- 1Addictions Division, Centre for Addiction and Mental Health, Toronto, ON, Canada
- 2Institute of Medical Science, University of Toronto, Toronto, ON, Canada
- 3Department of Family & Community Medicine, University of Toronto, Toronto, ON, Canada
- 4Department of Pharmacology & Toxicology, University of Toronto, Toronto, ON, Canada
- 5Division of Brain and Therapeutics, Department of Psychiatry, University of Toronto, Toronto, ON, Canada
- 6Campbell Family Mental Health Research Institute, Centre for Addiction and Mental Health, Toronto, ON, Canada
- 7Krembil Centre for Neuroinformatics, Centre for Addiction and Mental Health, Toronto, ON, Canada
Due to size and accessibility, most information about the habenula is derived from rodent studies. To better understand the molecular signature of the habenula we characterized the genes that have high expression in the habenula. We compared anatomical expression profiles of three normal adult human brains and four fetal brains. We used gene set enrichment analyses to determine if genes annotated to specific molecular functions, cellular components, and biological processes are enriched in the habenula. We also tested gene sets related to depression and addiction to determine if they uniquely involve the habenula. As expected, we observed high habenular expression of GPR151, nicotinic cholinergic receptors, and cilia-associated genes (medial division). Genes identified in genetic studies of smoking and associated with nicotine response were enriched in the habenula. Genes associated with major depressive disorder did not have enriched expression in the habenula but genes negatively correlated with hedonic well-being were, providing a link to anhedonia. We observed enrichment of genes associated with diseases that are comorbid with addictions (hematopoiesis, thrombosis, liver cirrhosis, pneumonia, and pulmonary fibrosis) and depression (rheumatoid arthritis, multiple sclerosis, and kidney disease). These inflammatory diseases mark a neuroimmune signature that is supported by genes associated with mast cells, acute inflammatory response, and leukocyte migration. We also found enrichment of cytochrome p450 genes suggesting the habenula is uniquely sensitive to endogenous and xenobiotic compounds. Our results suggest the habenula receives negative reward signals from immune and drug processing molecules. This is consistent with the habenular role in the “anti-reward” system and suggests it may be a key bridge between autoimmune disorders, drug use, and psychiatric diseases.
Introduction
The habenula, located in the epithalamus, links midbrain and hindbrain regions to the forebrain. It’s considered to be at a crossroads, allowing it to act as a conductor of cognitive behaviors (Lecourtier and Kelly, 2007; Hikosaka et al., 2008). Landmark work in monkeys by Matsumoto and Hikosaka (2007) found that habenula neurons provide negative reward signals to midbrain dopamine neurons. Human functional neuroimaging results also find that the habenula encodes negative motivational value (Lawson et al., 2014). However, due to its small size and accessibility, most information about the habenula is derived from animal studies. Specifically, the habenular connections to the dopamine and serotonin systems; and its associations with motivation have spurred its study in animal models of depression and addiction. These studies have been reviewed in the context of psychiatric disease (Hikosaka, 2010; Proulx et al., 2014; Fakhoury, 2017), drug addiction (Lecca et al., 2014; Velasquez et al., 2014; McLaughlin et al., 2017) and withdrawal (Meye et al., 2017).
At the molecular level, gene expression images and profiles of the mouse and rat brain have been used to characterize the habenula (Wagner et al., 2016a,b). By determining which genes are specifically expressed in the habenula these studies have been able to define subregions and link molecular processes. In the human brain, transcriptomic profiling has been used to characterize genes differentially expressed in postmortem studies of depression, addiction, and other psychiatric diseases. However, these studies commonly focus on the frontal cortex or limbic regions (Mistry and Pavlidis, 2010; Colantuoni et al., 2011; Ding et al., 2015; Labonté et al., 2017). While the habenula has been linked to psychiatric disease, its transcriptional profile has not been analyzed in normal or diseased brains beyond a comparison of the 100 most specifically expressed genes (Boulos et al., 2017).
Large-scale efforts by the Allen Institute for Brain Science and their collaborators have led to the creation of genome-wide gene expression atlases of the human brain (Hawrylycz et al., 2012; Miller et al., 2014a). These atlases are anatomically comprehensive, providing transcriptomic profiles of over 200 regions that include the lateral and medial habenula. Unlike past studies of the human habenula which are limited by the resolution of MR imaging, this transcriptomic data enables analysis at the molecular scale.
In this present study, we used human transcriptomic data to determine which genes are specifically expressed in the lateral and medial habenula. We sought to learn if these genes were associated with addiction and depression relative to other brain regions. We also took a broader approach by assessing which cellular components, molecular processes, or functions and disease-associated genes were uniquely expressed in the habenula.
Materials and Methods
Adult Human Brain Gene Expression Data
Gene expression data that comprehensively covers the adult human brain was obtained from the Allen Human Brain Atlas (Hawrylycz et al., 2012). From the six brains assayed in the Atlas, we used only data from the three that had habenula samples (all male). Specifically, the lateral and medial habenula was acquired from two donor brains (ID:9861/H0351.2001, 24 years old; ID:12876/H0351.1009, 57 years old), and one with only a lateral sample (ID:15697/H0351.1016, 55 years old). Postmortem blood was tested for the presence of therapeutic drugs and drugs with abuse potential by the Allen Institute. Caffeine (all 3 donors), theobromine (2 donors), and atropine (1 donor) were detected in the donors at levels that are not considered toxicologically significant. The expression data were extensively annotated and normalized by the Allen Institute for Brain Science (adjusted for array-specific biases, batch, and dissection method). Data from the three donor brains contained 2,674 spatially resolved gene expression profiles, providing expression information for 217 unique named brain regions. Custom 64K Agilent microarrays were used to assay genome-wide expression. The 58,692 probes were filtered to 48,170 that mapped to a gene symbol in the Allen annotations. Details of the procedures used by the Allen Institute researchers are available in the Allen Human Brain Atlas technical white paper1.
Prenatal Human Gene Expression Data
The BrainSpan consortium has created a similar transcriptomic atlas for the normal mid-gestational human brain (Miller et al., 2014a). The medial and lateral habenular nuclei were acquired in all four of the prenatal specimens used for this atlas (15–21 postconception weeks, 3 females and one male). These four specimens passed several exclusion criteria and no neuropathological defects were found by the consortium. Data from the four specimens contained 1,203 spatially resolved gene expression samples, providing expression information for 516 unique named brain regions. The same custom 64K Agilent microarrays that were used for the adult atlas were used to profile expression. Details of the procedures used by the Allen Institute researchers are available in the Brainspan Atlas of the Developing Human Brain technical white paper2.
Region-Specific Expression Analysis
The limma software package was used to detect probes that are specifically expressed in the lateral and medial habenula (Ritchie et al., 2015). For each microarray probe, linear models were fit with coefficients for donor and region of interest for the lateral and medial habenula separately. In other words, expression of given probe across the complete set of expression measurements (adult: 2,674, fetal: 1,203) was predicted with a donor indicator variable and if the sampled region was the lateral and medial habenula (calculated separately). The empirical Bayes moderation method implemented in limma was used to calculate moderated t statistics and corresponding p-values (shrinks variances to a common value).
Estimation of Cell-Type Proportion
The markerGeneProfile (MGP) R package was used to estimate cell type proportions from the expression profiles (Mancarci et al., 2017). This method uses the first principal component obtained from the expression of a set of cell-type specific markers to estimate the relative abundance of a cell type. For cell-type markers, we used genes from a study of healthy temporal cortex tissue that was obtained during resective surgery (Darmanis et al., 2015). This study provided the top 21 most enriched genes for astrocytes, neurons, oligodendrocytes, oligodendrocyte precursors, microglia and endothelial cell-types (via transcriptomic clustering, Supplementary Table S3; (Darmanis et al., 2015)). Default parameters for the mgpEstimate function were used with the geneTransform function set to the identity function. Proportions were estimated separately for each donor brain. Within a brain, proportions were mean averaged across multiple samples for each brain region. Regional proportions for each cell-type were then scaled and then mean averaged across the brains. Ranks were then computed across all regions for a specific cell-type to provide relative values of estimated proportions.
Gene Set Enrichment Analysis
To summarize the probe level statistics we used the probe to gene mappings provided by the Allen Institute (Hawrylycz et al., 2012; Miller et al., 2014b). When many probes map to the same gene, we summarized the region-specific expression results by choosing the probe with the lowest p-value to represent the gene. For a given region of interest, p-values were combined with the direction of effect for the 20,779 genes (signed p-values). This provided a ranking from the gene with the most significant specific expression to the gene with the most significant depleted expression in the region. This genome-wide ranking allowed us to test if the genes that were specifically expressed in the habenula were enriched for a given gene set using the area under the receiver operator curve (AUROC) statistic. This non-parametric measure is frequently used for enrichment testing (Gillis et al., 2010). The AUROC for a set of genes is equivalent to the probability that a gene associated with that set will be found first in the genome-wide ranking compared to an unassociated gene. The ranking we used arranges genes from strongest specific to depleted expression. In this context, AUROC > 0.5 for a gene set means that these genes have specific expression in the habenula. For AUROC < 0.5 the reverse is true, with a bias toward depleted expression in the habenula. Given our focus on up-regulation, we only examined groups with AUROC > 0.5 (one-sided tests). AUROC values were generated using the tmod analysis package in R (Weiner and Domaszewska, 2016). The Mann–Whitney U test was used to determine statistical significance (one-sided). We used the Benjamini–Hochberg false discovery rate (FDR) procedure for multiple test correction (Benjamini and Hochberg, 1995) to adjust for the many tested gene sets. We performed no or limited multiple test correction for gene sets associated with depression and addiction. This a priori focus is marked by the inclusion of this article in the Frontiers Special Research Topic titled “Lateral Habenula: Role in Depression and Addiction”.
We also employ a specificity test to determine if the enriched gene sets are not representing broad differences that are significant across many other brain regions. For example, habenula specific gene sets may simply represent expression differences between the cortex and the rest of the brain. To assess specificity we ran the region-specific expression and gene set enrichment procedures for 516 fetal and 217 named brain regions in the expression datasets. For each gene set, we counted the number of regions with an AUROC value higher than the habenula result. Gene sets that have the highest specificity in the habenula subregions were considered to uniquely characterize the region.
Gene Ontology Sets
The Gene Ontology (GO) classifies genes into sets associated with specific biological processes, molecular functions, and cellular locations to formally model biological systems (Ashburner et al., 2000). The GO database was accessed through the GO.db and org.Hs.eg.db packages in R (Carlson, 2017a,b). Annotations were dated March 29, 2017. We calculated the AUROC values for GO groups containing between 10 and 200 genes, after filtering for genes present in the Allen microarray data (6,623 GO groups annotating 14,530 unique genes).
Disease-Associated Gene Sets
Disease-associated gene sets were obtained from the DisGeNET database which integrates several sources (Piñero et al., 2017). The curated gene-disease association file was downloaded in May 2018. Similar to the GO sets, we filtered for disease-associated gene sets with 10 to 200 genes after the intersection with our results (1,849 disease-associated gene sets covering 5,899 unique genes).
To complement the heterogeneous mix of sources in the DisGeNET we obtained genes from five large genetic studies focused on addiction or depression. For cannabis associated genes we used the seven genes harboring the genome-wide significant loci from the analysis of lifetime cannabis use [Table 1 in (Pasman et al., 2018)]. We additionally used the 35 genes that were significant in the gene-based analysis from Table 2 (genes found in the S-PrediXcan analysis only were excluded because they were linked to a specific tissue). The nine genes near the 14 genome-wide loci associated with alcohol consumption were obtained from Table 1 of Clarke et al. (2017). Genes associated with smoking were obtained from Minicã et al. (2017). Specifically, genes associated with ever smoking (3 genes), quantity smoked (14) and smoking cessation (2) were obtained from Table 1. Four genes linked to substance use disorder and five associated with opioid use disorder were obtained from Table 1 in a review of genetic studies by Jensen (2016). For depression, we used the 71 genes that harbor the 44 significant loci identified in the largest genetic study of major depression to date [Table 2 in (Wray et al., 2018)].
We additionally tested genes from transcriptomic studies of well-being and depression. These studies examined gene expression profiles of blood. The 27 genes from a study of recurrent major depression by Mostafavi et al. (2014) were split into up- and down- regulated lists (Table 2). For well-being, genes associated with hedonic well-being were obtained from Supplementary File D01 of Fredrickson et al. (2013).
Availability
Scripts, supplementary tables, and data files for reproducing the analyses are available online at https://github.com/leonfrench/HumanHabenula and https://figshare.com/articles/Transcriptomic_characterization_of_the_human_habenula_highlights_drug_metabolism_and_the_neuroimmune_system/6756728.
Results
Lateral Habenula
We first tested for specific expression in the lateral habenula in the adult brain data. Of the 48,170 tested microarray probes, 514 were significantly up-regulated in the lateral habenula (pFDR < 0.05, Supplementary Table S1). These probes mapped to 405 genes, with the top 20 presented in Table 1. Both of the probes mapping to the most significant gene, GPR151, separate habenula from all other assayed structures (Figure 1A). In the fetal brain, 184 probes were significantly upregulated, representing 127 genes. Four genes were in both the fetal and adult top 20 most significantly up-regulated lists (GPR151, POU4F1, ONECUT1, and CHRNB3). Strong overlap was observed more broadly with 42 genes significantly up-regulated in both the fetal and adult expression profiles of the lateral habenula (hypergeometric test, p < 10-39).
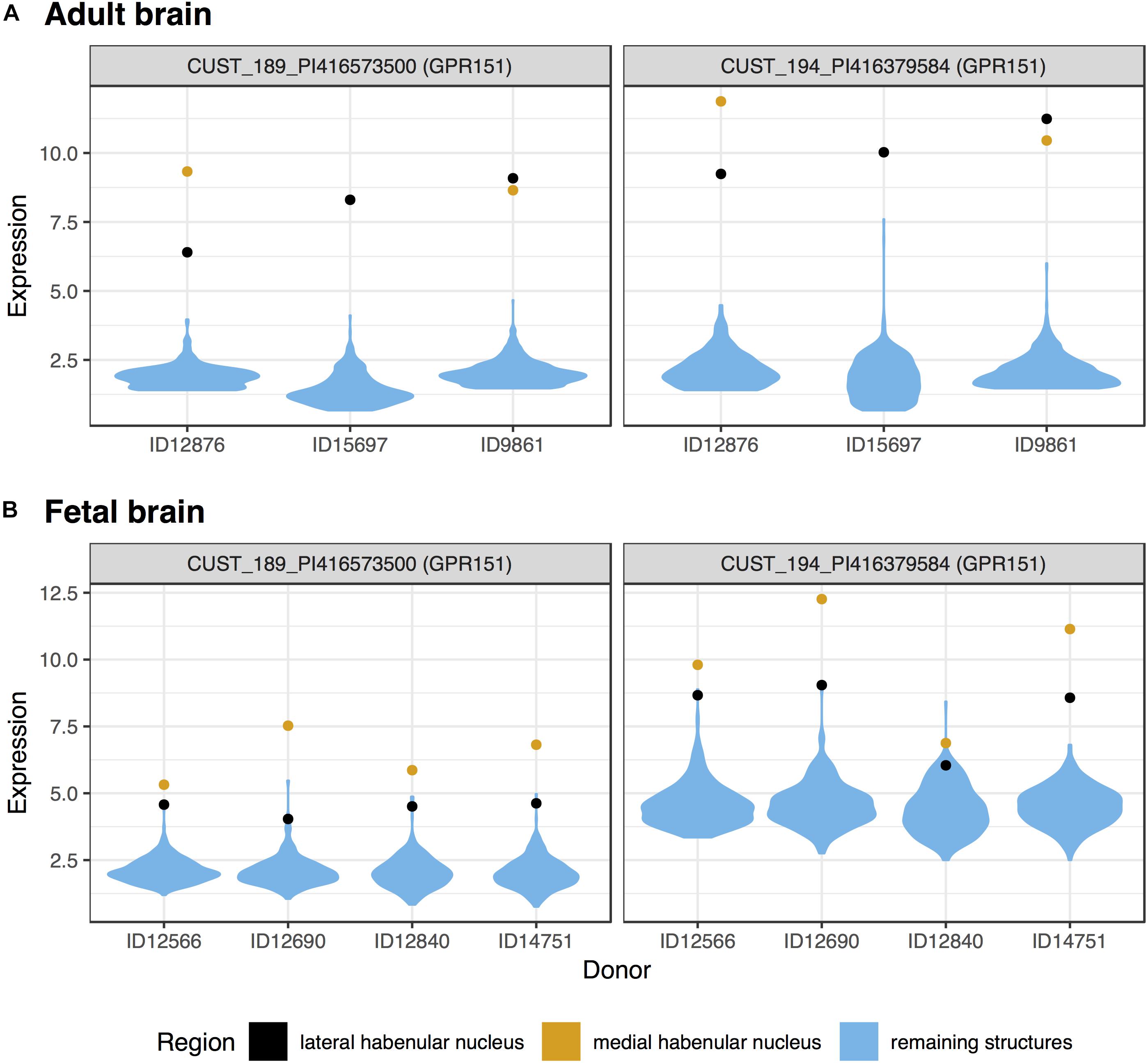
FIGURE 1. Violin plots of GPR151 expression in the adult (A) and fetal (B) brains. Expression (log2 intensity) is plotted on the y-axis for each of the two probes for GPR151. Donor identification numbers are marked in the x-axis. Expression in the lateral habenula is marked in black with orange marking the medial division. The distribution of expression across the remaining sampled regions is shown in blue.
Medial Habenula
In the medial habenula, the 624 significant up-regulated probes mapped to 427 genes (pFDR < 0.05, Supplementary Table S2). GPR151 was the most specifically expressed gene in both the fetal and adult data for the medial division (Figure 1 and Table 2). In the fetal brain, 924 probes that map to 624 genes were upregulated in the medial habenula. In comparison to the lateral habenula, there was a stronger overlap between the adult and fetal results. Specifically, six genes overlapped within the top 20 lists (GPR151, CYP3A4, CYP3A5, POU4F1, CHRNB3, and CAPN8) and 160 were in both sets of significantly upregulated genes (hypergeometric test, p < 10-132).
Cytochrome P450 Isoenzymes
Surprisingly, three of the four members of the cytochrome P450 family 3 subfamily A, were in the top 20 most significantly up-regulated genes for the medial habenula (CYP3A4, CYP3A5, and CYP3A7; Table 2). CYP3A4 and CYP3A5 were also in the fetal top 20 (mapped probes are plotted in Figure 2). This finding is unique as none of the other 217 brain regions have 3 or more of the 61 cytochrome P450 genes in their top 20 lists (CYP3A5 is in the lateral habenula top 20, adult data).
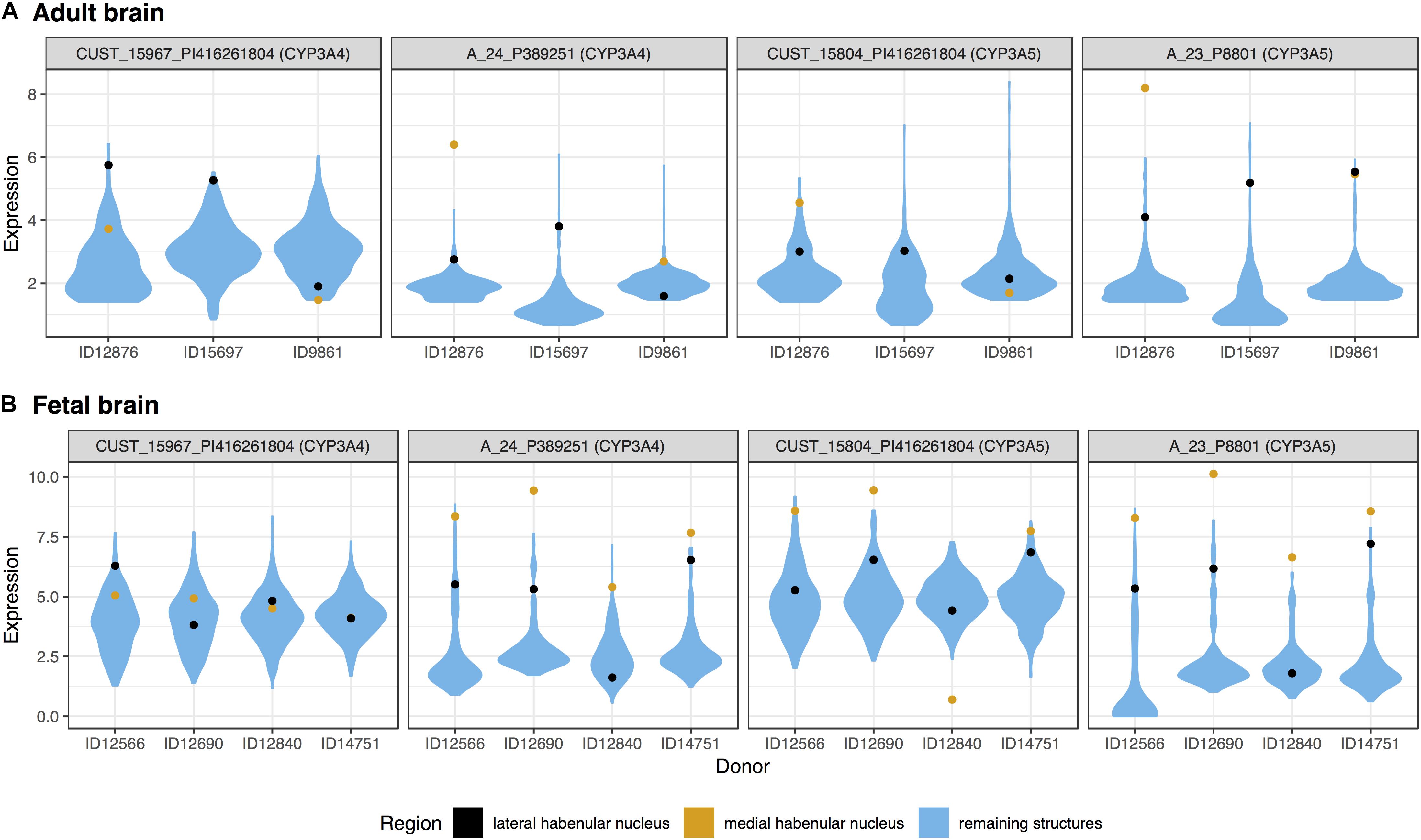
FIGURE 2. Violin plots of CYP3A4 and CYP3A5 expression in the adult (A) and fetal (B) brains. Expression (log2 intensity) is plotted individually for each probe on the y-axis (gene symbols in parenthesis). Donor identification numbers are marked in the x-axis. Expression in the lateral habenula is marked in black with orange marking the medial division. The distribution of expression across the remaining brain regions is shown in blue.
We extended this finding genome-wide by using the AUROC method to test the complete ranking of genes. Specifically, AUROC for the 61 cytochrome P450 genes was greater than 0.59 in the lateral and medial habenula (both p < 0.01). The four CYP3A subfamily genes had a stronger enrichment signal with AUROC above 0.85 in both divisions (p < 0.005). In the context of the other brain regions, only 5 other regions have higher AUROC values for the CYP3A genes. We named this count of brain-wide relative enrichment the “specificity rank” (lower is more specific). While the broad cytochrome P450 signal was not strong in the fetal data (both AUROC = 0.55, p > 0.08), the 3A subfamily genes were enriched in both divisions of the developing habenula (AUROC > 0.89, p < 0.005, specificity rank < 27). Across both divisions and datasets, CYP3A4, CYP3A5, and CYP3A7 were consistently ranked within the top 227 most enriched genes while CYP3A43 shows little enrichment (ranked within the top 6,444–11,744 genes). In summary, the CYP3A genes were specifically expressed in the fetal and adult habenula.
Estimated Cell Type Proportions
We next used the markerGeneProfile tool to estimate cell-type proportions (Mancarci et al., 2017). These estimates were built from top marker genes obtained from a study of the adult human temporal cortex. Due to the difference in brain region, we only used the estimates to roughly characterize the cell-type composition of the habenula in the context of the other regions in the adult expression atlas. For both divisions, microglia had the highest estimated proportion with 15 and 18 regions having a higher estimate for the lateral and medial habenula, respectively. Astrocyte (specificity rank = 33) and endothelial cells (specificity rank = 35) also had above average estimated proportions the lateral habenula. In the medial habenula, endothelial cells were enriched (specificity rank = 58) with the remaining four cell types with above average estimated proportions (Supplementary Table S3).
Addiction Focused Gene Set Enrichment Analyses
First, we tested GO terms related to drugs of abuse for enrichment in the adult habenula. Genes that were annotated to amphetamine, morphine, or cocaine response were not significantly enriched in the lateral or medial adult habenula (27–51 genes per group). The 47 genes annotated with the response to nicotine term were significantly enriched in the lateral (AUROC = 0.653, puncorrected < 0.00015) and medial habenula (AUROC = 0.614, puncorrected < 0.005). Brain-wide, this enrichment was specific with lateral habenula having the highest AUROC value of all 217 regions tested (specificity rank = 0). The medial finding was also relatively unique with a specificity rank of 10 (which includes the lateral habenula). Genes annotated to the response to alcohol term were significantly enriched in the medial (187 genes, AUROC = 0.54, puncorrected < 0.02, specificity rank = 30), but not the lateral habenula. In total, 12 GO terms have alcohol in their title. Of those terms, genes annotated to positive regulation of alcohol biosynthetic process were significantly enriched in both the medial and lateral habenula after correcting for the 12 tests (pFDR < 0.005, AUROC > 0.67, specificity rank < 6). An additional two terms were enriched in the lateral but not medial habenula (‘regulation of alcohol biosynthetic process’ and ‘primary alcohol metabolic process’).
We next tested disease annotations related to addiction using the adult data. Of the eight gene sets with alcohol in their title, none were significantly enriched in the medial habenula and three had significant enrichment without correction for multiple testing in the lateral division (Non-alcoholic Fatty Liver Disease, Fetal Alcohol Spectrum Disorders and Fetal Alcohol Syndrome; puncorrected < 0.05 and pFDR < 0.08). No significant enrichment was found for marijuana, cocaine or amphetamine abuse or dependence related gene sets.
To complement the disease associations from the DisGeNet database we tested genes from large genome-wide association studies of addiction. For addiction associated gene sets, none were significantly enriched after multiple test correction for the eight sets in the medial or lateral adult habenula data. For the lateral habenula, two genes associated with smoking cessation (SLC25A21 [ranked 848], SEMA6D [ranked 1128], puncorrected < 0.02, AUROC = 0.95, specificity rank = 4), 14 associated with quantity of cigarettes smoked (puncorrected < 0.03, AUROC = 0.65, specificity rank = 13) and four genes associated with broad illicit drug use (puncorrected < 0.05, AUROC = 0.75, specificity rank = 3) were nominally enriched. The habenula specific genes associated with quantity smoked were cholinergic receptors (CHRNA3 and CHRNB4) and major histocompatibility complex proteins (HLA-DPA1 and HLA-DPB1). For the fetal lateral habenula, only the seven cannabis associated genes were enriched (puncorrected < 0.01, AUROC = 0.77, specificity rank = 12). For the medial division in the adult dataset, the four genes associated with broad illicit drug use were the most enriched and specific (puncorrected < 0.02, AUROC = 0.84, specificity rank = 0). The two smoking cessation associated genes, SLC25A21 (ranked: 1021) and SEMA6D (ranked: 4285), were also enriched in the medial habenula data (puncorrected < 0.04, AUROC = 0.87, specificity rank = 20). These two sets were also enriched in the fetal data (puncorrected < 0.05 for both). The top-ranked set in the fetal medial data were the genes associated with quantity of cigarettes smoked (14 genes, puncorrected < 0.005, pFDR < 0.04 [corrected for 8 sets], AUROC = 0.7, specificity rank = 6). Thus, some enrichment was observed for the genes harboring genetic variants associated with broad illicit drug use, cannabis, and smoking but not for alcohol consumption or opioid use.
Depression Focused Gene Set Enrichment Analyses
Of the four disease gene sets from DisGeNet that are related to depression, only “Depressive Symptoms” was significantly enriched for lateral habenula specific expression (29 genes, AUROC = 0.6, puncorrected < 0.05, specificity rank = 0). Genes harboring genetic loci that were associated with major depressive disorder in the largest genetic study to date were not enriched in the medial or lateral habenula (Wray et al., 2018). This enrichment for depressive symptoms and the central role of the habenula in the hedonic neurocircuit motivated an analysis of genes that were differentially expressed in a study of well-being (Graziane et al., 2018). Genes that were up-regulated in the blood of people with low levels of hedonic well-being were enriched in both divisions of the adult habenula (33 genes, p < 0.0005, AUROC > 0.67, specificity rank < 7, Figure 3). These genes that were negatively correlated with hedonic well-being were also enriched in the fetal medial habenula (p < 0.005, specificity rank = 1). In contrast, no significant enrichment was observed for genes up-regulated with hedonic well-being or genes differentially expressed in blood of individuals with recurrent major depression. Overall, we found enrichment for genes associated with depressive symptoms, including hedonic well-being but not genes linked to major depressive disorder.
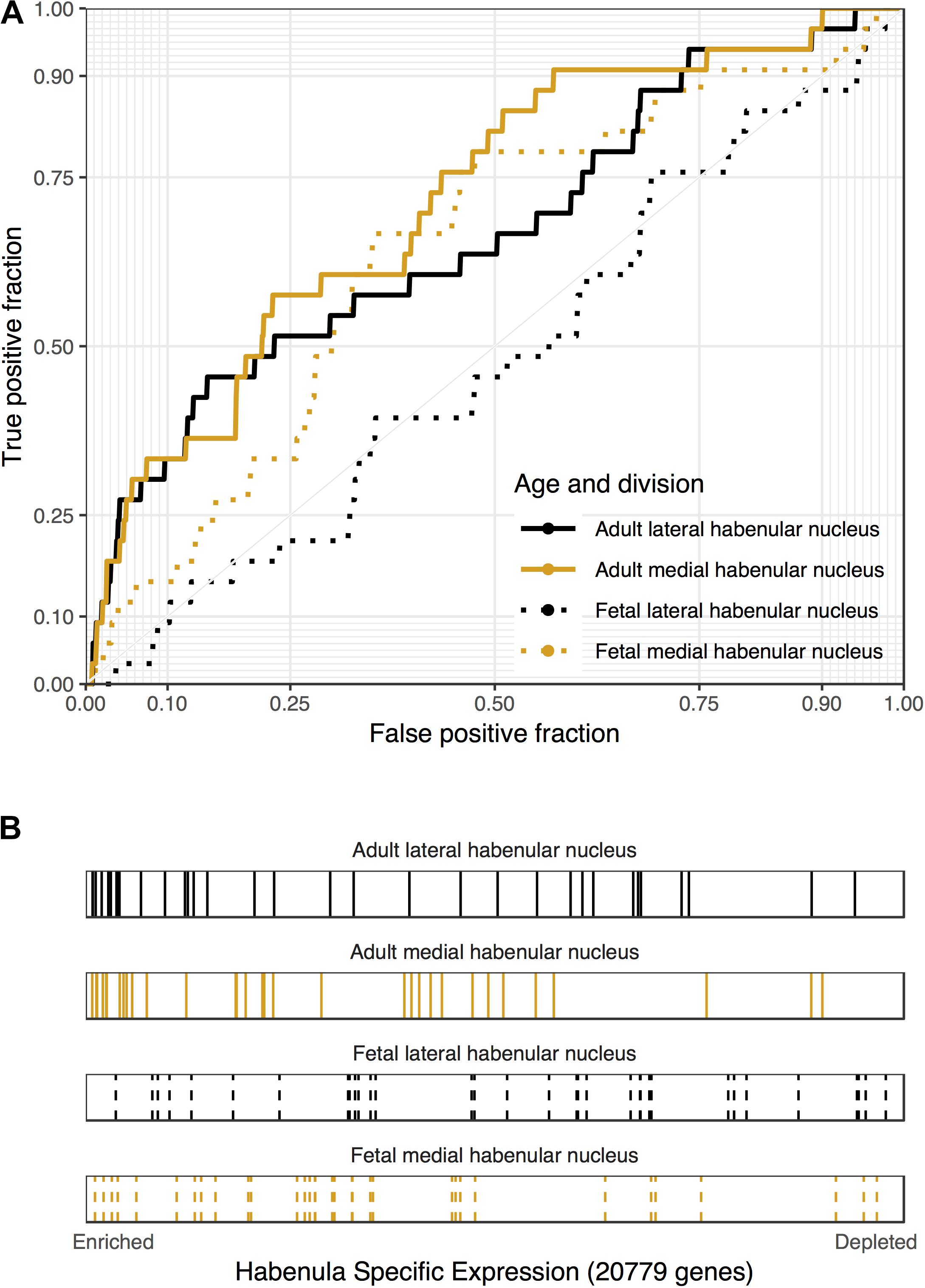
FIGURE 3. Associations between genes that are down-regulated in the blood of people with high levels of hedonic well-being and habenular specific expression. (A) ROC curves showing the proportion of hedonia down-regulated genes that overlap (y-axis, true positive fraction) in varying lengths of the habenula specific gene rankings (approximated by the x-axis, false positive fraction). (B) Distributions of the hedonia down-regulated genes across the habenular specific gene rankings with each gene representing a single colored line. Color marks the lateral (black) and medial (orange) habenula rankings. Dashed lines are used for the fetal datasets.
Gene Ontology Enrichment
We next used all the Gene Ontology annotations to obtain a broader characterization of the molecular functions, cellular components, and biological processes with high expression in the habenula. We applied the same procedures used for the above analyses with the addition of multiple test correction for the 6,623 GO groups used. For the lateral habenula, 981 groups were significant after correction and the top enriched GO groups cover a broad range of annotations (full list in Supplementary Table S4). The top result was the 196 genes annotated to the humoral immune response (pFDR < 10-11, AUROC > 0.66, specificity rank = 3). Ten immune-related GO groups also appear within the top 15 with other groups representing peptidase regulation, glycosaminoglycan binding, odorant binding, and G-protein coupled peptide receptor activity (all pFDR < 10-7, specificity rank < 13). For the medial habenula, 333 groups were significant after multiple test correction (full list in Supplementary Table S5). The most significant group was cilium movement (60 genes, pFDR < 10-10, AUROC > 0.79, specificity rank = 4), which was joined by five other cilium or axoneme related gene sets in the top 15. This enrichment for cilia associated genes was expected because the medial habenula borders the 3rd ventricle. Within that top list, the remaining groups were immune (regulation of mononuclear cell proliferation and response to interferon-gamma) or nucleosome related (all pFDR < 10-5, specificity rank < 37). Comparing the top 15 GO groups for the lateral and medial divisions revealed four shared immune-related groups: response to interferon-gamma, MHC protein complex, regulation of mononuclear cell proliferation and regulation of lymphocyte proliferation. Overall, stronger enrichment was found in the lateral habenula but this may be due to differing sample sizes (3 lateral versus 2 medial samples).
To filter the large number of significant GO groups, we selected groups that have a higher AUROC value than any other assayed region and were also enriched in the fetal samples (specificity rank = 0 and fetal puncorrected < 0.05). In the lateral habenula, applying the specificity leaves 114 of the 981 significant groups. As shown in Table 3, 38 groups were also enriched in the fetal brain samples (binomial test p < 10-16). While this list was primarily immune-related, acetylcholine binding and response to nicotine also appear. For the medial habenula, only four groups survive this filter (Table 4). To provide an extended list, we relaxed the specificity criteria to allow the gene group to have a higher AUROC value in one other brain region (specificity rank ≤ 1). Of the 31 groups with this specificity level, 22 were also significantly enriched in the fetal medial habenula at an puncorrected of 0.05 or lower (binomial test p < 10-16). These groups were primarily immune-related but also include two groups related to apoptosis via cysteine-type endopeptidase activity (Table 4).
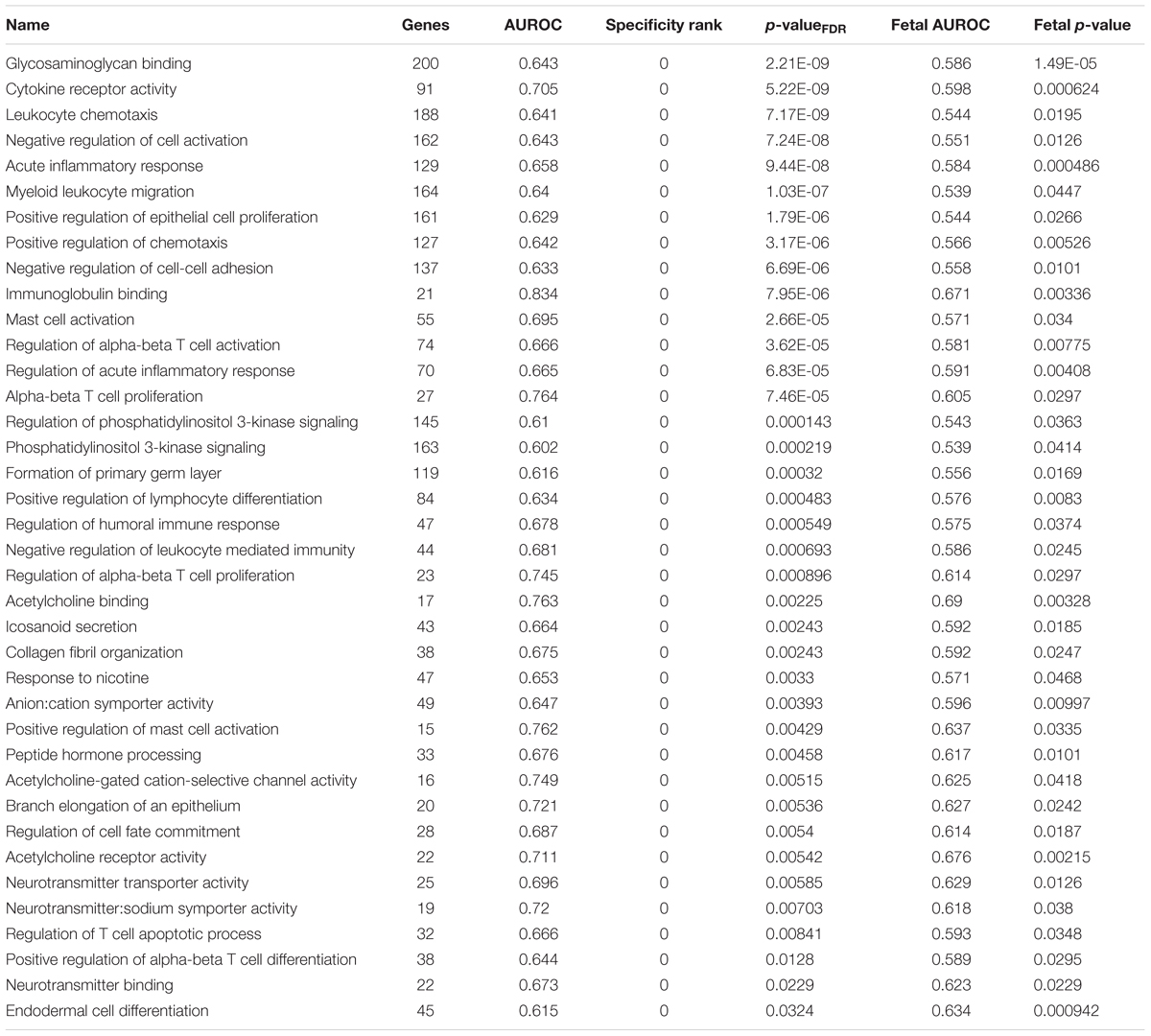
TABLE 3. Gene Ontology (GO) groups enriched for uniquely specific expression in the adult lateral habenula which validate in the fetal brain.
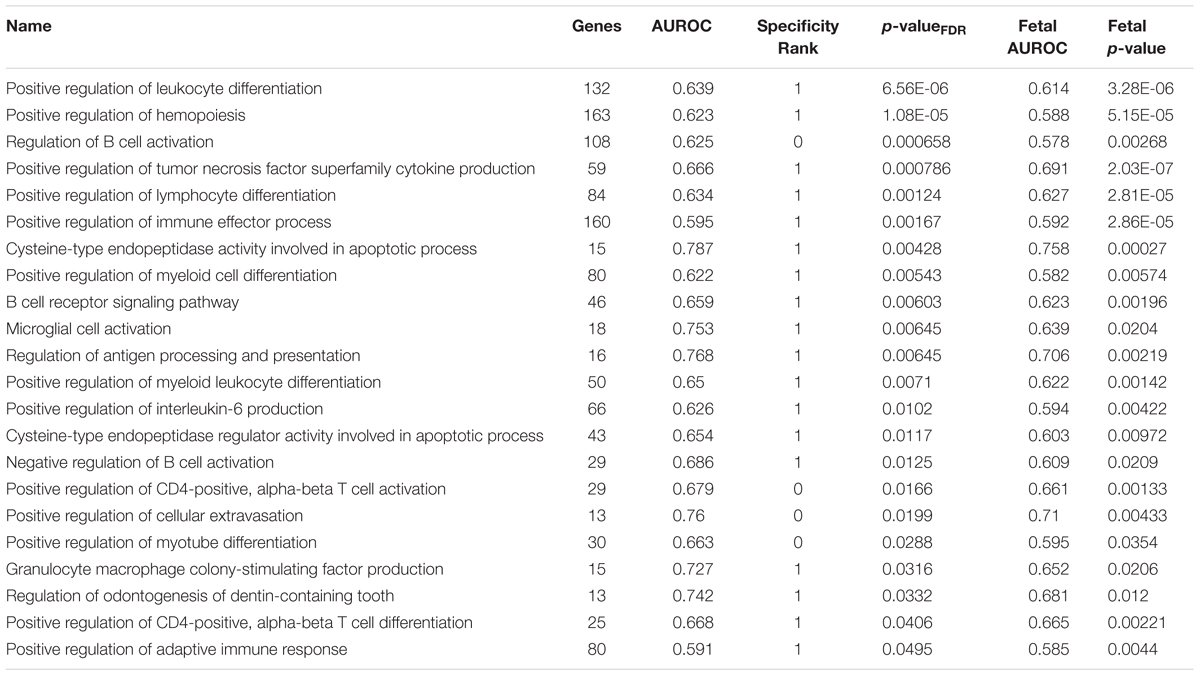
TABLE 4. Gene Ontology groups enriched for uniquely specific expression in the adult medial habenula which validate in the fetal brain.
Disease-Associated Gene Enrichment
Beyond the addiction and depression sets of interest, several disease-associated gene sets ranked higher. Of the 1,849 tested sets, the top 10 most enriched in the lateral habenula are presented in Table 5 and include disorders that often result from addictions (Table 5, full list in Supplementary Table S6). In agreement with the gene ontology results, the top diseases for the medial habenula were primarily related to cilium dysfunction (Supplementary Table S7). Four diseases occurred in the top ten for both divisions (influenza, pneumonia, calcinosis and IgA glomerulonephritis).
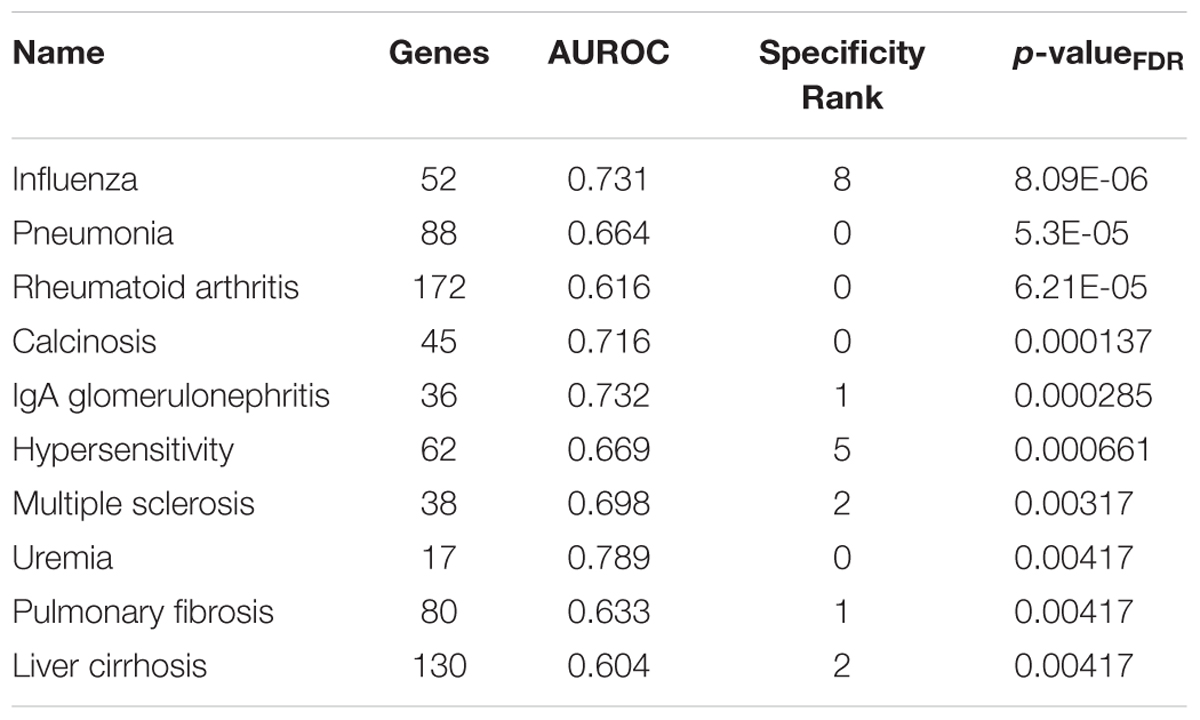
TABLE 5. Top 10 disease-associated gene sets enriched for specific expression in the adult lateral habenula.
Discussion
This study characterized the human lateral and medial habenula at the molecular scale. We tested for specific enrichment of genes associated with addiction and depression before performing a broader analysis. In agreement with a single-cell expression study of the larval and adult zebrafish habenula, our findings significantly overlapped between the adult and fetal results (Pandey et al., 2018). While focused on the adult habenula, we used the fetal data to validate key findings. Data from other brain regions was also used to determine specificity. In summary, we found the habenula specifically expressed genes associated with nicotine and smoking; cannabis; drug metabolism and clearance; acute immune response, mast cells, and inflammatory diseases; cilium; hedonic well-being; and hematopoiesis. We did not find strong or specific enrichment of genes associated with other drugs of abuse; learning, memory, and attention; nor major depressive disorder.
Top-Ranked Genes
At the level of individual genes, we found the expected enrichment of cholinergic receptors with the nicotinic β3 and β4 subunits having the highest specificity in the lateral and medial division, respectively. Supporting translational potential, the β4 subunit was shown to limit nicotinic acetylcholine receptor activity and regulate nicotine aversion in studies of the mouse medial habenula (Frahm et al., 2011; Slimak et al., 2014). Combined, the 17 genes in the acetylcholine binding GO group that encode 14 nicotinic subunits, the muscarinic 3 receptor, acetylcholinesterase, and solute carrier family 18 member A3 (acetylcholine transporter) are specifically and uniquely enriched in the lateral habenula. However, the medial habenula had a higher number of significantly enriched cholinergic receptor genes after multiple test correction (nicotinic subunits α3, α4, α6, and β4). In the mouse medial habenula, receptors containing the α6 subunit were involved in nicotine withdrawal-induced anxiety (Pang et al., 2016). While habenular α5 subunit signaling has been shown to limit nicotine intake in rats, CHRNA5 is not specifically expressed in the human habenula (Fowler et al., 2011). A recent mouse study suggests the α5 subunit may be important for nicotine preference and aversion in another region, the interpeduncular nucleus, which receives inputs from the medial habenula (Ables et al., 2017; Morton et al., 2018).
Confirming animal expression studies (Broms et al., 2015; Wagner et al., 2016a), the most specifically expressed gene in the habenula is a G protein-coupled receptor 151 (GPR151) which separated habenula expression from any other assayed region in the adult data. Similarly, specific expression of GPR139 was observed, in agreement with rat and human expression patterns (Liu et al., 2015) (75th and 168th most specific gene the lateral and medial habenula, respectively). A recent study demonstrated that activation of GPR139 reduces alcohol intake in alcohol-dependent rats (Kononoff et al., 2018). Transient Receptor Potential Cation Channel Subfamily M Member 8 (TRPM8), was highly expressed in both the lateral and medial habenula (ranked 3rd and 8th in the medial and lateral divisions, respectively). This gene encodes a cation channel which is activated by menthol, providing a candidate molecule for studies examining the neurobiological effects of menthol on cigarette smokers (Gandhi et al., 2009; Brody et al., 2013; Henderson et al., 2016). Overall, the top-ranked genes confirm rodent studies and mark targets for future studies of addiction.
Cytochrome P450 Enzymes and Drug Metabolism
Genes encoding cytochrome P450 enzymes were highly expressed in the medial habenula which has also been observed in rodent studies (Norris et al., 1996; Birnie et al., 2013). The metabolic functions of P450 enzymes are required to clear foreign and toxic compounds from the body. P450 substrates include therapeutic drugs, toxins, carcinogens, and some endogenous molecules that include testosterone and estrogen. For example, Cytochrome P450 Family 3 Subfamily A Member 4 (CYP3A4) is estimated to be involved in the metabolism of over half of all therapeutic drugs (Ioannides, 1996; Robertson et al., 2003). This is of note as CYP3A4 was the 13th most specifically expressed gene for the adult medial habenula. For CYP3A7, we found more specific habenular expression in the adult when compared to the fetal data, which contrasts studies of the liver that found high fetal expression (Hakkola et al., 2001). In addition, UDP Glucuronosyltransferase Family 2 Member B15 (UGT2B15), the 3rd most specifically expressed gene in the lateral habenula, is also involved in the elimination of toxic compounds (xenobiotic and endogenous) (Stingl et al., 2014). Furthermore, ADH7, an alcohol dehydrogenase gene was enriched in the medial habenula (27th most specific gene). The combination of these enriched genes and the habenular border with the third ventricle suggest the habenula is uniquely sensitive to endogenous and xenobiotic compounds. This agrees with studies finding that the habenula is important for learning the adverse effects of drugs such as ethanol and nicotine (Fowler and Kenny, 2014; Haack et al., 2014). Beyond drugs of abuse, this association with drug metabolism may underlie links between therapeutic drugs and depression that are of increasing importance (Patten and Barbui, 2004; Qato et al., 2018).
Mast Cells and Neuroimmune Associations
In the lateral habenula, genes annotated to mast cell activation were uniquely and specifically enriched as a set. Mast cells are first responders of the immune system, reside in the brain, and can cross the blood–brain barrier (Dong et al., 2014). Mast cells contain granules that can quickly release their stored contents in response to external stimuli. The contents of these granules are packed together with the help of glycosaminoglycans (Mulloy et al., 2017). Genes that bind glycosaminoglycan were the most significant and specifically enriched gene set in the lateral habenula. Mast cells respond to steroids and regulate the blood-brain barrier in the medial habenula of doves (Zhuang et al., 1996; Wilhelm, 2011). Mast cells react to xenobiotics, have been proposed as a paradigm for testing toxic effects of neurotropic drugs, and have been suggested to have detoxifying roles in the brain (Ibrahim, 1974; Purcell et al., 1996). More directly, habenular mast cells numbers are increased when rats are injected with cyclophosphamide, a toxic immunosuppressant and chemotherapeutic drug (Servière et al., 2003). This mast cell response was coupled with suppression of motor behavior. This key study connects our findings of enrichment of drug metabolism and mast cell associated genes with the habenula in an experimental setting.
Consistent with habenula being a source in negative reward signals, mast cells respond to aversive stimuli such as toxins, venoms, and physical stimuli (Galli et al., 2016; Kempuraj et al., 2017). Central nervous system mast cells are also associated with chronic subordination stress (Cirulli et al., 1998) and anxiety (Nautiyal et al., 2008). Patients with mastocytosis, a condition in which mast cells accumulate, are more likely to be depressed (Moura et al., 2011). A study of mastocytosis patients has also associated inflammatory markers with high depression scores (Georgin-Lavialle et al., 2016). In the context of addiction, naloxone-induced morphine withdrawal increases mast cell numbers in the thalamus (Taiwo et al., 2004) and intravenous heroin increases markers of mast cell degranulation (Rook et al., 2006). Overall, our findings suggest that the habenula is a key location for neuroimmune interactions (Forsythe and Bienenstock, 2012).
Beyond mast cells, we also observed enrichment of primarily immune-associated gene sets in Tables 3, 4. This can be partly explained by the relatively high estimated proportion of microglia cells. While 15 regions had higher estimated proportions of microglia these immune-associated gene sets were most strongly enriched in the habenula (specificity rank = 0), suggesting that these signals were not merely due to higher proportions of microglia. In agreement, a past study of the Interleukin 18 cytokine observed expression in medial habenula neurons (Sugama et al., 2002). One of most strongly enriched gene groups was ‘response to interferon-gamma,’ which is of interest given the recent associations between interferon-γ and social behavior (Filiano et al., 2016). Interferon-γ is also expressed in habenular neurons after influenza infection (Mori et al., 2001). Two other gene sets of note are ‘myeloid leukocyte migration’ and ‘positive regulation of cellular extravasation’ which supports findings of brain leukocyte infiltration from the cerebrospinal fluid into the habenula and other regions in rodents (Schmitt et al., 2012). More specific studies that have focused on pro-inflammatory molecules have associated the habenula with depressive symptoms and chronic restraint stress (Sugama et al., 2002; Zhao et al., 2018). Recent advances in single-cell sequencing may help determine which cell-types are contributing to this unique neuroimmune signature in the habenula.
Depression
Genes associated with major depressive disorder that were identified in the largest genome-wide association study to date were not specifically expressed in the habenula (Wray et al., 2018). In addition, genes differentially expressed in the blood of individuals with recurrent major depression in comparison to controls (Mostafavi et al., 2014), were not specifically expressed in the habenula. Genes associated with depressive symptoms in the DisGeNet database were specifically and uniquely enriched in the lateral habenula. Also, genes negatively correlated with hedonic well-being were enriched in the medial and lateral habenula. This finding links the habenula to anhedonia, a key symptom of depression. Genes positively correlated with hedonic well-being were not enriched, suggesting the leukocyte source of these correlated genes was not driving the signal. In support, several studies have associated the habenula with hedonic pathways. In mice, the medial habenula was shown to regulate of hedonic state (Hsu et al., 2014). In patients with cancer-associated weight loss reduced functional connectivity was observed between the habenula and nucleus accumbens during a reward task (Maldonado et al., 2018). Differences in habenula volume has been associated with anhedonia symptoms in depressed and healthy individuals (Lawson et al., 2017). Habenula activation during a punishment task in unmedicated major depressive disorder patients was more strongly associated with anhedonia than overall depressive symptoms (Liu et al., 2017). Overall, we observed molecular associations with depressive symptoms and hedonic well-being but not genes directly linked to major depressive disorder.
Our broad analysis of disease-associated gene sets provided indirect links to depression. In the lateral habenula, we observed enrichment of several inflammatory diseases that align with the gene ontology analysis. In the context of depression, we note that that neuroinflammation has been associated with depression in mouse and human studies (Miller and Raison, 2016; Setiawan et al., 2018; Zhao et al., 2018). Genes associated with rheumatoid arthritis were the third most enriched set with no other region having a stronger association. A meta-analysis found that patients with rheumatoid arthritis have a higher prevalence of depression (Dickens et al., 2002). Similarly, genes associated with multiple sclerosis were enriched in the lateral habenula and depression is more common in multiple sclerosis patients (Patten et al., 2003). In addition, mast cells were found to be essential for disease progression in a mouse model of multiple sclerosis (Secor et al., 2000). IgA glomerulonephritis, an inflammatory kidney disease, was the 5th most enriched disease gene set. Genes associated with uremia were also specifically enriched (8th most enriched), which results from loss of kidney function. In patients with chronic renal disease, depression is the most common psychiatric condition (Fabrazzo and De Santo, 2006). Again, these results may be explained by a higher proportion of microglia in the habenula, but again we note that several other regions have a higher estimated number of microglia and these disease genes sets are very specific to the habenula. We also note that genes associated with influenza were top-ranked. Past studies have found that habenula neurons are selectively targeted by the influenza virus, resulting in behavioral changes after infection and clearance (Mori et al., 1999, 2001; Beraki et al., 2005). This suggests that neurons may underlie some of these inflammatory disease associations in the habenula. While these associations are interesting, more direct studies are needed to test the pathophysiological role of the habenula in these diseases.
Addiction
Related to addiction and drugs of abuse we found limited specific expression in the habenula. We observed some enrichment of genes related to nicotine response and genetic studies of smoking behavior. Cannabis receptor 1 (CNR1) is significantly enriched in the adult lateral habenula and genes harboring genetic variants associated with cannabis use were enriched in the lateral division of the fetal brain but not the medial habenula in the adult or fetal data. In a rat model of nicotine addiction, habenular CB1 receptor activity in the habenula was linked to depressive-like behavior and recovery from nicotine dependence (Simonnet et al., 2017). In the context of nicotine, we note that the expression of molecules in regions that connect to the habenula play key roles. Specifically, rodent studies of glucagon-like peptide-1 in the nucleus tractus solitarius (Tuesta et al., 2017), dopamine D3 receptors in the basolateral amygdala (Khaled et al., 2014), and corticotropin releasing factor receptor-1 in the interpeduncular nucleus (Zhao-Shea et al., 2015) have linked nicotine intake, seeking, and withdrawal, respectively.
We found some habenular specific expression for genes involved in the regulation of alcohol biosynthesis but no enrichment of genes associated with alcohol consumption nor alcohol abuse. Also, we did not observe specific habenular expression of genes associated with amphetamine, morphine, or cocaine responses and related disorders. Past findings of increased habenula activity after administration of these drugs may be mediated by the cytochrome P450 enzymes. For example, the effect of cocaine on neurodevelopment was found to be mediated by CYP3A5 (Lee et al., 2017). Also, the negative findings are possibly due to limited understanding of which molecules are involved in drug withdrawal and relapse, which the habenula is thought to play a key role (Velasquez et al., 2014; López et al., 2018).
In the context of addiction, we note that genes involved in the positive regulation of hemopoiesis are strongly enriched in the medial habenula. Hematopoiesis or hemopoiesis is the production of blood cells. A phenome-wide association study of clonal hematopoiesis has associated it with smoking, addiction and psychiatric disease (Zink et al., 2017). In addition, increases of several hematological factors which included cell type counts were observed in opium and heroin users (Haghpanah et al., 2010). A related result was enrichment of genes associated with thrombosis (12th most significantly enriched disease gene set in the lateral habenula). In the context of addiction, deep vein thrombosis is frequently caused by intravenous drug use (Cornford et al., 2011; Kwiatkowska et al., 2015). We also note that several of the lateral habenula disease-associated gene sets are associated with addictions: pneumonia [smoking and alcohol abuse (Grau et al., 2014)], liver cirrhosis (alcohol abuse), pulmonary fibrosis (smoking), arthritis (smoking), uremia and IgA glomerulonephritis [cocaine and alcohol abuse (Cecchin and De Marchi, 1996; Jaffe and Kimmel, 2006)]. Our analysis cannot determine the role, if any, played by the habenula or the enriched genes in the pathophysiology of these diseases or their associations with substance abuse. Nonetheless, these links between habenular gene expression, hemopoiesis, and the sequelae of addictions suggest complex relationships that warrant further investigation.
Feeding and Energy Balance
An analysis of habenula specific expression in the rat brain highlighted genes associated with feeding and energy balance (Wagner et al., 2016a). Habenula activity has also been inversely associated with food hedonics in rodents (Kenny, 2011). In our gene set analysis, we did not observe enrichment of genes associated with eating behavior or energy regulation. However, we note that melanocortin 4 receptor (MC4R), was the 26th most significantly enriched gene in the lateral habenula. In addition, the five genes in the FTO obesity cluster were significantly enriched in the medial habenula which is primarily driven by the iroquois homeobox 5 and 6 genes (AUROC = 0.82, specificity rank = 14). Beyond MC4R and the FTO cluster, which were the two most significant genetic loci associated with body mass index, a larger set of genes harboring genetic variants associated with body mass index were not enriched in the habenula (Locke et al., 2015). Therefore, molecular studies of the habenula in the context of obesity should target the melanocortin system and iroquois homeobox genes.
Conclusion
Numerous review articles have focused on the habenula but few mention the immune system or drug metabolism. Our results highlighted these underappreciated aspects of the region, suggesting the habenula is tuned to respond to negative signals from acute infection or xenobiotic compounds. This suggests that future studies of the human habenula should test effects of medication use. Our focused analysis highlighted genes associated with nicotine and cannabis but we did not observe associations with other specific drugs beyond a signal associated with broad substance abuse. It’s possible that habenular responses to these drugs may be mediated by the general drug metabolism genes that are enriched, or may be due to the limited understanding of the molecular mechanisms underlying withdrawal. Related to depression we observed an association with hedonic well-being but not broader depression. Across all diseases, we observed associations with inflammatory disorders which is mirrored by Gene Ontology analysis. While we estimate the habenula has an above average number of microglia, these specific neuroimmune associations are stronger and supported by animal studies. Overall, our results from the healthy brain suggest the habenula may be a key bridge to aid understanding of drug-induced depression and the role of inflammation in depression. More generally, our results suggest the habenula receives negative reward signals from immune and drug processing molecules. This is consistent with the habenular role in the “anti-reward” system.
Author Contributions
BLF assisted in the interpretation of the data, manuscript writing, and editing. LF conceived the study, completed all analysis, interpreted the data, and drafted the manuscript.
Funding
This study was supported by the CAMH Foundation, and a National Science and Engineering Research Council of Canada (NSERC) Discovery Grant to LF.
Conflict of Interest Statement
The authors declare that the research was conducted in the absence of any commercial or financial relationships that could be construed as a potential conflict of interest.
Acknowledgments
We thank the Allen Institute for Brain Science for creating the Allen Human Brain Atlas. We thank Rachel Tyndale, Franziska Wagner, Henry A. Lester, and Derek Howard for helpful comments and suggestions. Additionally, we thank Derek Howard for help with initial data preparation and Mehrab Ali for editing help.
Footnotes
- ^http://help.brain-map.org/display/humanbrain/Documentation
- ^http://help.brain-map.org/display/devhumanbrain/Documentation
References
Ables, J. L., Görlich, A., Antolin-Fontes, B., Wang, C., Lipford, S. M., Riad, M. H., et al. (2017). Retrograde inhibition by a specific subset of interpeduncular α5 nicotinic neurons regulates nicotine preference. Proc. Natl. Acad. Sci. U.S.A. 114, 13012–13017. doi: 10.1073/pnas.1717506114
Ashburner, M., Ball, C. A., Blake, J. A., Botstein, D., Butler, H., Cherry, J. M., et al. (2000). Gene ontology: tool for the unification of biology. the gene ontology consortium. Nat. Genet. 25, 25–29. doi: 10.1038/75556
Benjamini, Y., and Hochberg, Y. (1995). Controlling the false discovery rate: a practical and powerful approach to multiple testing. J. R. Stat. Soc. Ser. B Stat. Methodol. 57, 289–300.
Beraki, S., Aronsson, F., Karlsson, H., Ogren, S. O, and Kristensson, K. (2005). Influenza A virus infection causes alterations in expression of synaptic regulatory genes combined with changes in cognitive and emotional behaviors in mice. Mol. Psychiatry 10, 299–308. doi: 10.1038/sj.mp.4001545
Birnie, M., Morrison, R., Camara, R., and Strauss, K. I. (2013). Temporal changes of cytochrome p450 (cyp) and eicosanoid-related gene expression in the rat brain after traumatic brain injury. BMC Genomics 14:303. doi: 10.1186/1471-2164-14-303
Boulos, L. J., Darcq, E., and Kieffer, B. L. (2017). Translating the habenula-from rodents to humans. Biol. Psychiatry 81, 296–305. doi: 10.1016/j.biopsych.2016.06.003
Brody, A. L., Mukhin, A. G., La Charite, J., Ta, K., Farahi, J., Sugar, C. A., et al. (2013). Up-regulation of nicotinic acetylcholine receptors in menthol cigarette smokers. Int. J. Neuropsychopharmacol. 16, 957–966. doi: 10.1017/S1461145712001022
Broms, J., Antolin-Fontes, B., Tingström, A., and Ibañez-Tallon, I. (2015). Conserved expression of the GPR151 receptor in habenular axonal projections of vertebrates. J. Comp. Neurol. 523, 359–380. doi: 10.1002/cne.23664
Carlson, M. (2017a). A Set of Annotation Maps Describing the Entire Gene Ontology. Available at: ftp://ctan.uib.no/pub/bioconductor/2.7/data/annotation/html/GO.db.html
Carlson, M. (2017b). org.Hs.eg.db: Genome Wide Annotation for Human. Available at: http://bioconductor.org/packages/org.Hs.eg.db/
Cecchin, E., and De Marchi, S. (1996). Alcohol misuse and renal damage. Addict. Biol. 1, 7–17. doi: 10.1080/1355621961000124656
Cirulli, F., Pistillo, L., de Acetis, L., Alleva, E., and Aloe, L. (1998). Increased number of mast cells in the central nervous system of adult male mice following chronic subordination stress. Brain Behav. Immun. 12, 123–133. doi: 10.1006/brbi.1998.0505
Clarke, T.-K., Adams, M. J., Davies, G., Howard, D. M., Hall, L. S., Padmanabhan, S., et al. (2017). Genome-wide association study of alcohol consumption and genetic overlap with other health-related traits in UK biobank (N=112117). Mol. Psychiatry 22, 1376–1384. doi: 10.1038/mp.2017.153
Colantuoni, C., Lipska, B. K., Ye, T., Hyde, T. M., Tao, R., Leek, J. T., et al. (2011). Temporal dynamics and genetic control of transcription in the human prefrontal cortex. Nature 478, 519–523. doi: 10.1038/nature10524
Cornford, C S., Mason, J. M., and Inns, F. (2011). Deep vein thromboses in users of opioid drugs: incidence, prevalence, and risk factors. Br. J. Gen. Pract. 61, e781-86. doi: 10.3399/bjgp11X613115
Darmanis, S., Sloan, S. A., Zhang, Y., Enge, M., Caneda, C., Shuer, L. M., et al. (2015). A survey of human brain transcriptome diversity at the single cell level. Proc. Natl. Acad. Sci. U.S.A. 112, 7285–7290. doi: 10.1073/pnas.1507125112
Dickens, C., McGowan, L., Clark-Carter, D., and Creed, F. (2002). Depression in rheumatoid arthritis: a systematic review of the literature with meta-analysis. Psych. Med. 64, 52–60. doi: 10.1097/00006842-200201000-00008
Ding, Y., Chang, L. C., Wang, X., Guilloux, J. P., Parrish, J., Oh, H., et al. (2015). Molecular and genetic characterization of depression: overlap with other psychiatric disorders and aging. Mol. Neuropsychiatry 1, 1–12. doi: 10.1159/000369974
Dong, H., Zhang, X., and Qian, Y. (2014). Mast cells and neuroinflammation. Med. Sci. Monitor Basic Res. 20, 200–206. doi: 10.12659/MSMBR.893093
Fabrazzo, M., and De Santo, R. M. (2006). Depression in chronic kidney disease. Semin. Nephrol. 26, 56–60. doi: 10.1016/j.semnephrol.2005.06.012
Fakhoury, M. (2017). The habenula in psychiatric disorders: more than three decades of translational investigation. Neurosci. Biobehav. Rev. 83, 721–735. doi: 10.1016/j.neubiorev.2017.02.010
Filiano, A. J., Xu, Y., Tustison, N. J., Marsh, R. L., Baker, W., Smirnov, I., et al. (2016). Unexpected role of interferon-γ in regulating neuronal connectivity and social behaviour. Nature 535, 425–429. doi: 10.1038/nature18626
Forsythe, P., and Bienenstock, J. (2012). The mast cell-nerve functional unit: a key component of physiologic and pathophysiologic responses. Chem. Immunol. Allergy 98, 196–221. doi: 10.1159/000336523
Fowler, C D., and Kenny, P. J. (2014). Nicotine aversion: neurobiological mechanisms and relevance to tobacco dependence vulnerability. Neuropharmacology 76(Pt B), 533–544. doi: 10.1016/j.neuropharm.2013.09.008
Fowler, C. D., Lu, Q., Johnson, P. M., Marks, M. J., et al. (2011). Habenular α5 nicotinic receptor subunit signalling controls nicotine intake. Nature 471, 597–601. doi: 10.1038/nature09797
Frahm, S., Slimak, M. A., Ferrarese, L., Santos-Torres, J., Antolin-Fontes, B., Auer, S., et al. (2011). Aversion to nicotine is regulated by the balanced activity of β4 and α5 nicotinic receptor subunits in the medial habenula. Neuron 70, 522–535. doi: 10.1016/j.neuron.2011.04.013
Fredrickson, B. L., Grewen, K. M., Coffey, K. A., Algoe, S. B. Firestine, A. M., Arevalo, J., et al. (2013). A functional genomic perspective on human well-being. Proc. Natl. Acad. Sci. U.S.A. 110, 13684–13689. doi: 10.1073/pnas.1305419110
Galli, S. J., Starkl, P., Marichal, T., and Tsai, M. (2016). Mast cells and ige in defense against venoms: possible ‘good side’ of allergy?” Allergol. Int. 65, 3–15. doi: 10.1016/j.alit.2015.09.002
Gandhi, K. K., Foulds, J., Steinberg, M. B., Lu, S.-E., and Williams, J. M. (2009). Lower quit rates among african american and latino menthol cigarette smokers at a tobacco treatment clinic. Int. J. Clin. Pract. 63, 360–367. doi: 10.1111/j.1742-1241.2008.01969.x
Georgin-Lavialle, S., Moura, D. S., Salvador, A., Chauvet-Gelinier, J.-C., Launay, J.-M., Côté, F., et al. (2016). Mast cells’ involvement in inflammation pathways linked to depression: evidence in mastocytosis. Mol. Psychiatry 21, 1511–1516. doi: 10.1038/mp.2015.216
Gillis, J., Mistry, M., and Pavlidis, P. (2010). Gene function analysis in complex data sets using ermine. J. Nat. Protoc. 5, 1148–1159. doi: 10.1038/nprot.2010.78
Grau, I., Ardanuy, C., Calatayud, L., Schulze, M. H., Liñares, J., and Pallares, R. (2014). Smoking and alcohol abuse are the most preventable risk factors for invasive pneumonia and other pneumococcal infections. Int. J. Infect. Dis. 25, 59–64. doi: 10.1016/j.ijid.2013.12.013
Graziane, N. M., Neumann, P. A., and Dong, Y. (2018). A focus on reward prediction and the lateral habenula: functional alterations and the behavioral outcomes induced by drugs of abuse. Front. Synaptic Neurosci. 10:12. doi: 10.3389/fnsyn.2018.00012
Haack, A. K., Sheth, C., Schwager, A. L., Sinclair, M. S., Tandon, S., and Taha, S. A. (2014). Lesions of the lateral habenula increase voluntary ethanol consumption and operant self-administration, block yohimbine-induced reinstatement of ethanol seeking, and attenuate ethanol-induced conditioned taste aversion. PLoS One 9:e92701. doi: 10.1371/journal.pone.0092701
Haghpanah, T., Afarinesh, M., and Divsalar, K. (2010). A review on hematological factors in opioid-dependent people (opium and Heroin) after the withdrawal period. Addict. Health 2, 9–16.
Hakkola, J., Raunio, H., Purkunen, R., Saarikoski, S., Vähäkangas, K., Pelkonen, O., et al. (2001). Cytochrome P450 3A expression in the human fetal liver: evidence that CYP3A5 is expressed in only a limited number of fetal livers. Biol. Neonate 80, 193–201. doi: 10.1159/000047142
Hawrylycz, M. J., Lein, E. S., Guillozet-Bongaarts, A. L., Shen, E. H., Ng, L., Miller, J. A., et al. (2012). An anatomically comprehensive atlas of the adult human brain transcriptome. Nature 489, 391–399. doi: 10.1038/nature11405
Henderson, B. J., Wall, T. R., Henley, B. M., Kim, C. H., Nichols, W. A., Moaddel, R., et al. (2016). Menthol alone upregulates midbrain nAChRs, alters nAChR subtype stoichiometry, alters dopamine neuron firing frequency, and prevents nicotine reward. J. Neurosci. 36, 2957–2974. doi: 10.1523/JNEUROSCI.4194-15.2016
Hikosaka, O. (2010). The habenula: from stress evasion to value-based decision-making. Nat. Rev. Neurosci. 11, 503–513. doi: 10.1038/nrn2866
Hikosaka, O., Sesack, S. R., Lecourtier, L., and Shepard, P. D. (2008). Habenula: crossroad between the basal ganglia and the limbic system. J. Neurosci. 28, 11825–11829. doi: 10.1523/JNEUROSCI.3463-08.2008
Hsu, Y. W. A., Wang, S. D., Wang, S., Morton, G., Zariwala, H. A., de la Iglesia, H. O., et al. (2014). Role of the dorsal medial habenula in the regulation of voluntary activity, motor function, hedonic state, and primary reinforcement. J. Neurosci. 34, 11366–11384. doi: 10.1523/JNEUROSCI.1861-14.2014
Ibrahim, M. Z. M. (1974). The mast cells of the mammalian central nervous system: part 1. morphology, distribution and histochemistry. J. Neurol. Sci. 21, 431–478. doi: 10.1016/0022-510X(74)90043-4
Ioannides, C. (1996). Cytochromes P450: Metabolic and Toxicological Aspects. Boca Raton, FL: CRC Press.
Jaffe, J. A., and Kimmel, P. L. (2006). Chronic nephropathies of cocaine and heroin abuse: a critical review. Clin. J. Am. Soc. Nephrol. 1, 655–667. doi: 10.2215/CJN.00300106
Jensen, K. P. (2016). A review of genome-wide association studies of stimulant and opioid use disorders. Mol. Neuropsychiatry 2, 37–45. doi: 10.1159/000444755
Kempuraj, D., Selvakumar, G. P., Thangavel, R., Ahmed, M. E., Zaheer, S., Raikwar, S. P., et al. (2017). Mast cell activation in brain injury, stress, and post-traumatic stress disorder and alzheimer’s disease pathogenesis. Front. Neurosci. 11:703. doi: 10.3389/fnins.2017.00703
Kenny, P. J. (2011). Reward mechanisms in obesity: new insights and future directions. Neuron 69, 664–679. doi: 10.1016/j.neuron.2011.02.016
Khaled, M. A., Pushparaj, A., Di Ciano, P., Diaz, J., and Le Foll, B. (2014). Dopamine D3 receptors in the basolateral amygdala and the lateral habenula modulate cue-induced reinstatement of nicotine seeking. Neuropsychopharmacology 39, 3049–3058. doi: 10.1038/npp.2014.158
Kononoff, J., Kallupi, M., Kimbrough, A., Conlisk, D., de Guglielmo, G., and George, O. (2018). Systemic and intra-habenular activation of the orphan g protein-coupled receptor GPR139 decreases compulsive-like alcohol drinking and hyperalgesia in alcohol-dependent rats. eNeuro 5:ENEURO.0153-18.2018.
Kwiatkowska, W., Knysz, B., Gąsiorowski, J., and Witkiewicz, W. (2015). Deep vein thrombosis of the lower limbs in intravenous drug users. Postepy Hig. Med. Dosw. 69, 510–520. doi: 10.5604/17322693.1150215
Labonté, B., Engmann, O., Purushothaman, I., Menard, C., Wang, J., Tan, C., et al. (2017). Sex-specific transcriptional signatures in human depression. Nat. Med. 23, 1102–1111. doi: 10.1038/nm.4386
Lawson, R. P., Nord, C. L., Seymour, B., Thomas, D. L., Dayan, P. S., Pilling, S., et al. (2017). Disrupted habenula function in major depression. Mol. Psychiatry 22, 202–208. doi: 10.1038/mp.2016.81
Lawson, R. P., Seymour, B., Loh, E., Lutti, A., Dolan, R. J., Dayan, P., et al. (2014). The habenula encodes negative motivational value associated with primary punishment in humans. Proc. Natl. Acad. Sci. U.S.A. 111, 11858–11863. doi: 10.1073/pnas.1323586111
Lecca, S., Meye, F. J., and Mameli, M. (2014). The lateral habenula in addiction and depression: an anatomical, synaptic and behavioral overview. Eur. J. Neurosci. 39, 1170–1178. doi: 10.1111/ejn.12480
Lecourtier, L., and Kelly, P. H. (2007). A conductor hidden in the orchestra? Role of the habenular complex in monoamine transmission and cognition. Neurosci. Biobehav. Rev. 31, 658–672. doi: 10.1016/j.neubiorev.2007.01.004
Lee, C.-T., Chen, J., Kindberg, A. A., Spivak, C. E., Williams, M. P., Richie, C. T., et al. (2017). CYP3A5 mediates effects of cocaine on human neocorticogenesis: studies using an in vitro 3D self-organized hPSC model with a single cortex-like unit. Neuropsychopharmacology 42, 774–784. doi: 10.1038/npp.2016.156
Liu, C., Bonaventure, P., Lee, G., Nepomuceno, D., Kuei, C., Wu, J., et al. (2015). GPR139, an orphan receptor highly enriched in the habenula and septum, is activated by the essential amino acids L-Tryptophan and L-Phenylalanine. Mol. Pharmacol. 88, 911–925. doi: 10.1124/mol.115.100412
Liu, W.-H., Valton, V., Wang, L.-Z., Zhu, Y.-H., and Roiser, Y.-H. (2017). Association between habenula dysfunction and motivational symptoms in unmedicated major depressive disorder. Soc. Cognit. Affect. Neurosci. 12, 1520–1533. doi: 10.1093/scan/nsx074
Locke, A. E., Kahali, B., Berndt, S. I., Justice, A. E., Pers, T. H., Day, F. R., et al. (2015). Genetic studies of body mass index yield new insights for obesity biology. Nature 518, 197–206. doi: 10.1038/nature14177
López, A. J., Jia, Y., White, A. O., Kwapis, J. L., Espinoza, M., Hwang, P., et al. (2018). Medial habenula cholinergic signaling regulates cocaine-associated relapse-like behavior. Addict. Biol. [Epub ahead of print]. doi: 10.1111/adb.12605
Maldonado, M., Molfese, D. L., Viswanath, H., Curtis, K., Jones, A., Hayes, T. G., Marcelli, M., et al. (2018). The habenula as a novel link between the homeostatic and hedonic pathways in cancer-associated weight loss: a pilot study. J. Cachexia Sarcopen. Muscle 9, 497–504. doi: 10.1002/jcsm.12286
Mancarci, B. O., Toker, L., Tripathy, S. J., Li, B., Rocco, B., Sibille, E., et al. (2017). Cross-laboratory analysis of brain cell type transcriptomes with applications to interpretation of bulk tissue data. eNeuro 4:ENEURO.0212-17.2017.
Matsumoto, M., and Hikosaka, O. (2007). Lateral habenula as a source of negative reward signals in dopamine neurons. Nature 447, 1111–1115. doi: 10.1038/nature05860
McLaughlin, I., Dani, J. A., and De Biasi, M. (2017). The medial habenula and interpeduncular nucleus circuitry is critical in addiction, anxiety, and mood regulation. J. Neurochem. 142 (Suppl. 2), 130–143. doi: 10.1111/jnc.14008
Meye, F. J., Trusel, M., Soiza-Reilly, M., and Mameli, M. (2017). Neural circuit adaptations during drug withdrawal – Spotlight on the lateral habenula. Pharmacol. Biochem. Behav. 162, 87–93. doi: 10.1016/j.pbb.2017.08.007
Miller, A. H., and Raison, C. L. (2016). The role of inflammation in depression: from evolutionary imperative to modern treatment target. Nat. Rev. Immunol. 16, 22–34. doi: 10.1038/nri.2015.5
Miller, J. A., Ding, S.-L., Sunkin, S. M., Smith, K. A., Ng, L., Szafer, A., et al. (2014a). Transcriptional landscape of the prenatal human brain. Nature 508, 199–206. doi: 10.1038/nature13185
Miller, J. A., Menon, V., Goldy, J., Kaykas, A., Lee, C.-K., Smith, K. A., et al. (2014b). Improving reliability and absolute quantification of human brain microarray data by filtering and scaling probes using RNA-Seq. BMC Genomics 15:154. doi: 10.1186/1471-2164-15-154
Minicã, C. C., Mbarek, H., Pool, R., Dolan, C. V., Boomsma, D. I., and Vink, J. M. (2017). Pathways to smoking behaviours: biological insights from the tobacco and genetics consortium meta-analysis. Mol. Psychiatry 22, 82–88. doi: 10.1038/mp.2016.20
Mistry, M., and Pavlidis, P. (2010). A cross-laboratory comparison of expression profiling data from normal human postmortem brain. Neuroscience 167, 384–395. doi: 10.1016/j.neuroscience.2010.01.016
Mori, I., Diehl, A. D., Chauhan, A., Ljunggren, H. G., and Kristensson, K. (1999). Selective targeting of habenular, thalamic midline and monoaminergic brainstem neurons by neurotropic influenza A virus in mice. J. Neurovirol. 5, 355–362. doi: 10.3109/13550289909029476
Mori, I., Hossain, M. J., Takeda, K., Okamura, H., Imai, Y., Kohsaka, S., and Kimura, Y. (2001). Impaired microglial activation in the brain of IL-18-gene-disrupted mice after neurovirulent influenza A virus infection. Virology 287, 163–170. doi: 10.1006/viro.2001.1029
Morton, G., Nasirova, N., Sparks, D. W., Brodsky, M., Sivakumaran, S., Lambe, E. K., et al. (2018). Chrna5-expressing neurons in the interpeduncular nucleus mediate aversion primed by prior stimulation or nicotine exposure. J. Neurosci. doi: 10.1523/JNEUROSCI.0023-18.2018 [Epub ahead of print].
Mostafavi, S., Battle, A., Zhu, X., Potash, J. B., Weissman, M. M., Shi, J., et al. (2014). Type I interferon signaling genes in recurrent major depression: increased expression detected by whole-blood RNA sequencing. Mol. Psychiatry 19, 1267–1274. doi: 10.1038/mp.2013.161
Moura, D. S., Sultan, S., Georgin-Lavialle, S., Pillet, N., Montestruc, F., Gineste, P., et al. (2011). Depression in patients with mastocytosis: prevalence, features and effects of masitinib therapy. PLoS One 6:e26375. doi: 10.1371/journal.pone.0026375
Mulloy, B., Lever, R., and Page, C. P. (2017). Mast cell glycosaminoglycans. Glycoconj. J. 34, 351–361. doi: 10.1007/s10719-016-9749-0
Nautiyal, K. M., Ribeiro, A. C., Pfaff, D. W., and Silver, R. (2008). Brain mast cells link the immune system to anxiety-like behavior. Proc. Natl. Acad. Sci. U.S.A. 105, 18053–18057. doi: 10.1073/pnas.0809479105
Norris, P. J., Hardwick, J. P., and Emson, P. C. (1996). Regional distribution of cytochrome P450 2D1 in the rat central nervous system. J. Comp. Neurol. 366, 244–258. doi: 10.1002/(SICI)1096-9861(19960304)366:2<244::AID-CNE5>3.0.CO;2-5
Pandey, S., Shekhar, K., Regev, A., and Schier, A. F. (2018). Comprehensive identification and spatial mapping of habenular neuronal types using single-cell RNA-Seq. Curr. Biol. CB 28, 1052.e7–1065.e7. doi: 10.1016/j.cub.2018.02.040
Pang, X., Liu, L., Ngolab, J., Zhao-Shea, R., McIntosh, J. M., Gardner, P. D., et al. (2016). Habenula cholinergic neurons regulate anxiety during nicotine withdrawal via nicotinic acetylcholine receptors. Neuropharmacology 107, 294–304. doi: 10.1016/j.neuropharm.2016.03.039
Pasman, J. A., Verweij, K. J. H., Gerring, Z., Stringer, S., Sanchez-Roige, S., Treur, J. L., et al. (2018). GWAS of lifetime cannabis use reveals new risk loci, genetic overlap with psychiatric traits, and a causal influence of schizophrenia. Nat. Neurosci. 21, 1161–1170. doi: 10.1038/s41593-018-0206-1
Patten, S. B., and Barbui, C. (2004). Drug-induced depression: a systematic review to inform clinical practice. Psychother. Psychosomat. 73, 207–215. doi: 10.1159/000077739
Patten, S. B., Beck, C. A., Williams, J. V., Barbui, A. C., and Metz. L. M. (2003). Major depression in multiple sclerosis: a population-based perspective. Neurology 61, 1524–1527. doi: 10.1212/01.WNL.0000095964.34294.B4
Piñero, J., Bravo,À., Queralt-Rosinach, N., Gutiérrez-Sacristán, A., Deu-Pons, J., Centeno, E., et al. (2017). DisGeNET: a comprehensive platform integrating information on human disease-associated genes and variants. Nucleic Acids Res. 45, D833–D839. doi: 10.1093/nar/gkw943
Proulx, C. D., Hikosaka, O., and Malinow, R. (2014). Reward processing by the lateral habenula in normal and depressive behaviors. Nat. Neurosci. 17, 1146–1152. doi: 10.1038/nn.3779
Purcell, W. M., Westgate, C., and Atterwill, C. K. (1996). Rat brain mast cells: an in vitro paradigm for assessing the toxic effects of neurotropic therapeutics. Neurotoxicology 17, 845–850.
Qato, D. M., Ozenberger, K., and Olfson, M. (2018). Prevalence of prescription medications with depression as a potential adverse effect among adults in the United States. JAMA J. Am. Med. Assoc. 319, 2289–2298. doi: 10.1001/jama.2018.6741
Ritchie, M. E., Phipson, B., Wu, D., Hu, Y., Law, C. W., Shi, W., et al. (2015). Limma powers differential expression analyses for RNA-sequencing and microarray studies. Nucleic Acids Res. 43:e47. doi: 10.1093/nar/gkv007
Robertson, G. R., Field, J., Goodwin, B., Bierach, S., Tran, M., Lehnert, A., et al. (2003). Transgenic mouse models of human CYP3A4 gene regulation. Mol. Pharmacol. 64, 42–50. doi: 10.1124/mol.64.1.42
Rook, E. J., van Zanten, A. P., Van den Brink, W., van Ree, J. M., and Beijnen, J. H. (2006). Mast cell mediator tryptase levels after inhalation or intravenous administration of high doses pharmaceutically prepared heroin. Drug Alcohol. Depend. 85, 185–190. doi: 10.1016/j.drugalcdep.2006.04.013
Schmitt, C., Strazielle, N., and Ghersi-Egea, J. F. (2012). Brain leukocyte infiltration initiated by peripheral inflammation or experimental autoimmune encephalomyelitis occurs through pathways connected to the csf-filled compartments of the forebrain and midbrain. J. Neuroinflamm. 9:187. doi: 10.1186/1742-2094-9-187
Secor, V. H., Secor, W. E., Gutekunst, C. A., and Brown, M. A. (2000). Mast cells are essential for early onset and severe disease in a murine model of multiple sclerosis. J. Exp. Med. 191, 813–822. doi: 10.1084/jem.191.5.813
Servière, J., Dubayle, D., and Menétrey. D. (2003). Increase of rat medial habenular mast cell numbers by systemic administration of cyclophosphamide. Toxicol. Lett. 145, 143–152. doi: 10.1016/S0378-4274(03)00264-9
Setiawan, E., Attwells, S., Wilson, A. A., Mizrahi, R., Rusjan, P. M., Miler, L., et al. (2018). Association of translocator protein total distribution volume with duration of untreated major depressive disorder: a cross-sectional study. Lancet. Psychiatry 5, 339–347. doi: 10.1016/S2215-0366(18)30048-8
Simonnet, A., Zamberletti, E., Cador, M., Rubino, T., and Caillé, S. (2017). Chronic FAAH inhibition during nicotine abstinence alters habenular CB1 receptor activity and precipitates depressive-like behaviors. Neuropharmacology 113, 252–259. doi: 10.1016/j.neuropharm.2016.10.007
Slimak, M. A., Ables, J. L., Frahm, S., Antolin-Fontes, B., Santos-Torres, J., Moretti, M., et al. (2014). Habenular expression of rare missense variants of the β4 nicotinic receptor subunit alters nicotine consumption. Front. Hum. Neurosci. 8:12. doi: 10.3389/fnhum.2014.00012
Stingl, J. C., Bartels, H., Viviani, R., Lehmann, M. L., and Brockmöller, J. (2014). Relevance of UDP-glucuronosyltransferase polymorphisms for drug dosing: a quantitative systematic review. Pharmacol. Therapeut. 141, 92–116. doi: 10.1016/j.pharmthera.2013.09.002
Sugama, S., Cho, B. P., Baker, H., Joh, T. H., Lucero, J., and Conti, B. (2002). Neurons of the superior nucleus of the medial habenula and ependymal cells express IL-18 in rat CNS. Brain Res. 958, 1–9. doi: 10.1016/S0006-8993(02)03363-2
Taiwo, O. B., Kovács, K. J., Sperry, L. C., and Larson, A. A. (2004). Naloxone-induced morphine withdrawal increases the number and degranulation of mast cells in the thalamus of the mouse. Neuropharmacology 46, 824–835. doi: 10.1016/j.neuropharm.2003.11.022
Tuesta, L. M., Chen, Z., Duncan, A., Fowler, C. D., Ishikawa, M., Lee, B. R., et al. (2017). GLP-1 acts on habenular avoidance circuits to control nicotine intake. Nat. Neurosci. 20, 708–716. doi: 10.1038/nn.4540
Velasquez, K. M., Molfese, D. L., and Salas, R. (2014). The role of the habenula in drug addiction. Front. Hum. Neurosci. 8:174. doi: 10.3389/fnhum.2014.00174
Wagner, F., Bernard, R., Derst, C., French, L., and Veh, R. W. (2016a). Microarray analysis of transcripts with elevated expressions in the rat medial or lateral habenula suggest fast GABAergic excitation in the medial habenula and habenular involvement in the regulation of feeding and energy balance. Brain Struct. Funct. 221, 4663–4689.
Wagner, F., French, L., and Veh, R. W. (2016b). Transcriptomic-anatomic analysis of the mouse habenula uncovers a high molecular heterogeneity among neurons in the lateral complex, while gene expression in the medial complex largely obeys subnuclear boundaries. Brain Struct. Funct. 221, 39–58. doi: 10.1007/s00429-014-0891-9
Weiner, J. III, and Domaszewska, T. (2016). Tmod: an R package for general and multivariate enrichment analysis. PeerJ 4:e2420v1. doi: 10.7287/peerj.preprints.2420v1
Wilhelm, M. (2011). Neuro-immune interactions in the dove brain. Gen. Comp. Endocrinol. 172, 173–180. doi: 10.1016/j.ygcen.2011.03.018
Wray, N. R., Ripke, S., Mattheisen, M., Trzaskowski, M., Byrne, E. M., Abdellaoui, A., et al. (2018). Genome-wide association analyses identify 44 risk variants and refine the genetic architecture of major depression. Nat. Genet. 50, 668–681. doi: 10.1038/s41588-018-0090-3
Zhao, Y. W., Pan, Y. Q., Tang, M. M., and Lin, W. J. (2018). Blocking p38 signaling reduces the activation of pro-inflammatory cytokines and the phosphorylation of p38 in the habenula and reverses depressive-like behaviors induced by neuroinflammation. Front. Pharmacol. 9:511. doi: 10.3389/fphar.2018.00511
Zhao-Shea, R., DeGroot, S. R., Liu, L., Vallaster, M., Pang, X., Su, Q., et al. (2015). Increased CRF signalling in a ventral tegmental area-interpeduncular nucleus-medial habenula circuit induces anxiety during nicotine withdrawal. Nat. Commun. 6:6770. doi: 10.1038/ncomms7770
Zhuang, X., Silverman, A. J., and Silver, R. (1996). Brain mast cell degranulation regulates blood-brain barrier. J. Neurobiol. 31, 393–403. doi: 10.1002/(SICI)1097-4695(199612)31:4<393::AID-NEU1>3.0.CO;2-4
Keywords: transcriptomics, habenula, addiction, depression, neuroimmune, mast cell, drug metabolism, cannabis
Citation: Le Foll B and French L (2018) Transcriptomic Characterization of the Human Habenula Highlights Drug Metabolism and the Neuroimmune System. Front. Neurosci. 12:742. doi: 10.3389/fnins.2018.00742
Received: 10 July 2018; Accepted: 26 September 2018;
Published: 31 October 2018.
Edited by:
Fereshteh S. Nugent, Uniformed Services University of the Health Sciences, United StatesReviewed by:
Lucia Carboni, Università degli Studi di Bologna, ItalyEnrico Domenici, University of Trento, Italy
Copyright © 2018 Le Foll and French. This is an open-access article distributed under the terms of the Creative Commons Attribution License (CC BY). The use, distribution or reproduction in other forums is permitted, provided the original author(s) and the copyright owner(s) are credited and that the original publication in this journal is cited, in accordance with accepted academic practice. No use, distribution or reproduction is permitted which does not comply with these terms.
*Correspondence: Leon French, bGVvbmZyZW5jaEBnbWFpbC5jb20=