- 1Medical Psychological Center, The Second Xiangya Hospital, Central South University, Changsha, China
- 2Center for Studies of Psychological Application, School of Psychology, South China Normal University, Guangzhou, China
- 3Medical Psychological Institute, Central South University, Changsha, China
- 4Department of Radiology, The Second Xiangya Hospital, Central South University, Changsha, China
Background: Emerging evidences supported the hypothesis that emotional dysregulation results from aberrant connectivity within the fronto-limbic neural networks in patients with borderline personality disorder (BPD). Considering its important role in emotional regulation, the anterior cingulate cortex (ACC) has not yet been fully explored in BPD patients. Therefore, using the seed-based resting state functional connectivity (rsFC) and probabilistic fiber tracking, we aimed to explore the alterations of functional and structural connectivity (SC) of the ACC in patients with BPD.
Methods: A cohort of 50 unmedicated, young BPD patients and 54 sex-, age-, and education-matched healthy controls (HCs) completed psychological tests and underwent rs-fMRI and diffuse tensor imaging (DTI) scanning. Rs-FC analysis and probabilistic fiber tracking were used to plot SC and FC of the ACC.
Results: With the left ACC selected as a seed, BPD patients exhibited increased rsFC and abnormal SC with the right middle frontal gyrus (MFG), and decreased rsFC with the left middle temporal gyrus (MTG), compared with HCs. Additionally, negative cognitive emotion regulation and depressive symptoms both correlated negatively with the rsFC of the left ACC in BPD patients.
Conclusion: Abnormal SC and FC of the ACC underlie the deficient emotional regulation circuitry in BPD patients. Such alterations may be important biomarkers of BPD and thus could point to potential BPD treatment targets.
Introduction
Borderline personality disorder is a common psychiatric disorder, characterized by a pervasive pattern of emotional lability, impulsivity, interpersonal difficulties, identity disturbances, and disturbed cognition (Leichsenring et al., 2011). Emotional dysregulation, which is considered a core pathological feature of BPD (Gratz and Roemer, 2004; Dsm-5, 2013), has been attributed to weakened inhibitory effects of the prefrontal cortex (PFC) on hyperactive limbic brain regions in response to emotional stimuli (Krause-Utz et al., 2014b; Schulze et al., 2016).
The PFC involvement in emotion regulation has been supported by neuroimaging studies in which control of emotion-related behavior was related to the activation of several frontal regions, including the ACC, dorsolateral PFC, and ventral medial PFC (Koenigsberg et al., 2009; Krause-Utz et al., 2012; Kamphausen et al., 2013; Ruocco et al., 2013). For example, activation of the ACC and other prefrontal structures (e.g., dorsolateral PFC and medial or orbital PFC) has been found to be consistently decreased during emotional processing in BPD patients compared with HCs (Silbersweig et al., 2007; Kraus et al., 2010; Smoski et al., 2011). Importantly, decreased PFC activation was reported to be more pronounced in response to negative emotional stimuli than to neutral stimuli (Schulze et al., 2016).
Apart from aberrant functional alterations of the ACC and other frontal structures, there also have been reported structural and biochemical aberration in these frontal lobes in BPD studies. Decreased gray matter volume (Hazlett et al., 2005; Minzenberg et al., 2008; Schulze et al., 2016) and thickness (Rüsch et al., 2007) in ACC, and reduced gray matter volumes in the orbitofrontal cortex and dorsolateral PFC (Chanen et al., 2008; Soloff et al., 2012), have been reported in patients with BPD, compared with HCs. In addition, BPD patients have increased glutamate and N-acetylaspartate concentrations in the left ACC (Hoerst et al., 2010; Rüsch et al., 2010a) and decreased N-acetylaspartate concentrations in the dorsolateral PFC (Tebartz van Elst et al., 2001).
Previous neuroimaging studies on BPD suggested that abnormal functional activity in frontal regions might be interrelated rather than independent (New et al., 2007; Niedtfeld et al., 2012; Kamphausen et al., 2013; Krause-Utz et al., 2014a). The frontal lobes and the ACC in particular played important roles in emotional cognitive control, and their deactivation was related strongly to negative emotional processing in BPD patients (Bush et al., 2000). Anatomically, the ACC has rich structural and functional connections with other regions in the frontal lobes. For example, the subgenual cingulate gyrus (part of the ventral medial cingulate gyrus) receives afferent projections from the orbital cortex and has a close relationship with part of the orbital–frontal cortex (Rüsch et al., 2010b). These connections help to integrate sensory information, coordinate autonomic nervous responses, and regulate emotion and behavior (Kringelbach, 2005). However, few studies have investigated alterations in SC or FC of emotion-related brain regions in BPD, especially the ACC. In one study investigating interhemispheric SC in BPD with a novel fiber-tracking approach, impaired connectivity between the left and right ACCs was observed (Rüsch et al., 2010b). Further investigations focusing on the ACC and other prefrontal areas involved in emotion regulation should be performed to confirm whether there is impaired interhemispheric connectivity in these patients (Rüsch et al., 2007, 2010b).
In this study, we used a multimodal approach combining rsFC and probabilistic fiber tracking with the bilateral ACC as seeds, to explore SC and FC of the ACC with other frontal regions, and how they relate to BPD-relevant psychological variables, namely negative affect intensity, negative cognitive emotion regulation strategies, depression, and anxiety. To exclude the influence of age, drug use, and comorbidity, we used a young BPD group with no antipsychotic drug use and no comorbidities. The severity of depression and anxiety symptoms was controlled as covariates to limit the influence of possible confounding effects.
Materials and Methods
Subjects
Patients diagnosed with BPD were recruited from an outpatient clinic affiliated with the Second Xiangya Hospital of Central South University in Changsha, Hunan province, China. HCs were recruited by advertisements from the surrounding communities. BPD diagnostic assessments were performed by two well-qualified psychiatrists using the structured clinical interview for axis II disorders (SCID II, First and Gibbon, 2004) of the Diagnostic and Statistical Manual of Mental Disorders, Fourth Edition (DSM-IV). Each patient received the Structured Clinical Interview for DSM-IV Axis I disorders (SCID I, First and Gibbon, 2004) to exclude past or current Axis I diagnoses (e.g., major depressive disorder, bipolar disorder, schizophrenia, delusional disorder, and schizo-affective disorder). The exclusion criteria for HCs were past or current history of any DSM-IV axis I or axis II disorder, a history of epilepsy or serious trauma, current medical problems, and family history of psychiatric disorders among first-degree relatives.
The study cohort included 50 patients with BPD and 54 age-, sex-, and education-matched HCs. All participants completed the rs-fMRI and T1 scanning; and of the 104 participants, 46 BPD patients and 44 HCs were scanned with DTI sequences. This study was approved by the ethics committee of the Central South University, and each participant signed an informed consent form at enrollment.
Scales
Prior to neuroimaging, all participants underwent several psychometric assessments, including the Personality Diagnostic Questionnaire (PDQ-4+), Short Affect Intensity Scale (SAIS), Cognitive Emotion Regulation Questionnaire (CERQ), Center for Epidemiologic Studies Depression Scale (CES-D), and State-Trait Anxiety Inventory (STAI). The PDQ-4+, a self-rated personality disorder questionnaire, includes 12 sub-scales corresponding to 12 kinds of personality disorders. The borderline sub-scale contains nine items adopting 0–1 scoring method (i.e., Yes or No), and the total score ranges from 0 to 9, which ≥5 indicates a screened positive BPD (Hyler et al., 1992). The SAIS was used to assess affect intensity, which includes three dimensions (positive intensity, negative affectivity, and serenity; Geuens and De Pelsmacker, 2002; Zhong et al., 2010). Only the negative affectivity score (range, 1–6) assessing negative emotional reactions (e.g., nervousness, worry, sadness, and fear) was used in the current study. The CERQ is used to measure cognitive emotion regulation strategies with positive and negative subscales (Zhu et al., 2008). In the current study, only the negative subscale was used to assess subjects’ negative emotion regulation strategies (e.g., self-blame, rumination, catastrophization, and blaming others). The STAI and the CES-D were used to assess anxiety and depression levels, respectively (Shek, 1993; Xiao et al., 2016).
MRI Parameters
Magnetic resonance imaging data were collected on a 3.0-T Philips Ingenia, in which foam pads were used to position and immobilize each subject’s head within the coil. High-resolution anatomical T1-weighted scans were obtained with the following parameters: repetition time (TR) = 7.44 ms; echo time (TE) = 3.46 ms; flip angle = 8°; sagittal slices = 301; slice thickness = 1.2 mm; slice spacing = 0.6 mm; matrix size = 240 × 240; volume = 1; and voxel size = 1 × 1 × 1 mm3.
Resting state functional magnetic resonance imaging images were obtained with following parameters: TR = 2000 ms; TE = 30 ms; flip angle = 90°; slices = 36; slice thickness = 4 mm; slice spacing = 4 mm; matrix size = 128 × 128; volumes = 200; volume interval = 2 s; and voxel size = 2 × 2 × 2 mm3. While being scanned, all participants were asked to relax, keep their eyes closed, and stay awake.
Diffuse tensor imaging images were acquired with the following parameters: TR = 6176 ms; TE = 79 ms; flip angle = 90°; slices = 60; slice thickness = 2.5 mm; slice spacing = 2.5 mm; and matrix size = 144 × 144, voxel size = 2 × 2 × 2 mm3. Diffusion-sensitizing gradient encoding was applied in 32 directions with a diffusion-weighted factor of b = 700 s/mm2 and two b0 (b = 0) images. Images were acquired parallel to the anterior and posterior commissure.
Imaging Data Processing
Rs-fMRI Processing
Resting state functional magnetic resonance imaging data were analyzed in DPARSF (Data Processing Assistant for rs-fMRI software1; Yan and Zang, 2010), based on Statistical Parametric Mapping 12 (SPM122) in MATLAB (Release 2017, The MathWorks, Inc., Natick, MA, United States). Data processing included the following steps: convert DICOM images to NIfTI files; remove the first 10 time points to minimize the influence of instability in the initial signals; slice timing with the 18th slice as the reference; spatial realignment for head motion correction (subjects with head motion >2.0 mm or >2.0° were excluded); register each subject’s fMRI images to their segmented high-resolution T1-weighted anatomical images; regress nuisance variables, including white matter (WM) and cerebral spinal fluid signals; normalize fMRI images to standard Montreal Neurological Institute (MNI) templates with a resolution of 3 × 3 × 3 mm3; smooth with a 4-mm full-width-half-maximum Gaussian kernel; linear detrend to discard physiological noise and drift from scanner instabilities and head motion; band-pass filtering (0.01–0.08 Hz); and calculate rsFC with the bilateral ACC selected as seeds, which were made by the Anatomical Automatic Labeling 90 regions (AAL90) templates to quantify the relationship between the seeds and other brain regions.
DTI Processing
Diffuse tensor imaging data were processed on the FMRIB’s Diffusion Toolbox-FDT v2.0 toolbox from FSL-FMRIB Software Library, FMRIB, Oxford, United Kingdom3 (Jenkinson et al., 2012). DTI data processing included the following steps: correct for eddy current distortion and head motion; fit a diffusion tensor model using the DTI fit in the FMRIB Diffusion Toolbox generating individual FA and MD; calculate within-voxel probability density functions of the principal diffusion direction using FSL’s BEDPOSTX tool using Markov Chain Monte Carlo sampling, which also accounts for the possibility of crossing fibers within a voxel (Woolrich et al., 2009); conduct probabilistic fiber tracking with PROBTRACKX implemented in FSL (Behrens et al., 2007), which repeatedly samples the distribution at each voxel to produce “streamlines” that connect voxels between selected seed regions (5000 streamline samples, 0.5 mm step length, curvature threshold = 0.2).
Probabilistic Fiber Tracking Analysis
Probabilistic fiber tracking analysis was based on the rsFC results and mainly investigated SC between which had abnormal functional connectivities in rs-fMRI analysis, i.e., the left ACC was selected as the seed, and the right MFG and left MTG as the targets. All selections were made by the AAL90 templates in FSL. A single image (fdt_paths) per participant was generated to visualize WM tracts connecting seed and target regions after data processing. The total number of seed-to-target WM tracts (i.e., waytotal) was also generated to calculate the connective probability or tract strength, which defined as the number of tracts from the seed, divided by the number of tracts that reach the target (van den Bos et al., 2014; Yuan et al., 2018).
After probabilistic tracking, generated fdt_paths images were further thresholded based on the individual maximum connectivity value within a tract. The maximum connectivity value was obtained with fslstats and voxels, which had values of >5% of the maximum connectivity value were kept in the analysis (Bennett et al., 2011; Hirsiger et al., 2016; Yuan et al., 2017). A common tract was created from tracts existed in ≥75% of the participants. Then, we extracted individual FA and MD of the WM tracts by de-projecting the common tracts to individual diffusion images (Ethofer et al., 2012; Gschwind et al., 2012).
Statistical Analysis
Statistical analyses were conducted in SPSS 20.0 (SPSS Inc., Chicago, IL, United States) and the SPM12 toolbox of MATLAB. To compare group differences in demographic and psychological variables, Chi-squared tests and independent-sample t-tests were conducted. To investigate the significantly altered rsFC, two sample t-tests were performed in SPM12 with depression and anxiety scores as covariates. All resulting maps were family-wise error (FWE) corrected for the whole brain. To explore potential group differences in SC (i.e., probability) and integrity (i.e., FA and MD), analysis of covariance (ANCOVA) was performed with age, depression, and anxiety levels controlled. Pearson’s correlations were conducted to investigate potential associations between emotional characteristics (i.e., psychometric scores including SAIS_N, CERQ_N, CES-D, TAI, and SAI) and neuroimaging indices (i.e., rsFC values, tract probability, mean FA, and MD) in the BPD group. A threshold of P < 0.05 was considered significant.
Results
Demographic and Psychological Characteristics
Age, sex, and education level did not differ significantly between the BPD and HC groups. The BPD group had significantly higher SAIS negative affectivity, CERQ negative subscale, CES-D, and STAI scores than the HC group (Table 1).
RsFC
Borderline personality disorder patients exhibited significantly increased rsFC of the ACC with the right MFG and decreased rsFC with the left MTG compared with HCs. In addition, the BPD group had lower connectivities between both ACC sides and the corpus collosum (CC) than HCs (Table 2 and Figure 1).
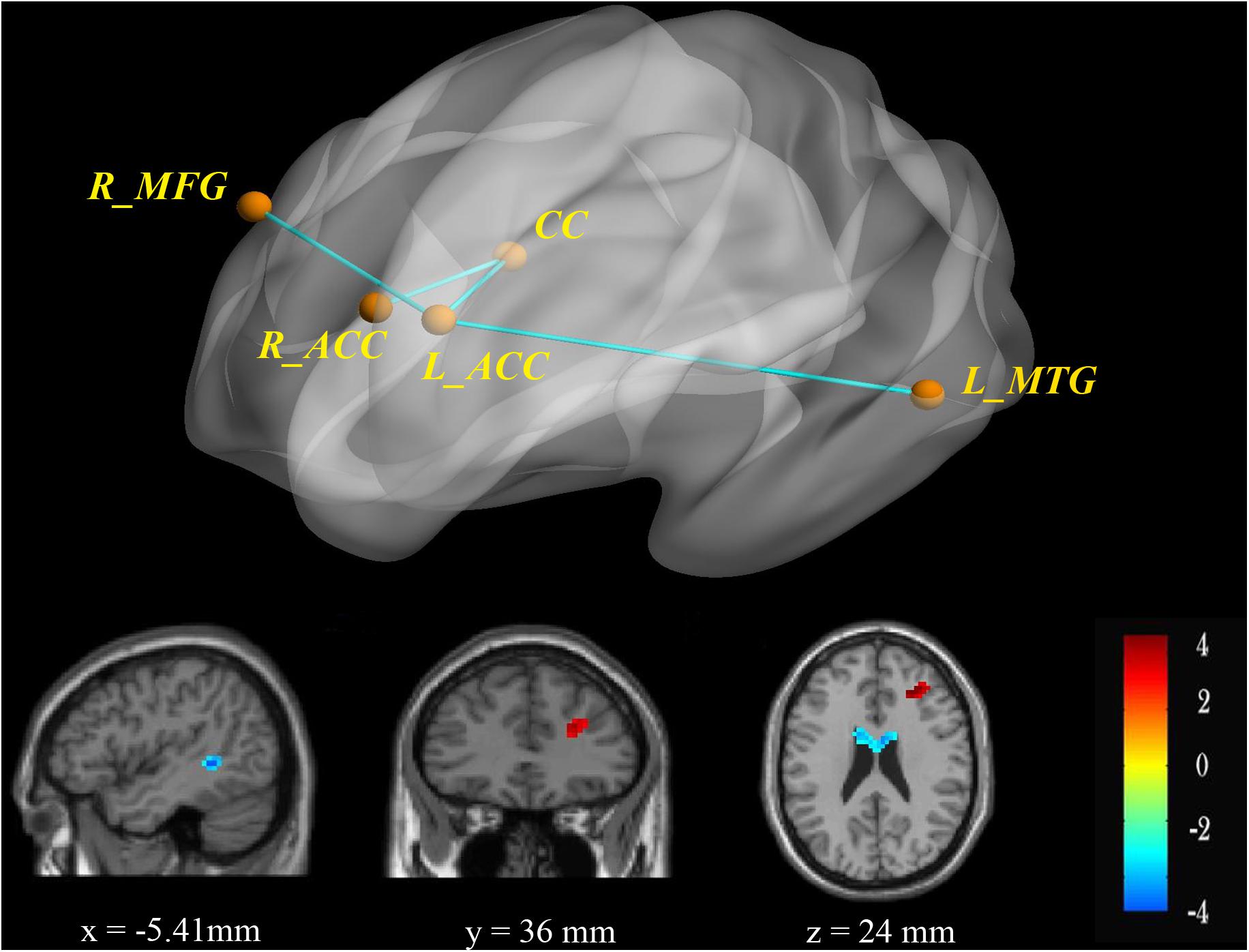
Figure 1. RsFC of the bilateral ACC as seeds. There was increased (red) rsFC between the left ACC and right MFG, as well as decreased (blue) rsFC between the left ACC and left MTG in the BPD group compared with the HC group. FWE-corrected p < 0.05 significance criterion. Color bar indicates the T-score.
SC
Compared with HCs, the BPD group had significantly decreased FA values in left ACC-to-right MFG tract (Table 3 and Figure 2), findings suggestive of impaired fiber integrity. The tract probability of left ACC-to-right MFG and left ACC-to-left MTG tract did not differ between the two groups.
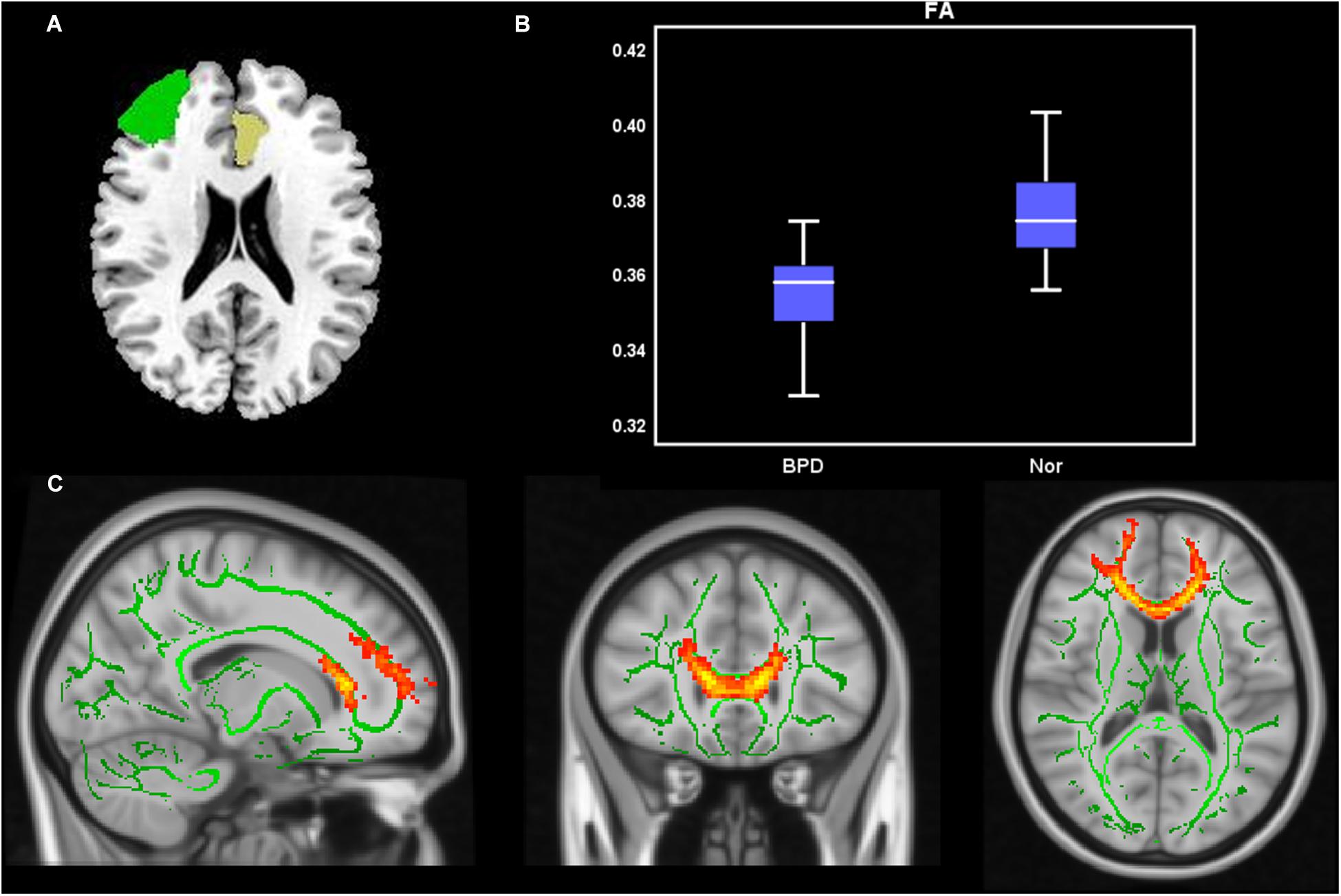
Figure 2. Probabilistic fiber tracking from the left ACC to the right MFG. (A) The seed (left ACC, yellow) and target (right MFG, green) were based on the AAL90 template and back-projected to the individual’s native space in sagittal, coronal, and axial planes. (B) Group comparisons of FA and MD values for fiber bundles passing though the left ACC to the right MFG (p < 0.05). Y-axis presents the mean of FA and MD values. The error bars present the standard error of mean. (C) Fiber distribution: fibers starting from the left ACC passed through the CC and the right ACC and then entered the right MFG.
Associations Between Imaging Parameters and Psychological Variables in BPD Patients
Pearson correlation analyses showed that CERQ-negative subscale scores correlated negatively with rsFC between the left ACC and CC (r = −0.59, P = 0.032), and CES-D scores correlated negatively with rsFC between the left ACC and right MFG (r = −0.37, P = 0.037) after multiple comparisons with Bonferroni correction. There were no other significant correlations between psychological variables (negative affective SAIS or STAI) and imaging parameters (rsFC values, tract probability, mean FA, or MD).
Discussion
In the current study, combining rsFC and probabilistic fiber tracking with the bilateral ACC as seeds, we explored the SC and FC of the ACC and examined correlations between abnormal neuroimaging indices and psychological variables in BPD patients. We found that patients with BPD exhibited abnormal SC and FC in the emotion-regulation-related ACC-CC-frontal neural circuit. These findings suggest that abnormal SC and FC in frontal–limbic structures of the brain could mediate emotional dysregulation in patients with BPD.
SC and FC Between the Left ACC and Right MFG
The ACC and PFC are key brain regions in emotional regulation and impulsiveness (Davidson and Irwin, 1999). Previous on-task fMRI studies utilizing emotion regulation strategies (e.g., restraint and reappraisal) have suggested that the ACC and other frontal regions (i.e., orbital frontal cortex, dorsolateral PFC, dorsomedial PFC, and ventrolateral PFC) are involved in emotional regulation processing (Beauregard et al., 2001; Lévesque et al., 2003; Ochsner et al., 2004; Phan et al., 2005; Urry et al., 2006). More specifically, the MFG, a component of the dorsolateral PFC, is particularly important in mood regulation and suppression of unwanted memories (Kluetsch et al., 2012; Krause-Utz et al., 2014a). In the current study, we found increased left ACC-to-right MFG FC in patients with BPD, consistent with previous findings (Krause-Utz et al., 2012). Based on rsFC data, we plotted the left ACC-to-right MFG fiber bundle in BPD patients using probabilistic fiber tracking and found that left ACC efferents passed through the CC and projected to the contralateral MFG, consistent with normal anatomy (Rüsch et al., 2010b). Importantly, we found that WM integrity from the left ACC to the right MFG was impaired with decreased FA in the BPD group compared with the HC group. The presently observed altered rsFC and impaired WM fasciculus integrity between the ACC and MFG suggest that emotional regulation circuitry may be deficient in BPD patients.
FC Between the Left ACC and Left MTG
Alterations in the brain default model network (DMN), including the medial PFC, ACC, cuneus/precuneus, MTG, thalamus, and insular cortex, which are located mainly along the midline of the brain, were found in a prior rs-fMRI study in BPD patients (Wolf et al., 2011). The ACC and MTG are important for numerous important DMN functions, including: separating internal cognition from external stimulus processing; autobiographical memory processing; and monitoring of cognitive, emotional, and somatosensory states (Buckner et al., 2008; Spreng et al., 2009; Napadow et al., 2010). In a previous rs-fMRI study, we found decreased connectivity–amplitude coupling in the left MTG, suggesting that the left MTG may be a functionally impaired hub in BPD (Lei et al., 2018). The MTG also has decreased rs activation (Wingenfeld et al., 2009) and increased activity during emotional processing in BPD patients (Guitart-Masip et al., 2009). Together with previous studies (Wolf et al., 2011; Lei et al., 2017), our study found decreased rsFC between the left ACC and the left MTG in BPD patients, which suggested disturbed DMN and emotional processing in patients with BPD.
SC and FC Between the ACC and CC
The CC mediates communication between the hemispheres (Luders et al., 2010). The WM integrity of the CC genu and body has previously been shown to be impaired in BPD patients (Salvador et al., 2016). Moreover, the CC isthmus was found to be thinner in BPD patients than in HCs, suggesting that inter-hemispheric SC may be affected in BPD (Rüsch et al., 2007). The present finding of decreased rsFC between the bilateral ACC and the CC is consistent with the possibility that SC between the cerebral hemispheres may be abnormal in BPD patients. Because the ACC is involved in regulating emotion and behavior (Minzenberg et al., 2007; Silbersweig et al., 2007; Koenigsberg et al., 2009; Niedtfeld et al., 2012; Perez et al., 2016; Schulze et al., 2016), decreased interhemispheric connectivity between the left and right ACCs may be a pathological correlate of BPD.
Psychometric Correlates of ACC Abnormalities in BPD
Patients with BPD have been shown consistently to be hypersensitive to negative emotional stimuli, with emotional dysregulation and negative regulatory strategies that are often accompanied by anxiety and depression (Yen et al., 2002; Niedtfeld et al., 2010; Schulze et al., 2016; Yang et al., 2018). The present findings of significantly higher SAIS-negative affectivity, CERQ-negative subscale, CES-D, and STAI scores in BPD patients than HCs are consistent with heightened negative intensity, depression, and anxiety levels as well as reliance on negative emotion cognition regulation strategies in BPD.
A frontal–limbic system suppression model in which there is reduced PFC control of hyperactive limbic areas has emerged as a favored hypothesis of the neurological basis of emotional dysregulation in BPD (Agrawal et al., 2004). More specifically, amygdalar hyperactivity with hypoactivity in the ACC and PFC (medial, orbital, and dorsolateral) is typical during negative emotional processes in BPD (Silbersweig et al., 2007; Perez et al., 2016; Baczkowski et al., 2017). In this study, cognitive emotion regulation scores correlated negatively with left ACC-CC rsFC, while depressive scores correlated negatively with left ACC–right MFG rsFC. These results provide further support for the view that emotional regulation circuitry may be impaired in BPD patients.
Limitations
Although we combined rsFC and SC analyses to investigate links between function and structure in BPD, which had not been thoroughly assessed before, this study had some limitations. Firstly, FC was assessed in a rs rather than in an on-task state. We are planning future studies that will focus on FC during the performance of emotion-related tasks. Secondly, because this study focused primarily on the emotion-related ACC and associated frontal regions, connectivities among other emotion-related limbic structures (e.g., amygdala) remain to be investigated further to provide a more comprehensive understanding of brain connectivity in BPD.
Conclusion
In this study, using rs-fMRI and probabilistic fiber tracking, we combined SC and FC data to investigate BPD alterations in ACC-based circuitry. With the left ACC selected as a seed, BPD patients exhibited increased rsFC and abnormal SC with the right MFG, and decreased rsFC with the left MTG, compared with HCs. Further, rsFC of the left ACC correlated negatively with negative cognitive emotion regulation and depressive symptoms in BPD patients. Given that the ACC plays an important role in emotional cognitive control, abnormal connectivity of the ACC supports the possibility that BPD may be characterized by deficient emotional regulation circuitry. Collectively, our data suggest that connectivity of these brain regions may be important imaging biomarkers in BPD populations, which could have clinical implications for treatment of the disorder.
Ethics Statement
This study was approved by the ethics committee of the Central South University, and each participant signed an informed consent form at enrollment.
Author Contributions
XL analyzed the data and wrote the manuscript. BZ, HY, WP, QL, and YZ collected the data. MZ, CT, and JY gave the idea and revised the manuscript. SY also helped the manuscript in revision.
Funding
This study was supported by grant from the National Natural Science Foundation of China (Grant No. 81370034).
Conflict of Interest Statement
The authors declare that the research was conducted in the absence of any commercial or financial relationships that could be construed as a potential conflict of interest.
Abbreviations
ACC, anterior cingulate cortex; BPD, borderline personality disorder; CC, corpus collosum; DTI, diffusion tensor imaging; FA, fractional anisotropy; FC, functional connectivity; HCs, healthy controls; MD, mean diffusivity; MFG, middle frontal gyrus; MTG, middle temporal gyrus; PFC, prefrontal cortex; rs-fMRI, resting state functional magnetic resonance imaging; SC, structural connectivity; WM, white matter.
Footnotes
- ^ http://rfmri.org/DPARSF
- ^ http://www.fil.ion.ucl.ac.uk/spm/
- ^ https://fsl.fmrib.ox.ac.uk/fsl/fslwiki/FSL
References
Agrawal, H. R., Gunderson, J., Holmes, B. M., and Lyons-Ruth, K. (2004). Attachment studies with borderline patients: a review. Harv. Rev. Psychiatry 12, 94–104. doi: 10.1080/10673220490447218
Baczkowski, B. M., van Zutphen, L., Siep, N., Jacob, G. A., Domes, G., Maier, S., et al. (2017). Deficient amygdala–prefrontal intrinsic connectivity after effortful emotion regulation in borderline personality disorder. Eur. Arch. Psychiatry Clin. Neurosci. 267, 551–565. doi: 10.1007/s00406-016-0760-z
Beauregard, M., Lévesque, J., and Bourgouin, P. (2001). Neural correlates of conscious self-regulation of emotion. J. Neurosci. 21, 1–6.
Behrens, T. E. J., Berg, H. J., Jbabdi, S., Rushworth, M. F. S., and Woolrich, M. W. (2007). Probabilistic diffusion tractography with multiple fibre orientations: what can we gain? Neuroimage 34, 144–155. doi: 10.1016/j.neuroimage.2006.09.018
Bennett, I. J., Madden, D. J., Vaidya, C. J., Howard, J. H., and Howard, D. V. (2011). White matter integrity correlates of implicit sequence learning in healthy aging. Neurobiol. Aging 32, 2317.e1–2317.e12. doi: 10.1016/j.neurobiolaging.2010.03.017
Buckner, R. L., Andrews-Hanna, J. R., and Schacter, D. L. (2008). The brain’s default network: anatomy, function, and relevance to disease. Ann. N. Y. Acad. Sci. 1124, 1–38. doi: 10.1196/annals.1440.011
Bush, G., Luu, P., and Posner, M. I. (2000). Bush_2000_conitive and emotional influence in anterior cingulate cortex. Trends Cogn. Sci. 4, 215–222. doi: 10.1016/s1364-6613(00)01483-2
Chanen, A. M., Velakoulis, D., Carison, K., Gaunson, K., Wood, S. J., Yuen, H. P., et al. (2008). Orbitofrontal, amygdala and hippocampal volumes in teenagers with first-presentation borderline personality disorder. Psychiatry Res. - Neuroimaging 163, 116–125. doi: 10.1016/j.pscychresns.2007.08.007
Davidson, R. J., and Irwin, W. (1999). The functional neuroanatomy of emotion and affective style. Trends Cogn. Sci. 3, 11–21. doi: 10.1016/S1364-6613(98)01265-1260
Dsm-5 (2013). Diagnostic and Statistical Manual of Mental Disorders (DSM-5), 5th Edn. Washington, DC: American Psychiatric Association.
Ethofer, T., Bretscher, J., Gschwind, M., Kreifelts, B., Wildgruber, D., and Vuilleumier, P. (2012). Emotional voice areas: anatomic location, functional properties, and structural connections revealed by combined fMRI/DTI. Cereb. Cortex 22, 191–200. doi: 10.1093/cercor/bhr113
First, M. B., and Gibbon, M. (2004). “The structured clinical interview for DSM-IV axis i disorders (SCID-I) and the structured clinical interview for DSM-IV axis II disorders (SCID-II),”in Comprehensive Handbook Of Psychological Assessment Personality Assessment, M. J. Hilsenroth, and D. L. Segal, (Hoboken, NJ: John Wiley & Sons Inc), 134–143.
Geuens, M., and De Pelsmacker, P. (2002). Developing a short affect intensity scale. Psychol. Rep. 91, 657–670. doi: 10.2466/pr0.2002.91.2.657
Gratz, K. L., and Roemer, L. (2004). Multidimensional assessment of emotion regulation and dysregulation: development, factor structure, and initial validation of the difficulties in emotion regulation scale. J. Psychopathol. Behav. Assess. 26, 41–54. doi: 10.1023/B:JOBA.0000007455.08539.94
Gschwind, M., Pourtois, G., Schwartz, S., Van De Ville, D., and Vuilleumier, P. (2012). White-matter connectivity between face-responsive regions in the human brain. Cereb. Cortex 22, 1564–1576. doi: 10.1093/cercor/bhr226
Guitart-Masip, M., Pascual, J. C., Carmona, S., Hoekzema, E., Bergé, D., Pérez, V., et al. (2009). Neural correlates of impaired emotional discrimination in borderline personality disorder: an fMRI study. Prog. Neuro Psychopharmacol. Biol. Psychiatry 33, 1537–1545. doi: 10.1016/j.pnpbp.2009.08.022
Hazlett, E. A., New, A. S., Newmark, R., Haznedar, M. M., Lo, J. N., Speiser, L. J., et al. (2005). Reduced anterior and posterior cingulate gray matter in borderline personality disorder. Biol. Psychiatry 58, 614–623. doi: 10.1016/j.biopsych.2005.04.029
Hirsiger, S., Koppelmans, V., Mérillat, S., Liem, F., Erdeniz, B., Seidler, R. D., et al. (2016). Structural and functional connectivity in healthy aging: associations for cognition and motor behavior. Hum. Brain Mapp. 37, 855–867. doi: 10.1002/hbm.23067
Hoerst, M., Weber-Fahr, W., Tunc-Skarka, N., Ruf, M., Bohus, M., Schmahl, C., et al. (2010). Correlation of glutamate levels in the anterior cingulate cortex with self-reported impulsivity in patients with borderline personality disorder and healthy controls. Arch. Gen. Psychiatry 67, 946–954. doi: 10.1001/archgenpsychiatry.2010.93
Hyler, S. E., Skodol, A. E., Oldham, J. M., Kellman, H. D., and Doidge, N. (1992). Validity of the personality diagnostic questionnaire-revised: a replication in an outpatient sample. Compr. Psychiatry 33, 73–77. doi: 10.1016/0010-440X(92)90001-90007
Jenkinson, M., Beckmann, C. F., Behrens, T. E. J., Woolrich, M. W., and Smith, S. M. (2012). FSL. Neuroimage 62, 782–790. doi: 10.1016/j.neuroimage.2011.09.015
Kamphausen, S., Schröder, P., Maier, S., Bader, K., Feige, B., Kaller, C. P., et al. (2013). Medial prefrontal dysfunction and prolonged amygdala response during instructed fear processing in borderline personality disorder. World J. Biol. Psychiatry 14, 307–318. doi: 10.3109/15622975.2012.665174
Kluetsch, R. C., Schmahl, C., Niedtfeld, I., Densmore, M., Calhoun, V. D., Daniels, J., et al. (2012). Alterations in default mode network connectivity during pain processing in borderline personality disorder. Arch. Gen. Psychiatry 69, 993–1002. doi: 10.1001/archgenpsychiatry.2012.476
Koenigsberg, H. W., Siever, L. J., Lee, H., Pizzarello, S., New, A. S., Goodman, M., et al. (2009). Neural correlates of emotion processing in borderline personality disorder. Psychiatry Res. Neuroimaging 172, 192–199. doi: 10.1016/j.pscychresns.2008.07.010
Kraus, A., Valerius, G., Seifritz, E., Ruf, M., Bremner, J. D., Bohus, M., et al. (2010). Script-driven imagery of self-injurious behavior in patients with borderline personality disorder: a pilot FMRI study. Acta Psychiatr. Scand. 121, 41–51. doi: 10.1111/j.1600-0447.2009.01417.x
Krause-Utz, A., Oei, N. Y. L., Niedtfeld, I., Bohus, M., Spinhoven, P., Schmahl, C., et al. (2012). Influence of emotional distraction on working memory performance in borderline personality disorder. Psychol. Med. 42, 2181–2192. doi: 10.1017/S0033291712000153
Krause-Utz, A., Veer, I. M., Rombouts, S. A. R. B., Bohus, M., Schmahl, C., and Elzinga, B. M. (2014a). Amygdala and anterior cingulate resting-state functional connectivity in borderline personality disorder patients with a history of interpersonal trauma. Psychol. Med. 44, 2889–2901. doi: 10.1017/S0033291714000324
Krause-Utz, A., Winter, D., Niedtfeld, I., and Schmahl, C. (2014b). The latest neuroimaging findings in borderline personality disorder topical collection on personality disorders. Curr. Psychiatry Rep. 16:348. doi: 10.1007/s11920-014-0438-z
Kringelbach, M. L. (2005). The human orbitofrontal cortex: linking reward to hedonic experience. Nat. Rev. Neurosci. 6, 691–702. doi: 10.1038/nrn1747
Lei, X., Liao, Y., Zhong, M., Peng, W., Liu, Q., Yao, S., et al. (2018). Functional connectivity density, local brain spontaneous activity, and their coupling strengths in patients with borderline personality disorder. Front. Psychiatry 9:342. doi: 10.3389/fpsyt.2018.00342
Lei, X., Zhong, M., Liu, Y., Jin, X., Zhou, Q., Xi, C., et al. (2017). A resting-state fMRI study in borderline personality disorder combining amplitude of low frequency fluctuation, regional homogeneity and seed based functional connectivity. J. Affect. Disord. 218, 299–305. doi: 10.1016/j.jad.2017.04.067
Leichsenring, F., Leibing, E., Kruse, J., New, A. S., and Leweke, F. (2011). Borderline personality disorder. Lancet 377, 74–84. doi: 10.1016/S0140-6736(10)61422-61425
Lévesque, J., Eugène, F., Joanette, Y., Paquette, V., Mensour, B., Beaudoin, G., et al. (2003). Neural circuitry underlying voluntary suppression of sadness. Biol. Psychiatry 53, 502–510. doi: 10.1016/S0006-3223(02)01817-1816
Luders, E., Thompson, P. M., and Toga, A. W. (2010). The development of the corpus callosum in the healthy human brain. J. Neurosci. 30, 10985–10990. doi: 10.1523/JNEUROSCI.5122-09.2010
Minzenberg, M. J., Fan, J., New, A. S., Tang, C. Y., and Siever, L. J. (2007). Fronto-limbic dysfunction in response to facial emotion in borderline personality disorder: an event-related fMRI study. Psychiatry Res. Neuroimaging 155, 231–243. doi: 10.1016/j.pscychresns.2007.03.006
Minzenberg, M. J., Fan, J., New, A. S., Tang, C. Y., and Siever, L. J. (2008). Frontolimbic structural changes in borderline personality disorder. J. Psychiatr. Res. 42, 727–733. doi: 10.1016/j.jpsychires.2007.07.015
Napadow, V., LaCount, L., Park, K., As-Sanie, S., Clauw, D. J., and Harris, R. E. (2010). Intrinsic brain connectivity in fibromyalgia is associated with chronic pain intensity. Arthritis Rheum. 62, 2545–2555. doi: 10.1002/art.27497
New, A. S., Hazlett, E. A., Buchsbaum, M. S., Goodman, M., Mitelman, S. A., Newmark, R., et al. (2007). Amygdala-prefrontal disconnection in borderline personality disorder. Neuropsychopharmacology 32, 1629–1640. doi: 10.1038/sj.npp.1301283
Niedtfeld, I., Kirsch, P., Schulze, L., Herpertz, S. C., Bohus, M., and Schmahl, C. (2012). Functional connectivity of pain-mediated affect regulation in borderline personality disorder. PLoS One 7:e33293. doi: 10.1371/journal.pone.0033293
Niedtfeld, I., Schulze, L., Kirsch, P., Herpertz, S. C., Bohus, M., and Schmahl, C. (2010). Affect regulation and pain in borderline personality disorder: a possible link to the understanding of self-injury. Biol. Psychiatry 68, 383–391. doi: 10.1016/j.biopsych.2010.04.015
Ochsner, K. N., Ray, R. D., Cooper, J. C., Robertson, E. R., Chopra, S., Gabrieli, J. D. E., et al. (2004). For better or for worse: neural systems supporting the cognitive down- and up-regulation of negative emotion. Neuroimage 23, 483–499. doi: 10.1016/j.neuroimage.2004.06.030
Perez, D. L., Vago, D. R., Pan, H., Root, J., Tuescher, O., Fuchs, B. H., et al. (2016). Frontolimbic neural circuit changes in emotional processing and inhibitory control associated with clinical improvement following transference-focused psychotherapy in borderline personality disorder. Psychiatry Clin. Neurosci. 70, 51–61. doi: 10.1111/pcn.12357
Phan, K. L., Fitzgerald, D. A., Nathan, P. J., Moore, G. J., Uhde, T. W., and Tancer, M. E. (2005). Neural substrates for voluntary suppression of negative affect: a functional magnetic resonance imaging study. Biol. Psychiatry 57, 210–219. doi: 10.1016/j.biopsych.2004.10.030
Ruocco, A. C., Amirthavasagam, S., Choi-Kain, L. W., and McMain, S. F. (2013). Neural correlates of negative emotionality in borderline personality disorder: an activation-likelihood-estimation meta-analysis. Biol. Psychiatry 73, 153–160. doi: 10.1016/j.biopsych.2012.07.014
Rüsch, N., Boeker, M., Büchert, M., Glauche, V., Bohrmann, C., Ebert, D., et al. (2010a). Neurochemical alterations in women with borderline personality disorder and comorbid attention-deficit hyperactivity disorder. World J. Biol. Psychiatry 11, 372–381. doi: 10.3109/15622970801958331
Rüsch, N., Bracht, T., Kreher, B. W., Schnell, S., Glauche, V., Il’yasov, K. A., et al. (2010b). Reduced interhemispheric structural connectivity between anterior cingulate cortices in borderline personality disorder. Psychiatry Res. - Neuroimaging 181, 151–154. doi: 10.1016/j.pscychresns.2009.08.004
Rüsch, N., Luders, E., Lieb, K., Zahn, R., Ebert, D., Thompson, P. M., et al. (2007). Corpus callosum abnormalities in women with borderline personality disorder and comorbid attention-deficit hyperactivity disorder. J. Psychiatry Neurosci. 32, 417–422.
Salvador, R., Vega, D., Pascual, J. C., Marco, J., Canales-Rodríguez, E. J., Aguilar, S., et al. (2016). Converging medial frontal resting state and diffusion-based abnormalities in borderline personality disorder. Biol. Psychiatry 79, 107–116. doi: 10.1016/j.biopsych.2014.08.026
Schulze, L., Schmahl, C., and Niedtfeld, I. (2016). Neural correlates of disturbed emotion processing in borderline personality disorder: a multimodal meta-analysis. Biol. Psychiatry 79, 97–106. doi: 10.1016/j.biopsych.2015.03.027
Shek, D. T. L. (1993). The chinese version of the state-trait anxiety inventory: its relationship to different measures of psychological well-being. J. Clin. Psychol. 49, 349–358. doi: 10.1002/1097-4679(199305)49:3<349::aid-jclp2270490308>3.0.co;2-j
Silbersweig, D., Clarkin, J. F., Goldstein, M., Kernberg, O. F., Tuescher, O., Levy, K. N., et al. (2007). Failure of frontolimbic inhibitory function in the context of negative emotion in borderline personality disorder. Am. J. Psychiatry 164, 1832–1841. doi: 10.1176/appi.ajp.2007.06010126
Smoski, M. J., Salsman, N., Wang, L., Smith, V., Lynch, T. R., Dager, S. R., et al. (2011). Functional imaging of emotion reactivity in opiate-dependent borderline personality disorder. Personal. Disord. Theory, Res. Treat. 2, 230–241. doi: 10.1037/a0022228
Soloff, P. H., Pruitt, P., Sharma, M., Radwan, J., White, R., and Diwadkar, V. A. (2012). Structural brain abnormalities and suicidal behavior in borderline personality disorder. J. Psychiatr. Res. 46, 516–525. doi: 10.1016/j.jpsychires.2012.01.003
Spreng, R. N., Mar, R. A., and Kim, A. S. N. (2009). The common neural basis of autobiographical memory, prospection, navigation, theory of mind, and the default mode: a quantitative meta-analysis. J. Cogn. Neurosci. 21, 489–510. doi: 10.1162/jocn.2008.21029
Tebartz van Elst, L., Thiel, T., Hesslinger, B., Lieb, K., Bohus, M., Hennig, J., et al. (2001). Subtle prefrontal neuropathology in a pilot magnetic resonance spectroscopy study in patients with borderline personality disorder. J. Neuropsychiatry Clin. Neurosci. 13, 511–514. doi: 10.1176/appi.neuropsych.13.4.511
Urry, H. L., Van Reekum, C. M., Johnstone, T., Kalin, N. H., Thurow, M. E., Schaefer, H. S., et al. (2006). Amygdala and ventromedial prefrontal cortex are inversely coupled during regulation of negative affect and predict the diurnal pattern of cortisol secretion among older adults. J. Neurosci. 26, 4415–4425. doi: 10.1523/JNEUROSCI.3215-05.2006
van den Bos, W., Rodriguez, C. A., Schweitzer, J. B., and McClure, S. M. (2014). Connectivity strength of dissociable striatal tracts predict individual differences in temporal discounting. J. Neurosci. 34, 10298–10310. doi: 10.1523/JNEUROSCI.4105-13.2014
Wingenfeld, K., Rullkoetter, N., Mensebach, C., Beblo, T., Mertens, M., Kreisel, S., et al. (2009). Neural correlates of the individual emotional stroop in borderline personality disorder. Psychoneuroendocrinology 34, 571–586. doi: 10.1016/j.psyneuen.2008.10.024
Wolf, R. C., Sambataro, F., Vasic, N., Schmid, M., Thomann, P. A., Bienentreu, S. D., et al. (2011). Aberrant connectivity of resting-state networks in borderline personality disorder. J. Psychiatry Neurosci. 36, 402–411. doi: 10.1503/jpn.100150
Woolrich, M. W., Jbabdi, S., Patenaude, B., Chappell, M., Makni, S., Behrens, T., et al. (2009). Bayesian analysis of neuroimaging data in FSL. Neuroimage 45, S173–S186. doi: 10.1016/j.neuroimage.2008.10.055
Xiao, J., Qiu, Y., He, Y., Cui, L., Auerbach, R. P., McWhinnie, C. M., et al. (2016). “Weakest link” as a cognitive vulnerability within the hopelessness theory of depression in chinese university students. Stress Heal. 32, 20–27. doi: 10.1002/smi.2571
Yan, C., and Zang, Y. (2010). DPARSF: a MATLAB toolbox for “pipeline” data analysis of resting-state fMRI. Front. Syst. Neurosci. 4:13. doi: 10.3389/fnsys.2010.00013
Yang, H., Lei, X., Zhong, M., Zhou, Q., Ling, Y., Jungkunz, M., et al. (2018). Psychometric properties of the Chinese version of the brief borderline symptom list in undergraduate students and clinical patients. Front. Psychol. 9:605. doi: 10.3389/fpsyg.2018.00605
Yen, S., Shea, M., and Battle, C. (2002). Traumatic exposure and posttraumatic stress disorder in borderline, schizotypal, avoidant, and obsessive-compulsive. J. Nerv Ment Dis. 190, 510–518. doi: 10.1097/01.NMD.0000026620.66764.78
Yuan, K., Yu, D., Bi, Y., Wang, R., Li, M., Zhang, Y., et al. (2017). The left dorsolateral prefrontal cortex and caudate pathway: new evidence for cue-induced craving of smokers. Hum. Brain Mapp. 38, 4644–4656. doi: 10.1002/hbm.23690
Yuan, K., Zhao, M., Yu, D., Manza, P., Volkow, N. D., Wang, G. J., et al. (2018). Striato-cortical tracts predict 12-h abstinence-induced lapse in smokers. Neuropsychopharmacology 43, 2452–2458. doi: 10.1038/s41386-018-0182-x
Zhong, M., Yi, J., Ling, Y., Zhu, X., and Yao, S. (2010). The reliability and validity of the short affect intensity scale-chinese version. J. Chin. Clin. Psychol. 18, 556–558.
Keywords: borderline personality disorder, emotion, anterior cingulate cortex, resting state functional connectivity, probabilistic fiber tracking
Citation: Lei X, Zhong M, Zhang B, Yang H, Peng W, Liu Q, Zhang Y, Yao S, Tan C and Yi J (2019) Structural and Functional Connectivity of the Anterior Cingulate Cortex in Patients With Borderline Personality Disorder. Front. Neurosci. 13:971. doi: 10.3389/fnins.2019.00971
Received: 09 January 2019; Accepted: 29 August 2019;
Published: 13 September 2019.
Edited by:
Nicoletta Cera, University of Porto, PortugalReviewed by:
Simon Maier, University of Freiburg, GermanyYanbin Jia, The First Affiliated Hospital of Jinan University, China
Roberta Rossi, IRCCS Istituto Centro San Giovanni di Dio Fatebenefratelli, Italy
Copyright © 2019 Lei, Zhong, Zhang, Yang, Peng, Liu, Zhang, Yao, Tan and Yi. This is an open-access article distributed under the terms of the Creative Commons Attribution License (CC BY). The use, distribution or reproduction in other forums is permitted, provided the original author(s) and the copyright owner(s) are credited and that the original publication in this journal is cited, in accordance with accepted academic practice. No use, distribution or reproduction is permitted which does not comply with these terms.
*Correspondence: Changlian Tan, dGFuY2hhbmdsaWFuQGNzdS5lZHUuY24=; dGFuY2hhbmdsaWFueHlAYWxpeXVuLmNvbQ==; Jinyao Yi, amlueWFveWlAY3N1LmVkdS5jbg==
†These authors have contributed equally to this work as co-first authors