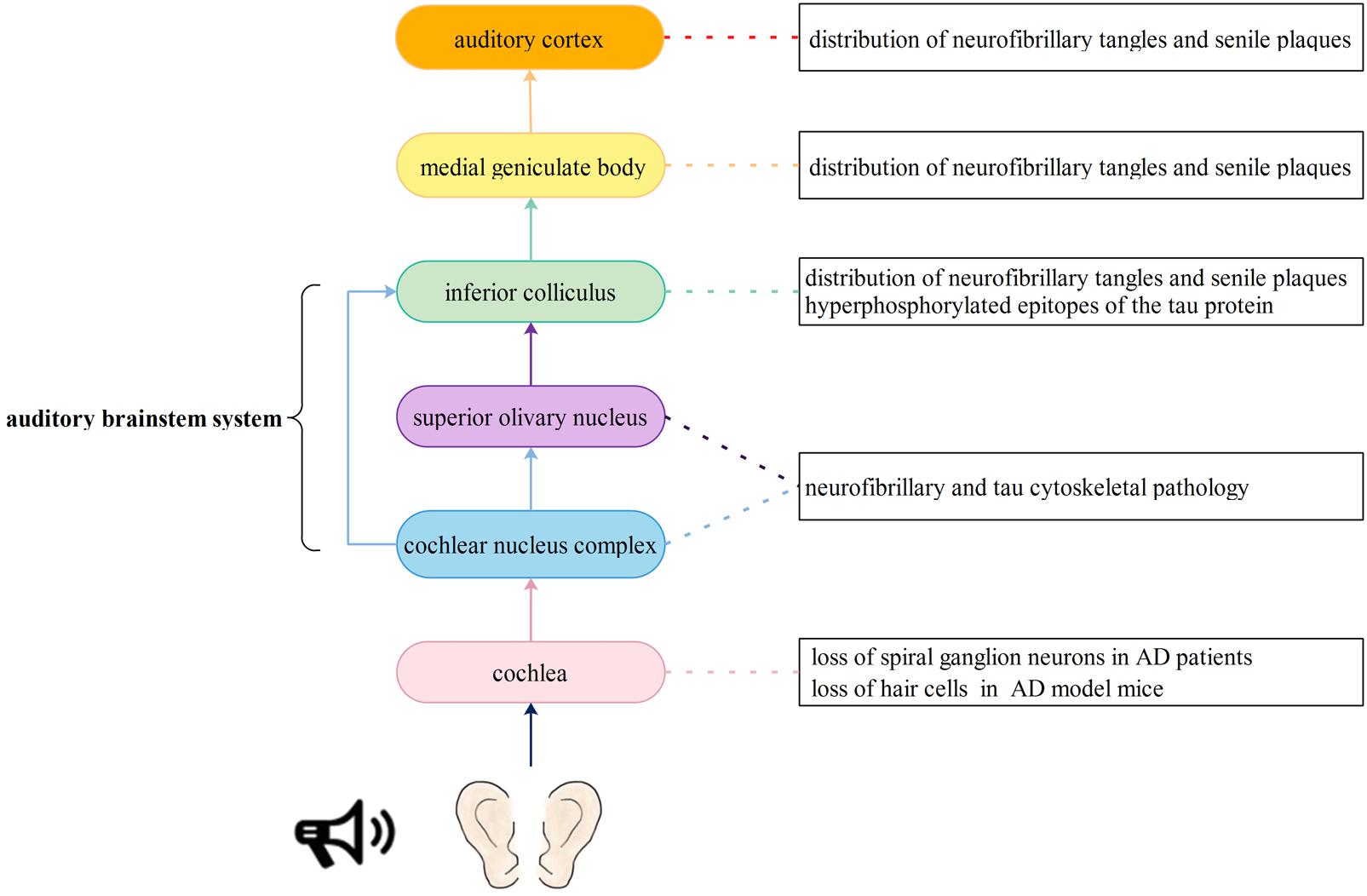
FIGURE 1. Auditory signal pathway and possible insults to the auditory nervous system caused by Alzheimer’s disease. The auditory nervous pathway originates from spiral ganglion cells in cochlea. Then, the axons of spiral ganglion cells project to the cochlear nucleus complex. Next, most of the axons from the cochlear nucleus complex cross the midline and ascend in the contralateral lateral lemniscus, terminating in the inferior colliculus and medial geniculate body. Another part of axons project to the superior olivary nucleus and then terminate in the inferior colliculus and medial geniculate body. Finally, all ascending neurons form an auditory radiation and terminate in the auditory center of the transverse temporal gyrus. Every link in the auditory pathway may display pathological changes associated with AD, thus leading to hearing impairment.
Cochlear Pathology
The production and conduction of auditory signals in the cochlea mainly involve hair cells and spiral neurons. Wang and Wu (2015) reported that a significant loss of SGNs, rather than hair cells, could be found in the cochlea of 9- and 12-month-old 3xTg-AD model mice. However, O’Leary et al. (2017) found that in 5xFAD model mice (a kind of AD model mice), outer and inner hair cells also showed significantly greater losses at the apical and basal ends of the basilar membrane than wild-type mice at 15–16 months of age. Omata et al. (2016) produced new transgenic mouse models [Tg(Math1E-Aβ42Arc)1Lt/Tg(Math1E-Aβ42)1Lt], which overexpress Aβ or Aβ-related proteins in cochlear hair cells, and Tg (MathE-MAPT) 1Lt, which express human tau (2N4R) in cochlear hair cells. These transgenic mice showed auditory dysfunction, especially in high-frequency sound perception, and in these mice, expression of Aβ and tau was correlated with hearing impairment as well as hair cell loss. Consequently, it is speculated that abnormal deposition of Aβ and overexpression of tau protein in cochlea hair cells may have a synergistic effect on hearing impairment.
Pathology of the Medial Geniculate Body
The ventral nucleus of the medial geniculate body is one of the most important relay stations in the ascending auditory pathway, and it receives fibers from neurons in the central nucleus of the inferior colliculus. Sinha et al. (1993) found that SPs and NFTs were extensively distributed throughout not only the ventral nucleus of the medial geniculate body but also the central nucleus of the inferior colliculus in AD patients at autopsy. Similarly, Rüb et al. (2016) found conspicuous AD-related cytoskeletal pathology in the inferior colliculus, superior olive and dorsal cochlear nucleus. Parvizi et al. (2001) also found β-amyloid and hyperphosphorylated epitopes of the tau protein in the inferior and superior colliculus and autonomic, monoaminergic, cholinergic, and classical reticular nucleus.
Auditory Cortex Pathology
The human auditory cortex is mainly located in the superior temporal gyrus (Hackett, 2015). In the early stage of AD, brain atrophy involved the temporal lobe, especially the hippocampus and other brain regions, including the central auditory cortex and its related functional nuclei. In addition to the relay stations in the auditory pathway, such as the medial geniculate body and inferior colliculus, SPs and NFTs have also been observed in the primary auditory cortex and association area of the auditory cortex (Sinha et al., 1993). Recently, expression of VEGF was found to be reduced in the superior temporal, hippocampal, and brainstem regions of AD patients (Provias and Jeynes, 2014). VEGF is an important endothelial growth factor that is responsible for vascular angiogenesis, remodeling, and maintenance of the blood–brain barrier (Sondell et al., 1999). It stimulates axonal outgrowth, thus promoting cell survival and Schwann cell proliferation in the peripheral nervous system (Rosenstein et al., 2010). Moreover, VEGF repairs hair cell damage caused by noise, drugs, or certain diseases, such as otitis media and acoustic neuroma (London and Gurgel, 2014). The results mentioned above imply that reduced expression of VEGF may cause abnormalities in the structure and function of blood vessels and neurons in the auditory cortex of patients with AD, leading to a severe hearing loss. In addition to the pathological changes in auditory pathways, mouse models and patients with AD also showed increased ABR thresholds, and a greater hearing loss was related to higher adjusted relative odds of having dementia (Uhlmann et al., 1989; Goll et al., 2011; O’Leary et al., 2017).
ARHL May Be a Risk Factor for Cognitive Decline, Dementia or AD
Defects in sensory systems, including the olfactory, visual or auditory systems, are thought to be highly associated with age-related neurodegenerative diseases (Benarroch, 2010). Impairments in peripheral and central auditory organs have been linked to accelerated cognitive decline (Bernabei et al., 2014; Amieva et al., 2015), incident cognitive impairment (Deal et al., 2017), dementia and AD (Gallacher et al., 2012; Panza et al., 2015; Taljaard et al., 2016). Therefore, audiometric testing may serve as a useful method for evaluating cognitive function, dementia and AD. After a 12-year follow-up study, Lin et al. (2011) noted that hearing loss was independently related to incident dementia after eliminating the influence of age, sex, race, education, hypertension, and other factors in 639 participants aged from 36 to 90 years old, and the attribute risk of dementia related to hearing loss reached 36.4%. In 2013, they further proposed that hearing loss was independently associated with accelerated cognitive decline (Lin et al., 2013). In addition to the peripheral auditory function, Gates et al. (2010) proposed that central auditory dysfunction and executive dysfunction might also give rise to neurodegenerative processes. CAP dysfunction in one ear was related to a sixfold increase in the risk of cognitive decline in later life after a 6-year follow-up study (Gates et al., 1996). Panza et al. (2015) concluded from many clinical trials that a CAP deficit might be an early sign of cognitive decline, and that CAP testing could be used to evaluate cognitive function in the future. Other researchers believe that hearing defects may play a part in producing mental symptoms, such as social isolation and loneliness, by reducing an individual’s contact with the outside world due to communication impairments caused by ARHL (Bennett et al., 2006). Evidence from epidemiological studies suggests that hearing loss is a modifiable factor, and with the help of appropriate and timely treatment, cognitive decline can be decelerated and daily activities can be facilitated (Behrman et al., 2014). Cognitive performance was found to be improved in auditory rehabilitation with the use of cochlear implants or hearing aids, which suggests that interventions that aim to restore hearing may be an effective way to alleviate cognitive disorders in late life (Mulrow et al., 1990; Castiglione et al., 2016). Individuals with greater hearing loss might receive the most cognitive benefit from hearing aids (Meister et al., 2015). Although many researches have shown that hearing loss may increase the risk of cognitive decline, as mentioned above, there is still much debate regarding whether there is a link between hearing loss and dementia. Gennis et al. (1991) found that hearing loss had no significant relation with cognitive function after a 5-year follow-up of 224 people aged over 60 years with no serious underlying disease. In addition, some studies have also found no evidence of improvement in behavioral symptoms, functional status, or quality of later life by providing hearing aids to hearing-impaired AD patients (Adrait et al., 2017; Nguyen et al., 2017).
In addition to the above clinical trials, patients with hearing loss have also exhibited significant reductions in the gray matter volume of the cortex related to hearing, attention and emotion, as revealed by morphometry, functional magnetic resonance (fMRI) imaging or EEG studies (Wong et al., 2010; Peelle et al., 2011; Eckert et al., 2012; Cardin, 2016). With fMRI, Peelle et al. (2011) found that a decline of peripheral auditory acuity not only led to a loss of gray matter volume in the primary auditory cortex but also contributed to down regulation of neural activity in the course of language processing. EEG studies have revealed that the greater the degree of hearing loss, the more sluggish the cortical response (Cardin, 2016). These results suggest that changes in the anatomy and function of the brain associated with hearing loss might be a reason for the increased incidence of cognitive decline and dementia. In animal experiments, Yu et al. (2011) found that when C57BL/6J mice developed profound hearing loss by 42–44 weeks of age, their cognitive function also declined, as evaluated by the Morris water maze and that the ultrastructure of the synapses of the CA3 region of the hippocampus also changed, including an increase in the synaptic cleft width and decrease of the thickness of the postsynaptic density. Therefore, we believe that there may be a certain connection between ARHL and dementia. The pathological changes of the hippocampus associated with hearing loss may lay the foundation for the incidence of cognitive decline and dementia. Similarly, Wayne and Johnsrude (2015) also proposed that there may be a common mechanism for both diseases and, to some extent, hearing loss may be an early manifestation of the underlying pathological changes.
ARHL and AD May Result From Mitochondrial Dysfunction and Changes in Certain Signal Pathways
The role of mitochondrial dysfunction in the pathogenesis of dementia is hotly debated. Manczak et al. (2006) found that oligomeric forms of Aβ are mainly aggregated in the mitoplast (the inner membrane plus the matrix) of mitochondria inside neurons of the cortex and hippocampus. In a transgenic mouse model of AD, the hydrogen peroxide levels were greatly increased and cytochrome c oxidase activity was decreased in a transgenic mouse model of AD. These changes were directly associated with the levels of soluble Aβ, which suggest that soluble Aβ may cause more production of hydrogen peroxide and impair mitochondrial metabolism in the development and progression of AD. By contrast, aggregation of ROS may cause mtDNA mutations or deletions, and mitochondrial dysfunction is correlated with the development of ARHL (Yamasoba, 2009; Yamasoba et al., 2013). In recent decades, an increasing number of researchers have begun to pay attention to the function of the mitochondria in an effort to explore whether there is a common pathway in mitochondrial oxidative metabolism that can cause both dementia and hearing loss. If there is a common pathway between them, it may provide new ideas for preventing and treating AD as well as hearing loss.
ROS/VEGF Pathway
Reactive oxygen species, such as O2- and H2O2, can activate VEGF, which is important for angiogenesis and neuron protection. VEGF transmits signals to endothelial cells mainly through two tyrosine kinase receptors, VEGFR-1 (Flt-1) and VEGFR-2 (Flk-1) (Rosenstein et al., 2010). Research has shown that flt-1(-/-) embryos have defective sprouting from the dorsal aorta (Kearney et al., 2004). Exogenous H2O2 administered to human umbilical vein endothelial cells to simulate ROS production in cells increases mitochondrial (mtROS) through Serine 36 phosphorylation of p66Shc (Kim et al., 2017). Mitochondria in endothelial cells are also implicated in ROS signaling transactivation of VEGFR2 or VEGF-induced cell migration. Inhibition of mitochondrial respiration with rotenone and oligomycin can attenuate H2O2-induced tyrosine phosphorylation of VEGFR2 in bovine aortic endothelial cells (Chen et al., 2004). When cells are faced with a hypoxic environment, both VEGF production and VEGFR2 expression are upregulated and the generation of hydrogen peroxide is triggered by mitochondria under hypoxic conditions (Chandel et al., 1998; Colavitti et al., 2002). VEGF may also promote neurogenesis, neuronal patterning, neuroprotection and glial growth by acting on blood vessels. VEGF was found to be upregulated when the central nervous system suffered from injuries, and exogenous application of VEGF facilitated central nervous system angiogenesis (Rosenstein et al., 2010). Wang et al. (2007) found that neurogenesis and neuromigration were enhanced under ischemic condition in VEGF-overexpressing transgenic mice. In addition, the use of VEGF-specific antibodies that blocked its normal function in a stab injury model led to an increase in lesion size and reduction of angiogenic and astroglial activity in the striatum (Krum and Khaibullina, 2003).
As mentioned above, VEGF levels are reduced in the superior temporal, hippocampal, and brainstem regions in AD patients (Provias and Jeynes, 2014). VEGF is also decreased in aged ARHL mice (Picciotti et al., 2004). Therefore, as a downstream signal of mitochondrial regulation, VEGF is likely to be a common factor in the molecular mechanisms of dementia and presbycusis. Since VEGF has the function of neuroprotection, it also exerts many positive effects during the pathogenesis of AD. Expression of VEGF in the brain can increase transiently in the early stage of AD, implicating some compensatory mechanisms to counter pathological changes, such as insufficient vascularity and reduced perfusion (Kalaria et al., 1998). Exogenous VEGF was administered to transgenic mice by Burger et al. (2009) who found that VEGF modulated β-secreatase1 (BACE1) and reduced soluble Aβ1–40 and Aβ1–42. In addition, VEGF was found to bind to amyloid plaques with high affinity, most likely causing a deficiency of available and free VEGF under hypoperfusion conditions and possibly contributing to neurodegeneration and vascular dysfunction in the progression of AD (Yang et al., 2004). The decreased VEGF levels in the brain may affect the function of memory and cognition. The cognitive function of APP/PS1 mice was improved after intraperitoneal injection of VEGF (Wang et al., 2011). Intra-hippocampal administration of a VEGF receptor blocker may negatively affect long-term memory (Pati et al., 2009). Donepezi, an acetylcholinesterase inhibitor, exhibits beneficial effects in Alzheimer’s disease through activation of the PI3K/Akt/HIF-1α/VEGF pathway (Kakinuma et al., 2010). By reviewing the relevant literature, we found that VEGF is not only associated with the occurrence of dementia, but may also play an important role in many inner ear diseases. In studies, VEGF has been shown to contribute to the development of vestibular schwannoma and otitis media with effusion, and the use of a VEGF antagonist can alleviate hearing loss in these two diseases (Lim and Birck, 1971; Koutsimpelas et al., 2007; Plotkin et al., 2009; Cheeseman et al., 2011). However, the specific function of VEGF in ARHL requires further investigation. Among the many risk factors of ARHL, exposure to noise and ototoxic drugs, such as kanamycin or cisplatin, has been reported to lead to significant cochlear vascular changes, including an increase in vascular permeability, alterations in cochlear blood flow and vasoconstriction (Hawkins et al., 1972). Acoustic trauma was also found to structurally change blood vessels by disrupting the cochlear blood–barrier (Shi, 2009). In a study exploring noise-induced sensorineural damage, upregulation of VEGF was observed in the stria vascularis, spiral ligament and SGNs of guinea pigs as early as 1 day after noise exposure, and it also preceded the gradual recovery of inner ear function. Therefore, VEGF may also enhance neuron survival during the course of ARHL (Picciotti et al., 2006).
In short, aging often causes reduced ROS clearance and increased ROS activity (Kaur et al., 2016). Accumulation of ROS in cells can cause mtDNA mutations and mitochondrial dysfunction and then result in decreased levels of VEGF. Decreased levels of VEGF may lead to Aβ deposition and hamper the repair of hair cells and spiral ganglions in the inner ear as well as the reconstruction of blood vessels in the brain, resulting in dementia or presbycusis. Further studies are needed to determine whether there is a time order of the occurrence of both diseases and whether hearing loss caused by a decrease of VEGF will further increase the risk of dementia and its progression (Figure 2).
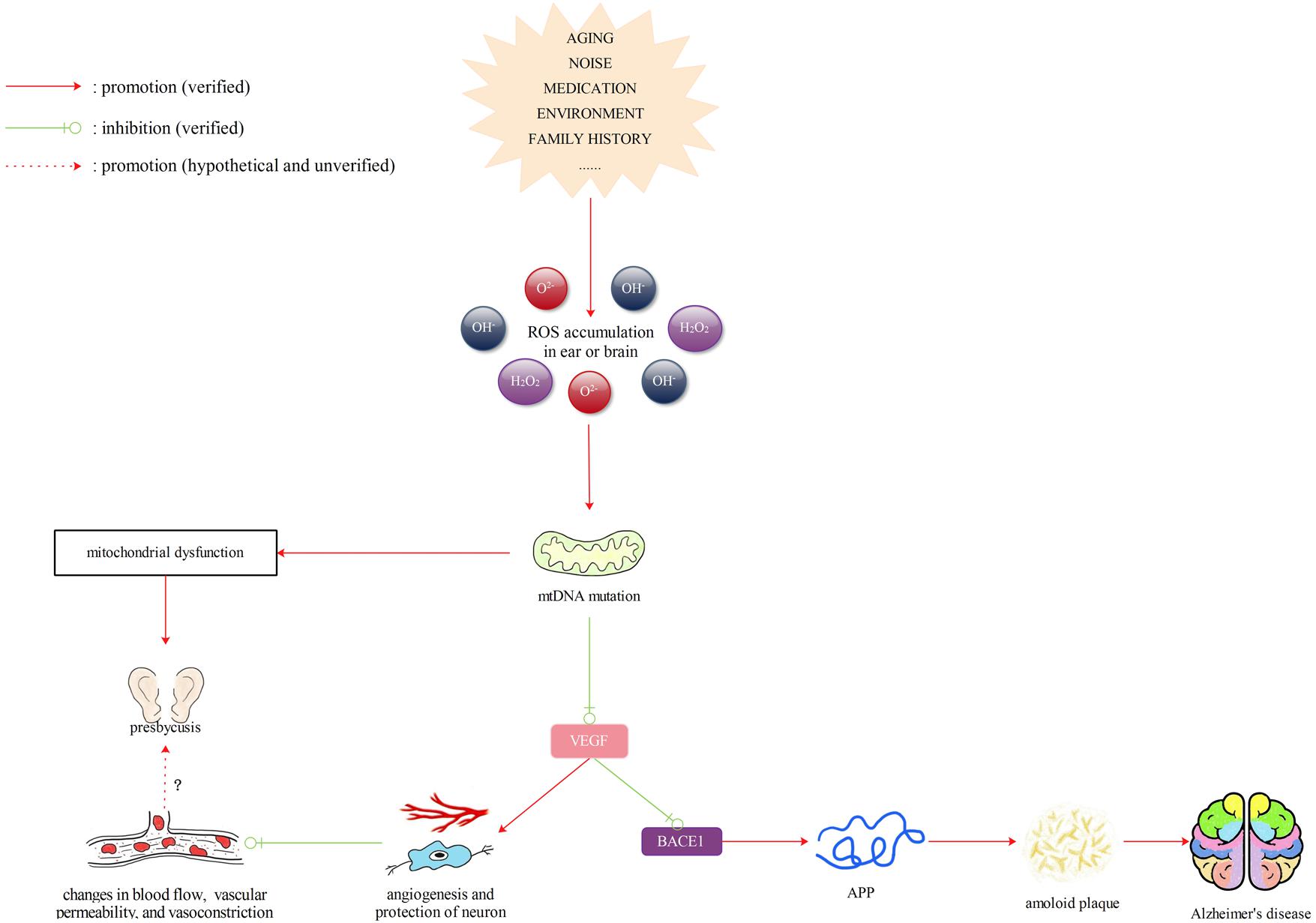
FIGURE 2. Reactive oxygen species (ROS)/VEGF pathway. Aging often causes reduced ROS clearance and increased ROS activity. Accumulation of ROS in cells can cause mtDNA mutations and mitochondrial dysfunction and then result in decreased levels of VEGF. Decreased levels of VEGF may lead to Aβ deposition and hamper the repair of hair cells and spiral ganglions in the inner ear as well as the reconstruction of blood vessels in the brain, resulting in dementia or presbycusis. Further studies are still needed to determine whether there is a time order of the occurrence of both diseases and whether hearing loss caused by a decrease of VEGF will further increase the risk of dementia and its progression.
SIRT1/PGC-1α (or FNDC5) Pathway
Amyloid plaques primarily consist of Aβ1–42 and Aβ23–35 (Glenner and Wong, 1984; Gruden et al., 2007). BDNF is a versatile and multifunctional growth factor that is indispensable in a wide range of adaptive processes during human brain development, ranging from regulation of synapse formation and plasticity to neuronal differentiation and better cognitive function (Phillips et al., 1991; Park and Poo, 2013). BDNF precursors and mature BDNF were found to be reduced in the brain of patients in the pre-clinical stages of AD (Peng et al., 2005). Xia et al. (2017) found that both the protein and mRNA levels of BDNF were reduced in APP/PS1 transgenic mice as well as in the hippocampus and cerebral cortex of C57BL/6 mice after injection of Aβ1–42 oligomer. BDNF exerts protective effects in the process of neurodegeneration. In the case of AD, BDNF shifts APP processing toward the α-secretase pathway rather than the β-secretase pathway to repair the neurotoxic effects of Aβ in the brain (Holback et al., 2005). Moreover, BDNF activation is responsible for the tau dephosphorylation, thus inhibiting aggregation of the tau protein in NFTs (Murer et al., 1999; Elliott et al., 2005). A previous study showed that PPARγ coactivator-1 (PGC-1α) and its downstream activated membrane protein, FNDC5, could modulate the BDNF levels together in the PGC-1α (-/-) mouse model (Wrann et al., 2013).
PGC-1α is important in the control of cellular energy metabolic pathways (Finck and Kelly, 2006). It is more highly expressed in cells that are rich in mitochondria and is one of the factors involved in mitochondria synthesis and respiratory gene regulation (Kelly and Scarpulla, 2004). Moreover, PGC-1α also plays an important role in inhibiting neurodegeneration. PGC-1α was reported to protect against the neurodegenerative effects induced by MTPT (1-methyl-4-phenyl-1, 2, 3, 6-tetrahydropyridine) and enhance ROS detoxification (St-Pierre et al., 2006). In addition, PGC-1α can promote spinogenesis and synaptogenesis in cultured hippocampal neurons (Cheng et al., 2012). SIRT1 is a NAD+-dependent protein deacetylase located upstream of PGC-1α. It plays a crucial role in maintaining cellular homeostasis by regulating neuron survival and death, glucose metabolism, insulin sensitivity, and mitochondrial synthesis (Lagouge et al., 2006; Guarente, 2013). PCG-1α deacetylation mediated by SIRT1is required for the activation of mitochondrial fatty acid oxidation genes (McCarty et al., 2015).
Reduced levels of PGC-1α and FNDC5 were also found in Neuro-2a cells treated with Aβ oligomers in vitro (Xia et al., 2017). However, when PGC-1α and FNDC5 were overexpressed, the reduction in BDNF caused by Aβ oligomers was reversed, suggesting that the inhibitory action of Aβ on BDNF levels was mediated by a PGC-1α-/FNDC5-dependent pathway. With the help of BDNF treatment, deposition of Aβ in the brain was restrained and the cognitive decline was postponed in APP/PS1transgenic mice. SIRT1 can modulate Aβ metabolism by regulating the processing of amyloid precursor protein (APP) in AD progression (Kumar et al., 2013). Wang et al. (2013) found that treatment with the SIRT1-activating agent resveratrol prevented the generation of Aβ through a SIRT1-dependent mechanism. They also showed that both in vitro and in vivo experiments, downregulation of PGC-1α or PGC-1β gene transcription using specific small interfering RNA (siRNA) resulted in augmented expression of β-secreatase1 (BACE1), a protein responsible for cleaving APP. In addition, overexpression of PGC-1α decreased BACE1 protein expression in the hippocampi of transgenic mice (Wang et al., 2013). The dysfunction in the SIRT1-PGC-1α pathway not only linked to AD, but also influenced the function of hair cells and caused hearing loss. Expression of SIRT1, SIRT3, and SIRT5 was reduced in the cochlea of 22-month-old CBA/J mice (Takumida et al., 2016). A previous study revealed that SIRT1 was the direct target gene of miR-29 (Xu et al., 2014) and that expression of miR-29b was upregulated in the ARHL mouse model (Zhang et al., 2013). Xue et al. (2016) found an age-dependent downregulation in SIRT1 and PGC-1α protein levels, as well as mitochondrial dysfunction in the cochlea of aged mice. In vitro experiments showed that overexpression of miR-29b in HEI-OC1 cells inhibited SIRT-1 and PGC-1α protein levels, causing mitochondrial dysfunction and hair cell apoptosis, whereas in miR-29b knockdown cells, the SIRT1 and PGC-1α protein levels, as well as their mRNA levels, were all significantly upregulated (Xue et al., 2016). Additionally, overexpression of SIRT1 markedly promoted PGC-1α expression in HEI-OC1 cells, inhibited cell apoptosis, and boosted cell proliferation. Consequently, it was hypothesized that miR-29b/SIRT1/PGC-1α signaling most likely plays a role in regulating hair cells apoptosis and in the pathogenesis of ARHL.
From the above research, we propose that expression of miR-29b may be increased in the inner ear with aging, which reduces the expression of its target SIRT1 and affects downstream PGC-1α and FNDC5. Reduced expression of SIRT1- PGC-1α may impair the synthesis and respiratory function of mitochondria, thereby causing neuronal degeneration and cell apoptosis, thus leading to presbycusis. At the same time, reduced expression of SIRT1- PGC-1α in the brain can cause increased production of Aβ and reduced production of BDNF and thus contribute to the incidence of AD. Though no previous study has explored the changes of miR-29b in the brains of AD patients, we believe that SIRT1/PGC-1α (FNDC5) may be a possible drug target to prevent patients with ARHL from developing AD. However, SIRT1 has recently been reported to play a completely adversarial role in the early onset of ARHL in C57BL/6 mice (Han et al., 2016). Whether SIRT1 has a positive or negative effect on ARHL requires further investigation (Figure 3).
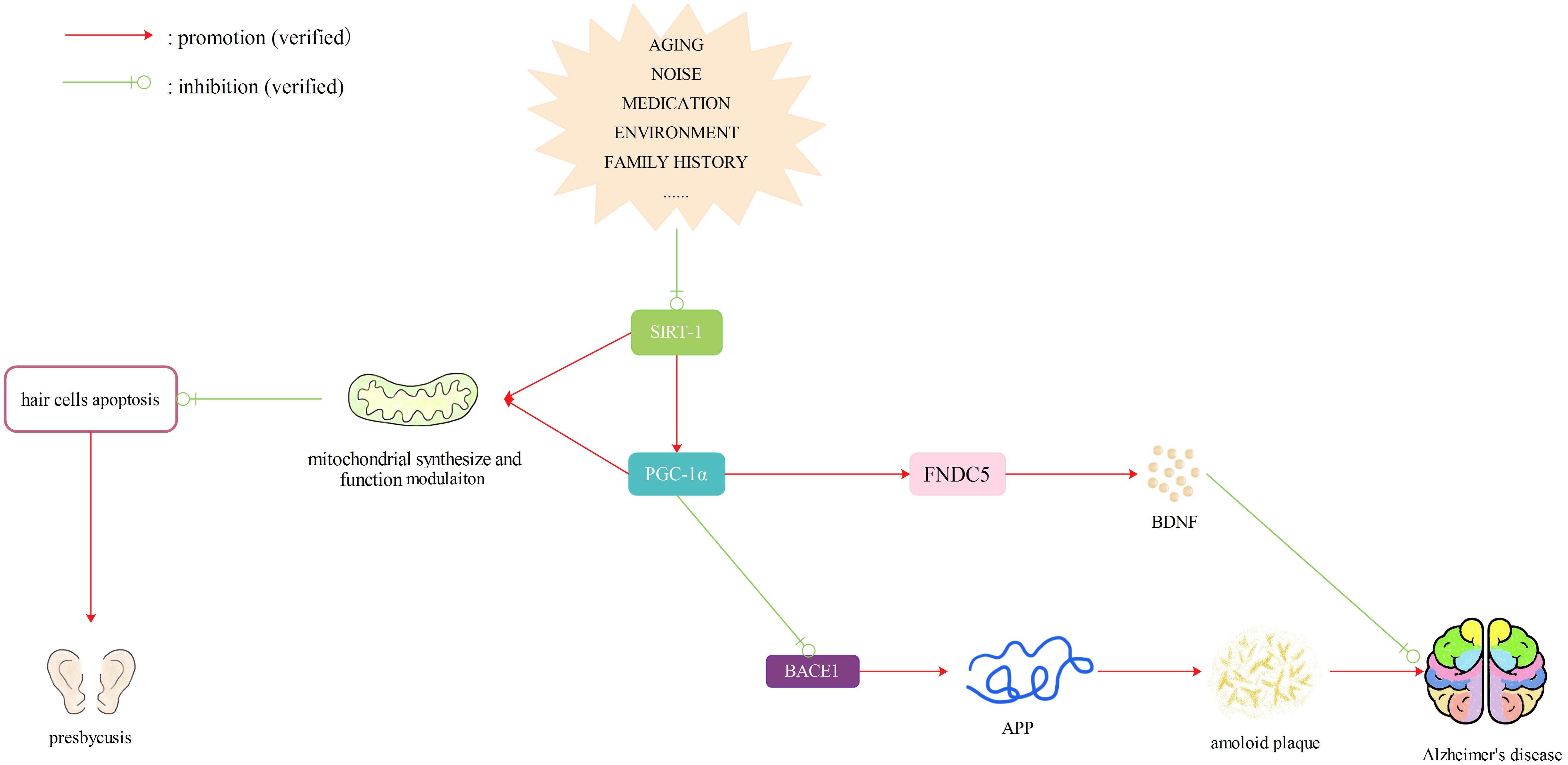
FIGURE 3. SIRTl/PGC-1α (FNDC5) pathway. Reduced expression of SIRT1- PGC-1α caused by many factors may impair the synthesis and respiratory function of mitochondria, thereby causing neuronal degeneration and cell apoptosis, thus leading to presbycusis. At the same time, reduced expression of SIRT1- PGC-lα in the brain can cause increased production of Aβ and reduced production of BDNF and thus contribute to the incidence of dementia. Therefore, SIRT1/PGC-lα (FNDC5) may be a possible drug target to prevent patients with ARHL from developing AD.
LKB1 and CaMKKβ/AMPK Pathway
AMP-activated protein kinase (AMPK) is a major regulator of cellular energy homeostasis and a central player in glucose and lipid metabolism (Daval et al., 2006; Hardie, 2008). First, when the human body is faced with hypoxia, oxidative stress or ischemia, AMPK is activated and phosphorylated by LKB1 complex or CaMKKβ, accompanied by microenvironment changes, such as an elevated AMP/ATP ratio and increased Ca2+ levels, respectively, thus promoting cellular glucose uptake, fatty acid β-oxidation, glucose transporter 4 synthesis and mitochondrial synthesis (Viollet et al., 2009). Activation of AMPK can lead to more fatty acids entering the mitochondria as well as stimulate the synthesis of ATP (Saha and Ruderman, 2003). It can also promote ATP synthesis by suppressing upregulation of ATPase inhibitory factor 1 (IF1) and stimulating the activity of the oxidative respiratory chain (Vazquez-Martin et al., 2013). Second, AMPK can phosphorylate the PGC-1α protein at threonine-177 and serine-538 to directly regulate mitochondrial synthesis (Jager et al., 2007) or deacetylate PGC-1α via SIRT1activation to modulate mitochondria and lipid utilization genes (Canto et al., 2010). Third, AMPK can improve mitochondrial autophagy due to reactive oxygen damage (Pauly et al., 2012). Therefore, the LKB1 (or CaMKKβ)/AMPK pathway and its association with the SIRT1/PGC-1α pathway are closely related to the function and synthesis of mitochondria.
AMP-activated protein kinase has also been shown to play a key role in regulating neurodegenerative diseases via autophagy of mitochondria (Vingtdeux et al., 2011). Transient glutamate exposure during ischemia or stroke leads to rapid and transient AMPK activation with an increase in Glucose Transporter 3 trafficking, which exerts a neuroprotective effect by increasing the ATP/AMP ratio and decreasing cytosolic Ca2+ levels (Weisova et al., 2011). In the progression of AD, AMPK activation may downregulate the generation of Aβ by modulating APP processing (Won et al., 2010). At the same time, AMPK is also a physiological tau kinase, and direct stimulation by AICAR (AMPK activator) inhibits tau phosphorylation (Chakraborty et al., 2008; Greco et al., 2009a,b). Moreover, AMPK activation can suppress the mTOR signaling pathway and then enhance cell autophagy and lysosomal degradation of Aβ (Anekonda et al., 2011; Vingtdeux et al., 2011). Sodium hydrosulfide was found to alleviate cell apoptosis in the auditory cortex through the CaMKKβ/AMPK pathways, suggesting that AMPK is a crucial factor in neuron degeneration of the auditory cortex in the central nervous system (Chen et al., 2017). Therefore, AMPK may be a neoteric determinant in neurodegenerative diseases and one of the possible targets for drugs aimed at AD and ARHL. Another upstream kinase of AMPK, LKB1, contributes to maintaining the development and structural stability of cochlear hair cells and stereocilia. Men et al. (2015) found that LKB1 is required for the development and maintenance of hair cell stereociliary bundles and is expressed from the cuticular plate to the nuclei of hair cells. The ABR and DPOAEs thresholds were significantly higher in Atoh1-LKB1-/- mice than those in control mice, although there were no obvious differences between mutant mice and control mice in gross morphology (Men et al., 2015). However, whether expression of LKB1 will change during the aging process and affect the process of ARHL is still unknown.
AMP-activated protein kinase is capable of inhibiting c-Jun N-terminal protein kinase (JNK) activity in neurons (Schulz et al., 2008). However, prolonged elevation of phosphorylated (p)-AMPK can trigger chronic activation of JNK, thereby causing upregulation of the proapoptotic protein Bim (Bcl-2 interacting mediator of cell death) and subsequently leading to apoptosis in neuronal and pancreatic cells, suggesting that cell fate regulation by AMPK is complex (Kefas et al., 2004; Yun et al., 2005; Weisova et al., 2011). Hill et al. (2016) proposed that long-term phosphorylation of AMPK caused by noise exposure could inversely lead to a decreased auditory function together with hair cell and synaptic ribbon loss. After CBA/J mice were exposed to 98 dB of noise, immunolabeling for p-AMPKα showed a stronger change in IHCs and marginal elevation in OHCs, while no obvious changes were detected in pillar cells. In non-exposed control animals, p-AMPKα was weak in hair cells and stronger in pillar cells. As a result, we can speculate that activation of AMPK may exert a dual influence, which may have an opposite effect on cell death and survival.
Therefore, we can hypothesize that decreased activity of AMPK will lead to abnormalities in mitochondrial synthesis and normal functions, such as oxidative respiration, as well as alterations of autophagy, which render cells unable to handle changes in the microenvironment. Decreased activity of AMPK will also cause less degradation of Aβ and more phosphorylation of tau. LKB1 and CaMKKβ are required for the activation of AMPK and are important for the stability of stereocilia in hair cells and protection of auditory cortex neurons, respectively. Although there is currently no evidence showing that LKB1 and CaMKKβ change in the ear or brain due to aging, the environment, diet or other factors, we speculate that if expression of LKB1 and CaMKKβ is altered by the above factors, they would not only cause hearing loss but also affect downstream phosphorylation of AMPK, leading to AD. In addition, the dual effect of AMPK leads us to suggest a novel concept: AMPK may be phosphorylated in the brains of AD patients for a long time in response to intracellular environmental changes, which may damage the auditory cortex and accelerate hearing loss. Therefore, LKB1 and CaMKKβ/AMPK may also be a new target for drugs to protect patients with ARHL from dementia (Figure 4).
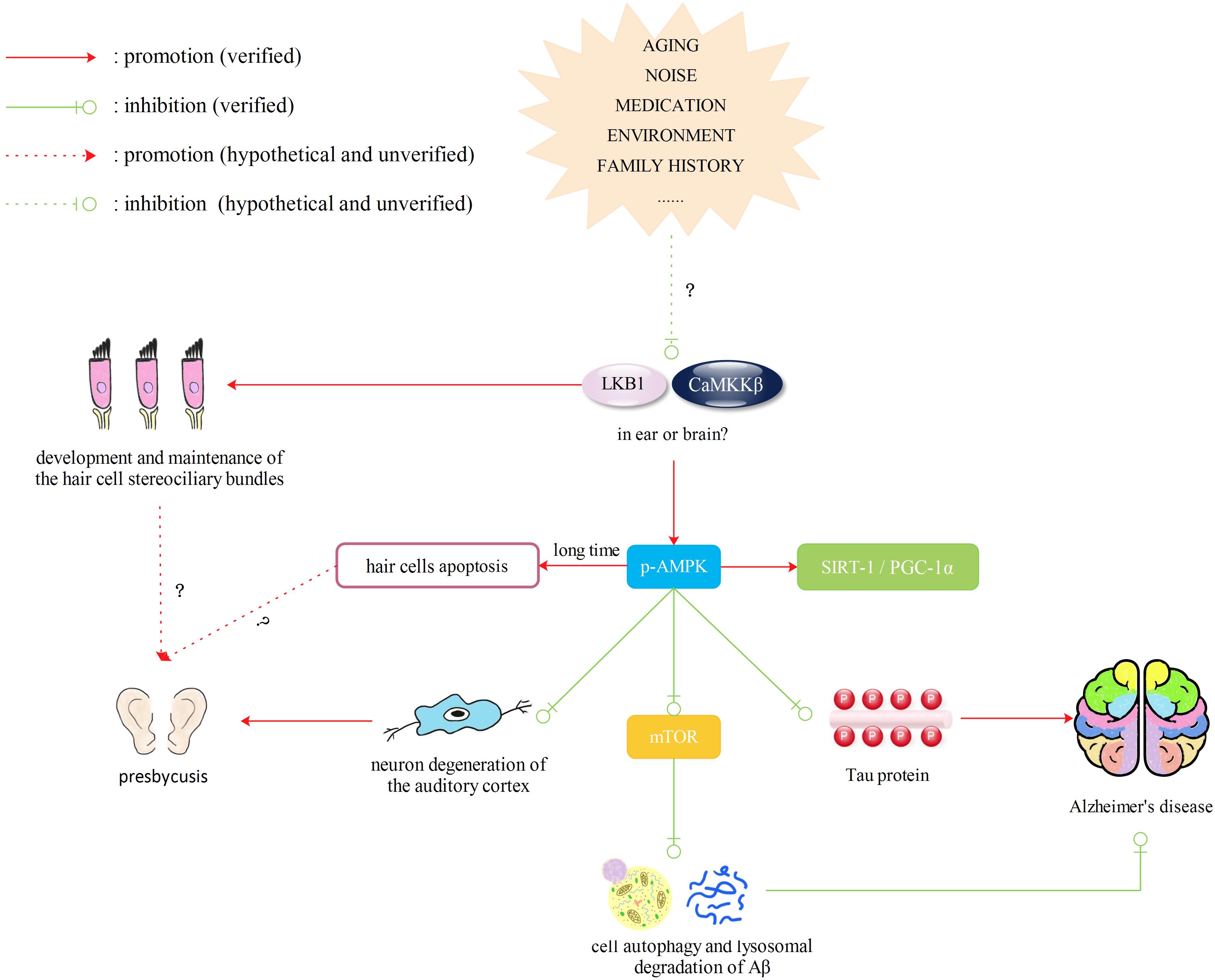
FIGURE 4. Liver kinase B1 (LKB1) and CaMKKβ/AMPK pathway. Decreased activity of AMPK will lead to abnormalities in mitochondrial synthesis and normal functions, such as oxidative respiration, as well as alterations of autophagy, which render cells unable to handle changes in the microenvironment. Decreased activity of AMPK will also cause less degradation of Aβ and more phosphorylation of tau. LKB1 and CaMKKβ are required for the activation of AMPK and are important for the stability of stereocilia in hair cells and protection of auditory cortex neurons, respectively. Although there is currently no evidence showing that LKB1 and CaMKKβ change in the ear or brain due to aging, the environment, diet or other factors, we speculate that if expression of LKB 1 and CaMKKβ is altered by the above factors, they would not only cause hearing loss but also affect downstream phosphorylation of AMPK, leading to AD. However, the activation of AMPK may exert a dual influence, which may have an opposite effect on cell death and survival.
The above-mentioned publications may guide us in studying the relationship between dementia and ARHL. We speculate that expression or activity of SIRT1/PGC-1α and LKB1/AMPK may be changed by factors such as aging, the environment and medication. The expression or activity change may cause dysfunction of mitochondrial synthesis, oxidative respiration, and autophagy, leading to abnormalities of mtROS signal transduction and activation of downstream VEGFR2 transcription, followed by the development of both dementia and presbycusis. These two diseases may also influence the progression of each other as they have common pathological pathways and common targets. Undoubtedly, more research is necessary to confirm the role of these pathways. If we can find a common mechanism and verify that hearing loss is an early manifestation of dementia, it would be meaningful for the early diagnosis and prevention of dementia.
Conclusion
Due to the non-renewable characteristic of neurons, there are no completely effective methods for curing dementia or presbycusis. With the increasing aging population, the number of people suffering from AD or other dementias and hearing disability will also increase. The factors that influence dementia and age-related hearing impairment are highly complex and involve not only age but also the environment, genetics, lifestyle, drugs and other factors. As a result, considering how to delay or prevent the incidence of dementia and how to delay the neurodegeneration in patients with dementia is important. Although pathological changes of the auditory system have been observed in patients or mice with AD, as mentioned in Section “Pathological Changes of the Auditory System in Patients with AD,” and a group of epidemiological studies have revealed that ARHL may be a risk factor for cognitive decline, dementia or AD, as mentioned in Section “ARHL May be a Risk Factor for Cognitive Decline, Dementia or AD,” the specific molecular mechanism linking these diseases is still unknown. It is also still not clear whether the relationship is unidirectional or bidirectional or if they are both the clinical manifestations of aging. Therefore, in vivo experiments are necessary if we are to explore the relationship between AD and ARHL at the molecular biology level. For example, we may make full use of transgenic AD mouse models, such as APP/PS1 mice, 3xTg-AD mice and 5x-FAD mice, to explore whether AD can cause more severe auditory dysfunction compared to non-transgenic mice in the same background. To explore whether ARHL can cause cognitive changes, C57BL6/J mice (a strain that develops late-onset sensorineural hearing impairment) (Noben-Trauth et al., 2003) and CBA/CaJ mice (a strain that has normal hearing throughout life) are good experiment and control groups, respectively. To our expectation, pathological changes have been found in some AD mouse models and cognitive decline has been verified in old C57BL6/J mice compared to CBA/CaJ mice of the same age, according to existing research. Although these are only patterns of manifestations, they support our belief that certain correlations really exist between ARHL and cognitive decline, dementia or AD. The next step is to explore the molecular mechanism, and with the help of a series of molecular biological techniques, we can try to validate whether the expression of proteins such as VEGF, Sirt1, PGC-1α, LKB1, CaMKKβ or AMPK has the same tendency of variation in the peripheral auditory system, central auditory system, temporal lobes and hippocampus. However, we need to overcome obstacles, such as the long time period needed for experiments, since AD and ARHL model mice require at least 6 months or more to exhibit their phenotype. In addition, we need a large sample size due to the inherent variability of the cognitive assessment made by the Morris water maze or other behavioral experiments on mice. In brief, if a common specific target or pathway is found, drugs aimed at this common target can be developed, which will be of great practical value for the early diagnosis and prevention of dementia and presbycusis.
Author Contributions
MX and YShu: substantial contributions to the conception or design of the work. YShe, BY, PC, QW, and CF: drafting the work or revising it critically for important intellectual content agreement to be accountable for all aspects of the work in ensuring that questions related to the accuracy or integrity of any part of the work are appropriately investigated and resolved. MX: final approval of the version to be published.
Funding
This work was supported by the National Natural Science Foundation of China (Grant Nos. 81271088 and 81670926), Science and Technology Commission of Shanghai Municipality, China (Grant Nos. 15411950303 and 14DZ2260300), and Doctoral Innovation Fund of Shanghai Jiaotong University School of Medicine (Grant No. BXJ201722).
Conflict of Interest Statement
The authors declare that the research was conducted in the absence of any commercial or financial relationships that could be construed as a potential conflict of interest.
Abbreviations
Aβ, amyloid β-protein; AD, Alzheimer’s disease; AMPK, AMP-activated protein kinase; ApoE, apolipoprotein E; ARHL, age-related hearing loss; BACE1, β-secreatase1; BDNF, brain-derived neurotrophic factor; CaMKKβ, calcium/calmodulin-dependent protein kinase kinaseβ; CAP, central auditory processing; EEG, electroencephalogram; FNDC5, fibronectin type III domain-containing protein 5; LKB1, liver kinase B1; mtDNA, mitochondrial DNA; NFTs, neurofibrillary tangles; p66Shc, 66-kilodahon isoform of Shc gene products; PCG-1α, PPARγ coactivator-1; ROS, reactive oxygen species; SGNs, spiral ganglion neurons; SPs, senile plaques; VEGF, vascular endothelial growth factor.
References
Adrait, A., Perrot, X., Nguyen, M. F., Gueugnon, M., Petitot, C., Collet, L., et al. (2017). Do hearing aids influence behavioral and psychological symptoms of dementia and quality of life in hearing impaired Alzheimer’s disease patients and their caregivers? J. Alzheimers Dis. 58, 109–121. doi: 10.3233/JAD-160792
Aitkin, L. M., and Webster, W. R. (1971). Tonotopic organization in the medial geniculate body of the cat. Brain Res. 26, 402–405. doi: 10.1016/S0006-8993(71)80015-X
Amieva, H., Ouvrard, C., Giulioli, C., Meillon, C., Rullier, L., and Dartigues, J. F. (2015). Self-reported hearing loss, hearing AIDS, and cognitive decline in elderly adults: a 25-year study. J. Am. Geriatr. Soc. 63, 2099–2104. doi: 10.1111/jgs.13649
Anekonda, T. S., Wadsworth, T. L., Sabin, R., Frahler, K., Harris, C., Petriko, B., et al. (2011). Phytic acid as a potential treatment for Alzheimer’s pathology: evidence from animal and in vitro models. J. Alzheimers Dis. 23, 21–35. doi: 10.3233/JAD-2010-101287
Behrman, S., Chouliaras, L., and Ebmeier, K. P. (2014). Considering the senses in the diagnosis and management of dementia. Maturitas 77, 305–310. doi: 10.1016/j.maturitas.2014.01.003
Benarroch, E. E. (2010). Olfactory system: functional organization and involvement in neurodegenerative disease. Neurology 75, 1104–1109. doi: 10.1212/WNL.0b013e3181f3db84
Bennett, D. A., Schneider, J. A., Tang, Y., Arnold, S. E., and Wilson, R. S. (2006). The effect of social networks on the relation between Alzheimer’s disease pathology and level of cognitive function in old people: a longitudinal cohort study. Lancet Neurol. 5, 406–412. doi: 10.1016/S1474-4422(06)70417-3
Bernabei, R., Bonuccelli, U., Maggi, S., Marengoni, A., Martini, A., Memo, M., et al. (2014). Hearing loss and cognitive decline in older adults: questions and answers. Aging Clin. Exp. Res. 26, 567–573. doi: 10.1007/s40520-014-0266-3
Burger, S., Noack, M., Kirazov, L. P., Kirazov, E. P., Naydenov, C. L., Kouznetsova, E., et al. (2009). Vascular endothelial growth factor (VEGF) affects processing of amyloid precursor protein and beta-amyloidogenesis in brain slice cultures derived from transgenic Tg2576 mouse brain. Int. J. Dev. Neurosci. 27, 517–523. doi: 10.1016/j.ijdevneu.2009.06.011
Canto, C., Jiang, L. Q., Deshmukh, A. S., Mataki, C., Coste, A., Lagouge, M., et al. (2010). Interdependence of AMPK and SIRT1 for metabolic adaptation to fasting and exercise in skeletal muscle. Cell Metab. 11, 213–219. doi: 10.1016/j.cmet.2010.02.006
Cardin, V. (2016). Effects of aging and adult-onset hearing loss on cortical auditory regions. Front. Neurosci. 10:199. doi: 10.3389/fnins.2016.00199
Castiglione, A., Benatti, A., Velardita, C., Favaro, D., Padoan, E., Severi, D., et al. (2016). Aging, cognitive decline and hearing loss: effects of auditory rehabilitation and training with hearing aids and cochlear implants on cognitive function and depression among older adults. Audiol. Neurootol. 21(Suppl. 1), 21–28. doi: 10.1159/000448350
Chakraborty, G., Saito, M., Mao, R. F., Wang, R., Vadasz, C., and Saito, M. (2008). Lithium blocks ethanol-induced modulation of protein kinases in the developing brain. Biochem. Biophys. Res. Commun. 367, 597–602. doi: 10.1016/j.bbrc.2008.01.004
Chandel, N. S., Maltepe, E., Goldwasser, E., Mathieu, C. E., Simon, M. C., and Schumacker, P. T. (1998). Mitochondrial reactive oxygen species trigger hypoxia-induced transcription. Proc. Natl. Acad. Sci. U.S.A. 95, 11715–11720. doi: 10.1073/pnas.95.20.11715
Cheeseman, M. T., Tyrer, H. E., Williams, D., Hough, T. A., Pathak, P., Romero, M. R., et al. (2011). HIF-VEGF pathways are critical for chronic otitis media in Junbo and Jeff mouse mutants. PLoS Genet. 7:e1002336. doi: 10.1371/journal.pgen.1002336
Chen, K., Thomas, S. R., Albano, A., Murphy, M. P., and Keaney, J. J. (2004). Mitochondrial function is required for hydrogen peroxide-induced growth factor receptor transactivation and downstream signaling. J. Biol. Chem. 279, 35079–35086. doi: 10.1074/jbc.M404859200
Chen, X., Zhao, X., Cai, H., Sun, H., Hu, Y., Huang, X., et al. (2017). The role of sodium hydrosulfide in attenuating the aging process via PI3K/AKT and CaMKKbeta/AMPK pathways. Redox Biol. 12, 987–1003. doi: 10.1016/j.redox.2017.04.031
Cheng, A., Wan, R., Yang, J. L., Kamimura, N., Son, T. G., Ouyang, X., et al. (2012). Involvement of PGC-1alpha in the formation and maintenance of neuronal dendritic spines. Nat. Commun. 3:1250. doi: 10.1038/ncomms2238
Colavitti, R., Pani, G., Bedogni, B., Anzevino, R., Borrello, S., Waltenberger, J., et al. (2002). Reactive oxygen species as downstream mediators of angiogenic signaling by vascular endothelial growth factor receptor-2/KDR. J. Biol. Chem. 277, 3101–3108. doi: 10.1074/jbc.M107711200
Daval, M., Ferre, P., and Foufelle, F. (2006). AMPK, an active player in the control of metabolism. J. Soc. Biol. 200, 99–105. doi: 10.1051/jbio:2006013
Dawes, P., Emsley, R., Cruickshanks, K. J., Moore, D. R., Fortnum, H., Edmondson-Jones, M., et al. (2015). Hearing loss and cognition: the role of hearing AIDS, social isolation and depression. PLoS One 10:e0119616. doi: 10.1371/journal.pone.0119616
de Carvalho, I. A., Beard, J., Chatterji, S., Jordan, J. E., and Harvey, A. (2015). World Report on aging and Health. Available at: www.who.int/entity/ageing/publications/world-report-2015/en/
Deal, J. A., Betz, J., Yaffe, K., Harris, T., Purchase-Helzner, E., Satterfield, S., et al. (2017). Hearing impairment and incident dementia and cognitive decline in older adults: the health ABC study. J. Gerontol. A Biol. Sci. Med. Sci. 72, 703–709. doi: 10.1093/gerona/glw069
Eckert, M. A., Cute, S. L., Vaden, K. J., Kuchinsky, S. E., and Dubno, J. R. (2012). Auditory cortex signs of age-related hearing loss. J. Assoc. Res. Otolaryngol. 13, 703–713. doi: 10.1007/s10162-012-0332-5
Elliott, E., Atlas, R., Lange, A., and Ginzburg, I. (2005). Brain-derived neurotrophic factor induces a rapid dephosphorylation of tau protein through a PI-3 Kinase signalling mechanism. Eur. J. Neurosci. 22, 1081–1089. doi: 10.1111/j.1460-9568.2005.04290.x
Finck, B. N., and Kelly, D. P. (2006). PGC-1 coactivators: inducible regulators of energy metabolism in health and disease. J. Clin. Invest. 116, 615–622. doi: 10.1172/JCI27794
Gallacher, J., Ilubaera, V., Ben-Shlomo, Y., Bayer, A., Fish, M., Babisch, W., et al. (2012). Auditory threshold, phonologic demand, and incident dementia. Neurology 79, 1583–1590. doi: 10.1212/WNL.0b013e31826e263d
Gates, G. A., Cobb, J. L., Linn, R. T., Rees, T., Wolf, P. A., and D’Agostino, R. B. (1996). Central auditory dysfunction, cognitive dysfunction, and dementia in older people. Arch. Otolaryngol. Head Neck Surg. 122, 161–167. doi: 10.1001/archotol.1996.01890140047010
Gates, G. A., Gibbons, L. E., McCurry, S. M., Crane, P. K., Feeney, M. P., and Larson, E. B. (2010). Executive dysfunction and presbycusis in older persons with and without memory loss and dementia. Cogn. Behav. Neurol. 23, 218–223. doi: 10.1097/WNN.0b013e3181d748d7
Gates, G. A., and Mills, J. H. (2005). Presbycusis. Lancet 366, 1111–1120. doi: 10.1016/S0140-6736(05)67423-5
Gennis, V., Garry, P. J., Haaland, K. Y., Yeo, R. A., and Goodwin, J. S. (1991). Hearing and cognition in the elderly. New findings and a review of the literature. Arch. Intern. Med. 151, 2259–2264. doi: 10.1001/archinte.1991.00400110105021
Glenner, G. G., and Wong, C. W. (1984). Alzheimer’s disease: initial report of the purification and characterization of a novel cerebrovascular amyloid protein. Biochem. Biophys. Res. Commun. 120, 885–890. doi: 10.1016/S0006-291X(84)80190-4
Goll, J. C., Kim, L. G., Hailstone, J. C., Lehmann, M., Buckley, A., Crutch, S. J., et al. (2011). Auditory object cognition in dementia. Neuropsychologia 49, 2755–2765. doi: 10.1016/j.neuropsychologia.2011.06.004
Greco, S. J., Sarkar, S., Casadesus, G., Zhu, X., Smith, M. A., Ashford, J. W., et al. (2009a). Leptin inhibits glycogen synthase kinase-3beta to prevent tau phosphorylation in neuronal cells. Neurosci. Lett. 455, 191–194. doi: 10.1016/j.neulet.2009.03.066
Greco, S. J., Sarkar, S., Johnston, J. M., and Tezapsidis, N. (2009b). Leptin regulates tau phosphorylation and amyloid through AMPK in neuronal cells. Biochem. Biophys. Res. Commun. 380, 98–104. doi: 10.1016/j.bbrc.2009.01.041
Gruden, M. A., Davidova, T. B., Malisauskas, M., Sewell, R. D., Voskresenskaya, N. I., Wilhelm, K., et al. (2007). Differential neuroimmune markers to the onset of Alzheimer’s disease neurodegeneration and dementia: autoantibodies to Abeta((25-35)) oligomers, S100b and neurotransmitters. J. Neuroimmunol. 186, 181–192. doi: 10.1016/j.jneuroim.2007.03.023
Guarente, L. (2013). Introduction: sirtuins in aging and diseases. Methods Mol. Biol. 1077, 3–10. doi: 10.1007/978-1-62703-637-5_1
Hackett, T. A. (2015). Anatomic organization of the auditory cortex. Handb. Clin. Neurol. 129, 27–53. doi: 10.1016/B978-0-444-62630-1.00002-0
Han, C., Linser, P., Park, H. J., Kim, M. J., White, K., Vann, J. M., et al. (2016). Sirt1 deficiency protects cochlear cells and delays the early onset of age-related hearing loss in C57BL/6 mice. Neurobiol. Aging 43, 58–71. doi: 10.1016/j.neurobiolaging.2016.03.023
Hardie, D. G. (2008). AMPK: a key regulator of energy balance in the single cell and the whole organism. Int. J. Obes. 32(Suppl. 4), S7–S12. doi: 10.1038/ijo.2008.116
Hawkins, J. J., Johnsson, L. G., and Preston, R. E. (1972). Cochlear microvasculature in normal and damaged ears. Laryngoscope 82, 1091–1104. doi: 10.1288/00005537-197207000-00001
Hill, K., Yuan, H., Wang, X., and Sha, S. H. (2016). Noise-induced loss of hair cells and cochlear synaptopathy are mediated by the activation of AMPK. J. Neurosci. 36, 7497–7510. doi: 10.1523/JNEUROSCI.0782-16.2016
Holback, S., Adlerz, L., and Iverfeldt, K. (2005). Increased processing of APLP2 and APP with concomitant formation of APP intracellular domains in BDNF and retinoic acid-differentiated human neuroblastoma cells. J. Neurochem. 95, 1059–1068. doi: 10.1111/j.1471-4159.2005.03440.x
Hyman, B. T. (1998). New neuropathological criteria for Alzheimer disease. Arch. Neurol. 55, 1174–1176. doi: 10.1001/archneur.55.9.1174
Idrizbegovic, E., Hederstierna, C., Dahlquist, M., Kampfe, N. C., Jelic, V., and Rosenhall, U. (2011). Central auditory function in early Alzheimer’s disease and in mild cognitive impairment. Age. Ageing 40, 249–254. doi: 10.1093/ageing/afq168
Jager, S., Handschin, C., St-Pierre, J., and Spiegelman, B. M. (2007). AMP-activated protein kinase (AMPK) action in skeletal muscle via direct phosphorylation of PGC-1alpha. Proc. Natl. Acad. Sci. U.S.A. 104, 12017–12022. doi: 10.1073/pnas.0705070104
Kakinuma, Y., Furihata, M., Akiyama, T., Arikawa, M., Handa, T., Katare, R. G., et al. (2010). Donepezil, an acetylcholinesterase inhibitor against Alzheimer’s dementia, promotes angiogenesis in an ischemic hindlimb model. J. Mol. Cell. Cardiol. 48, 680–693. doi: 10.1016/j.yjmcc.2009.11.010
Kalaria, R. N., Cohen, D. L., Premkumar, D. R., Nag, S., LaManna, J. C., and Lust, W. D. (1998). Vascular endothelial growth factor in Alzheimer’s disease and experimental cerebral ischemia. Brain Res. Mol. Brain Res. 62, 101–105. doi: 10.1016/S0169-328X(98)00190-9
Kaur, A., Webster, M. R., Marchbank, K., Behera, R., Ndoye, A., Kugel, C. R., et al. (2016). sFRP2 in the aged microenvironment drives melanoma metastasis and therapy resistance. Nature 532, 250–254. doi: 10.1038/nature17392
Kay, D. W., Roth, M., and Beamish, P. (1964). Old age mental disorders in newcastle upon Tyne. II. A study of possible social and medical causes. Br. J. Psychiatry 110, 668–682. doi: 10.1192/bjp.110.468.668
Kearney, J. B., Kappas, N. C., Ellerstrom, C., DiPaola, F. W., and Bautch, V. L. (2004). The VEGF receptor flt-1 (VEGFR-1) is a positive modulator of vascular sprout formation and branching morphogenesis. Blood 103, 4527–4535. doi: 10.1182/blood-2003-07-2315
Kefas, B. A., Cai, Y., Kerckhofs, K., Ling, Z., Martens, G., Heimberg, H., et al. (2004). Metformin-induced stimulation of AMP-activated protein kinase in beta-cells impairs their glucose responsiveness and can lead to apoptosis. Biochem. Pharmacol. 68, 409–416. doi: 10.1016/j.bcp.2004.04.003
Kelly, D. P., and Scarpulla, R. C. (2004). Transcriptional regulatory circuits controlling mitochondrial biogenesis and function. Genes Dev. 18, 357–368. doi: 10.1101/gad.1177604
Kim, Y., Kim, S., Tatsunami, R., Yamamura, H., Fukai, T., and Ushio-Fukai, M. (2017). ROS-induced ROS release orchestrated by Nox4, Nox2, and mitochondria in VEGF signaling and angiogenesis. Am. J. Physiol. Cell Physiol. 312, C749–C764. doi: 10.1152/ajpcell.00346.2016
Kong, W. J., Hu, Y. J., Wang, Q., Wang, Y., Han, Y. C., Cheng, H. M., et al. (2006). The effect of the mtDNA4834 deletion on hearing. Biochem. Biophys. Res. Commun. 344, 425–430. doi: 10.1016/j.bbrc.2006.03.060
Koutsimpelas, D., Stripf, T., Heinrich, U. R., Mann, W. J., and Brieger, J. (2007). Expression of vascular endothelial growth factor and basic fibroblast growth factor in sporadic vestibular schwannomas correlates to growth characteristics. Otol. Neurotol. 28, 1094–1099. doi: 10.1097/MAO.0b013e31814b2787
Krum, J. M., and Khaibullina, A. (2003). Inhibition of endogenous VEGF impedes revascularization and astroglial proliferation: roles for VEGF in brain repair. Exp. Neurol. 181, 241–257. doi: 10.3201/eid0906.020485
Kumar, R., Chaterjee, P., Sharma, P. K., Singh, A. K., Gupta, A., Gill, K., et al. (2013). Sirtuin1: a promising serum protein marker for early detection of Alzheimer’s disease. PLoS One 8:e61560. doi: 10.1371/journal.pone.0061560
Lagouge, M., Argmann, C., Gerhart-Hines, Z., Meziane, H., Lerin, C., Daussin, F., et al. (2006). Resveratrol improves mitochondrial function and protects against metabolic disease by activating SIRT1 and PGC-1alpha. Cell 127, 1109–1122. doi: 10.1016/j.cell.2006.11.013
Lim, D. J., and Birck, H. (1971). Ultrastructural pathology of the middle ear mucosa in serous otitis media. Ann. Otol. Rhinol. Laryngol. 80, 838–853. doi: 10.1177/000348947108000611
Lin, F. R., Metter, E. J., O’Brien, R. J., Resnick, S. M., Zonderman, A. B., and Ferrucci, L. (2011). Hearing loss and incident dementia. Arch. Neurol. 68, 214–220. doi: 10.1001/archneurol.2010.362
Lin, F. R., Yaffe, K., Xia, J., Xue, Q., Harris, T. B., Purchase-Helzner, E., et al. (2013). Hearing loss and cognitive decline in older adults. JAMA Intern. Med. 173, 293–299. doi: 10.1001/jamainternmed.2013.1868
Livingston, G., Sommerlad, A., Orgeta, V., Costafreda, S. G., Huntley, J., Ames, D., et al. (2017). Dementia prevention, intervention, and care. Lancet 390, 2673–2734. doi: 10.1016/S0140-6736(17)31363-6
London, N. R., and Gurgel, R. K. (2014). The role of vascular endothelial growth factor and vascular stability in diseases of the ear. Laryngoscope 124, E340–E346. doi: 10.1002/lary.24564
Lonsbury-Martin, B. L., Martin, G. K., and Hannley, M. T. (2009). “Physiology of the auditory and vestibular systems,” in The Ballenger’s Otolaryngology: Head and Neck Surgery, 17th Edn, eds B. James, J. R. Snow, and P. Ashley Wackym (Shelton, CT: People’s Medical Publishing House), 45–80.
Manczak, M., Anekonda, T. S., Henson, E., Park, B. S., Quinn, J., and Reddy, P. H. (2006). Mitochondria are a direct site of A beta accumulation in Alzheimer’s disease neurons: implications for free radical generation and oxidative damage in disease progression. Hum. Mol. Genet. 15, 1437–1449. doi: 10.1093/hmg/ddl066
Markaryan, A., Nelson, E. G., and Hinojosa, R. (2009). Quantification of the mitochondrial DNA common deletion in presbycusis. Laryngoscope 119, 1184–1189. doi: 10.1002/lary.20218
Masters, C. L., Bateman, R., Blennow, K., Rowe, C. C., Sperling, R. A., and Cummings, J. L. (2015). Alzheimer’s disease. Nat. Rev. Dis. Primers 1:15056. doi: 10.1038/nrdp.2015.56
Mathers, C. D., and Loncar, D. (2005). Updated Projections of Global Mortality and Burden of Disease, 2002-2030: Data Sources, Methods and Results. Geneva: World Health Organization.
McCarty, M. F., DiNicolantonio, J. J., and O’Keefe, J. H. (2015). Ketosis may promote brain macroautophagy by activating Sirt1 and hypoxia-inducible factor-1. Med. Hypotheses 85, 631–639. doi: 10.1016/j.mehy.2015.08.002
Meister, H., Rählmann, S., Walger, M., Margolf-Hackl, S., and Kießling, J. (2015). Hearing aid fitting in older persons with hearing impairment: the influence of cognitive function, age, and hearing loss on hearing aid benefit. Clin. Interv. Aging 10, 435–443. doi: 10.2147/CIA.S77096
Men, Y., Zhang, A., Li, H., Zhang, T., Jin, Y., Li, H., et al. (2015). LKB1 is required for the development and maintenance of stereocilia in inner ear hair cells in mice. PLoS One 10:e0135841. doi: 10.1371/journal.pone.0135841
Mills, J. H., Megerian, C. A., and Lambert, P. R. (2009). “Presbyacusis and presbyastasis,” in the Ballenger’s Otolaryngology: Head and Neck Surgery, 17th Edn, eds B. James, J. R. Snow, and P. Ashley Wackym (Shelton, CT: People’s Medical Publishing House), 333–343.
Mulrow, C. D., Aguilar, C., Endicott, J. E., Tuley, M. R., Velez, R., Charlip, W. S., et al. (1990). Quality-of-life changes and hearing impairment. A randomized trial. Ann. Intern. Med. 113, 188–194. doi: 10.7326/0003-4819-113-3-188
Murer, M. G., Boissiere, F., Yan, Q., Hunot, S., Villares, J., Faucheux, B., et al. (1999). An immunohistochemical study of the distribution of brain-derived neurotrophic factor in the adult human brain, with particular reference to Alzheimer’s disease. Neuroscience 88, 1015–1032. doi: 10.1016/S0306-4522(98)00219-X
Nguyen, M. F., Bonnefoy, M., Adrait, A., Gueugnon, M., Petitot, C., Collet, L., et al. (2017). Efficacy of hearing aids on the cognitive status of patients with Alzheimer’s disease and hearing loss: a multicenter controlled randomized trial. J. Alzheimers Dis. 58, 123–137. doi: 10.3233/JAD-160793
Noben-Trauth, K., Zheng, Q. Y., and Johnson, K. R. (2003). Association of cadherin 23 with polygenic inheritance and genetic modification of sensorineural hearing loss. Nat. Genet. 35, 21–23. doi: 10.1038/ng1226
O’Leary, T. P., Shin, S., Fertan, E., Dingle, R. N., Almuklass, A., Gunn, R. K., et al. (2017). Reduced acoustic startle response and peripheral hearing loss in the 5xFAD mouse model of Alzheimer’s disease. Genes Brain Behav. 16, 554–563. doi: 10.1111/gbb.12370
Omata, Y., Tharasegaran, S., Lim, Y. M., Yamasaki, Y., Ishigaki, Y., Tatsuno, T., et al. (2016). Expression of amyloid-beta in mouse cochlear hair cells causes an early-onset auditory defect in high-frequency sound perception. Aging 8, 427–439. doi: 10.18632/aging.100899
Panza, F., Solfrizzi, V., and Logroscino, G. (2015). Age-related hearing impairment – a risk factor and frailty marker for dementia and AD. Nat. Rev. Neurol. 11, 166–175. doi: 10.1038/nrneurol.2015.12
Park, H., and Poo, M. M. (2013). Neurotrophin regulation of neural circuit development and function. Nat. Rev. Neurosci. 14, 7–23. doi: 10.1038/nrn3379
Parvizi, J., Van Hoesen, G. W., and Damasio, A. (2001). The selective vulnerability of brainstem nuclei to Alzheimer’s disease. Ann. Neurol. 49, 53–66. doi: 10.1002/1531-8249(200101)49:1<53::AID-ANA30>3.0.CO;2-Q
Pati, S., Orsi, S. A., Moore, A. N., and Dash, P. K. (2009). Intra-hippocampal administration of the VEGF receptor blocker PTK787/ZK222584 impairs long-term memory. Brain Res. 1256, 85–91. doi: 10.1016/j.brainres.2008.11.086
Pauly, M., Daussin, F., Burelle, Y., Li, T., Godin, R., Fauconnier, J., et al. (2012). AMPK activation stimulates autophagy and ameliorates muscular dystrophy in the mdx mouse diaphragm. Am. J. Pathol. 181, 583–592. doi: 10.1016/j.ajpath.2012.04.004
Peelle, J. E., Troiani, V., Grossman, M., and Wingfield, A. (2011). Hearing loss in older adults affects neural systems supporting speech comprehension. J. Neurosci. 31, 12638–12643. doi: 10.1523/JNEUROSCI.2559-11.2011
Peng, S., Wuu, J., Mufson, E. J., and Fahnestock, M. (2005). Precursor form of brain-derived neurotrophic factor and mature brain-derived neurotrophic factor are decreased in the pre-clinical stages of Alzheimer’s disease. J. Neurochem. 93, 1412–1421. doi: 10.1111/j.1471-4159.2005.03135.x
Phillips, H. S., Hains, J. M., Armanini, M., Laramee, G. R., Johnson, S. A., and Winslow, J. W. (1991). BDNF mRNA is decreased in the hippocampus of individuals with Alzheimer’s disease. Neuron 7, 695–702. doi: 10.1016/0896-6273(91)90273-3
Picciotti, P., Torsello, A., Wolf, F. I., Paludetti, G., Gaetani, E., and Pola, R. (2004). Age-dependent modifications of expression level of VEGF and its receptors in the inner ear. Exp. Gerontol. 39, 1253–1258. doi: 10.1016/j.exger.2004.06.003
Picciotti, P. M., Fetoni, A. R., Paludetti, G., Wolf, F. I., Torsello, A., Troiani, D., et al. (2006). Vascular endothelial growth factor (VEGF) expression in noise-induced hearing loss. Hear. Res. 214, 76–83. doi: 10.1016/j.heares.2006.02.004
Plotkin, S. R., Stemmer-Rachamimov, A. O., Barker, F. N., Halpin, C., Padera, T. P., Tyrrell, A., et al. (2009). Hearing improvement after bevacizumab in patients with neurofibromatosis type 2. N. Engl. J. Med. 361, 358–367. doi: 10.1056/NEJMoa0902579
Provias, J., and Jeynes, B. (2014). Reduction in vascular endothelial growth factor expression in the superior temporal, hippocampal, and brainstem regions in Alzheimer’s disease. Curr. Neurovasc. Res. 11, 202–209. doi: 10.2174/1567202611666140520122316
Rosenstein, J. M., Krum, J. M., and Ruhrberg, C. (2010). VEGF in the nervous system. Organogenesis 6, 107–114. doi: 10.4161/org.6.2.11687
Rüb, U., Stratmann, K., Heinsen, H., Turco, D. D., Seidel, K., Dunnen, W. D., et al. (2016). The brainstem tau cytoskeletal pathology of Alzheimer’s disease: a brief historical overview and description of its anatomical distribution pattern, evolutional features, pathogenetic and clinical relevance. Curr Alzheimer Res 13, 1178–1197. doi: 10.2174/1567205013666160606100509
Saha, A. K., and Ruderman, N. B. (2003). Malonyl-CoA and AMP-activated protein kinase: an expanding partnership. Mol. Cell. Biochem. 253, 65–70. doi: 10.1023/A:1026053302036
Schulz, E., Dopheide, J., Schuhmacher, S., Thomas, S. R., Chen, K., Daiber, A., et al. (2008). Suppression of the JNK pathway by induction of a metabolic stress response prevents vascular injury and dysfunction. Circulation 118, 1347–1357. doi: 10.1161/CIRCULATIONAHA.108.784298
Shi, X. (2009). Cochlear pericyte responses to acoustic trauma and the involvement of hypoxia-inducible factor-1alpha and vascular endothelial growth factor. Am. J. Pathol. 174, 1692–1704. doi: 10.2353/ajpath.2009.080739
Sinha, U. K., Hollen, K. M., Rodriguez, R., and Miller, C. A. (1993). Auditory system degeneration in Alzheimer’s disease. Neurology 43, 779–785. doi: 10.1212/WNL.43.4.779
Sondell, M., Lundborg, G., and Kanje, M. (1999). Vascular endothelial growth factor has neurotrophic activity and stimulates axonal outgrowth, enhancing cell survival and Schwann cell proliferation in the peripheral nervous system. J. Neurosci. 19, 5731–5740. doi: 10.1523/JNEUROSCI.19-14-05731.1999
St-Pierre, J., Drori, S., Uldry, M., Silvaggi, J. M., Rhee, J., Jager, S., et al. (2006). Suppression of reactive oxygen species and neurodegeneration by the PGC-1 transcriptional coactivators. Cell 127, 397–408. doi: 10.1016/j.cell.2006.09.024
Takumida, M., Takumida, H., Katagiri, Y., and Anniko, M. (2016). Localization of sirtuins (SIRT1-7) in the aged mouse inner ear. Acta Otolaryngol. 136, 120–131. doi: 10.3109/00016489.2015.1093172
Taljaard, D. S., Olaithe, M., Brennan-Jones, C. G., Eikelboom, R. H., and Bucks, R. S. (2016). The relationship between hearing impairment and cognitive function: a meta-analysis in adults. Clin. Otolaryngol. 41, 718–729. doi: 10.1111/coa.12607
Tavanai, E., and Mohammadkhani, G. (2017). Role of antioxidants in prevention of age-related hearing loss: a review of literature. Eur. Arch. Otorhinolaryngol. 274, 1821–1834. doi: 10.1007/s00405-016-4378-6
Uhlmann, R. F., Larson, E. B., Rees, T. S., Koepsell, T. D., and Duckert, L. G. (1989). Relationship of hearing impairment to dementia and cognitive dysfunction in older adults. JAMA 261, 1916–1919. doi: 10.1001/jama.1989.03420130084028
Vazquez-Martin, A., Corominas-Faja, B., Cufi, S., Vellon, L., Oliveras-Ferraros, C., Menendez, O. J., et al. (2013). The mitochondrial H+-ATP synthase and the lipogenic switch: new core components of metabolic reprogramming in induced pluripotent stem (iPS) cells. Cell Cycle 12, 207–218. doi: 10.4161/cc.23352
Vingtdeux, V., Chandakkar, P., Zhao, H., D’Abramo, C., Davies, P., and Marambaud, P. (2011). Novel synthetic small-molecule activators of AMPK as enhancers of autophagy and amyloid-beta peptide degradation. FASEB J. 25, 219–231. doi: 10.1096/fj.10-167361
Viollet, B., Lantier, L., Devin-Leclerc, J., Hebrard, S., Amouyal, C., Mounier, R., et al. (2009). Targeting the AMPK pathway for the treatment of Type 2 diabetes. Front. Biosci. 14, 3380–3400. doi: 10.2741/3460
Wang, P., Xie, Z. H., Guo, Y. J., Zhao, C. P., Jiang, H., Song, Y., et al. (2011). VEGF-induced angiogenesis ameliorates the memory impairment in APP transgenic mouse model of Alzheimer’s disease. Biochem. Biophys. Res. Commun. 411, 620–626. doi: 10.1016/j.bbrc.2011.07.003
Wang, R., Li, J. J., Diao, S., Kwak, Y. D., Liu, L., Zhi, L., et al. (2013). Metabolic stress modulates Alzheimer’s beta-secretase gene transcription via SIRT1-PPARgamma-PGC-1 in neurons. Cell Metab. 17, 685–694. doi: 10.1016/j.cmet.2013.03.016
Wang, S. E., and Wu, C. H. (2015). Physiological and histological evaluations of the cochlea between 3xTg-AD mouse model of Alzheimer’s diseases and R6/2 mouse model of Huntington’s diseases. Chin. J. Physiol. 58, 359–366. doi: 10.4077/CJP.2015.BAD334
Wang, Y., Jin, K., Mao, X. O., Xie, L., Banwait, S., Marti, H. H., et al. (2007). VEGF-overexpressing transgenic mice show enhanced post-ischemic neurogenesis and neuromigration. J. Neurosci. Res. 85, 740–747. doi: 10.1002/jnr.21169
Wayne, R. V., and Johnsrude, I. S. (2015). A review of causal mechanisms underlying the link between age-related hearing loss and cognitive decline. Ageing Res. Rev. 23, 154–166. doi: 10.1016/j.arr.2015.06.002
Weisova, P., Davila, D., Tuffy, L. P., Ward, M. W., Concannon, C. G., and Prehn, J. H. (2011). Role of 5’-adenosine monophosphate-activated protein kinase in cell survival and death responses in neurons. Antioxid. Redox Signal. 14, 1863–1876. doi: 10.1089/ars.2010.3544
Won, J. S., Im, Y. B., Kim, J., Singh, A. K., and Singh, I. (2010). Involvement of AMP-activated-protein-kinase (AMPK) in neuronal amyloidogenesis. Biochem. Biophys. Res. Commun. 399, 487–491. doi: 10.1016/j.bbrc.2010.07.081
Wong, P. C., Ettlinger, M., Sheppard, J. P., Gunasekera, G. M., and Dhar, S. (2010). Neuroanatomical characteristics and speech perception in noise in older adults. Ear Hear. 31, 471–479. doi: 10.1097/AUD.0b013e3181d709c2
World health Organization [WHO] (2017). Deafness and Hearing Loss. Available at: http://www.who.int/mediacentre/factsheets/fs300/en/
Wortmann, M. (2012). Dementia: a global health priority – highlights from an ADI and world health organization report. Alzheimers Res. Ther. 4:40. doi: 10.1186/alzrt143
Wrann, C. D., White, J. P., Salogiannnis, J., Laznik-Bogoslavski, D., Wu, J., Ma, D., et al. (2013). Exercise induces hippocampal BDNF through a PGC-1alpha/FNDC5 pathway. Cell Metab. 18, 649–659. doi: 10.1016/j.cmet.2013.09.008
Xia, D. Y., Huang, X., Bi, C. F., Mao, L. L., Peng, L. J., and Qian, H. R. (2017). PGC-1alpha or FNDC5 is involved in modulating the effects of abeta1-42 oligomers on suppressing the expression of BDNF, a beneficial factor for inhibiting neuronal apoptosis, abeta deposition and cognitive decline of APP/PS1 Tg mice. Front. Aging Neurosci. 9:65. doi: 10.3389/fnagi.2017.00065
Xu, Z., Zhang, L., Fei, X., Yi, X., Li, W., and Wang, Q. (2014). The miR-29b-Sirt1 axis regulates self-renewal of mouse embryonic stem cells in response to reactive oxygen species. Cell. Signal. 26, 1500–1505. doi: 10.1016/j.cellsig.2014.03.010
Xue, T., Wei, L., Zha, D. J., Qiu, J. H., Chen, F. Q., Qiao, L., et al. (2016). miR-29b overexpression induces cochlear hair cell apoptosis through the regulation of SIRT1/PGC-1alpha signaling: Implications for age-related hearing loss. Int. J. Mol. Med. 38, 1387–1394. doi: 10.3892/ijmm.2016.2735
Yamasoba, T. (2009). [Molecular mechanism of age-related hearing loss: toward its prevention]. Nihon Jibiinkoka Gakkai Kaiho 112, 414–421. doi: 10.3950/jibiinkoka.112.414
Yamasoba, T., Lin, F. R., Someya, S., Kashio, A., Sakamoto, T., and Kondo, K. (2013). Current concepts in age-related hearing loss: epidemiology and mechanistic pathways. Hear. Res. 303, 30–38. doi: 10.1016/j.heares.2013.01.021
Yang, S. P., Bae, D. G., Kang, H. J., Gwag, B. J., Gho, Y. S., and Chae, C. B. (2004). Co-accumulation of vascular endothelial growth factor with beta-amyloid in the brain of patients with Alzheimer’s disease. Neurobiol. Aging 25, 283–290. doi: 10.1016/S0197-4580(03)00111-8
Yu, Y. F., Zhai, F., Dai, C. F., and Hu, J. J. (2011). The relationship between age-related hearing loss and synaptic changes in the hippocampus of C57BL/6J mice. Exp. Gerontol. 46, 716–722. doi: 10.1016/j.exger.2011.04.007
Yun, H., Lee, M., Kim, S. S., and Ha, J. (2005). Glucose deprivation increases mRNA stability of vascular endothelial growth factor through activation of AMP-activated protein kinase in DU145 prostate carcinoma. J. Biol. Chem. 280, 9963–9972. doi: 10.1074/jbc.M412994200
Keywords: presbycusis, Alzheimer’s disease, dementia, ROS, VEGF, PGC-1α, AMPK, mitochondria
Citation: Shen Y, Ye B, Chen P, Wang Q, Fan C, Shu Y and Xiang M (2018) Cognitive Decline, Dementia, Alzheimer’s Disease and Presbycusis: Examination of the Possible Molecular Mechanism. Front. Neurosci. 12:394. doi: 10.3389/fnins.2018.00394
Received: 15 December 2017; Accepted: 22 May 2018;
Published: 08 June 2018.
Edited by:
Claude Alain, Rotman Research Institute (RRI), CanadaCopyright © 2018 Shen, Ye, Chen, Wang, Fan, Shu and Xiang. This is an open-access article distributed under the terms of the Creative Commons Attribution License (CC BY). The use, distribution or reproduction in other forums is permitted, provided the original author(s) and the copyright owner are credited and that the original publication in this journal is cited, in accordance with accepted academic practice. No use, distribution or reproduction is permitted which does not comply with these terms.
*Correspondence: Mingliang Xiang, bWluZ2xpYW5neGlhbmdAMTYzLmNvbQ== Yilai Shu, eWlsYWlzaHVAMTYzLmNvbQ==
†These authors have contributed equally to this work.