- 1Department of Pediatric Dentistry, Guanghua School of Stomatology, Hospital of Stomatology, Sun Yat-sen University, Guangzhou, China
- 2Guangdong Provincial Key Laboratory of Stomatology, Guangzhou, China
- 3Department of Oral Anatomy and Physiology, Guanghua School of Stomatology, Hospital of Stomatology, Sun Yat-sen University, Guangzhou, China
The activation of opioid receptors by exogenous or endogenous opioids can produce significant analgesic effects in peripheral tissues. Numerous researchers have demonstrated the expression of peripheral opioid receptors (PORs) and endogenous opioid peptides (EOPs) in the orofacial region. Growing evidence has shown the involvement of PORs and immune cell-derived EOPs in the modulation of orofacial pain. In this review, we discuss the role of PORs and EOPs in orofacial pain and the possible cellular mechanisms involved. Furthermore, the potential development of therapeutic strategies for orofacial pain is also summarized.
Introduction
It has been well established that opioid receptors are widely distributed throughout the central (spinal cord, brain) and peripheral (trigeminal and dorsal root ganglia) sensory nervous systems (Machelska and Celik, 2020). Activation of opioid receptors by opioids can play a significant role in inflammatory and neuropathic pain. A growing body of literature has noted that interactions involving peripheral opioid receptors (PORs) and immune cell-derived endogenous opioid peptides (EOPs) produce potent analgesia in painful conditions (Machelska and Stein, 2006; Hua, 2016). Furthermore, the analgesic effects of PORs and EOPs occur without central side effects, such as respiratory depression, nausea, constipation, pruritus, addiction, and tolerance (Stein, 2016). PORs and EOPs have been shown to be involved in orofacial pain (Liu et al., 2020). This review provides evidence for the role of PORs and immune cell-derived EOPs in orofacial pain. Possible cellular mechanisms involving PORs and EOPs in modulating orofacial pain are also discussed. In addition, this review summarizes potential development of therapeutic strategies for orofacial pain control.
The Opioid System
Opioid Receptors
Opioid receptors are classified into three main subtypes: μ-receptor (MOR), δ-receptor (DOR) and κ-receptor (KOR) (Cox, 2013; Stevens, 2015). These three opioid receptors belong to the family of G-nucleotide binding protein-coupled receptors (GPCRs) and are encoded by three genes: Oprm1, Oprd1, and Oprk1 (Law et al., 2013). The structure of opioid receptors consists of seven transmembrane domains, three intracellular hydrophobic loops, three extracellular loops, an extracellular amino terminus, and an intracellular carboxyl terminus (Sobczak et al., 2014).
Opioid receptors are synthesized in the dorsal root ganglion (DRG) and transported to central and peripheral nerve terminals. In the peripheral nervous system, opioid receptors are expressed in the cell bodies and peripheral terminals of primary afferent neurons and sympathetic postganglionic terminals (Stein, 2003; Sehgal et al., 2011). In addition to the nervous system, opioid receptors are also expressed by immune cells, such as lymphocytes, macrophages, and granulocytes (Chuang et al., 1995a,b; Celik et al., 2016; Jiao et al., 2018). MOR, DOR, and KOR proteins can be detected in astrocytes and oligodendrocytes in the mouse brain (Stiene-Martin et al., 2001), but no study has shown the expression of opioid receptors in satellite glial cells. More research is needed to verify the presence of opioid receptors in glial cells.
Endogenous Opioid Peptides
Classical EOPs are categorized as endorphins, enkephalins, and dynorphins, which are derived from three precursor proteins, proopiomelanocortin (POMC), proenkephalin (PENK), and prodynorphin (PDYN), respectively (Stein, 2018). These opioid peptides exhibit different affinity and selectivity for MOR (endorphins, enkephalins), DOR (enkephalins, endorphins) and KOR (dynorphins) (Machelska, 2007). It has been demonstrated that EOPs are expressed in the central and peripheral nervous systems, neuroendocrine tissues, and immune cells (Labuz et al., 2009; Stein and Machelska, 2011; Basso et al., 2015; Table 1).
Moreover, EOPs or their precursors are also found in glial cells. Several studies have identified the expression of opioid peptides in cultured primary spinal microglia and astrocytes (Vilijn et al., 1988; Shinoda et al., 1989; Hauser et al., 1990). Spinal cord microglia-derived β-endorphins have been shown to participate in inflammatory, neuropathic, and bone cancer pain (Gong et al., 2014; Fan et al., 2016; Huang et al., 2017; Wu et al., 2018; Mao et al., 2019). However, there are no studies reported concerning the expression of opioid peptides in satellite glial cells.
Opioid-releasing immune cells mainly include macrophages/monocytes, granulocytes, and lymphocytes (Mousa et al., 2004, 2007; Pannell et al., 2016). The expression of EOPs and their precursor proteins in immune cells is increased during inflammation (Cabot et al., 1997; Boue et al., 2011; Hua, 2016). In the initial phase of inflammation, the major opioid-producing leukocytes are granulocytes, while macrophages/monocytes and lymphocytes predominate at later stages of inflammation (Rittner et al., 2001; Brack et al., 2004a). IL-4 can upregulate POMC expression and β-endorphin production in lymphocytes, which contributes to the inhibition of inflammatory pain via activation of PORs (Busch-Dienstfertig et al., 2012). Enkephalins released by CD4(+) T lymphocytes relieve visceral inflammatory pain (Basso et al., 2018). Another study indicates that increased levels of methionine-enkephalin (Met-enkephalin) and dynorphin A produce analgesic effects against long-lasting inflammatory pain induced by complete Freund’s adjuvant (CFA) (Jiang et al., 2015). The analgesic effects of immune cell-derived EOPs have also been verified for neuropathic and cancer pain (Baamonde et al., 2006; Labuz et al., 2009, 2010).
Modulation of Orofacial Pain via PORS and EOPS
Orofacial Pain
Orofacial pain is the common name of various disorders, ranging from inflammatory diseases to neuropathic pain syndromes, and which refers to pain associated with the head, face, neck, and intraoral structures (Ayoub et al., 2018; Feher et al., 2019). The prevalence of orofacial pain is approximately 17 to 26%, of which 7–11% is classified as chronic (Benoliel and Sharav, 2010). Inflammatory mediators produced during orofacial inflammation and peripheral nerve damage or infection can evoke pain via activation and sensitization of specialized trigeminal primary afferent neurons called nociceptors (Rittner et al., 2008; Matsuda et al., 2019). Nociceptors mainly comprise myelinated Aδ and non-myelinated C fibers, and their cell bodies are located in the trigeminal ganglion (TG) (Sessle, 2011). After activation by noxious stimuli, these nociceptive fibers conduct nerve impulses from their nociceptive endings into the somatosensory and limbic cortices through the spinal trigeminal nucleus and thalamus.
Upon peripheral inflammation or nerve injury, numerous non-neuronal cells, such as endothelial cells, fibroblasts, dendritic cells, mast cells, macrophages, and Schwann cells are activated and release many mediators (Machelska, 2011). The inflammatory mediators involved in the activation of nociceptors include, but are not limited to, cytokines, chemokines, nerve growth factor, bradykinin, prostaglandins, and ATP (Rittner and Stein, 2005). Activation of various ion channels and protein kinases, such as transient receptor potential vanilloid 1 (TRPV1), protein kinase A, and mitogen-activated protein kinases (MAPKs), also contribute to the development of pain and hyperalgesia (Aley and Levine, 1999; Obata and Noguchi, 2004; Moore et al., 2018). A better understanding of the underlying orofacial inflammatory and neuropathic pain mechanism will allow the development of novel therapeutic strategies for such painful conditions.
The Role of PORs and EOPs in Orofacial Pain
Accumulating evidence has shown that PORs are expressed in orofacial tissue. An early study indicated the immunohistochemical localization of DOR in various peripheral tissues, including the cornea, eyelid, and lip (Wenk and Honda, 1999). A number of investigations have demonstrated increased expression of MOR protein via western blot and immunofluorescent staining in TG under acute muscle pain conditions (Bagues et al., 2018; Zhang et al., 2018). The upregulation of MOR in TG is observed in rat orofacial inflammatory pain models utilizing RT-PCR at the mRNA level and western blot at the protein level (Nunez et al., 2007; Zhang et al., 2014). The levels of MOR mRNA detected by qPCR and of protein detected by immunohistochemistry and western blot are elevated in the rat orofacial persistent pain model (Bai et al., 2015). Immunohistochemical experiments and qPCR revealed that the androgen receptor regulates MOR expression in the TG under acute masseter pain conditions (Lee et al., 2016). The level of MOR in the anterior synovial membrane is higher than that in the posterior synovial membrane in the non-inflamed temporomandibular joint (TMJ) using non-radiographic in situ hybridization and immunohistochemistry (Hayashi et al., 2002). Moreover, the application of peripherally acting KOR agonists suppresses formalin-induced TMJ nociceptive behavior, indicating the location of functional KOR within the rat TMJ (Clemente et al., 2004). Several immunohistochemical studies have revealed the localization of MOR and DOR in human and rat dental pulp (Jaber et al., 2003; Fristad et al., 2006). Furthermore, the expression of MOR, DOR, and KOR has been verified in primary human oral epithelial cells by RT-PCR and immunocytochemical techniques (Charbaji et al., 2012; Table 2).
Notably, activation of PORs can produce antinociceptive effects on orofacial inflammatory and neuropathic pain. The contribution of PORs to antinociception has been demonstrated in orofacial masseter pain (Sanchez et al., 2010; Saloman et al., 2011). The antinociceptive effects mediated by PORs were also assessed in rat TMJ pain models (Macedo et al., 2016; Coura et al., 2017). Activation of local opioid receptors by enkephalin attenuates nociceptive behavior in a rat model of trigeminal neuropathic pain (Meunier et al., 2005). Low doses of naloxone have been shown to produce peripheral antinociceptive effects by activating DOR and KOR in in vivo and in vitro models of trigeminal nociception (Capuano et al., 2010). Furthermore, therapeutic ultrasound is effective in alleviating experimental trigeminal neuropathic pain, with a mechanism involving opioid receptor activation (Savernini et al., 2012). Clinical studies have also assessed the efficacy of peripheral morphine analgesia in dental surgery-induced pain, oral mucositis pain, and temporomandibular disorder with myofascial pain (Likar et al., 2001; Nielsen et al., 2012; Kang et al., 2018).
Meanwhile, various investigations have indicated the involvement of EOPs in orofacial pain. An early chromatography study suggested the distribution of enkephalin precursor proteins in the nuclear, microsomal, and supernatant fractions of rat dental pulp tissue (Wei et al., 1991). Another immunostaining assay has shown that endomorphin-2 is basally expressed in TG, trigeminal nucleus caudalis, and periodontium in rats (Liu et al., 2019). Moreover, clinical studies have revealed increased expression levels of β-endorphins and Met-enkephalin in human dental pulp with asymptomatic inflammation or controlled orthodontic intrusive forces by radioimmunoassay (Chavarría-Bolaños et al., 2014, 2015). The administration of endomorphin-2 to both TG and periodontal tissues alleviates orofacial pain induced by tooth movement (Liu et al., 2019). Local overproduction of PENK-derived peptides in TG sensory neurons via a genomic herpes simplex virus-derived vector evokes a potent antiallodynic effect on trigeminal neuropathic pain by activating PORs (Meunier et al., 2005). In addition, resveratrol has been shown to inhibit the nociceptive jaw-opening reflex via the endogenous opioid system (Kokuba et al., 2017). Clinical trials have also demonstrated that β-endorphin levels are correlated with TMJ pain (Feldreich et al., 2012, 2017). Notably, β-endorphin and dynorphin released from leukocytes cells are involved in the antinociceptive effect of 15-deoxy-Δ12,14-prostaglandin J2 (15d-PGJ2) in formalin-induced TMJ pain (Macedo et al., 2016). Neutrophil-mediated β-endorphin also produces antinociceptive effects on acute oral cancer pain in rats (Scheff et al., 2018, 2019; Table 3). To date, few studies have focused on the EOPs released by immune cells in orofacial pain, which deserves further investigation.
Mechanisms of Action
Migration of Opioid Peptide-Containing Immune Cells to Inflamed/Injured Tissue
It has been confirmed that granulocyte colony-stimulating factor significantly increases neutrophil infiltration in mouse tongues with oral cancer (Scheff et al., 2019). Administration of 1% thioglycollate in the TMJ enhances the recruitment of opioid peptide-containing leukocytes, especially macrophages, in TMJ periarticular tissue (Macedo et al., 2016). The upregulation of macrophages and lymphocytes can be observed following trigeminal nerve injury (Moreau et al., 2017). The recruitment of immune cells from the circulation into sites of nerve damage or inflamed tissue is a multistep process involving various adhesion molecules located on immune cells and vascular endothelium (Machelska, 2011; Iwaszkiewicz et al., 2013). Initially, circulating immunocytes roll along the vascular endothelial cell wall, mediated predominantly by P- and E-selectins on endothelial cells and L-selectin on immunocytes (Machelska et al., 2004). Subsequently, immunocytes are activated by chemokines secreted by endothelial and inflammatory cells and which are present on the luminal surface of the vascular endothelium (Stein et al., 2003; Busch-Dienstfertig and Stein, 2010). The expression level and avidity of integrins are upregulated, which mediates adhesion between immunocytes and endothelial cells via intercellular adhesion molecule-1 (ICAM-1) (Machelska et al., 2002; Machelska, 2007). Finally, the immunocytes migrate through the endothelium, directed predominantly by platelet-endothelial cell adhesion molecule-1 (PECAM-1) (Ley et al., 2007).
Studies have found that P-selectin is upregulated in hamster oral mucositis models, as is ICAM-1 in inflammatory process involving dental pulp (Chang et al., 2019; Mafra et al., 2019). Upregulation of P-selectin and PECAM-1, and co-expression of L-selectin and β-endorphin, are observed in immunocytes within inflamed tissues (Mousa et al., 2000). Furthermore, the depletion of neutrophil-expressed β-endorphin and Met-enkephalin induced by antibodies results in nociceptive behavior in mouse oral cancer pain models (Scheff et al., 2018). Pretreatment with antagonists of selectins, ICAM-1, integrins, and chemokines leads to decreased number of opioid peptide-containing cells in inflamed tissues and weakens the peripheral analgesic effect (Brack et al., 2004b; Machelska, 2007; Stein and Machelska, 2011). Additionally, intrathecal morphine results in a reduction in the number of β-endorphin-containing cells within the inflamed tissues, and peripheral endogenous antinociceptive effects are also significantly decreased. These findings suggest the role of central mechanisms in modulating peripheral endogenous opioid analgesia (Schmitt et al., 2003). However, another study has indicated the direct binding involving lymphocytes and cultured sensory neurons via adhesion molecules such as ICAM-1 and neural cell adhesion molecule (NCAM) (Hua et al., 2006), may be necessary to release EOPs within the effective range of PORs to produce adequate analgesia (Hua, 2016). Currently, few investigations have focused on the migration of immune cells expressing EOPs into inflamed/injured tissue upon orofacial pain, which requires further intensive study.
Release of EOPs From Immune Cells
In peripheral inflamed tissues or damaged nerves, opioid-containing immune cells release EOPs and then migrate to local lymph nodes (Hua and Cabot, 2010). Various factors trigger the release of EOPs by immune cells. Inflammatory mediators, such as corticotropin-releasing factor (CRF) and interleukin-1β (IL-1β), can stimulate the secretion of EOPs by immunocytes (Schafer et al., 1994; Cabot et al., 2001). Dental pulp injuries significantly increase CRF receptor expression in a rat model, which is correlated with localized increases in leukocytes and β-endorphins (Rutz et al., 2007; Uhrich et al., 2015). Activation of CXC chemokine receptor 2 (CXCR2) by chemokines (CXCL8 in humans; CXCL1 and CXCL2/3 in rats) also contributes to the increased release of EOPs from immune cells (Rittner et al., 2006a,b). Moreover, noradrenaline, stressful stimuli (e.g., experimental swim stress, surgery), and exogenous opioids have been shown to play a role in the release of leucocyte-derived opioid peptides (Binder et al., 2004; Machelska, 2007; Celik et al., 2016). Interestingly, one investigation demonstrated that peroxisome proliferator activated receptor-γ located in leukocytes, upon activation by 15d-PGJ2, releases β-endorphin and dynorphin into TMJ tissue (Macedo et al., 2016). More studies are needed to confirm the molecular modulation mechanism involved in opioid peptide release by orofacial inflamed/injured tissues.
Furthermore, it has been demonstrated that EOPs are released from immune cells in a calcium-dependent manner (Rittner et al., 2006a). Activation of leucocyte opioid receptors by exogenous agonists promotes the activation of phospholipase C (PLC), which then hydrolyzes phosphatidylinositol-4,5-bisphosphate (PIP2) into inositol-3 phosphate (IP3) and diacylglycerol (DAG) (Celik et al., 2016). The binding of IP3 to IP3 receptors (IP3R) in the endoplasmic reticulum (ER) leads to mobilization of intracellular Ca2+, which then accelerates the secretion of EOPs (Celik et al., 2016). Phosphokinase C (PKC) activated by DAG may have a small effect on opioid peptide release (Feske, 2007). Evidence indicates that activation of CXCR2 by chemokines leads to opioid peptide secretion regulated predominantly by IP3R-triggered Ca2+ release from the ER, partially by phosphoinositol-3-kinase (PI3K), and by p38 mitogen-activated protein kinase (p38 MAPK) (Rittner et al., 2006a, 2007, 2009a,b). Then, the EOPs packaged in vesicular structures are translocated to the cell membrane (Mousa et al., 2004). Subsequently, EOPs bind to opioid receptors on the peripheral terminals of sensory neurons, resulting in pain inhibition (Figure 1).
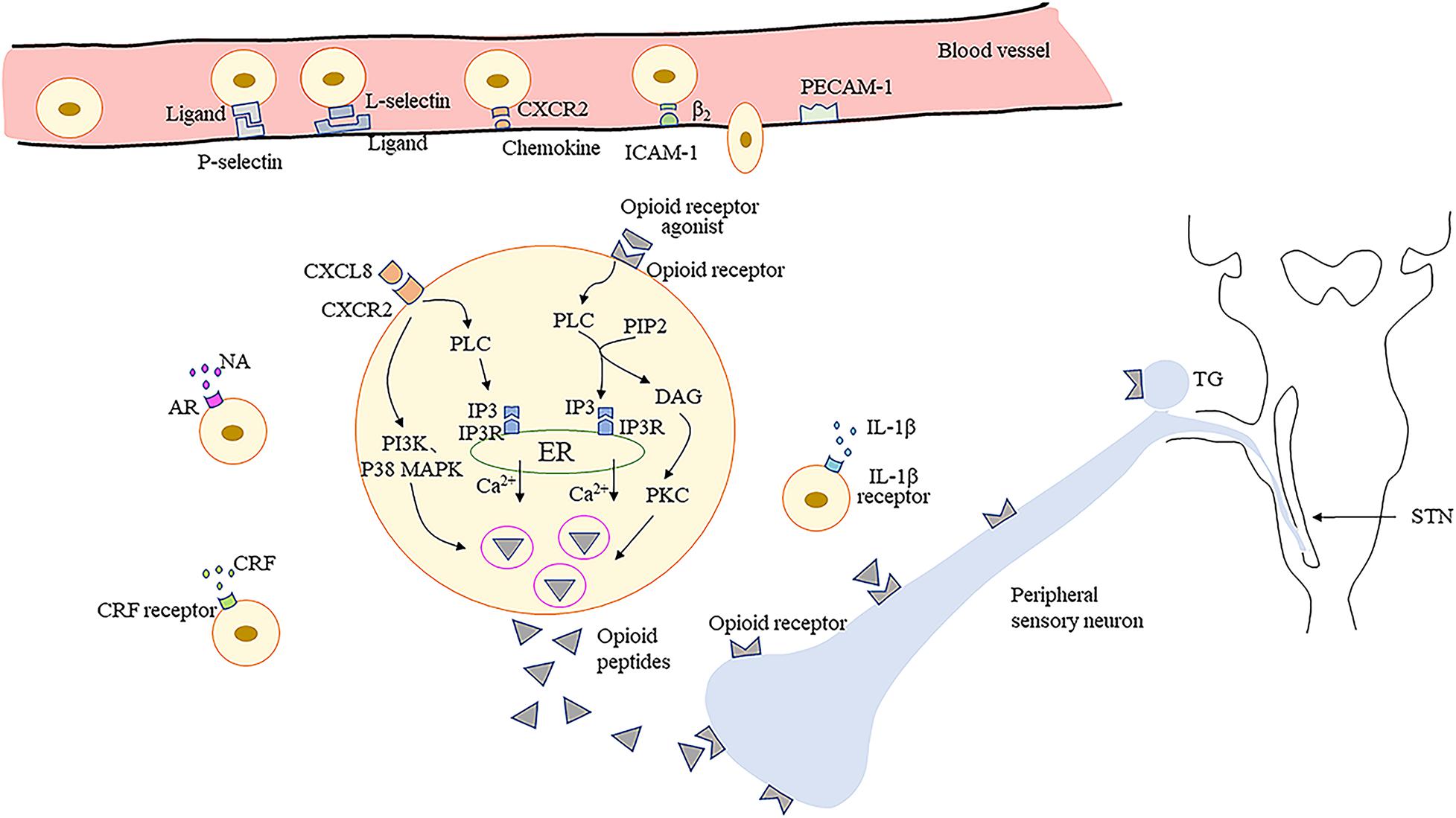
Figure 1. Migration of opioid peptide-containing immune cells and release of opioid peptides within peripheral inflamed or damaged tissues. Opioid peptide-containing immune cells migrate into inflamed or damaged tissues mediated by adhesion molecules. Release of opioid peptides is triggered by chemokines, inflammatory mediators or NA. Opioid peptide secretion is regulated predominantly by IP3R-triggered Ca2+ release from ER, partially by PI3K, and by p38 MAPK. Then opioid peptides bind to opioid receptors on peripheral terminals of sensory neurons resulting in pain inhibition. ICAM-1, intercellular adhesion molecule-1; PECAM-1, platelet-endothelial cell adhesion molecule-1; CRF, corticotropin-releasing factor; IL-1β, interleukin-1β; CXCR2, the CXC chemokine receptor 2; NA, noradrenaline; AR, adrenergic receptors; PLC, phospholipase C; PIP2, phosphatidylinositol-4,5-bisphosphate; IP3, inositol-3 phosphate; DAG, diacylglycerol; IP3R, inositol-3 phosphate receptors; ER, endoplasmic reticulum; PKC, phosphokinase C; PI3K, phosphoinositol-3-kinase; p38 MAPK, p38 mitogen-activated protein kinase; TG, trigeminal ganglion; STN, spinal trigeminal nucleus.
Activation of PORs by Endogenous and Exogenous Opioids
Inflammatory conditions or nerve injuries increase the synthesis of opioid receptors in DRG neurons and enhance the peripherally directed axonal transport of opioid receptors (Sehgal et al., 2011; Stein and Machelska, 2011; Pettinger et al., 2013). Studies have revealed that bradykinin pretreatment induces a rapid and significant increase in the trafficking of DOR to the plasma membrane in cultured TG neurons (Patwardhan et al., 2005; Pettinger et al., 2013). PI3K expression has been demonstrated to promote the export of endogenous DOR in primary TG neurons (Shiwarski et al., 2017). Inflammation induces a decrease in extracellular pH, which enhances the interaction of PORs with G proteins and downstream signaling pathways (Vetter et al., 2006). Inflammation also induces the sprouting of opioid receptor-bearing peripheral nerve endings and damage to the perineural barrier, which facilitates access of opioid receptor agonists to PORs (Rittner et al., 2012). These factors enhance the analgesic effect of opioids in inflamed peripheral tissues. The underlying mechanism of neuropathic pain is relevant to inflammatory pain, as nerve injury is usually accompanied by inflammation. However, further research into ligand accessibility, affinity, coupling, and signaling of PORs in neuropathic pain conditions is still needed (Stein and Machelska, 2011).
After activation by endogenous or exogenous agonists, opioid receptors couple to heterotrimeric Gi/o proteins forming trimeric G protein complexes, which then dissociate into Gα and Gβγ subunits to interact with various downstream effectors. The Gα subunit inhibits adenylyl cyclase (AC) and cyclic adenosine monophosphate (cAMP) production (Brust et al., 2015). Hence, protein kinase A activity is suppressed due to decreased cAMP production, which causes the inhibition of numerous receptors or ion channels such as hyperpolarization-activated cyclic nucleotide-gated (HCN) channels, TRPV1, acid-sensing ion channels, and two pore domain channels (Francois and Scherrer, 2018). It has been reported that the application of protease-activated receptor-2 agonists induces antinociceptive effects of DOR by inhibiting cAMP accumulation in a capsaicin-evoked orofacial pain model (Patwardhan et al., 2006). Moreover, activation of opioid receptors can also inhibit voltage-gated calcium channel (VGCC) activity and open G protein-coupled inwardly rectifying K+ (GIRK) channels or ATP-dependent K+ (KATP) channels via the Gβγ subunit (Currie, 2010; Wang et al., 2010; Nockemann et al., 2013). GIRK and KATP channel subunits are expressed in TG neurons and are involved in orofacial muscle pain mediated by DOR (Niu et al., 2011; Saloman et al., 2011; Chung et al., 2014). Additionally, the MAPK pathway may also play a role in formalin-induced orofacial inflammatory pain (Huang et al., 2015; Kurose et al., 2017; Zhang et al., 2018). The L-arginine/NO/cGMP pathway also participates in opioid-mediated antinociception in response to orofacial pain (Napimoga et al., 2008; Clemente-Napimoga et al., 2009; Coura et al., 2017). In brief, activation of PORs leads to cellular hyperpolarization and suppresses excitability of peripheral sensory neurons, as well as to the decreased release of excitatory mediators, including substance P, calcitonin gene-related peptide (CGRP), and glutamate (Jin et al., 2006; Beaudry et al., 2011; Snyder et al., 2018; Figure 2).
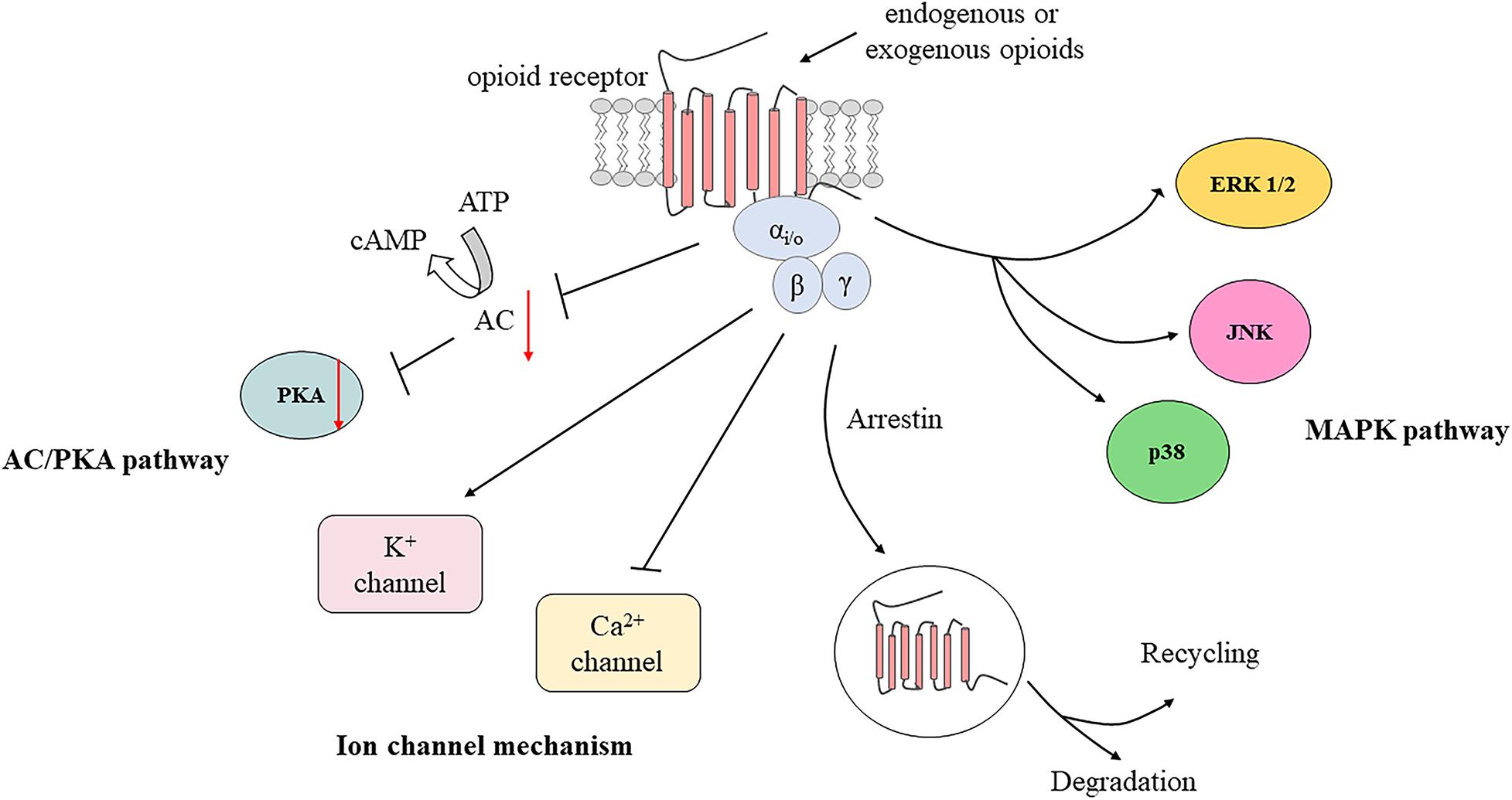
Figure 2. Activation of peripheral opioid receptors by endogenous and exogenous opioids. AC, adenylyl cyclase; cAMP, cyclic adenosine monophosphate; PKA, protein kinase A; ERK 1/2, extracellular signal regulated kinase 1 and 2; JNK, c-Jun N-terminal kinase; MAPK, mitogen-activated protein kinase.
Furthermore, exogenous opioid agonists injected close to peripheral nerves can produce analgesia by activating opioid receptors on neurons and immune cells (Plein and Rittner, 2018). Intriguingly, the activation of opioid receptors in leucocytes also induces the release of EOPs (Celik et al., 2016). Leucocyte-derived opioid peptides, in turn, induce analgesia by binding to opioid receptors on peripheral nerve sensory terminals. Therefore, the development of novel therapeutic strategies based on this neuron-immune cell analgesic pathway is important for orofacial pain treatment.
Strategies for Orofacial Pain Control
Currently, medications for orofacial pain mainly include tricyclic antidepressants, serotonin–noradrenaline reuptake inhibitors, opioids, and non-steroidal anti-inflammatory drugs (Clark et al., 2016). However, these therapeutic agents are often ineffective in relieving pain and are associated with various adverse effects, such as respiratory depression, constipation, cardiotoxicity, and dizziness (Fornasari, 2017; Hossain et al., 2020). Undoubtedly, opioids are the most potent drugs for moderate-to-severe pain control (Wiffen et al., 2017), and drugs based on PORs are effective for pain relief without central side effects. Studies have reported that peripherally acting opioids significantly attenuate inflammatory and neuropathic pain (Tiwari et al., 2016, 2018; Bruce et al., 2019). Notably, peripherally acting opioids attenuate pain involving the dopaminergic and endocannabinoid systems, which require further intensive study (Zubrzycki et al., 2019; Vaidya et al., 2021). Interactions between immune cell-derived EOPs and PORs also provide novel insights into pharmaceutical development and alternative strategies for orofacial pain treatment. There are a range of approaches that can be used to enhance peripheral opioid analgesia based on EOPs and PORs in the orofacial region.
Targeting EOPs in Immune Cells
It would be highly desirable to identify strategies for augmenting the production and release of opioid peptides in inflamed and damaged tissues. First, gene therapy is expected to an effective way to achieve long-term expression of EOPs. Administration of virus-based vectors expressing PENK into TG and whisker pad increases enkephalin expression and then produces analgesic effects in orofacial neuropathic and muscle pain models (Meunier et al., 2005; Tzabazis et al., 2014; Kramer et al., 2015; Ma et al., 2016; Meidahl et al., 2017). Common-employed vectors consist of plasmids, non-replicating adenoviruses, adeno-associated viruses, herpes simplex virus (HSV), and non-plasmid and non-viral DNA vectors (Machelska and Celik, 2018). Similarly, non-viral delivery of Oprm1 into the cancer microenvironment produces endogenous analgesia through the secretion of β-endorphin in a preclinical oral cancer model (Yamano et al., 2017). Second, it is very important to enhance opioid production and release EOPs from immune cells into orofacial inflamed/injured tissue (Macedo et al., 2016; Scheff et al., 2019). Although inhibition of immune responses alleviates pain by decreasing inflammatory mediators at the beginning of inflammation or neuropathy, immunosuppressive interventions may exacerbate pain when peripheral inflammation and pain are already established (Machelska, 2011; Machelska and Celik, 2020). Therefore, it is significant to enhance the production and release of EOPs. It’s worth noting that anti-adhesion and anti-chemokine treatments may exacerbate pain due to the vital roles of adhesion molecules and chemokines in the migration of opioid-containing immune cells to injured tissue and the release of EOPs in inflamed tissue (Busch-Dienstfertig and Stein, 2010). Additionally, the application of enkephalinase inhibitors, such as neprilysin and aminopeptidase N, will effectively increase the duration and magnitude of analgesia by preventing released opioid peptide degradation (Carbone and Poole, 2020). How to translate these findings into clinical therapies for orofacial pain requires further investigation.
Targeting PORs in Inflamed/Injured Tissue
The development of peripherally restricted opioid agonists has become a research hotspot for chronic pain treatment. Peripheral acting opioid receptor agonists have been reported to alleviate visceral pain and spinal nerve injury at pre-clinical level (Guan et al., 2008; Arendt-Nielsen et al., 2009). A mixed opioid DN-9 (Tyr-D.Ala-Gly-NMe.Phe-Gly-Pro-Gln-Arg-Phe-NH2) is developed to attenuate orofacial formalin pain via MOR and KOR (Zhang et al., 2018). Chemical modification of opioid receptor agonists is very important for exerting their effects on pain control. Low PH-dependent agonists or nanocarrier-based opioids favor receptor activation in the acid environments of inflamed and damaged tissues (Machelska and Celik, 2018). NFEPP [(±)-N-(3-fluoro-1-phenethylpiperidine-250 4-yl)-N-phenyl propionamide] is a fluorinated fentanyl derivative with maximal activity at low pH, which produces analgesia by activating PORs in rat paw inflammation and surgical incision models (Spahn et al., 2017). Furthermore, positive allosteric modulators potentiate receptor signaling by binding GPCRs at distinct sites to orthosteric ligands, such as endogenous and standard exogenous ligands (Machelska and Celik, 2018; Carbone et al., 2019). Augment the synthesis and transport of opioid receptors in peripheral tissues is also helpful in promoting analgesia. Although accumulating evidence exists regarding the functional roles of these approaches in pain modulation, little has been assessed in orofacial pain models.
Conclusion
Inflammation or injury of peripheral nerves causes an increase in the expression of PORs on peripheral nerve terminals and production of immunocyte-derived EOPs. The activation of PORs by exogenous agonists or EOPs can produce analgesia in orofacial tissues without central side effects. However, investigations focused on peripheral opioid analgesia in orofacial pain are relatively fewer than those in trunk or limb pain. The precise mechanisms of analgesic effects induced by PORs and EOPs remain to be elucidated. Therefore, more animal and clinical studies are needed to identify the function and mechanisms involving PORs and EOPs in orofacial pain. The next challenges include developing peripherally restricted opioid agonists, accelerating the release of immune cell-derived EOPs to peripheral tissues, and augmenting the synthesis of opioid receptors on peripheral neurons. These findings may provide potential therapeutic strategies for the enhancement of analgesic efficacy in the treatment of orofacial pain.
Author Contributions
QL designed and drafted the manuscript and figure. LM and SY analyzed the data. FH, WF, and HH revised the manuscript. All authors read and approved the final manuscript.
Funding
This work was supported partly by the National Natural Science Foundation of China (Grant Nos. 81870737 and 81771098) and Guangdong Financial Fund for High-Caliber Hospital Construction (Grant No. 174-2018-XMZC-0001-03-0125/D-02).
Conflict of Interest
The authors declare that the research was conducted in the absence of any commercial or financial relationships that could be construed as a potential conflict of interest.
References
Aley, K. O., and Levine, J. D. (1999). Role of protein kinase A in the maintenance of inflammatory pain. J. Neurosci. 19, 2181–2186.
Arendt-Nielsen, L., Olesen, A. E., Staahl, C., Menzaghi, F., Kell, S., Wong, G. Y., et al. (2009). Analgesic efficacy of peripheral kappa-opioid receptor agonist CR665 compared to oxycodone in a multi-modal, multi-tissue experimental human pain model: selective effect on visceral pain. Anesthesiology 111, 616–624. doi: 10.1097/ALN.0b013e3181af6356
Ayoub, L. J., Seminowicz, D. A., and Moayedi, M. (2018). A meta-analytic study of experimental and chronic orofacial pain excluding headache disorders. Neuroimage Clin. 20, 901–912. doi: 10.1016/j.nicl.2018.09.018
Baamonde, A., Lastra, A., Juárez, L., García-Suárez, O., Meana, A., Hidalgo, A., et al. (2006). Endogenous beta-endorphin induces thermal analgesia at the initial stages of a murine osteosarcoma. Peptides 27, 2778–2785. doi: 10.1016/j.peptides.2006.07.004
Bagues, A., Martin, M. I., Higuera-Matas, A., Esteban-Hernandez, J., Ambrosio, E., and Sanchez-Robles, E. M. (2018). Mu-Opioid receptors in ganglia, but not in muscle, mediate peripheral analgesia in rat muscle pain. Anesth. Analg. 126, 1369–1376. doi: 10.1213/ANE.0000000000002717
Bai, X., Zhang, X., Li, Y., Lu, L., Li, B., and He, X. (2015). Sex differences in peripheral mu-opioid receptor mediated analgesia in rat orofacial persistent pain model. PLoS One 10:e0122924. doi: 10.1371/journal.pone.0122924
Basso, L., Bourreille, A., and Dietrich, G. (2015). Intestinal inflammation and pain management. Curr. Opin. Pharmacol. 25, 50–55. doi: 10.1016/j.coph.2015.11.004
Basso, L., Garnier, L., Bessac, A., Boue, J., Blanpied, C., Cenac, N., et al. (2018). T-lymphocyte-derived enkephalins reduce Th1/Th17 colitis and associated pain in mice. J. Gastroenterol. 53, 215–226. doi: 10.1007/s00535-017-1341-2
Beaudry, H., Dubois, D., and Gendron, L. (2011). Activation of spinal mu- and delta-opioid receptors potently inhibits substance P release induced by peripheral noxious stimuli. J. Neurosci. 31, 13068–13077. doi: 10.1523/JNEUROSCI.1817-11.2011
Benoliel, R., and Sharav, Y. (2010). Chronic orofacial pain. Curr. Pain Headache Rep. 14, 33–40. doi: 10.1007/s11916-009-0085-y
Binder, W., Mousa, S. A., Sitte, N., Kaiser, M., Stein, C., and Schafer, M. (2004). Sympathetic activation triggers endogenous opioid release and analgesia within peripheral inflamed tissue. Eur. J. Neurosci. 20, 92–100. doi: 10.1111/j.1460-9568.2004.03459.x
Boue, J., Blanpied, C., Brousset, P., Vergnolle, N., and Dietrich, G. (2011). Endogenous opioid-mediated analgesia is dependent on adaptive T cell response in mice. J. Immunol. 186, 5078–5084. doi: 10.4049/jimmunol.1003335
Brack, A., Labuz, D., Schiltz, A., Rittner, H. L., Machelska, H., Schafer, M., et al. (2004a). Tissue monocytes/macrophages in inflammation: hyperalgesia versus opioid-mediated peripheral antinociception. Anesthesiology 101, 204–211. doi: 10.1097/00000542-200407000-00031
Brack, A., Rittner, H. L., Machelska, H., Leder, K., Mousa, S. A., Schafer, M., et al. (2004b). Control of inflammatory pain by chemokine-mediated recruitment of opioid-containing polymorphonuclear cells. Pain 112, 229–238. doi: 10.1016/j.pain.2004.08.029
Bruce, D. J., Peterson, C. D., Kitto, K. F., Akgun, E., Lazzaroni, S., Portoghese, P. S., et al. (2019). Combination of a delta-opioid receptor agonist and loperamide produces peripherally-mediated analgesic synergy in mice. Anesthesiology 131, 649–663. doi: 10.1097/ALN.0000000000002840
Brust, T. F., Conley, J. M., and Watts, V. J. (2015). Galpha(i/o)-coupled receptor-mediated sensitization of adenylyl cyclase: 40 years later. Eur. J. Pharmacol. 763(Pt B), 223–232. doi: 10.1016/j.ejphar.2015.05.014
Brust, T. F., Morgenweck, J., Kim, S. A., Rose, J. H., Locke, J. L., Schmid, C. L., et al. (2016). Biased agonists of the kappa opioid receptor suppress pain and itch without causing sedation or dysphoria. Sci. Signal. 9:ra117. doi: 10.1126/scisignal.aai8441
Busch-Dienstfertig, M., Labuz, D., Wolfram, T., Vogel, N. N., and Stein, C. (2012). JAK-STAT1/3-induced expression of signal sequence-encoding proopiomelanocortin mRNA in lymphocytes reduces inflammatory pain in rats. Mol. Pain 8:83. doi: 10.1186/1744-8069-8-83
Busch-Dienstfertig, M., and Stein, C. (2010). Opioid receptors and opioid peptide-producing leukocytes in inflammatory pain–basic and therapeutic aspects. Brain Behav. Immun. 24, 683–694. doi: 10.1016/j.bbi.2009.10.013
Cabot, P. J., Carter, L., Gaiddon, C., Zhang, Q., Schafer, M., Loeffler, J. P., et al. (1997). Immune cell-derived beta-endorphin. Production, release, and control of inflammatory pain in rats. J. Clin. Invest. 100, 142–148. doi: 10.1172/JCI119506
Cabot, P. J., Carter, L., Schafer, M., and Stein, C. (2001). Methionine-enkephalin-and Dynorphin A-release from immune cells and control of inflammatory pain. Pain 93, 207–212. doi: 10.1016/s0304-3959(01)00322-0
Capuano, A., De Corato, A., Treglia, M., Tringali, G., Curro, D., Dello Russo, C., et al. (2010). Peripheral antinociceptive effects of low doses of naloxone in an in vivo and in vitro model of trigeminal nociception. Neuropharmacology 58, 784–792. doi: 10.1016/j.neuropharm.2009.12.012
Carbone, S. E., and Poole, D. P. (2020). Inflammation without pain: Immune-derived opioids hold the key. Neurogastroenterol. Motil. 32:e13787. doi: 10.1111/nmo.13787
Carbone, S. E., Veldhuis, N. A., Gondin, A. B., and Poole, D. P. (2019). G protein-coupled receptor trafficking and signaling: new insights into the enteric nervous system. Am. J. Physiol. Gastrointest. Liver Physiol. 316, G446–G452. doi: 10.1152/ajpgi.00406.2018
Celik, M., Labuz, D., Henning, K., Busch-Dienstfertig, M., Gaveriaux-Ruff, C., Kieffer, B. L., et al. (2016). Leukocyte opioid receptors mediate analgesia via Ca(2+)-regulated release of opioid peptides. Brain Behav. Immun. 57, 227–242. doi: 10.1016/j.bbi.2016.04.018
Chan, H. C. S., McCarthy, D., Li, J., Palczewski, K., and Yuan, S. (2017). Designing Safer analgesics via Mu-opioid receptor pathways. Trends Pharmacol. Sci. 38, 1016–1037. doi: 10.1016/j.tips.2017.08.004
Chang, M. C., Lin, S. I., Pan, Y. H., Lin, L. D., Wang, Y. L., Yeung, S. Y., et al. (2019). IL-1beta-induced ICAM-1 and IL-8 expression/secretion of dental pulp cells is differentially regulated by IRAK and p38. J. Formos. Med. Assoc. 118, 1247–1254. doi: 10.1016/j.jfma.2018.11.015
Charbaji, N., Schafer-Korting, M., and Kuchler, S. (2012). Morphine stimulates cell migration of oral epithelial cells by delta-opioid receptor activation. PLoS One 7:e42616. doi: 10.1371/journal.pone.0042616
Chavarria-Bolanos, D., Flores-Reyes, H., Lombana-Sanchez, N., Cerda-Cristerna, B., and Pozos-Guillen, A. (2015). Sensory neuropeptides and endogenous opioids expression in human dental pulp with asymptomatic inflammation: in vivo study. Mediators Inflamm. 2015:879126. doi: 10.1155/2015/879126
Chavarría-Bolaños, D., Martinez-Zumaran, A., Lombana, N., Flores-Reyes, H., and Pozos-Guillen, A. (2014). Expression of substance P, calcitonin gene-related peptide, beta-endorphin and methionine-enkephalin in human dental pulp tissue after orthodontic intrusion: a pilot study. Angle Orthod. 84, 521–526. doi: 10.2319/060313-423.1
Chuang, L. F., Chuang, T. K., Killam, K. F. Jr., Qiu, Q., Wang, X. R., Lin, J. J., et al. (1995a). Expression of kappa opioid receptors in human and monkey lymphocytes. Biochem. Biophys. Res. Commun. 209, 1003–1010. doi: 10.1006/bbrc.1995.1597
Chuang, T. K., Killam, K. F. Jr., Chuang, L. F., Kung, H. F., Sheng, W. S., Chao, C. C., et al. (1995b). Mu opioid receptor gene expression in immune cells. Biochem. Biophys. Res. Commun. 216, 922–930. doi: 10.1006/bbrc.1995.2709
Chung, M. K., Cho, Y. S., Bae, Y. C., Lee, J., Zhang, X., and Ro, J. Y. (2014). Peripheral G protein-coupled inwardly rectifying potassium channels are involved in delta-opioid receptor-mediated anti-hyperalgesia in rat masseter muscle. Eur. J. Pain 18, 29–38. doi: 10.1002/j.1532-2149.2013.00343.x
Clark, G. T., Padilla, M., and Dionne, R. (2016). Medication treatment efficacy and chronic orofacial pain. Oral Maxillofac. Surg. Clin. North Am. 28, 409–421. doi: 10.1016/j.coms.2016.03.011
Clemente, J. T., Parada, C. A., Veiga, M. C., Gear, R. W., and Tambeli, C. H. (2004). Sexual dimorphism in the antinociception mediated by kappa opioid receptors in the rat temporomandibular joint. Neurosci. Lett. 372, 250–255. doi: 10.1016/j.neulet.2004.09.048
Clemente-Napimoga, J. T., Pellegrini-da-Silva, A., Ferreira, V. H., Napimoga, M. H., Parada, C. A., and Tambeli, C. H. (2009). Gonadal hormones decrease temporomandibular joint kappa-mediated antinociception through a down-regulation in the expression of kappa opioid receptors in the trigeminal ganglia. Eur. J. Pharmacol. 617, 41–47. doi: 10.1016/j.ejphar.2009.06.036
Coura, C. O., Chaves, H. V., do Val, D. R., Vieira, L. V., Silveira, F. D., Dos Santos Lopes, F. M., et al. (2017). Mechanisms involved in antinociception induced by a polysulfated fraction from seaweed Gracilaria cornea in the temporomandibular joint of rats. Int. J. Biol. Macromol. 97, 76–84. doi: 10.1016/j.ijbiomac.2017.01.017
Cox, B. M. (2013). Recent developments in the study of opioid receptors. Mol. Pharmacol. 83, 723–728. doi: 10.1124/mol.112.083279
Currie, K. P. (2010). G protein modulation of CaV2 voltage-gated calcium channels. Channels (Austin) 4, 497–509. doi: 10.4161/chan.4.6.12871
Dripps, I. J., Chen, R., Shafer, A. M., Livingston, K. E., Disney, A., Husbands, S. M., et al. (2020). Pharmacological properties of delta-opioid receptor-mediated behaviors: agonist efficacy and receptor reserve. J. Pharmacol. Exp. Ther. 374, 319–330. doi: 10.1124/jpet.119.262717
Fan, H., Li, T. F., Gong, N., and Wang, Y. X. (2016). Shanzhiside methylester, the principle effective iridoid glycoside from the analgesic herb Lamiophlomis rotata, reduces neuropathic pain by stimulating spinal microglial beta-endorphin expression. Neuropharmacology 101, 98–109. doi: 10.1016/j.neuropharm.2015.09.010
Feher, G., Nemeskeri, Z., Pusch, G., Zadori, I., Bank, G., Gurdan, Z., et al. (2019). [Chronic orofacial pain]. Orv. Hetil. 160, 1047–1056. doi: 10.1556/650.2019.31432
Feldreich, A., Ernberg, M., Lund, B., and Rosen, A. (2012). Increased beta-endorphin levels and generalized decreased pain thresholds in patients with limited jaw opening and movement-evoked pain from the temporomandibular joint. J. Oral Maxillofac. Surg. 70, 547–556. doi: 10.1016/j.joms.2011.09.013
Feldreich, A., Ernberg, M., and Rosen, A. (2017). Reduction in maximum pain after surgery in temporomandibular joint patients is associated with decreased beta-endorphin levels - a pilot study. Int. J. Oral Maxillofac. Surg. 46, 97–103. doi: 10.1016/j.ijom.2016.08.010
Feske, S. (2007). Calcium signalling in lymphocyte activation and disease. Nat. Rev. Immunol. 7, 690–702. doi: 10.1038/nri2152
Fornasari, D. (2017). Pharmacotherapy for neuropathic pain: a review. Pain Ther. 6(Suppl. 1) 25–33. doi: 10.1007/s40122-017-0091-4
Francois, A., and Scherrer, G. (2018). Delta opioid receptor expression and function in primary afferent somatosensory neurons. Handb. Exp. Pharmacol. 247, 87–114. doi: 10.1007/164_2017_58
Fristad, I., Berggreen, E., and Haug, S. R. (2006). Delta (delta) opioid receptors in small and medium-sized trigeminal neurons supporting the dental pulp of rats. Arch. Oral Biol. 51, 273–281. doi: 10.1016/j.archoralbio.2005.08.007
Gong, N., Xiao, Q., Zhu, B., Zhang, C. Y., Wang, Y. C., Fan, H., et al. (2014). Activation of spinal glucagon-like peptide-1 receptors specifically suppresses pain hypersensitivity. J. Neurosci. 34, 5322–5334. doi: 10.1523/JNEUROSCI.4703-13.2014
Grim, T. W., Acevedo-Canabal, A., and Bohn, L. M. (2020). Toward directing opioid receptor signaling to refine opioid therapeutics. Biol. Psychiatry 87, 15–21. doi: 10.1016/j.biopsych.2019.10.020
Guan, Y., Johanek, L. M., Hartke, T. V., Shim, B., Tao, Y. X., Ringkamp, M., et al. (2008). Peripherally acting mu-opioid receptor agonist attenuates neuropathic pain in rats after L5 spinal nerve injury. Pain 138, 318–329. doi: 10.1016/j.pain.2008.01.004
Hauser, K. F., Osborne, J. G., Stiene-Martin, A., and Melner, M. H. (1990). Cellular localization of proenkephalin mRNA and enkephalin peptide products in cultured astrocytes. Brain Res. 522, 347–353. doi: 10.1016/0006-8993(90)91482-v
Hayashi, K., Sugisaiki, M., Ota, S., and Tanabe, H. (2002). mu-Opioid receptor mRNA expression and immunohistochemical localization in the rat temporomandibular joint. Peptides 23, 889–893. doi: 10.1016/s0196-9781(02)00015-3
Helal, M. A., Habib, E. S., and Chittiboyina, A. G. (2017). Selective kappa opioid antagonists for treatment of addiction, are we there yet? Eur. J. Med. Chem. 141, 632–647. doi: 10.1016/j.ejmech.2017.10.012
Hossain, M. Z., Ando, H., Unno, S., and Kitagawa, J. (2020). Targeting peripherally restricted cannabinoid receptor 1, cannabinoid receptor 2, and endocannabinoid-degrading enzymes for the treatment of neuropathic pain including neuropathic orofacial pain. Int. J. Mol. Sci. 21:1423. doi: 10.3390/ijms21041423
Hua, S. (2016). Neuroimmune interaction in the regulation of peripheral opioid-mediated analgesia in inflammation. Front. Immunol. 7:293. doi: 10.3389/fimmu.2016.00293
Hua, S., and Cabot, P. J. (2010). Mechanisms of peripheral immune-cell-mediated analgesia in inflammation: clinical and therapeutic implications. Trends Pharmacol. Sci. 31, 427–433. doi: 10.1016/j.tips.2010.05.008
Hua, S., Hermanussen, S., Tang, L., Monteith, G. R., and Cabot, P. J. (2006). The neural cell adhesion molecule antibody blocks cold water swim stress-induced analgesia and cell adhesion between lymphocytes and cultured dorsal root ganglion neurons. Anesth. Analg. 103, 1558–1564. doi: 10.1213/01.ane.0000243410.61451.c1
Huang, J., Lv, Y., Fu, Y., Ren, L., Wang, P., Liu, B., et al. (2015). Dynamic regulation of delta-opioid receptor in rat trigeminal ganglion neurons by lipopolysaccharide-induced acute pulpitis. J. Endod. 41, 2014–2020. doi: 10.1016/j.joen.2015.09.011
Huang, Q., Mao, X. F., Wu, H. Y., Liu, H., Sun, M. L., Wang, X., et al. (2017). Cynandione A attenuates neuropathic pain through p38beta MAPK-mediated spinal microglial expression of beta-endorphin. Brain Behav. Immun. 62, 64–77. doi: 10.1016/j.bbi.2017.02.005
Iwaszkiewicz, K. S., Schneider, J. J., and Hua, S. (2013). Targeting peripheral opioid receptors to promote analgesic and anti-inflammatory actions. Front. Pharmacol. 4:132. doi: 10.3389/fphar.2013.00132
Jaber, L., Swaim, W. D., and Dionne, R. A. (2003). Immunohistochemical localization of mu-opioid receptors in human dental pulp. J. Endod. 29, 108–110. doi: 10.1097/00004770-200302000-00005
Jiang, Y. L., He, X. F., Shen, Y. F., Yin, X. H., Du, J. Y., Liang, Y. I., et al. (2015). Analgesic roles of peripheral intrinsic met-enkephalin and dynorphin A in long-lasting inflammatory pain induced by complete Freund’s adjuvant in rats. Exp. Ther. Med. 9, 2344–2348. doi: 10.3892/etm.2015.2407
Jiao, X., Wang, X., Wang, R., Geng, J., Liu, N., Chen, H., et al. (2018). Rules to activate CD8(+)T cells through regulating subunits of opioid receptors by methionine enkephalin (MENK). Int. Immunopharmacol. 65, 76–83. doi: 10.1016/j.intimp.2018.09.040
Jin, Y. H., Nishioka, H., Wakabayashi, K., Fujita, T., and Yonehara, N. (2006). Effect of morphine on the release of excitatory amino acids in the rat hind instep: Pain is modulated by the interaction between the peripheral opioid and glutamate systems. Neuroscience 138, 1329–1339. doi: 10.1016/j.neuroscience.2005.12.049
Jutkiewicz, E. M., Rice, K. C., Traynor, J. R., and Woods, J. H. (2005). Separation of the convulsions and antidepressant-like effects produced by the delta-opioid agonist SNC80 in rats. Psychopharmacology (Berl.) 182, 588–596. doi: 10.1007/s00213-005-0138-9
Kang, S. K., Lee, Y. H., Park, H., Ro, J. Y., and Auh, Q. S. (2018). Effects of intramuscular morphine in men and women with temporomandibular disorder with myofascial pain. Oral Dis. 24, 1591–1598. doi: 10.1111/odi.12919
Kokuba, S., Takehana, S., Oshima, K., Shimazu, Y., and Takeda, M. (2017). Systemic administration of the dietary constituent resveratrol inhibits the nociceptive jaw-opening reflex in rats via the endogenous opioid system. Neurosci. Res. 119, 1–6. doi: 10.1016/j.neures.2017.01.005
Kramer, P. R., Umorin, M., and Bellinger, L. L. (2015). Attenuation of myogenic orofacial nociception and mechanical hypersensitivity by viral mediated enkephalin overproduction in male and female rats. BMC Neurol. 15:34. doi: 10.1186/s12883-015-0285-5
Kurose, M., Imbe, H., Nakatani, Y., Hasegawa, M., Fujii, N., Takagi, R., et al. (2017). Bilateral increases in ERK activation at the spinomedullary junction region by acute masseter muscle injury during temporomandibular joint inflammation in the rats. Exp. Brain Res. 235, 913–921. doi: 10.1007/s00221-016-4852-9
Labuz, D., Schmidt, Y., Schreiter, A., Rittner, H. L., Mousa, S. A., and Machelska, H. (2009). Immune cell-derived opioids protect against neuropathic pain in mice. J. Clin. Invest. 119, 278–286. doi: 10.1172/JCI36246
Labuz, D., Schreiter, A., Schmidt, Y., Brack, A., and Machelska, H. (2010). T lymphocytes containing beta-endorphin ameliorate mechanical hypersensitivity following nerve injury. Brain Behav. Immun. 24, 1045–1053. doi: 10.1016/j.bbi.2010.04.001
Law, P. Y., Reggio, P. H., and Loh, H. H. (2013). Opioid receptors: toward separation of analgesic from undesirable effects. Trends Biochem. Sci. 38, 275–282. doi: 10.1016/j.tibs.2013.03.003
Lee, K. S., Zhang, Y., Asgar, J., Auh, Q. S., Chung, M. K., and Ro, J. Y. (2016). Androgen receptor transcriptionally regulates mu-opioid receptor expression in rat trigeminal ganglia. Neuroscience 331, 52–61. doi: 10.1016/j.neuroscience.2016.06.023
Ley, K., Laudanna, C., Cybulsky, M. I., and Nourshargh, S. (2007). Getting to the site of inflammation: the leukocyte adhesion cascade updated. Nat. Rev. Immunol. 7, 678–689. doi: 10.1038/nri2156
Likar, R., Koppert, W., Blatnig, H., Chiari, F., Sittl, R., Stein, C., et al. (2001). Efficacy of peripheral morphine analgesia in inflamed, non-inflamed and perineural tissue of dental surgery patients. J. Pain Symptom Manage. 21, 330–337. doi: 10.1016/s0885-3924(01)00251-2
Liu, Q., Fan, W., He, H., and Huang, F. (2020). The role of peripheral opioid receptors in orofacial pain. Oral Dis. doi: 10.1111/odi.13435 [Epub ahead of print].
Liu, S., Liu, L., Jiang, Y., Zhou, J., Hu, H., Wu, Z., et al. (2019). Effect of endomorphin-2 on orofacial pain induced by orthodontic tooth movement in rats. Eur. J. Oral Sci. 127, 408–416. doi: 10.1111/eos.12640
Ma, F., Wang, C., Yoder, W. E., Westlund, K. N., Carlson, C. R., Miller, C. S., et al. (2016). Efficacy of herpes simplex virus vector encoding the human preproenkephalin gene for treatment of facial pain in mice. J. Oral Facial Pain Headache 30, 42–50. doi: 10.11607/ofph.1512
Macedo, C. G., Napimoga, M. H., Rocha-Neto, L. M., Abdalla, H. B., and Clemente-Napimoga, J. T. (2016). The role of endogenous opioid peptides in the antinociceptive effect of 15-deoxy(Delta12,14)-prostaglandin J2 in the temporomandibular joint. Prostaglandins Leukot. Essent. Fatty Acids 110, 27–34. doi: 10.1016/j.plefa.2016.05.009
Machelska, H. (2007). Targeting of opioid-producing leukocytes for pain control. Neuropeptides 41, 355–363. doi: 10.1016/j.npep.2007.06.001
Machelska, H. (2011). Dual peripheral actions of immune cells in neuropathic pain. Arch. Immunol. Ther. Exp. (Warsz) 59, 11–24. doi: 10.1007/s00005-010-0106-x
Machelska, H., Brack, A., Mousa, S. A., Schopohl, J. K., Rittner, H. L., Schafer, M., et al. (2004). Selectins and integrins but not platelet-endothelial cell adhesion molecule-1 regulate opioid inhibition of inflammatory pain. Br. J. Pharmacol. 142, 772–780. doi: 10.1038/sj.bjp.0705837
Machelska, H., and Celik, M. O. (2018). Advances in achieving opioid analgesia without side effects. Front. Pharmacol. 9:1388. doi: 10.3389/fphar.2018.01388
Machelska, H., and Celik, M. O. (2020). Opioid receptors in immune and glial cells-implications for pain control. Front. Immunol. 11:300. doi: 10.3389/fimmu.2020.00300
Machelska, H., Mousa, S. A., Brack, A., Schopohl, J. K., Rittner, H. L., Schafer, M., et al. (2002). Opioid control of inflammatory pain regulated by intercellular adhesion molecule-1. J. Neurosci. 22, 5588–5596. doi: 10.1523/JNEUROSCI.22-13-05588.2002
Machelska, H., and Stein, C. (2006). Leukocyte-derived opioid peptides and inhibition of pain. J. Neuroimmune Pharmacol. 1, 90–97. doi: 10.1007/s11481-005-9002-2
Mafra, C., Vasconcelos, R. C., de Medeiros, C., Leitao, R. F. C., Brito, G. A. C., Costa, D., et al. (2019). Gliclazide prevents 5-FU-induced oral mucositis by reducing oxidative stress, inflammation, and P-selectin adhesion molecules. Front. Physiol. 10:327. doi: 10.3389/fphys.2019.00327
Mao, X. F., Wu, H. Y., Tang, X. Q., Ali, U., Liu, H., and Wang, Y. X. (2019). Activation of GPR40 produces mechanical antiallodynia via the spinal glial interleukin-10/beta-endorphin pathway. J. Neuroinflammation 16:84. doi: 10.1186/s12974-019-1457-9
Matsuda, M., Huh, Y., and Ji, R. R. (2019). Roles of inflammation, neurogenic inflammation, and neuroinflammation in pain. J. Anesth. 33, 131–139. doi: 10.1007/s00540-018-2579-4
Meidahl, A. C., Klukinov, M., Tzabazis, A. Z., Sorensen, J. C., and Yeomans, D. C. (2017). Nasal application of HSV encoding human preproenkephalin blocks craniofacial pain in a rat model of traumatic brain injury. Gene Ther. 24, 482–486. doi: 10.1038/gt.2017.55
Meunier, A., Latremoliere, A., Mauborgne, A., Bourgoin, S., Kayser, V., Cesselin, F., et al. (2005). Attenuation of pain-related behavior in a rat model of trigeminal neuropathic pain by viral-driven enkephalin overproduction in trigeminal ganglion neurons. Mol. Ther. 11, 608–616. doi: 10.1016/j.ymthe.2004.12.011
Moore, C., Gupta, R., Jordt, S. E., Chen, Y., and Liedtke, W. B. (2018). Regulation of pain and itch by TRP channels. Neurosci. Bull. 34, 120–142. doi: 10.1007/s12264-017-0200-8
Moreau, N., Dieb, W., Mauborgne, A., Bourgoin, S., Villanueva, L., Pohl, M., et al. (2017). Hedgehog pathway-mediated vascular alterations following trigeminal nerve injury. J. Dent. Res. 96, 450–457. doi: 10.1177/0022034516679395
Mousa, S. A., Machelska, H., Schafer, M., and Stein, C. (2000). Co-expression of beta-endorphin with adhesion molecules in a model of inflammatory pain. J. Neuroimmunol. 108, 160–170. doi: 10.1016/s0165-5728(00)00284-8
Mousa, S. A., Shakibaei, M., Sitte, N., Schäfer, M., and Stein, C. (2004). Subcellular pathways of beta-endorphin synthesis, processing, and release from immunocytes in inflammatory pain. Endocrinology 145, 1331–1341. doi: 10.1210/en.2003-1287
Mousa, S. A., Straub, R. H., Schafer, M., and Stein, C. (2007). Beta-endorphin, Met-enkephalin and corresponding opioid receptors within synovium of patients with joint trauma, osteoarthritis and rheumatoid arthritis. Ann. Rheum. Dis. 66, 871–879. doi: 10.1136/ard.2006.067066
Napimoga, M. H., Souza, G. R., Cunha, T. M., Ferrari, L. F., Clemente-Napimoga, J. T., Parada, C. A., et al. (2008). 15d-prostaglandin J2 inhibits inflammatory hypernociception: involvement of peripheral opioid receptor. J. Pharmacol. Exp. Ther. 324, 313–321. doi: 10.1124/jpet.107.126045
Nielsen, B. N., Aagaard, G., Henneberg, S. W., Schmiegelow, K., Hansen, S. H., and Romsing, J. (2012). Topical morphine for oral mucositis in children: dose finding and absorption. J. Pain Symptom Manage. 44, 117–123. doi: 10.1016/j.jpainsymman.2011.06.029
Niu, K., Saloman, J. L., Zhang, Y., and Ro, J. Y. (2011). Sex differences in the contribution of ATP-sensitive K+ channels in trigeminal ganglia under an acute muscle pain condition. Neuroscience 180, 344–352. doi: 10.1016/j.neuroscience.2011.01.045
Nockemann, D., Rouault, M., Labuz, D., Hublitz, P., McKnelly, K., Reis, F. C., et al. (2013). The K(+) channel GIRK2 is both necessary and sufficient for peripheral opioid-mediated analgesia. EMBO Mol. Med. 5, 1263–1277. doi: 10.1002/emmm.201201980
Nunez, S., Lee, J. S., Zhang, Y., Bai, G., and Ro, J. Y. (2007). Role of peripheral mu-opioid receptors in inflammatory orofacial muscle pain. Neuroscience 146, 1346–1354. doi: 10.1016/j.neuroscience.2007.02.024
Obata, K., and Noguchi, K. (2004). MAPK activation in nociceptive neurons and pain hypersensitivity. Life Sci. 74, 2643–2653. doi: 10.1016/j.lfs.2004.01.007
Pannell, M., Labuz, D., Celik, M. O., Keye, J., Batra, A., Siegmund, B., et al. (2016). Adoptive transfer of M2 macrophages reduces neuropathic pain via opioid peptides. J. Neuroinflammation 13:262. doi: 10.1186/s12974-016-0735-z
Patwardhan, A. M., Berg, K. A., Akopain, A. N., Jeske, N. A., Gamper, N., Clarke, W. P., et al. (2005). Bradykinin-induced functional competence and trafficking of the delta-opioid receptor in trigeminal nociceptors. J. Neurosci. 25, 8825–8832. doi: 10.1523/jneurosci.0160-05.2005
Patwardhan, A. M., Diogenes, A., Berg, K. A., Fehrenbacher, J. C., Clarke, W. P., Akopian, A. N., et al. (2006). PAR-2 agonists activate trigeminal nociceptors and induce functional competence in the delta opioid receptor. Pain 125, 114–124. doi: 10.1016/j.pain.2006.05.007
Pettinger, L., Gigout, S., Linley, J. E., and Gamper, N. (2013). Bradykinin controls pool size of sensory neurons expressing functional delta-opioid receptors. J. Neurosci. 33, 10762–10771. doi: 10.1523/JNEUROSCI.0123-13.2013
Plein, L. M., and Rittner, H. L. (2018). Opioids and the immune system - friend or foe. Br. J. Pharmacol. 175, 2717–2725. doi: 10.1111/bph.13750
Rittner, H. L., Amasheh, S., Moshourab, R., Hackel, D., Yamdeu, R. S., Mousa, S. A., et al. (2012). Modulation of tight junction proteins in the perineurium to facilitate peripheral opioid analgesia. Anesthesiology 116, 1323–1334. doi: 10.1097/ALN.0b013e318256eeeb
Rittner, H. L., Brack, A., Machelska, H., Mousa, S. A., Bauer, M., Schäfer, M., et al. (2001). Opioid peptide-expressing leukocytes: identification, recruitment, and simultaneously increasing inhibition of inflammatory pain. Anesthesiology 95, 500–508. doi: 10.1097/00000542-200108000-00036
Rittner, H. L., Brack, A., and Stein, C. (2008). Pain and the immune system. Br. J. Anaesth. 101, 40–44. doi: 10.1093/bja/aen078
Rittner, H. L., Hackel, D., Voigt, P., Mousa, S., Stolz, A., Labuz, D., et al. (2009a). Mycobacteria attenuate nociceptive responses by formyl peptide receptor triggered opioid peptide release from neutrophils. PLoS Pathog. 5:e1000362. doi: 10.1371/journal.ppat.1000362
Rittner, H. L., Hackel, D., Yamdeu, R. S., Mousa, S. A., Stein, C., Schafer, M., et al. (2009b). Antinociception by neutrophil-derived opioid peptides in noninflamed tissue–role of hypertonicity and the perineurium. Brain Behav. Immun. 23, 548–557. doi: 10.1016/j.bbi.2009.02.007
Rittner, H. L., Labuz, D., Richter, J. F., Brack, A., Schafer, M., Stein, C., et al. (2007). CXCR1/2 ligands induce p38 MAPK-dependent translocation and release of opioid peptides from primary granules in vitro and in vivo. Brain Behav. Immun. 21, 1021–1032. doi: 10.1016/j.bbi.2007.05.002
Rittner, H. L., Labuz, D., Schaefer, M., Mousa, S. A., Schulz, S., Schäfer, M., et al. (2006a). Pain control by CXCR2 ligands through Ca2+-regulated release of opioid peptides from polymorphonuclear cells. FASEB J. 20, 2627–2629. doi: 10.1096/fj.06-6077fje
Rittner, H. L., Mousa, S. A., Labuz, D., Beschmann, K., Schäfer, M., Stein, C., et al. (2006b). Selective local PMN recruitment by CXCL1 or CXCL2/3 injection does not cause inflammatory pain. J. Leukoc. Biol. 79, 1022–1032. doi: 10.1189/jlb.0805452
Rittner, H. L., and Stein, C. (2005). Involvement of cytokines, chemokines and adhesion molecules in opioid analgesia. Eur. J. Pain 9, 109–112. doi: 10.1016/j.ejpain.2004.05.009
Rutz, J. C., Hatton, J. F., Hildebolt, C., Wells, J. E., and Rowland, K. C. (2007). Localized increases in corticotropin-releasing factor receptors in pulp after dental injury. J. Endod. 33, 1319–1324. doi: 10.1016/j.joen.2007.08.009
Saloman, J. L., Niu, K. Y., and Ro, J. Y. (2011). Activation of peripheral delta-opioid receptors leads to anti-hyperalgesic responses in the masseter muscle of male and female rats. Neuroscience 190, 379–385. doi: 10.1016/j.neuroscience.2011.05.062
Sanchez, E. M., Bagues, A., and Martin, M. I. (2010). Contributions of peripheral and central opioid receptors to antinociception in rat muscle pain models. Pharmacol. Biochem. Behav. 96, 488–495. doi: 10.1016/j.pbb.2010.07.009
Savernini, A., Savernini, N., de Amaral, F. A., Romero, T. R., Duarte, I. D., and de Castro, M. S. (2012). Assay of therapeutic ultrasound induced-antinociception in experimental trigeminal neuropathic pain. J. Neurosci. Res. 90, 1639–1645. doi: 10.1002/jnr.23056
Schafer, M., Carter, L., and Stein, C. (1994). Interleukin 1 beta and corticotropin-releasing factor inhibit pain by releasing opioids from immune cells in inflamed tissue. Proc. Natl. Acad. Sci. U.S.A. 91, 4219–4223. doi: 10.1073/pnas.91.10.4219
Scheff, N. N., Alemu, R. G., Klares, R. III, Wall, I. M., Yang, S. C., Dolan, J. C., et al. (2019). Granulocyte-colony stimulating factor-induced neutrophil recruitment provides opioid-mediated endogenous anti-nociception in female mice with oral squamous cell carcinoma. Front. Mol. Neurosci. 12:217. doi: 10.3389/fnmol.2019.00217
Scheff, N. N., Bhattacharya, A., Dowse, E., Dang, R. X., Dolan, J. C., Wang, S., et al. (2018). Neutrophil-mediated endogenous analgesia contributes to sex differences in oral cancer pain. Front. Integr. Neurosci. 12:52. doi: 10.3389/fnint.2018.00052
Schmitt, T. K., Mousa, S. A., Brack, A., Schmidt, D. K., Rittner, H. L., Welte, M., et al. (2003). Modulation of peripheral endogenous opioid analgesia by central afferent blockade. Anesthesiology 98, 195–202. doi: 10.1097/00000542-200301000-00030
Sehgal, N., Smith, H. S., and Manchikanti, L. (2011). Peripherally acting opioids and clinical implications for pain control. Pain Phys. 14, 249–258.
Sessle, B. J. (2011). Peripheral and central mechanisms of orofacial inflammatory pain. Int. Rev. Neurobiol. 97, 179–206. doi: 10.1016/B978-0-12-385198-7.00007-2
Shinoda, H., Marini, A. M., Cosi, C., and Schwartz, J. P. (1989). Brain region and gene specificity of neuropeptide gene expression in cultured astrocytes. Science 245, 415–417. doi: 10.1126/science.2569236
Shiwarski, D. J., Darr, M., Telmer, C. A., Bruchez, M. P., and Puthenveedu, M. A. (2017). PI3K class II alpha regulates delta-opioid receptor export from the trans-Golgi network. Mol. Biol. Cell 28, 2202–2219. doi: 10.1091/mbc.E17-01-0030
Snyder, L. M., Chiang, M. C., Loeza-Alcocer, E., Omori, Y., Hachisuka, J., Sheahan, T. D., et al. (2018). Kappa opioid receptor distribution and function in primary afferents. Neuron 99, 1274–1288.e6. doi: 10.1016/j.neuron.2018.08.044
Sobczak, M., Salaga, M., Storr, M. A., and Fichna, J. (2014). Physiology, signaling, and pharmacology of opioid receptors and their ligands in the gastrointestinal tract: current concepts and future perspectives. J. Gastroenterol. 49, 24–45. doi: 10.1007/s00535-013-0753-x
Spahn, V., Del Vecchio, G., Labuz, D., Rodriguez-Gaztelumendi, A., Massaly, N., Temp, J., et al. (2017). A nontoxic pain killer designed by modeling of pathological receptor conformations. Science 355, 966–969. doi: 10.1126/science.aai8636
Stein, C. (2016). Opioid Receptors. Annu. Rev. Med. 67, 433–451. doi: 10.1146/annurev-med-062613-093100
Stein, C. (2018). New concepts in opioid analgesia. Expert Opin. Investig. Drugs 27, 765–775. doi: 10.1080/13543784.2018.1516204
Stein, C., and Machelska, H. (2011). Modulation of peripheral sensory neurons by the immune system: implications for pain therapy. Pharmacol. Rev. 63, 860–881. doi: 10.1124/pr.110.003145
Stein, C., Schäfer, M., and Machelska, H. (2003). Attacking pain at its source: new perspectives on opioids. Nat. Med. 9, 1003–1008. doi: 10.1038/nm908
Stevens, C. W. (2015). Bioinformatics and evolution of vertebrate nociceptin and opioid receptors. Vitam. Horm. 97, 57–94. doi: 10.1016/bs.vh.2014.10.002
Stiene-Martin, A., Knapp, P. E., Martin, K., Gurwell, J. A., Ryan, S., Thornton, S. R., et al. (2001). Opioid system diversity in developing neurons, astroglia, and oligodendroglia in the subventricular zone and striatum: impact on gliogenesis in vivo. Glia 36, 78–88.
Tiwari, V., Anderson, M., Yang, F., Tiwari, V., Zheng, Q., He, S. Q., et al. (2018). Peripherally acting mu-Opioid receptor agonists attenuate ongoing pain-associated behavior and spontaneous neuronal activity after nerve injury in rats. Anesthesiology 128, 1220–1236. doi: 10.1097/ALN.0000000000002191
Tiwari, V., Yang, F., He, S. Q., Shechter, R., Zhang, C., Shu, B., et al. (2016). Activation of peripheral mu-opioid receptors by dermorphin [D-Arg2, Lys4] (1-4) amide leads to modality-preferred inhibition of neuropathic pain. Anesthesiology 124, 706–720. doi: 10.1097/ALN.0000000000000993
Tzabazis, A. Z., Klukinov, M., Feliciano, D. P., Wilson, S. P., and Yeomans, D. C. (2014). Gene therapy for trigeminal pain in mice. Gene Ther. 21, 422–426. doi: 10.1038/gt.2014.14
Uhrich, E., Gautam, M., Hatton, J., and Rowland, K. (2015). Corticotropin releasing factor receptor expression in painful human dental pulp. Arch. Oral Biol. 60, 1048–1053. doi: 10.1016/j.archoralbio.2015.03.008
Vaidya, S., Shantanu, P. A., and Tiwari, V. (2021). Attenuation of ongoing neuropathic pain by peripheral acting opioid involves activation of central dopaminergic neurocircuitry. Neurosci. Lett. doi: 10.1016/j.neulet.2021.135751 [Epub ahead of print].
Vetter, I., Kapitzke, D., Hermanussen, S., Monteith, G. R., and Cabot, P. J. (2006). The effects of pH on beta-endorphin and morphine inhibition of calcium transients in dorsal root ganglion neurons. J. Pain 7, 488–499. doi: 10.1016/j.jpain.2006.01.456
Vicente-Sanchez, A., Segura, L., and Pradhan, A. A. (2016). The delta opioid receptor tool box. Neuroscience 338, 145–159. doi: 10.1016/j.neuroscience.2016.06.028
Vilijn, M. H., Vaysse, P. J., Zukin, R. S., and Kessler, J. A. (1988). Expression of preproenkephalin mRNA by cultured astrocytes and neurons. Proc. Natl. Acad. Sci. U.S.A. 85, 6551–6555. doi: 10.1073/pnas.85.17.6551
Wang, H. B., Zhao, B., Zhong, Y. Q., Li, K. C., Li, Z. Y., Wang, Q., et al. (2010). Coexpression of delta- and mu-opioid receptors in nociceptive sensory neurons. Proc. Natl. Acad. Sci. U.S.A. 107, 13117–13122. doi: 10.1073/pnas.1008382107
Wei, E. Q., Kudo, T., and Inoki, R. (1991). Subcellular distribution of enkephalin precursor proteins in rat dental pulp and the usefulness as a substrate for enkephalin-producing enzymes. Life Sci. 48, 667–674. doi: 10.1016/0024-3205(91)90542-j
Wenk, H. N., and Honda, C. N. (1999). Immunohistochemical localization of delta opioid receptors in peripheral tissues. J. Comp. Neurol. 408, 567–579. doi: 10.1002/(sici)1096-9861(19990614)408:4<567::aid-cne10<3.0.co;2-q
Wiffen, P. J., Wee, B., Derry, S., Bell, R. F., and Moore, R. A. (2017). Opioids for cancer pain – an overview of Cochrane reviews. Cochrane Database Syst. Rev. 7:CD012592. doi: 10.1002/14651858.CD012592.pub2
Wu, H. Y., Mao, X. F., Tang, X. Q., Ali, U., Apryani, E., Liu, H., et al. (2018). Spinal interleukin-10 produces antinociception in neuropathy through microglial β-endorphin expression, separated from antineuroinflammation. Brain Behav. Immun. 73, 504–519. doi: 10.1016/j.bbi.2018.06.015
Yamano, S., Viet, C. T., Dang, D., Dai, J., Hanatani, S., Takayama, T., et al. (2017). Ex vivo nonviral gene delivery of mu-opioid receptor to attenuate cancer-induced pain. Pain 158, 240–251. doi: 10.1097/j.pain.0000000000000750
Zhang, T., Zhao, W., Zhang, M., Xu, B., Shi, X., Zhang, Q., et al. (2018). Analgesic activities of the mixed opioid and NPFF receptors agonist DN-9 in a mouse model of formalin-induced orofacial inflammatory pain. Peptides 110, 30–39. doi: 10.1016/j.peptides.2018.10.010
Zhang, X., Zhang, Y., Asgar, J., Niu, K. Y., Lee, J., Lee, K. S., et al. (2014). Sex differences in mu-opioid receptor expression in trigeminal ganglia under a myositis condition in rats. Eur. J. Pain 18, 151–161. doi: 10.1002/j.1532-2149.2013.00352.x
Zhu, L., Cui, Z., Zhu, Q., Zha, X., and Xu, Y. (2018). Novel opioid receptor agonists with reduced morphine-like side effects. Mini. Rev. Med. Chem. 18, 1603–1610. doi: 10.2174/1389557518666180716124336
Keywords: peripheral opioid receptors, endogenous opioid peptides, orofacial pain, immune cells, neuroimmune
Citation: Liu Q, He H, Mai L, Yang S, Fan W and Huang F (2021) Peripherally Acting Opioids in Orofacial Pain. Front. Neurosci. 15:665445. doi: 10.3389/fnins.2021.665445
Received: 08 February 2021; Accepted: 12 April 2021;
Published: 04 May 2021.
Edited by:
Parisa Gazerani, Oslo Metropolitan University, NorwayReviewed by:
Christoph Stein, Charité – Universitätsmedizin Berlin, GermanyVinod Tiwari, Indian Institute of Technology (BHU), India
Copyright © 2021 Liu, He, Mai, Yang, Fan and Huang. This is an open-access article distributed under the terms of the Creative Commons Attribution License (CC BY). The use, distribution or reproduction in other forums is permitted, provided the original author(s) and the copyright owner(s) are credited and that the original publication in this journal is cited, in accordance with accepted academic practice. No use, distribution or reproduction is permitted which does not comply with these terms.
*Correspondence: Fang Huang, aGZhbmdAbWFpbC5zeXN1LmVkdS5jbg==; Wenguo Fan, ZmFud2VuZ0BtYWlsLnN5c3UuZWR1LmNu