- 1Clinical College of Ophthalmology, Tianjin Medical University, Tianjin, China
- 2Tianjin Key Lab of Ophthalmology and Visual Science, Tianjin Eye Hospital, Tianjin, China
- 3Tianjin Eye Hospital Optometric Center, Tianjin, China
- 4Nankai University Affiliated Eye Hospital, Nankai University, Tianjin, China
Objective: To study the effect of treatment zone (TZ) decentration on axial length growth (ALG) in adolescents after wearing the orthokeratology lenses (OK lenses).
Materials and methods: This retrospective clinical study selected 251 adolescents who were fitted OK lenses at the Clinical College of Ophthalmology, Tianjin Medical University (Tianjin, China) from January 2018–December 2018 and wore them continuously for >12 months. The age of the subjects was 8–15 years, spherical equivalent (SE): −1.00 to −5.00 diopter (D), and astigmatism ≤ 1.50 D. The corneal topography were recorded at baseline and 1-, 6-, and 12-month visits, and the axial length (AL) were recorded at baseline and 6-, 12-month visits. The data of the right eye were collected for statistical analysis.
Results: The subjects were divided into three groups according to the decentration distance of the TZ after wearing lenses for 1 month: 56 cases in the mild (<0.5 mm), 110 in the moderate (0.5–1.0 mm), and 85 in the severe decentration group (>1.0 mm). A significant difference was detected in the ALG between the three groups after wearing lenses for 6 and 12 months (F = 10.223, P < 0.001; F = 13.380, P < 0.001, respectively). Among these, the 6- and 12-month ALG of the mild decentration group was significantly higher than that of the other two groups. Multivariable linear regression analysis showed that age, baseline SE, and 1-month decentration distance associated with the 12-month ALG (P < 0.001, P < 0.001, and P = 0.001, respectively).
Conclusion: The decentration of the TZ of the OK lens affected the growth of the AL in adolescents, i.e., the greater the decentration, the slower the ALG.
Introduction
Currently, myopia accounts for about 30% of the global population and is expected to rise to 50% by 2050 (Holden et al., 2016). Similarly, the current situation of myopia is not optimistic in China. The overall myopia rate of children and adolescents is about 50%, and that in high school students is 80%, among which about 10–20% is high myopia (Chen M. et al., 2018; Dong et al., 2020). The condition is irreversible and incurable once it occurs, and high myopia causes various complications, such as cataract, glaucoma, retinal detachment, and macular degeneration (Flitcroft, 2012; Tsai et al., 2019). In the population with high myopia, the risk of fundus lesions is >40 times higher than emmetropia (Hu et al., 2020). These complications are closely related to the excessive axial length growth (ALG) in high myopia. The goal of myopia control is to slow the progression and prevent a low myopia from becoming a moderate or high myopia (Bullimore and Brennan, 2019).
Orthokeratology lenses (OK lenses) are rigid gas-permeable contact lenses (RGPCL) with a special reverse geometric design. These lenses can reshape the front surface of the cornea during sleep, temporarily reduce myopia, and improve visual acuity (Lian et al., 2014). Recent studies (Cho et al., 2005; Kakita et al., 2011) have confirmed that compared to single-vision spectacle, OK lenses can reduce the ALG by 40–60%. However, some OK lens wearers experienced that the center of the OK lens treatment zone (TZ) is often inconsistent with the center of the pupil. Presently, only a few studies have assessed the influence of TZ decentration on ALG with a small sample size (Wang and Yang, 2019; Chen et al., 2020).
The present study aimed to observe the effect of different decentration states of the lenses on the ALG after wearing OK lenses and explore the association between the ALG and individual factors, such as age, gender, spherical equivalent (SE), astigmatism, corneal curvature, corneal astigmatism, pupil diameter, TZ decentration distance, and TZ decentration direction.
Materials and methods
Subjects
This retrospective clinical study was conducted to collect adolescent myopia subjects who underwent OK lens fitting at the Clinical College of Ophthalmology, Tianjin Medical University (Tianjin, China), from January 2018–December 2018. The inclusion criteria were as follows: (1) Age: 8–15 years; (2) Subjective refraction under cycloplegia: −5.00 diopter (D) ≤ SE ≤ −1.00 D, astigmatism ≤ 1.50 D; (3) The flat K (FK) of the cornea was 39.00D ∼ 46.00D; (4) Monocular corrected visual acuity of OK lenses ≥ 0.8 (Visual acuity expressed as decimal visual acuity); (5) Both eyes were fitted with OK lenses for the first time and worn continuously for ≥12 months. Exclusion criteria: (1) Stop wearing the lenses for ≥7 days for any reason during the follow-up, including corneal and conjunctival adverse events, lens broken, and lens parameter adjustment, etc; (2) Obvious keratoconjunctival complications, glare, diplopia, or any other symptoms during the follow-up; (3) Contraindications to wearing contact lenses for ocular and systemic diseases. This study adhered to the tenets of the Declaration of Helsinki and was approved by the Institutional Ethical Committee Review Board of Tianjin Eye Hospital (Scientific Research Review No. 2022005). The right eye data were collected for statistical analysis to avoid the symbiosis of binocular myopia.
Fitting of the orthokeratology lenses
All subjects were fitted by a team of one ophthalmologist and three optometrists. The OK lenses were fitted according to the manufacturer’s instructions. The OK lenses selected in this study had a four-zone reverse-geometric design (Euclid Systems Orthokeratology; Euclid System, Herndon, VA, USA) manufactured at the Boston Equalens II material, with a nominal DK 90 × 10–11 (cm2/s) (mlO2/mL ⋅ kPa–1), and the lenses diameters were 10.0–11.2 mm. The difference of the total diameter of the lens was located in the fitting arc. The diameter of the optical zone fixed at 6.2 mm; the width of the reverse arc was 0.5 mm; the width of the fitting arc ranged from 0.9 to 1.5 mm, and the curvature range of the fitting arc ranged from 39.00D to 46.00D; the width of the peripheral arc was 0.5 mm, the curvature radius of the peripheral arc was 11.5 mm.
Refraction and corneal curvature examination
Cycloplegic refraction was performed at baseline. Three drops of 0.5% Topicamide/0.5% deoxyepinephrine hydrochloride were dropped at 5-min intervals. Subjective refraction was assessed at least 30 min after the last drop of eye drops. SE = spherical diopter + 1/2 cylindrical diopter. The FK and steep K (SK) of the cornea were measured by an auto refraction keratometer (ARK-510A, Nidek, Aichi, Japan).
Measurement of axial length
The AL was measured at baseline and at 6- and 12-month follow-up using an ocular biometric (IOL-Master 500, Carl Zeiss, Ag, Jena, Germany). Five consecutive measurements were recorded, and the average value was taken for data analysis at each follow-up. The difference between the AL and the baseline value at each follow-up was considered the ALG.
Corneal topography and evaluation of treatment zone decentration
Corneal topography (TMS-4, Tomey, Nagoya, Japan) was measured after four consecutive measurements of each eye; the best imaging quality and good gaze were selected for data analysis. All measurements were conducted by the same technician. The decentration distance and direction were calculated according to the method of Guo et al. (2022): (1) decentration distance: the TZ was defined as the area enclosed by points with 0 diopter changes on the tangential subtractive topography maps, and the tolerance of each zero point at the edge of the TZ was ± 0.10 D. Briefly, the refractive power change was obtained by hovering the cursor above each reference point and the measurement shown on the image was recorded. As shown in Figure 1A, the area surrounded by the red circle is the TZ, line segments AB and CD were the horizontal and vertical diameters of the TZ, respectively. They intersect at O point, which was the central point of the TZ. When the cursor is hovered over the O point and the topographic map software, it automatically displays the distance between the O point and the pupil center, i.e., the decentration distance. This decentration distance was divided into mild decentration (<0.5 mm), medium decentration (0.5–1 mm), and severe decentration (>1.0 mm) according to the method of Tsai and Lin (2000). (2) The position of the central point of the TZ on the coordinate axis was calculated as follows: the pupil center was taken as the origin, and the horizontal (X)-axis and vertical (Y)-axis were set up, as shown in Figure 1B. The decentration angle of the central point of the TZ (O point) was displayed by the topographic map software (α-angle), i.e., the included angle between the connecting line between the center of the TZ and the pupil center and the X-axis. The TZ decentration along X-axis and Y-axis was calculated as follows: X = cos α × decentration distance, Y = sinα × decentration distance, with positive signs representing nasal or superior decentration.
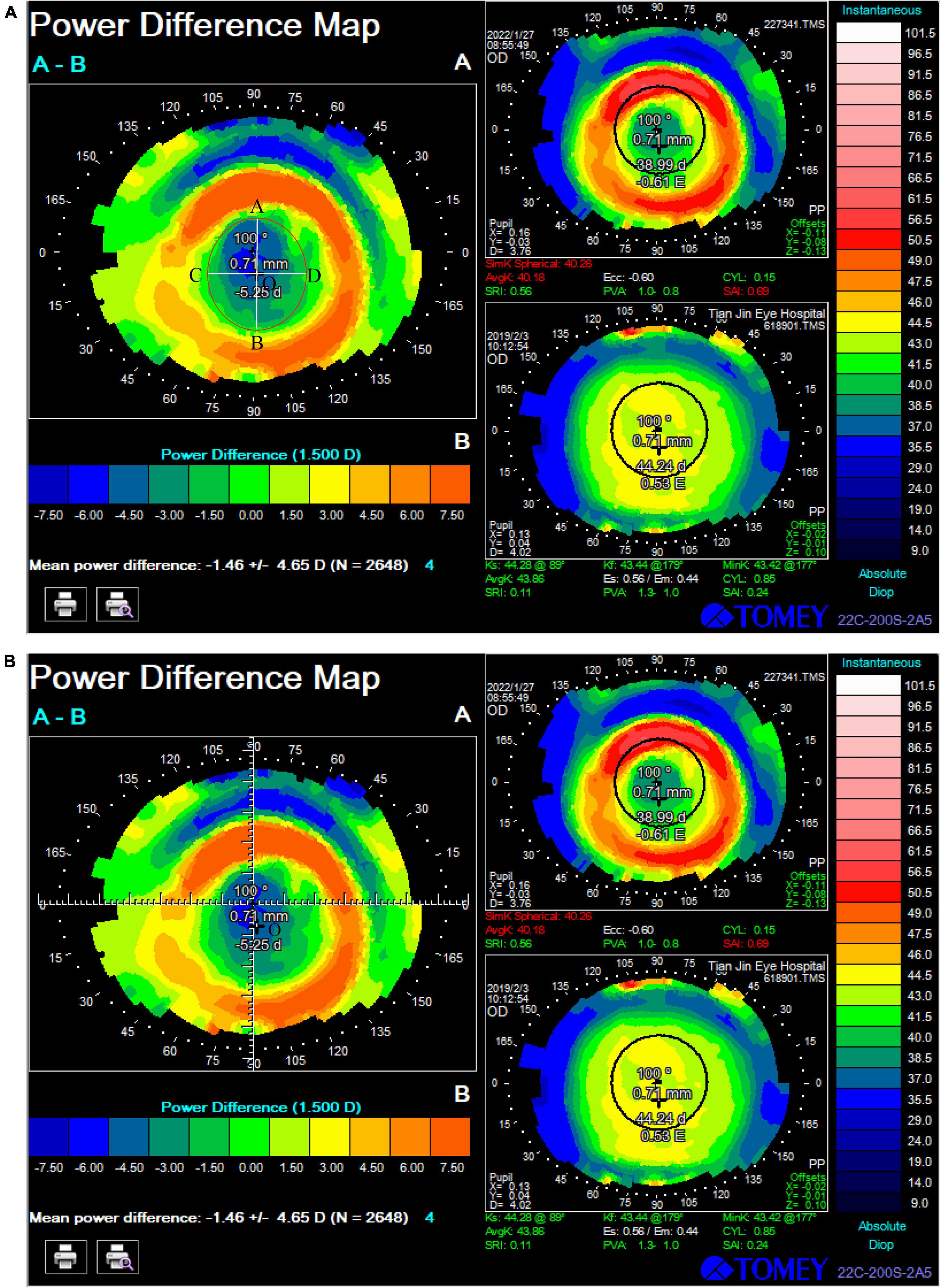
Figure 1. Calculation method of treatment zone decentration: (A) Decentration distance: the area surrounded by the red circle is the treatment zone; line segments AB and CD were the horizontal and vertical diameters of the treatment zone; O point was the central point of the treatment zone. The distance from O point to the center of the pupil is 0.71 mm. (B) The position of the central point of the treatment zone (O point) on the coordinate axis: the pupil center was taken as the origin, and the horizontal (X)-axis and vertical (Y)-axis were set up. The decentration angle of O point was displayed by the topographic map software, which was 100°, The treatment zone decentration along X-axis and Y-axis was calculated as follows: X = cos 100 × 0.71, Y = –sin 100 × 0.71.
Pupil diameter
Pupil diameter were determined with Corneal topography (TMS-4, Tomey, Nagoya, Japan). In the Tomey topography system, pupil diameter value was provided for each map. Corneal topographic maps were measured in a windowless examination room with lighting of approximate 300–310 lx. In this study, pupil diameters were recorded from baseline topographic maps of subjects for statistical analysis.
Measurements and follow-up visits
After dispensing the lens, the subjects were instructed to wear and care for them properly. Moreover, the subjects were advised to wear their OK lenses every night for 8–10 h. The examinations were performed before any lens wear (baseline) and 1 day, 1 week, 1 month, and 3 months, and then every 3 months until 1 year. Each follow-up included naked visual acuity, subjective and objective refraction (ARK-1, Nidek, Aichi, Japan), slit-lamp anterior segment examination (SL-D701, Topcon, Yamagata, Japan), and corneal topography. The AL was measured at 6- and 12-month follow-ups. Corneal topography data at 1-, 6-, and 12-month visits and AL at 6- and 12-month visits were used for statistical analysis.
Statistical analysis
SPSS statistical software (version 20.0, IBM SPSS, Chicago, IL, USA) was used for data analysis. Quantitative data were tested for normality using quantile-quantile plots, described as mean ± standard deviation, and the classification data were described as the number of cases and percentages. Repeated measures analysis of variance (ANOVA) was used to compare the decentration distance at different follow-ups after wearing OK lenses. For consistent comparison between baseline parameters of different groups, one-way ANOVA was used for continuous variables, and the chi-square test was used for classification variables. The 6- and 12-month ALG between different groups was compared by one-way ANOVA. Pearson’s correlation analysis was used to assess the simple association between the 12-month ALG and decentration distance achieved at 1-month visit, and Spearman’s correlation analysis was used to assess the association between the 12-month ALG and decentration direction. This association was further examined using linear regression analysis. Variables, including baseline age, gender, SE, astigmatism, FK, SK, corneal astigmatism, pupil diameter, and 1-month decentration distance and direction were first examined using univariate linear regression analysis. Variables with statistically significant associations (P < 0.05) with the 12-month ALG in univariate analyses were entered into the multivariate regression model to further test whether it has statistical significance on the 12-month ALG. P < 0.05 was considered a statistically significant difference.
Results
Baseline data
A total of 251 subjects (251 eyes) were enrolled in this study, including 112 (44.6%) males and 139 (55.4%) females. The mean baseline age was 10.47 ± 1.97 years, the mean SE was −3.23 ± 1.14 D, and the mean astigmatism was −0.55 ± 0.58 D. FK and SK were 42.82 ± 1.29 D and 44.03 ± 1.40 D, respectively. The mean corneal astigmatism was 1.21 ± 0.56 D, and the mean baseline AL was 24.94 ± 0.83 mm. The mean baseline pupil diameter was 4.14 ± 0.30 mm.
Decentration distance at different follow-up visits
The decentration distance at 1-, 6-, and 12-month visits was 0.83 ± 0.32 mm, 0.84 ± 0.33 mm, and 0.84 ± 0.32 mm, respectively. The difference was not statistically significant (F = 2.877, P = 0.066), indicating stable decentration of the lenses at the 1-month visit. The subsequent studies were statistically analyzed based on the data of the 1-month visit.
Decentration distribution
After wearing the lens for 1 month, the decentration direction of the TZ was as follows: superior 2 cases (0.8%), superonasal 4 cases (1.6%), nasal 5 cases (2.0%), inferonasal 9 cases (3.6%), inferior 9 cases (3.6%), inferotemporal 129 cases (51.4%), temporal 36 cases (14.3%), and superotemporal 57 cases (22.7%). Among these, inferotemporal decentration was the most commonly observed phenotype. Figure 2 is the distribution of the center of the treatment zone for all subjects.
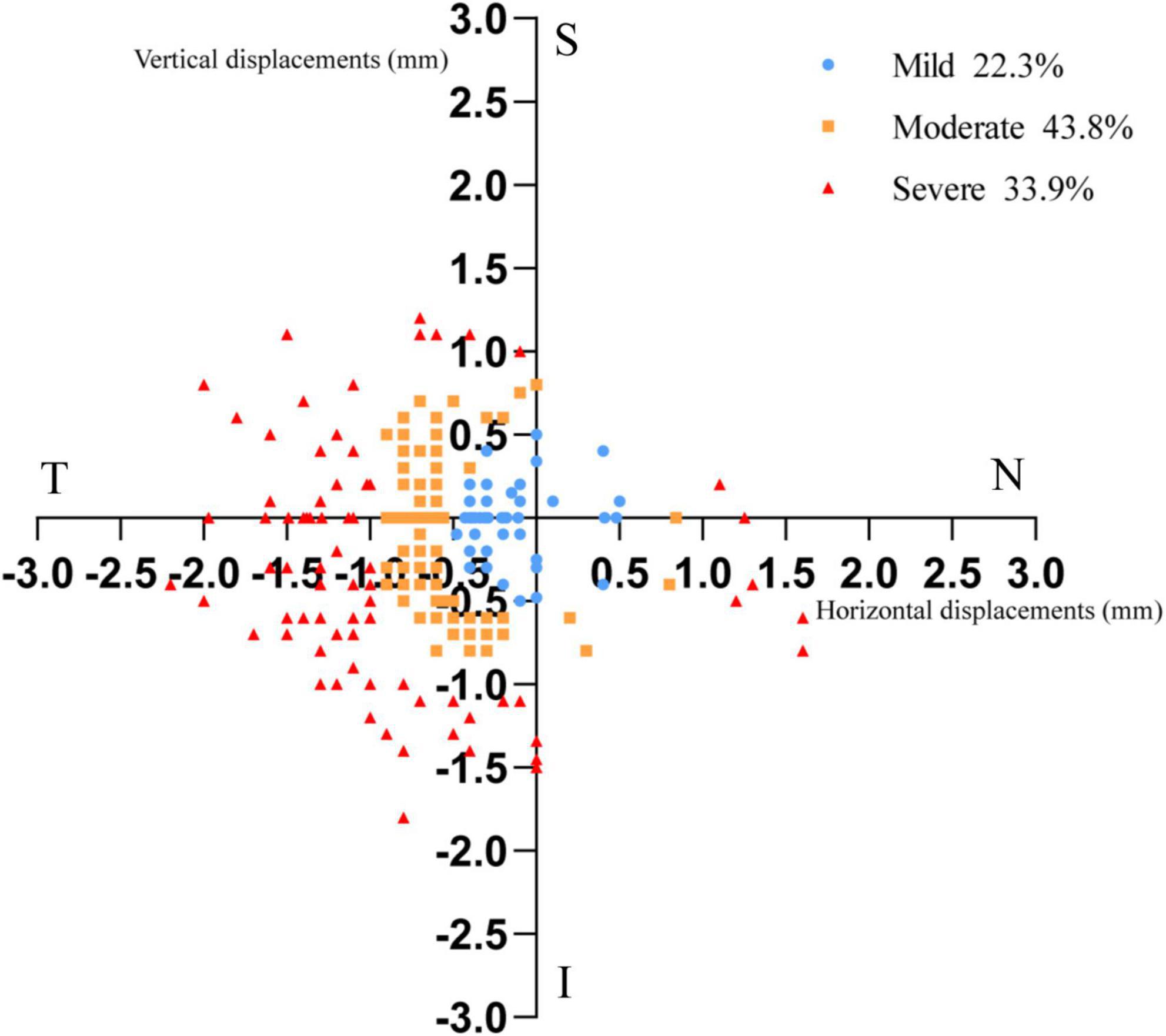
Figure 2. The distribution of the center of the treatment zone for all subjects. Each point depicted in the figure represents the location of the center point of each subject’s treatment zone in an axis with the pupil center as the origin. Each plotted point shows the distance and angle of the center of treatment zone from the pupil center. The spacing of each grid on the coordinate axis represents 0.5 mm on the cornea. (T: temple; N: nasal; S: superior; I: inferior).
Baseline data in different groups
At 1-month visit, 56 (22.3%) cases in the mild decentration group (30 males and 26 females), with a mean decentration of 0.41 ± 0.09 mm; 110 (43.8%) cases in the moderate decentration group (51 males and 59 females), with a mean decentration of 0.76 ± 0.13 mm; and 85 (33.9%) cases in the severe decentration group (31 males and 54 females), with a mean decentration of 1.20 ± 0.14 mm were assimilated. No significant difference was detected in the gender between different groups (χ2 = 3.918, P = 0.141), while astigmatism, SK, and corneal astigmatism showed statistical significance among the three groups (P < 0.001, P = 0.030, and P < 0.001, respectively). The other baseline parameters did not show any significant difference among the three groups (P > 0.05), as shown in Table 1.
Axial length growth
Statistically significant differences were observed in the 6- and 12-month ALG among the three groups, as shown in Table 2.
Moreover, the 6- and 12-month ALG of the mild decentration group was significantly higher than that of the moderate (P = 0.004 and P < 0.001) and the severe decentration groups (P < 0.001 and P < 0.001), while no significant difference was noted between the moderate and severe decentration groups (P = 0.194, P = 0.244), as shown in Figure 3.
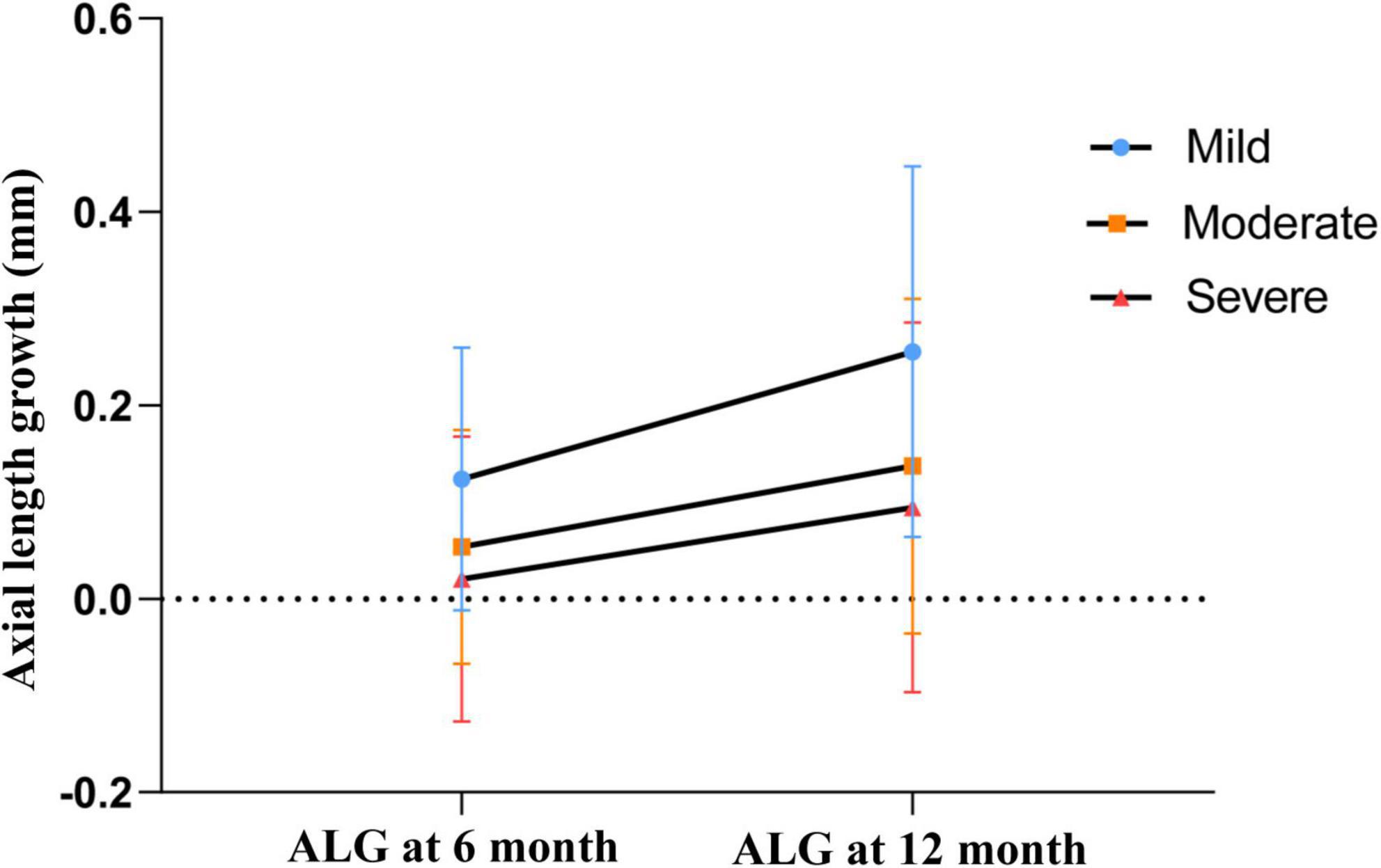
Figure 3. 6- and 12-month axial length growth among different groups. The mean values are represented by blue dots (mild decentration group), orange squares (moderate decentration group) and red triangles (severe decentration group), respectively. Error bars represent standard deviation of the mean.
12-month axial length growth and individual factors
The 12-month ALG showed a significantly simple negative association with the 1-month decentration distance (r = −0.289, P < 0.001), but showed no association with 1-month decentration direction (r = −0.028, P = 0.657), as shown in Figure 4.
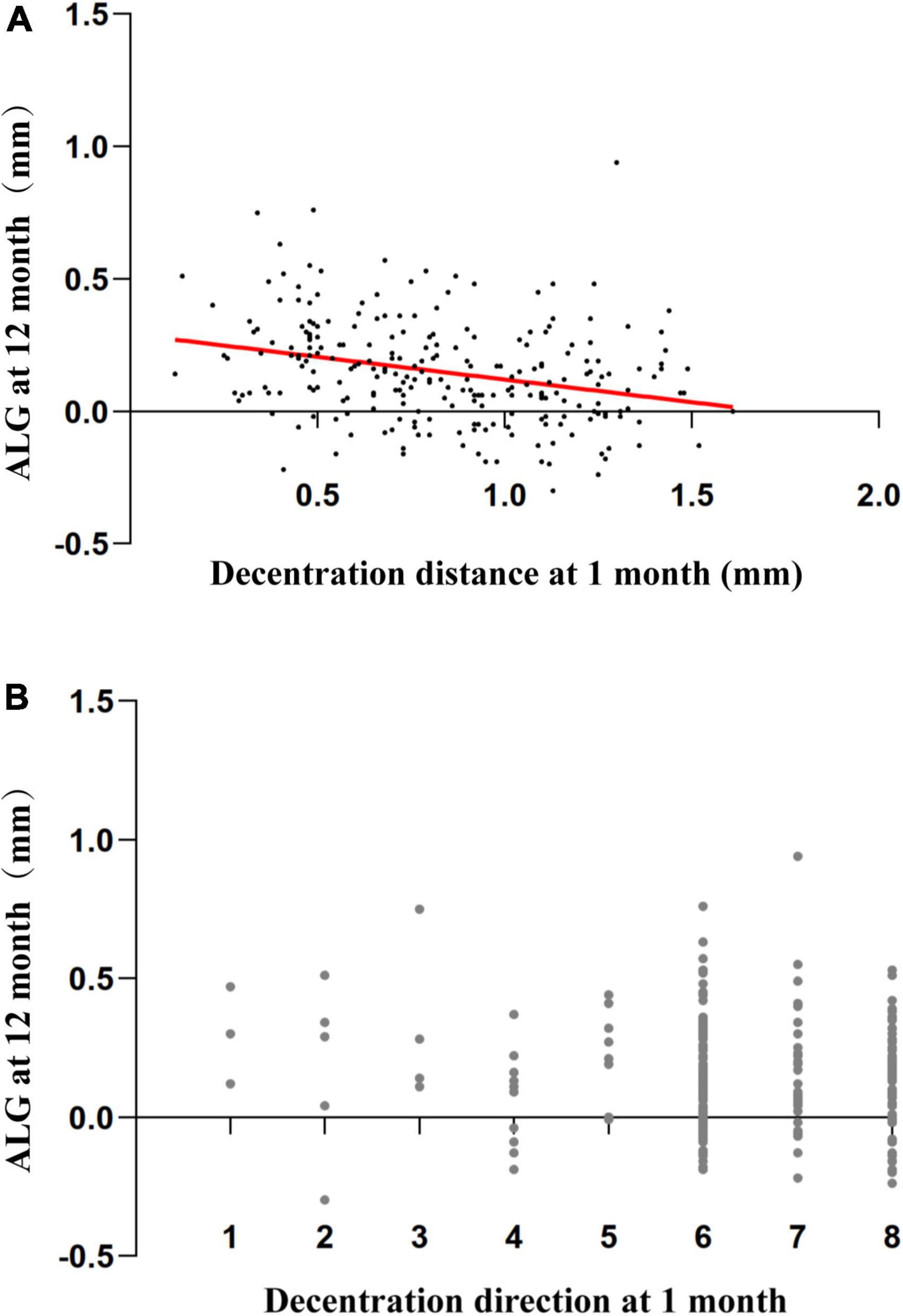
Figure 4. Association between 12-month axial length growth and decentration factors. (A) Scatterplots demonstrating the association between the 12-month axial length growth and 1-month decentration distance. There was a significant association between the parameters (Pearson’s correlation analysis: r = –0.289, P < 0.001) (B) Scatterplots demonstrating the association between the 12-month axial length growth and 1-month decentration direction (1: superior; 2: superonasal; 3: nasal; 4: inferonasal; 5: inferior; 6: inferotemporal; 7: temporal; 8: superotemporal). There was no association between 12-month axial length growth and 1-month decentration direction (Spearman’s correlation analysis: r = –0.028, P = 0.657).
Baseline age, SE, and 1-month decentration distance were significantly associated with 12-month ALG in univariate and multivariate linear regression analysis. Corneal astigmatism was associated with 12-month ALG in the univariate analysis, which became statistically insignificant in the multivariate regression model. Pupil diameter has no statistically associated with 12-month ALG in univariate or multivariate regression analyses, as shown in Table 3.
Discussion
In the present study, the 6- and 12-month ALG of the mild decentration group was significantly higher than that of the moderate and severe decentration groups. Baseline age and 1-month decentration negatively associated with 12-month ALG, while baseline SE is positively associated with 12-month ALG in univariate and multivariate linear regression analysis. However, the decentration direction and pupil diameter did not affect ALG. Corneal astigmatism was only associated with 12-month ALG in univariate regression analysis but not significantly in multivariate regression analysis.
The distribution of the treatment zone decentration
This study showed that the distribution of decentration direction is most common in the inferotemporal, followed by the superotemporal and the temporal, which is similar to the previous findings (Hiraoka et al., 2009; Chen J. et al., 2018). The elevated nasal and temporal sides of the human cornea are asymmetric. Typically, the nasal side is higher than the temporal side (Jaisankar et al., 2020). Hence, the lens is prone to deviate to the temporal side, which is relatively low in elevation. Chen et al. (2017) analyzed the corneal elevation data collected at the 8-mm chord and confirmed the inferotemporal quadrant to be the paracentral corneal region lowest in elevation. Therefore, our study showed the inferotemporal decentration was most common (as shown in Figure 2), that is consistent with previous findings.
Treatment zone decentration and axial length growth
The exact mechanism by which OK lens delays the progression of myopia remains unclear. The OK lens induced relative corneal refractive power shift (RCRPS) to produce myopic defocusing on the peripheral retina, thereby delaying the growth of AL, i.e., the “peripheral defocusing hypothesis,” which has been recognized by several investigators (Charman et al., 2006; Kang and Swarbrick, 2016; Santodomingo-Rubido et al., 2017). Recent studies have shown that the spatial distribution of relative corneal refractive power (RCRP) is more significant than a simple sum in delaying AL progression. Yang et al. (2021) and Jiang et al. (2021), respectively confirmed that the closer the RCRP is to the center of the cornea and the faster RCRP reaches the peak, the slower the ALG. The TZ decentration increases the corneal asymmetry and shifts the RCRP on one side of the cornea toward the center (Wang et al., 2018, 2021). Lin et al. (2021, 2022) demonstrated that the TZ decentration was significantly positively associated with summed RCRP within 1–2 mm chord radius, and RCRP was negatively associated with ALG. Therefore, a high TZ decentration can delay AL progression.
Another opinion was that there was a threshold of RCRP. Only when the defocus exceeds this threshold will the “stop” signal start to delay the ALG (Kang and Swarbrick, 2016). For the same average RCRP, the greater the variation in all directions, the more uneven the distribution and the slower the ALG (Gu et al., 2019). The current study did not find any association between decentration direction and 12-month ALG. Thus, it could be deduced that the RCRP need not reach or be above the threshold in every direction but only for a specific direction to reach the threshold. The larger the decentration distance of the lens, the more uneven the corneal shape and the greater chance for RCRP to reach the threshold, thus delaying ALG.
In the process of corneal reshaping, the characteristic morphological changes of the cornea will also increase the higher-order aberrations (Lau et al., 2020b). This phenomenon suggests that mechanisms other than myopic defocus may be involved in the inhibitory effect of the OK lenses on ALG (Lau et al., 2020a). Hiraoka et al. (2015) showed that the aberrations also played a major role in ALG delay after wearing the OK lenses; among all aberration factors, coma has the closest association with ALG. Some studies have shown that the aberration changes caused by lens decentration were mainly increased coma and spherical aberration (Lau et al., 2020b). These findings indicated that asymmetric corneal shapes, rather than concentric and radially symmetric shapes, have a considerable effect on the retardation of AL. Lin et al. (2020) indicated that increased myopia defocuses in the temporal retina is caused by the decentration of lenses toward the temporal side, resulting in 3 diopters myopic defocus of the temporal retina, while astigmatism and higher-order aberration also increased. Therefore, we hypothesized that the combination of these changes slowed the ALG after the TZ decentration.
Other factors and axial length growth
In the current study, greater baseline corneal astigmatism was related to slower axial growth in univariate regression analysis. This could be attributed to larger corneal astigmatism leading to lens decentration (Chen et al., 2017; Gu et al., 2019; Jiang et al., 2019). However, the association became statistically insignificant after adjusting for the effects of age, SE, and decentration distance using multivariate linear regression analysis. Therefore, the decentration of the TZ and not corneal astigmatism affected ALG.
The ALG of myopic children might be affected by several factors. Previous studies have shown that age is a baseline parameter significantly associated with axial growth, and the younger the age, the faster the axial growth (Zhong et al., 2015; Chen et al., 2020; Lin et al., 2021; Sun et al., 2022), which is consistent with the current findings. However, the association between ALG and baseline SE is also controversial (Vincent et al., 2021). Some studies reported a significant negative association between ALG and baseline SE, as the subjects had a wide range of baseline SE, usually between −0.75 D and −6.00 D (Wang et al., 2017; Lin et al., 2021; Yang et al., 2021). While in the studies that reported a lack of association between the two factors (Zhong et al., 2015; Hu et al., 2019), the subjects’ baseline SE was in a limited intermediate range between −1.00 D and −4.00 D. In this study, subjects had −1.00 D to −5.00 D range of baseline SE, and the sample size was large. The results showed a significant negative association between ALG and baseline SE.
Since the pupil diameter will affect the measurement of peripheral refraction (Mathur and Atchison, 2013; Romashchenko et al., 2020) and higher-order aberration (Applegate et al., 2007), the pupil diameter is a factor that must be considered when studying the influencing factors of ALG after wearing OK lenses. Our study showed that baseline pupil diameter do not affecting 12-month ALG. Two other longitudinal studies (Wang et al., 2017; Zhao et al., 2020), similar to our findings, found no association between pupil diameter and ALG in children Treated with OK lenses. Conversely, the study of Chen et al. (2012) showed that after 2 years’ follow-up, larger pupil diameter in dark environment was significantly associated with smaller ALG. In another comparative study of European children wearing OK lenses and single-vision spectacle (Santodomingo-Rubido et al., 2013), there was negative association between pupil diameter and ALG in children in the OK lens group. We found that studies showing an association between pupil diameter and ALG, measured pupil diameter in the dark. While the measurement of our baseline pupil diameter is based on the detection value of Tomey topographic software, which is measured in a bright environment. During the daytime, particularly near work tasks (Gislen et al., 2008), children’s pupil diameters are much smaller than that in dark environments. Therefore, studying the relationship between pupil diameter and ALG in bright environment is more instructive for clinical work.
Treatment zone decentration and adverse events
Severe Ortho-K lens decentration increased the risk of corneal adverse events, such as staining and indentation in the corneal epithelium (Maseedupally et al., 2016). In addition, severe TZ decentration increased the high-order aberration (Chen J. et al., 2018), reduced the contrast sensitivity (Hiraoka et al., 2009), and caused visual interference, such as glare and glowering (Nti and Berntsen, 2020). Therefore, in clinical practice, increasing the TZ decentration is not encouraged.
Limitations of this current study
Nevertheless, the present study has several limitations. Firstly, this is a retrospective study, and many factors, such as family history in refractive errors, duration of outdoor activities, and habits of reading, were not recorded and could potentially interfere with the findings. Secondly, according to the literature, we speculated that the decentration of the TZ increased the RCRP, but we did not measure the RCRP in different decentration groups, and hence the association between the decentration and the RCRP could not be tested. Thirdly, we also speculated that the decentration of the TZ increased the higher-order aberration; however, there was no measured aberration value. These speculations would be substantiated in future prospective studies with a rigorous design.
Conclusion
In conclusion, the TZ decentration of OK lenses can affect the ALG in adolescent myopia patients, and the greater the decentration distance, the slower the ALG. Thus, delaying the ALG by artificially creating decentration is not advocated.
Data availability statement
The original contributions presented in this study are included in the article/supplementary material, further inquiries can be directed to the corresponding author.
Ethics statement
This study adhered to the tenets of the Declaration of Helsinki and was reviewed and approved by the Institutional Ethical Committee Review Board of Tianjin Eye Hospital (Scientific Research Review No. 2022005). This is a retrospective clinical study, and the data have been anonymized. The requirement for informed consent was therefore waived.
Author contributions
SZ was responsible for data collection and analysis and manuscript drafting. XL contributed to the study design and revision of the manuscript. HZ and XY contributed to the data collection. LL provided the administrative, technical, and material support. SL conducted the statistical analysis. All authors made substantial intellectual contributions to this study and reviewed and approved the final version of the manuscript.
Funding
This present study was sponsored by Tianjin Health Research Project (No. TJWJ2021MS040), the National Natural Science Foundation of China (82171024), and the Tianjin Science and Technology Foundation (20JCYBJC01450). This study was also supported by the Tianjin Key Medical Discipline (Specialty) Construction Project.
Conflict of interest
The authors declare that the research was conducted in the absence of any commercial or financial relationships that could be construed as a potential conflict of interest.
Publisher’s note
All claims expressed in this article are solely those of the authors and do not necessarily represent those of their affiliated organizations, or those of the publisher, the editors and the reviewers. Any product that may be evaluated in this article, or claim that may be made by its manufacturer, is not guaranteed or endorsed by the publisher.
References
Applegate, R. A., Donnelly, W. J. III, Marsack, J. D., Koenig, D. E., and Pesudovs, K. (2007). Three-dimensional relationship between high-order root-mean-square wavefront error, pupil diameter, and aging. J. Opt. Soc. Am. A Opt. Image Sci. Vis. 24, 578–587. doi: 10.1364/josaa.24.000578
Bullimore, M. A., and Brennan, N. A. (2019). Myopia control: Why each diopter matters. Optom. Vis. Sci. 96, 463–465. doi: 10.1097/OPX.0000000000001367
Charman, W. N., Mountford, J., Atchison, D. A., and Markwell, E. L. (2006). Peripheral refraction in orthokeratology patients. Optom. Vis. Sci. 83, 641–648. doi: 10.1097/01.opx.0000232840.66716.af
Chen, J., Huang, W., Zhu, R., Jiang, J., and Li, Y. (2018). Influence of overnight orthokeratology lens fitting decentration on corneal topography reshaping. Eye Vis. 5:5. doi: 10.1186/s40662-018-0100-7
Chen, M., Wu, A., Zhang, L., Wang, W., Chen, X., Yu, X., et al. (2018). The increasing prevalence of Myopia and High Myopia among high school students in Fenghua city, eastern China: A 15-year population-based survey. BMC Ophthalmol. 18:159. doi: 10.1186/s12886-018-0829-8
Chen, R., Chen, Y., Lipson, M., Kang, P., Lian, H., Zhao, Y., et al. (2020). The Effect of Treatment Zone Decentration on Myopic Progression during Or-thokeratology. Curr. Eye Res. 45, 645–651. doi: 10.1080/02713683.2019.1673438
Chen, Z., Niu, L., Xue, F., Qu, X., Zhou, Z., Zhou, X., et al. (2012). Impact of pupil diameter on axial growth in orthokeratology. Optom. Vis. Sci. 89, 1636–1640. doi: 10.1097/OPX.0b013e31826c1831
Chen, Z., Xue, F., Zhou, J., Qu, X., Zhou, X., Shanghai, O., et al. (2017). Prediction of Orthokeratology Lens Decentration with Corneal Elevation. Optom. Vis. Sci. 94, 903–907. doi: 10.1097/OPX.0000000000001109
Cho, P., Cheung, S. W., and Edwards, M. (2005). The longitudinal orthokeratology research in children (LORIC) in Hong Kong: A pilot study on refractive changes and myopic control. Curr. Eye Res. 30, 71–80. doi: 10.1080/02713680590907256
Dong, L., Kang, Y. K., Li, Y., Wei, W. B., and Jonas, J. B. (2020). Prevalence and time trends of Myopia in children and adolescents in China: A systemic review and meta-analysis. Retina 40, 399–411. doi: 10.1097/IAE.0000000000002590
Flitcroft, D. I. (2012). The complex interactions of retinal, optical and environmental factors in Myopia aetiology. Prog. Retin. Eye Res. 31, 622–660. doi: 10.1016/j.preteyeres.2012.06.004
Gislen, A., Gustafsson, J., and Kroger, R. H. (2008). The accommodative pupil responses of children and young adults at low and intermediate levels of ambient illumination. Vision Res. 48, 989–993. doi: 10.1016/j.visres.2008.01.022
Gu, T., Gong, B., Lu, D., Lin, W., Li, N., He, Q., et al. (2019). Influence of corneal topographic parameters in the decentration of orthokeratology. Eye Contact Lens 45, 372–376. doi: 10.1097/ICL.0000000000000580
Guo, B., Wu, H., Cheung, S. W., and Cho, P. (2022). Manual and software-based measurements of treatment zone parameters and characteristics in children with slow and fast axial elongation in orthokeratology. Ophthalmic Physiol. Opt. 42, 773–785. doi: 10.1111/opo.12981
Hiraoka, T., Kakita, T., Okamoto, F., and Oshika, T. (2015). Influence of ocular wavefront aberrations on axial length elongation in myopic children treated with overnight orthokeratology. Ophthalmology 122, 93–100. doi: 10.1016/j.ophtha.2014.07.042
Hiraoka, T., Mihashi, T., Okamoto, C., Okamoto, F., Hirohara, Y., and Oshika, T. (2009). Influence of induced decentered orthokeratology lens on ocular higher-order wavefront aberrations and contrast sensitivity function. J. Cataract Refract. Surg. 35, 1918–1926. doi: 10.1016/j.jcrs.2009.06.018
Holden, B. A., Fricke, T. R., Wilson, D. A., Jong, M., Naidoo, K. S., Sankaridurg, P., et al. (2016). Global prevalence of Myopia and High Myopia and temporal trends from 2000 through 2050. Ophthalmology 123, 1036–1042. doi: 10.1016/j.ophtha.2016.01.006
Hu, Y., Ding, X., Guo, X., Chen, Y., Zhang, J., and He, M. (2020). Association of Age at Myopia Onset With Risk of High Myopia in Adulthood in a 12-Year Follow-up of a Chinese Cohort. JAMA Ophthalmol. 138, 1129–1134. doi: 10.1001/jamaophthalmol.2020.3451
Hu, Y., Wen, C., Li, Z., Zhao, W., Ding, X., and Yang, X. (2019). Areal summed corneal power shift is an important determinant for axial length elongation in myopic children treated with overnight orthokeratology. Br. J. Ophthalmol. 103, 1571–1575. doi: 10.1136/bjophthalmol-2018-312933
Jaisankar, D., Liu, Y., Kollbaum, P., Jaskulski, M., Gifford, P., Suheimat, M., et al. (2020). Nasal-temporal asymmetry in peripheral refraction with an aspheric Myopia control contact lens. Biomed. Opt. Express 11, 7376–7394. doi: 10.1364/BOE.406101
Jiang, F., Huang, X., Xia, H., Wang, B., Lu, F., Zhang, B., et al. (2021). The spatial distribution of relative corneal refractive power shift and axial growth in myopic children: Orthokeratology versus multifocal contact lens. Front. Neurosci. 15:686932. doi: 10.3389/fnins.2021.686932
Jiang, J., Lian, L., Wang, F., Zhou, L., Zhang, X., and Song, E. (2019). Comparison of toric and spherical orthokeratology lenses in patients with Astigmatism. J. Ophthalmol. 2019:4275269. doi: 10.1155/2019/4275269
Kakita, T., Hiraoka, T., and Oshika, T. (2011). Influence of overnight orthokeratology on axial elongation in childhood Myopia. Invest. Ophthalmol. Vis. Sci. 52, 2170–2174. doi: 10.1167/iovs.10-5485
Kang, P., and Swarbrick, H. (2016). New perspective on Myopia control with orthokeratology. Optom. Vis. Sci. 93, 497–503. doi: 10.1097/OPX.0000000000000826
Lau, J. K., Vincent, S. J., Cheung, S. W., and Cho, P. (2020b). The influence of orthokeratology compression factor on ocular higher-order aberrations. Clin. Exp. Optom. 103, 123–128. doi: 10.1111/cxo.12933
Lau, J. K., Vincent, S. J., Cheung, S. W., and Cho, P. (2020a). Higher-Order Aberrations and Axial Elongation in Myopic Children Treated With Orthokeratology. Invest. Ophthalmol. Vis. Sci. 61:22. doi: 10.1167/iovs.61.2.22
Lian, Y., Shen, M., Huang, S., Yuan, Y., Wang, Y., Zhu, D., et al. (2014). Corneal reshaping and wavefront aberrations during overnight orthokeratology. Eye Contact Lens 40, 161–168. doi: 10.1097/ICL.0000000000000031
Lin, W., Gu, T., Bi, H., Du, B., Zhang, B., and Wei, R. (2022). The treatment zone decentration and corneal refractive profile changes in children undergoing orthokeratology treatment. BMC Ophthalmol. 22:177. doi: 10.1186/s12886-022-02396-w
Lin, W., Li, N., Gu, T., Tang, C., Liu, G., Du, B., et al. (2021). The treatment zone size and its decentration influence axial elongation in children with orthokeratology treatment. BMC Ophthalmol. 21:362. doi: 10.1186/s12886-021-02123-x
Lin, Z., Duarte-Toledo, R., Manzanera, S., Lan, W., Artal, P., and Yang, Z. (2020). Two-dimensional peripheral refraction and retinal image quality in orthokeratology lens wearers. Biomed. Opt. Express 11, 3523–3533. doi: 10.1364/BOE.397077
Maseedupally, V. K., Gifford, P., Lum, E., Naidu, R., Sidawi, D., Wang, B., et al. (2016). Treatment Zone Decentration During Orthokeratology on Eyes with Corneal Toricity. Optom. Vis. Sci. 93, 1101–1111. doi: 10.1097/OPX.0000000000000896
Mathur, A., and Atchison, D. A. (2013). Peripheral refraction patterns out to large field angles. Optom. Vis. Sci. 90, 140–147. doi: 10.1097/OPX.0b013e31827f1583
Nti, A. N., and Berntsen, D. A. (2020). Optical changes and visual performance with orthokeratology. Clin. Exp. Optom. 103, 44–54. doi: 10.1111/cxo.12947
Romashchenko, D., Rosen, R., and Lundstrom, L. (2020). Peripheral refraction and higher order aberrations. Clin. Exp. Optom. 103, 86–94. doi: 10.1111/cxo.12943
Santodomingo-Rubido, J., Villa-Collar, C., Gilmartin, B., and Gutierrez-Ortega, R. (2013). Factors preventing Myopia progression with orthokeratology correction. Optom. Vis. Sci. 90, 1225–1236. doi: 10.1097/OPX.0000000000000034
Santodomingo-Rubido, J., Villa-Collar, C., Gilmartin, B., Gutierrez-Ortega, R., and Sugimoto, K. (2017). Long-term efficacy of orthokeratology contact lens wear in controlling the progression of childhood Myopia. Curr. Eye Res. 42, 713–720. doi: 10.1080/02713683.2016.1221979
Sun, L., Li, Z. X., Chen, Y., He, Z. Q., and Song, H. X. (2022). The effect of orthokeratology treatment zone decentration on Myopia progression. BMC Ophthalmol. 22:76. doi: 10.1186/s12886-022-02310-4
Tsai, A. S. H., Wong, C. W., Lim, L., Yeo, I., Wong, D., Wong, E., et al. (2019). Pediatric retinial detachment in an asian population with high prevalence of Myopia: Clinical characteristics, surgical outcomes, and prognostic factors. Retina 39, 1751–1760. doi: 10.1097/IAE.0000000000002238
Tsai, Y. Y., and Lin, J. M. (2000). Ablation centration after active eye-tracker-assisted photorefractive keratectomy and laser in situ keratomileusis. J. Cataract Refract. Surg. 26, 28–34. doi: 10.1016/s0886-3350(99)00328-4
Vincent, S. J., Cho, P., Chan, K. Y., Fadel, D., Ghorbani-Mojarrad, N., Gonzalez-Meijome, J. M., et al. (2021). CLEAR - Orthokeratology. Cont. Lens Anterior. Eye 44, 240–269. doi: 10.1016/j.clae.2021.02.003
Wang, A., and Yang, C. (2019). Influence of overnight orthokeratology lens treatment zone decentration on Myopia progression. J. Ophthalmol. 2019:2596953. doi: 10.1155/2019/2596953
Wang, B., Naidu, R. K., and Qu, X. (2017). Factors related to axial length elongation and Myopia progression in orthokeratology practice. PLoS One 12:e0175913. doi: 10.1371/journal.pone.0175913
Wang, D., Wen, D., Zhang, B., Lin, W., Liu, G., Du, B., et al. (2021). The association between fourier parameters and clinical parameters in myopic children undergoing orthokeratology. Curr. Eye Res. 46, 1637–1645. doi: 10.1080/02713683.2021.1917619
Wang, J., Yang, D., Bi, H., Du, B., Lin, W., Gu, T., et al. (2018). A New Method to Analyze the Relative Corneal Refractive Power and Its Association to Myopic Progression Control With Orthokeratology. Transl. Vis. Sci. Technol. 7:17. doi: 10.1167/tvst.7.6.17
Yang, X., Bi, H., Li, L., Li, S., Chen, S., Zhang, B., et al. (2021). The effect of relative corneal refractive power shift distribution on axial length growth in myopic children undergoing orthokeratology treatment. Curr. Eye Res. 46, 657–665. doi: 10.1080/02713683.2020.1820528
Zhao, Y., Hu, P., Chen, D., and Ni, H. (2020). Is it possible to predict progression of childhood Myopia using short-term axial change after orthokeratology? Eye Contact Lens 46, 136–140. doi: 10.1097/ICL.0000000000000665
Keywords: orthokeratology, myopia, axial length, decentration, refraction
Citation: Zhang S, Zhang H, Li L, Yang X, Li S and Li X (2022) Effect of treatment zone decentration on axial length growth after orthokeratology. Front. Neurosci. 16:986364. doi: 10.3389/fnins.2022.986364
Received: 05 July 2022; Accepted: 29 September 2022;
Published: 20 October 2022.
Edited by:
Pablo De Gracia, Midwestern University, United StatesReviewed by:
Daniela Lopes-Ferreira, Centro de Física das Universidades do Minho e Porto, PortugalKeyur Savla, University of Alabama at Birmingham, United States
Copyright © 2022 Zhang, Zhang, Li, Yang, Li and Li. This is an open-access article distributed under the terms of the Creative Commons Attribution License (CC BY). The use, distribution or reproduction in other forums is permitted, provided the original author(s) and the copyright owner(s) are credited and that the original publication in this journal is cited, in accordance with accepted academic practice. No use, distribution or reproduction is permitted which does not comply with these terms.
*Correspondence: Xuan Li, eHVhbmxpMDhAeWFob28uY29t