- 1Patton State Hospital, San Bernardino, CA, United States
- 2Department of Psychiatry, University of California, Riverside, Riverside, CA, United States
- 3Department of Anthropology, Stanford University, Stanford, CA, United States
- 4Department of Medicine, Shiraz University, Shiraz, Iran
- 5Instituto Nacional de Cardiologia Ignacio Chavez, Mexico City, Mexico
- 6Department of Medicine, The University of Texas Rio Grande Valley, Edinburg, TX, United States
Human immunodeficiency virus (HIV)-associated neurocognitive disorders (HAND) comprise a group of illnesses marked by memory and behavioral dysfunction that can occur in up to 50% of HIV patients despite adequate treatment with combination antiretroviral drugs. Iron dyshomeostasis exacerbates HIV-1 infection and plays a major role in Alzheimer’s disease pathogenesis. In addition, persons living with HIV demonstrate a high prevalence of neurodegenerative disorders, indicating that HAND provides a unique opportunity to study ferroptosis in these conditions. Both HIV and combination antiretroviral drugs increase the risk of ferroptosis by augmenting ferritin autophagy at the lysosomal level. As many viruses and their proteins exit host cells through lysosomal exocytosis, ferroptosis-driving molecules, iron, cathepsin B and calcium may be released from these organelles. Neurons and glial cells are highly susceptible to ferroptosis and neurodegeneration that engenders white and gray matter damage. Moreover, iron-activated microglia can engage in the aberrant elimination of viable neurons and synapses, further contributing to ferroptosis-induced neurodegeneration. In this mini review, we take a closer look at the role of iron in the pathogenesis of HAND and neurodegenerative disorders. In addition, we describe an epigenetic compensatory system, comprised of bromodomain-containing protein 4 (BRD4) and microRNA-29, that may counteract ferroptosis by activating cystine/glutamate antiporter, while lowering ferritin autophagy and iron regulatory protein-2. We also discuss potential interventions for lysosomal fitness, including ferroptosis blockers, lysosomal acidification, and cathepsin B inhibitors to achieve desirable therapeutic effects of ferroptosis-induced neurodegeneration.
HIGHLIGHTS
- HIV Patients With HAND Often Develop Early Neurodegeneration and Iron Dysmetyabolism, Likely Implicating Ferroptosis in this pathology.
- Many viruses, including HIV-1, exploit the host endosomal-lysosomal system to acquire iron and egress host cells.
- Both HIV-1 and cART can disrupt the lysosomes, promoting ferroptosis by releasing cathepsin B, iron, and Ca2+.
- Dysfunctional lysosomes impair both ferritinophagy and myelin synthesis, increasing the risk of neuronal and glial ferroptosis.
- An epigenetic BRD4/miR-29 system may oppose ferroptosis by boosting SLC7A11 and lowering ferritin autophagy.
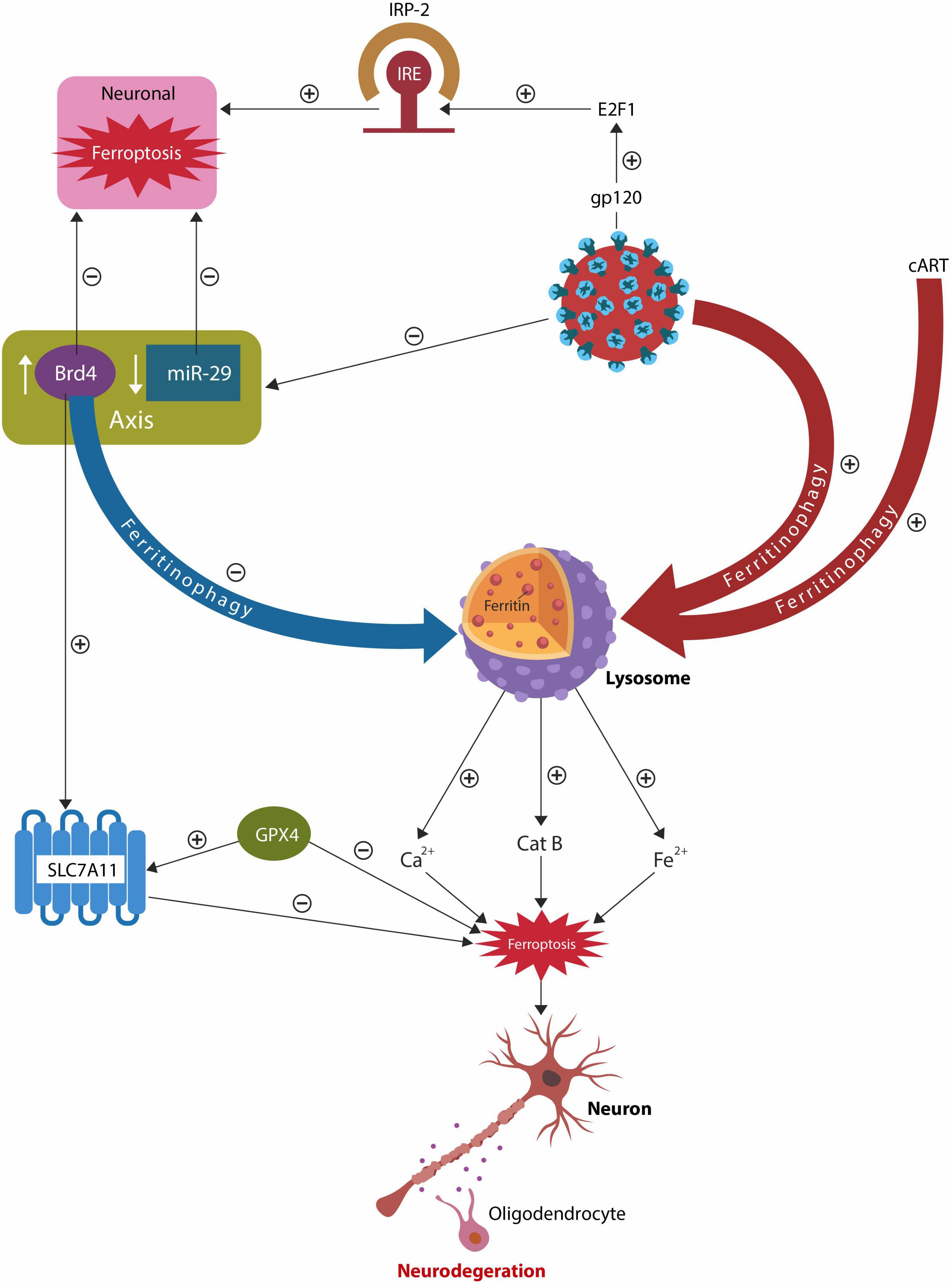
Graphical Abstract. Both HIV-1 and cART alter the lysosomes, increasing intracellular iron and the risk of ferroptosis. Dysfunctional lysosomes release the ferroptosis drivers iron, Ca2+ and cathepsin B (catB), promoting neuronal and oligodendrocyte loss, reflected in the white and gray matter pathology. The host responds to lysosomal damage by activating an epigenetic axis comprised of bromodomain 4 (BRD4) and microRNA-29 family (miR-29) that promptly suppresses lysosomal function, lowering ferritinophagy. As there is an inverse relationship between miR-29 and BRD4, HIV-1 inhibition of miR-29, upregulates BRD4, blocking ferritinophagy. The BRD4/miR-29 system also inhibits iron regulatory protein-2 (IRP-2) and augments cystine/glutamate antiporter xCT (SLC7A11), lowering the odds of ferroptosis.
Introduction
HIV-associated neurocognitive disorder (HAND), encountered in up to 50% of HIV patients, is characterized by cognitive deficits that may occur despite adequate treatment with combination antiretroviral therapy (cART) (Ru and Tang, 2017). Although the severity of HAND is lowered by cART, people living with HIV (PLWH), continue to display high rates of cognitive impairment and often develop Alzheimer’s disease (AD) earlier in life compared to the general population (Calcagno et al., 2021; Sharma, 2021). As high intracellular iron worsens HIV-1 prognosis and iron proteins are upregulated in HAND, ferroptosis-induced neurodegeneration (FIN) may contribute to this disorder (Patton et al., 2017; Milanini et al., 2019).
Viruses require iron for replication and often obtain this nutrient by targeting the iron-rich organelles, mitochondria, and lysosomes, disrupting their function, including ferritinophagy and myelination (Chang et al., 2015).
Ferroptosis is a programmed cell death triggered by iron-mediated lipid peroxidation in the absence of antioxidants glutathione (GSH) or glutathione peroxidase 4 (GPX4). Under normal circumstances, iron is stored in ferritin, a protein that undergoes lysosomal autophagy to release this biometal as needed. Dysfunctional ferritinophagy triggers toxic oxidative stress by upregulating intracellular iron, promoting pathology, including neurodegeneration. Aside from iron, ferroptosis can be triggered by low uptake of cysteine or glutamine via SLC7A11, an amino acid transporter specific for cysteine and glutamate, as well as the loss of GPX4 (Tang et al., 2021). Viral infections associated with increased iron absorption or upregulation of intracellular iron are likely to result in ferroptosis. Ferroptotic cell death is characterized by the release of damage-associated molecular patterns (DAMPs) that trigger immunogenicity and neuroinflammation, hallmarks of both HAND and AD (Smail and Brew, 2018; Sun et al., 2018). Lysosomes, the master regulators of iron metabolism, control ferroptosis via ferritin autophagy (ferritinophagy), a process characterized by iron release (Rizzollo et al., 2021). Many viruses hijack the endosomal-lysosomal system (ELS) to acquire iron, precipitating ferroptosis and FIN (Gao et al., 2017).
Recent studies have found that both cART and the HIV-1 antigen, trans-activator of transcription (Tat), alter the host ELS, upregulating ferritinophagy and iron release (Fredericksen et al., 2002; Hui et al., 2012; Tripathi et al., 2019; Cao et al., 2021) (Graphical Abstract). In addition, virus or cART-induced lysosomal dysfunction can activate microglial cells that often eliminate healthy neurons and synapses, further contributing to neurodegeneration (Tripathi et al., 2019; Kapralov et al., 2020; Cao et al., 2021; Miyanishi et al., 2021). Microglia are highly susceptible to ferroptosis and harbor latent HIV-1, therefore ferroptotic disintegration of these cells release DAMPs, triggering neuroinflammation (Lisi et al., 2016; Chivero et al., 2017; Shankaran et al., 2017; Wallet et al., 2019; Borrajo et al., 2021). Indeed, iron-activated microglia and macrophages, documented in HIV-1 infection, are believed to drive HAND pathology (Boelaert et al., 1996; Kenkhuis et al., 2021). In addition, microglia release cathepsin B (CatB), a protein associated with premature brain aging, neurotoxicity, and the accumulation of pathological, hyperphosphorylated Tau (pTau) (von Bernhardi et al., 2015; Nakanishi, 2020). Interestingly, as CatB possesses endopeptidase activity, the SARS-CoV-2 virus hijacks this protein to activate the S (spike) antigen, increasing infectivity (Schornberg et al., 2006; Mitrović et al., 2016; Huang et al., 2020; Padmanabhan et al., 2020). Moreover, individuals with HIV-1 infection on long-term cART present with higher brain deposition of pTau, linking this virus and its treatment to the risk of developing tauopathies (Anthony et al., 2006; Brown et al., 2014; Rao and Adlard, 2018; Mangan, 2021). These findings indicate that HIV-1 and CART induce lysosomal damage and predispose to FIN as elevated intracellular iron increases the odds of lipid peroxidation and ferroptotic cell death (Jiang et al., 2021).
Lysosomes are iron-rich subcellular organelles specialized in the degradation of proteins derived from autophagy, endocytosis, and phagocytosis (Platt et al., 2012; Figure 1). Aside from recycling endogenous biomolecule, autophagy also eliminates malignant and virus-infected cells, actively participating in host immunity (Choi et al., 2018). Many viruses, including SARS-CoV-2 and HIV Tat protein exploit the ELS to enter and exit host cells, disrupting this pathway and predisposing to FIN (Fan and He, 2016; Khan et al., 2020; Chen D. et al., 2021). Indeed, viruses that exploit the lysosome alter local pH and membrane permeability, facilitating the release of ferroptosis drivers iron, calcium (Ca2+) and CatB (Hui et al., 2012; Gorshkov et al., 2021; Nagakannan et al., 2021; Pedrera et al., 2021). For example, recent studies linked cytosolic Ca2+ upregulation to both ferroptosis and excitotoxicity, connecting the two metals to cell death (Gleitze et al., 2021; Pedrera et al., 2021). In addition, HIV has been reported to generate massive reactive oxygen and nitrogen species (RONS) associated with HAND, likely by aberrant microglial activation (Borrajo et al., 2021).
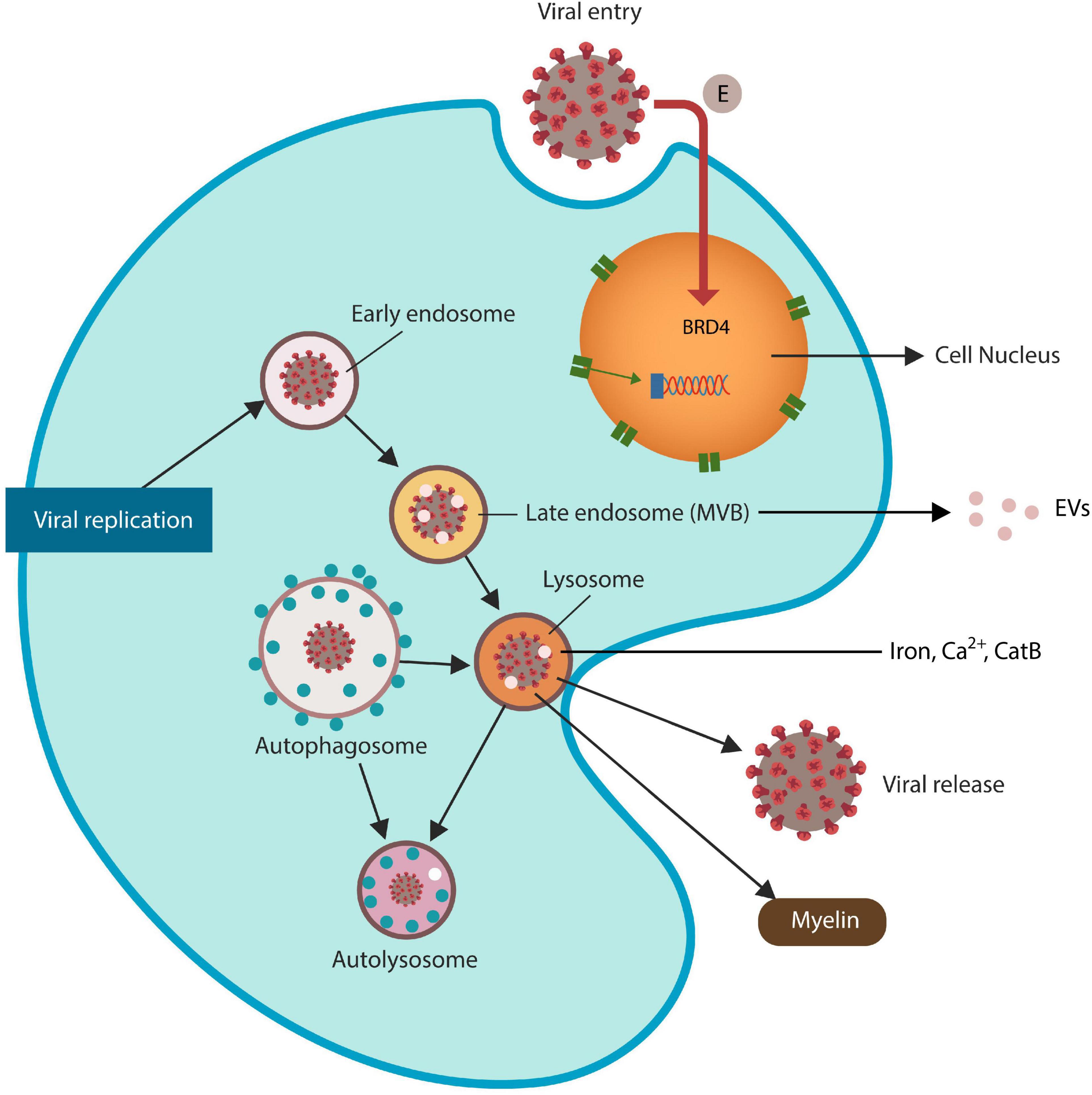
Figure 1. SARS-CoV-2 virus and HIV Tat antigen ingress host cells via ELS. The SARS-CoV-2 envelope (E) protein is a direct inhibitor of BRD4, increasing the risk of ferroptosis. Viruses that exploit ELS to egress host cells may disrupt lysosomal exocytosis of myelin and Tau protein (not shown). Late endosomes generate extracellular vesicles (EVs) that can spread viral proteins to the neighboring cells. Dysfunctional lysosomes may “leak” ferroptosis-driving molecules, including iron, Ca2+ and CatB, contributing to ferroptosis-induced neurodegeneration (FIN).
Recent studies have reported that lysosomal exocytosis is required for oligodendrocytes (OLGc) and Schwan cells myelination, suggesting that dysfunctional ELS could lead to white matter damage in HAND and AD (Shen et al., 2016). Others have connected dysfunctional ELS with the dissemination and seeding of pTau, further implicating this system in tauopathies (Tanaka et al., 2019; Jiang and Bhaskar, 2020; Polanco et al., 2021; Sebastián-Serrano et al., 2022).
In this mini review, we take a closer look at the virus induced ELS dysfunction in the pathogenesis of HAND and neurodegenerative disorders. In addition, we propose that FIN, triggered by HIV-1 infection and long-term cART use, may be counteracted by an epigenetic system comprised of bromodomain protein 4 (BRD4) and microRNA-29 (miR 29) that inhibits ferritinophagy by several mechanisms, including direct antiviral action, lysosomal suppression, SLC7A11 activation, and iron regulatory protein-2 (IRP-2) inhibition. We also discuss potential interventions for lysosomal fitness, including V-ATPase inhibitors, ferroptosis blockers, and CatB inhibitors.
Ferroptosis and the Endosomal-Lysosomal System
The ELS is comprised of intracellular vesicular compartments, including early endosomes, recycling endosomes, and late endosomes [also called multivesicular bodies (MVBs)] that merge with lysosomes. Autophagosomes also join the lysosomes to recycle their cargoes (Figure 1). Many viruses, including HIV-1 and SARS-CoV-2, acquire iron by usurping the lysosome, a process that upregulates ferritinophagy, iron release and the risk of FIN (Hui et al., 2012; Chauhan and Khandkar, 2015; Hu et al., 2015; Yambire et al., 2019; Blaess et al., 2020; Figure 1).
As autophagy controls viral infections by catabolizing infected cells along with the virus, many viruses have developed the ability to evade immunity by manipulating autophagy. For example, HIV-1 hijacks the ELS by Tat, Nef, and ENV antigens interaction with the autophagy proteins or mammalian target of rapamycin (mTor) components (Nardacci et al., 2017).
Lysosomal exocytosis, a mechanism of content secretion into the extracellular space, is mediated by lysosomal fusion with the cell plasma membrane that enables cargo release. HIV-1 and SARS-CoV-2 hijack the ELS to enter and egress host cells, disrupting vesicular homeostasis, including lysosomal exocytosis, and local pH (Hui et al., 2012; Nardacci et al., 2017; Blaess et al., 2020). Recent studies have reported that myelin biosynthesis requires adequate lysosomal exocytosis, suggesting that dysfunctional ELS may lead to both gray and white matter pathology (Chen D. et al., 2021; Kreher et al., 2021). Indeed, viruses that exit host cells through the lysosomes may disrupt myelination, promoting HAND and neurodegenerative disorders (Jensen et al., 2015; Buratta et al., 2020). Along these lines, novel neuroimaging studies have detected white matter changes in the prodromal phase of AD [prior to the development of pTau or beta-amyloid (Aβ)], indicating that myelin pathology is more common in his disorder than previously thought (Nasrabady et al., 2018; Pichet Binette et al., 2021). Moreover, a growing body of evidence has demonstrated myelin breakdown and dysfunctional OLGc progenitor cells (OPCs) in HAND and AD, linking both conditions to white matter pathology (Kimura-Kuroda et al., 1994; Bernardo et al., 1997; Lackner et al., 2010; Jensen et al., 2019). Furthermore, as OLGc are the predominant iron-containing cells in the brain and highly susceptible to ferroptosis, their demise may increase local iron, predisposing to FIN (Nobuta et al., 2019; Jhelum et al., 2020). Also, the ELS-released pTau, iron, Ca2+, CatB, and myelin maintain the neurodegenerative and HAND pathology (Joy et al., 2010; Chen et al., 2012; Xu Y. et al., 2021).
Recent studies found that elevated intracellular iron increases pTau and its aggregation, linking tauopathies to dysfunctional ELS (Brown et al., 2014; Rao and Adlard, 2018). In addition, iron dyshomeostasis and excessive pTau, documented in HAND, AD, and traumatic brain injury (TBI), implicate ELS in these pathologies (Canzoniero and Snider, 2005; Ravi et al., 2016; Cantres-Rosario et al., 2019; Hook et al., 2020; Nagakannan et al., 2021; Pedrera et al., 2021). Furthermore, as pTau acts as a cell-penetrating peptide, it may trigger cell-cell fusion and senescence, probably accounting for the accelerated aging and early development of AD in PLWH on long term cART (Ferrell and Giunta, 2014; Veloria et al., 2017; Osorio et al., 2022). Along these lines, HIV infected macrophages were shown to enter the brain and secrete neurotoxic CatB, triggering premature senescence, ferroptosis and FIN (Cantres-Rosario et al., 2019). Excessive CatB was also associated with cancer, connecting ELS dysfunction to tumorigenesis and metastases (Gondi and Rao, 2013; Ruan et al., 2015). On the other hand, CatB inhibitors may avert HAND, TBI, and cancer (Ha et al., 2012; Kos et al., 2014; Hook et al., 2015; Zenón-Meléndez et al., 2022). Cathepsin B was also associated with autoimmune disorders and osteoporosis, suggesting that a better understanding of this protein and its inhibitors could improve the treatment of several disorders that lack specific therapies (Toomey et al., 2014; Li et al., 2017; Ansari et al., 2022). In addition, several antipsychotic agents possess anticancer and antiviral properties, suggesting that these pathologies may intersect at the ELS level (Daniel et al., 2001; Kuzu et al., 2017; Girgis and Lieberman, 2021; Lu et al., 2021) (discussed in the section Psychotropic drugs). Other novel studies show that viral infections may precipitate ferroptosis by hijacking Ca2+ channels and pumps, suggesting a role for calcium channel blockers in the treatment of viral infections (Chen et al., 2019; Jayaseelan and Paramasivam, 2020; Straus et al., 2021). Indeed, as HIV Tat antigen upregulates pTau, it likely promotes FIN (Tripathi et al., 2016; Majerníková et al., 2021; Wang et al., 2022). Moreover, HIV infection was shown to increase apolipoprotein ε4 (ApoE4), an iron-upregulated biomolecule and AD risk factor, suggesting FIN involvement (Ayton et al., 2015; Diouf et al., 2019; Kagerer et al., 2020). Furthermore, as viruses upregulate both ApoE4 and iron, they may be capable of triggering FIN directly (Corder et al., 1998; Burt et al., 2008; Kuo et al., 2020; Gkouskou et al., 2021; Yim et al., 2022).
Taken together, ELS is situated at the crossroad of viral infections, cancer, and neuropsychiatric illness, probably explaining the beneficial effect of lysosomal therapeutics in these pathologies.
The BRD4/MiR-29 Compensatory System
Bromodomains are chromatin-associated molecules that interact with acetylated lysine residues on histone proteins, regulating numerous cellular processes, including replication, genome repair and the autophagic lysosomal function (Mujtaba et al., 2007; Fujisawa and Filippakopoulos, 2017; Sakamaki et al., 2017; Li et al., 2018). BRD4 is an epigenetic reader that regulates gene expression by forming a complex with the positive transcription elongation factor b (P-TEFb), promoting RNA polymerase II (Pol II), a mediator of DNA-dependent RNA synthesis (Jung et al., 2014). Novel studies have implicated BRD4 in various pathologies, ranging from inflammation, to cancer, CNS, and viral diseases (Zhu et al., 2012; Korb et al., 2015; Hajmirza et al., 2018). In preclinical studies, bromodomain and extra-terminal motif (BET) inhibitors (BETis) were shown to limit the progression of several cancers, rendering this protein a pharmacological target.
Recently, however, pan-BRD4 blockers, such as JQ1, were found detrimental as they trigger ferroptosis, damaging the genome, immunity and white matter (Chiu et al., 2017; Donati et al., 2018; Mita and Mita, 2020). On the other hand, domain-specific BETis appear to have fewer adverse effects and some have already been approved for clinical use (Pérez-Salvia and Esteller, 2017; Petretich et al., 2020). BRD4 negatively regulates ferroptosis by activating xCT, repairing the DNA, and inducing senescence-associated secretory phenotype (SASP), a ferroptosis resistant cellular program (Tasdemir et al., 2016; Sui et al., 2019; Lam et al., 2020; Tang et al., 2022). In addition, BRD4 promotes iron sequestration in ferritin to withhold it from pathogens, likely implicating this protein in nutritional immunity (Sui et al., 2019). Moreover, BRD4 displays direct antiviral properties, including inhibition of HIV Tat antigen, hence viruses must neutralize this protein to thrive (Wang et al., 2020; Alamer et al., 2021; Xu X. et al., 2021; Table 1). Indeed, several viruses, including HIV and SARS-CoV-2 have developed the ability to usurp BRD4, overcoming nutritional immunity (Chen I. P. et al., 2021). For example, the E (envelope) protein of SARS-CoV-2 virus inhibits BRD4, neutralizing the function of this epigenetic reader (Gordon et al., 2020). As BRD4 represses lysosomal autophagy, including ferritinophagy, viral hijacking of this protein may directly induce ferroptosis (Sakamaki et al., 2017). Moreover, BRD4 protects mitochondria by safeguarding the transcription of mitochondrial genes located in the nucleus (Kim et al., 2020). This is significant as earlier studies have implicated mitochondria and BRD4 in memory formation, suggesting a direct mechanism for virus-mediated neurodegeneration (Korb et al., 2015; Khacho et al., 2017; Zhang et al., 2022).
MicroRNAs (miRs) are non-coding ribonucleic acids (RNAs) that regulate gene expression by interacting with the 3′ untranslated region (3′ UTR) of target mRNAs (O’Brien et al., 2018). MiR-29 family, comprised of miR-29a, miR-29b, and miR-29c, displays antiviral properties, including inhibition of HIV-1 Nef protein (Ahluwalia et al., 2008; Adoro et al., 2015; Monteleone et al., 2015). In addition, IL-21/miR-29 axis was associated with HIV-1 latency, suggesting that enhancing this pathway may eradicate the virus from reservoirs (Frattari et al., 2017). Indeed, to counteract its antiviral action, HIV-1 has developed the ability to inhibit miR-29 via Tat antigen, promoting viral latency (Bennasser et al., 2005; Ruelas and Greene, 2013; Monteleone et al., 2015). As there is an inverse relationship between miR-29 and BRD4, HIV-1-mediated miR-29 downregulation lowers ferritinophagy by increasing BRD4 (Kohnken et al., 2018; Huang et al., 2019). Recently, miR-29 was found to lower intracellular iron by inhibiting iron regulatory protein 2 (IRP-2), lowering the risk of ferroptosis and FIN (Ripa et al., 2017) (Graphical Abstract). A recent preclinical study associated miR-29 family with gene expression in aging brain, showing that this miR lowers the expression of IRP-2 and the signaling with the iron responsive element (IRE), decreasing the intracellular iron load (Ripa et al., 2017). Opposing this mir-29 action, the HIV-1 glycoprotein 120 (gp 120) activates IRP-2 via E2F transcription factor 1 (E2F1), precipitating ferrocytosis (Shimizu et al., 2007). This is significant as several studies demonstrated low miR-29 expression in AD, linking this miR to the pathogenesis of neurodegenerative disorders and HAND (Lu et al., 2016; Müller et al., 2016; Pereira et al., 2016; Jahangard et al., 2020). Moreover, BRD4 has inhibitory effects on HIV-1 Tat protein, blocking viral latency and indicating that BRD4/miR-29 manipulation could eradicate latent HIV-1 (Zhu et al., 2012; Huang et al., 2017; Table 1).
Taken together, BRD4/miR-29 may comprise an epigenetic system that opposes FIN by several mechanisms, including direct antiviral action, iron sequestration in ferritin, IRP-2 downregulation, and suppression of lysosomal function, including ferritinophagy. As BRD4/miR-29 axis withholds iron from pathogens, we speculate that this system may drive nutritional immunity.
Potential Interventions
The role of ferroptosis in viral infections is closely connected to the concept of nutritional immunity, intracellular iron sequestration to withhold it from pathogens (Núñez et al., 2018). Although protective against infections, iron sequestration may escalate the risk of ferroptosis as it places this biometal in the proximity of lipids, increasing the risk of peroxidation (Chao et al., 2020; Chen et al., 2020). For this reason, lowering neuronal ferroptosis by upregulating BRD4 may decrease ferritinophagy and neuronal ferroptosis.
In this section, we focus on pharmacological agents that may lower the FIN risk at the ELS level.
V-Atpase Inhibitors
Under normal circumstances, lysosomal pH must be highly acidic (4.5–5.5) for protein degradation to occur. This is accomplished via vacuolar (H+) ATPase (or V-ATPase) that pumps protons into the organelle to lower its pH (Song et al., 2020). Several viruses and their antigens, including HIV-1 Nef protein, usurp V-ATPase, increasing ferritin autophagy and intracellular iron, as well as the risk of ferroptosis and FIN (Lu et al., 1998; Castro-Gonzalez et al., 2021).
Over the past two decades, several V-ATPase inhibitors have been developed, including concanamycin A, bafilomycin A1, saliphenylhalamide, and quinazolines (Garcia-Rodriguez et al., 2015). Quinazolines were the newest addition to the armamentarium of V-ATPase inhibitors. They are small electrophilic molecules that comprise the common denominator of over 150 naturally occurring alkaloids with numerous biological properties (Chen et al., 2017). These agents possess anti-HIV, anticancer and anti-neurodegenerative properties, implicating ELS dysfunction in these pathologies (Colacurcio and Nixon, 2016; Hu et al., 2018; Whitton et al., 2018; Le-Nhat-Thuy et al., 2020). Indeed, the quinazolinone compound, PBT434 possess iron chelating properties suggesting that lowering this biometal may benefit the patients with these conditions (Bailey et al., 2021).
N-Acetylcysteine
Recent studies have shown that N-acetylcysteine (NAC) can reverse cART-induced microglial activation and inhibit ferritinophagy (Tripathi et al., 2020). NAC is a widely used drug, primarily as an antidote for acetaminophen overdose, but possesses many other beneficial effects, including correcting the oxidative stress-mediated ELS dysfunction, suggesting that it may reverse some neurological complications of HAND (Tripathi et al., 2020). Interestingly, NAC has been evaluated for its senolytic properties against brain aging, indicating potential efficacy against HAND and AD (Tardiolo et al., 2018). In addition, NAC possesses anticancer and anti-HIV, properties, further linking ELS to these pathologies (Roederer et al., 1992; Deng et al., 2019). Moreover, as NAC supplementation increases GSH and GPX, it may also suppress ferroptosis and FIN (Karuppagounder et al., 2018; Hu et al., 2021).
Aryl-Thiazole Compounds
Aryl-thiazole compounds are novel agents that include N2-[2-chloro-4(3,4,5-trimethoxy phenyl azetidin-1-yl]-N4-(substituted aryl)-1,3-thiazole-2,4-diamine (4a–g), a lipid peroxidation blocker, indicating potential beneficial effect against FIN (Djukic et al., 2018). Aryl-thiazole compounds possess antiviral, anticancer and anti-neurodegenerative properties by modulating γ-secretase (Lu et al., 2009; Dawood et al., 2015; Bhattarai et al., 2021). In addition, (3Z)-3-(2-[4-(aryl)-1,3-thiazol-2-yl]hydrazin-1-ylidene)-2,3-dihydro-1H-indol-2-one presents with reverse transcriptase inhibiting properties, potentially lowering HIV-1 replication (Meleddu et al., 2015).
Pyridine, Acetamide, and Benzohydrazine
Pyridine, acetamide, and benzohydrazine are CatB inhibitors with antiviral, anti-neurodegenerative and anticancer properties (Prachayasittikul et al., 2017; Alizadeh and Ebrahimzadeh, 2021; Chitranshi et al., 2021). As CatB is a ferroptosis driver, the inhibitors of this cysteine protease may benefit the patients with AD and HAND. In addition, these molecules were shown to modulate the HIV-1 gene expression and inhibit Tat protein, suggesting antiviral properties (Balachandran et al., 2017). Other recently developed CatB inhibitors are nitroxoline (8-hydroxy 5-nitroquinoline) derivatives, inhibitors of endopeptidase and exopeptidase activities of this enzyme. These drugs have established antibiotic, anticancer and anti-neurodegenerative properties (Knez et al., 2015).
Ferrostatin-1 and Liproxstatin-1
Ferrostatin-1 (Fer-1) and liproxstatin-1 (Lip-1) comprise a new generation of ferroptosis inhibitors that function as radical traps and scavengers of lipid hydroperoxyl groups (Miotto et al., 2020). These agents are potential COVID-19 treatments, while their inhibitors have been studied as ferroptosis inducers in cancer cells (Subburayan et al., 2020; Yang and Lai, 2020). Moreover, Fer-1 and Lip-1 have been evaluated for efficacy against viral infections and neurodegeneration (Chen K. et al., 2021; Wang et al., 2021).
Iron Chelators
Iron chelation was demonstrated to protect lysosomal membranes against peroxidative injury, lowering the risk of ferroptosis (Kurz et al., 2006). Indeed, iron chelators are currently being assessed for efficacy against neurodegenerative disorders, including AD (Dusek et al., 2016) (NCT03234686). These agents have shown antiviral effects, especially against HIV-1 and SARS-CoV-2, suggesting that withholding iron from pathogens can be an efficient anti-infectious strategy (van Asbeck et al., 2001; Chhabra et al., 2020). By protecting lysosomal membranes, chelation therapy lowers CatB cytosolic “escape” and subsequent neurotoxicity (Lin et al., 2010). In addition, iron chelators have shown beneficial effects in cancer, probably by decreasing mitochondrial energy production and starving the tumors (Fryknäs et al., 2016).
Chloroquine and Hydroxychloroquine
The anti-malaria drugs, chloroquine (CQ) and hydroxychloroquine (HCQ) are autophagy blockers that accumulate in the ELS, neutralizing luminal pH. These compounds possess antiviral and anticancer properties, probably by blocking lysosomal exocytosis (Neely et al., 2003; Halcrow et al., 2019). Interestingly, recent studies in patients with rheumatoid arthritis (RA) showed that prolonged treatment with CQ and HCQ decreased the risk of Parkinson’s disease (PD), indicating anti-neurodegenerative properties (Paakinaho et al., 2022). Indeed, as CQ and HCQ inhibit CatB, a protein implicated in the pathogenesis of both RA and PD, it is likely that these drugs inhibit CatB (Hashimoto et al., 2001; Tsujimura et al., 2015; Mahoney-Sánchez et al., 2021; Zhao et al., 2022).
Psychotropic Drugs
Second generation antipsychotic drugs, and several antidepressants were shown to possess antioxidant properties by upregulating GSH, lowering the risk of ferroptosis (Quincozes-Santos et al., 2010; Caruso et al., 2020). In addition, as GSH possesses antiviral properties, psychotropic drugs may enhance autophagy, facilitating the clearance of cancerous and pathogen-infected cells (Fraternale et al., 2006). Indeed, as some psychotropics enter cells via ELS, they may eliminate the viruses they encounter throughout this pathway (Benton et al., 2010; Canfrán-Duque et al., 2016; Schneider et al., 2016; Vela, 2020; Fred et al., 2022). Moreover, as several psychotropic drugs display anticancer properties, they likely inhibit CatB, lowering the cytotoxicity of this protein (Varalda et al., 2020). Furthermore, newly synthesized ferroptosis inhibitors, the phenothiazine analogs, alter autophagy, probably explaining their efficacy against neurodegenerative disorders (Liu et al., 2020; Posso et al., 2022). Interestingly, the fast-acting antidepressant drug, ketamine, was found to upregulate miR-29, linking depression to ferroptosis, suggesting that the BRD4/miR-29 system may have antidepressant effects (Stolke et al., 1980; Paul et al., 2014; Wan et al., 2018).
Cathepsin B Inhibitors
CatB dysfunction was associated with several pathologies, including autoimmune disorders, cancer, drug addiction, neurodegeneration, and viral infections (Toomey et al., 2014; Li et al., 2017; Ansari et al., 2022). For example, the anthelminthic drug, niclosamide, demonstrates anticancer, antiviral, and neuroprotective properties, likely by inhibiting CatB and restoring ELS homeostasis (Circu et al., 2016; Goulding et al., 2021; Weiss et al., 2021). Indeed, niclosamide was found to inhibit HIV-1 proliferation and activate PTEN-induced kinase 1 (PINK1), indicating potential benefit in neurodegenerative disorders and likely HAND (Niyomdecha et al., 2020; Jang et al., 2022). Interestingly, sigma-1 (Sig1R) agonists, such as BD1047, were shown to downregulate CatB, ameliorating HAND, especially in cocaine users (López et al., 2019). Along this line, fluvoxamine, a potent Sig1R agonist, with antiviral properties may be a yet unknown CatB inhibitor.
Conclusion
HIV-1 infection and long-term cART, enhance lysosomal ferritinophagy, releasing excessive amounts of iron, Ca2+ and CatB that may trigger FIN. The host responds to this insult by activating an epigenetic system comprised of BRD4/miR-29 that blocks dysfunctional lysosomes and iron release via IRP-2 and SLC7A11. As BRD4 is upregulated by low miR-29 and HIV-1 inhibits miR-29, ferritinophagy is downregulated, lowering FIN. In addition, as BRD4 inhibits HIV-1 Tat protein, modulation of BRD4/miR-29 system may eradicate latent HIV-1 from reservoirs, such as microglia and macrophages.
Author Contributions
All authors listed have made a substantial, direct, and intellectual contribution to the work, and approved it for publication.
Conflict of Interest
The authors declare that the research was conducted in the absence of any commercial or financial relationships that could be construed as a potential conflict of interest.
Publisher’s Note
All claims expressed in this article are solely those of the authors and do not necessarily represent those of their affiliated organizations, or those of the publisher, the editors and the reviewers. Any product that may be evaluated in this article, or claim that may be made by its manufacturer, is not guaranteed or endorsed by the publisher.
References
Adoro, S., Cubillos-Ruiz, J., Chen, X., Deruaz, M., Vrbanac, V. D., Song, M., et al. (2015). IL-21 induces antiviral microRNA-29 in CD4 T cells to limit HIV-1 infection. Nat. Commun. 6:7562. doi: 10.1038/ncomms8562
Ahluwalia, J. K., Khan, S. Z., Soni, K., Rawat, P., Gupta, A., Hariharan, M., et al. (2008). Human cellular microRNA hsa-miR-29a interferes with viral nef protein expression and HIV-1 replication. Retrovirology 5:117. doi: 10.1186/1742-4690-5-117
Alamer, E., Zhong, C., and Hajnik, R. (2021). Modulation of BRD4 in HIV epigenetic regulation: implications for finding an HIV cure. Retrovirology 18:3. doi: 10.1186/s12977-020-00547-9
Alizadeh, S. R., and Ebrahimzadeh, M. A. (2021). Antiviral activities of pyridine fused and pyridine containing heterocycles, a review (from 2000 to 2020). Mini Rev. Med. Chem. 21, 2584–2611. doi: 10.2174/1389557521666210126143558
Ansari, M. A., Nadeem, A., Alshammari, M. A., Attia, S. M., Bakheet, S. A., Khan, M. R., et al. (2022). Cathepsin B inhibitor alleviates Th1, Th17, and Th22 transcription factor signaling dysregulation in experimental autoimmune encephalomyelitis. Exp. Neurol. 351:113997. doi: 10.1016/j.expneurol.2022.113997
Anthony, I. C., Ramage, S. N., Carnie, F. W., Simmonds, P., and Bell, J. E. (2006). Accelerated Tau deposition in the brains of individuals infected with human immunodeficiency virus-1 before and after the advent of highly active anti-retroviral therapy. Acta Neuropathol. 111, 529–538. doi: 10.1007/s00401-006-0037-0
Ayton, S., Faux, N. G., and Bush, A. I. Alzheimer’s Disease Neuroimaging Initiative (2015). Ferritin levels in the cerebrospinal fluid predict Alzheimer’s disease outcomes and are regulated by APOE. Nat. Commun. 6:6760. doi: 10.1038/ncomms7760
Bailey, D. K., Clark, W., and Kosman, D. J. (2021). The iron chelator, PBT434, modulates transcellular iron trafficking in brain microvascular endothelial cells. PLoS One 16:e0254794. doi: 10.1371/journal.pone.0254794
Balachandran, A., Wong, R., Stoilov, P., Pan, S., Blencowe, B., Cheung, P., et al. (2017). Identification of small molecule modulators of HIV-1 Tat and Rev protein accumulation. Retrovirology 14:7. doi: 10.1186/s12977-017-0330-0
Bennasser, Y., Le, S. Y., Benkirane, M., and Jeang, K. T. (2005). Evidence that HIV-1 encodes an siRNA and a suppressor of RNA silencing. Immunity 22, 607–619. Erratum in: Immunity. 2005;22(6):773 doi: 10.1016/j.immuni.2005.03.010
Benton, T., Lynch, K., Dubé, B., Gettes, D. R., Tustin, N. B., Lai, J. P., et al. (2010). Selective serotonin reuptake inhibitor suppression of HIV infectivity and replication. Psychosom. Med. 72, 925–932. doi: 10.1097/PSY.0b013e3181f883ce
Bernardo, A., Agresti, C., and Levi, G. (1997). HIV-gp120 affects the functional activity of oligodendrocytes and their susceptibility to complement. J. Neurosci. Res. 50, 946–957. doi: 10.1002/(SICI)1097-4547(19971215)50:6<946::AID-JNR5<3.0.CO;2-D
Bhattarai, S., Liu, L., and Wolfe, M. S. (2021). Discovery of aryl aminothiazole γ-secretase modulators with novel effects on amyloid β-peptide production. Bioorg. Med. Chem. Lett. 54:128446. doi: 10.1016/j.bmcl.2021.128446
Blaess, M., Kaiser, L., Sauer, M., Csuk, R., and Deigner, H. P. (2020). COVID-19/SARS-CoV-2 infection: lysosomes and lysosomotropism implicate new treatment strategies and personal risks. Int. J. Mol. Sci. 21:4953. doi: 10.3390/ijms21144953
Boelaert, J. R., Weinberg, G. A., and Weinberg, E. D. (1996). Altered iron metabolism in HIV infection: mechanisms, possible consequences, and proposals for management. Infect. Agents Dis. 5, 36–46.
Borrajo, A., Spuch, C., Penedo, M. A., Olivares, J. M., and Agís-Balboa, R. C. (2021). Important role of microglia in HIV-1 associated neurocognitive disorders and the molecular pathways implicated in its pathogenesis. Ann. Med. 53, 43–69. doi: 10.1080/07853890.2020.1814962
Brown, L. A., Scarola, J., Smith, A. J., Sanberg, P. R., Tan, J., Giunta, B., et al. (2014). The role of tau protein in HIV-associated neurocognitive disorders. Mol. Neurodegen. 9:40. doi: 10.1186/1750-1326-9-40
Buratta, S., Tancini, B., Sagini, K., Delo, F., Chiaradia, E., Urbanelli, L., et al. (2020). Lysosomal exocytosis, exosome release and secretory autophagy: the autophagic- and endo-lysosomal systems go extracellular. Int. J. Mol. Sci. 21:2576. doi: 10.3390/ijms21072576
Burt, T. D., Agan, B. K., Marconi, V. C., He, W., Kulkarni, H., Mold, J. E., et al. (2008). Apolipoprotein (apo) E4 enhances HIV-1 cell entry in vitro, and the APOE epsilon4/epsilon4 genotype accelerates HIV disease progression. Proc. Natl. Acad. Sci. U.S.A. 105, 8718–8723. doi: 10.1073/pnas.0803526105
Calcagno, A., Celani, L., Trunfio, M., Orofino, G., Imperiale, D., Atzori, C., et al. (2021). Alzheimer dementia in people living with HIV. Neurol. Clin. Pract. 11, e627–e633. doi: 10.1212/CPJ.0000000000001060
Canfrán-Duque, A., Barrio, L. C., Lerma, M., de la Peña, G., Serna, J., Pastor, O., et al. (2016). First-generation antipsychotic haloperidol alters the functionality of the late Endosomal/Lysosomal compartment in vitro. Int. J. Mol. Sci. 17:404. doi: 10.3390/ijms17030404
Cantres-Rosario, Y. M., Ortiz-Rodríguez, S. C., Santos-Figueroa, A. G., Plaud, M., Negron, K., Cotto, B., et al. (2019). HIV infection induces extracellular Cathepsin B uptake and damage to neurons. Sci. Rep. 9:8006. doi: 10.1038/s41598-019-44463-1
Canzoniero, L. M., and Snider, B. J. (2005). Calcium in Alzheimer’s disease pathogenesis: too much, too little or in the wrong place? J. Alzheimers Dis. 8, 147–154; discussion 209–215. doi: 10.3233/jad-2005-8207
Cao, D., Khanal, S., Wang, L., Li, Z., Zhao, J., Nguyen, L. N., et al. (2021). A matter of life or death: productively infected and Bystander CD4 T cells in early HIV infection. Front. Immunol. 11:626431. doi: 10.3389/fimmu.2020.626431
Caruso, G., Grasso, M., Fidilio, A., Tascedda, F., Drago, F., and Caraci, F. (2020). Antioxidant properties of second-generation antipsychotics: focus on microglia. Pharmaceuticals (Basel) 13:457. doi: 10.3390/ph13120457
Castro-Gonzalez, S., Shi, Y., Colomer-Lluch, M., Song, Y., Mowery, K., Almodovar, S., et al. (2021). HIV-1 Nef counteracts autophagy restriction by enhancing the association between BECN1 and its inhibitor BCL2 in a PRKN-dependent manner. Autophagy 17, 553–577. doi: 10.1080/15548627.2020.1725401
Chang, H. C., Bayeva, M., Taiwo, B., Palella, F. J. Jr., Hope, T. J., and Ardehali, H. (2015). Short communication: high cellular iron levels are associated with increased HIV infection and replication. AIDS Res. Hum. Retroviruses 31, 305–312. doi: 10.1089/aid.2014.0169
Chao, Y. K., Chang, S. Y., and Grimm, C. (2020). Endo-Lysosomal cation channels and infectious diseases. Rev. Physiol. Biochem. Pharmacol. [Online ahead of print], doi: 10.1007/112_2020_31
Chauhan, A., and Khandkar, M. (2015). Endocytosis of human immunodeficiency virus 1 (HIV-1) in astrocytes: a fiery path to its destination. Microb. Pathog. 78, 1–6. doi: 10.1016/j.micpath.2014.11.003
Chen, D., Zheng, Q., Sun, L., Ji, M., Li, Y., Deng, H., et al. (2021). ORF3a of SARS-CoV-2 promotes lysosomal exocytosis-mediated viral egress. Dev. Cell. 56, 3250.e5–3263.e5. doi: 10.1016/j.devcel.2021.10.006
Chen, G., Zhang, Z., Wei, Z., Cheng, Q., Li, X., Li, W., et al. (2012). Lysosomal exocytosis in Schwann cells contributes to axon remyelination. Glia 60, 295–305. doi: 10.1002/glia.21263
Chen, I.P., Longbotham, J. E., McMahon, S., Suryawanshi, R. K., Carlson-Stevermer, J., Gupta, M., et al. (2021). Viral E protein neutralizes BET protein-mediated post-entry antagonism of SARS-CoV-2. bioRxiv [preprint] 2021.11.14.468537. doi: 10.1101/2021.11.14.468537
Chen, K., Jiang, X., Wu, M., Cao, X., Bao, W., and Zhu, L. Q. (2021). Ferroptosis, a potential therapeutic target in Alzheimer’s disease. Front. Cell Dev. Biol. 9:704298. doi: 10.3389/fcell.2021.704298
Chen, X., Cao, R., and Zhong, W. (2019). Host calcium channels and pumps in viral infections. Cells 9:94. doi: 10.3390/cells9010094
Chen, X., Yu, C., Kang, R., and Tang, D. (2020). Iron metabolism in ferroptosis. Front. Cell Dev. Biol. 8:590226. doi: 10.3389/fcell.2020.590226
Chen, Y. C., Backus, K. M., Merkulova, M., Yang, C., Brown, D., Cravatt, B. F., et al. (2017). Covalent modulators of the vacuolar ATPase. J. Am. Chem. Soc. 139, 639–642. doi: 10.1021/jacs.6b12511
Chhabra, R., Saha, A., Chamani, A., Schneider, N., Shah, R., and Nanjundan, M. (2020). Iron pathways and iron chelation approaches in viral, microbial, and fungal infections. Pharmaceuticals (Basel) 13:275. doi: 10.3390/ph13100275
Chitranshi, N., Kumar, A., Sheriff, S., Gupta, V., Godinez, A., Saks, D., et al. (2021). Identification of Novel Cathepsin B inhibitors with implications in Alzheimer’s disease: computational refining and biochemical evaluation. Cells 10:1946. doi: 10.3390/cells10081946
Chiu, L. Y., Gong, F., and Miller, K. M. (2017). Bromodomain proteins: repairing DNA damage within chromatin. Philos. Trans. R. Soc. Lond. B Biol. Sci. 372:20160286. doi: 10.1098/rstb.2016.0286
Chivero, E. T., Guo, M. L., Periyasamy, P., Liao, K., Callen, S. E., and Buch, S. (2017). HIV-1 Tat primes and activates microglial NLRP3 inflammasome-mediated neuroinflammation. J. Neurosci. 37, 3599–3609. doi: 10.1523/JNEUROSCI.3045-16.2017
Choi, Y., Bowman, J. W., and Jung, J. U. (2018). Autophagy during viral infection — a double-edged sword. Nat. Rev. Microbiol. 16, 341–354. doi: 10.1038/s41579-018-0003-6
Circu, M. L., Dykes, S. S., Carroll, J., Kelly, K., Galiano, F., Greer, A., et al. (2016). A novel high content imaging-based screen identifies the Anti-helminthic niclosamide as an inhibitor of lysosome anterograde trafficking and prostate cancer cell invasion. PLoS One 11:e0146931. Erratum in: PLoS One. 2016;11(3):e0151718 doi: 10.1371/journal.pone.0146931
Colacurcio, D. J., and Nixon, R. A. (2016). Disorders of lysosomal acidification-The emerging role of v-ATPase in aging and neurodegenerative disease. Ageing Res. Rev. 32, 75–88. doi: 10.1016/j.arr.2016.05.004
Corder, E., Robertson, K., and Lannfelt, L. (1998). HIV-infected subjects with the E4 allele for APOE have excess dementia and peripheral neuropathy. Nat. Med. 4, 1182–1184. doi: 10.1038/2677
Daniel, W. A., Wójcikowski, J., and Pałucha, A. (2001). Intracellular distribution of psychotropic drugs in the grey and white matter of the brain: the role of lysosomal trapping. Br. J. Pharmacol. 134, 807–814. doi: 10.1038/sj.bjp.0704319
Dawood, K. M., Eldebss, T. M., El-Zahabi, H. S., and Yousef, M. H. (2015). Synthesis and antiviral activity of some new bis-1,3-thiazole derivatives. Eur. J. Med. Chem. 102, 266–276. doi: 10.1016/j.ejmech.2015.08.005
Deng, J., Liu, A. D., Hou, G. Q., Zhang, X., Ren, K., Chen, X. Z., et al. (2019). N-acetylcysteine decreases malignant characteristics of glioblastoma cells by inhibiting Notch2 signaling. J. Exp. Clin. Cancer Res. 38:2. doi: 10.1186/s13046-018-1016-8
Diouf, I., Fazlollahi, A., Bush, A. I., and Ayton, S. Alzheimer’s Disease Neuroimaging Initiative (2019). Cerebrospinal fluid ferritin levels predict brain hypometabolism in people with underlying β-amyloid pathology. Neurobiol. Dis. 124, 335–339. doi: 10.1016/j.nbd.2018.12.010
Djukic, M., Fesatidou, M., Xenikakis, I., Geronikaki, A., Angelova, V. T., Savic, V., et al. (2018). In vitro antioxidant activity of thiazolidinone derivatives of 1,3-thiazole and 1,3,4-thiadiazole. Chem. Biol. Interact. 286, 119–131. doi: 10.1016/j.cbi.2018.03.013
Donati, B., Lorenzini, E., and Ciarrocchi, A. (2018). BRD4 and Cancer: going beyond transcriptional regulation. Mol. Cancer 17:164. doi: 10.1186/s12943-018-0915-9
Dusek, P., Schneider, S. A., and Aaseth, J. (2016). Iron chelation in the treatment of neurodegenerative diseases. J. Trace Elem. Med. Biol. 38, 81–92. doi: 10.1016/j.jtemb.2016.03.010
Fan, Y., and He, J. J. (2016). HIV-1 Tat promotes lysosomal exocytosis in astrocytes and contributes to astrocyte-mediated tat neurotoxicity. J. Biol. Chem. 291, 22830–22840. doi: 10.1074/jbc.M116.731836
Ferrell, D., and Giunta, B. (2014). The impact of HIV-1 on neurogenesis: implications for HAND. Cell Mol. Life Sci. 71, 4387–4392. doi: 10.1007/s00018-014-1702-4
Fraternale, A., Paoletti, M. F., Casabianca, A., Oiry, J., Clayette, P., Vogel, J. U., et al. (2006). Antiviral and immunomodulatory properties of new pro-glutathione (GSH) molecules. Curr. Med. Chem. 13, 1749–1755. doi: 10.2174/092986706777452542
Frattari, G., Aagaard, L., and Denton, P. W. (2017). The role of miR-29a in HIV-1 replication and latency. J. Virus Erad. 3, 185–191. doi: 10.1016/s2055-6640(20)30322-8
Fred, S. M., Kuivanen, S., and Ugurlu, H. (2022). Antidepressant and antipsychotic drugs reduce viral infection by SARS-CoV-2 and fluoxetine shows antiviral activity against the novel variants in vitro. Front. Pharmacol. 12:755600. doi: 10.3389/fphar.2021.755600
Fredericksen, B. L., Wei, B. L., Yao, J., Luo, T., and Garcia, J. V. (2002). Inhibition of endosomal/lysosomal degradation increases the infectivity of human immunodeficiency virus. J. Virol. 76, 11440–11446. doi: 10.1128/jvi.76.22.11440-11446.2002
Fryknäs, M., Zhang, X., Bremberg, U., Senkowski, W., Olofsson, M. H., Brandt, P., et al. (2016). Iron chelators target both proliferating and quiescent cancer cells. Sci. Rep. 6:38343. doi: 10.1038/srep38343
Fujisawa, T., and Filippakopoulos, P. (2017). Functions of bromodomain-containing proteins and their roles in homeostasis and cancer. Nat. Rev. Mol. Cell Biol. 18, 246–262. doi: 10.1038/nrm.2016.143
Gao, Y., Chen, Y., Zhan, S., Zhang, W., Xiong, F., and Ge, W. (2017). Comprehensive proteome analysis of lysosomes reveals the diverse function of macrophages in immune responses. Oncotarget 8, 7420–7440. doi: 10.18632/oncotarget.14558
Garcia-Rodriguez, J., Mendiratta, S., White, M. A., Xie, X. S., and De Brabander, J. K. (2015). Synthesis and structure-activity studies of the V-ATPase inhibitor saliphenylhalamide (SaliPhe) and simplified analogs. Bioorg. Med. Chem. Lett. 25, 4393–4398. doi: 10.1016/j.bmcl.2015.09.021
Girgis, R. R., and Lieberman, J. A. (2021). Anti-viral properties of antipsychotic medications in the time of COVID-19. Psychiatry Res. 295:113626. doi: 10.1016/j.psychres.2020.113626
Gkouskou, K., Vasilogiannakopoulou, T., Andreakos, E., Davanos, N., Gazouli, M., Sanoudou, D., et al. (2021). COVID-19 enters the expanding network of apolipoprotein E4-related pathologies. Redox Biol. 41:101938. doi: 10.1016/j.redox.2021.101938
Gleitze, S., Paula-Lima, A., Núñez, M. T., and Hidalgo, C. (2021). The calcium-iron connection in ferroptosis-mediated neuronal death. Free Radic Biol. Med. 175, 28–41. doi: 10.1016/j.freeradbiomed.2021.08.231
Gondi, C. S., and Rao, J. S. (2013). Cathepsin B as a cancer target. Expert Opin. Ther. Targets 17, 281–291. doi: 10.1517/14728222.2013.740461
Gordon, D. E., Jang, G. M., and Bouhaddou, M. (2020). A SARS-CoV-2 protein interaction map reveals targets for drug repurposing. Nature 583, 459–468. doi: 10.1038/s41586-020-2286-9
Gorshkov, K., Chen, C. Z., Bostwick, R., Rasmussen, L., Tran, B. N., Cheng, Y. S., et al. (2021). The SARS-CoV-2 cytopathic effect is blocked by lysosome alkalizing small molecules. ACS Infect. Dis. 7, 1389–1408. doi: 10.1021/acsinfecdis.0c00349
Goulding, S. R., Lévesque, M., Sullivan, A. M., Collins, L. M., and O’Keeffe, G. W. (2021). Quinacrine and niclosamide promote neurite growth in midbrain dopaminergic neurons through the canonical BMP-smad pathway and protect against neurotoxin and α-Synuclein-Induced neurodegeneration. Mol. Neurobiol. 58, 3405–3416. doi: 10.1007/s12035-021-02351-8
Ha, S. D., Park, S., Hattlmann, C. J., Barr, S. D., and Kim, S. O. (2012). Inhibition or deficiency of cathepsin B leads defects in HIV-1 Gag pseudoparticle release in macrophages and HEK293T cells. Antiviral Res. 93, 175–184. doi: 10.1016/j.antiviral.2011.11.009
Hajmirza, A., Emadali, A., Gauthier, A., Casasnovas, O., Gressin, R., and Callanan, M. B. (2018). BET family protein BRD4: an emerging actor in NFκB signaling in inflammation and cancer. Biomedicines 6:16. doi: 10.3390/biomedicines6010016
Halcrow, P., Datta, G., Ohm, J. E., Soliman, M. L., Chen, X., and Geiger, J. D. (2019). Role of endolysosomes and pH in the pathogenesis and treatment of glioblastoma. Cancer Rep. 2:e1177. doi: 10.1002/cnr2.1177
Hashimoto, Y., Kakegawa, H., Narita, Y., Hachiya, Y., Hayakawa, T., Kos, J., et al. (2001). Significance of cathepsin B accumulation in synovial fluid of rheumatoid arthritis. Biochem. Biophys. Res. Commun. 283, 334–339. doi: 10.1006/bbrc.2001.4787
Hook, G., Jacobsen, J. S., Grabstein, K., Kindy, M., and Hook, V. (2015). Cathepsin B is a new drug target for traumatic brain injury therapeutics: evidence for E64d as a promising lead drug candidate. Front. Neurol. 6:178. doi: 10.3389/fneur.2015.00178
Hook, V., Yoon, M., Mosier, C., Ito, G., Podvin, S., Head, B. P., et al. (2020). Cathepsin B in neurodegeneration of Alzheimer’s disease, traumatic brain injury, and related brain disorders. Biochim. Biophys. Acta Proteins Proteom. 1868:140428. doi: 10.1016/j.bbapap.2020.140428
Hu, C. J., Chen, Y. T., Fang, Z. S., Chang, W. S., and Chen, H. W. (2018). Antiviral efficacy of nanoparticulate vacuolar ATPase inhibitors against influenza virus infection. Int. J. Nanomed. 13, 8579–8593. doi: 10.2147/IJN.S185806
Hu, M., Zhang, Y., Ma, S., Li, J., Wang, X., Liang, M., et al. (2021). Suppression of uterine and placental ferroptosis by N-acetylcysteine in a rat model of polycystic ovary syndrome. Mol. Hum. Reprod. 27:gaab067. doi: 10.1093/molehr/gaab067
Hu, Y. B., Dammer, E. B., Ren, R. J., and Wang, G. (2015). The endosomal-lysosomal system: from acidification and cargo sorting to neurodegeneration. Transl. Neurodegener. 4:18. doi: 10.1186/s40035-015-0041-1
Huang, H., Liu, S., Jean, M., Simpson, S., Huang, H., Merkley, M., et al. (2017). A novel bromodomain inhibitor reverses HIV-1 latency through specific binding with BRD4 to promote Tat and P-TEFb association. Front. Microbiol. 8:1035. doi: 10.3389/fmicb.2017.01035
Huang, Y., Yang, C., Xu, X. F., Xu, W., and Liu, S. (2020). Structural and functional properties of SARS-CoV-2 spike protein: potential antivirus drug development for COVID-19. Acta Pharmacol. Sin. 41, 1141–1149. doi: 10.1038/s41401-020-0485-4
Huang, Y. H., Kuo, H. C., Yang, Y. L., and Wang, F. S. (2019). MicroRNA-29a is a key regulon that regulates BRD4 and mitigates liver fibrosis in mice by inhibiting hepatic stellate cell activation. Int. J. Med. Sci. 16, 212–220. doi: 10.7150/ijms.29930
Hui, L., Chen, X., Haughey, N. J., and Geiger, J. D. (2012). Role of endolysosomes in HIV-1 Tat-induced neurotoxicity. ASN Neuro 4, 243–252. doi: 10.1042/AN20120017
Jahangard, Y., Monfared, H., Moradi, A., Zare, M., Mirnajafi-Zadeh, J., and Mowla, S. J. (2020). Therapeutic effects of transplanted exosomes containing miR-29b to a rat model of Alzheimer’s disease. Front. Neurosci. 14:564. doi: 10.3389/fnins.2020.00564
Jang, H. J., Kim, Y. Y., Lee, K. M., Shin, J. E., and Yun, J. (2022). The PINK1 activator niclosamide mitigate mitochondrial dysfunction and thermal hypersensitivity in a paclitaxel-induced drosophila model of peripheral neuropathy. Biomedicines 10:863. doi: 10.3390/biomedicines10040863
Jayaseelan, V. P., and Paramasivam, A. (2020). Repurposing calcium channel blockers as antiviral drugs. J. Cell Commun. Signal. 14, 467–468. doi: 10.1007/s12079-020-00579-y
Jensen, B. K., Monnerie, H., and Mannell, M. V. (2015). Altered oligodendrocyte maturation and myelin maintenance: the role of antiretrovirals in HIV-associated neurocognitive disorders. J. Neuropathol. Exp. Neurol. 74, 1093–1118. doi: 10.1097/NEN.0000000000000255
Jensen, B. K., Roth, L. M., Grinspan, J. B., and Jordan-Sciutto, K. L. (2019). White matter loss and oligodendrocyte dysfunction in HIV: a consequence of the infection, the antiretroviral therapy or both? Brain Res. 1724:146397. doi: 10.1016/j.brainres.2019.146397
Jhelum, P., Santos-Nogueira, E., Teo, W., Haumont, A., Lenoël, I., Stys, P. K., et al. (2020). Ferroptosis mediates cuprizone-induced loss of oligodendrocytes and demyelination. J. Neurosci. 40, 9327–9341. doi: 10.1523/JNEUROSCI.1749-20.2020
Jiang, S., and Bhaskar, K. (2020). Degradation and transmission of Tau by autophagic-endolysosomal networks and potential therapeutic targets for tauopathy. Front. Mol. Neurosci. 13:586731. doi: 10.3389/fnmol.2020.586731
Jiang, X., Stockwell, B. R., and Conrad, M. (2021). Ferroptosis: mechanisms, biology and role in disease. Nat. Rev. Mol. Cell. Biol. 22, 266–282. doi: 10.1038/s41580-020-00324-8
Joy, B., Sivadasan, R., Abraham, T. E., John, M., Sobhan, P. K., and Seervi, M. (2010). Lysosomal destabilization and cathepsin B contributes for cytochrome c release and caspase activation in embelin-induced apoptosis. Mol. Carcinog. 49, 324–336. doi: 10.1002/mc.20599
Jung, M., Philpott, M., Müller, S., Schulze, J., Badock, V., Eberspächer, U., et al. (2014). Affinity map of bromodomain protein 4 (BRD4) interactions with the histone H4 tail and the small molecule inhibitor JQ1. J. Biol. Chem. 289, 9304–9319. doi: 10.1074/jbc.M113.523019
Kagerer, S. M., van Bergen, J. M. G., Li, X., Quevenco, F. C., Gietl, A. F., Studer, S., et al. (2020). APOE4 moderates effects of cortical iron on synchronized default mode network activity in cognitively healthy old-aged adults. Alzheimers Dement (Amst.) 12:e12002. doi: 10.1002/dad2.12002
Kapralov, A. A., Yang, Q., Dar, H. H., Tyurina, Y. Y., Anthonymuthu, T. S., Kim, R., et al. (2020). Redox lipid reprogramming commands susceptibility of macrophages and microglia to ferroptotic death. Nat. Chem. Biol. 16, 278–290. doi: 10.1038/s41589-019-0462-8
Karuppagounder, S. S., Alin, L., Chen, Y., Brand, D., Bourassa, M. W., Dietrich, K., et al. (2018). N-acetylcysteine targets 5 lipoxygenase-derived, toxic lipids and can synergize with prostaglandin E2 to inhibit ferroptosis and improve outcomes following hemorrhagic stroke in mice. Ann. Neurol. 84, 854–872. doi: 10.1002/ana.25356
Kenkhuis, B., Somarakis, A., de Haan, L., Dzyubachyk, O., IJsselsteijn, M. E., de Miranda, N. F. C. C., et al. (2021). Iron loading is a prominent feature of activated microglia in Alzheimer’s disease patients. Acta Neuropathol. Commun. 9:27. doi: 10.1186/s40478-021-01126-5
Khacho, M., Clark, A., Svoboda, D. S., MacLaurin, J. G., Lagace, D. C., Park, D. S., et al. (2017). Mitochondrial dysfunction underlies cognitive defects as a result of neural stem cell depletion and impaired neurogenesis. Hum. Mol. Genet. 26, 3327–3341. doi: 10.1093/hmg/ddx217
Khan, N., Chen, X., and Geiger, J. D. (2020). Role of endolysosomes in severe acute respiratory syndrome Coronavirus-2 infection and coronavirus disease 2019 pathogenesis: implications for potential treatments. Front. Pharmacol. 11:595888. doi: 10.3389/fphar.2020.595888
Kim, S. Y., Zhang, X., Schiattarella, G. G., Altamirano, F., Ramos, T. A. R., French, K. M., et al. (2020). Epigenetic Reader BRD4 (Bromodomain-Containing Protein 4) governs nucleus-encoded mitochondrial transcriptome to regulate cardiac function. Circulation 142, 2356–2370. doi: 10.1161/CIRCULATIONAHA.120.047239
Kimura-Kuroda, J., Nagashima, K., and Yasui, K. (1994). Inhibition of myelin formation by HIV-1 gp120 in rat cerebral cortex culture. Arch. Virol. 137, 81–99. doi: 10.1007/BF01311175
Knez, D., Brus, B., Coquelle, N., Sosič, I., Šink, R., Brazzolotto, X., et al. (2015). Structure-based development of nitroxoline derivatives as potential multifunctional anti-Alzheimer agents. Bioorg. Med. Chem. 23, 4442–4452. doi: 10.1016/j.bmc.2015.06.010
Kohnken, R., Wen, J., Mundy-Bosse, B., McConnell, K., Keiter, A., Grinshpun, L., et al. (2018). Diminished microRNA-29b level is associated with BRD4-mediated activation of oncogenes in cutaneous T-cell lymphoma. Blood 131, 771–781. doi: 10.1182/blood-2017-09-805663
Korb, E., Herre, M., Zucker-Scharff, I., Darnell, R. B., and Allis, C. D. (2015). BET protein Brd4 activates transcription in neurons and BET inhibitor Jq1 blocks memory in mice. Nat. Neurosci. 18, 1464–1473. doi: 10.1038/nn.4095
Kos, J., Mitrović, A., and Mirković, B. (2014). The current stage of cathepsin B inhibitors as potential anticancer agents. Future Med. Chem. 6, 1355–1371. doi: 10.4155/fmc.14.73
Kreher, C., Favret, J., Maulik, M., and Shin, D. (2021). Lysosomal functions in glia associated with neurodegeneration. Biomolecules 11:400. doi: 10.3390/biom11030400
Kuo, C. L., Pilling, L. C., Atkins, J. L., Masoli, J. A. H., Delgado, J., Kuchel, G. A., et al. (2020). APOE e4 genotype predicts severe COVID-19 in the UK biobank community cohort. J. Gerontol. A Biol. Sci. Med. Sci. 75, 2231–2232. doi: 10.1093/gerona/glaa131
Kurz, T., Gustafsson, B., and Brunk, U. T. (2006). Intralysosomal iron chelation protects against oxidative stress-induced cellular damage. FEBS J. 273, 3106–3117. doi: 10.1111/j.1742-4658.2006.05321.x
Kuzu, O. F., Gowda, R., Noory, M. A., and Robertson, G. P. (2017). Modulating cancer cell survival by targeting intracellular cholesterol transport. Br. J. Cancer 117, 513–524. doi: 10.1038/bjc.2017.200
Lackner, P., Kuenz, B., Reindl, M., Morandell, M., Berger, T., Schmutzhard, E., et al. (2010). Antibodies to myelin oligodendrocyte glycoprotein in HIV-1 associated neurocognitive disorder: a cross-sectional cohort study. J. Neuroinflamm. 7:79. doi: 10.1186/1742-2094-7-79
Lam, F. C., Kong, Y. W., Huang, Q., Vu Han, T. L., Maffa, A. D., Kasper, E. M., et al. (2020). BRD4 prevents the accumulation of R-loops and protects against transcription–replication collision events and DNA damage. Nat. Commun. 11:4083. doi: 10.1038/s41467-020-17503-y
Le-Nhat-Thuy, G., Nguyen Thi, N., Pham-The, H., Dang Thi, T. A., Nguyen Thi, H., Nguyen Thi, T. H., et al. (2020). Synthesis and biological evaluation of novel quinazoline-triazole hybrid compounds with potential use in Alzheimer’s disease. Bioorg. Med. Chem. Lett. 30:127404. doi: 10.1016/j.bmcl.2020.127404
Li, X., Baek, G., Ramanand, S. G., Sharp, A., Gao, Y., Yuan, W., et al. (2018). BRD4 promotes DNA repair and mediates the formation of TMPRSS2-ERG gene rearrangements in prostate cancer. Cell Rep. 22, 796–808. doi: 10.1016/j.celrep.2017.12.078
Li, Y. Y., Fang, J., and Ao, G. Z. (2017). Cathepsin B and L inhibitors: a patent review (2010 - present). Expert Opin. Ther. Pat. 27, 643–656. doi: 10.1080/13543776.2017.1272572
Lin, Y., Epstein, D. L., and Liton, P. B. (2010). Intralysosomal iron induces lysosomal membrane permeabilization and cathepsin D-mediated cell death in trabecular meshwork cells exposed to oxidative stress. Invest. Ophthalmol. Vis. Sci. 51, 6483–6495. doi: 10.1167/iovs.10-5410
Lisi, L., Laudati, E., Miscioscia, T. F., Dello Russo, C., Topai, A., and Navarra, P. (2016). Antiretrovirals inhibit arginase in human microglia. J. Neurochem. 136, 363–372. doi: 10.1111/jnc.13393
Liu, J., Bandyopadhyay, I., Zheng, L., Khdour, O. M., and Hecht, S. M. (2020). Antiferroptotic activity of phenothiazine analogues: a novel therapeutic strategy for oxidative stress related disease. ACS Med. Chem. Lett. 11, 2165–2173. doi: 10.1021/acsmedchemlett.0c00293
López, O. V., Gorantla, S., Segarra, A. C., Andino Norat, M. C., Álvarez, M., Skolasky, R. L., et al. (2019). Sigma-1 receptor antagonist (BD1047) decreases Cathepsin B secretion in HIV-infected macrophages exposed to cocaine. J. Neuroimmune Pharmacol. 14, 226–240. doi: 10.1007/s11481-018-9807-4
Lu, J., Hou, Y., Ge, S., Wang, X., Wang, J., Hu, T., et al. (2021). Screened antipsychotic drugs inhibit SARS-CoV-2 binding with ACE2 in vitro. Life Sci. 266:118889. doi: 10.1016/j.lfs.2020.118889
Lu, P., Qu, X., and Shen, Y. (2016). The BET inhibitor OTX015 reactivates latent HIV-1 through P-TEFb. Sci. Rep. 6:24100. doi: 10.1038/srep24100
Lu, X., Yu, H., Liu, S. H., Brodsky, F. M., and Peterlin, B. M. (1998). Interactions between HIV1 Nef and vacuolar ATPase facilitate the internalization of CD4. Immunity 8, 647–656. doi: 10.1016/s1074-7613(00)80569-5
Lu, Y., Li, C. M., Wang, Z., Ross, C. R. II, Chen, J., Dalton, J. T., et al. (2009). Discovery of 4-substituted methoxybenzoyl-aryl-thiazole as novel anticancer agents: synthesis, biological evaluation, and structure-activity relationships. J. Med. Chem. 52, 1701–1711. doi: 10.1021/jm801449a
Mahoney-Sánchez, L., Bouchaoui, H., Ayton, S., Devos, D., Duce, J. A., and Devedjian, J. C. (2021). Ferroptosis and its potential role in the physiopathology of Parkinson’s Disease. Prog. Neurobiol. 196:101890. doi: 10.1016/j.pneurobio.2020.101890
Majerníková, N., den Dunnen, W. F. A., and Dolga, A. M. (2021). The potential of ferroptosis-targeting therapies for Alzheimer’s disease: from mechanism to transcriptomic analysis. Front. Aging Neurosci. 13:745046. doi: 10.3389/fnagi.2021.745046
Mangan, D. (2021). Iron: an underrated factor in aging. Aging (Albany NY) 13, 23407–23415. doi: 10.18632/aging.203612
Meleddu, R., Distinto, S., Corona, A., Bianco, G., Cannas, V., Esposito, F., et al. (2015). (3Z)-3-(2-[4-(aryl)-1,3-thiazol-2-yl]hydrazin-1-ylidene)-2,3-dihydro-1H-indol-2-one derivatives as dual inhibitors of HIV-1 reverse transcriptase. Eur. J. Med. Chem. 93, 452–460.
Milanini, B., Samboju, V., Cobigo, Y., Paul, R., Javandel, S., Hellmuth, J., et al. (2019). Longitudinal brain atrophy patterns and neuropsychological performance in older adults with HIV-associated neurocognitive disorder compared with early Alzheimer’s disease. Neurobiol. Aging 82, 69–76. doi: 10.1016/j.neurobiolaging.2019.07.006
Miotto, G., Rossetto, M., Di Paolo, M. L., Orian, L., Venerando, R., Roveri, A., et al. (2020). Insight into the mechanism of ferroptosis inhibition by ferrostatin-1. Redox Biol. 28:101328. doi: 10.1016/j.redox.2019.101328
Mita, M. M., and Mita, A. C. (2020). Bromodomain inhibitors a decade later: a promise unfulfilled? Br. J. Cancer 123, 1713–1714. doi: 10.1038/s41416-020-01079-x
Mitrović, A., Mirković, B., Sosič, I., Gobec, S., and Kos, J. (2016). Inhibition of endopeptidase and exopeptidase activity of cathepsin B impairs extracellular matrix degradation and tumour invasion. Biol. Chem. 397, 165–174. doi: 10.1515/hsz-2015-0236
Miyanishi, K., Sato, A., Kihara, N., Utsunomiya, R., and Tanaka, J. (2021). Synaptic elimination by microglia and disturbed higher brain functions. Neurochem. Int. 142:104901. doi: 10.1016/j.neuint.2020.104901
Monteleone, K., Selvaggi, C., Cacciotti, G., Falasca, F., Mezzaroma, I., D’Ettorre, G., et al. (2015). MicroRNA-29 family expression and its relation to antiviral immune response and viro-immunological markers in HIV-1-infected patients. BMC Infect. Dis. 15:51. doi: 10.1186/s12879-015-0768-4
Mujtaba, S., Zeng, L., and Zhou, M. M. (2007). Structure and acetyl-lysine recognition of the bromodomain. Oncogene 26, 5521–5527. doi: 10.1038/sj.onc.1210618
Müller, M., Jäkel, L., Bruinsma, I. B., Claassen, J. A., Kuiperij, H. B., and Verbeek, M. M. (2016). MicroRNA-29a is a candidate biomarker for Alzheimer’s disease in cell-free cerebrospinal fluid. Mol. Neurobiol. 53, 2894–2899. doi: 10.1007/s12035-015-9156-8
Nagakannan, P., Islam, M. I., Conrad, M., and Eftekharpour, E. (2021). Cathepsin B is an executioner of ferroptosis. Biochim. Biophys. Acta Mol. Cell. Res. 1868:118928. doi: 10.1016/j.bbamcr.2020.118928
Nakanishi, H. (2020). Microglial cathepsin B as a key driver of inflammatory brain diseases and brain aging. Neural Regen. Res. 15, 25–29. doi: 10.4103/1673-5374.264444
Nardacci, R., Ciccosanti, F., Marsella, C., Ippolito, G., Piacentini, M., and Fimia, G. M. (2017). Role of autophagy in HIV infection and pathogenesis. J. Intern. Med. 281, 422–432. doi: 10.1111/joim.12596
Nasrabady, S. E., Rizvi, B., Goldman, J. E., and Brickman, A. M. (2018). White matter changes in Alzheimer’s disease: a focus on myelin and oligodendrocytes. Acta Neuropathol. Commun. 6:22. doi: 10.1186/s40478-018-0515-3
Neely, M., Kalyesubula, I., Bagenda, D., Myers, C., and Olness, K. (2003). Effect of chloroquine on human immunodeficiency virus (HIV) vertical transmission. Afr. Health Sci. 3, 61–67.
Niyomdecha, N., Suptawiwat, O., Boonarkart, C., Jitobaom, K., and Auewarakul, P. (2020). Inhibition of human immunodeficiency virus type 1 by niclosamide through mTORC1 inhibition. Heliyon 6:e04050. doi: 10.1016/j.heliyon.2020.e04050
Nobuta, H., Yang, N., Ng, Y. H., Marro, S. G., Sabeur, K., Chavali, M., et al. (2019). Oligodendrocyte death in Pelizaeus-Merzbacher disease is rescued by iron chelation. Cell Stem Cell 25, 531.e6–541.e6. doi: 10.1016/j.stem.2019.09.003
Núñez, G., Sakamoto, K., and Soares, M. P. (2018). Innate nutritional immunity. J. Immunol. 201, 11–18. doi: 10.4049/jimmunol.1800325
O’Brien, J., Hayder, H., Zayed, Y., and Peng, C. (2018). Overview of MicroRNA biogenesis, mechanisms of actions, and circulation. Front. Endocrinol. (Lausanne) 9:402. doi: 10.3389/fendo.2018.00402
Osorio, C., Sfera, A., Anton, J. J., Thomas, K. G., Andronescu, C. V., Li, E., et al. (2022). Virus-Induced membrane fusion in neurodegenerative disorders. Front. Cell. Infect. Microbiol. 12:845580. doi: 10.3389/fcimb.2022.845580
Paakinaho, A., Koponen, M., Tiihonen, M., Kauppi, M., Hartikainen, S., and Tolppanen, A. M. (2022). Disease-modifying antirheumatic drugs and risk of parkinson disease: nested case-control study of people with rheumatoid arthritis. Neurology 98, e1273–e1281. doi: 10.1212/WNL.0000000000013303
Padmanabhan, P., Desikan, R., and Dixit, N. M. (2020). Targeting TMPRSS2 and Cathepsin B/L together may be synergistic against SARS-CoV-2 infection. PLoS Comput. Biol. 16:e1008461. doi: 10.1371/journal.pcbi.1008461
Patton, S. M., Wang, Q., and Hulgan, T. (2017). Cerebrospinal fluid (CSF) biomarkers of iron status are associated with CSF viral load, antiretroviral therapy, and demographic factors in HIV-infected adults. Fluids Barriers CNS 14:11. doi: 10.1186/s12987-017-0058-1
Paul, R. K., Singh, N. S., Khadeer, M., Moaddel, R., Sanghvi, M., Green, C. E., et al. (2014). (R,S)-Ketamine metabolites (R,S)-norketamine and (2S,6S)-hydroxynorketamine increase the mammalian target of rapamycin function. Anesthesiology 121, 149–159. doi: 10.1097/ALN.0000000000000285
Pedrera, L., Espiritu, R. A., and Ros, U. (2021). Ferroptotic pores induce Ca2+ fluxes and ESCRT-III activation to modulate cell death kinetics. Cell Death Differ. 28, 1644–1657. doi: 10.1038/s41418-020-00691-x
Pereira, P. A., Tomás, J. F., Queiroz, J. A., Figueiras, A. R., and Sousa, F. (2016). Recombinant pre-miR-29b for Alzheimer’s disease therapeutics. Sci. Rep. 6:19946. doi: 10.1038/srep19946
Pérez-Salvia, M., and Esteller, M. (2017). Bromodomain inhibitors and cancer therapy: from structures to applications. Epigenetics 12, 323–339. doi: 10.1080/15592294.2016.1265710
Petretich, M., Demont, E. H., and Grandi, P. (2020). Domain-selective targeting of BET proteins in cancer and immunological diseases. Curr. Opin. Chem. Biol. 57, 184–193. doi: 10.1016/j.cbpa.2020.02.003
Pichet Binette, A., Theaud, G., Rheault, F., Roy, M., Collins, D. L., Levin, J., et al. (2021). Bundle-specific associations between white matter microstructure and Aβ and tau pathology in preclinical Alzheimer’s disease. Elife 10:e62929. doi: 10.7554/eLife.62929
Platt, F. M., Boland, B., and van der Spoel, A. C. (2012). The cell biology of disease: lysosomal storage disorders: the cellular impact of lysosomal dysfunction. J. Cell. Biol. 199, 723–734. doi: 10.1083/jcb.201208152
Polanco, J. C., Hand, G. R., Briner, A., Li, C., and Götz, J. (2021). Exosomes induce endolysosomal permeabilization as a gateway by which exosomal tau seeds escape into the cytosol. Acta Neuropathol. 141, 235–256. doi: 10.1007/s00401-020-02254-3
Posso, M. C., Domingues, F. C., Ferreira, S., and Silvestre, S. (2022). Development of phenothiazine hybrids with potential medicinal interest: a review. Molecules 27:276. doi: 10.3390/molecules27010276
Prachayasittikul, S., Pingaew, R., Worachartcheewan, A., Sinthupoom, N., Prachayasittikul, V., Ruchirawat, S., et al. (2017). Roles of pyridine and pyrimidine derivatives as privileged scaffolds in anticancer agents. Mini Rev. Med. Chem. 17, 869–901. doi: 10.2174/1389557516666160923125801
Quincozes-Santos, A., Bobermin, L. D., Tonial, R. P., Bambini-Junior, V., Riesgo, R., and Gottfried, C. (2010). Effects of atypical (risperidone) and typical (haloperidol) antipsychotic agents on astroglial functions. Eur. Arch. Psychiatry Clin. Neurosci. 260, 475–481. doi: 10.1007/s00406-009-0095-0
Rao, S. S., and Adlard, P. A. (2018). Untangling Tau and Iron: exploring the interaction between Iron and Tau in neurodegeneration. Front. Mol. Neurosci. 11:276. doi: 10.3389/fnmol.2018.00276
Ravi, S., Peña, K. A., Chu, C. T., and Kiselyov, K. (2016). Biphasic regulation of lysosomal exocytosis by oxidative stress. Cell Calcium. 60, 356–362. doi: 10.1016/j.ceca.2016.08.002
Ripa, R., Dolfi, L., Terrigno, M., Pandolfini, L., Savino, A., Arcucci, V., et al. (2017). MicroRNA miR-29 controls a compensatory response to limit neuronal iron accumulation during adult life and aging. BMC Biol. 15:9. doi: 10.1186/s12915-017-0354-x
Rizzollo, F., More, S., Vangheluwe, P., and Agostinis, P. (2021). The lysosome as a master regulator of iron metabolism. Trends Biochem. Sci. 46, 960–975. doi: 10.1016/j.tibs.2021.07.003
Roederer, M., Ela, S. W., Staal, F. J., Herzenberg, L. A., and Herzenberg, L. A. (1992). N-acetylcysteine: a new approach to anti-HIV therapy. AIDS Res. Hum. Retroviruses 8, 209–217. doi: 10.1089/aid.1992.8.209
Ru, W., and Tang, S. J. (2017). HIV-associated synaptic degeneration. Mol. Brain 10:40. doi: 10.1186/s13041-017-0321-z
Ruan, H., Hao, S., Young, P., and Zhang, H. (2015). Targeting Cathepsin B for cancer therapies. Horiz Cancer Res. 56, 23–40.
Ruelas, D. S., and Greene, W. C. (2013). An integrated overview of HIV-1 latency. Cell 155, 519–529. doi: 10.1016/j.cell.2013.09.044
Sakamaki, J. I., Wilkinson, S., Hahn, M., Tasdemir, N., O’Prey, J., Clark, W., et al. (2017). Bromodomain protein BRD4 is a transcriptional repressor of autophagy and lysosomal function. Mol. Cell. 66, 517.e9–532.e9. doi: 10.1016/j.molcel.2017.04.027
Schneider, J. L., Miller, A. M., and Woesner, M. E. (2016). Autophagy and schizophrenia: a closer look at how dysregulation of neuronal cell homeostasis influences the pathogenesis of schizophrenia. Einstein J. Biol. Med. 31, 34–39. doi: 10.23861/EJBM201631752
Schornberg, K., Matsuyama, S., Kabsch, K., Delos, S., Bouton, A., and White, J. (2006). Role of endosomal cathepsins in entry mediated by the Ebola virus glycoprotein. J. Virol. 80, 4174–4178. doi: 10.1128/JVI.80.8.4174-4178.2006
Sebastián-Serrano, A., Merchán-Rubira, J., Di Lauro, C., Bianchi, C., Soria-Tobar, L., Narisawa, S., et al. (2022). TNAP upregulation is a critical factor in Tauopathies and its blockade ameliorates neurotoxicity and increases life-expectancy. Neurobiol. Dis. 165:105632. doi: 10.1016/j.nbd.2022.105632
Shankaran, P., Madlenakova, M., Hajkova, V., Jilich, D., Svobodova, I., Horinek, A., et al. (2017). Effects of heme degradation products on reactivation of latent HIV-1. Acta Virol. 61, 86–96. doi: 10.4149/av_2017_01_86
Sharma, I. (2021). Interrogating the impact of combination antiretroviral therapies on HIV-associated neurocognitive disorders. HIV Med. 22, 783–790. doi: 10.1111/hiv.13142
Shen, Y. T., Gu, Y., Su, W. F., Zhong, J. F., Jin, Z. H., Gu, X. S., et al. (2016). Rab27b is involved in lysosomal exocytosis and proteolipid protein trafficking in oligodendrocytes. Neurosci. Bull. 32, 331–340. doi: 10.1007/s12264-016-0045-6
Shimizu, S., Khan, M. Z., Hippensteel, R. L., Parkar, A., Raghupathi, R., and Meucci, O. (2007). Role of the transcription factor E2F1 in CXCR4-mediated neurotoxicity and HIV neuropathology. Neurobiol. Dis. 25, 17–26. doi: 10.1016/j.nbd.2006.08.004
Smail, R. C., and Brew, B. J. (2018). HIV-associated neurocognitive disorder. Handb. Clin. Neurol. 152, 75–97. doi: 10.1016/B978-0-444-63849-6.00007-4
Song, Q., Meng, B., Xu, H., and Mao, Z. (2020). The emerging roles of vacuolar-type ATPase-dependent Lysosomal acidification in neurodegenerative diseases. Transl. Neurodegener. 9:17. doi: 10.1186/s40035-020-00196-0
Stolke, D., Weidner, A., and Dietz, H. (1980). Das Verhalten lysosomaler Enzyme des Hirngewebes auf die Narkose unter Ketamine [The effect of ketamine on the lysosomal enzymes of the brain tissue (author’s transl)]. Anasth. Intensivther. Notfallmed. 15, 224–227. doi: 10.1055/s-2007-1005142
Straus, M. R., Bidon, M. K., Tang, T., Jaimes, J. A., Whittaker, G. R., and Daniel, S. (2021). Inhibitors of L-Type calcium channels show therapeutic potential for treating SARS-CoV-2 infections by preventing virus entry and spread. ACS Infect. Dis. 7, 2807–2815. doi: 10.1021/acsinfecdis.1c00023
Subburayan, K., Thayyullathil, F., Pallichankandy, S., Cheratta, A. R., and Galadari, S. (2020). Superoxide-mediated ferroptosis in human cancer cells induced by sodium selenite. Transl. Oncol. 13:100843. doi: 10.1016/j.tranon.2020.100843
Sui, S., Zhang, J., Xu, S., Wang, Q., Wang, P., and Pang, D. (2019). Ferritinophagy is required for the induction of ferroptosis by the bromodomain protein BRD4 inhibitor (+)-JQ1 in cancer cells. Cell Death Dis. 10:331. doi: 10.1038/s41419-019-1564-7
Sun, Y., Zheng, Y., Wang, C., and Liu, Y. (2018). Glutathione depletion induces ferroptosis, autophagy, and premature cell senescence in retinal pigment epithelial cells. Cell Death Dis. 9:753. doi: 10.1038/s41419-018-0794-4
Tanaka, Y., Yamada, K., and Satake, K. (2019). Seeding activity-based detection uncovers the different release mechanisms of seed-competent tau versus inert tau via lysosomal exocytosis. Front. Neurosci. 13:1258. doi: 10.3389/fnins.2019.01258
Tang, D., Chen, X., Kang, R., and Kroemer, G. (2021). Ferroptosis: molecular mechanisms and health implications. Cell Res. 31, 107–125. doi: 10.1038/s41422-020-00441-1
Tang, X., Chen, W., Liu, H., Liu, N., Chen, D., Tian, D., et al. (2022). Research progress on SLC7A11 in the regulation of cystine/cysteine metabolism in tumors. Oncol. Lett. 23:47. doi: 10.3892/ol.2021.13165
Tardiolo, G., Bramanti, P., and Mazzon, E. (2018). Overview on the effects of N-Acetylcysteine in neurodegenerative diseases. Molecules 23:3305. doi: 10.3390/molecules23123305
Tasdemir, N., Banito, A., Roe, J. S., Alonso-Curbelo, D., Camiolo, M., Tschaharganeh, D. F., et al. (2016). BRD4 connects enhancer remodeling to senescence immune surveillance. Cancer Discov. 6, 612–629. doi: 10.1158/2159-8290.CD-16-0217
Toomey, C. B., Cauvi, D. M., Hamel, J. C., Ramirez, A. E., and Pollard, K. M. (2014). Cathepsin B regulates the appearance and severity of mercury-induced inflammation and autoimmunity. Toxicol. Sci. 142, 339–349. doi: 10.1093/toxsci/kfu189
Tripathi, A., Thangaraj, A., Chivero, E. T., Periyasamy, P., Burkovetskaya, M. E., Niu, F., et al. (2020). N-Acetylcysteine reverses antiretroviral-mediated microglial activation by attenuating autophagy-lysosomal dysfunction. Front. Neurol. 11:840. doi: 10.3389/fneur.2020.00840
Tripathi, A., Thangaraj, A., Chivero, E. T., Periyasamy, P., Callen, S., Burkovetskaya, M. E., et al. (2019). Antiretroviral-Mediated microglial activation involves dysregulated autophagy and lysosomal dysfunction. Cells 8:1168. doi: 10.3390/cells8101168
Tripathi, M., Yadav, S., Kumar, V., Kumar, R., Tripathi, M., Gaikwad, S., et al. (2016). HIV encephalitis with subcortical tau deposition: imaging pathology in vivo using F-18 THK 5117. Eur. J. Nucl. Med. Mol. Imaging 43, 2456–2457. doi: 10.1007/s00259-016-3473-7
Tsujimura, A., Taguchi, K., Watanabe, Y., Tatebe, H., Tokuda, T., Mizuno, T., et al. (2015). Lysosomal enzyme cathepsin B enhances the aggregate forming activity of exogenous α-synuclein fibrils. Neurobiol. Dis. 73, 244–253. doi: 10.1016/j.nbd.2014.10.011
van Asbeck, B. S., Georgiou, N. A., van der Bruggen, T., Oudshoorn, M., Nottet, H. S., and Marx, J. J. (2001). Anti-HIV effect of iron chelators: different mechanisms involved. J. Clin. Virol. 20, 141–147. doi: 10.1016/s1386-6532(00)00122-0
Varalda, M., Antona, A., and Bettio, V. (2020). Psychotropic drugs show anticancer activity by disrupting mitochondrial and lysosomal function. Front. Oncol. 10:562196. doi: 10.3389/fonc.2020.562196
Vela, J. M. (2020). Repurposing Sigma-1 receptor ligands for COVID-19 therapy? Front. Pharmacol. 11:582310. doi: 10.3389/fphar.2020.582310
Veloria, J. R., Li, L., Breen, G. A. M., and Goux, W. J. (2017). Novel cell model for tauopathy induced by a Cell-permeable Tau-related peptide. ACS Chem. Neurosci. 8, 2734–2745. doi: 10.1021/acschemneuro.7b00275
von Bernhardi, R., Eugenín-von Bernhardi, L., and Eugenín, J. (2015). Microglial cell dysregulation in brain aging and neurodegeneration. Front. Aging Neurosci. 7:124. doi: 10.3389/fnagi.2015.00124
Wallet, C., De Rovere, M., and Van Assche, J. (2019). Microglial cells: the main HIV-1 reservoir in the brain. Front. Cell Infect. Microbiol. 9:362. doi: 10.3389/fcimb.2019.00362
Wan, Y. Q., Feng, J. G., Li, M., Wang, M. Z., Liu, L., Liu, X., et al. (2018). Prefrontal cortex miR-29b-3p plays a key role in the antidepressant-like effect of ketamine in rats. Exp. Mol. Med. 50, 1–14. doi: 10.1038/s12276-018-0164-4
Wang, J., Li, G. L., Ming, S. L., Wang, C. F., Shi, L. J., Su, B. Q., et al. (2020). BRD4 inhibition exerts anti-viral activity through DNA damage-dependent innate immune responses. PLoS Pathog. 16:e1008429. doi: 10.1371/journal.ppat.1008429
Wang, M. P., Joshua, B., Jin, N. Y., Du, S. W., and Li, C. (2021). Ferroptosis in viral infection: the unexplored possibility. Acta Pharmacol. Sin. [Online ahead of print], doi: 10.1038/s41401-021-00814-1
Wang, S., Jiang, Y., Liu, Y., Liu, Q., Sun, H., Mei, M., et al. (2022). Ferroptosis promotes microtubule-associated protein tau aggregation via GSK-3β activation and proteasome inhibition. Mol. Neurobiol. 59, 1486–1501. doi: 10.1007/s12035-022-02731-8
Weiss, A., Touret, F., Baronti, C., Gilles, M., Hoen, B., Nougairède, A., et al. (2021). Niclosamide shows strong antiviral activity in a human airway model of SARS-CoV-2 infection and a conserved potency against the Alpha (B.1.1.7), Beta (B.1.351) and Delta variant (B.1.617.2). PLoS One 16:e0260958. doi: 10.1371/journal.pone.0260958
Whitton, B., Okamoto, H., Packham, G., and Crabb, S. J. (2018). Vacuolar ATPase as a potential therapeutic target and mediator of treatment resistance in cancer. Cancer Med. 7, 3800–3811. doi: 10.1002/cam4.1594
Xu, X., Mann, M., Qiao, D., Li, Y., Zhou, J., and Brasier, A. R. (2021). Bromodomain containing protein 4 (BRD4) regulates expression of its interacting coactivators in the innate response to respiratory syncytial virus. Front. Mol. Biosci. 8:728661. doi: 10.3389/fmolb.2021.728661
Xu, Y., Du, S., Marsh, J. A., Horie, K., Sato, C., Ballabio, A., et al. (2021). TFEB regulates lysosomal exocytosis of tau and its loss of function exacerbates tau pathology and spreading. Mol. Psychiatry 26, 5925–5939. doi: 10.1038/s41380-020-0738-0
Yambire, K. F., Rostosky, C., Watanabe, T., Pacheu-Grau, D., Torres-Odio, S., Sanchez-Guerrero, A., et al. (2019). Impaired lysosomal acidification triggers iron deficiency and inflammation in vivo. Elife 8:e51031. doi: 10.7554/eLife.51031
Yang, M., and Lai, C. L. (2020). SARS-CoV-2 infection: can ferroptosis be a potential treatment target for multiple organ involvement? Cell Death Discov. 6:130. doi: 10.1038/s41420-020-00369-w
Yim, Y., Choi, J. D., Cho, J. H., Moon, Y., Han, S. H., and Moon, W. J. (2022). Magnetic susceptibility in the deep gray matter may be modulated by apolipoprotein E4 and age with regional predilections: a quantitative susceptibility mapping study. Neuroradiology [Online ahead of print], doi: 10.1007/s00234-021-02859-9
Zenón-Meléndez, C. N., Carrasquillo Carrión, K., Cantres Rosario, Y., Roche Lima, A., and Meléndez, L. M. (2022). Inhibition of Cathepsin B and SAPC secreted by HIV-infected macrophages reverses common and unique apoptosis pathways. J. Proteome Res. 21, 301–312. doi: 10.1021/acs.jproteome.1c00187
Zhang, S., Bai, P., Lei, D., Liang, Y., Zhen, S., Bakiasi, G., et al. (2022). Degradation and inhibition of epigenetic regulatory protein BRD4 exacerbate Alzheimer’s disease-related neuropathology in cell models. J. Biol. Chem. 298:101794.
Zhao, T., Yang, Q., Xi, Y., Xie, Z., Shen, J., Li, Z., et al. (2022). Ferroptosis in rheumatoid arthritis: a potential therapeutic strategy. Front. Immunol. 13:779585. doi: 10.3389/fimmu.2022.779585
Keywords: ferroptosis, neurodegenerative disorders, iron, BRD4, miR-29
Citation: Sfera A, Thomas KG, Andronescu CV, Jafri N, Sfera DO, Sasannia S, Zapata-Martín del Campo CM and Maldonado JC (2022) Bromodomains in Human-Immunodeficiency Virus-Associated Neurocognitive Disorders: A Model of Ferroptosis-Induced Neurodegeneration. Front. Neurosci. 16:904816. doi: 10.3389/fnins.2022.904816
Received: 25 March 2022; Accepted: 19 April 2022;
Published: 12 May 2022.
Edited by:
Isabella Zanella, University of Brescia, ItalyReviewed by:
Lindsay Festa, University of Pennsylvania, United StatesLarisa Tratnjek, University of Ljubljana, Slovenia
Copyright © 2022 Sfera, Thomas, Andronescu, Jafri, Sfera, Sasannia, Zapata-Martín del Campo and Maldonado. This is an open-access article distributed under the terms of the Creative Commons Attribution License (CC BY). The use, distribution or reproduction in other forums is permitted, provided the original author(s) and the copyright owner(s) are credited and that the original publication in this journal is cited, in accordance with accepted academic practice. No use, distribution or reproduction is permitted which does not comply with these terms.
*Correspondence: Adonis Sfera, ZHIuc2ZlcmFAZ21haWwuY29t