- 1Department of Neuropharmacology, QPS Austria GmbH, Grambach, Austria
- 2Institute for Biology, Karl-Franzens-Universität Graz, Graz, Austria
- 3Department of Pharmacy-Drug Sciences, University of Bari Aldo Moro, Bari, Italy
Introduction: Autism spectrum disorder (ASD) is a persistent neurodevelopmental condition characterized by two core behavioral symptoms: impaired social communication and interaction, as well as stereotypic, repetitive behavior. No distinct cause of ASD is known so far; however, excitatory/inhibitory imbalance and a disturbed serotoninergic transmission have been identified as prominent candidates responsible for ASD etiology.
Methods: The GABAB receptor agonist R-Baclofen and the selective agonist for the 5HT7 serotonin receptor LP-211 have been reported to correct social deficits and repetitive behaviors in mouse models of ASD. To evaluate the efficacy of these compounds in more details, we treated BTBR T+ Itpr3tf/J and B6.129P2-Fmr1tm1Cgr/J mice acutely with R-Baclofen or LP-211 and evaluated the behavior of animals in a series of tests.
Results: BTBR mice showed motor deficits, elevated anxiety, and highly repetitive behavior of self-grooming. Fmr1-KO mice exhibited decreased anxiety and hyperactivity. Additionally, Fmr1-KO mice’s ultrasonic vocalizations were impaired suggesting a reduced social interest and communication of this strain. Acute LP-211 administration did not affect the behavioral abnormalities observed in BTBR mice but improved repetitive behavior in Fmr1-KO mice and showed a trend to change anxiety of this strain. Acute R-Baclofen treatment improved repetitive behavior only in Fmr1-KO mice.
Conclusion: Our results add value to the current available data on these mouse models and the respective compounds. Yet, additional studies are needed to further test R-Baclofen and LP-211 as potential treatments for ASD therapy.
1. Introduction
Autism spectrum disorder (ASD) is a group of complex lifelong neurodevelopmental conditions affecting around 1−1.5% of the global population (Bougeard et al., 2021; Zeidan et al., 2022). The male-female incidence of ASD is 4:1 to 3:1 which has been repetitively observed and the focus of the ASD research lies therefore on the male population (Loomes et al., 2017; Posserud et al., 2021). Hallmark ASD symptoms are categorized as unusual reciprocal social communication and impaired communication as well as stereotypic, repetitive behaviors with restricted interest. Seizure, anxiety, and hyperactivity are comorbid ASD symptoms with an incidence of 40−78% (Murray, 2010; Zaboski and Storch, 2018). One important hypothesis consistent with these comorbidities is an imbalance of excitatory/inhibitory (E/I) neurotransmission in ASD (Geschwind and Levitt, 2007; Bourgeron, 2009; Gogolla et al., 2014; Uzunova et al., 2016; Culotta and Penzes, 2020; Canitano and Palumbi, 2021). Reduced GABAergic neurotransmission and fewer GABAergic interneurons are summarized as reduced GABAergic action in the autistic brain that has been detected both in humans and animal models (Kubas et al., 2012; Han et al., 2014; Cochran et al., 2015; Silverman et al., 2015; Robertson et al., 2016; Möhrle et al., 2021). Furthermore, genetic evidence strongly supports that the E/I imbalance effects ASD pathogenesis (Delahanty et al., 2011; Bonnet-Brilhault et al., 2016; Guo et al., 2017; Noroozi et al., 2018).
Hyperserotonemia is the first and most replicated biochemical abnormality identified in autistic individuals (Schain and Freedman, 1961; Anderson et al., 1987; Folk and Long, 1988; Leboyer et al., 1999; Pagan et al., 2014; Muller et al., 2016). The disturbed serotoninergic (5-hydroxytryptamine, 5-HT) system was identified as an underlying cause of neuropsychiatric disorders such as ASD, and manipulation of 5-HT signaling was consequentially shown to improve ASD-like behaviors in animal models (Kolevzon et al., 2006; Leopoldo et al., 2011; Costa et al., 2012, 2018; Lacivita et al., 2017; Saitow et al., 2020; Andersson et al., 2021; Lee et al., 2021).
BTBR T+ Itpr3tf/J mice (BTBR) are a widely used inbred mouse model that robustly exhibits ASD phenotypes of highly repetitive behaviors (Silverman et al., 2010a,2015), anxiety, exaggerated stress response (Benno et al., 2009; Pobbe et al., 2011), as well as social impairments (Silverman et al., 2015; Amodeo et al., 2021). Social approach impairment in BTBR mice can be improved by the application of the GABAAR-active neurosteroid ganaxolone (Kazdoba et al., 2016). Another GABAergic compound, clonazepam, improved sociability in BTBR mice (Han et al., 2014). The selective GABAB receptor agonist, R-Baclofen, is reported to reverse social deficits and repetitive behavior in BTBR mice (Silverman et al., 2015). This mouse model is shown to have reduced serotonin transporter (SERT) activity in various brain regions as well as increased 5-HT1A receptor levels in the hippocampus (Speranza et al., 2017).
Another model to investigate ASD is the Fragile X syndrome (FXS) mouse model. FXS is the most frequent form of inherited intellectual disability and monogenic cause of ASD affecting 1/4,000 male and 1/7,000 female subjects (Rogers et al., 2001; Kazdoba et al., 2014). FXS is caused by a CGG repeat mutation on the X chromosome containing the FMR1 gene resulting in hypermethylation and therefore silencing of this gene leading to reduced levels of the Fragile X mental retardation protein (FMR1P) (Telias, 2019). Fmr1-knock out (KO) mice present hyperactivity, repetitive behavior, seizure, and social impairments as well as altered anxiety, thus recapitulating FXS symptoms of humans (Bakker et al., 1994; Spencer et al., 2005; Mineur et al., 2006; Olmos-Serrano et al., 2011; Pietropaolo et al., 2011; Rotschafer et al., 2012; Dolan et al., 2013; Qin et al., 2015). Increased dendritic spine density and abnormal spinal morphology are known neuronal features in Fmr1-KO mice (Comery et al., 1997; Booker et al., 2019). These mice also present alterations in Group I metabotropic glutamate receptor type 5 (mGlu5) and GABAergic system deficits in the cortex, hippocampus, amygdala, striatum, and subiculum (Paluszkiewicz et al., 2011; Booker et al., 2019; Afshar et al., 2022). Decreased expression of GABAergic signaling combined with an increase in glutamatergic pathway activity results in hyperexcitability of these mice (Gibson et al., 2008; Paluszkiewicz et al., 2011).
Chemicals correcting this hyperexcitation could be useful as potential therapeutic to treat FXS-related symptoms. R-Baclofen (STX209), the most potent enantiomer of Baclofen (Lal et al., 2009), has already been used to alleviate FXS behaviors by an acute treatment as well as to correct spine density by a chronic treatment (Henderson et al., 2012). Moreover, the acute R-Baclofen treatment was shown to significantly improve auditory-evoked gamma oscillations, but not the impaired sociability in Fmr1-KO mice (Sinclair et al., 2017).
Based on results from preclinical studies, clinical studies on R-Baclofen were performed, resulting in some side effects such as sedation of the animals, but it was concluded that R-Baclofen is a potential candidate to improve sociability in FXS patients (Berry-Kravis et al., 2012). A phase 3 clinical study with R-Baclofen that was based on caregivers’ questionnaires, however, reported a lack of efficacy in FXS patients (Berry-Kravis et al., 2017). No further clinical studies reported the effect of R-Baclofen in FXS patients since then.
Moreover, FXS-related behaviors in mice are improved by serotonergic compounds. One such compound is LP-211 that reverses mGluR mediated long-term depression (LTD) in the hippocampus of wild type and Fmr1-KO mice and improves learning deficits as well as repetitive behavior in these mice (Costa et al., 2012, 2015). Recent work from Costa et al. (2021) further confirmed the LP-211 effect on mGluR-LTD and revealed that this compound requires cyclin-dependent kinase 5 to rescue abnormal plasticity in Fmr1-KO mice.
In the present study, we characterize BTBR and Fmr1-KO mice for their ASD-related behaviors by assessing locomotor activity, anxiety-like, and repetitive behaviors. In addition, the communication of Fmr1-KO mice is tested using the ultrasonic vocalization test. Furthermore, we evaluate the efficacy of the highly discussed GABAergic agonist R-Baclofen and the serotonergic compound LP-211 in both mouse models to explore whether treatment with these compounds can rescue ASD-associated behavioral phenotypes.
2. Materials and methods
2.1. Animals
Breeding pairs of homozygous female and hemizygous male B6.129P2-Fmr1TM1Cgr/J mice (Fmr1-KO; #:003025) (The Dutch-Belgian Fragile X Consortium, 1994), and BTBR T+ Itpr3tf/J mice (BTBR; #:002282) (Petkov et al., 2004; Timmermans et al., 2017) were purchased from Jackson Laboratories, Bar Harbor, ME, USA while C57BL/6JRj (B6) breeding pairs were purchased from Janvier Labs, Le Genest-Saint-Isle, France (# SC-C57J-F). All mice were further bred at the AAALAC-accredited animal facility of QPS Austria GmbH, Grambach, Austria. All animals were housed in single-sex groups of 3−5 mice per cage and kept in ventilated cages under a 12:12 h dark-light cycle and a temperature of 21 ± 1°C at 40 to 70% humidity. Dried pelleted standard rodent chow (Altromin®, Lage, Germany) and water were provided ad libitum. All behavioral tests were performed during the light cycle phase (07:00 a.m.−4:00 p.m.). Each test was continuously performed at the same time of the day. Animals were allocated to experimental groups according to their strain and initial body weight. The use of animals was subject to the statutory provision under the Austrian Animal Welfare Act and was approved by the Styrian government, Austria (ABT13-67744/2020).
2.2. Experimental design
A total of 45 male BTBR and Fmr1-KO mice with a starting age of 4 weeks were allocated to receive either LP-211 (LP; 3 mg/kg, provided by M. Leopoldo, Bari, Italy. Purity > 98%), R-Baclofen (Bf; 1.5 mg/kg, Sigma Aldrich, St. Louis, MO, USA, G013) or vehicle (PEG400 20% v/v + Tween80 10% v/v + 0.9% saline). In addition, control groups of 15 male B6 animals received vehicle only. All animals were treated with an intraperitoneal injection 30 min before each behavioral test performed after age of 5 weeks as these strains are expected to exhibit relevant ASD behaviors mostly from this age (Leopoldo et al., 2011; Silverman et al., 2015).
Based on previous findings of these mouse models (Kazdoba et al., 2014; Silverman et al., 2015; Meyza and Blanchard, 2017; Costa et al., 2018; Zeidler et al., 2018), the behavioral test battery as well as compound dosing selection were designed as indicated in Figure 1. BTBR and Fmr1-KO mice are biologically and developmentally different, thus a different experimental design was applied for these animals. Each of these strains show ASD phenotypes such as modulated anxiety, at particular age stages, the testing timeline was therefore adjusted for each strain accordingly. Therefore, each analyzed parameter was scheduled based on phenotypic characteristics of BTBR and Fmr1-KO mice. All animals were weaned on postnatal day 21. Before each behavioral test, animals were habituated to the test room for 45 min.
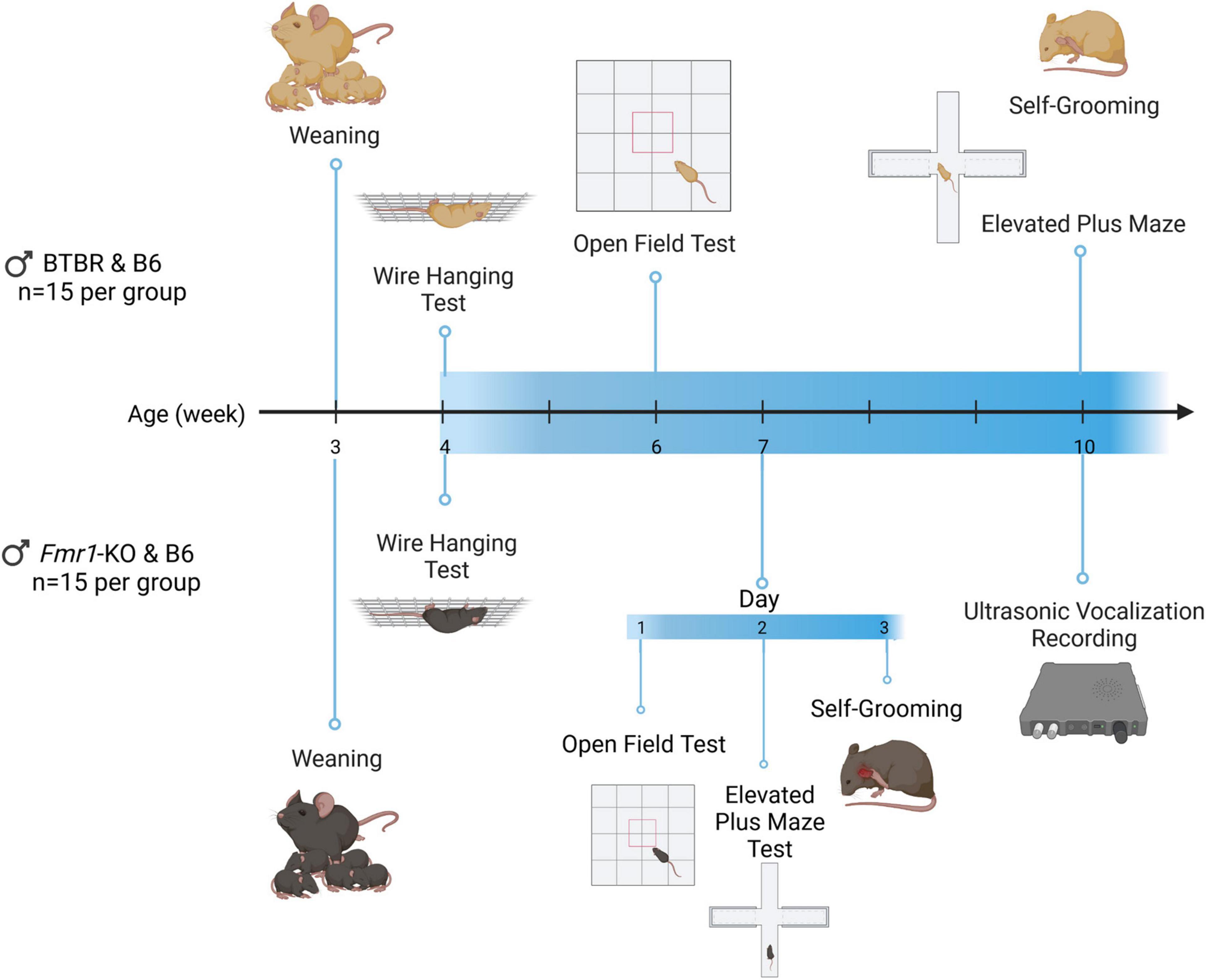
Figure 1. Timeline of BTBR and Fmr1-KO mice experiments. Animals were weaned at the end of week 3. Wire hanging, open field, grooming, and elevated plus maze test were performed at the age of 4, 6, and 10 weeks for BTBR mice. For Fmr1-KO mice, the wire hanging test was performed at the age of 4 weeks. The open field, grooming and elevated plus maze were performed on the 1st to 3rd day of week 7, respectively. Ultrasonic vocalization recording was performed at week 10. Created with BioRender.com.
Animal’s body weight was recorded every 7 days throughout the whole study. Veterinarian care was provided to animals that lost body weight by providing daily wet food pellets on the cage floor starting from day of weaning (postnatal day 21) until 6 weeks of age.
2.2.1. Wire hanging test
The wire hanging test was performed to detect neuromuscular abnormalities of muscle strength within the cut-off time of 300 sec (Hashemi et al., 2007; van Putten, 2014). For the test, a wire cage lid was taped with duct tape around the perimeter to prevent the mouse from walking off the edge. The animal was then placed on the cage grid. The grid was afterward slightly shaken so the animal tightly grabs the wires. Then, the grid was turned upside down. The wire cage lid was held at a height of approximately 50−60 cm above a soft underlay. This distance was high enough to prevent the mouse from jumping down, but not high enough to cause harm in the event of a fall. The latency to fall off the grid was measured in seconds.
2.2.2. Open field test
Mice were subjected to the open field test to explore locomotion and anxiety (Seibenhener and Wooten, 2015). In brief, each mouse was placed in the center of the Plexiglas open field box (48 × 48 cm) surrounded by two rows of infrared photograph beams to detect motion and rearing (standing on the hind paws) and given 30 min to freely explore the box at day light room lighting conditions. The vertical and horizontal movements of animals were recorded within 5 min intervals by a computer-operating tracking system (TSE-System®, Berlin, Germany). Multiple parameters such as activity, hyperactivity, moved distance, thigmotaxis, rearing number, and duration, and time spent in the center of the box were calculated automatically by the ActiMot Software (TSE-System®, Berlin, Germany).
Activity: Time in seconds animals were active with a speed of > 5 m/s. Hyperactivity: Time in seconds animals were active with a speed of > 15 m/s. Moved distance: total distance that was moved by the animal during the test time measured in centimeters. Thigmotaxis: relative time in seconds that the animal spent in the periphery versus the center of the arena indicating an anxiety-like behavior of the animal when spending more time in the periphery. Rearing number and duration: total number and time in seconds that animals spent standing on the hind legs to observe the environment.
2.2.3. Elevated plus maze test
In addition to the open field test, general anxiety-like behavior of animals was evaluated with the elevated plus maze test (Lister, 1987). In brief, each mouse was allowed to freely explore the elevated plus maze apparatus consisting of two open arms (25 × 5 cm), two enclosed arms (25 × 5 × 16 cm), and a central zone (5 × 5 cm) for 5 min while being video recorded. The cumulative time spent and the frequency of entry in either arm or the central zone of the maze were evaluated by the Noldus EthoVision XT 8.5 (Wageningen, Netherlands) software for the whole 5 min test duration. The test was performed under red light illumination.
2.2.4. Self-grooming test
Animals were scored for spontaneous self-grooming behavior (Silverman et al., 2010b; Kalueff et al., 2016). In brief, each mouse was placed in a clean cage, free of bedding, for 15 min and video recorded at day light room lighting conditions. The first 5 min were considered habituation and the following 10 min were evaluated as test session. The cumulative duration of self-grooming and number of grooming episodes were scored with Noldus Observer XT 15 (Wageningen, Netherlands) software.
2.2.5. Ultrasonic vocalizations
2.2.5.1. Familiarization of the male mouse with the smell of female
One week before ultrasonic vocalization (USV) recording, male mice were familiarized with female odor by placing 1 male with an estrous female mouse in a clean cage covered with fresh bedding for 5 min. Mice were observed carefully to prevent any sexual activity.
2.2.5.2. Estrous cycle induction in female mice
Only urine of females in the estrous cycle were used to excite male mice. To induce estrus cycle, adult female C57BL/6JRj mice were subcutaneously injected with β-estradiol (1 μg/50 μl, E-8515, Sigma Aldrich, St. Louis, MO, USA) and progesterone (16 mg/ml, P-0130, Sigma Aldrich, St. Louis, MO, USA) in corn oil (C-8267, Sigma Aldrich, St. Louis, MO, USA) each 48 and 6 h before familiarization or performance of the actual USV recording (Edwards, 1970; Nah et al., 2011).
2.2.5.3. Urine collection from estrous females
To collect urine, each female mouse was grasped, and the urine was collected on small pieces of filter paper. This filter paper was placed in the clean cage containing the male study mouse right before pressing the start button of the recording program.
2.2.5.4. USV recording day
On the test day, animals were habituated in a separate soundproof room. Before recording in the same room, each mouse was placed in a cage with fresh bedding. Recordings were performed with an UltraSoundGate Condenser Microphone (CM16; Avisoft Bioacoustics, Nordbahn, Germany), connected via an Avisoft UltraSoundGate 416 USB Audio device (Avisoft Bioacoustics, Nordbahn, Germany) to a personal computer. Acoustic data were recorded with a sampling rate of 250,000 Hz in 16-bit format. After placing a filter paper with urine of an estrous female in the mouse cage, the recording was started under red light condition. Vocal emission was recorded for 5 min.
2.2.5.5. USV data analysis
For analysis, recordings were transferred to SASLab Pro (Avisoft Bioacoustics, Nordbahn, Germany), and a fast Fourier transformation was conducted (512 FFT length, 100% frame, Hamming window and 75%-time window overlap). For automatic parameter measurement setup, the maximum changes were set to 10 pixels with 5 ms minimum duration and 10 ms hold time. The total number of calls and the latency to initiate the first call were measured and evaluated for the whole 5 min of the recordings.
2.3. Statistical analysis
Data analysis was performed by GraphPad Prism™ 9.2 (GraphPad Software Inc., San Diego, CA, USA). All graphs present group means with standard error of the mean (SEM). The significance level was set at p < 0.05. Group means were compared using One-way or Two-way analysis of variance (ANOVA), with a subsequent post hoc; Dunn’s Multiple Comparison or Fischer’s LSD test. For comparison of two groups, Student’s t-test was performed. Significances were defined as *p < 0.05; **p < 0.01; ***p < 0.001.
Pearson’s Correlation Coefficient (r) was used to compare animals’ body weight and latency to fall off the wire in the wire suspension test. Moreover, the results of the Pearson’s Correlation test of BTBR and Fmr1-KO mice were compared using Fischer’s Z transformation (Eid et al., 2011). To this aim, the online tool https://www.psychometrica.de/correlation.html#fisher was used. Z- and p-values are provided.
The exact group size and utilized statistical tests are mentioned in the figured legends.
All raw data of this study are provided in Supplementary Table 1.
3. Results
3.1. Wire hanging test reveals motor deficits of BTBR mice starting at the early stage of life
The wire hanging test was performed as part of the general health check before treatment start of the efficacy study. Based on body weight results, mice were allocated to treatment groups, so that all groups would present similar body weight averages. The wire hanging test evaluating neuromuscular changes was performed at 4 weeks of age. The body weight of all animals was measured immediately thereafter and correlated with the wire hanging results. Results show that BTBR mice fell significantly earlier off the wire compared to B6 mice (Figure 2A; p ≤ 0.001). Body weights of BTBR mice was significantly higher compared to B6 mice (Figure 2B; p = 0.004). In addition, results of the wire hanging test were correlated with body weight data (Figures 2C, D). There was a significant negative correlation of body weight and the latency to fall in BTBR mice (Figure 2C; r = −0.6082; p ≤ 0.0001) but no correlation of these parameters in B6 mice (Figure 2D; r = 0.3872; p = 0.2137). Moreover, there was a significant difference between correlations of time hanging and body weight in BTBR mice and the B6 control group (z = −3.028; p = 0.001).
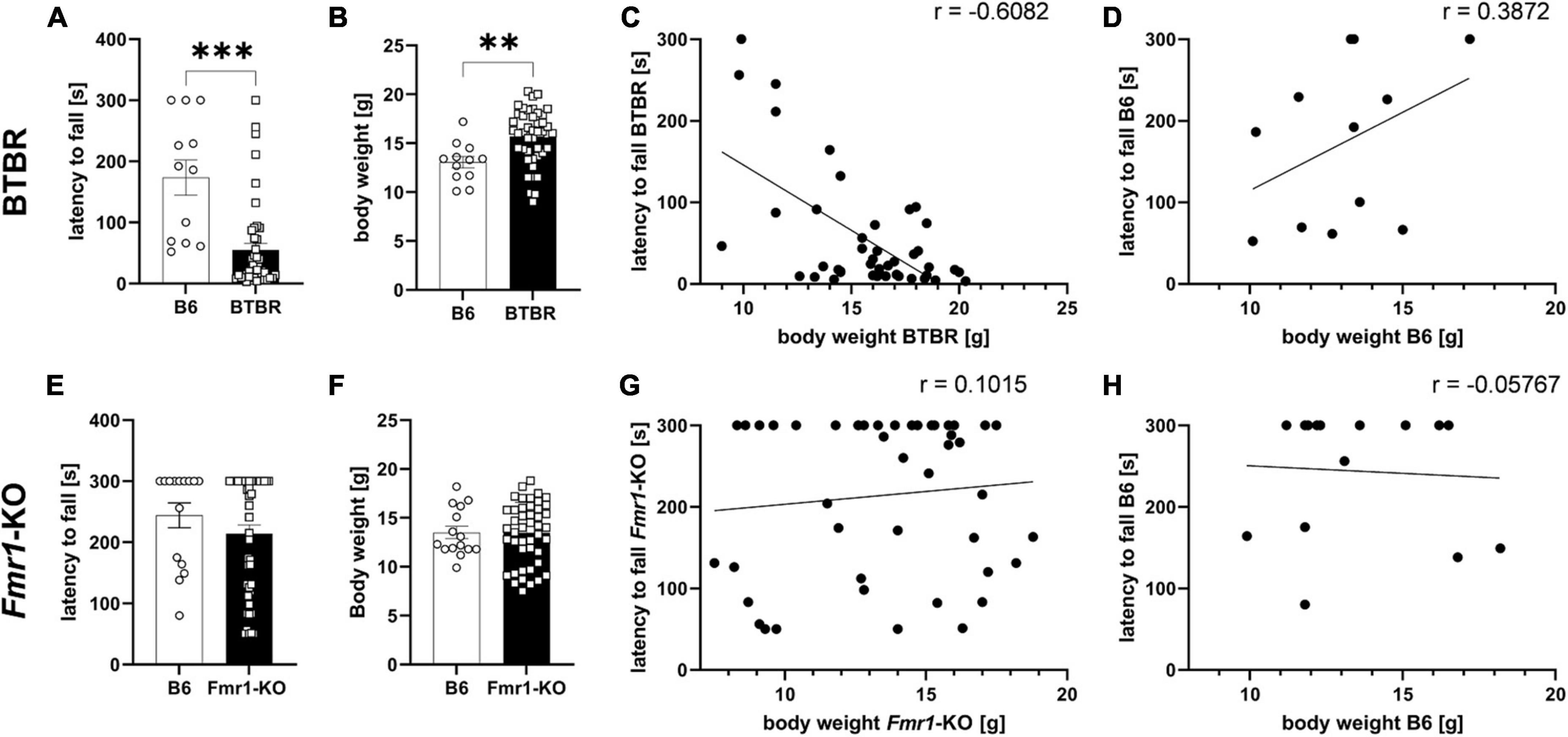
Figure 2. Wire hanging test. Latency to fall off the wire (A,E), body weight measurements after the wire hanging test (B,F), correlation curves of body weight and latency to fall off the wire (C,D,G,H) of BTBR (A–D) and Fmr1-KO (E–H) compared to B6 mice at 4 weeks of age. Cut-off time of 300 s. Unpaired t-test; B6: n = 15, BTBR/Fmr1-KO: n = 45, mean ± SEM. **p < 0.01, *** p < 0.001.
The Fmr1-KO mice, however, did not reveal any motor dysfunction by showing a comparable latency to fall and body weight levels compared to B6 control mice (Figures 2E, F). There was no significant correlation between body weight and the latency to fall in Fmr1-KO (Figure 2G; r = 0.1015; p = 0.5072) and B6 (Figure 2H; r = −0.05767; p = 0.8382). There was no significant difference between correlations of latency to fall off the wire and body weight in Fmr1-KO mice and the B6 control group (z = 0.488; p = 0.313).
3.2. BTBR mice’s body weight is decreased by R-Baclofen treatment
Weekly body weight measurement of Fmr1-KO and BTBR mice showed that BTBR mice are heavier than B6 mice (Figure 3A; ANOVA: genotype, p < 0.001; age, p < 0.001; interaction, p < 0.001; post hoc test, p = 0.001). After one treatment with R-Baclofen at the age of 6 weeks and 2 treatments at the age of 10 weeks, BTBR mice lost about 10% of body weight at the age of 9 and 10 weeks (Figure 3A; p ≤ 0.03). Veterinarian care was provided to animals that lost body weight by providing daily wet food pellets on the cage floor until animals gained back the lost body weight. LP-211 treatment did not affect BTBR animals’ body weight.
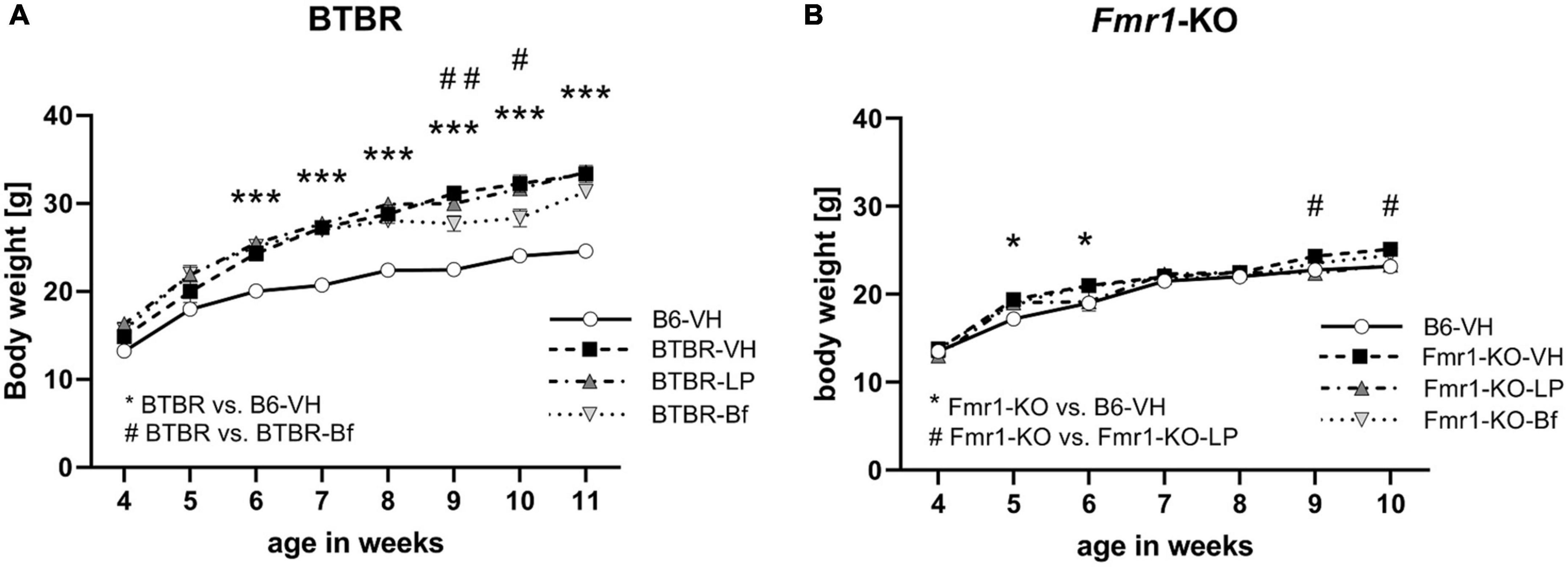
Figure 3. Weekly body weight measurements in BTBR (A) and Fmr1-KO (B) animals after treatment with LP-211, R-Baclofen, or vehicle. Two-way ANOVA followed by uncorrected Fisher’s LSD post hoc test. n = 15 per group, Mean ± SEM. All versus BTBR/Fmr1-KO vehicle-treated (VH) mice. */#p < 0.05, ##p < 0.01, ***p < 0.001.
Fmr1-KO mice showed a similar progression of body weight gain compared to B6 mice. Wet food pellets were provided to animals that lost body weight. Fmr1-KO mice showed higher body weights in week 5 and 6 than B6 mice but differences faded with age (Figure 3B; ANOVA: genotype, p = 0.42; age p < 0.001; interaction, p = 0.002; post hoc test, p = 0.04). In week 9 and 10, Fmr1-KO mice treated with LP-211 were heavier, as the vehicle-treated Fmr1-KO mice seem to stop gaining weight (Figure 3B; p = 0.04). R-Baclofen treatment did not affect the body weight of Fmr1-KO mice.
From this point onward, animals were treated with R-Baclofen, LP-211 or vehicle 30 min prior to each test.
3.3. Hyperactivity in Fmr1-KO and anxiety in BTBR mice are measurable in the open field test
Locomotor activity was measured during a 30 min trial in BTBR and B6 mice at the age of 6 weeks. Hyperactivity did not show a difference between BTBR and B6 mice (Figures 4A, B). Consistent with activity and hyperactivity levels, there were no differences observed in the moved distance between BTBR and B6 mice. Acute LP-211 and R-Baclofen treatment did not affect these parameters in BTBR mice (Figures 4A-C).
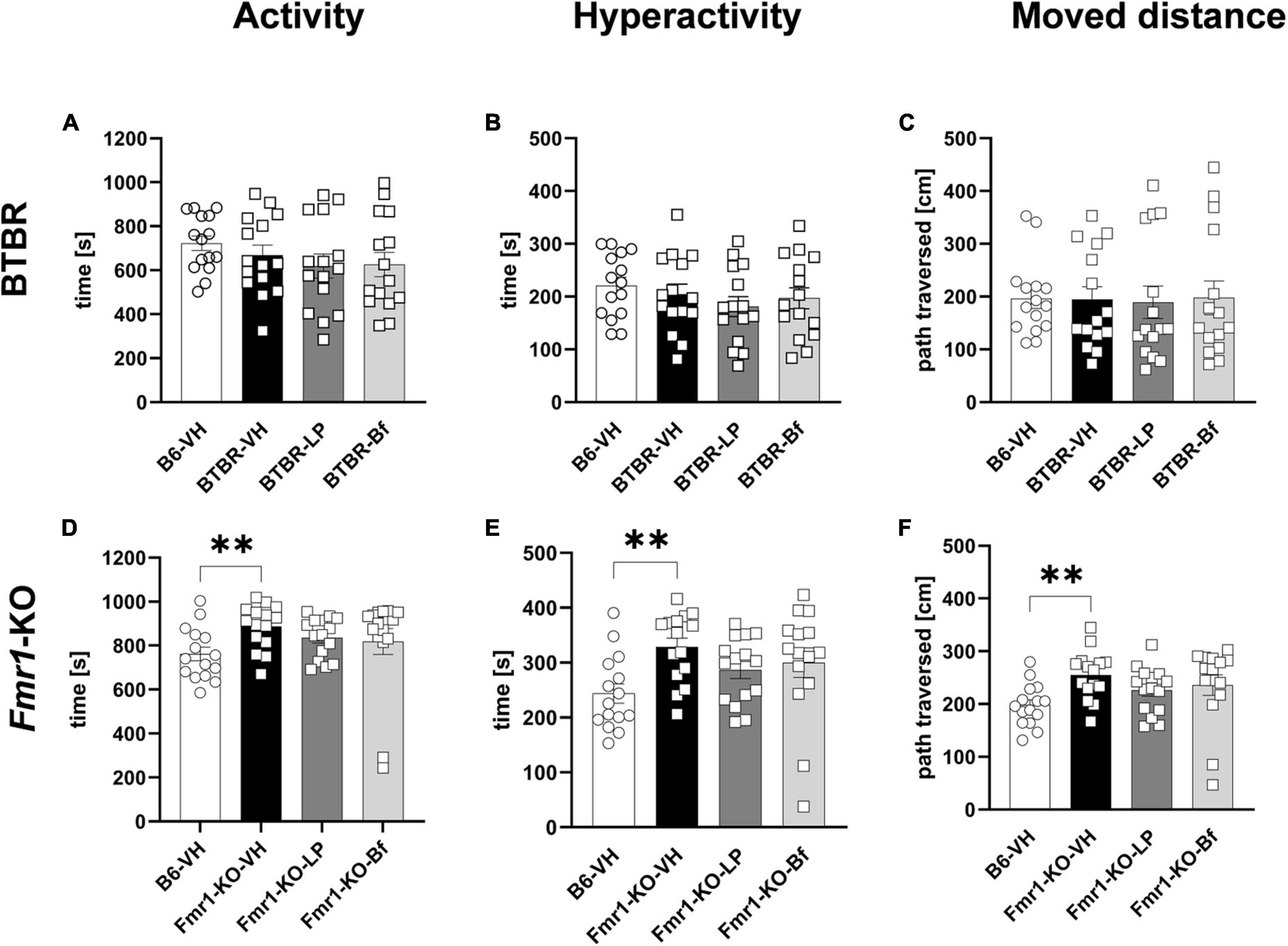
Figure 4. Locomotor activity of BTBR and Fmr1-KO mice in the open field test. Activity (A,D), hyperactivity (B,E), and moved distance during a 30 min trial (C,F) of BTBR (A–C) and Fmr1-KO (D–F) mice. One-way ANOVA followed by uncorrected Fisher’s LSD (D,F) or uncorrected Dunn’s (E) post hoc test. n = 15 per group. Mean ± SEM. All groups versus BTBR/Fmr1-KO vehicle-treated (VH) mice. **p < 0.01.
Fmr1-KO mice at 7 weeks of age, however, displayed a significantly higher activity and hyperactivity (Figures 4D, E; p = 0.004; p = 0.003, respectively) compared to B6 mice during the 30 min open field test. Fmr1-KO mice moved a longer distance (Figure 4F; p = 0.002) than B6 mice in the open field box.
Evaluation of parameters related to anxiety showed a significantly higher thigmotaxis (Figure 5A; p = 0.001) and shorter time spent in the center of the box (Figure 5B; p = 0.001) in BTBR mice compared to B6 mice. Anxiety in BTBR mice was further confirmed by the observed lower rearing number and rearing duration compared to B6 mice (Figures 5C, D; p ≤ 0.001). Acute LP-211 and R-Baclofen did not alleviate anxiety-like behavior observed in BTBR mice compared to vehicle-treated BTBR mice (Figures 5A-D).
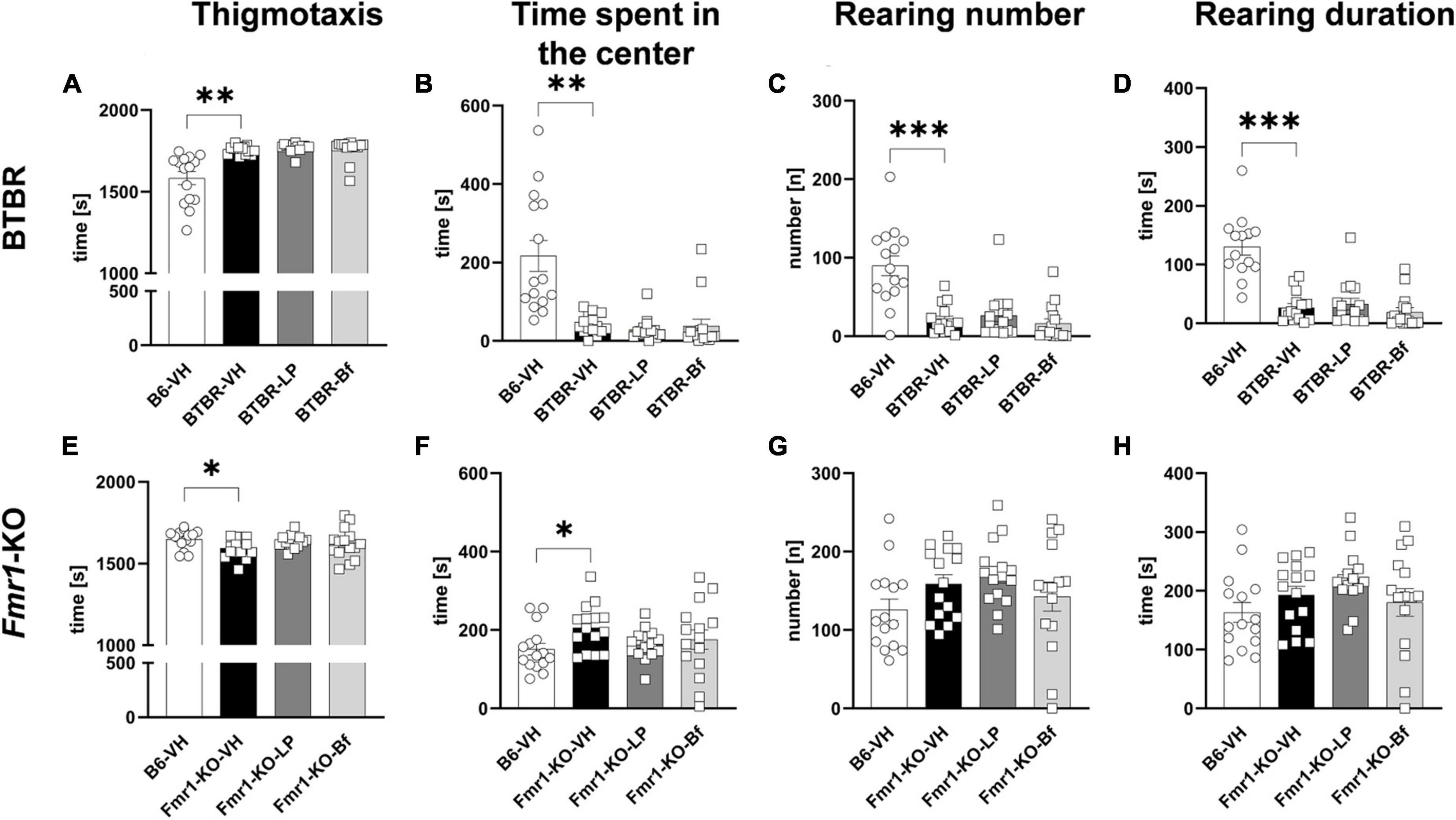
Figure 5. Anxiety measurement of BTBR and Fmr1-KO mice in the open field test. Thigmotaxis (A,E), time spent in the center of the open field box (B,F), rearing number (C,G), and rearing duration (D,H) of BTBR (A–D), and Fmr1-KO (E–H) mice. One-way ANOVA followed by uncorrected Fisher’s LSD (E–H) or uncorrected Dunn’s (A–D) post hoc test. n = 15 per group. In graph (D), one value had to be excluded from B6-VH group due to a software error. Mean ± SEM. All groups vs. BTBR/Fmr1-KO vehicle-treated (VH) mice. *p < 0.05, **p < 0.01, *** p < 0.001.
Fmr1-KO mice presented significantly less thigmotaxis compared to B6 mice (Figure 5E; p = 0.03). Moreover, low anxiety in Fmr1-KO mice was validated by the longer time animals spent in the center of the open field box compared to B6 mice (Figure 5F; p = 0.03). The rearing activity was comparable between Fmr1-KO and B6 mice. Acute treatment of LP-211 and R-Baclofen did not affect these parameters in Fmr1-KO mice, indicating no sedating effect of these drugs at the applied doses (Figures 5E-H).
3.4. Fmr1-KO mice display reduced anxious behavior in the elevated plus maze test
Based on the observed results from the open field test, we aimed to further explore anxiety-like behavior by the widely used elevated plus maze (EPM) test in BTBR and Fmr1-KO mice at the age of 10 and 7 weeks, respectively. BTBR mice spent the same amount of time in open and closed arms and the center of the maze compared to B6 mice (Figures 6A–C). Fmr1-KO mice spent significantly more time in the open arms of the EPM compared to B6 mice (Figure 6D; p = 0.03). The cumulative duration in the closed arms, as well as central zone of the maze was similar between Fmr1-KO and B6 mice (Figures 6E, F; p = 0.06 and p = 0.68, respectively).
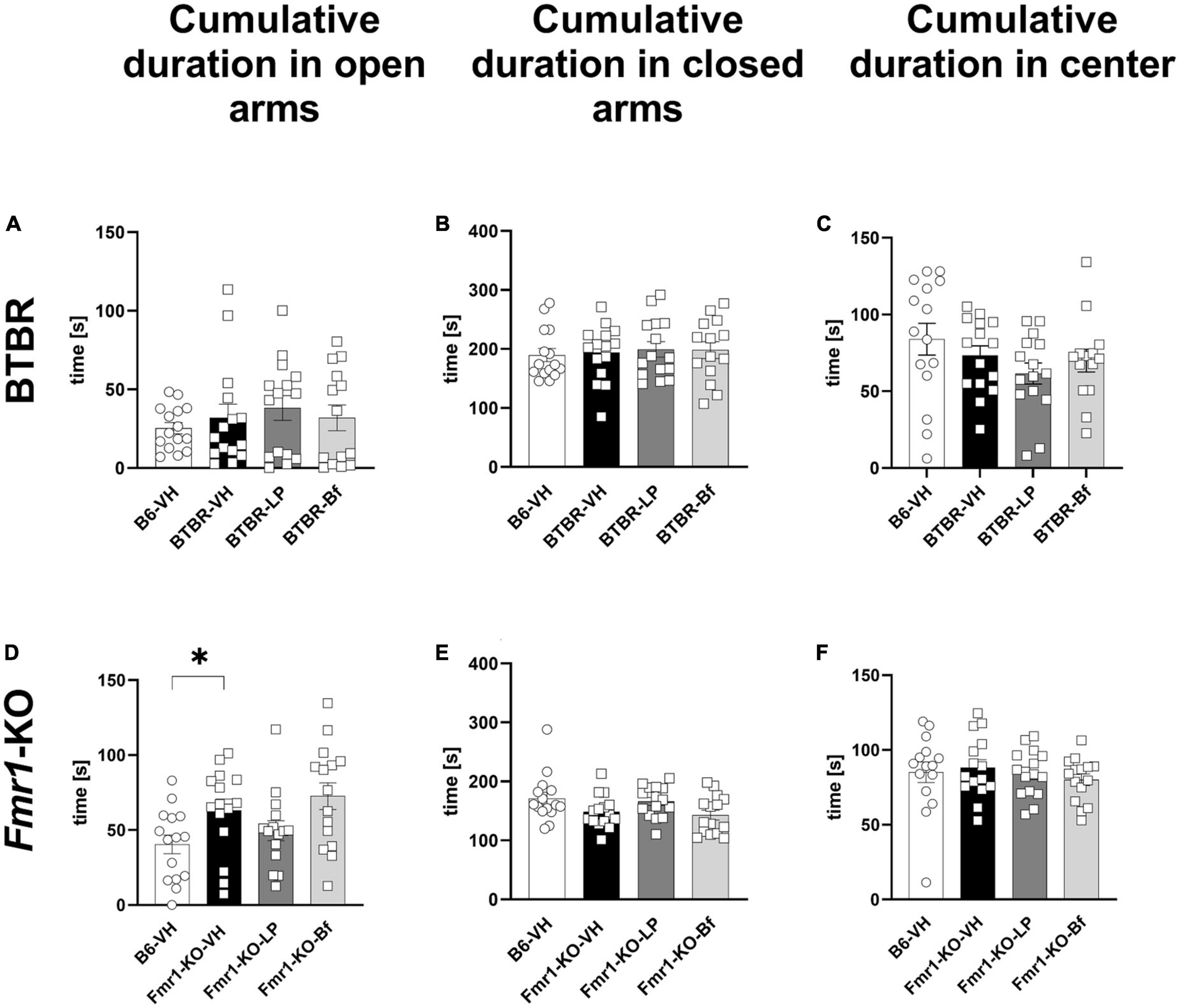
Figure 6. Cumulative duration in closed arms, open arms, and central zone of the elevated plus maze test of BTBR and Fmr1-KO mice. Total time spent in open arms (A,D), closed arms (B,E) and central zone (C,F) in seconds during a 5-min session. One-way ANOVA followed by uncorrected Fisher’s LSD (A,B,D–F) or uncorrected Dunn’s (C) post hoc test. n = 15 per group. Mean ± SEM. All groups vs. BTBR/Fmr1-KO vehicle-treated (VH) mice. *p < 0.05.
Examining the number of entries, BTBR mice entered the open arms as many times as B6 mice (Figure 7A). However, BTBR mice entered the closed arms more frequently than B6 mice (Figure 7B; p = 0.004). Furthermore, the BTBR mice entered the central zone of the maze more frequently than B6 mice (Figure 7C; p = 0.006). Acute LP-211 treatment of BTBR mice had neither significant effect on the number of entries to the closed arms nor the central zone of the EPM compared to the vehicle-treated BTBR (Figures 7B, C). There were no changes observed upon R-Baclofen injection in BTBR mice for the cumulative durations as well as frequencies to enter the open, closed, or central zone of the EPM compared to vehicle-treated BTBR mice. The frequency to enter the open arms was significantly higher in Fmr1-KO than B6 mice (Figure 7D; p = 0.003), while the frequency to enter the closed arms was similar between B6 and Fmr1-KO mice (Figure 7E; p = 0.07). None of the treatments was able to modulate this parameter in Fmr1-KO mice. Fmr1-KO mice entered the central zone of the EPM significantly more often compared to B6 mice (Figure 7F; p = 0.03). A decreased frequency to enter the open arms was observed in LP-211 treated Fmr1-KO mice compared to vehicle-treated Fmr1-KO mice (Figure 7D; p = 0.03). R-Baclofen treatment did not change the frequency of entries in open and closed arms and central zone of the EPM in Fmr1-KO mice compared to vehicle-treated Fmr1-KO mice.
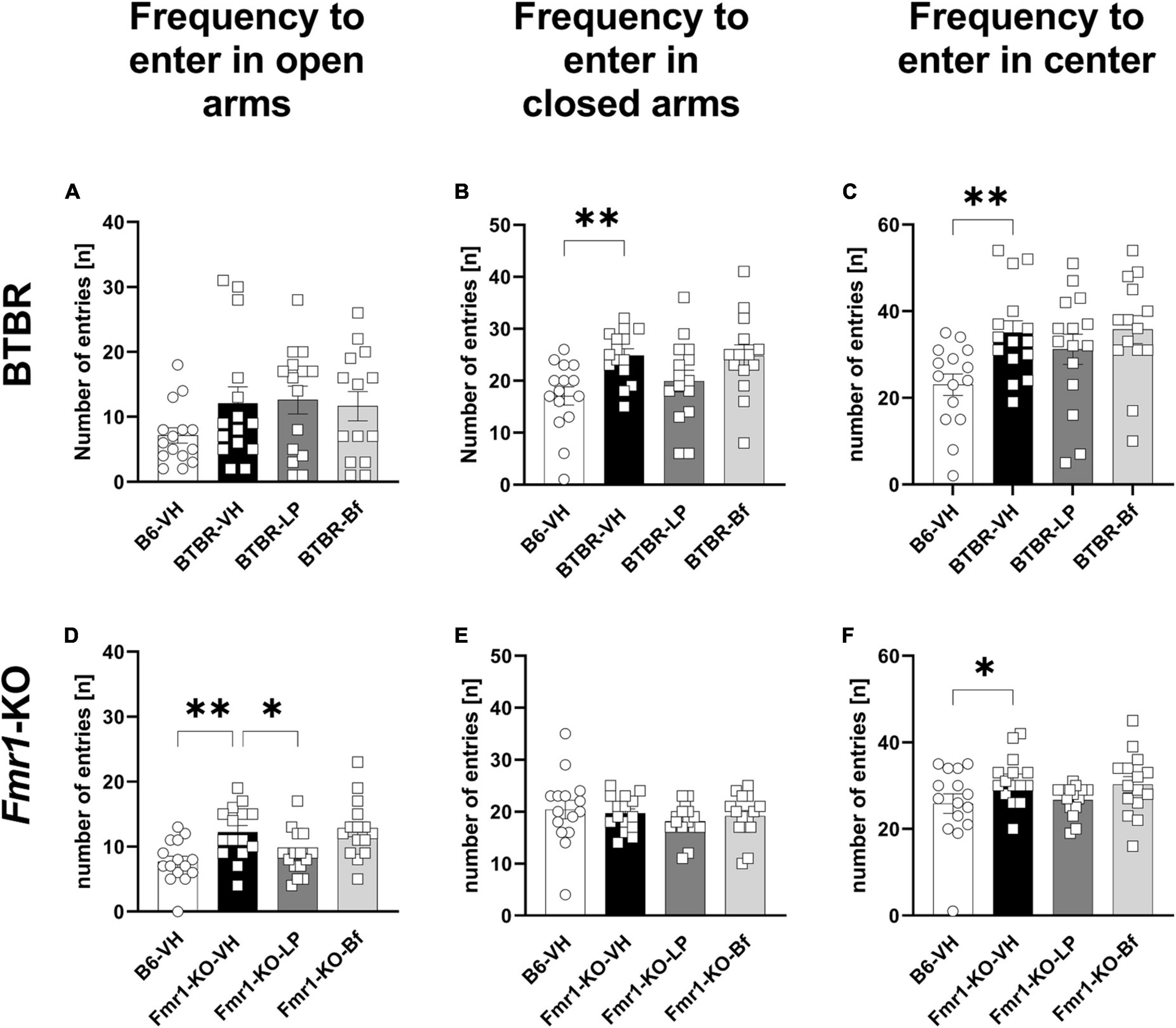
Figure 7. Frequency of entries to closed arms, open arms, and central zone of the elevated plus maze of BTBR and Fmr1-KO mice. Total number of entries to open arms (A,D), closed arms (B,E) and central zone (C,F) during a 5 min session under red light. One-way ANOVA followed by uncorrected Fisher’s LSD (B,C,F) or uncorrected Dunn’s (A,D,E) post hoc test. n = 15 per group. Mean ± SEM. All groups versus BTBR/Fmr1-KO vehicle-treated (VH) mice. *p < 0.05, **p < 0.01.
3.5. Acute R-Baclofen and LP-211 treatment reduced grooming behavior in Fmr1-KO mice
Repetitive behavior was monitored by evaluating self-grooming in a 10 min session in BTBR and Fmr1-KO mice at the age of 10 and 7 weeks, respectively. BTBR mice spent significantly more time self-grooming compared to B6 mice (Figure 8A; p ≤ 0.001); however, there was no significant difference observed in the number of grooming episodes (Figure 8B). None of the compounds was able to reverse these parameters in BTBR mice.
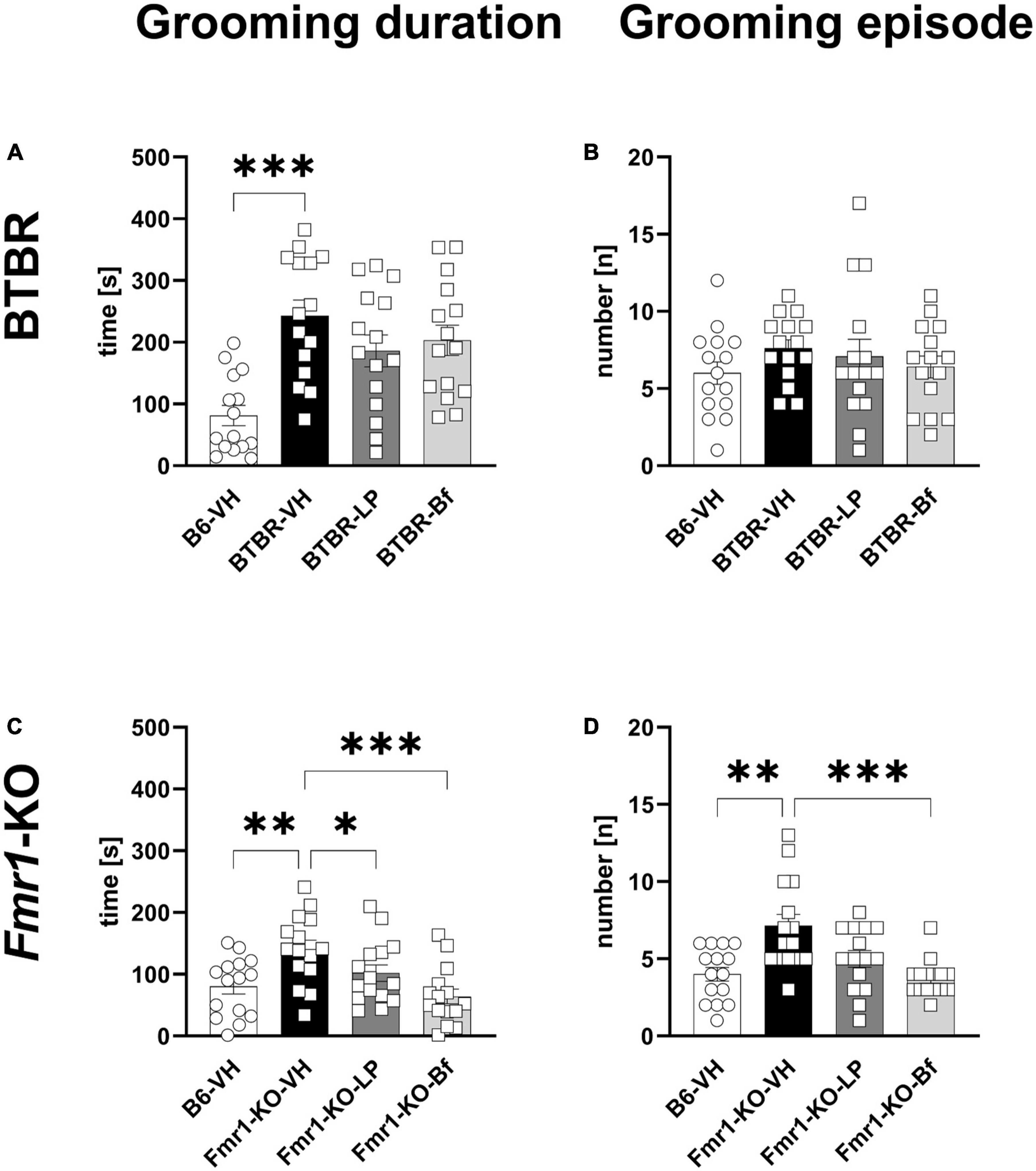
Figure 8. Self-grooming behavior of BTBR and Fmr1-KO mice. Total self-grooming duration (A,C) and total grooming episodes (B,D) during the 10 min recording session in BTBR (A,B) and Fmr1-KO (C,D) mice. One-way ANOVA followed by uncorrected Fisher’s LSD (C) or uncorrected Dunn’s (A–D) post hoc test. n = 15 per group. Mean ± SEM. All groups versus BTBR/Fmr1-KO vehicle-treated (VH) mice. *p < 0.05, **p < 0.01, *** p < 0.001.
Fmr1-KO mice spent a significantly longer time grooming with a higher number of grooming episodes during the 10 min of recording (Figures 8C, D; p = 0.002, p = 0.002, respectively). Acute R-Baclofen treatment significantly ameliorated the grooming duration as well as number of grooming episodes in Fmr1-KO mice (Figures 8C, D; p ≤ 0.001; p ≤ 0.001, respectively). Acute LP-211 treatment significantly reduced the grooming duration in Fmr1-KO mice (Figure 8C; p = 0.04).
3.6. Impaired ultrasonic vocalization emission in Fmr1-KO mice did not improve by R-Baclofen or LP-211 treatment
Since R-Baclofen and LP-211 treatment only alleviated repetitive behavior in Fmr1-KO mice, we further studied the effect of these compounds on the social interest and communication test by measuring ultrasonic vocalization in these mice. This test was chosen to measure social communication as results of this test are less variable and more reliable compared to other tests such as the automated three-chamber social interaction test as less interventions to the test animal are needed to perform the test.
Male Fmr1-KO mice were exposed to fresh urine of estrous females and USV calls were recorded for 5 min at the age of 10 weeks. A significantly lower number of calls was observed in Fmr1-KO mice regardless of treatment compared to B6 mice (Figure 9A; p = 0.01). The latency to initiate the first call did not differ between Fmr1-KO and B6 mice and did not depend on the treatment (Figure 9B). R-Baclofen and LP-211 treatment did not affect these parameters in Fmr1-KO mice compared to vehicle-treated Fmr1-KO mice.
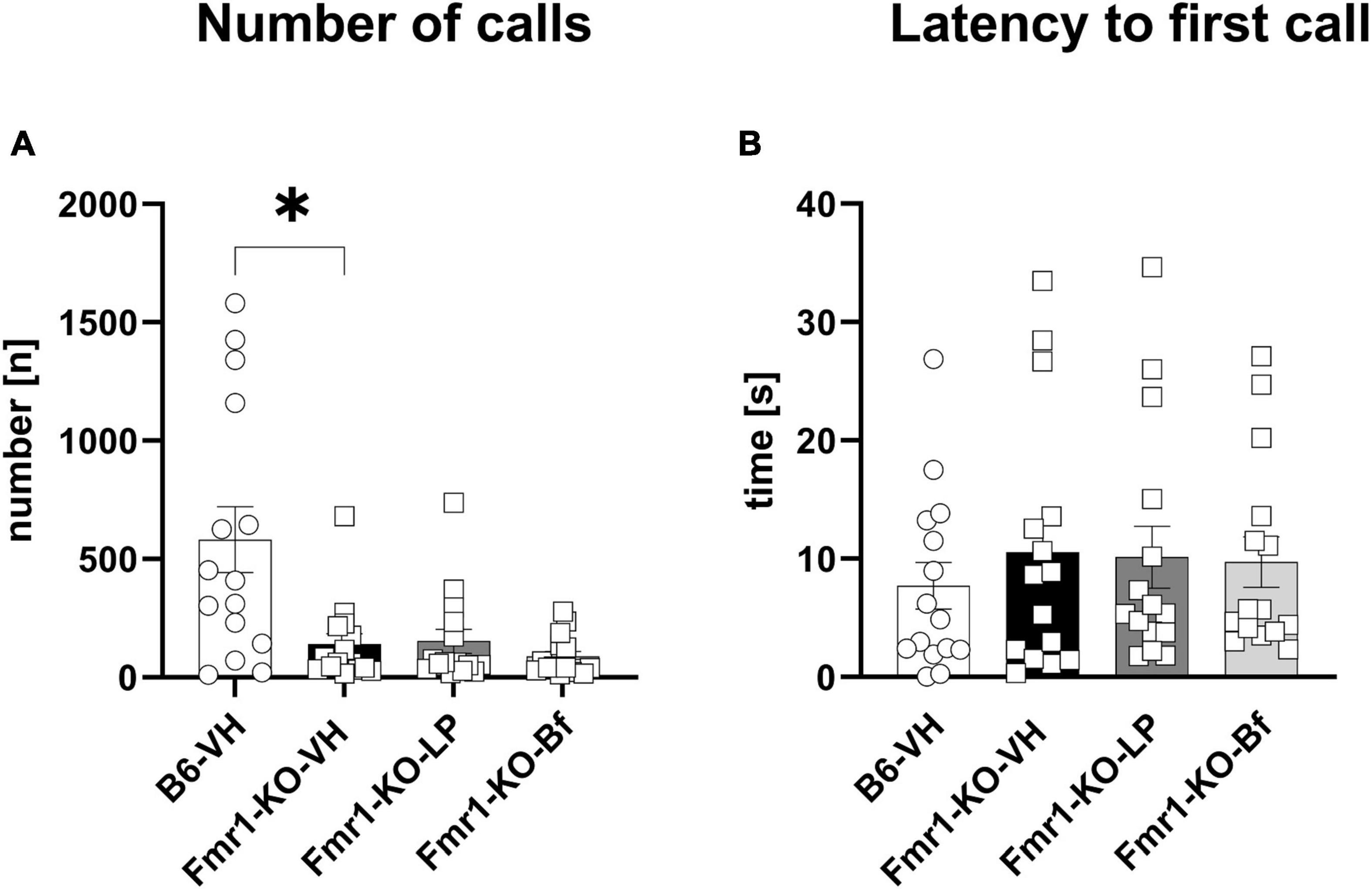
Figure 9. Ultrasonic vocalization emission recordings while exposed to fresh urine of an estrous female. The number of emitted calls (A) and latency to initiate the first call (B) were measured. One-way ANOVA followed by uncorrected Dunn’s post hoc test. n = 15 per group. Mean ± SEM. All groups versus Fmr1-KO vehicle-treated (VH) mice. *p < 0.05.
Overall, the results of the behavioral characterization and the efficacy study showed that male BTBR mice at the age of 4−10 weeks are a suitable model to study ASD symptoms, such as motor deficits, repetitive behavior, anxiety, and stress-like behavior. Acute R-Baclofen and LP-211 treatment could not ameliorate these alterations in BTBR mice. Fmr1-KO mice presented ASD-like behavior in terms of hyperactivity, repetitive behavior as well as communication impairments at the age of 4−10 weeks. Acute R-Baclofen and LP-211 treatment ameliorated repetitive behavior in Fmr1-KO mice.
4. Discussion
In the present study, we behaviorally characterized two different ASD mouse models, BTBR and Fmr1-KO, and evaluated the efficacy of the GABAB receptor agonist R-Baclofen and the serotonin 5-HT7 receptor agonist LP-211 in these models. Animals were tested for locomotor activity, anxiety, stereotypic, and repetitive behaviors. In addition, the sociability of Fmr1-KO mice was evaluated within the treatment efficacy part of the study.
4.1. Behavioral characterization of BTBR mice
Motor dysfunction in BTBR mice has been previously shown by Xiao et al. (2020) evaluating it from 2 weeks to 5 months of age. Researchers showed that abnormal cerebellar development could be an important factor related to motor dysfunction as well as dystonia-like behavior in these animals. Here we report motor abnormalities in BTBR mice at the age of 4 weeks as analyzed in the wire hanging test, that negatively correlated with body weights, suggesting that the body weight has a strong impact on motor abilities: only 4 out of 45 BTBR mice could hold the wire for more than 200 s. We thus conclude that motor disability in BTBR mice starts at an early age.
Approximately half of the children with ASD meet the diagnostic criteria of hyperactivity as well as attention-deficit disorder (Murray, 2010), thus evaluating locomotor activity in ASD animal models is an important parameter to evaluate their translatability. Here, when measuring the locomotor activity in the non-social context of the open field test, 6 weeks old BTBR mice presented no differences in activity and hyperactivity. A previous report of the same test performed with 22 days old BTBR mice showed that they traveled more than B6 controls in the first 15 min of the test session that was performed under red light (McFarlane et al., 2008). Furthermore, another study showed that 8 weeks old BTBR mice present hyperactivity compared to wild type control mice by an increased distance moved in all zones in the open field test (Xiao et al., 2020).
However, another study measured the distance moved in the open field test in 15 months old male BTBR mice for 30 min under room lightening, in which BTBR mice moved a comparable distance than B6 controls (Jasien et al., 2014). Differences in these results can be explained by different test settings like the age of animals, duration of recording, and room light condition. Overall, BTBR mice appear to present hyperactivity behavior at a young age until early adolescence but lose this phenotype during adulthood.
We further evaluated the anxiety level of BTBR mice by measuring anxiety parameters in the open field and EPM at the age of 6 and 10 weeks, respectively. BTBR mice presented a high anxiety profile both in open field and EPM test. When considering the total amount of the time spent in the open field box, which is a sum of thigmotaxis and time spent in the center, BTBR mice strongly exhibited anxious behavior by spending most of the time at the outer boundaries of the box and the least time in the center. Previous reports showed an anxiogenic profile of 14−17 weeks old BTBR mice by using the EPM as animals showed a significantly lower number of entries in the open arms. Authors mention that the lightening of the test room was a stressor to which BTBR mice show a strong response, manifesting high stress-like behavior in BTBR mice (Pobbe et al., 2011). Moreover, it is worth to mention that BTBR mice are shown to have impaired photoreceptor function and therefore present atypical visual perception which mimics some subset of ASD patients (Cheng et al., 2020). Another research group reported anxiety in 8−12 months old BTBR mice compared to wild type mice by the elevated open platform test, where BTBR mice spent more time at the edges and less time in the center of the open platform (Chao et al., 2018). Another study assessing anxiety-like behavior in aged (19−21 months old) BTBR mice showed a decreased anxiety-like behavior compared to existing results in younger mice by showing that animals spent less time in the closed arms of the EPM (O’Connor et al., 2021). We thus conclude that BTBR mice exhibit anxiety and stress-like behavior at adolescent age.
Furthermore, we confirmed repetitive behavior in the self-grooming test, as 10 weeks old BTBR mice spent a longer time self-grooming compared to controls. These results are consistent with previous reports testing BTBR mice for self-grooming from postnatal day 18 to 60 (McFarlane et al., 2008) as well as weeks 7 to 8 (Amodeo et al., 2014). Furthermore, O’Connor et al. (2021) showed higher repetitive behavior of self-grooming in 19−21 months old BTBR mice compared to wild type mice. We thus conclude that BTBR mice exhibit repetitive behavior at adolescent age, and, based on previous reports, maintain this behavior lifelong.
4.2. Behavioral characterization of Fmr1-KO mice
Based on a study by Pietropaolo et al. (2011), the B6 background is more suitable to study core autism symptoms of social impairments, repetitive behaviors, as well as hyperactivity compared to the FVB background. Here, Fmr1-KO mice on a B6 background were thus used to evaluate ASD-like behaviors. The results obtained from the behavioral characterization of Fmr1-KO mice are consistent with previous reports (Michalon et al., 2012; Kramvis et al., 2013; Hébert et al., 2014; Kazdoba et al., 2014; Gantois et al., 2017; Gaudissard et al., 2017). In summary, Fmr1-KO mice did not show any motor dysfunction and presented comparable body weights as B6 mice. Although a micro-computerized tomography analysis performed by Leboucher et al. (2019) showed that the deficiency in the Fmr1 gene impacts skeleton and bone morphology in 16 weeks old Fmr1-KO mice, it does not influence the animal’s body weight and motor performance. Moreover, in Fmr1-KO mice the body weight does not correlate with the latency to fall off the wire in the wire suspension test. Thus, a heavier mouse does not necessarily fall off earlier compared to a lighter mouse.
The observed differences in the latency to fall off the wire in control animals of BTBR and Fmr1-KO studies could be caused by the different breeding pairs that were used for each study.
Results of the behavioral characterization of Fmr1-KO mice in the open field and EPM test indicate hyperactivity as well as lower anxiety in this mouse model consistent with previous reports performed in 2−5 months old Fmr1-KO mice using the same tests (Mineur et al., 2002; Qin et al., 2002; Saré et al., 2016). Studies evaluating overlapping anxiety and hyperactivity in humans show that around 50% of adults and up to 30% of children with attention deficit hyperactivity disorder (ADHS) meet both diagnostic criteria (Pliszka, 2000; Michelini et al., 2015; Mulraney et al., 2018; van der Meer et al., 2018). Though it must be pointed out that the complexity of neuropsychiatric disorder symptoms such as hyperactivity such as excessive activity, decreased sleep need, disturbed behavior, etc., challenges the interpretation of data from animal studies (Nestler and Hyman, 2010; Yen et al., 2013). Hence, the lower anxiety-like behavior observed in Fmr1-KO mice detected by a higher frequency to enter and more time spent in the open arms of the EPM test could be affected by the higher activity and hyperactivity observed in these mice. Although hyperactivity might influence the results of anxiety analyses, evaluation of Fmr1-KO mice in the EPM and open field test definitively demonstrates that these animals have a reduced anxiety.
Repetitive behaviors are considered a key characteristic of ASD (Caldwell-Harris, 2021), thus reproducing them in animal models is crucial for the use of these models for further ASD research. Fmr1-KO mice showed repetitive behaviors by spending more time as well as more episodes of self-grooming compared to controls. These data are consistent with previous reports about the grooming behavior of 3 months old Fmr1-KO mice on a B6 background (Mines et al., 2010; Pietropaolo et al., 2011).
Results from the ultrasonic vocalization recording of 10 weeks old Fmr1-KO mice revealed impaired sociability and communication deficits in this mouse model. Some studies showed a similar average quantity of calls from adult Fmr1-KO compared to wild type mice. One example is the study where Fmr1-KO mice were bred on an FVB background and USV was recorded while exposing mice to fresh urine collected from estrus female mice for 5 min (Hodges et al., 2017). Moreover, another study reports Fmr1-KO on B6 background at the age of 2−3 months while directly exposed to receptive female mice for 2 min (Belagodu et al., 2016). Another study reported a reduced rate of ultrasonic calls during mating for 10 min by 2−3 months old Fmr1-KO mice on an FVB background compared to wild type mice and thus on a different background than the one used here (Rotschafer et al., 2012). We can conclude that not only age but also the genetic background as well as the test setting, e. g., female mice vs. urine of female mice (Hoffmann et al., 2009) as well as exposure time can affect the outcome of the ultrasonic vocalization test of adult male Fmr1-KO mice (Premoli et al., 2021).
4.3. R-Baclofen treatment
R-Baclofen, a GABAB receptor agonist, has been already used in various clinical and preclinical studies to ameliorate ASD phenotypes (Cryan et al., 2004; Erickson et al., 2014; Qin et al., 2015; Berry-Kravis et al., 2017; Veenstra-VanderWeele et al., 2017; Stoppel et al., 2018; Möhrle et al., 2021). The applied drug concentration is critical, especially in studies measuring behavioral changes where deleterious side effects such as sedation or hyperactivity need to be avoided. A study showed a paradoxical effect of chronic R-Baclofen not only in the Fmr1-KO mouse model but also in B6 control mice by increasing activity (Zeidler et al., 2018). Another study reported the sedative effects of R-Baclofen in higher doses such as 3−5 mg/kg intraperitoneal treatment in the BTBR and C58 ASD mouse models (Silverman et al., 2015). Therefore, in the current study, the concentration of 1.5 mg/kg was carefully selected to induce disease ameliorating effects while preventing any side effects. Concentrations less than 3 mg/kg have been reported to be effectless on locomotor activity, repetitive behavior, as well as sociability in B6 mice (Silverman et al., 2015), therefore, no B6 mice were treated with R-Baclofen in the current study.
In 2005, Silverman et al. (2015) evaluated the efficacy of R-Baclofen on both sexes of BTBR mice at the age of 10−12 weeks with a single dose of 1 and 3 mg/kg 60 min prior to testing. Both doses improved sociability in BTBR mice but only the 3 mg/kg dose could improve the repetitive behavior of these mice. The same research group showed in an earlier study that 1 mg/kg of R-Baclofen did not reverse the social impairment of male-female interaction in BTBR mice (Silverman et al., 2015). In the present study, BTBR vehicle-treated mice showed higher anxiety and higher repetitive grooming behavior. R-Baclofen treatment did not improve these parameters. Thus, it might be possible that each behavioral assay requires a different optimal dose of R-Baclofen to be effective. Chronic treatment with R-Baclofen might be more useful, not only because ASD is a lifelong disorder and would require constant treatment, but it would also blunt the observed side effects when using higher concentrations. As discussed by Silverman et al. (2015), comprehensive studies conducting both acute and chronic treatments for each behavioral assay would be the most effective way of assessing compound efficacy.
In Fmr1-KO mice, there were no improvements in hyperactivity, anxiety level, and communication detected after R-Baclofen treatment. However, acute R-Baclofen injection could significantly reduce the repetitive grooming behavior in Fmr1-KO mice. While both BTBR and Fmr1-KO are used in ASD research, (Silverman et al., 2015) they are genetically and behaviorally very different (Nestler and Hyman, 2010; Meyza and Blanchard, 2017; Nikvarz et al., 2017; Niu et al., 2017). Therefore, the different effects of R-Baclofen on BTBR and Fmr1-KO mice’ behavior are not unexpected. Nonetheless, as previously mentioned, a more efficient way to study compound effects, is to study it separately for each behavioral assay considering both acute and chronic administrations.
4.4. LP-211 treatment
The serotonin 5-HT7 receptor agonist LP-211 has already been used to alleviate object recognition memory and stereotypic behavior by an acute administration of 3 mg/kg in 3–4 months old Fmr1-KO mice but did not affect wild type mice (Costa et al., 2018). In addition, Costa et al. (2015, 2018) showed that activation of the 5-HT7 receptor by LP-211 reversed mGluR-LTD in the hippocampal region of Fmr1-KO mice and prevented internalization of AMPA receptors by stimulating the adenylate cyclase and protein kinase A. LP-211 not only reversed mGluR-LTD in Fmr1-KO mice but also in wild type animals, exhibiting the potential of this compound to modulate synaptic plasticity impairments in Fmr1-KO mice (Costa et al., 2015). In 2020, Armstrong et al. (2020) showed that improvement of pharmacological properties and pharmacokinetics of a serotonergic compound, a combination of 5-HT1A, 5-HT2C, and 5-HT7 receptor agonists, can ameliorate social deficits, prevent audiogenic seizures, and decrease repetitive behavior in male and female Fmr1-KO mice on a FVB background. Chronic treatment with a low dose of LP-211 was further shown to improve Rett-syndrome-related phenotypes such as memory deficits and anxiety (De Filippis et al., 2014, 2015). Since LP-211 was already shown to penetrate the brain within 30 min after intraperitoneal injection (Hedlund et al., 2010; Costa et al., 2018), we used acute injections of 3 mg/kg of LP-211 30 min before each testing in Fmr1-KO mice resulting in significantly reduced anxious behavior in Fmr1-KO mice.
We were able to reproduce the positive effect of an acute LP-211 administration on repetitive behavior in Fmr1-KO mice even at a younger age than previously used (Costa et al., 2018). Additionally, Lacivita et al. (2020) synthesized and evaluated a variety of long-chain arylpiperazine compounds with biased selectivity to 5-HT7R that showed higher drug-like properties and a high affinity to distinctive 5-HT7 receptors resulting in a significantly improved repetitive behavior in 12–16 weeks old Fmr1-KO mice.
Altogether, previous reports conclude that LP-211 corrects synaptic plasticity, abnormal intracellular signaling, as well as memory and repetitive behavior. From our current report, we can further conclude that LP-211 rescues some of the behavioral abnormalities including repetitive behaviors in Fmr1-KO mice and it seems that LP-211 can also be used to ameliorate anxiety (Leopoldo et al., 2011; Costa et al., 2015, 2018; Lacivita et al., 2017).
We did not detect a positive effect of LP-211 on behavioral abnormalities in BTBR mice. Although, previous reports showed serotoninergic axon density reduction in the hippocampus (Guo and Commons, 2017) and a rescue of repetitive behavior in BTBR mice after acute treatment with a 5-HT6 receptor blocker (Amodeo et al., 2021). Finally, we can conclude that repetitive behavior seemed to be linked to the serotoninergic system as reported previously and that serotoninergic compounds could improve this behavior in ASD mouse models such as Fmr1-KO mice (Lewis and Kim, 2009; Muller et al., 2016). Further research is needed to improve the pharmacological properties and study the effect of serotoninergic compounds such as LP-211 in other ASD mouse models.
So far, no FDA-approved compound exists to improve core symptoms of ASD. Animal studies are critical and valuable tools to investigate the treatment effects of already existing pharmacological compounds and to develop new drug candidates. Our preclinical data from the characterization of BTBR and Fmr1-KO mice by using a similar but strain-specific behavioral test battery supports the use of these mouse models for ASD research. Moreover, evaluating the efficacy of two existing pharmacological tools showed the value of these strains for future novel therapeutics. We could show some of the core and secondary symptoms in these models, and also point out how precisely mouse models can mimic the ASD phenotypic spectrum with a wide diversity in abnormality severity of abnormality. We observed strong hyperactivity in Fmr1-KO mice, but a moderate level in BTBR mice. Measurements of anxiety-like behavior revealed high levels of this behavior in BTBR mice, but lower anxious behavior in Fmr1-KO mice which could be explained as a side effect of the observed hyperactivity. Repetitive behavior was detected in both models. In addition, Fmr1-KO mice showed impaired communication confirmed by ultrasonic vocalization measurements.
Altogether these observations support the comorbidity of ASD core symptoms with motor dysfunction (Bougeard et al., 2021; Posserud et al., 2021), hyperactivity, and anxiety in ASD individuals (Avni et al., 2018; Nimmo-Smith et al., 2020). Our data support the idea that the GABAergic and serotoninergic systems play an important role for ASD phenotypic symptoms. We could improve the repetitive behavior, one of the core symptoms of ASD, observed in Fmr1-KO mice with only a single injection of a low dose of R-Baclofen or LP-211.
Although ASD symptoms of BTBR and Fmr1-KO mice and the impact of R-Baclofen and LP-211 have been studied previously, only a fraction of the age-related ASD phenotype of these models are understood so far. Moreover, as observed in the current study, each compound mostly shows a slight effect on the behavioral abnormalities of BTBR and Fmr1-KO mice. A combined treatment of R-Baclofen and LP-211 should be considered as a strategy for future ASD studies.
Data availability statement
The original contributions presented in this study are included in the article/Supplementary material, further inquiries can be directed to the corresponding author.
Ethics statement
This animal study was reviewed and approved by Amt der Steiermärkischen Landesregierung, Abteilung 13 – Umwelt und Raumordnung Austria.
Author contributions
SS designed, performed, analyzed all the experiments, prepared, and corrected the manuscript. SF performed the main revision and corrected the manuscript. MD supervised SS and contributed to the experimental design. RR interpreted the results. ML and EL provided the LP-211 compound and reviewed the manuscript. BC supervised SS and reviewed the manuscript. BH-P and MP conceived the study, interpreted the data, and edited the manuscript. All authors contributed to the article and approved the submitted version.
Funding
This work was supported by the Austrian Research Promotion Agency (FFG: 875225) as well as QPS Austria GmbH.
Acknowledgments
We greatly thank the whole research team of QPS Austria GmbH, primarily our colleagues operating the animal facility for their unlimited support.
Conflict of interest
SS, SF, MD, RR, MP, and BH-P were employed by QPS Austria GmbH.
The remaining authors declare that the research was conducted in the absence of any commercial or financial relationships that could be construed as a potential conflict of interest.
Publisher’s note
All claims expressed in this article are solely those of the authors and do not necessarily represent those of their affiliated organizations, or those of the publisher, the editors and the reviewers. Any product that may be evaluated in this article, or claim that may be made by its manufacturer, is not guaranteed or endorsed by the publisher.
Supplementary material
The Supplementary Material for this article can be found online at: https://www.frontiersin.org/articles/10.3389/fnins.2023.1087788/full#supplementary-material
References
Afshar, S., Lule, S., Yuan, G., Qu, X., Pan, C., Whalen, M., et al. (2022). Longitudinal PET studies of mGluR5 in FXS using an FMR1 knockout mouse model. Transl. Neurosci. 13, 80–92. doi: 10.1515/tnsci-2022-0217
Amodeo, D. A., Oliver, B., Pahua, A., Hitchcock, K., Bykowski, A., Tice, D., et al. (2021). Serotonin 6 receptor blockade reduces repetitive behavior in the BTBR mouse model of autism spectrum disorder. Pharmacol. Biochem. Behav. 200:173076. doi: 10.1016/j.pbb.2020.173076
Amodeo, D. A., Yi, J., Sweeney, J., and Ragozzino, M. (2014). Oxotremorine treatment reduces repetitive behaviors in BTBR T+ tf/J mice. Front. Synaptic Neurosci. 6:17. doi: 10.3389/fnsyn.2014.00017
Anderson, G. M., Feibel, F. C., and Cohen, D. J. (1987). Determination of serotonin in whole blood, platelet-rich plasma, platelet-poor plasma and plasma ultrafiltrate. Life Sci. 40, 1063–1070. doi: 10.1016/0024-3205(87)90568-6
Andersson, M., Tangen, Ä, Farde, L., Bölte, S., Halldin, C., Borg, J., et al. (2021). Serotonin transporter availability in adults with autism—a positron emission tomography study. Mol. Psychiatry 26, 1647–1658. doi: 10.1038/s41380-020-00868-3
Armstrong, J. L., Casey, A., Saraf, T., Mukherjee, M., Booth, R., and Canal, C. (2020). (S)-5-(2’-Fluorophenyl)-N,N-dimethyl-1,2,3,4-tetrahydronaphthalen-2-amine, a serotonin receptor modulator, possesses anticonvulsant, prosocial, and anxiolytic-like properties in an Fmr1 knockout mouse model of fragile X syndrome and autism spectrum disorder. ACS Pharmacol. Transl. Sci. 3, 509–523. doi: 10.1021/acsptsci.9b00101
Avni, E., Ben-Itzchak, E., and Zachor, D. A. (2018). The presence of comorbid ADHD and anxiety symptoms in autism spectrum disorder: Clinical presentation and predictors. Front. Psychiatry 9:717. doi: 10.3389/fpsyt.2018.00717
Bakker, C. E., Verheij, C., Willemsen, R., van der Helm, R., Oerlemans, F., Vermey, M., et al. (1994). Fmr1 knockout mice: A model to study fragile X mental 926 retardation. Cell 78, 23–33. doi: 10.1016/0092-8674(94)90569-X
Belagodu, A. P., Johnson, A. M., and Galvez, R. (2016). Characterization of ultrasonic vocalizations of Fragile X mice. Behav. Brain Res. 310, 76–83. doi: 10.1016/j.bbr.2016.04.016
Benno, R., Smirnova, Y., Vera, S., Liggett, A., and Schanz, N. (2009). Exaggerated responses to stress in the BTBR T+tf/J mouse: An unusual behavioral phenotype. Behav. Brain Res. 197, 462–465. doi: 10.1016/j.bbr.2008.09.041
Berry-Kravis, E. M., Hessl, D., Rathmell, B., Zarevics, P., Cherubini, M., Walton-Bowen, K., et al. (2012). Effects of STX209 (arbaclofen) on neurobehavioral function in children and adults with fragile X syndrome: A randomized, controlled, phase 2 trial. Sci. Transl. Med. 4:152ra127. doi: 10.1126/scitranslmed.3004214
Berry-Kravis, E., Hagerman, R., Visootsak, J., Budimirovic, D., Kaufmann, W., Cherubini, M., et al. (2017). Arbaclofen in fragile X syndrome: Results of phase 3 trials. J. Neurodev. Disord. 9:3. doi: 10.1186/s11689-016-9181-6
Bonnet-Brilhault, F., Alirol, S., Blanc, R., Bazaud, S., Marouillat, S., Thépault, R., et al. (2016). GABA/Glutamate synaptic pathways targeted by integrative genomic and electrophysiological explorations distinguish autism from intellectual disability. Mol. Psychiatry 21, 411–418. doi: 10.1038/mp.2015.75
Booker, S. A., Domanski, A. P. F., Dando, O. R., Jackson, A. D., Isaac, J. T. R., Hardingham, G. E., et al. (2019). Altered dendritic spine function and integration in a mouse model of fragile X syndrome. Nat. Commun. 10:4813.
Bougeard, C., Picarel-Blanchot, F., Schmid, R., Campbell, R., and Buitelaar, J. (2021). Prevalence of autism spectrum disorder and co-morbidities in children and adolescents: A systematic literature review. Front. Psychiatry 12:744709. doi: 10.3389/fpsyt.2021.744709
Bourgeron, T. (2009). A synaptic trek to autism. Curr. Opin. Neurobiol. 19, 231–234. doi: 10.1016/j.conb.2009.06.003
Caldwell-Harris, C. L. (2021). An explanation for repetitive motor behaviors in autism: Facilitating inventions via trial-and-error discovery. Front. Psychiatry 12:657774. doi: 10.3389/fpsyt.2021.657774
Canitano, R., and Palumbi, R. (2021). Excitation/inhibition modulators in autism spectrum disorder: Current clinical research. Front. Neurosci. 15:753274. doi: 10.3389/fnins.2021.753274
Chao, O. Y., Yunger, R., and Yang, Y. M. (2018). Behavioral assessments of BTBR T+Itpr3tf/J mice by tests of object attention and elevated open platform: Implications for an animal model of psychiatric comorbidity in autism. Behav. Brain Res. 347, 140–147. doi: 10.1016/j.bbr.2018.03.014
Cheng, N., Pagtalunan, E., Abushaibah, A., Naidu, J., Stell, W., Rho, J., et al. (2020). Atypical visual processing in a mouse model of autism. Sci. Rep. 10:12390. doi: 10.1038/s41598-020-68589-9
Cochran, D., Sikoglu, E., Hodge, S., Edden, R., Foley, A., Kennedy, D., et al. (2015). Relationship among Glutamine, γ-aminobutyric acid, and social cognition in autism spectrum disorders. J. Child Adolesc. Psychopharmacol. 25, 314–322. doi: 10.1089/cap.2014.0112
Comery, T. A., Harris, J., Willems, P., Oostra, B., Irwin, S., Weiler, I., et al. (1997). Abnormal dendritic spines in fragile X knockout mice: Maturation and pruning deficits. Proc. Natl. Acad. Sci. 94, 5401–5404. doi: 10.1073/pnas.94.10.5401
Costa, L., Sardone, L., Bonaccorso, C., D’Antoni, S., Spatuzza, M., Gulisano, W., et al. (2018). Activation of serotonin 5-HT7 receptors modulates hippocampal synaptic plasticity by stimulation of adenylate cyclases and rescues learning and behavior in a mouse model of fragile X syndrome. Front. Mol. Neurosci. 11:353. doi: 10.3389/fnmol.2018.00353
Costa, L., Sardone, L., Lacivita, E., Leopoldo, M., and Ciranna, L. (2015). Novel agonists for serotonin 5-HT7 receptors reverse metabotropic glutamate receptor-mediated long-term depression in the hippocampus of wild-type and Fmr1 KO mice, a model of Fragile X Syndrome. Front. Behav. Neurosci. 9:65. doi: 10.3389/fnbeh.2015.00065
Costa, L., Spatuzza, M., D’Antoni, S., Bonaccorso, C., Trovato, C., Musumeci, S., et al. (2012). Activation of 5-HT7 serotonin receptors reverses metabotropic glutamate receptor-mediated synaptic plasticity in wild-type and Fmr1 knockout mice, a model of Fragile X syndrome. Biol. Psychiatry 72, 924–933. doi: 10.1016/j.biopsych.2012.06.008
Costa, L., Tempio, A., Lacivita, E., Leopoldo, M., and Ciranna, L. (2021). Serotonin 5-HT7 receptors require cyclin-dependent kinase 5 to rescue hippocampal synaptic plasticity in a mouse model of Fragile X Syndrome. Eur. J. Neurosci. 54, 4124–4132. doi: 10.1111/ejn.15246
Cryan, J. F., Kelly, P., Chaperon, F., Gentsch, C., Mombereau, C., Lingenhoehl, K., et al. (2004). Behavioral Characterization of the novel GABAB receptor-positive modulator GS39783 (<em>N</em>,<em>N</em>’-Dicyclopentyl-2-methylsulfanyl-5-nitro-pyrimidine-4,6-diamine): Anxiolytic-like activity without side effects associated with baclofen or benzodiazepines. J. Pharmacol. Exp. Ther. 310, 952–963. doi: 10.1124/jpet.104.066753
Culotta, L., and Penzes, P. (2020). Exploring the mechanisms underlying excitation/inhibition imbalance in human iPSC-derived models of ASD. Mol. Autism 11:32. doi: 10.1186/s13229-020-00339-0
De Filippis, B., Chiodi, V., Adriani, W., Lacivita, E., Mallozzi, C., Leopoldo, M., et al. (2015). Long-lasting beneficial effects of central serotonin receptor 7 stimulation in female mice modeling Rett syndrome. Front. Behav. Neurosci. 9:86. doi: 10.3389/fnbeh.2015.00086
De Filippis, B., Nativio, P., Fabbri, A., Ricceri, L., Adriani, W., Lacivita, E., et al. (2014). Pharmacological stimulation of the brain serotonin receptor 7 as a novel therapeutic approach for Rett syndrome. Neuropsychopharmacology 39, 2506–2518. doi: 10.1038/npp.2014.105
Delahanty, R. J., Kang, J., Brune, C., Kistner, E., Courchesne, E., Cox, N., et al. (2011). Maternal transmission of a rare GABRB3 signal peptide variant is associated with autism. Mol. Psychiatry 16, 86–96. doi: 10.1038/mp.2009.118
Dolan, B. M., Duron, S., Campbell, D., Vollrath, B., Shankaranarayana, R. B., Ko, H., et al. (2013). Rescue of fragile X syndrome phenotypes in Fmr1 KO mice by the small-molecule PAK inhibitor FRAX486. Proc. Natl. Acad. Sci. 110, 5671–5676. doi: 10.1073/pnas.1219383110
Edwards, D. A. (1970). Induction of estrus in female mice: Estrogen-progesterone interactions. Horm. Behav. 1, 299–304. doi: 10.1016/0018-506X(70)90022-X
Eid, M., Gollwitzer, M., and Schmitt, M. (2011). Statistik und Forschungsmethoden: Lehrbuch. Mit Online-Materialien. Weinheim: Beltz.
Erickson, C. A., Veenstra-Vanderweele, J., Melmed, R., McCracken, J., Ginsberg, L., Sikich, L., et al. (2014). STX209 (arbaclofen) for autism spectrum disorders: An 8-week open-label study. J. Autism Dev. Disord. 44, 958–964. doi: 10.1007/s10803-013-1963-z
Folk, G. E. Jr., and Long, J. P. (1988). Serotonin as a neurotransmitter: A review. Comp. Biochem. Physiol. C Comp. Pharmacol. Toxicol. 91, 251–257. doi: 10.1016/0742-8413(88)90193-4
Gantois, I., Khoutorsky, A., Popic, J., Aguilar-Valles, A., Freemantle, E., Cao, R., et al. (2017). Metformin ameliorates core deficits in a mouse model of fragile X syndrome. Nat. Med. 23, 674–677. doi: 10.1038/nm.4335
Gaudissard, J., Ginger, M., Premoli, M., Memo, M., Frick, A., and Pietropaolo, S. (2017). Behavioral abnormalities in the Fmr1-KO2 mouse model of fragile X syndrome: The relevance of early life phases. Autism Res. 10, 1584–1596. doi: 10.1002/aur.1814
Geschwind, D. H., and Levitt, P. (2007). Autism spectrum disorders: Developmental disconnection syndromes. Curr. Opin. Neurobiol. 17, 103–111. doi: 10.1016/j.conb.2007.01.009
Gibson, J. R., Bartley, A., Hays, S., and Huber, K. (2008). Imbalance of neocortical excitation and inhibition and altered UP states reflect network hyperexcitability in the mouse model of fragile X syndrome. J. Neurophysiol. 100, 2615–2626. doi: 10.1152/jn.90752.2008
Gogolla, N., Takesian, A., Feng, G., Fagiolini, M., and Hensch, T. (2014). Sensory integration in mouse insular cortex reflects GABA circuit maturation. Neuron 83, 894–905. doi: 10.1016/j.neuron.2014.06.033
Guo, H., Peng, Y., Hu, Z., Li, Y., Xun, G., Ou, J., et al. (2017). Genome-wide copy number variation analysis in a Chinese autism spectrum disorder cohort. Sci. Rep. 7:44155. doi: 10.1038/srep44155
Guo, Y. P., and Commons, K. G. (2017). Serotonin neuron abnormalities in the BTBR mouse model of autism. Autism Res. 10, 66–77. doi: 10.1002/aur.1665
Han, S., Tai, C., Jones, C., Scheuer, T., and Catterall, W. (2014). Enhancement of Inhibitory Neurotransmission by GABAA receptors having α2,3-subunits ameliorates behavioral deficits in a mouse model of autism. Neuron 81, 1282–1289. doi: 10.1016/j.neuron.2014.01.016
Hashemi, E., Sahbaie, P., Davies, M., Clark, J., and DeLorey, T. (2007). Gabrb3 gene deficient mice exhibit increased risk assessment behavior, hypotonia and expansion of the plexus of locus coeruleus dendrites. Brain Res. 1129, 191–199. doi: 10.1016/j.brainres.2006.10.050
Hébert, B., Pietropaolo, S., Même, S., Laudier, B., Laugeray, A., Doisne, N., et al. (2014). Rescue of fragile X syndrome phenotypes in Fmr1KO mice by a BKCa channel opener molecule. Orphanet J. Rare Dis. 9:124. doi: 10.1186/s13023-014-0124-6
Hedlund, P. B., Leopoldo, M., Caccia, S., Sarkisyan, G., Fracasso, C., Martelli, G., et al. (2010). LP-211 is a brain penetrant selective agonist for the serotonin 5-HT(7) receptor. Neurosci. Lett. 481, 12–16. doi: 10.1016/j.neulet.2010.06.036
Henderson, C., Wijetunge, L., Kinoshita, M., Shumway, M., Hammond, R., Postma, F., et al. (2012). Reversal of disease-related pathologies in the fragile X mouse model by selective activation of GABAB receptors with arbaclofen. Sci. Transl. Med. 4:152ra128. doi: 10.1126/scitranslmed.3004218
Hodges, S. L., Nolan, S., Reynolds, C., and Lugo, J. (2017). Spectral and temporal properties of calls reveal deficits in ultrasonic vocalizations of adult Fmr1 knockout mice. Behav. Brain Res. 332, 50–58. doi: 10.1016/j.bbr.2017.05.052
Hoffmann, F., Musolf, K., and Penn, D. J. (2009). Freezing urine reduces its efficacy for eliciting ultrasonic vocalizations from male mice. Physiol. Behav. 96, 602–605. doi: 10.1016/j.physbeh.2008.12.014
Jasien, J. M., Daimon, C., Wang, R., Shapiro, B., Martin, B., and Maudsley, S. (2014). The effects of aging on the BTBR mouse model of autism spectrum disorder. Front. Aging Neurosci. 6:225. doi: 10.3389/fnagi.2014.00225
Kalueff, A. V., Stewart, A., Song, C., Berridge, K., Graybiel, A., and Fentress, J. (2016). Neurobiology of rodent self-grooming and its value for translational neuroscience. Nat. Rev. Neurosci. 17, 45–59. doi: 10.1038/nrn.2015.8
Kazdoba, T. M., Hagerman, R., Zolkowska, D., Rogawski, M., and Crawley, J. (2016). Evaluation of the neuroactive steroid ganaxolone on social and repetitive behaviors in the BTBR mouse model of autism. Psychopharmacology 233, 309–323. doi: 10.1007/s00213-015-4115-7
Kazdoba, T. M., Leach, P., Silverman, J., and Crawley, J. (2014). Modeling fragile X syndrome in the Fmr1 knockout mouse. Intractable Rare Dis. Res. 3, 118–133. doi: 10.5582/irdr.2014.01024
Kolevzon, A., Mathewson, K. A., and Hollander, E. (2006). Selective serotonin reuptake inhibitors in autism: A review of efficacy and tolerability. J. Clin. Psychiatry 67, 407–414. doi: 10.4088/JCP.v67n0311
Kramvis, I., Mansvelder, H., Loos, M., and Meredith, R. (2013). Hyperactivity, perseveration and increased responding during attentional rule acquisition in the Fragile X mouse model. Front. Behav. Neurosci. 7:172. doi: 10.3389/fnbeh.2013.00172
Kubas, B., Kułak, W., Sobaniec, W., Tarasow, E., Lebkowska, U., and Walecki, J. (2012). Metabolite alterations in autistic children: A 1H MR spectroscopy study. Adv. Med. Sci. 57, 152–156. doi: 10.2478/v10039-012-0014-x
Lacivita, E., Niso, M., Stama, M., Arzuaga, A., Altamura, C., Costa, L., et al. (2020). Privileged scaffold-based design to identify a novel drug-like 5-HT7 receptor-preferring agonist to target Fragile X syndrome. Eur. J. Med. Chem. 199:112395. doi: 10.1016/j.ejmech.2020.112395
Lacivita, E., Perrone, R., Margari, L., and Leopoldo, M. (2017). Targets for drug therapy for autism spectrum disorder: Challenges and future directions. J. Med. Chem. 60, 9114–9141. doi: 10.1021/acs.jmedchem.7b00965
Lal, R., Sukbuntherng, J., Tai, E., Upadhyay, S., Yao, F., Warren, M., et al. (2009). Arbaclofen placarbil, a novel < < cps:it > em < /cps:it > > < cps:it > R < /cps:it > < /em>-baclofen prodrug: Improved absorption, distribution, metabolism, and elimination properties compared with < < cps:it > em < /cps:it > > < cps:it > R < /cps:it > < /em>-Baclofen. J. Pharmacol. Exp. Ther. 330, 911–921. doi: 10.1124/jpet.108.149773
Leboucher, A., Bermudez-Martin, P., Mouska, X., Amri, E., Pisani, D., and Davidovic, L. (2019). Fmr1-deficiency impacts body composition, skeleton, and bone microstructure in a mouse model of fragile X syndrome. Front. Endocrinol. 10:678. doi: 10.3389/fendo.2019.00678
Leboyer, M., Philippe, A., Bouvard, M., Guilloud-Bataille, M., Bondoux, D., Tabuteau, F., et al. (1999). Whole blood serotonin and plasma beta-endorphin in autistic probands and their first-degree relatives. Biol. Psychiatry 45, 158–163. doi: 10.1016/S0006-3223(97)00532-5
Lee, J., Avramets, D., Jeon, B., and Choo, H. (2021). Modulation of serotonin receptors in neurodevelopmental disorders: Focus on 5-HT7 receptor. Molecules 26:3348. doi: 10.3390/molecules26113348
Leopoldo, M., Lacivita, E., Berardi, F., Perrone, R., and Hedlund, P. (2011). Serotonin 5-HT7 receptor agents: Structure-activity relationships and potential therapeutic applications in central nervous system disorders. Pharmacol. Ther. 129, 120–148. doi: 10.1016/j.pharmthera.2010.08.013
Lewis, M., and Kim, S. J. (2009). The pathophysiology of restricted repetitive behavior. J. Neurodev. Disord. 1, 114–132. doi: 10.1007/s11689-009-9019-6
Lister, R. G. (1987). The use of a plus-maze to measure anxiety in the mouse. Psychopharmacology 92, 180–185. doi: 10.1007/BF00177912
Loomes, R., Hull, L., and Mandy, W. P. L. (2017). What is the male-to-female ratio in autism spectrum disorder? A systematic review and meta-analysis. J. Am. Acad. Child Adolesc. Psychiatry 56, 466–474. doi: 10.1016/j.jaac.2017.03.013
McFarlane, H. G., Kusek, G., Yang, M., Phoenix, J., Bolivar, V., and Crawley, J. (2008). Autism-like behavioral phenotypes in BTBR T+tf/J mice. Genes Brain Behav. 7, 152–163. doi: 10.1111/j.1601-183X.2007.00330.x
Meyza, K. Z., and Blanchard, D. C. (2017). The BTBR mouse model of idiopathic autism – current view on mechanisms. Neurosci. Biobehav. Rev. 76(Pt A), 99–110. doi: 10.1016/j.neubiorev.2016.12.037
Michalon, A., Sidorov, M., Ballard, T., Ozmen, L., Spooren, W., Wettstein, J., et al. (2012). Chronic pharmacological mGlu5 inhibition corrects fragile X in adult mice. Neuron 74, 49–56. doi: 10.1016/j.neuron.2012.03.009
Michelini, G., Eley, T., Gregory, A., and McAdams, T. (2015). Aetiological overlap between anxiety and attention deficit hyperactivity symptom dimensions in adolescence. J. Child Psychol. Psychiatry 56, 423–431. doi: 10.1111/jcpp.12318
Mines, M. A., Yuskaitis, C., King, M., Beurel, E., and Jope, R. (2010). GSK3 influences social preference and anxiety-related behaviors during social interaction in a mouse model of fragile X syndrome and autism. PLoS One 5:e9706. doi: 10.1371/journal.pone.0009706
Mineur, Y. S., Huynh, L. X., and Crusio, W. E. (2006). Social behavior deficits in the Fmr1 mutant mouse. Behav. Brain Res. 168, 172–175. doi: 10.1016/j.bbr.2005.11.004
Mineur, Y. S., Sluyter, F., de Wit, S., Oostra, B., and Crusio, W. (2002). Behavioral and neuroanatomical characterization of the Fmr1 knockout mouse. Hippocampus 12, 39–46. doi: 10.1002/hipo.10005
Möhrle, D., Wang, W., Whitehead, S., and Schmid, S. (2021). GABAB receptor agonist R-baclofen reverses altered auditory reactivity and filtering in the Cntnap2 Knock-out rat. Front. Integr. Neurosci. 15:710593. doi: 10.3389/fnint.2021.710593
Muller, C. L., Anacker, A. M. J., and Veenstra-VanderWeele, J. (2016). The serotonin system in autism spectrum disorder: From biomarker to animal models. Neuroscience 321, 24–41. doi: 10.1016/j.neuroscience.2015.11.010
Mulraney, M., Schilpzand, E., Anderson, V., Nicholson, J., Efron, D., Hazell, P., et al. (2018). Correlates of anxiety in 6- to 8-year-old children with ADHD: A community-based study. J. Atten. Disord. 22, 425–434. doi: 10.1177/1087054716638510
Murray, M. J. (2010). Attention-deficit/hyperactivity disorder in the context of autism spectrum disorders. Curr. Psychiatry Rep. 12, 382–388. doi: 10.1007/s11920-010-0145-3
Nah, W. H., Park, M. J., and Gye, M. C. (2011). Effects of early prepubertal exposure to bisphenol A on the onset of puberty, ovarian weights, and estrous cycle in female mice. Clin. Exp. Reprod. Med. 38, 75–81. doi: 10.5653/cerm.2011.38.2.75
Nestler, E. J., and Hyman, S. E. (2010). Animal models of neuropsychiatric disorders. Nat. Neurosci. 13, 1161–1169. doi: 10.1038/nn.2647
Nikvarz, N., Alaghband-Rad, J., Tehrani-Doost, M., Alimadadi, A., and Ghaeli, P. (2017). Comparing efficacy and side effects of memantine vs. Risperidone in the treatment of autistic disorder. Pharmacopsychiatry 50, 19–25. doi: 10.1055/s-0042-108449
Nimmo-Smith, V., Heuvelman, H., Dalman, C., Lundberg, M., Idring, S., Carpenter, P., et al. (2020). Anxiety disorders in adults with autism spectrum disorder: A population-based study. J. Autism Dev. Disord. 50, 308–318. doi: 10.1007/s10803-019-04234-3
Niu, M., Han, Y., Dy, A., Du, J., Jin, H., Qin, J., et al. (2017). Autism symptoms in fragile X syndrome. J. Child Neurol. 32, 903–909. doi: 10.1177/0883073817712875
Noroozi, R., Taheri, M., Ghafouri-Fard, S., Bidel, Z., Omrani, M., Moghaddam, A., et al. (2018). Meta-analysis of GABRB3 gene polymorphisms and susceptibility to autism spectrum disorder. J. Mol. Neurosci. 65, 432–437. doi: 10.1007/s12031-018-1114-2
O’Connor, R., van De Wouw, M., Moloney, G., Ventura-Silva, A., O’Riordan, K., Golubeva, A., et al. (2021). Strain differences in behaviour and immunity in aged mice: Relevance to Autism. Behav. Brain Res. 399:113020. doi: 10.1016/j.bbr.2020.113020
Olmos-Serrano, J. L., Corbin, J. G., and Burns, M. P. (2011). The GABA(A) receptor agonist THIP ameliorates specific behavioral deficits in the mouse model of fragile X syndrome. Dev. Neurosci. 33, 395–403. doi: 10.1159/000332884
Pagan, C., Delorme, R., Callebert, J., Goubran-Botros, H., Amsellem, F., Drouot, X., et al. (2014). The serotonin-N-acetylserotonin-melatonin pathway as a biomarker for autism spectrum disorders. Transl. Psychiatry 4:e479. doi: 10.1038/tp.2014.120
Paluszkiewicz, S. M., Martin, B. S., and Huntsman, M. M. (2011). Fragile X syndrome: The GABAergic system and circuit dysfunction. Dev. Neurosci. 33, 349–364. doi: 10.1159/000329420
Petkov, P. M., Cassell, M., Sargent, E., Donnelly, C., Robinson, P., Crew, V., et al. (2004). Development of a SNP genotyping panel for genetic monitoring of the laboratory mouse. Genomics 83, 902–911. doi: 10.1016/j.ygeno.2003.11.007
Pietropaolo, S., Guilleminot, A., Martin, B., D’Amato, F., and Crusio, W. (2011). Genetic-background modulation of core and variable autistic-like symptoms in Fmr1 knock-out mice. PLoS One 6:e17073. doi: 10.1371/journal.pone.0017073
Pliszka, S. R. (2000). Patterns of psychiatric comorbidity with attention-deficit/hyperactivity disorder. Child Adolesc. Psychiatr. Clin. N. Am. 9, 525–540. doi: 10.1016/S1056-4993(18)30105-6
Pobbe, R. L., Defensor, E., Pearson, B., Bolivar, V., Blanchard, D., and Blanchard, R. (2011). General and social anxiety in the BTBR T+ tf/J mouse strain. Behav. Brain Res. 216, 446–451. doi: 10.1016/j.bbr.2010.08.039
Posserud, M.-B., Skretting Solberg, B., Engeland, A., Haavik, J., and Klungsøyr, K. (2021). Male to female ratios in autism spectrum disorders by age, intellectual disability and attention-deficit/hyperactivity disorder. Acta Psychiatr. Scand. 144, 635–646. doi: 10.1111/acps.13368
Premoli, M., Memo, M., and Bonini, S. A. (2021). Ultrasonic vocalizations in mice: Relevance for ethologic and neurodevelopmental disorders studies. Neural Regen. Res. 16, 1158–1167. doi: 10.4103/1673-5374.300340
Qin, M., Huang, T., Kader, M., Krych, L., Xia, Z., Burlin, T., et al. (2015). R-baclofen reverses a social behavior deficit and elevated protein synthesis in a mouse model of fragile X syndrome. Int. J. Neuropsychopharmacol. 18:yv034. doi: 10.1093/ijnp/pyv034
Qin, M., Kang, J., and Smith, C. B. (2002). Increased rates of cerebral glucose metabolism in a mouse model of fragile X mental retardation. Proc. Natl. Acad. Sci. U.S.A. 99, 15758–15763. doi: 10.1073/pnas.242377399
Robertson, C., Ratai, E., and Kanwisher, N. (2016). Reduced GABAergic action in the autistic brain. Curr. Biol. 26, 80–85. doi: 10.1016/j.cub.2015.11.019
Rogers, S. J., Wehner, D. E., and Hagerman, R. (2001). The behavioral phenotype in fragile X: Symptoms of autism in very young children with fragile X syndrome, idiopathic autism, and other developmental disorders. J. Dev. Behav. Pediatr. 22, 409–417. doi: 10.1097/00004703-200112000-00008
Rotschafer, S. E., Trujillo, M., Dansie, L., Ethell, I., and Razak, K. (2012). Minocycline treatment reverses ultrasonic vocalization production deficit in a mouse model of Fragile X Syndrome. Brain Res. 1439, 7–14. doi: 10.1016/j.brainres.2011.12.041
Saitow, F., Takumi, T., and Suzuki, H. (2020). Upregulated 5-HT1A receptor-mediated currents in the prefrontal cortex layer 5 neurons in the 15q11–13 duplication mouse model of autism. Mol. Brain 13:115. doi: 10.1186/s13041-020-00655-9
Saré, R. M., Levine, M., and Smith, C. B. (2016). Behavioral phenotype of Fmr1 knock-out mice during active phase in an altered light/dark cycle. eNeuro 3, ENEURO.35–ENEURO.16. doi: 10.1523/ENEURO.0035-16.2016
Schain, R. J., and Freedman, D. X. (1961). Studies on 5-hydroxyindole metabolism in autistic and other mentally retarded children. J. Pediatr. 58, 315–320. doi: 10.1016/S0022-3476(61)80261-8
Seibenhener, M. L., and Wooten, M. C. (2015). Use of the open field maze to measure locomotor and anxiety-like behavior in mice. J. Vis. Exp. 96, e52434–e52434. doi: 10.3791/52434
Silverman, J. L., Pride, M., Hayes, J., Puhger, K., Butler-Struben, H., Baker, S., et al. (2015). GABAB receptor agonist R-baclofen reverses social deficits and reduces repetitive behavior in two mouse models of autism. Neuropsychopharmacology 40, 2228–2239. doi: 10.1038/npp.2015.66
Silverman, J. L., Tolu, S., Barkan, C., and Crawley, J. (2010a). Repetitive self-grooming behavior in the BTBR mouse model of autism is blocked by the mGluR5 antagonist MPEP. Neuropsychopharmacology 35, 976–989. doi: 10.1038/npp.2009.201
Silverman, J. L., Yang, M., Lord, C., and Crawley, J. N. (2010b). Behavioural phenotyping assays for mouse models of autism. Nat. Rev. Neurosci. 11, 490–502. doi: 10.1038/nrn2851
Sinclair, D., Featherstone, R., Naschek, M., Nam, J., Du, A., Wright, S., et al. (2017). GABA-B agonist baclofen normalizes auditory-evoked neural oscillations and behavioral deficits in the < < cps:it > em < /cps:it > > < cps:it > Fmr1 < /cps:it > < /em> knockout mouse model of fragile X syndrome. eneuro 4, ENEURO.380–ENEURO.316. doi: 10.1523/ENEURO.0380-16.2017
Spencer, C. M., Alekseyenko, O., Serysheva, E., Yuva-Paylor, L., and Paylor, R. (2005). Altered anxiety-related and social behaviors in the Fmr1 knockout mouse model of fragile X syndrome. Genes Brain Behav. 4, 420–430. doi: 10.1111/j.1601-183X.2005.00123.x
Speranza, L., Labus, J., Volpicelli, F., Guseva, D., Lacivita, E., Leopoldo, M., et al. (2017). Serotonin 5-HT7 receptor increases the density of dendritic spines and facilitates synaptogenesis in forebrain neurons. J. Neurochem. 141, 647–661. doi: 10.1111/jnc.13962
Stoppel, L. J., Kazdoba, T., Schaffler, M., Preza, A., Heynen, A., Crawley, J., et al. (2018). R-Baclofen reverses cognitive deficits and improves social interactions in two lines of 16p11.2 deletion mice. Neuropsychopharmacology 43, 513–524. doi: 10.1038/npp.2017.236
Telias, M. (2019). Molecular mechanisms of synaptic dysregulation in fragile X syndrome and autism spectrum disorders. Front. Mol. Neurosci. 12:51. doi: 10.3389/fnmol.2019.00051
The Dutch-Belgian Fragile X Consortium (1994). Fmr1 knockout mice: A model to study fragile X mental retardation. The dutch-belgian fragile X consortium. Cell 78, 23–33.
Timmermans, S., Montagu, M., and Van Libert, C. (2017). Complete overview of protein-inactivating sequence variations in 36 sequenced mouse inbred strains. Proc. Natl. Acad. Sci. U.S.A. 114, 9158–9163. doi: 10.1073/pnas.1706168114
Uzunova, G., Pallanti, S., and Hollander, E. (2016). Excitatory/inhibitory imbalance in autism spectrum disorders: Implications for interventions and therapeutics. World J. Biol. Psychiatry 17, 174–186. doi: 10.3109/15622975.2015.1085597
van der Meer, D., Hoekstra, P., van Rooij, D., Winkler, A., van Ewijk, H., Heslenfeld, D., et al. (2018). Anxiety modulates the relation between attention-deficit/hyperactivity disorder severity and working memory-related brain activity. World J. Biol. Psychiatry 19, 450–460. doi: 10.1080/15622975.2017.1287952
van Putten, M. (2014). The use of hanging wire tests to monitor muscle strength and condition over time. in “TREAT-NMD SOP DMD_M.2.1.004.” Leiden: Treat-NMD Neuromuscular Network.
Veenstra-VanderWeele, J., Cook, E., King, B., Zarevics, P., Cherubini, M., Walton-Bowen, K., et al. (2017). Arbaclofen in children and adolescents with autism spectrum disorder: A randomized, controlled, phase 2 trial. Neuropsychopharmacology 42, 1390–1398. doi: 10.1038/npp.2016.237
Xiao, R., Zhong, H., Li, X., Ma, Y., Zhang, R., Wang, L., et al. (2020). Abnormal cerebellar development is involved in dystonia-like behaviors and motor dysfunction of autistic BTBR mice. Front. Cell Dev. Biol. 8:231. doi: 10.3389/fcell.2020.00231
Yen, Y.-C., Anderzhanova, E., Bunck, M., Schuller, J., Landgraf, R., and Wotjak, C. (2013). Co-segregation of hyperactivity, active coping styles, and cognitive dysfunction in mice selectively bred for low levels of anxiety. Front. Behav. Neurosci. 7:103. doi: 10.3389/fnbeh.2013.00103
Zaboski, B. A., and Storch, E. A. (2018). Comorbid autism spectrum disorder and anxiety disorders: A brief review. Future Neurol. 13, 31–37. doi: 10.2217/fnl-2017-0030
Zeidan, J., Fombonne, E., Scorah, J., Ibrahim, A., Durkin, M., Saxena, S., et al. (2022). Global prevalence of autism: A systematic review update. Autism Res. 15, 778–790. doi: 10.1002/aur.2696
Keywords: autism spectrum disorders, locomotor behavior, anxiety, repetitive behavior, ultrasonic vocalization, GABAB receptor agonist, 5-HT7 receptor agonist
Citation: Sharghi S, Flunkert S, Daurer M, Rabl R, Chagnaud BP, Leopoldo M, Lacivita E, Hutter-Paier B and Prokesch M (2023) Evaluating the effect of R-Baclofen and LP-211 on autistic behavior of the BTBR and Fmr1-KO mouse models. Front. Neurosci. 17:1087788. doi: 10.3389/fnins.2023.1087788
Received: 02 November 2022; Accepted: 07 March 2023;
Published: 30 March 2023.
Edited by:
Carla Denise Bonan, Pontifical Catholic University of Rio Grande do Sul, BrazilReviewed by:
David Alcantara-Gonzalez, Nathan Kline Institute for Psychiatric Research, United StatesSusanne Schmid, Western University, Canada
Copyright © 2023 Sharghi, Flunkert, Daurer, Rabl, Chagnaud, Leopoldo, Lacivita, Hutter-Paier and Prokesch. This is an open-access article distributed under the terms of the Creative Commons Attribution License (CC BY). The use, distribution or reproduction in other forums is permitted, provided the original author(s) and the copyright owner(s) are credited and that the original publication in this journal is cited, in accordance with accepted academic practice. No use, distribution or reproduction is permitted which does not comply with these terms.
*Correspondence: Shirin Sharghi, U2hpcmluLnNoYXJnaGlAcXBzLmNvbQ==