- Department of Experimental Medicine, University of Perugia, Perugia, Italy
Mounting experimental evidence demonstrate that sex neuroactive steroids (neurosteroids) are essential for memory formation. Neurosteroids have a profound impact on the function and structure of neural circuits and their local synthesis is necessary for the induction of both long-term potentiation (LTP) and long-term depression (LTD) of synaptic transmission and for neural spine formation in different areas of the central nervous system (CNS). Several studies demonstrated that in the hippocampus, 17β-estradiol (E2) is necessary for inducing LTP, while 5α-dihydrotestosterone (DHT) is necessary for inducing LTD. This contribution has been proven by administering sex neurosteroids in rodent models and by using blocking agents of their synthesis or of their specific receptors. The general opposite role of sex neurosteroids in synaptic plasticity appears to be dependent on their different local availability in response to low or high frequency of synaptic stimulation, allowing the induction of bidirectional synaptic plasticity. The relevant contribution of these neurosteroids to synaptic plasticity has also been described in other brain regions involved in memory processes such as motor learning, as in the case of the vestibular nuclei, the cerebellum, and the basal ganglia, or as the emotional circuit of the amygdala. The rapid effects of sex neurosteroids on neural synaptic plasticity need the maintenance of a tonic or phasic local steroid synthesis determined by neural activity but might also be influenced by circulating hormones, age, and gender. To disclose the exact mechanisms how sex neurosteroids participate in finely tuning long-term synaptic changes and spine remodeling, further investigation is required.
Introduction
Sex neurosteroids are a group of cholesterol-derived molecules that are synthesized de novo within the central nervous system (CNS) where they are able to exert local effects (Baulieu, 1981; Baulieu and Robel, 1990). Among these, estrogens and androgens influence the general development of neural circuits, motor adaptation, learning, memory (Luine and Frankfurt, 2012), emotional behavior (Altemus, 2017), and cognition (Luine, 2014), and they are implicated in some psychiatric disorders (Bangasser and Valentino, 2014) modulating the function of different areas of the brain such as the hippocampus, the amygdala, the vestibular and cerebellar systems, and the nucleus striatum (Beauchet, 2006; Andreescu et al., 2007; Colciago et al., 2015; Bender et al., 2017; Diotel et al., 2018; Mohamad et al., 2018; Wagner et al., 2018; Dieni et al., 2020; Luine and Frankfurt, 2020).
These actions have a major role in the induction and maintenance of neural connections during development and the entire life-span by directing synaptogenesis, dendritic spine formation, and functional long-term potentiation (LTP) or long-term depression (LTD) of synaptic transmission.
Enzymes responsible for the biosynthesis of neurosteroids from cholesterol are present in the nervous system (Mensah-Nyagan et al., 1999; Giatti et al., 2019). Testosterone is synthesized through conversion of cholesterol into pregnenolone, a neurosteroid that is subsequently metabolized, leading to formation of 5α-dihydrotestosterone (DHT) or 17β-estradiol (E2) by the action of the 5α-reductase or P450 aromatase enzymes, respectively. The local concentration of these neurosteroids was found to be significantly higher in some areas of the CNS (e.g., hippocampus) in respect to blood, where they bind to specific estrogen receptors (ERα, ERβ, and multiple isoforms) or to androgen receptors (AR) (Mukai et al., 2006; Hatanaka et al., 2015). These receptors are largely distributed in the CNS and their activation can elicit diverse genomic and non-genomic cell functions.
Besides the genomic effects that occur within hours, leading to up- or downregulation of gene transcription, E2 and DHT, the most active metabolite of testosterone, can also activate rapid intracellular signaling pathways, acting within seconds or minutes via extranuclear membrane-associated forms of receptors (Kawato, 2004). In neurons, membrane effects of E2 may lead to the stimulation of different enzymes such as phospholipase C (PLC) and protein kinase C (PKC) that in turn stimulate the production of IP3 and the elevation of intracellular calcium levels. These molecules may rapidly change the function of neurons by acting as second messengers leading to the activation of further enzymatic cascades including the src kinase and the MAPK/ERK pathway (Evinger and Levin, 2005; Srivastava, 2012; Murakami et al., 2018). As in the case of estrogens, evidence has been accumulated to involve rapid responses to androgens, dependent or independent on ARs (Foradori et al., 2008). Many cellular responses to androgens are transcription independent; in fact, activated ARs are able to associate with molecular substrates in the cytoplasm and the inner layer of the cell membrane to activate intracellular kinase cascades (Soma et al., 2018).
The activation of neural network within the CNS might produce rapid behavioral changes by stimulating estrogenic or androgenic sex neurosteroid signaling and rapidly modulating the enzyme function involved in the induction of long-term synaptic plasticity. Accordingly, the consolidation of hippocampal memory might be facilitated within minutes after treatments with estrogenic and androgenic neurosteroids or with agonists of their receptors, enhancing the performance on hippocampal memory tasks, as reported in experiments performed with rats and mice (Luine et al., 2003; Aubele et al., 2008; Benice and Raber, 2009; Inagaki et al., 2010, 2012; Babanejad et al., 2012; Phan et al., 2012, 2015; Jacome et al., 2016).
Here, we will review evidence supporting the immediate involvement of the most neuroactive estrogenic and androgenic neurosteroids, namely E2 and testosterone/DHT, in the induction of neuronal synaptic plasticity, the capability of neuronal circuitries to modify their function in response to environment-triggered electric signals. The electrophysiological actions of E2 and DHT on long-term synaptic plasticity will be presented.
Neural E2 Influences Hippocampal LTP
The role of E2 acting as a neurosteroid in memory formation has been described since the late 1980s and has been recently confirmed by different research groups (Ooishi et al., 2012; Di Mauro et al., 2015; Fester and Rune, 2015; Hasegawa et al., 2015; Lu et al., 2019; Tozzi et al., 2019). These studies were guided by the major general evidence that inhibition of E2 synthase (P450 aromatase) activity produced hippocampal-related memory deficits both in women and in female rodents (Bayer et al., 2015; Tuscher et al., 2016). They also pointed out that E2, synthesized in the CNS, regulates cognition and behavior independently of gender, assigning to the neural E2 signaling system a general modulator role of CNS function (Moradpour et al., 2006; Saldanha et al., 2011; Bailey et al., 2013, 2017; Tuscher et al., 2016; Fester et al., 2017). In the hippocampus, E2-mediated synaptic activity was found to be related with memory formation and early and late influence of E2 on long-term electrophysiological synaptic effects and on dendritic spine formation has been described in animal models (Kramar et al., 2009).
The question how E2 can regulate the physiology of neurons involved in memory is still a matter of debate. E2 can directly influence the electric membrane properties of neurons or it can affect synaptic transmission, with synaptic changes suggested to be strongly correlated to functional modifications of nervous system networks. For example, E2 administration changed very rapidly the neuronal excitability and the synaptic responses of hippocampal pyramidal neurons, also triggering both short- and long-term effects on glutamate-mediated signaling (Wong and Moss, 1991, 1992). These rapid effects, occurring a few minutes after administration of this neurosteroid, strongly suggest major non-genomic mechanisms of action for E2-dependent facilitation of LTP (Warren et al., 1995; Cordoba Montoya and Carrer, 1997; Good et al., 1999). Electrophysiological recordings of hippocampal rat slices evidenced that E2 facilitation of LTP is mediated by NMDAR currents with effects both in males and females (Foy et al., 1999), as confirmed by pharmacological inhibition of the E2- and NMDA-dependent LTP using GluN2B-containing NMDA receptor antagonists (Smith and McMahon, 2005, 2006). The action of the E2-mediated modulation of NMDAR-dependent LTP was found to involve several intracellular protein kinases and membrane-associated targets, as suggested by experiments where incubation of hippocampal slices with blockers of ERs, MAPK/ERK, PKA, PKC, PI3K, NR2B, or CaMKII did not allow the induction of LTP in the presence of known E2 concentrations (Hasegawa et al., 2015). However, most of the studies exploring the influence of hippocampal E2 on synaptic memory involved experimental protocols where E2 was applied exogenously, an approach that may have limited the possibility to determine the exact source of neural E2, leaving unsolved the question whether LTP induction in physiological conditions needs the presence of the E2 neurosteroid. An important advance in the knowledge on the E2 origin and the mechanism of E2-mediated LTP was provided by studies using an electrophysiological and pharmacological approach of investigation that involved the use of drugs inhibiting the E2 synthesis or blockers of the ERs in animal models. Electrophysiological experiments aimed at investigating local mechanisms of LTP induction by using blocking agents of E2 synthesis were first performed in brain slices of male rats containing the medial vestibular nuclei (Grassi et al., 2009). Here, it has been found that the acute pharmacological inhibition of P450 aromatase with letrozole or the acute blockade of ERs (Grassi et al., 2009) with ICI 182780 (Scarduzio et al., 2013) prevented the LTP induction. This approach was then extended to the hippocampus where the role of locally synthesized E2 was demonstrated in male rats by measuring the effect of blockade of the E2 synthesis or receptors on the synaptic field potentials evoked in the CA1 region (Grassi et al., 2011; Pettorossi et al., 2013a). In fact, the LTP amplitude was markedly reduced under block of E2 synthesis or receptors, suggesting a facilitatory role of E2 on LTP. Further experiments performed in single hippocampal pyramidal neurons evidenced that in most of the neurons of the CA1 region, E2 is necessary for inducing LTP because pharmacological ER blockade fully prevented this form of synaptic plasticity (Tozzi et al., 2019). These studies also allowed to explore the role of ER subtypes on LTP induction. The use of selective ERα and ERβ inhibitors demonstrated that these ERs are both involved, as the ERα blocker MPP and the ERβ blocker PHTPP were both individually able to reduce LTP and were able to fully prevent it when applied together (Tozzi et al., 2019). The role of E2 synthesis on hippocampal LTP was further explored in male rats with experiments inhibiting the P450 aromatase activity (Tanaka and Sokabe, 2012, 2013; Hojo et al., 2015) and more recently by proving that ERα stimulation is able to induce LTP (Clements and Harvey, 2020). The role of E2 in the LTP induction has also been confirmed in mice knock-out for the P450 aromatase of both sexes, providing direct evidence that in the hippocampus, neuron-derived E2 is able to rapidly regulate the P450 aromatase-dependent Akt-ERK and CREB-BDNF signaling, essential for normal expression of LTP and synaptic plasticity (Lu et al., 2019).
The influence of E2 on LTP induction was generally observed in the hippocampus of adult male rats; however, different research groups pointed to age-dependent effects, with E2-dependent LTP observed only in young animals (Foy et al., 1999; Bi et al., 2000; Hojo et al., 2008). The influence of E2 on LTP has been also hypothesized to be gender specific. When E2 synthesis was chronically inhibited with letrozole, the LTP induction was prevented in female rats but not in males. This gender-dependent effect was paralleled by reduction of spine formation (Vierk et al., 2012; Fester and Rune, 2015). However, in a subsequent paper, the same group provided evidence that P450 aromatase inhibition by letrozole, applied acutely, prevented LTP induction both in male and female animals, confirming a more general role of E2 in hippocampal LTP (Vierk et al., 2012).
Modulation of P450 Aromatase Affects LTP Induction
The pharmacological prevention of hippocampal LTP by inhibition of the E2 synthesis or of the ERs activity suggests that E2 is required during the induction phase of LTP, when neuronal afferences are activated by electrical stimulation. P450 aromatase is expressed at hippocampal level and it has been shown that its activation depends on neuronal activity (Kimoto et al., 2001; Hojo et al., 2004, 2008; Balthazart et al., 2006). Whether the P450 aromatase activity is responsible for a tonic synthesis of local E2 or is dependent on neural phasic inputs has not been established yet. Experimental evidences show that different high- or low-frequency stimulations in the rat hippocampus may account for bidirectional synaptic plasticity, with the synthesis of estrogen and androgens directly implicated in synaptic potentiation or depression, respectively (Tozzi et al., 2019). For example, whereas the inhibition of P450 aromatase impedes LTP induced by high-frequency stimulation, the concomitant presence of exogenous E2 allows a full LTP expression in a dose-dependent manner (Di Mauro et al., 2015, 2017). Moreover, while low-frequency stimulation normally depresses synaptic transmission (LTD), in the presence of exogenous E2, it is able to induce LTP. These evidences confirm that in the rat hippocampus E2 is a key factor for eliciting LTP and suggest that different stimulating frequency patterns might modulate the P450 aromatase activity and the local E2 neo-synthesis to sustain LTP (Di Mauro et al., 2015, 2017).
Different research groups described a relation between changes of intracellular Ca2+ concentrations and P450 aromatase activity, implying the modulation of E2 synthesis (Balthazart et al., 2005; Fester et al., 2016). However, the precise mechanism by which neuronal electrical activity may lead to a Ca2+-dependent increase or decrease of E2 synthesis is still unknown. Exogenous glutamate application and K+-induced membrane depolarization have been suggested to trigger neuronal calcium-induced calcium release from intracellular Ca2+ stores, leading to enhancement of Ca2+ concentrations. This has been shown to lead to the activation of Ca2+-dependent kinases, P450 aromatase phosphorylation, and subsequent inhibition of E2 synthesis (Balthazart et al., 2001, 2003, 2005; Balthazart and Ball, 2006; Charlier et al., 2015; Fester et al., 2016). Moreover, a mechanism involving the action of extracellular E2 on NMDAR and on voltage-activated Ca2+ channels has been proposed to regulate E2 synthesis by influencing P450 aromatase activity (Zhao et al., 2005; Fester et al., 2016; Figure 1). Other investigators suggested a different scenario, describing a direct role of the NMDAR activation in the enhancement of intracellular Ca2+ with opposite effects on P450 aromatase activity. It has been reported that NMDAR activation triggers Ca2+-dependent kinases activity and increases E2 synthesis by P450 aromatase stimulation (Hojo et al., 2004; Figure 1). One possible explanation that might account for these opposite findings is the different experimental modality employed to stimulate neurons. Different glutamate receptors’ activation and velocity of Ca2+ entry into the cell may lead to opposite phosphorylation–dephosphorylation processes. The induction of LTP under the enhanced E2 availability was prevented by an inhibitor of calcium–calmodulin-dependent protein kinase II (CaMKII), suggesting that E2 is able to potentiate NMDA receptor function inducing an increase of postsynaptic Ca2+ concentration that in turn activates CaMKII leading to LTP induction (Hasegawa et al., 2015; Figure 1).
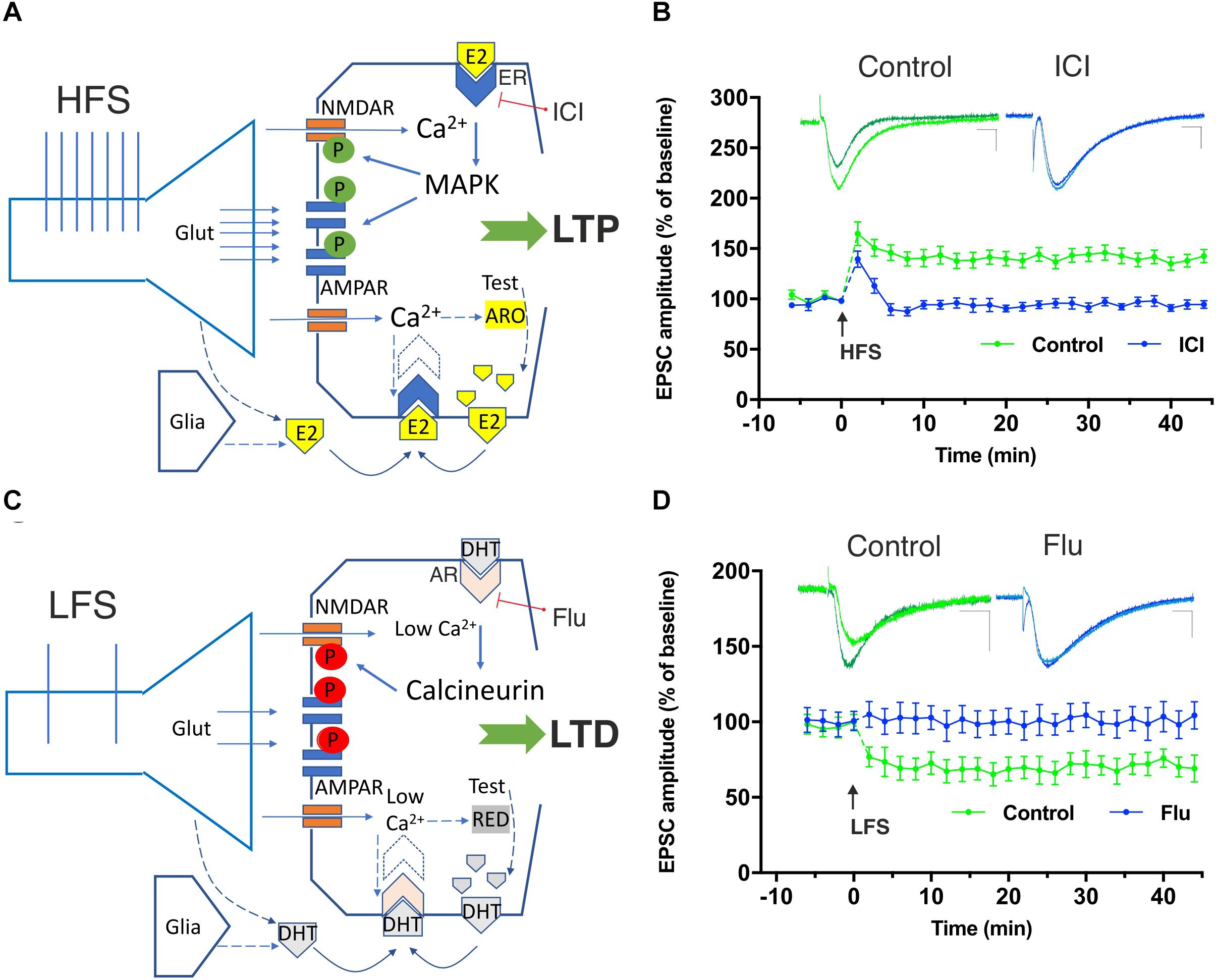
Figure 1. Involvement of neural 17β-estradiol (E2) and 5α-dihydrotestosterone (DHT), produced by testosterone (Test), in the induction of hippocampal high-frequency stimulation (HFS)–dependent long-term potentiation (LTP) and low-frequency stimulation (LFS)–dependent long-term depression (LTD). (A) The HFS-mediated glutamate (Glut) release stimulates large Ca2+ influx into dendritic spines through NMDA receptors. Ca2+ can activate calmodulin, PKC, and MAPK leading to phosphorylation (green symbols) of AMPA and NMDA glutamate receptors triggering LTP. Ca2+ is suggested to also stimulate P450 aromatase (ARO) activity increasing or decreasing the synthesis of E2, according to specific inputs to NMDARs and the Ca2+-dependent enzymes involved. After HFS, the synthesis of E2 may be locally enhanced and E2, released by presynaptic terminals and by the glia, may bind to membrane-gathered estrogen receptors (ERs) and to induce LTP through MAPK stimulation. (B) Graph showing the time course of the excitatory postsynaptic current (EPSC) amplitudes as percentage of the baseline, before and after an HFS is delivered to Schaffer collaterals in patch-clamp experiments from rat hippocampal CA1 pyramidal cells in control conditions (green time-course plot) and in the presence of the ER blocker ICI 182780 (10 μM, ICI) applied for the duration of the experiment (blue time-course plot). Note the LTP induced in control conditions but not in the presence of the ER blocking agent ICI. Superimposed representative traces showing an EPSC recorded before and 40 min after the HFS protocol in control conditions (green) and in the presence of ICI (blue). Scale bars: 10 ms; 50 pA. (C) LFS allows modest Ca2+ inflow into the postsynaptic neuron through NMDARs. Low Ca2+ levels are suggested to stimulate the 5α-reductase (RED)-dependent biosynthesis of DHT from testosterone (Lu et al., 2019). The locally synthesized DHT together with DHT released by presynaptic terminals and glia is suggested to activate membrane-gathered androgen receptors (AR) leading to the activation of calcineurin and to the dephosphorylation (red symbols) of different targets involved in LTD including the NMDAR. (D) Time-course graph of the EPSC amplitudes, before and after an LFS in control conditions (green time-course plot) and in the presence of the AR blocker flutamide (10 μM, Flu) applied for the duration of the experiment (blue time-course plot). Note the LTD induced in control conditions but not in the presence of the AR blocking agent Flu. Superimposed representative traces showing an EPSC recorded before and 40 min after the LFS protocol in control conditions (green) and in the presence of Flu (blue). Scale bars 10 ms; 50 pA. Modified by Tozzi et al. (2019).
Involvement of Neural E2 on Hippocampal LTD
The involvement of neural E2 on the scaling down of synaptic transmission, as in the case of LTD, has also been explored because the ability of E2 to modify synaptic plasticity via long-term depression may be an additional mechanism by which E2 can enhance learning and memory. In fact, E2 might act to improve memory by suppressing forgetting via an LTD-based mechanism.
Brain aging is generally associated to a decreased ability of memory processing and, in aged rats, to a facilitated hippocampal LTD. Accordingly, LTD amplitude was found to be larger in aged rats than in adults, and interestingly, treatments with E2 was reported to prevent this enhanced LTD in these aged animals but not in adults (Foy, 2001). Hippocampal LTD can be elicited by modest Ca2+ inflows entering into neurons via NMDA or metabotropic group I glutamate (mGluI) receptors (Shiroma et al., 2005). Interestingly, it has been found that the threshold for the induction of the NMDAR-dependent LTD could be enhanced or lowered by the stimulation of ERα or ERβ, respectively, confirming the role of ERs on LTD induction (Ooishi et al., 2012). Moreover, experiments also using ERα- and ERβ-knock-out mice confirmed that E2 may be able to rapidly enhance hippocampal LTD, presumably by a NMDAR- and ERα-dependent mechanism of action (Mukai et al., 2007; Murakami et al., 2015). However, electrophysiological experiments conducted in the presence of inhibitors of the E2 synthesizing enzyme or of the ERs suggested a minor role of E2 in hippocampal LTD because in the presence of these drugs, hippocampal LTD of male adult rats remained unchanged (Pettorossi et al., 2013a; Di Mauro et al., 2015, 2017).
Androgens Affect Hippocampal LTP and LTD
It is well known that testosterone and its more active metabolite DHT attenuate mild cognitive impairment in men, suggesting a role of androgens in sustaining synaptic memory (Hogervorst et al., 2004; Pike et al., 2006; Kang et al., 2014; Pan et al., 2016). The concentration of these neurosteroids appears to be positively related with hippocampal synaptic spine density, suggesting that androgens are required for hippocampus-related cognitive performances (Moffat et al., 2002; Ooishi et al., 2012). The effects of androgens on the induction of hippocampal LTP and LTD has been recently examined by using drugs able to inhibit ARs or the 5α-reductase, the enzyme catalyzing the conversion of testosterone into DHT. Experimental findings demonstrated that in the rat CA1 hippocampal area, LTD is fully prevented under pharmacological blockade of either the AR or the 5α-reductase. These pharmacological actions, however, were unable to influence LTP in the same brain region and suggest that in the hippocampus, androgens sustain LTD but not LTP induction (Grassi et al., 2011; Grassi et al., 2013; Pettorossi et al., 2013a; Di Mauro et al., 2015; Di Mauro et al., 2017; Tozzi et al., 2019; Figure 1). Accordingly, ARs were found to be expressed in the hippocampus at the postsynaptic level (Tabori et al., 2005; Hatanaka et al., 2009), implying that ARs may participate in androgen-induced LTD.
Because testosterone may be converted either into E2 or DHT, depending on the prevalence, respectively, of the P450 aromatase or 5α-reductase synthesizing activity, it is suggested that the modulation of these biosynthetic pathways might in turn promote LTP or LTD in neurons (Di Mauro et al., 2015, 2017). Moreover, it is possible that the conversion of testosterone into DHT might limit the E2 neo-synthesis and the consequent possibility to induce LTP. Moreover, it has been hypothesized that a specific frequency of neuronal stimulation might drive hippocampal metabolism of testosterone toward conversion into E2 or DHT to sustain, respectively, LTP or LTD induction (Di Mauro et al., 2015).
Long-term depression in neurons may be based on different mechanisms, most of them implying an NMDAR-, mGluR- or endocannabinoid-dependent signaling. Ca2+ is suggested to play a role in LTD entering into neurons by glutamate receptors such as NMDARs during synaptic electric stimulations. Low-frequency stimulations (LFS) would produce low Ca2+ increases through NMDARs in the postsynaptic dendritic spines (Figure 1). After Ca2+-calmodulin formation, sequential activation of protein phosphatase 2B (calcineurin), dephosphorylation of inhibitor-1, activation of protein phosphatase 1 (PP1), and dephosphorylation of ser845 on the AMPAR subunit GluA1 would lead to internalization of AMPARs from the synapse, changes of the conductance properties of these receptors ultimately inducing LTD (Mulkey et al., 1994). However, how androgens are involved in LTD induction has been poorly investigated. It has been proposed that LFS-induced LTD is established in the hippocampus via DHT binding to synaptic ARs on delivery of LFS, leading to calcineurin activation and NMDAR suppression, resulting in a decreased presence or dephosphorylation of AMPARs (Hasegawa et al., 2015).
Estrogenic and Androgenic Sex Neurosteroids Affect Vestibular LTP and LTD
The vestibular system is responsible for stabilizing the eyes and the body in space and is crucial for self-motion perception. It is involved in several plastic phenomena like the visuo-vestibular calibration (Lisberger and Miles, 1980), the vestibular compensation (Smith and Curthoys, 1989; Dutia, 2010), and the responsiveness to intense stimulation (Massot et al., 2012; Pettorossi et al., 2013b). Because LTP and LTD expression have been demonstrated in the vestibular nuclei and are likely involved in these adaptive responses (Grassi et al., 1995), the contribution of sex neurosteroids has been explored in medial vestibular nucleus (MVN).
Medial vestibular nucleus neurons express the E2 synthesizing enzyme P450 aromatase and both estrogen and androgen receptors. Specifically, immunoreactivity for ERα, ERβ, and AR have been found in these neurons, most of them co-localizing ERβ and AR (Grassi et al., 2013). Electrophysiological studies in rat MVN neurons showed that E2 affects synaptic transmission and neuronal excitability facilitating both the LTP of the primary vestibular afferents and the intrinsic membrane excitability (Grassi et al., 2007, 2009, 2010). Specifically, during LTP, E2 depresses the spontaneous action potential discharge in both regular (A type) and irregular (B type) discharging neurons, while the synaptic response to vestibular nerve stimulation is increased in B type neurons with a net effect of enhancement of the signal-to-noise ratio of synaptic response in these neurons, relative to resting activity of all MVN neurons. These combined effects may be necessary to specifically enhance the dynamic properties of neuronal activation of vestibular circuits. Interestingly, pharmacological inhibition of E2 synthesis by letrozole or antagonism of ERs by ICI 182780 was reported not only to prevent the induction of LTP but also to unmask LTD of synaptic plasticity, suggesting that the level of neural E2 is a key modulator of MVN neurons’ synaptic plasticity, being able to shift LTP into LTD according to the local availability of this neurosteroid (Grassi et al., 2009, 2010).
Because MVN neurons have been reported to also express androgens, it is plausible that androgens play an important role in LTD induction of synaptic plasticity also in MVN, as demonstrated in other brain regions such as the hippocampus. In fact, it has been shown that the pharmacological antagonism of ARs abolishes this form of synaptic plasticity in MVN neurons, as observed by electrophysiological recordings (Scarduzio et al., 2012, 2013). However, differently from what was observed in the hippocampus, the reduction of DHT synthesis from testosterone by pharmacological inhibition of 5α-reductase did not affect LTD, suggesting a more direct effect of testosterone and/or a greater sensitivity of MVN neurons to this neurosteroid with respect to DHT (Scarduzio et al., 2012, 2013).
Neural E2 Affects Cerebellar LTP and LTD
The cerebellum participates with the vestibular system to most of the adaptation observed in the gaze stability with multiple synaptic plasticity mechanisms (Lisberger and Miles, 1980). In particular, together with the vestibular nuclei, it is responsible for the vestibulo-ocular reflex calibration (Hogervorst et al., 2004). In this encephalic structure, the encoding gain increase and decrease adaptation is thought to be mediated by LTP occurring at the parallel fiber–Purkinje cell synapses (PF-LTP) and by LTD (PF-LTD) at the same or different synapse subset, respectively (Hansel et al., 2001; Boyden and Raymond, 2003; Boyden et al., 2004; Coesmans et al., 2004; Broussard et al., 2011).
The cerebellum expresses ERs, ARs, and the synthesizing enzymes for E2 and androgens (Sakamoto et al., 2003; Tsutsui et al., 2011; Hedges et al., 2012). The first evidence of the influence of neural E2 in cerebellar learning has been provided by a study in which synaptic plasticity at the Purkinje cell and the VOR adaptation was examined in ovariectomized mice, in ERβ knock-out female mice, and in male mice, after the administration of E2 (Andreescu et al., 2007). These authors found that E2 had relevant impact on the expression of VOR gain-down adaptation and regulated cerebellar synaptic plasticity influencing PF-LTP. First, it was shown that E2 enhanced the LTP amplitude at the PF–Purkinje cell synapse, while leaving LTD unaffected. Second, in Purkinje cells, ERβ activation by E2 significantly improved the gain-decrease adaptation of the VOR (Andreescu et al., 2007). In a subsequent study, the impact of E2 in the cerebellar synaptic plasticity was examined by pharmacological inhibition of E2 synthesis in male rats both in vitro at the PF–Purkinje cell synapses and in vivo evaluating the VOR adaptation (Dieni et al., 2018a,b). The application of the P450 aromatase inhibitor letrozole in the flocculus of cerebellar slices prevented the PF-LTP without affecting the PF-LTD impeding the adaptive gain reduction of the VOR. Together with the sex neurosteroid-mediated bidirectional vestibular synaptic plasticity, the cerebellum participates in the visuo-vestibular calibration of the VOR. It is likely that E2 facilitates the gain-increase of VOR by acting at the level of the vestibular system and the gain-down regulation by acting at the level of the cerebellum (Dieni et al., 2018a,b).
Neural E2 Affects Striatal LTP
Neural E2 exerts an important role in LTP induction in the nucleus striatum with E2 receptors diffusely expressed in the basal ganglia (Creutz and Kritzer, 2004; Krentzel et al., 2019). Electrophysiological experiments performed in neurons of the dorsal striatum of the male rat showed that E2 synthesis and ER activation are required for the induction of LTP in both spiny projection neurons (SPNs) and cholinergic interneurons because the pharmacological inhibition of P450 aromatase or antagonism of ERs completely prevented the LTP induction of SPNs with no effect on LTD or synaptic depotentiation (Tozzi et al., 2015). Because striatal dopamine (DA) release is critical for LTP induction in this brain structure (Calabresi et al., 2007) and based on the evidence that striatal LTP depends on E2 local synthesis, the interaction between E2 and DA in controlling SPNs’ LTP was explored. Tozzi et al. (2015) suggested that the E2 and DA signaling systems converge on the stimulation of the cAMP–PKA intracellular pathway to facilitate LTP induction in striatal neurons via a cooperation between the D1 DA receptor and the ERs (Tozzi et al., 2015). These findings were also supported by experiments showing a possible facilitatory influence of E2 in the dorsal striatum where DA release has been demonstrated to be potentiated by E2 (Song et al., 2019).
Neural E2 Affects LTP in the Amygdala
17β-estradiol is suggested to play a role in synaptic plasticity also in the amygdala, a brain structure where the presence of P450 aromatase has been reported (Zhao et al., 2007; Bender et al., 2017). The amygdala is considered a core nucleus of “emotional” memory and for the responses to emotion (Pape and Pare, 2010). Accordingly, dysfunction of its neuronal networks is implicated in pathological conditions such as depression and post-traumatic stress disorder (Tovote et al., 2015). Depression-like symptoms have been observed in women under treatment with P450 aromatase inhibitors providing evidence of the importance of the E2 signaling system in the amygdala-related physiology (Gallicchio et al., 2012).
In a recent study, Bender and colleagues (Bender et al., 2017) explored the possible influence of the E2 in the LTP induction of neurons of the basolateral amygdala, a brain region characterized by important synaptic plasticity and by the presence of P450 aromatase both in male and female rodents. Here, the authors found that, beside the effect on the spine density, pharmacological inhibition of P450 aromatase prevented the LTP induction in amygdala slices of female rodents but not in males. The gender-specific role of E2 in LTP of amygdala neurons points to the importance of conducting further studies in the field to better understand the sex-related differences observed in mood disorders and to take into account the side effects of P450 aromatase inhibitors.
The Role of Circulating Hormones
Whether circulating sex hormones affect the long-term synaptic changes induced by electrical neuronal activity in the brain is an intriguing possibility. E2 synthesized in the CNS may be affected by the estrous cycle in rodent, as reported in the rat hippocampus where neural E2 levels changed according to different phases of the cycle (Kato et al., 2013). Neural E2 changes were suggested to result by indirect access of fluctuating hematic progesterone into the brain and its subsequent conversion into E2 (Kato et al., 2013) or by changes of the phosphorylation status of P450 aromatase depending on fluctuation of different kinases related to synaptic plasticity (Balthazart and Ball, 2006; Hojo and Kawato, 2018). The resulting effect of neural E2 fluctuations appeared to be correlated in the rat hippocampus with the induction of LTP or LTD together with changes of dendritic spine density. In fact, it has been shown that the LTP amplitude changes are depending on the estrum phase in the hippocampus (Warren et al., 1995). Subsequent comparative studies on the occurrence and amplitude of LTP were performed in vestibular neurons of male and female rats (Pettorossi et al., 2011). Specifically, while HFS protocol was able to consistently induce LTP in male rats within seconds (fast-developing LTP), in females HFS protocol produced fast and slow LTP or even LTD. The amplitude and occurrence of LTP depended on the estrous phases, with minor probability to induce fast LTP in proestrus (Pettorossi et al., 2011). This effect might depend on the marked structural changes of the neurons during the estrous cycle, but it might also be a result of the influence of circulating hormones in the synthesis of the neurosteroids. It has been found, in fact, that the acute administration of testosterone that induces LTP in male can induce LTD in female only during the proestrus phase (Grassi et al., 2012). This suggests that in the presence of high levels of circulating E2 (proestrus), the conversion of testosterone into E2 is inhibited, whereas the synthesis of DHT is facilitated.
The interesting data obtained by analyzing the long-term responses in vestibular nuclei prompt to extend this study to the hippocampus by using a similar approach for understanding how memory and learning could be correlated with the expression of the neural synaptic plasticity. Changes in the thresholds of the induction of long-term phenomena might vary with behavioral changes, as reported in EEG experiments in women (Sumner et al., 2018).
Dendritic Spine Remodeling Is Associated to Hippocampal E2-Dependent LTP
Most of the studies on the role of sex neurosteroids in hippocampal synaptic plasticity demonstrated that E2 facilitates LTP induction and enhances dendritic spine formation. E2 has been reported to increase apical dendritic spine formation in projections that are closed to presynaptic terminals on hippocampal CA1 pyramidal cells. It has been reported that this action is achieved by activation of genomic ERs, most likely the ERα (Gould et al., 1990; Woolley et al., 1990, 1996; Woolley and McEwen, 1993; Murphy and Segal, 1996; Woolley, 1998; Hao et al., 2003; MacLusky et al., 2005). Accordingly, ERs activation is able to either decrease GABAergic inhibition (Murphy et al., 1998; Rudick and Woolley, 2001) and increase NMDAR expression and function (Weiland, 1992; Warren et al., 1995; Gazzaley et al., 1996; Cordoba Montoya and Carrer, 1997; Woolley et al., 1997; Good et al., 1999; Cyr et al., 2000, 2001; Bi et al., 2001; Rudick and Woolley, 2001), ultimately increasing the spine density of neurons. Hippocampal LTP have in fact been correlated to the simultaneous increase of both the spine density and the NMDAR-dependent synaptic transmission (Smith and McMahon, 2005, 2006), suggesting that E2 during LTP formation is able to trigger both morphological and functional changes. Synaptic changes induced by E2 during LTP were reported to affect the structure of neuronal circuitry by increasing the polymerization of filamentous actin proteins in the dendritic spines (Kramar et al., 2009) and by rapidly enhancing their head structure and density via activation of ERα, but not ERβ (Ooishi et al., 2012).
The morphological and electrophysiological changes triggered by E2 during LTP may be part of the same E2-dependent mechanism. Synergic effects consisting of formation of new spines and stabilization of synaptic strength in active mature synapses have in fact been reported to be necessary for long-term synaptic consolidation of neurotransmission (Toni et al., 1999; Yang et al., 2008). However, an alternative possibility considers the functional and structural events happening during E2-dependent LTP formation as two distinct phenomena, according to experiments of acute or chronic inhibition of E2 synthesis (Vierk et al., 2012; Fester and Rune, 2015).
Overall, even if it is possible to hypothesize that initial structural changes of dendritic spines induced by E2 precede a full LTP induction, the idea that neural E2 is able to trigger LTP more rapidly than any possible change of synaptic structure is likely to occur. In this scenario, the induction of LTP of synaptic transmission, involving the increased synthesis or availability of E2 during neuronal activity, might represent the early phase in the process that allows subsequent spine formation and consolidation (Yang et al., 2008; Kato et al., 2013; Dickens et al., 2014).
Interestingly, neural androgens may also increase spine structure suggesting that the machinery at the basis of synaptic plasticity needs structural improvement either for the induction of the E2-mediated LTP and for the induction of androgen-mediated LTD (Leranth et al., 2004; Hatanaka et al., 2009).
Thus, sex neurosteroids are suggested to influence long-term synaptic plasticity by complex mechanisms of action, with estrogens and androgens producing opposite effects on LTP and LTD induction, respectively, and both of them being responsible for the enhancement of dendritic spine formation. This reveals a multifaceted mechanism at the base of neurosteroid influence in synaptic plasticity. In fact, estrogens and androgens have opposite effects in the long-term synaptic events, being the LTP induced by E2 and the LTD by androgens, whereas both neurosteroids enhance dendritic spine formation.
Beside their contribution in synaptic plasticity, the evidence reported in this review of the literature underly the involvement of these molecules in the regulation of several behavioral aspects that are related to the motor system function, to emotional manifestations and cognition.
Author Contributions
AT and VP conceived and wrote the manuscript. LB reviewed the manuscript. All authors contributed to the figure preparation and critically revised the manuscript.
Conflict of Interest
The authors declare that the research was conducted in the absence of any commercial or financial relationships that could be construed as a potential conflict of interest.
Acknowledgments
The support of a Telethon Italy grant (GGP16131) to AT was gratefully acknowledged.
References
Altemus, M. (2017). Neuroendocrine networks and functionality. Psychiatr. Clin. North Am. 40, 189–200. doi: 10.1016/j.psc.2017.01.008
Andreescu, C. E., Milojkovic, B. A., Haasdijk, E. D., Kramer, P., De Jong, F. H., Krust, A., et al. (2007). Estradiol improves cerebellar memory formation by activating estrogen receptor beta. J. Neurosci. 27, 10832–10839. doi: 10.1523/JNEUROSCI.2588-07.2007
Aubele, T., Kaufman, R., Montalmant, F., and Kritzer, M. F. (2008). Effects of gonadectomy and hormone replacement on a spontaneous novel object recognition task in adult male rats. Horm. Behav. 54, 244–252. doi: 10.1016/j.yhbeh.2008.04.001
Babanejad, S., Naghdi, N., and Haeri Rohani, S. A. (2012). Microinjection of Dihydrotestosterone as a 5α-Reduced Metabolite of Testosterone into CA1 Region of Hippocampus Could Improve Spatial Learning in the Adult Male Rats. Iran. J. Pharm. Res. 11, 661–669.
Bailey, D. J., Ma, C., Soma, K. K., and Saldanha, C. J. (2013). Inhibition of hippocampal aromatization impairs spatial memory performance in a male songbird. Endocrinology 154, 4707–4714. doi: 10.1210/en.2013-1684
Bailey, D. J., Makeyeva, Y. V., Paitel, E. R., Pedersen, A. L., Hon, A. T., Gunderson, J. A., et al. (2017). Hippocampal aromatization modulates spatial memory and characteristics of the synaptic membrane in the male zebra finch. Endocrinology 158, 852–859. doi: 10.1210/en.2016-1692
Balthazart, J., Baillien, M., and Ball, G. F. (2001). Phosphorylation processes mediate rapid changes of brain aromatase activity. J. Steroid Biochem. Mol. Biol. 79, 261–277. doi: 10.1016/s0960-0760(01)00143-1
Balthazart, J., Baillien, M., and Ball, G. F. (2006). Rapid control of brain aromatase activity by glutamatergic inputs. Endocrinology 147, 359–366. doi: 10.1210/en.2005-0845
Balthazart, J., Baillien, M., Charlier, T. D., and Ball, G. F. (2003). Calcium-dependent phosphorylation processes control brain aromatase in quail. Eur. J. Neurosci. 17, 1591–1606. doi: 10.1046/j.1460-9568.2003.02598.x
Balthazart, J., Baillien, M., Charlier, T. D., and Ball, G. F. (2005). Effects of calmodulin on aromatase activity in the preoptic area. J. Neuroendocrinol. 17, 664–671. doi: 10.1111/j.1365-2826.2005.01355.x
Balthazart, J., and Ball, G. F. (2006). Is brain estradiol a hormone or a neurotransmitter? Trends Neurosci. 29, 241–249. doi: 10.1016/j.tins.2006.03.004
Bangasser, D. A., and Valentino, R. J. (2014). Sex differences in stress-related psychiatric disorders: neurobiological perspectives. Front. Neuroendocrinol. 35, 303–319. doi: 10.1016/j.yfrne.2014.03.008
Baulieu, E. E. (1981). “Steroid hormone in the brain: several mechanism?” in Steroid Hormone Regulation of the Brain, eds K. Fuxe, J. A. Gustafsson, and L. Wetterberg, (Oxford: Pergamon), 3–14.
Baulieu, E. E., and Robel, P. (1990). Neurosteroids: A new brain function? J. Steroid Biochem. Mol. Biol. 37, 395–403. doi: 10.1016/0960-0760(90)90490-c
Bayer, J., Rune, G., Schultz, H., Tobia, M. J., Mebes, I., Katzler, O., et al. (2015). The effect of estrogen synthesis inhibition on hippocampal memory. Psychoneuroendocrinology 56, 213–225. doi: 10.1016/j.psyneuen.2015.03.011
Beauchet, O. (2006). Testosterone and cognitive function: current clinical evidence of a relationship. Eur. J. Endocrinol. 155, 773–781. doi: 10.1530/eje.1.02306
Bender, R. A., Zhou, L., Vierk, R., Brandt, N., Keller, A., Gee, C. E., et al. (2017). Sex-dependent regulation of aromatase-mediated synaptic plasticity in the basolateral amygdala. J. Neurosci. 37, 1532–1545. doi: 10.1523/JNEUROSCI.1532-16.2016
Benice, T. S., and Raber, J. (2009). Dihydrotestosterone modulates spatial working-memory performance in male mice. J. Neurochem. 110, 902–911. doi: 10.1111/j.1471-4159.2009.06183.x
Bi, R., Broutman, G., Foy, M. R., Thompson, R. F., and Baudry, M. (2000). The tyrosine kinase and mitogen-activated protein kinase pathways mediate multiple effects of estrogen in hippocampus. Proc. Natl. Acad. Sci. U.S.A. 97, 3602–3607. doi: 10.1073/pnas.060034497
Bi, R., Foy, M. R., Vouimba, R. M., Thompson, R. F., and Baudry, M. (2001). Cyclic changes in estradiol regulate synaptic plasticity through the MAP kinase pathway. Proc. Natl. Acad. Sci. U.S.A. 98, 13391–13395. doi: 10.1073/pnas.241507698
Boyden, E. S., Katoh, A., and Raymond, J. L. (2004). Cerebellum-dependent learning: the role of multiple plasticity mechanisms. Annu. Rev. Neurosci. 27, 581–609. doi: 10.1146/annurev.neuro.27.070203.144238
Boyden, E. S., and Raymond, J. L. (2003). Active reversal of motor memories reveals rules governing memory encoding. Neuron 39, 1031–1042. doi: 10.1016/s0896-6273(03)00562-2
Broussard, D. M., Titley, H. K., Antflick, J., and Hampson, D. R. (2011). Motor learning in the VOR: the cerebellar component. Exp. Brain Res. 210, 451–463. doi: 10.1007/s00221-011-2589-z
Calabresi, P., Picconi, B., Tozzi, A., and Di Filippo, M. (2007). Dopamine-mediated regulation of corticostriatal synaptic plasticity. Trends Neurosci. 30, 211–219. doi: 10.1016/j.tins.2007.03.001
Charlier, T. D., Cornil, C. A., Patte-Mensah, C., Meyer, L., Mensah-Nyagan, A. G., and Balthazart, J. (2015). Local modulation of steroid action: rapid control of enzymatic activity. Front. Neurosci. 9:83. doi: 10.3389/fnins.2015.00083
Clements, L., and Harvey, J. (2020). Activation of oestrogen receptor alpha induces a novel form of LTP at hippocampal temporoammonic-CA1 synapses. Br. J. Pharmacol. 177, 642–655. doi: 10.1111/bph.14880
Coesmans, M., Weber, J. T., De Zeeuw, C. I., and Hansel, C. (2004). Bidirectional parallel fiber plasticity in the cerebellum under climbing fiber control. Neuron 44, 691–700. doi: 10.1016/j.neuron.2004.10.031
Colciago, A., Casati, L., Negri-Cesi, P., and Celotti, F. (2015). Learning and memory: steroids and epigenetics. J. Steroid Biochem. Mol. Biol. 150, 64–85. doi: 10.1016/j.jsbmb.2015.02.008
Cordoba Montoya, D. A., and Carrer, H. F. (1997). Estrogen facilitates induction of long term potentiation in the hippocampus of awake rats. Brain Res. 778, 430–438. doi: 10.1016/s0006-8993(97)01206-7
Creutz, L. M., and Kritzer, M. F. (2004). Mesostriatal and mesolimbic projections of midbrain neurons immunoreactive for estrogen receptor beta or androgen receptors in rats. J. Comp. Neurol. 476, 348–362. doi: 10.1002/cne.20229
Cyr, M., Ghribi, O., and Di Paolo, T. (2000). Regional and selective effects of oestradiol and progesterone on NMDA and AMPA receptors in the rat brain. J. Neuroendocrinol. 12, 445–452. doi: 10.1046/j.1365-2826.2000.00471.x
Cyr, M., Ghribi, O., Thibault, C., Morissette, M., Landry, M., and Di Paolo, T. (2001). Ovarian steroids and selective estrogen receptor modulators activity on rat brain NMDA and AMPA receptors. Brain Res. Brain Res. Rev. 37, 153–161. doi: 10.1016/s0165-0173(01)00115-1
Di Mauro, M., Tozzi, A., Calabresi, P., Pettorossi, V. E., and Grassi, S. (2015). Neo-synthesis of estrogenic or androgenic neurosteroids determine whether long-term potentiation or depression is induced in hippocampus of male rat. Front. Cell. Neurosci. 9:376. doi: 10.3389/fncel.2015.00376
Di Mauro, M., Tozzi, A., Calabresi, P., Pettorossi, V. E., and Grassi, S. (2017). Different synaptic stimulation patterns influence the local androgenic and estrogenic neurosteroid availability triggering hippocampal synaptic plasticity in the male rat. Eur. J. Neurosci. 45, 499–509. doi: 10.1111/ejn.13455
Dickens, M. J., de Bournonville, C., Balthazart, J., and Cornil, C. A. (2014). Relationships between rapid changes in local aromatase activity and estradiol concentrations in male and female quail brain. Horm. Behav. 65, 154–164. doi: 10.1016/j.yhbeh.2013.12.011
Dieni, C. V., Contemori, S., Biscarini, A., and Panichi, R. (2020). De novo synthesized estradiol: a role in modulating the cerebellar function. Int. J. Mol. Sci. 21:3316. doi: 10.3390/ijms21093316
Dieni, C. V., Ferraresi, A., Sullivan, J. A., Grassi, S., Pettorossi, V. E., and Panichi, R. (2018a). Acute inhibition of estradiol synthesis impacts vestibulo-ocular reflex adaptation and cerebellar long-term potentiation in male rats. Brain Struct. Funct. 223, 837–850. doi: 10.1007/s00429-017-1514-z
Dieni, C. V., Sullivan, J. A., Faralli, M., Contemori, S., Biscarini, A., Pettorossi, V. E., et al. (2018b). 17 beta-estradiol synthesis modulates cerebellar dependent motor memory formation in adult male rats. Neurobiol. Learn. Mem. 155, 276–286. doi: 10.1016/j.nlm.2018.08.011
Diotel, N., Charlier, T. D., Lefebvre, d’Hellencourt, C., Couret, D., Trudeau, V. L., et al. (2018). Steroid transport, local synthesis, and signaling within the brain: roles in neurogenesis, neuroprotection, and sexual behaviors. Front. Neurosci. 12:84. doi: 10.3389/fnins.2018.00084
Dutia, M. B. (2010). Mechanisms of vestibular compensation: recent advances. Curr. Opin. Otolaryngol. Head Neck Surg. 18, 420–424. doi: 10.1097/MOO.0b013e32833de71f
Evinger, A. J. III, and Levin, E. R. (2005). Requirements for estrogen receptor alpha membrane localization and function. Steroids 70, 361–363. doi: 10.1016/j.steroids.2005.02.015
Fester, L., Brandt, N., Windhorst, S., Prols, F., Blaute, C., and Rune, G. M. (2016). Control of aromatase in hippocampal neurons. J. Steroid Biochem. Mol. Biol. 160, 9–14. doi: 10.1016/j.jsbmb.2015.10.009
Fester, L., and Rune, G. M. (2015). Sexual neurosteroids and synaptic plasticity in the hippocampus. Brain Res. 1621, 162–169. doi: 10.1016/j.brainres.2014.10.033
Fester, L., Zhou, L., Ossig, C., Labitzke, J., Blaute, C., Bader, M., et al. (2017). Synaptopodin is regulated by aromatase activity. J. Neurochem. 140, 126–139. doi: 10.1111/jnc.13889
Foradori, C. D., Weiser, M. J., and Handa, R. J. (2008). Non-genomic actions of androgens. Front. Neuroendocrinol. 29:169–181. doi: 10.1016/j.yfrne.2007.10.005
Foy, M. R. (2001). 17beta-estradiol: effect on CA1 hippocampal synaptic plasticity. Neurobiol. Learn. Mem. 76, 239–252. doi: 10.1006/nlme.2001.4018
Foy, M. R., Xu, J., Xie, X., Brinton, R. D., Thompson, R. F., and Berger, T. W. (1999). 17beta-estradiol enhances NMDA receptor-mediated EPSPs and long-term potentiation. J. Neurophysiol. 81, 925–929. doi: 10.1152/jn.1999.81.2.925
Gallicchio, L., MacDonald, R., Wood, B., Rushovich, E., and Helzlsouer, K. J. (2012). Menopausal-type symptoms among breast cancer patients on aromatase inhibitor therapy. Climacteric 15, 339–349. doi: 10.3109/13697137.2011.620658
Gazzaley, A. H., Weiland, N. G., McEwen, B. S., and Morrison, J. H. (1996). Differential regulation of NMDAR1 mRNA and protein by estradiol in the rat hippocampus. J. Neurosci. 16, 6830–6838.
Giatti, S., Garcia-Segura, L. M., Barreto, G. E., and Melcangi, R. C. (2019). Neuroactive steroids, neurosteroidogenesis and sex. Prog. Neurobiol. 176, 1–17. doi: 10.1016/j.pneurobio.2018.06.007
Good, M., Day, M., and Muir, J. L. (1999). Cyclical changes in endogenous levels of oestrogen modulate the induction of LTD and LTP in the hippocampal CA1 region. Eur. J. Neurosci. 11, 4476–4480. doi: 10.1046/j.1460-9568.1999.00920.x
Gould, E., Woolley, C. S., Frankfurt, M., and McEwen, B. S. (1990). Gonadal steroids regulate dendritic spine density in hippocampal pyramidal cells in adulthood. J. Neurosci. 10, 1286–1291.
Grassi, S., Della Torre, G., Capocchi, G., Zampolini, M., and Pettorossi, V. E. (1995). The role of GABA in NMDA-dependent long term depression (LTD) of rat medial vestibular nuclei. Brain Res. 699, 183–191. doi: 10.1016/0006-8993(95)00895-w
Grassi, S., Frondaroli, A., Dieni, C., Dutia, M. B., and Pettorossi, V. E. (2007). Neurosteroid modulation of neuronal excitability and synaptic transmission in the rat medial vestibular nuclei. Eur J. Neurosci. 26, 23–32. doi: 10.1111/j.1460-9568.2007.05645.x
Grassi, S., Frondaroli, A., Dieni, C., Scarduzio, M., and Pettorossi, V. E. (2009). Long-term potentiation in the rat medial vestibular nuclei depends on locally synthesized 17beta-estradiol. J. Neurosci. 29, 10779–10783. doi: 10.1523/JNEUROSCI.1697-09.2009
Grassi, S., Frondaroli, A., Scarduzio, M., Dieni, C. V., Brecchia, G., Boiti, C., et al. (2012). Influence of sex and estrous cycle on synaptic responses of the medial vestibular nuclei in rats: role of circulating 17beta-estradiol. Brain Res. Bull. 87, 319–327. doi: 10.1016/j.brainresbull.2011.11.008
Grassi, S., Frondaroli, A., Scarduzio, M., Dutia, M. B., Dieni, C., and Pettorossi, V. E. (2010). Effects of 17beta-estradiol on glutamate synaptic transmission and neuronal excitability in the rat medial vestibular nuclei. Neuroscience 165, 1100–1114. doi: 10.1016/j.neuroscience.2009.11.039
Grassi, S., Scarduzio, M., Panichi, R., Dall’Aglio, C., Boiti, C., and Pettorossi, V. E. (2013). Opposite long-term synaptic effects of 17beta-estradiol and 5alpha-dihydrotestosterone and localization of their receptors in the medial vestibular nucleus of rats. Brain Res. Bull. 97, 1–7. doi: 10.1016/j.brainresbull.2013.05.006
Grassi, S., Tozzi, A., Costa, C., Tantucci, M., Colcelli, E., Scarduzio, M., et al. (2011). Neural 17beta-estradiol facilitates long-term potentiation in the hippocampal CA1 region. Neuroscience 192, 67–73. doi: 10.1016/j.neuroscience.2011.06.078
Hansel, C., Linden, D. J., and D’Angelo, E. (2001). Beyond parallel fiber LTD: the diversity of synaptic and non-synaptic plasticity in the cerebellum. Nat. Neurosci. 4, 467–475. doi: 10.1038/87419
Hao, J., Janssen, W. G., Tang, Y., Roberts, J. A., McKay, H., Lasley, B., et al. (2003). Estrogen increases the number of spinophilin-immunoreactive spines in the hippocampus of young and aged female rhesus monkeys. J. Comp. Neurol. 465, 540–550. doi: 10.1002/cne.10837
Hasegawa, Y., Hojo, Y., Kojima, H., Ikeda, M., Hotta, K., Sato, R., et al. (2015). Estradiol rapidly modulates synaptic plasticity of hippocampal neurons: Involvement of kinase networks. Brain Res. 1621, 147–161. doi: 10.1016/j.brainres.2014.12.056
Hatanaka, Y., Hojo, Y., Mukai, H., Murakami, G., Komatsuzaki, Y., Kim, J., et al. (2015). Rapid increase of spines by dihydrotestosterone and testosterone in hippocampal neurons: dependence on synaptic androgen receptor and kinase networks. Brain Res. 1621, 121–132. doi: 10.1016/j.brainres.2014.12.011
Hatanaka, Y., Mukai, H., Mitsuhashi, K., Hojo, Y., Murakami, G., Komatsuzaki, Y., et al. (2009). Androgen rapidly increases dendritic thorns of CA3 neurons in male rat hippocampus. Biochem. Biophys. Res. Commun. 381, 728–732. doi: 10.1016/j.bbrc.2009.02.130
Hedges, V. L., Ebner, T. J., Meisel, R. L., and Mermelstein, P. G. (2012). The cerebellum as a target for estrogen action. Front. Neuroendocrinol. 33, 403–411. doi: 10.1016/j.yfrne.2012.08.005
Hogervorst, E., De Jager, C., Budge, M., and Smith, A. D. (2004). Serum levels of estradiol and testosterone and performance in different cognitive domains in healthy elderly men and women. Psychoneuroendocrinology 29, 405–421. doi: 10.1016/s0306-4530(03)00053-2
Hojo, Y., Hattori, T. A., Enami, T., Furukawa, A., Suzuki, K., Ishii, H. T., et al. (2004). Adult male rat hippocampus synthesizes estradiol from pregnenolone by cytochromes P45017alpha and P450 aromatase localized in neurons. Proc. Natl. Acad. Sci. U.S.A. 101, 865–870. doi: 10.1073/pnas.2630225100
Hojo, Y., and Kawato, S. (2018). Neurosteroids in adult hippocampus of male and female rodents: biosynthesis and actions of sex steroids. Front. Endocrinol. 9:183. doi: 10.3389/fendo.2018.00183
Hojo, Y., Munetomo, A., Mukai, H., Ikeda, M., Sato, R., Hatanaka, Y., et al. (2015). Estradiol rapidly modulates spinogenesis in hippocampal dentate gyrus: involvement of kinase networks. Horm. Behav. 74, 149–156. doi: 10.1016/j.yhbeh.2015.06.008
Hojo, Y., Murakami, G., Mukai, H., Higo, S., Hatanaka, Y., Ogiue-Ikeda, M., et al. (2008). Estrogen synthesis in the brain–role in synaptic plasticity and memory. Mol. Cell. Endocrinol. 290, 31–43. doi: 10.1016/j.mce.2008.04.017
Inagaki, T., Frankfurt, M., and Luine, V. (2012). Estrogen-induced memory enhancements are blocked by acute bisphenol A in adult female rats: role of dendritic spines. Endocrinology 153, 3357–3367. doi: 10.1210/en.2012-1121
Inagaki, T., Gautreaux, C., and Luine, V. (2010). Acute estrogen treatment facilitates recognition memory consolidation and alters monoamine levels in memory-related brain areas. Horm. Behav. 58, 415–426. doi: 10.1016/j.yhbeh.2010.05.013
Jacome, L. F., Barateli, K., Buitrago, D., Lema, F., Frankfurt, M., and Luine, V. N. (2016). Gonadal hormones rapidly enhance spatial memory and increase hippocampal spine density in male rats. Endocrinology 157, 1357–1362. doi: 10.1210/en.2015-1959
Kang, L., Li, S., Xing, Z., Li, J., Su, Y., Fan, P., et al. (2014). Dihydrotestosterone treatment delays the conversion from mild cognitive impairment to Alzheimer’s disease in SAMP8 mice. Horm. Behav. 65, 505–515. doi: 10.1016/j.yhbeh.2014.03.017
Kato, A., Hojo, Y., Higo, S., Komatsuzaki, Y., Murakami, G., Yoshino, H., et al. (2013). Female hippocampal estrogens have a significant correlation with cyclic fluctuation of hippocampal spines. Front. Neural Circuits 7:149. doi: 10.3389/fncir.2013.00149
Kawato, S. (2004). Endocrine disrupters as disrupters of brain function: a neurosteroid viewpoint. Environ. Sci. 11, 1–14.
Kimoto, T., Tsurugizawa, T., Ohta, Y., Makino, J., Tamura, H., Hojo, Y., et al. (2001). Neurosteroid synthesis by cytochrome p450-containing systems localized in the rat brain hippocampal neurons: N-methyl-D-aspartate and calcium-dependent synthesis. Endocrinology 142, 3578–3589. doi: 10.1210/endo.142.8.8327
Kramar, E. A., Chen, L. Y., Rex, C. S., Gall, C. M., and Lynch, G. (2009). Estrogen’s place in the family of synaptic modulators. Mol. Cell. Pharmacol. 1, 258–262.
Krentzel, A. A., Barrett, L. R., and Meitzen, J. (2019). Estradiol rapidly modulates excitatory synapse properties in a sex- and region-specific manner in rat nucleus accumbens core and caudate-putamen. J. Neurophysiol. 122, 1213–1225. doi: 10.1152/jn.00264.2019
Leranth, C., Hajszan, T., and MacLusky, N. J. (2004). Androgens increase spine synapse density in the CA1 hippocampal subfield of ovariectomized female rats. J. Neurosci. 24, 495–499. doi: 10.1523/JNEUROSCI.4516-03.2004
Lisberger, S. G., and Miles, F. A. (1980). Role of primate medial vestibular nucleus in long-term adaptive plasticity of vestibuloocular reflex. J. Neurophysiol. 43, 1725–1745. doi: 10.1152/jn.1980.43.6.1725
Lu, Y., Sareddy, G. R., Wang, J., Wang, R., Li, Y., Dong, Y., et al. (2019). Neuron-derived estrogen regulates synaptic plasticity and memory. J. Neurosci. 39, 2792–2809. doi: 10.1523/JNEUROSCI.1970-18.2019
Luine, V., and Frankfurt, M. (2020). Estrogenic regulation of memory: the first 50 years. Horm. Behav. 121:104711. doi: 10.1016/j.yhbeh.2020.104711
Luine, V. N. (2014). Estradiol and cognitive function: past, present and future. Horm. Behav. 66, 602–618. doi: 10.1016/j.yhbeh.2014.08.011
Luine, V. N., and Frankfurt, M. (2012). Estrogens facilitate memory processing through membrane mediated mechanisms and alterations in spine density. Front. Neuroendocrinol. 33:388–402. doi: 10.1016/j.yfrne.2012.07.004
Luine, V. N., Jacome, L. F., and Maclusky, N. J. (2003). Rapid enhancement of visual and place memory by estrogens in rats. Endocrinology 144, 2836–2844. doi: 10.1210/en.2003-0004
MacLusky, N. J., Luine, V. N., Hajszan, T., and Leranth, C. (2005). The 17alpha and 17beta isomers of estradiol both induce rapid spine synapse formation in the CA1 hippocampal subfield of ovariectomized female rats. Endocrinology 146, 287–293. doi: 10.1210/en.2004-0730
Massot, C., Schneider, A. D., Chacron, M. J., and Cullen, K. E. (2012). The vestibular system implements a linear-nonlinear transformation in order to encode self-motion. PLoS Biol. 10:e1001365. doi: 10.1371/journal.pbio.1001365
Mensah-Nyagan, A. G., Do-Rego, J. L., Beaujean, D., Luu-The, V., Pelletier, G., and Vaudry, H. (1999). Neurosteroids: expression of steroidogenic enzymes and regulation of steroid biosynthesis in the central nervous system. Pharmacol. Rev. 51, 63–81.
Moffat, S. D., Zonderman, A. B., Metter, E. J., Blackman, M. R., Harman, S. M., and Resnick, S. M. (2002). Longitudinal assessment of serum free testosterone concentration predicts memory performance and cognitive status in elderly men. J. Clin. Endocrinol. Metab. 87, 5001–5007. doi: 10.1210/jc.2002-020419
Mohamad, N. V., Ima-Nirwana, S., and Chin, K. Y. (2018). A review on the effects of testosterone supplementation in hypogonadal men with cognitive impairment. Curr. Drug Targets 19, 898–906. doi: 10.2174/1389450118666170913162739
Moradpour, F., Naghdi, N., and Fathollahi, Y. (2006). Anastrozole improved testosterone-induced impairment acquisition of spatial learning and memory in the hippocampal CA1 region in adult male rats. Behav. Brain Res. 175, 223–232. doi: 10.1016/j.bbr.2006.08.037
Mukai, H., Takata, N., Ishii, H. T., Tanabe, N., Hojo, Y., Furukawa, A., et al. (2006). Hippocampal synthesis of estrogens and androgens which are paracrine modulators of synaptic plasticity: synaptocrinology. Neuroscience 138, 757–764. doi: 10.1016/j.neuroscience.2005.09.010
Mukai, H., Tsurugizawa, T., Murakami, G., Kominami, S., Ishii, H., Ogiue-Ikeda, M., et al. (2007). Rapid modulation of long-term depression and spinogenesis via synaptic estrogen receptors in hippocampal principal neurons. J. Neurochem. 100, 950–967. doi: 10.1111/j.1471-4159.2006.04264.x
Mulkey, R. M., Endo, S., Shenolikar, S., and Malenka, R. C. (1994). Involvement of a calcineurin/inhibitor-1 phosphatase cascade in hippocampal long-term depression. Nature 369, 486–488. doi: 10.1038/369486a0
Murakami, G., Hojo, Y., Kato, A., Komatsuzaki, Y., Horie, S., Soma, M., et al. (2018). Rapid nongenomic modulation by neurosteroids of dendritic spines in the hippocampus: androgen, oestrogen and corticosteroid. J. Neuroendocrinol. 30:e12561. doi: 10.1111/jne.12561
Murakami, G., Hojo, Y., Ogiue-Ikeda, M., Mukai, H., Chambon, P., Nakajima, K., et al. (2015). Estrogen receptor KO mice study on rapid modulation of spines and long-term depression in the hippocampus. Brain Res. 1621, 133–146. doi: 10.1016/j.brainres.2014.12.002
Murphy, D. D., Cole, N. B., Greenberger, V., and Segal, M. (1998). Estradiol increases dendritic spine density by reducing GABA neurotransmission in hippocampal neurons. J. Neurosci. 18, 2550–2559.
Murphy, D. D., and Segal, M. (1996). Regulation of dendritic spine density in cultured rat hippocampal neurons by steroid hormones. J. Neurosci. 16, 4059–4068.
Ooishi, Y., Kawato, S., Hojo, Y., Hatanaka, Y., Higo, S., Murakami, G., et al. (2012). Modulation of synaptic plasticity in the hippocampus by hippocampus-derived estrogen and androgen. J. Steroid Biochem. Mol. Biol. 131, 37–51. doi: 10.1016/j.jsbmb.2011.10.004
Pan, W., Han, S., Kang, L., Li, S., Du, J., and Cui, H. (2016). Effects of dihydrotestosterone on synaptic plasticity of the hippocampus in mild cognitive impairment male SAMP8 mice. Exp. Ther. Med. 12, 1455–1463. doi: 10.3892/etm.2016.3470
Pape, H. C., and Pare, D. (2010). Plastic synaptic networks of the amygdala for the acquisition, expression, and extinction of conditioned fear. Physiol. Rev. 90, 419–463. doi: 10.1152/physrev.00037.2009
Pettorossi, V. E., Di Mauro, M., Scarduzio, M., Panichi, R., Tozzi, A., Calabresi, P., et al. (2013a). Modulatory role of androgenic and estrogenic neurosteroids in determining the direction of synaptic plasticity in the CA1 hippocampal region of male rats. Physiol. Rep. 1:e00185. doi: 10.1002/phy2.185
Pettorossi, V. E., Frondaroli, A., and Grassi, S. (2011). Cyclic estrogenic fluctuation influences synaptic transmission of the medial vestibular nuclei in female rats. Acta Otolaryngol. 131, 434–439. doi: 10.3109/00016489.2010.536992
Pettorossi, V. E., Panichi, R., Botti, F. M., Kyriakareli, A., Ferraresi, A., Faralli, M., et al. (2013b). Prolonged asymmetric vestibular stimulation induces opposite, long-term effects on self-motion perception and ocular responses. J. Physiol. 591, 1907–1920. doi: 10.1113/jphysiol.2012.241182
Phan, A., Gabor, C. S., Favaro, K. J., Kaschack, S., Armstrong, J. N., MacLusky, N. J., et al. (2012). Low doses of 17β-estradiol rapidly improve learning and increase hippocampal dendritic spines. Neuropsychopharmacology 37, 2299–2309. doi: 10.1038/npp.2012.82
Phan, A., Suschkov, S., Molinaro, L., Reynolds, K., Lymer, J. M., Bailey, C. D., et al. (2015). Rapid increases in immature synapses parallel estrogen-induced hippocampal learning enhancements. Proc. Natl. Acad. Sci. U.S.A. 112, 16018–16023. doi: 10.1073/pnas.1522150112
Pike, C. J., Rosario, E. R., and Nguyen, T. V. (2006). Androgens, aging, and Alzheimer’s disease. Endocrine 29, 233–241.
Rudick, C. N., and Woolley, C. S. (2001). Estrogen regulates functional inhibition of hippocampal CA1 pyramidal cells in the adult female rat. J. Neurosci. 21, 6532–6543.
Sakamoto, H., Mezaki, Y., Shikimi, H., Ukena, K., and Tsutsui, K. (2003). Dendritic growth and spine formation in response to estrogen in the developing Purkinje cell. Endocrinology 144, 4466–4477. doi: 10.1210/en.2003-0307
Saldanha, C. J., Remage-Healey, L., and Schlinger, B. A. (2011). Synaptocrine signaling: steroid synthesis and action at the synapse. Endocr. Rev. 32, 532–549. doi: 10.1210/er.2011-0004
Scarduzio, M., Panichi, R., Pettorossi, V. E., and Grassi, S. (2012). The repetition timing of high frequency afferent stimulation drives the bidirectional plasticity at central synapses in the rat medial vestibular nuclei. Neuroscience 223, 1–11. doi: 10.1016/j.neuroscience.2012.07.039
Scarduzio, M., Panichi, R., Pettorossi, V. E., and Grassi, S. (2013). Synaptic long-term potentiation and depression in the rat medial vestibular nuclei depend on neural activation of estrogenic and androgenic signals. PLoS One 8:e80792. doi: 10.1371/journal.pone.0080792
Shiroma, S., Yamaguchi, T., and Kometani, K. (2005). Effects of 17beta-estradiol on chemically induced long-term depression. Neuropharmacology 49, 97–102. doi: 10.1016/j.neuropharm.2005.02.002
Smith, C. C., and McMahon, L. L. (2005). Estrogen-induced increase in the magnitude of long-term potentiation occurs only when the ratio of NMDA transmission to AMPA transmission is increased. J. Neurosci. 25, 7780–7791. doi: 10.1523/JNEUROSCI.0762-05.2005
Smith, C. C., and McMahon, L. L. (2006). Estradiol-induced increase in the magnitude of long-term potentiation is prevented by blocking NR2B-containing receptors. J. Neurosci. 26, 8517–8522. doi: 10.1523/JNEUROSCI.5279-05.2006
Smith, P. F., and Curthoys, I. S. (1989). Mechanisms of recovery following unilateral labyrinthectomy: a review. Brain Res. Brain Res. Rev. 14, 155–180. doi: 10.1016/0165-0173(89)90013-1
Soma, M., Kim, J., Kato, A., and Kawato, S. (2018). Src Kinase dependent rapid non-genomic modulation of hippocampal spinogenesis induced by androgen and estrogen. Front. Neurosci. 12:282. doi: 10.3389/fnins.2018.00282
Song, Z., Yang, H., Peckham, E. M., and Becker, J. B. (2019). Estradiol-induced potentiation of dopamine release in dorsal striatum following amphetamine administration requires estradiol receptors and mGlu5. eNeuro 6:ENEURO.0446-18.2019. doi: 10.1523/ENEURO.0446-18.2019
Srivastava, D. P. (2012). Two-step wiring plasticity–a mechanism for estrogen-induced rewiring of cortical circuits. J. Steroid Biochem. Mol. Biol. 131, 17–23. doi: 10.1016/j.jsbmb.2012.01.006
Sumner, R. L., Spriggs, M. J., McMillan, R. L., Sundram, F., and Kirk, I. J. (2018). Muthukumaraswamy SD. Neural plasticity is modified over the human menstrual cycle: combined insight from sensory evoked potential LTP and repetition suppression. Neurobiol. Learn. Mem. 155, 422–434. doi: 10.1016/j.nlm.2018.08.016
Tabori, N. E., Stewart, L. S., Znamensky, V., Romeo, R. D., Alves, S. E., McEwen, B. S., et al. (2005). Ultrastructural evidence that androgen receptors are located at extranuclear sites in the rat hippocampal formation. Neuroscience 130, 151–163. doi: 10.1016/j.neuroscience.2004.08.048
Tanaka, M., and Sokabe, M. (2012). Continuous de novo synthesis of neurosteroids is required for normal synaptic transmission and plasticity in the dentate gyrus of the rat hippocampus. Neuropharmacology 62, 2373–2387. doi: 10.1016/j.neuropharm.2012.02.007
Tanaka, M., and Sokabe, M. (2013). Bidirectional modulatory effect of 17beta-estradiol on NMDA receptors via ERalpha and ERbeta in the dentate gyrus of juvenile male rats. Neuropharmacology 75, 262–273. doi: 10.1016/j.neuropharm.2013.07.029
Toni, N., Buchs, P. A., Nikonenko, I., Bron, C. R., and Muller, D. (1999). LTP promotes formation of multiple spine synapses between a single axon terminal and a dendrite. Nature 402, 421–425. doi: 10.1038/46574
Tovote, P., Fadok, J. P., and Luthi, A. (2015). Neuronal circuits for fear and anxiety. Nat. Rev. Neurosci. 16, 317–331. doi: 10.1038/nrn3945
Tozzi, A., de Iure, A., Tantucci, M., Durante, V., Quiroga-Varela, A., Giampa, C., et al. (2015). Endogenous 17beta-estradiol is required for activity-dependent long-term potentiation in the striatum: interaction with the dopaminergic system. Front. Cell. Neurosci. 9:192. doi: 10.3389/fncel.2015.00192
Tozzi, A., Durante, V., Manca, P., Di Mauro, M., Blasi, J., Grassi, S., et al. (2019). Bidirectional synaptic plasticity is driven by sex neurosteroids targeting estrogen and androgen receptors in hippocampal CA1 pyramidal neurons. Front. Cell. Neurosci. 13:534. doi: 10.3389/fncel.2019.00534
Tsutsui, K., Ukena, K., Sakamoto, H., Okuyama, S., and Haraguchi, S. (2011). Biosynthesis, mode of action, and functional significance of neurosteroids in the purkinje cell. Front. Endocrinol. 2:61. doi: 10.3389/fendo.2011.00061
Tuscher, J. J., Szinte, J. S., Starrett, J. R., Krentzel, A. A., Fortress, A. M., Remage-Healey, L., et al. (2016). Inhibition of local estrogen synthesis in the hippocampus impairs hippocampal memory consolidation in ovariectomized female mice. Horm. Behav. 83, 60–67. doi: 10.1016/j.yhbeh.2016.05.001
Vierk, R., Glassmeier, G., Zhou, L., Brandt, N., Fester, L., Dudzinski, D., et al. (2012). Aromatase inhibition abolishes LTP generation in female but not in male mice. J. Neurosci. 32, 8116–8126. doi: 10.1523/JNEUROSCI.5319-11.2012
Wagner, B. A., Braddick, V. C., Batson, C. G., Cullen, B. H., Miller, L. E., and Spritzer, M. D. (2018). Effects of testosterone dose on spatial memory among castrated adult male rats. Psychoneuroendocrinology 89, 120–130. doi: 10.1016/j.psyneuen.2017.12.025
Warren, S. G., Humphreys, A. G., Juraska, J. M., and Greenough, W. T. (1995). LTP varies across the estrous cycle: enhanced synaptic plasticity in proestrus rats. Brain Res. 703, 26–30. doi: 10.1016/0006-8993(95)01059-9
Weiland, N. G. (1992). Estradiol selectively regulates agonist binding sites on the N-methyl-D-aspartate receptor complex in the CA1 region of the hippocampus. Endocrinology 131, 662–668. doi: 10.1210/endo.131.2.1353442
Wong, M., and Moss, R. L. (1991). Electrophysiological evidence for a rapid membrane action of the gonadal steroid, 17 beta-estradiol, on CA1 pyramidal neurons of the rat hippocampus. Brain Res. 543, 148–152. doi: 10.1016/0006-8993(91)91057-8
Wong, M., and Moss, R. L. (1992). Long-term and short-term electrophysiological effects of estrogen on the synaptic properties of hippocampal CA1 neurons. J. Neurosci. 12, 3217–3225.
Woolley, C. S. (1998). Estrogen-mediated structural and functional synaptic plasticity in the female rat hippocampus. Horm. Behav. 34, 140–148. doi: 10.1006/hbeh.1998.1466
Woolley, C. S., Gould, E., Frankfurt, M., and McEwen, B. S. (1990). Naturally occurring fluctuation in dendritic spine density on adult hippocampal pyramidal neurons. J. Neurosci. 10, 4035–4039.
Woolley, C. S., and McEwen, B. S. (1993). Roles of estradiol and progesterone in regulation of hippocampal dendritic spine density during the estrous cycle in the rat. J. Comp. Neurol. 336, 293–306. doi: 10.1002/cne.903360210
Woolley, C. S., Weiland, N. G., McEwen, B. S., and Schwartzkroin, P. A. (1997). Estradiol increases the sensitivity of hippocampal CA1 pyramidal cells to NMDA receptor-mediated synaptic input: correlation with dendritic spine density. J. Neurosci. 17, 1848–1859.
Woolley, C. S., Wenzel, H. J., and Schwartzkroin, P. A. (1996). Estradiol increases the frequency of multiple synapse boutons in the hippocampal CA1 region of the adult female rat. J. Comp. Neurol. 373, 108–117.
Yang, Y., Wang, X. B., Frerking, M., and Zhou, Q. (2008). Spine expansion and stabilization associated with long-term potentiation. J. Neurosci. 28, 5740–5751. doi: 10.1523/JNEUROSCI.3998-07.2008
Zhao, C., Fujinaga, R., Tanaka, M., Yanai, A., Nakahama, K., and Shinoda, K. (2007). Region-specific expression and sex-steroidal regulation on aromatase and its mRNA in the male rat brain: immunohistochemical and in situ hybridization analyses. J. Comp. Neurol. 500, 557–573. doi: 10.1002/cne.21193
Zhao, L., Chen, S., Ming Wang, J., and Brinton, R. D. (2005). 17beta-estradiol induces Ca2+ influx, dendritic and nuclear Ca2+ rise and subsequent cyclic AMP response element-binding protein activation in hippocampal neurons: a potential initiation mechanism for estrogen neurotrophism. Neuroscience 132, 299–311. doi: 10.1016/j.neuroscience.2004.11.054
Keywords: 17β-estradiol, testosterone, 5α-dihydrotestosterone, P450 aromatase, 5α-reductase, LTP, LTD, synaptic plasticity
Citation: Tozzi A, Bellingacci L and Pettorossi VE (2020) Rapid Estrogenic and Androgenic Neurosteroids Effects in the Induction of Long-Term Synaptic Changes: Implication for Early Memory Formation. Front. Neurosci. 14:572511. doi: 10.3389/fnins.2020.572511
Received: 14 June 2020; Accepted: 21 August 2020;
Published: 27 October 2020.
Edited by:
Takayoshi Ubuka, Waseda University, JapanReviewed by:
Yasushi Hojo, Saitama Medical University, JapanTetsuya Kimoto, The University of Tokyo, Japan
Copyright © 2020 Tozzi, Bellingacci and Pettorossi. This is an open-access article distributed under the terms of the Creative Commons Attribution License (CC BY). The use, distribution or reproduction in other forums is permitted, provided the original author(s) and the copyright owner(s) are credited and that the original publication in this journal is cited, in accordance with accepted academic practice. No use, distribution or reproduction is permitted which does not comply with these terms.
*Correspondence: Alessandro Tozzi, YWxlc3NhbmRyby50b3p6aUB1bmlwZy5pdA==