- 1Institute of Psychopharmacology, Central Institute of Mental Health, Medical Faculty Mannheim, University of Heidelberg, Mannheim, Germany
- 2Interdisciplinary Center for Neurosciences, University of Heidelberg, Heidelberg, Germany
- 3Department of Molecular and Cellular Biology, Harvard University, Cambridge, MA, United States
- 4Department of Molecular Neuroimaging, Central Institute of Mental Health, Medical Faculty Mannheim, University of Heidelberg, Mannheim, Germany
The potential of psychedelics to persistently treat substance use disorders is known since the 1960s. However, the biological mechanisms responsible for their therapeutic effects have not yet been fully elucidated. While it is known that serotonergic hallucinogens induce changes in gene expression and neuroplasticity, particularly in prefrontal regions, theories on how specifically this counteracts the alterations that occur in neuronal circuitry throughout the course of addiction are largely unknown. This narrative mini-review endeavors to synthesize well-established knowledge from addiction research with findings and theories regarding the neurobiological effects of psychedelics to give an overview of the potential mechanisms that underlie the treatment of substance use disorders with classical hallucinogenic compounds and point out gaps in the current understanding.
Introduction
In 2019, substance use disorders (SUDs) affected an estimated 2.2% of global population and continue to impose a substantial economic burden on society (Peacock et al., 2018; Castaldelli-Maia and Bhugra, 2022). Drug addiction is commonly described as a behavioral disorder featuring cycles of abuse, abstinence, and relapse, as well as negative affective states (Kalivas et al., 2009; Wolf, 2016; Koob, 2022). SUDs result from repeated exposure of a vulnerable brain to reinforcing drugs. This induces various transcriptional and epigenetic changes, altering neural pathways that mediate reward assignment, motivation, and executive control (Nestler, 2001; Lüscher and Malenka, 2011; Ruffle, 2014). The resulting behavioral changes are very stable, potentially persisting over the lifetime of an individual and leading to relapses even after years of abstinence (Spanagel, 2009).
Current pharmacotherapies for SUDs include agonist replacement, reduction of withdrawal symptoms, and inhibition of the rewarding properties of addictive drugs (Volpicelli et al., 1995; Warner and Shoaib, 2005; Lobmaier et al., 2010). However, so far, these approaches are limited to certain drugs or classes of drugs because they target receptors or enzymes specific to particular substances, instead of the underlying pathway modifications shared by all SUDs (Kreek et al., 2002; Nichols et al., 2017).
Recently, serotonergic hallucinogens (here also referred to as psychedelics), such as psilocybin, lysergic acid diethylamide (LSD), or N,N-dimethyltryptamine (DMT), have shown great promise in reducing symptoms of substance addictions and mood disorders, as indicated by a vast body of research and reviews (Dos Santos et al., 2018; Romeo et al., 2021; Bogenschutz et al., 2022; Calleja-Conde et al., 2022; Jones et al., 2022; Mendes et al., 2022; van der Meer et al., 2023). Hypotheses explaining the therapeutic efficacy of these drugs cover psychosocial models, large-scale effects on neural activity and network connectivity, neuroinflammatory mechanisms, as well as molecular/pharmacological actions and are, thus, as multilayered as the phenomenon of addiction (Tófoli and de Araujo, 2016; Koslowski et al., 2022; Teixeira et al., 2022; van Elk and Yaden, 2022). Among these explanations, the induction of structural neuroplasticity is often pointed out as the key mechanism (Mertens and Preller, 2021; Nutt et al., 2023). This mini-review focuses on neuroplastic changes in addiction circuitry and how they potentially lead to alleviation of symptom load.
The addicted brain
As addiction develops, successive adaptations in distinct but interconnected neurocircuits take place while compulsivity of drug intake gradually increases. The affected circuits comprise reward-related pathways, prefrontal control networks, and stress systems (Koob and Volkow, 2010).
Goal-directed behavior is, in part, controlled by dopamine (DA) release from the ventral tegmental area (VTA) into the nucleus accumbens (NAc) and the prefrontal cortex (PFC). This circuit is known as mesocorticolimbic system. Depending on its release site, DA codes for reward, anticipation, or motivation, and mediates associative learning or the activation of goal-directed behavior (Volkow et al., 2007). The reinforcing effects of addictive substances arise from their ability to stimulate DA release in mesolimbic areas, which substantially exceeds that caused by natural rewards, thereby creating strong incentive salience of drug cues (Hyman and Malenka, 2001). With repeated drug intake, epigenetic modifications alter gene expression profiles in mesocorticolimbic areas, leading to homeostatic adaptations. These include changes in synaptic morphology and dysregulation of dopamine receptors (Volkow et al., 2007; Robison and Nestler, 2011; Bidwell et al., 2019). Also, induction of the opioid peptide dynorphin inhibits DA release in the NAc via its action on κ-opioid receptors (Nestler, 2001). As a consequence of these alterations, the responsiveness of the reward system, and thus the motivation to pursue natural rewards, diminishes (Willuhn et al., 2010). Furthermore, in the later stages of addiction, mesocortical DA release initiates drug seeking (Kalivas and Volkow, 2005).
The PFC exerts top-down control over subcortical structures, like the striatum or the amygdala, and by that mediates higher-order cognitive functions, such as selection and coordination of complex behaviors as well as emotional regulation (Jackson and Moghaddam, 2001; Funahashi and Andreau, 2013). As addiction progresses, the ability of the PFC to choose behavioral options that favor long-term positive outcomes is compromised and immediate drug reward is preferred (Dalley et al., 2011). A structural correlate of this dysfunction is gray matter reduction in the PFC, which likely results from loss of dendritic complexity and spine density (Abernathy et al., 2010; DePoy et al., 2014; Mackey et al., 2018). Such atrophies correlate with the duration of drug abuse and impulsivity scores, implying disruption of executive function in the cortex as an underlying mechanism of escalating drug use (Qiu et al., 2013; Becker et al., 2015; Gröpper et al., 2016). Morphological alterations in the PFC change its neurotransmission, importantly leading to hyperactive efferents to the NAc. These corticoaccumbal projections become active upon mesocortical DA release and activate drug seeking (Kalivas and Volkow, 2005). A molecular hallmark of excessive corticoaccumbal transmission is significant down-regulation of metabotropic glutamate receptor subtype 2 (mGluR2) on cortical neurons (Meinhardt et al., 2013, 2021). mGluR2 serves as an inhibiting autoreceptor that modulates glutamatergic transmission (Kalivas et al., 2009). Cortical mGluR2 deficit was shown to be necessary and sufficient for impaired cognitive flexibility and elevated cue-induced drug seeking in ethanol-dependent rats (Meinhardt et al., 2021). Reduced mGlur2 expression was also found in human addicts and implied to play a role in other SUDs besides alcoholism (Jin et al., 2010; Meinhardt et al., 2013; Qian et al., 2019).
Besides that, the emotion and stress systems are crucially involved in the pathogenesis of SUDs (Koob and Schulkin, 2019). Regulation of affective states is achieved through prefrontal top-down control of the dorsal raphe nucleus (DRN) and the amygdala and is compromised in addiction (Vollenweider and Kometer, 2010; Lee et al., 2012; Wilcox et al., 2016). Amygdala and DRN control the activity of the hypothalamic–pituitary–adrenal (HPA) axis by modulating serotonin (5-hydroxytryptamine, 5-HT), corticotropin-releasing factor (CRF), and norepinephrine release in the hypothalamus (Feldman and Weidenfeld, 1998; Lowry, 2002). The HPA-axis mediates endocrine responses to stress and becomes increasingly sensitized during addiction, particularly during phases of abstinence (Koob and Schulkin, 2019). This seems to arise from excessive excitatory input from the extended amygdala, which in turn is attributable to a disruption of inhibitory control mechanisms within this structure (Kallupi et al., 2013; Stamatakis et al., 2014; Sharp, 2017). Consequently, the levels of stress-related neurotransmitters increase and negative affect becomes a driving force behind drug use (Koob, 2013). Stress-induced drug consumption is mediated by projections from the extended amygdala to the VTA and the NAc (Shaham et al., 2000; Kalivas and Volkow, 2005; Rinker et al., 2017). Structural and functional imaging studies support the notion that impaired prefrontal control over emotion and stress systems contributes to defective regulation of behavior (Li and Sinha, 2008; Qiu et al., 2013; Xiao et al., 2015). Additionally, chronic stress itself conduces to deleterious structural alterations and might exacerbate neuronal atrophy, and thus impairments in behavioral regulation, through reduction of dendritic complexity (Ansell et al., 2012; Lu et al., 2021).
To summarize, loss of control over drug consumption results from a series of adaptations in neurocircuits that regulate goal-directed behavior, executive function, and affective states. Abnormalities in all described circuits, resulting from genetic or environmental influences, have been suggested to elevate addiction vulnerability (Goldstein and Volkow, 2011; Shumay et al., 2012; Al’Absi et al., 2021).
Effects of psychedelics – Prefrontal plasticity
Agonism at the 5-HT-2A receptor (5HT2AR) is thought to be the most relevant mechanism of serotonergic hallucinogens for eliciting psychoactive effects and lasting behavioral changes (Benko and Vrankova, 2020; DiVito and Leger, 2020; Pędzich et al., 2022). 5HT2ARs are abundantly expressed at dendrites of glutamatergic PFC pyramidal neurons in layer V, projecting to regions such as the amygdala, VTA, or NAc (Mocci et al., 2014; de Veen et al., 2017; Nichols and Hendricks, 2020). Thus, one common hypothesis regarding the anti-addictive properties of psychedelics focuses on induced neuroplasticity in the PFC leading to functional and structural alterations which may help to recover the phenotype (Ross, 2012; DiVito and Leger, 2020; Peters and Olson, 2021).
A variety of pathways and immediate early genes related to cell growth are upregulated by psychedelics, including brain-derived neurotrophic factor (BDNF) (Olson, 2022). BDNF has been repeatedly suggested to be the key effector in inducing neuroplasticity and thus lasting changes in cognition and behavior (Perkins et al., 2021; Knudsen, 2022; Olson, 2022). This is, for example, underlined by the fact that the anti-depressive effects of the dissociative ketamine, which displays therapeutic effects similar to psychedelics, are blocked by BDNF knockout in mice (Autry et al., 2011). Comparable studies with psychedelics are still missing (Olson, 2022). However, BDNF-driven plasticity seems to be the convergent mechanism of ketamine and serotonergic hallucinogens, hence it is not unlikely that BDNF is equally crucial for the lasting behavioral effects of classical hallucinogens (Aleksandrova and Phillips, 2021). The suggested process behind psychedelic-induced plasticity is initiated by 5HT2AR agonism, leading to the depolarization of prefrontal pyramidal neurons and locally increased glutamate release in the PFC (Muschamp et al., 2004). This drives activation of glutamatergic α-amino-3-hydroxy-5-methyl-4-isoxazole propionic acid (AMPA) receptors and subsequent BNDF secretion (Jourdi et al., 2009). BDNF binds to tropomyosin receptor kinase B (TrkB), resulting in activation of mechanistic target of rapamycin (mTOR), which in turn upregulates BDNF synthesis in dendrites, thereby creating a positive feedback loop that allows for prolonged periods of plasticity (Takei et al., 2004; Ly et al., 2021; Olson, 2022). Such periods are characterized by increases in neurito-, spino-and synaptogenesis, which might counteract neuronal atrophy occurring throughout addiction, and hence improve executive functioning and affective regulation (Ly et al., 2018; Aleksandrova and Phillips, 2021; Raval et al., 2021).
Activity on 5HT2AR-mGluR2 dimers may be crucially important for therapeutic effects of psychedelics (Benko and Vrankova, 2020; Meinhardt et al., 2021). As described above, mGluR2 deficit is an important hallmark of uncontrolled drug consumption, associated with impaired cognitive flexibility and elevated craving in ethanol-dependent rats. Psilocybin administration rescued this behavioral phenotype by restoring mGluR2 expression and presumably rebalancing aberrant corticoaccumbal glutamate transmission (Meinhardt et al., 2021). The persistence of these changes and their relevance in human patients need to be validated in human studies. In addition to corticoaccumbal modulation, prefrontal 5HT2AR stimulation via psychedelics is known to acutely increase activity of serotonergic neurons in the DRN and dopaminergic neurons in the VTA (Puig et al., 2003; Pehek et al., 2006). Long-lasting changes in these projections are yet to be identified but might contribute to regaining control over affective states and impulses, respectively.
Adaptation of 5HT2AR density in prefrontal areas could constitute another important effect of psychedelic action (Vollenweider and Kometer, 2010; Bogenschutz and Johnson, 2016). As elevated 5HT2AR availability in the PFC is associated with mood dysregulation and impulsivity, which are known features of SUDs, normalizing 5HT2AR density could alleviate symptoms of addiction (Frokjaer et al., 2008; Shelton et al., 2009; Rosell et al., 2010). Rapid induction of tolerance, known as tachyphylaxis, is a commonly observed phenomenon with psychedelics and is often accompanied by downregulation of 5HT2AR signaling in cortical areas (Buckholtz et al., 1988; Gresch et al., 2005; Raval et al., 2021). Although this presents regulation of 5HT2AR as a compelling mechanism, 5HT2AR availability returns to baseline a few days after psychedelic intervention in preclinical studies, which speaks against this effect as a mediator of lasting change (Buckholtz et al., 1990; Raval et al., 2021). PET studies in humans could not identify a reliable trend regarding neocortical 5HT2AR availability, although individual-specific relationships between 5HT2AR regulation and therapeutic outcomes were implied by Erritzoe et al. (2011) and Madsen et al. (2020). Further studies are necessary to evaluate the role of 5HT2AR modulation in therapeutic effects of psychedelics and explore alternative mechanisms besides downregulation, such as redistribution within the cell.
Various clinical findings support the idea of functional and structural plasticity in the PFC as a key mechanism of psychedelic therapy. For example, studies on regular users of the DMT-containing plant brew ayahuasca showed enhanced cognitive capabilities related to executive functioning as well as increased cortical thickness in the anterior cingulate cortex, a region that is crucial for affect regulation (Stevens et al., 2011; Bouso et al., 2012, 2015). Causality between the use of psychedelics and cognitive improvements mediated by effects in the cortex is further implied by increases in cognitive flexibility and altered metabolism in the anterior cingulate cortex following psilocybin intervention in depressive patients (Doss et al., 2021). Clinically controlled studies for long-term structural effects of psychedelics are lacking but could generate valuable insights, specifically with a focus on patients suffering from mood or addictive disorders. Higher capacity for emotional regulation, as well as lower levels of anxiety and depressive moods, were found in studies comparing frequent users of psychedelics to controls (Thiessen et al., 2018; Lafrance et al., 2021). In a clinical imaging study, psilocybin reduced reactivity of the amygdala to affective stimuli beyond acute drug effects. This was accompanied by increased activity of prefrontal regions known to control amygdala responses, which implies a potential improvement in top-down control over emotional reactions (Barrett et al., 2020).
Effects of psychedelics – Direct effects on reward and stress systems
5HT2AR is also found in regions of reward and stress systems and directly contributes to regulation of goal-directed behavior and emotional states (de Veen et al., 2017; Nichols and Hendricks, 2020). Therefore, direct stimulation of these circuits might have therapeutic benefits besides top-down effects. One line of thought points toward effects of psychedelics on the mesolimbic DA system (Liester and Prickett, 2012; Ross, 2012; DiVito and Leger, 2020). Acutely, psychedelics elevate mesolimbic DA release, although not to a degree that renders them addictive (Vollenweider et al., 1999; Yan et al., 2000; Ross, 2012). Since persistent low-grade activity on dopamine 2 receptors (D2Rs) caused D2R upregulation in preclinical studies, it has been put forward that psychedelics could normalize D2R deficiency in the NAc by this effect (Boundy et al., 1995; Ross, 2012). Increases in D2R density caused by psychedelics were, indeed, identified in cell membranes from the mouse striatum. This effect was mediated by 5HT2AR, with which D2R forms heterodimers, and potentially caused by allosteric modulation of D2R signaling (Borroto-Escuela et al., 2014, 2021). As in the PFC, 5HT2AR availability in the striatum diminishes significantly after administration of psychedelics (Buckholtz et al., 1985). Since 5HT2AR positively regulates DA release in the mesolimbic pathway and repeated treatment with drugs of abuse seems to increase 5HT2AR sensitivity in the NAc, 5HT2AR downregulation might contribute to rebalancing of aberrant dopaminergic transmission (Pessia et al., 1994; Yan et al., 2000; Alex and Pehek, 2007). The persistence of changes in striatal DA release, D2R, and 5HT2AR density, as well as correlating behavioral alterations, requires further investigation. The same is true for changes in dynorphin levels. However, so far, one preclinical study implies that ayahuasca reduces the effects of ethanol on dynorphin activity (Almeida et al., 2022). Studies regarding psychedelic-induced 5HT2AR downregulation often focus on cortical areas, thus specific investigations in mesolimbic areas and their relation to therapeutic effects are lacking (Hall et al., 2000; Buchborn et al., 2015; Raval et al., 2021).
Furthermore, 5HT2AR is expressed in mood-regulating regions of the brain, such as amygdala or hypothalamus (Shi et al., 2008; Bombardi, 2014). Neuroimaging studies investigating acute effects of psychedelics in the amygdala revealed reduced activity during processing of fearful stimuli (Kraehenmann et al., 2015, 2016; Grimm et al., 2018). While such observations could be caused by top-down mechanisms, preclinical findings show that local stimulation of 5HT2AR in the amygdala is necessary and sufficient to suppress fear expression in rats, implying the presence of direct effects (Grimm et al., 2018; Barrett et al., 2020; Pędzich et al., 2022). Agonism at 5HT2ARs expressed at inhibitory interneurons in the amygdala could readjust their function and normalize hyperactive output to the stress system, like the hypothalamus, and thus re-balance the emotional state (Nichols and Hendricks, 2020). This effect could also decrease signaling to the VTA or the NAc and thereby stress-mediated drug seeking. Direct stimulation of 5HT2AR in the hypothalamus was shown to account for the neuroendocrine response to psychedelics, further supporting the notion that these substances display direct effects in stress-related regions (Hemrick-Luecke and Evans, 2002; Zhang et al., 2002). As use of psychedelics is associated with reduced levels of psychological distress in clinical and population studies, examinations of how this is reflected by long-term alterations in activity of the stress systems (e.g., HPA-axis) would be of interest (Hendricks et al., 2015; Johansen and Krebs, 2015; Doss et al., 2021).
Conclusion
Effects of psychedelics on addiction-related circuitry are diverse and include indirect as well as direct mechanisms in reward, stress, and emotion systems (see Table 1). Prefrontal plasticity supposedly re-establishes impaired top-down regulation of regions like the NAc, the VTA, DRN or the amygdala, which leads to increased control over emotions and impulses, thus reducing cue-and stress-induced drug intake and improving general mood (Vollenweider and Kometer, 2010; Bouso et al., 2015; Aday et al., 2020; see Figure 1). Specifically, rescue of mGluR2 expression was demonstrated to re-balance corticoaccumbal glutamate transmission and reduce craving (Meinhardt et al., 2021; see Figure 1). Direct effects in the limbic system might elevate DA-release and D2R-density, thereby normalizing the function of the reward system (Liester and Prickett, 2012; Ross, 2012; DiVito and Leger, 2020; see Figure 1). Acute effects in stress or emotion systems can partially be attributed to altered top-down regulation, however, local stimulation of the amygdala or the HPA-axis caused behavioral and neuroendocrine effects, respectively, as well (Zhang et al., 2002; Barrett et al., 2020; Pędzich et al., 2022). It is thus still unclear which proportion of the effects in subcortical structures are the consequence of top-down modifications and which part is caused via local action.
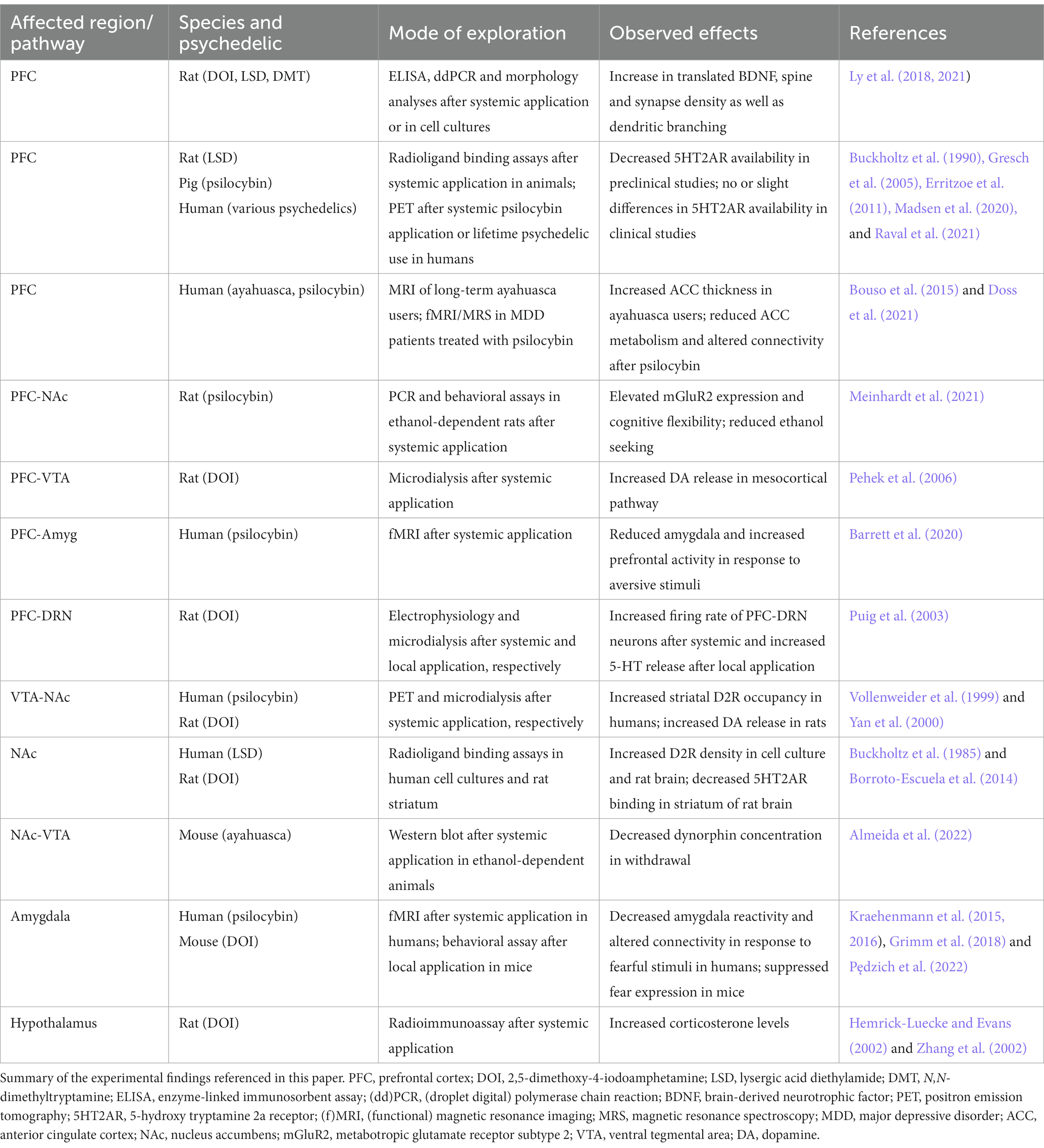
Table 1. Experimental evidence for psychedelic effects in key regions and pathways in the addicted brain.
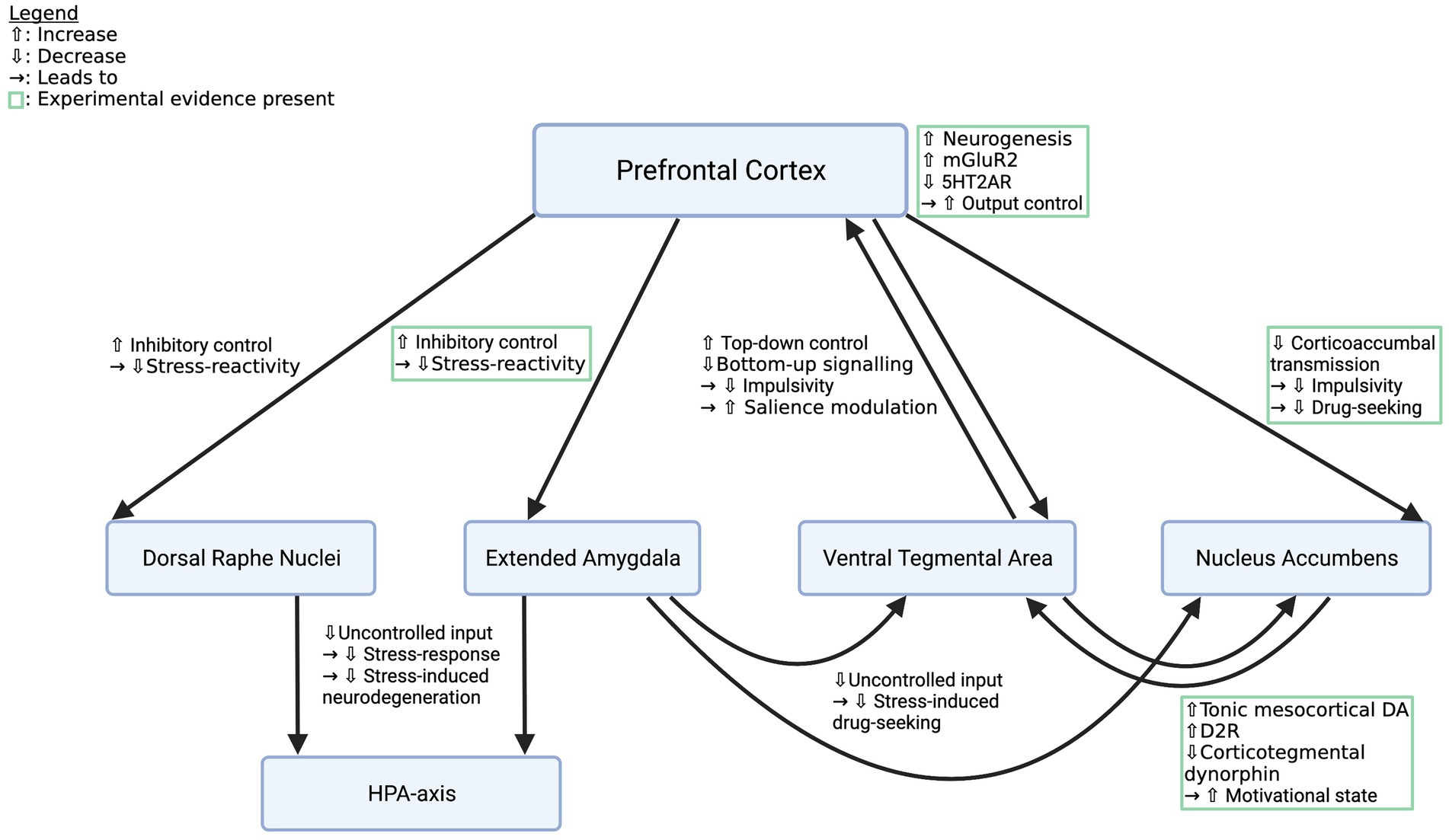
Figure 1. Effects of psychedelics on key pathways in the addicted brain. Depicted are crucial pathways that contribute to the behavioral and affective symptoms of SUDs and descriptions of how psychedelics supposedly alter their function to restore a healthy phenotype. Mechanisms listed in green boxes are backed up by experimental evidence, the other ones are deduced from knowledge about addiction circuitry and the effects of psychedelics. However, all pathways deserve closer examination. mGluR2, metabotropic glutamate receptor subtype 2; 5HT2AR, 5-hydroxy tryptamine 2a receptor; HPA-axis, hypothalamic–pituitary–adrenal axis. Created with BioRender.com.
Studies employing local administration of psychedelics to or local blocking of 5HT2AR in important emotion-and reward-hubs in combination with animal models of addiction could shed light on the role of bottom-up mechanisms in subcortical structures. Furthermore, studies elucidating top-down effects on addiction circuitry are needed. These could include investigation of synaptic plasticity in corticolimbic or corticostriatal projections, examination of local transmitter release in response to different stimuli (e.g., fear-provoking or drug cues) pre versus post-psychedelics, and correlating structural changes with behavior. Most studies so far focus on acute or short-term effects of serotonergic hallucinogens and the field could benefit from (pre)clinical studies that systematically investigate long-term alterations in the key pathways outlined in this paper (see Figure 1). Despite the existing gaps, the current state of knowledge implies that psychedelics induce profound changes in cognition and emotional processing which are accompanied by circuit modifications that foster improvement of SUDs in general and challenge the efficacy of currently available addiction pharmacotherapy (Fuentes et al., 2020).
Author contributions
MU collected the studies, wrote, and edited the manuscript, including creation of the figure and the table. MS and MM contributed to shaping the manuscript and editing it. All authors read and agreed upon the final manuscript.
Funding
Financial support for this work was provided by Bundesministerium für Bildung und Forschung (BMBF) funded ERA-NET Psi-Alc (FKZ: 01EW1908), Deutsche Forschungsgemeinschaft (DFG, German Research Foundation) Project-ID: ME 5279/3-1 and the publication funding program ‘Open Access Publikationskosten’ by the University of Heidelberg and DFG.
Conflict of interest
The authors declare that the research was conducted in the absence of any commercial or financial relationships that could be construed as a potential conflict of interest.
Publisher’s note
All claims expressed in this article are solely those of the authors and do not necessarily represent those of their affiliated organizations, or those of the publisher, the editors and the reviewers. Any product that may be evaluated in this article, or claim that may be made by its manufacturer, is not guaranteed or endorsed by the publisher.
References
Abernathy, K., Chandler, L. J., and Woodward, J. J. (2010). Alcohol and the prefrontal cortex. Int Rev Neurobiol 91, 289–320. doi: 10.1016/S0074-7742(10)91009-X
Aday, J. S., Bloesch, E. K., and Davoli, C. C. (2020). Can psychedelic drugs attenuate age-related changes in cognition and affect? J Cogn Enhance 4, 219–227. doi: 10.1007/s41465-019-00151-6
Al’Absi, M., Ginty, A. T., and Lovallo, W. R. (2021). Neurobiological mechanisms of early life adversity, blunted stress reactivity and risk for addiction. Neuropharmacology 188:108519. doi: 10.1016/j.neuropharm.2021.108519
Aleksandrova, L. R., and Phillips, A. G. (2021). Neuroplasticity as a convergent mechanism of ketamine and classical psychedelics. Trends Pharmacol. Sci. 42, 929–942. doi: 10.1016/j.tips.2021.08.003
Alex, K. D., and Pehek, E. A. (2007). Pharmacologic mechanisms of serotonergic regulation of dopamine neurotransmission. Pharmacol. Ther. 113, 296–320. doi: 10.1016/j.pharmthera.2006.08.004
Almeida, C. A. F., Pereira-Junior, A. A., Rangel, J. G., Pereira, B. P., Costa, K. C. M., Bruno, V., et al. (2022). Ayahuasca, a psychedelic beverage, modulates neuroplasticity induced by ethanol in mice. Behav. Brain Res. 416:113546. doi: 10.1016/j.bbr.2021.113546
Ansell, E. B., Rando, K., Tuit, K., Guarnaccia, J., and Sinha, R. (2012). Cumulative adversity and smaller gray matter volume in medial prefrontal, anterior cingulate, and insula regions. Biol. Psychiatry 72, 57–64. doi: 10.1016/j.biopsych.2011.11.022
Autry, A. E., Adachi, M., Nosyreva, E., Na, E. S., Los, M. F., Cheng, P., et al. (2011). NMDA receptor blockade at rest triggers rapid behavioural antidepressant responses. Nature, 475, 91–95. doi: 10.1038/nature10130
Barrett, F. S., Doss, M. K., Sepeda, N. D., Pekar, J. J., and Griffiths, R. R. (2020). Emotions and brain function are altered up to one month after a single high dose of psilocybin. Sci. Rep. 10:2214. doi: 10.1038/s41598-020-59282-y
Becker, B., Wagner, D., Koester, P., Tittgemeyer, M., Mercer-Chalmers-Bender, K., Hurlemann, R., et al. (2015). Smaller amygdala and medial prefrontal cortex predict escalating stimulant use. Brain 138, 2074–2086. doi: 10.1093/brain/awv113
Benko, J., and Vranková, S. (2020). Natural psychoplastogens as antidepressant agents. Molecules 25:1172. doi: 10.3390/molecules25051172
Bidwell, L. C., Karoly, H. C., Thayer, R. E., Claus, E. D., Bryan, A. D., Weiland, B. J., et al. (2019). DRD2 promoter methylation and measures of alcohol reward: functional activation of reward circuits and clinical severity. Addict. Biol. 24, 539–548. doi: 10.1111/adb.12614
Bogenschutz, M. P., and Johnson, M. W. (2016). Classic hallucinogens in the treatment of addictions. Prog. Neuro-Psychopharmacol. Biol. Psychiatry 64, 250–258. doi: 10.1016/j.pnpbp.2015.03.002
Bogenschutz, M. P., Ross, S., Bhatt, S., Baron, T., Forcehimes, A. A., Laska, E., et al. (2022). Percentage of heavy drinking days following psilocybin-assisted psychotherapy vs placebo in the treatment of adult patients with alcohol use disorder: a randomized clinical trial. JAMA Psychiat. 79, 953–962. doi: 10.1001/jamapsychiatry.2022.2096
Bombardi, C. (2014). Neuronal localization of the 5-HT2 receptor family in the amygdaloid complex. Front. Pharmacol. 5, 1–10. doi: 10.3389/fphar.2014.00068
Borroto-Escuela, D. O., Ambrogini, P., Narvaez, M., Di Liberto, V., Beggiato, S., Ferraro, L., et al. (2021). Serotonin heteroreceptor complexes and their integration of signals in neurons and Astroglia—relevance for mental diseases. Cells 10:1902. doi: 10.3390/cells10081902
Borroto-Escuela, D. O., Romero-Fernandez, W., Narvaez, M., Oflijan, J., Agnati, L. F., and Fuxe, K. (2014). Hallucinogenic 5-HT2AR agonists LSD and DOI enhance dopamine D2R protomer recognition and signaling of D2-5-HT2A heteroreceptor complexes. Biochem. Biophys. Res. Commun. 443, 278–284. doi: 10.1016/j.bbrc.2013.11.104
Boundy, V. A., Pacheco, M. A., Guan, W., and Molinoff, P. B. (1995). Agonists and antagonists differentially regulate the high affinity state of the D2L receptor in human embryonic kidney 293 cells. Mol. Pharmacol. 48, 956–964.
Bouso, J. C., González, D., Fondevila, S., Cutchet, M., Fernández, X., Ribeiro Barbosa, P. C., et al. (2012). Personality, psychopathology, life attitudes and neuropsychological performance among ritual users of Ayahuasca: a longitudinal study. PLoS One 7:e42421. doi: 10.1371/journal.pone.0042421
Bouso, J. C., Palhano-Fontes, F., Rodríguez-Fornells, A., Ribeiro, S., Sanches, R., Crippa, J. A. S., et al. (2015). Long-term use of psychedelic drugs is associated with differences in brain structure and personality in humans. Eur. Neuropsychopharmacol. 25, 483–492. doi: 10.1016/j.euroneuro.2015.01.008
Buchborn, T., Schröder, H., Dieterich, D. C., Grecksch, G., and Höllt, V. (2015). Tolerance to LSD and DOB induced shaking behaviour: differential adaptations of frontocortical 5-HT(2A) and glutamate receptor binding sites. Behav. Brain Res. 281, 62–68. doi: 10.1016/j.bbr.2014.12.014
Buckholtz, N. S., Freedman, D. X., and Middaugh, L. D. (1985). Daily LSD administration selectively decreases serotonin2 receptor binding in rat brain. Eur. J. Pharmacol. 109, 421–425. doi: 10.1016/0014-2999(85)90407-8
Buckholtz, N. S., Zhou, D. F., and Freedman, D. X. (1988). Serotonin2 agonist administration down-regulates rat brain serotonin2 receptors. Life Sci. 42, 2439–2445. doi: 10.1016/0024-3205(88)90342-6
Buckholtz, N. S., Zhou, D. F., Freedman, D. X., and Potter, W. Z. (1990). Lysergic acid diethylamide (LSD) administration selectively downregulates serotonin2 receptors in rat brain. Neuropsychopharmacology 3, 137–148.
Calleja-Conde, J., Morales-García, J. A., Echeverry-Alzate, V., Bühler, K. M., Giné, E., and López-Moreno, J. A. (2022). Classic psychedelics and alcohol use disorders: a systematic review of human and animal studies. Addict. Biol. 27:e13229. doi: 10.1111/adb.13229
Castaldelli-Maia, J. M., and Bhugra, D. (2022). Analysis of global prevalence of mental and substance use disorders within countries: focus on sociodemographic characteristics and income levels. Int. Rev. Psychiatry (Abingdon, England) 34, 6–15. doi: 10.1080/09540261.2022.2040450
Dalley, J. W., Everitt, B. J., and Robbins, T. W. (2011). Impulsivity, compulsivity, and top-down cognitive control. Neuron 69, 680–694. doi: 10.1016/j.neuron.2011.01.020
DePoy, L. M., Perszyk, R. E., Zimmermann, K. S., Koleske, A. J., and Gourley, S. L. (2014). Adolescent cocaine exposure simplifies orbitofrontal cortical dendritic arbors. Front. Pharmacol. 5:228. doi: 10.3389/fphar.2014.00228
de Veen, B. T. H., Schellekens, A. F. A., Verheij, M. M. M., and Homberg, J. R. (2017). Psilocybin for treating substance use disorders?. Expert Rev. Neurother. 17, 203–212. doi: 10.1080/14737175.2016.122083
DiVito, A. J., and Leger, R. F. (2020). Psychedelics as an emerging novel intervention in the treatment of substance use disorder: a review. Mol. Biol. Rep. 47, 9791–9799. doi: 10.1007/s11033-020-06009-x
Dos Santos, R. G., Bouso, J. C., Alcázar-Córcoles, M. Á., and Hallak, J. E. C. (2018). Efficacy, tolerability, and safety of serotonergic psychedelics for the management of mood, anxiety, and substance-use disorders: a systematic review of systematic reviews. Expert. Rev. Clin. Pharmacol. 11, 889–902. doi: 10.1080/17512433.2018.1511424
Doss, M. K., Považan, M., Rosenberg, M. D., Sepeda, N. D., Davis, A. K., Finan, P. H., et al. (2021). Psilocybin therapy increases cognitive and neural flexibility in patients with major depressive disorder. Transl. Psychiatry 11:574. doi: 10.1038/s41398-021-01706-y
Erritzoe, D., Frokjaer, V. G., Holst, K. K., Christoffersen, M., Johansen, S. S., Svarer, C., et al. (2011). In vivo imaging of cerebral serotonin transporter and Serotonin2A receptor binding in 3,4-Methylenedioxymethamphetamine (MDMA or “ecstasy”) and hallucinogen users. Arch. Gen. Psychiatry 68, 562–576. doi: 10.1001/archgenpsychiatry.2011.56
Feldman, S., and Weidenfeld, J. (1998). The excitatory effects of the amygdala on hypothalamo-pituitary-adrenocortical responses are mediated by hypothalamic norepinephrine, serotonin, and CRF-41. Brain Res. Bull. 45, 389–393. doi: 10.1016/s0361-9230(97)00384-5
Frokjaer, V. G., Mortensen, E. L., Nielsen, F. Å., Haugbol, S., Pinborg, L. H., Adams, K. H., et al. (2008). Frontolimbic serotonin 2A receptor binding in healthy subjects is associated with personality risk factors for affective disorder. Biol. Psychiatry 63, 569–576. doi: 10.1016/j.biopsych.2007.07.009
Fuentes, J. J., Fonseca, F., Elices, M., Farré, M., and Torrens, M. (2020). Therapeutic use of LSD in psychiatry: a systematic review of randomized-controlled clinical trials. Front. Psych. 10:943. doi: 10.3389/fpsyt.2019.00943
Funahashi, S., and Andreau, J. M. (2013). Prefrontal cortex and neural mechanisms of executive function. J. Physiol. Paris 107, 471–482. doi: 10.1016/j.jphysparis.2013.05.001
Goldstein, R. Z., and Volkow, N. D. (2011). Dysfunction of the prefrontal cortex in addiction: neuroimaging findings and clinical implications. Nat. Rev. Neurosci. 12, 652–669. doi: 10.1038/nrn3119
Gresch, P. J., Smith, R. L., Barrett, R. J., and Sanders-Bush, E. (2005). Behavioral tolerance to lysergic acid diethylamide is associated with reduced serotonin-2A receptor signaling in rat cortex. Neuropsychopharmacology 30, 1693–1702. doi: 10.1038/sj.npp.1300711
Grimm, O., Kraehenmann, R., Preller, K. H., Seifritz, E., and Vollenweider, F. X. (2018). Psilocybin modulates functional connectivity of the amygdala during emotional face discrimination. Eur. Neuropsychopharmacol. 28, 691–700. doi: 10.1016/j.euroneuro.2018.03.016
Gröpper, S., Spengler, S., Stuke, H., Gawron, C. K., Parnack, J., Gutwinski, S., et al. (2016). Behavioral impulsivity mediates the relationship between decreased frontal gray matter volume and harmful alcohol drinking: a voxel-based morphometry study. J. Psychiatr. Res. 83, 16–23. doi: 10.1016/j.jpsychires.2016.08.006
Hall, H., Farde, L., Halldin, C., Lundkvist, C., and Sedvall, G. (2000) Autoradiographic localization of 5-HT(2A) receptors in the human brain using [(3)H]M100907 and [(11)C]M100907, Synapse New York, NY, 38, 421–431. doi: 10.1002/1098-2396(20001215)38:4<421::AID-SYN7>3.0.CO;2-X
Hemrick-Luecke, S. K., and Evans, D. C. (2002). Comparison of the potency of MDL 100,907 and SB 242084 in blocking the serotonin (5-HT)2 receptor agonist-induced increases in rat serum corticosterone concentrations: evidence for 5-HT2A receptor mediation of the HPA axis. Neuropharmacology 42, 162–169. doi: 10.1016/S0028-3908(01)00166-6
Hendricks, P. S., Thorne, C. B., Clark, C. B., Coombs, D. W., and Johnson, M. W. (2015). Classic psychedelic use is associated with reduced psychological distress and suicidality in the United States adult population. J Psychopharmacol 29, 280–288. doi: 10.1177/0269881114565653
Hyman, S. E., and Malenka, R. C. (2001). Addiction and the brain: the neurobiology of compulsion and its persistence. Nat. Rev. Neurosci. 2, 695–703. doi: 10.1038/35094560
Jackson, M. E., and Moghaddam, B. (2001). Amygdala regulation of nucleus Accumbens dopamine output is governed by the prefrontal cortex. J. Neurosci. 21, 676–681. doi: 10.1523/JNEUROSCI.21-02-00676.2001
Jin, X., Semenova, S., Yang, L., Ardecky, R., Sheffler, D. J., Dahl, R., et al. (2010). The mGluR2 positive allosteric modulator BINA decreases cocaine self-administration and cue-induced cocaine-seeking and counteracts cocaine-induced enhancement of brain reward function in rats. Neuropsychopharmacology 35, 2021–2036. doi: 10.1038/npp.2010.82
Johansen, P.-Ø., and Krebs, T. S. (2015). Psychedelics not linked to mental health problems or suicidal behavior: a population study. J. Psychopharmacol. (Oxford, England) 29, 270–279. doi: 10.1177/0269881114568039
Jones, G., Ricard, J. A., Lipson, J., and Nock, M. K. (2022). Associations between classic psychedelics and opioid use disorder in a nationally-representative U.S. adult sample. Sci. Rep. 12:4099. doi: 10.1038/s41598-022-08085-4
Jourdi, H., Hsu, Y., Zhou, M., Qin, Q., Bi, X., and Baudry, M. (2009). Positive AMPA receptor modulation rapidly stimulates BDNF release and increases dendritic mRNA translation. J. Neurosci. 27, 8688–8697. doi: 10.1523/JNEUROSCI.6078-08.2009
Kalivas, P. W., Lalumiere, R. T., Knackstedt, L., and Shen, H. (2009). Glutamate transmission in addiction. Neuropharmacology 56, 169–173. doi: 10.1016/j.neuropharm.2008.07.011
Kalivas, P. W., and Volkow, N. D. (2005). The neural basis of addiction: a pathology of motivation and choice. Am. J. Psychiatry 162, 1403–1413. doi: 10.1176/appi.ajp.162.8.1403
Kallupi, M., Wee, S., Edwards, S., Whitfield, T. W., Oleata, C. S., Luu, G., et al. (2013). Kappa opioid receptor-mediated dysregulation of gamma-aminobutyric Acidergic transmission in the central amygdala in cocaine addiction. Biol. Psychiatry 74, 520–528. doi: 10.1016/j.biopsych.2013.04.028
Knudsen, G. M. (2022). Sustained effects of single doses of classical psychedelics in humans. Neuropsychopharmacology 48, 145–150. doi: 10.1038/s41386-022-01361-x
Koob, G. F. (2013). Addiction is a reward deficit and stress surfeit disorder. Front. Psych. 4:72. doi: 10.3389/fpsyt.2013.00072
Koob, G. F. (2022). “Anhedonia, Hyperkatifeia, and negative reinforcement in substance use disorders” in Anhedonia: preclinical, translational, and clinical integration. ed. D. A. Pizzagalli (Cham: Springer International Publishing Current Topics in Behavioral Neurosciences), 147–165. doi: 10.1007/7854_2021_288
Koob, G. F., and Schulkin, J. (2019). Addiction and stress: an allostatic view. Neurosci. Biobehav. Rev. 106, 245–262. doi: 10.1016/j.neubiorev.2018.09.008
Koob, G. F., and Volkow, N. D. (2010). Neurocircuitry of addiction. Neuropsychopharmacology 35, 217–238. doi: 10.1038/npp.2009.110
Koslowski, M., Johnson, M. W., Gründer, G., and Betzler, F. (2022). Novel treatment approaches for substance use disorders: therapeutic use of psychedelics and the role of psychotherapy. Curr. Addict. Rep. 9, 48–58. doi: 10.1007/s40429-021-00401-8
Kraehenmann, R., Preller, K. H., Scheidegger, M., Pokorny, T., Bosch, O. G., Seifritz, E., et al. (2015). Psilocybin-induced decrease in amygdala reactivity correlates with enhanced positive mood in healthy volunteers. Biol. Psychiatry 78, 572–581. doi: 10.1016/j.biopsych.2014.04.010
Kraehenmann, R., Schmidt, A., Friston, K., Preller, K. H., Seifritz, E., and Vollenweider, F. X. (2016). The mixed serotonin receptor agonist psilocybin reduces threat-induced modulation of amygdala connectivity. NeuroImage 11, 53–60. doi: 10.1016/j.nicl.2015.08.009
Kreek, M. J., LaForge, K. S., and Butelman, E. (2002). Pharmacotherapy of addictions. Nat. Rev. Drug Discov. 1, 710–726. doi: 10.1038/nrd897
Lafrance, A., Strahan, E., Bird, B. M., St. Pierre, M., and Walsh, Z. (2021). Classic psychedelic use and mechanisms of mental health: exploring the mediating roles of spirituality and emotion processing on symptoms of anxiety, depressed mood, and disordered eating in a community sample. J. Humanist. Psychol. doi: 10.1177/00221678211048049
Lee, H., Heller, A. S., van Reekum, C. M., Nelson, B., and Davidson, R. J. (2012). Amygdala-prefrontal coupling underlies individual differences in emotion regulation. NeuroImage 62, 1575–1581. doi: 10.1016/j.neuroimage.2012.05.044
Li, C. R., and Sinha, R. (2008). Inhibitory control and emotional stress regulation: neuroimaging evidence for frontal–limbic dysfunction in psycho-stimulant addiction. Neurosci. Biobehav. Rev. 32, 581–597. doi: 10.1016/j.neubiorev.2007.10.003
Liester, M. B., and Prickett, J. I. (2012). Hypotheses regarding the mechanisms of ayahuasca in the treatment of addictions. J. Psychoactive Drugs 44, 200–208. doi: 10.1080/02791072.2012.704590
Lobmaier, P., Gossop, M., Waal, H., and Bramness, J. (2010). The pharmacological treatment of opioid addiction--a clinical perspective. Eur. J. Clin. Pharmacol. 66, 537–545. doi: 10.1007/s00228-010-0793-6
Lowry, C. A. (2002). Functional subsets of serotonergic neurones: implications for control of the hypothalamic-pituitary-adrenal axis. J. Neuroendocrinol. 14, 911–923. doi: 10.1046/j.1365-2826.2002.00861.x
Lu, J., Tjia, M., Mullen, B., Cao, B., Lukasiewicz, K., Shah-Morales, S., et al. (2021). An analog of psychedelics restores functional neural circuits disrupted by unpredictable stress. Mol. Psychiatry 26, 6237–6252. doi: 10.1038/s41380-021-01159-1
Lüscher, C., and Malenka, R. C. (2011). Drug-evoked synaptic plasticity in addiction: from molecular changes to circuit remodeling. Neuron 69, 650–663. doi: 10.1016/j.neuron.2011.01.017
Ly, C., Greb, A. C., Cameron, L. P., Wong, J. M., Barragan, E. V., Wilson, P. C., et al. (2018). Psychedelics promote structural and functional neural plasticity. Cell Rep. 23, 3170–3182. doi: 10.1016/j.celrep.2018.05.022
Ly, C., Greb, A. C., Vargas, M. V., Duim, W. C., Grodzki, A. C. G., Lein, P. J., et al. (2021). Transient stimulation with psychoplastogens is sufficient to initiate neuronal growth. ACS Pharmacol Transl Sci 4, 452–460. doi: 10.1021/acsptsci.0c00065
Mackey, S., Allgaier, N., Chaarani, B., Spechler, P., Orr, C. J. B., Bunn, J., et al. (2018). Mega-analysis of gray matter volume in substance dependence: general and substance-specific regional effects. Am. J. Psychiatry 176, 119–128. doi: 10.1176/appi.ajp.2018.17040415
Madsen, M. K., Fisher, P. M., Stenbæk, D. S., Kristiansen, S., Burmester, D., Lehel, S., et al. (2020). A single psilocybin dose is associated with long-term increased mindfulness, preceded by a proportional change in neocortical 5-HT2A receptor binding. Eur. Neuropsychopharmacol. 33, 71–80. doi: 10.1016/j.euroneuro.2020.02.001
Meinhardt, M. W., Hansson, A. C., Perreau-Lenz, S., Bauder-Wenz, C., Stählin, O., Heilig, M., et al. (2013). Rescue of Infralimbic mGluR2 deficit restores control over drug-seeking behavior in alcohol dependence. J. Neurosci. 33, 2794–2806. doi: 10.1523/JNEUROSCI.4062-12.2013
Meinhardt, M. W., Pfarr, S., Fouquet, G., Rohleder, C., Meinhardt, M. L., Barroso-Flores, J., et al. (2021). Psilocybin targets a common molecular mechanism for cognitive impairment and increased craving in alcoholism. Sci. Adv. 7:eabh2399. doi: 10.1126/sciadv.abh2399
Mendes, F. R., Costa, C. D. S., Wiltenburg, V. D., Morales-Lima, G., Fernandes, J. A. B., and Filev, R. (2022). Classic and non-classic psychedelics for substance use disorder: a review of their historic, past and current research. Addict Neurosci 3:100025. doi: 10.1016/j.addicn.2022.100025
Mertens, L. J., and Preller, K. H. (2021). Classical psychedelics as therapeutics in psychiatry - current clinical evidence and potential therapeutic mechanisms in substance use and mood disorders. Pharmacopsychiatry 54, 176–190. doi: 10.1055/a-1341-1907
Mocci, G., Jiménez-Sánchez, L., Adell, A., Cortés, R., and Artigas, F. (2014) Expression of 5-HT2A receptors in prefrontal cortex pyramidal neurons projecting to nucleus accumbens. Potential relevance for atypical antipsychotic action. Neuropharmacology, 79, 49–58. doi: 10.1016/j.neuropharm.2013.10.021
Muschamp, J. W., Regina, M. J., Hull, E. M., Winter, J. C., and Rabin, R. A. (2004). Lysergic acid diethylamide and [−]-2,5-dimethoxy-4-methylamphetamine increase extracellular glutamate in rat prefrontal cortex. Brain Res. 1023, 134–140. doi: 10.1016/j.brainres.2004.07.044
Nestler, E. J. (2001). Molecular neurobiology of addiction. Am. J. Addict. 10, 201–217. doi: 10.1080/105504901750532094
Nichols, C. D., and Hendricks, P. S. (2020). “Chapter 49 – classic psychedelics as therapeutics for psychiatric disorders” in Handbook of behavioral neuroscience. eds. C. P. Müller and K. A. Cunningham (Elsevier: Handbook of the Behavioral Neurobiology of Serotonin), 959–966. doi: 10.1016/B978-0-444-64125-0.00049-9
Nichols, D. E., Johnson, M. W., and Nichols, C. D. (2017). Psychedelics as medicines: an emerging New paradigm. Clin. Pharmacol. Ther. 101, 209–219. doi: 10.1002/cpt.557
Nutt, D., Spriggs, M., and Erritzoe, D. (2023). Psychedelics therapeutics: what we know, what we think, and what we need to research. Neuropharmacology 223:109257. doi: 10.1016/j.neuropharm.2022.109257
Olson, D. E. (2022). Biochemical mechanisms underlying psychedelic-induced neuroplasticity. Biochemistry 61, 127–136. doi: 10.1021/acs.biochem.1c00812
Peacock, A., Leung, J., Larney, S., Colledge, S., Hickman, M., Rehm, J., et al. (2018). Global statistics on alcohol, tobacco and illicit drug use: 2017 status report. Addiction 113, 1905–1926. doi: 10.1111/add.14234
Pędzich, B. D., Rubens, S., Sekssaoui, M., Pierre, A., Van Schuerbeek, A., Marin, P., et al. (2022). Effects of a psychedelic 5-HT2A receptor agonist on anxiety-related behavior and fear processing in mice. Neuropsychopharmacology 47, 1304–1314. doi: 10.1038/s41386-022-01324-2
Pehek, E. A., Nocjar, C., Roth, B. L., Byrd, T. A., and Mabrouk, O. S. (2006). Evidence for the preferential involvement of 5-HT2A serotonin receptors in stress-and drug-induced dopamine release in the rat medial prefrontal cortex. Neuropsychopharmacology 31, 265–277. doi: 10.1038/sj.npp.1300819
Perkins, D., Sarris, J., Rossell, S., Bonomo, Y., Forbes, D., Davey, C., et al. (2021). Medicinal psychedelics for mental health and addiction: advancing research of an emerging paradigm. Aust. N. Z. J. Psychiatry 55, 1127–1133. doi: 10.1177/0004867421998785
Pessia, M., Jiang, Z. G., North, R. A., and Johnson, S. W. (1994). Actions of 5-hydroxytryptamine on ventral tegmental area neurons of the rat in vitro. Brain Res. 654, 324–330. doi: 10.1016/0006-8993(94)90495-2
Peters, J., and Olson, D. E. (2021). Engineering Safer Psychedelics for Treating Addiction. Neurosci. Insights, 16, doi: 10.1177/26331055211033847
Puig, M. V., Celada, P., Díaz-Mataix, L., and Artigas, F. (2003). In vivo modulation of the activity of pyramidal neurons in the rat medial prefrontal cortex by 5-HT2A receptors: relationship to thalamocortical afferents. Cereb Cortex (1991 13, 870–882. doi: 10.1093/cercor/13.8.870
Qian, Z., Wu, X., Qiao, Y., Shi, M., Liu, Z., Ren, W., et al. (2019). Downregulation of mGluR2/3 receptors during morphine withdrawal in rats impairs mGluR2/3-and NMDA receptor-dependent long-term depression in the nucleus accumbens. Neurosci. Lett. 690, 76–82. doi: 10.1016/j.neulet.2018.10.018
Qiu, Y., Jiang, G., Su, H., Lv, X., Tian, J., Li, L., et al. (2013). The impulsivity behavior is correlated with prefrontal cortex gray matter volume reduction in heroin-dependent individuals. Neurosci. Lett. 538, 43–48. doi: 10.1016/j.neulet.2013.01.019
Raval, N. R., Johansen, A., Donovan, L. L., Ros, N. F., Ozenne, B., Hansen, H. D., et al. (2021). A single dose of psilocybin increases synaptic density and decreases 5-HT2A receptor density in the pig brain. Int. J. Mol. Sci. 22:E835. doi: 10.3390/ijms22020835
Rinker, J. A., Marshall, S. A., Mazzone, C. M., Lowery-Gionta, E. G., Gulati, V., Pleil, K. E., et al. (2017). Extended amygdala to ventral tegmental area Corticotropin-releasing factor circuit controls binge ethanol intake. Biol. Psychiatry 81, 930–940. doi: 10.1016/j.biopsych.2016.02.029
Robison, A. J., and Nestler, E. J. (2011). Transcriptional and epigenetic mechanisms of addiction. Nat. Rev. Neurosci. 12, 623–637. doi: 10.1038/nrn3111
Romeo, B., Hermand, M., Pétillion, A., Karila, L., and Benyamina, A. (2021). Clinical and biological predictors of psychedelic response in the treatment of psychiatric and addictive disorders: a systematic review. J. Psychiatr. Res. 137, 273–282. doi: 10.1016/j.jpsychires.2021.03.002
Rosell, D. R., Thompson, J. L., Slifstein, M., Xu, X., Frankle, W. G., New, A. S., et al. (2010). Increased serotonin 2A receptor availability in the orbitofrontal cortex of physically aggressive personality disordered patients. Biol. Psychiatry 67, 1154–1162. doi: 10.1016/j.biopsych.2010.03.013
Ross, S. (2012). Serotonergic hallucinogens and emerging targets for addiction pharmacotherapies. Psychiatr. Clin. North Am. 35, 357–374. doi: 10.1016/j.psc.2012.04.002
Ruffle, J. K. (2014). Molecular neurobiology of addiction: what’s all the (Δ)FosB about? Am. J. Drug Alcohol Abuse 40, 428–437. doi: 10.3109/00952990.2014.933840
Shaham, Y., Erb, S., and Stewart, J. (2000). Stress-induced relapse to heroin and cocaine seeking in rats: a review. Brain Res. Brain Res. Rev. 33, 13–33. doi: 10.1016/s0165-0173(00)00024-2
Sharp, B. M. (2017). Basolateral amygdala and stress-induced hyperexcitability affect motivated behaviors and addiction. Transl. Psychiatry 7:e1194. doi: 10.1038/tp.2017.161
Shelton, R. C., Sanders-Bush, E., Manier, D. H., and Lewis, D. A. (2009). Elevated 5-HT 2A receptors in postmortem prefrontal cortex in major depression is associated with reduced activity of protein kinase A. Neuroscience 158, 1406–1415. doi: 10.1016/j.neuroscience.2008.11.036
Shi, J., Landry, M., Carrasco, G. A., Battaglia, G., and Muma, N. A. (2008). Sustained treatment with a 5-HT2A receptor agonist causes functional desensitization and reductions in agonist-labeled 5-HT2A receptors despite increases in receptor protein levels in rats. Neuropharmacology 55, 687–692. doi: 10.1016/j.neuropharm.2008.06.001
Shumay, E., Fowler, J. S., Wang, G.-J., Logan, J., Alia-Klein, N., Goldstein, R. Z., et al. (2012). Repeat variation in the human PER2 gene as a new genetic marker associated with cocaine addiction and brain dopamine D2 receptor availability. Transl. Psychiatry 2:e86. doi: 10.1038/tp.2012.11
Spanagel, R. (2009). Alcoholism: a systems approach from molecular physiology to addictive behavior. Physiol. Rev. 89, 649–705. doi: 10.1152/physrev.00013.2008
Stamatakis, A. M., Sparta, D. R., Jennings, J. H., McElligott, Z. A., Decot, H., and Stuber, G. D. (2014). Amygdala and bed nucleus of the stria terminalis circuitry: implications for addiction-related behaviors. Neuropharmacology 76, 320–328. doi: 10.1016/j.neuropharm.2013.05.046
Stevens, F. L., Hurley, R. A., and Taber, K. H. (2011). Anterior cingulate cortex: unique role in cognition and emotion. J. Neuropsychiatry Clin. Neurosci. 23, 121–125. doi: 10.1176/jnp.23.2.jnp121
Takei, N., Inamura, N., Kawamura, M., Namba, H., Hara, K., Yonezawa, K., et al. (2004). Brain-derived neurotrophic factor induces mammalian target of rapamycin-dependent local activation of translation machinery and protein synthesis in neuronal dendrites. J. Neurosci. Res. 24, 9760–9769. doi: 10.1523/JNEUROSCI.1427-04.2004
Teixeira, P. J., Johnson, M. W., Timmermann, C., Watts, R., Erritzoe, D., Douglass, H., et al. (2022). Psychedelics and health behaviour change. J. Psychopharmacol. (Oxford, England) 36, 12–19. doi: 10.1177/02698811211008554
Thiessen, M. S., Walsh, Z., Bird, B. M., and Lafrance, A. (2018). Psychedelic use and intimate partner violence: The role of emotion regulation J. Psychopharmacol. 32, 749–755. doi: 10.1177/0269881118771782
Tófoli, L. F., and de Araujo, D. B. (2016). “Chapter seven – treating addiction: perspectives from EEG and imaging studies on psychedelics” in International review of neurobiology. eds. N. M. Zahr and E. T. Peterson (Academic Press: Imaging the Addicted Brain), 157–185. doi: 10.1016/bs.irn.2016.06.005
van der Meer, P. B., Fuentes, J. J., Kaptein, A. A., Schoones, J. W., de Waal, M. M., Goudriaan, A. E., et al. (2023). Therapeutic effect of psilocybin in addiction: a systematic review. Front. Psych. 14:1134454. doi: 10.3389/fpsyt.2023.1134454
van Elk, M., and Yaden, D. B. (2022). Pharmacological, neural, and psychological mechanisms underlying psychedelics: a critical review. Neurosci. Biobehav. Rev. 140:104793. doi: 10.1016/j.neubiorev.2022.104793
Volkow, N. D., Fowler, J. S., Wang, G.-J., Swanson, J. M., and Telang, F. (2007). Dopamine in drug abuse and addiction: results of imaging studies and treatment implications. Arch. Neurol. 64, 1575–1579. doi: 10.1001/archneur.64.11.1575
Vollenweider, F. X., and Kometer, M. (2010). The neurobiology of psychedelic drugs: implications for the treatment of mood disorders. Nat. Rev. Neurosci. 11, 642–651. doi: 10.1038/nrn2884
Vollenweider, F. X., Vontobel, P., Hell, D., and Leenders, K. L. (1999). 5-HT modulation of dopamine release in basal ganglia in psilocybin-induced psychosis in man--a PET study with [11C]raclopride. Neuropsychopharmacol 20, 424–433. doi: 10.1016/S0893-133X(98)00108-0
Volpicelli, J. R., Watson, N. T., King, A. C., Sherman, C. E., and O’Brien, C. P. (1995). Effect of naltrexone on alcohol high in alcoholics. Am. J. Psychiatry 152, 613–615. doi: 10.1176/ajp.152.4.613
Warner, C., and Shoaib, M. (2005). How does bupropion work as a smoking cessation aid? Addict. Biol. 10, 219–231. doi: 10.1080/13556210500222670
Wilcox, C. E., Pommy, J. M., and Adinoff, B. (2016). Neural circuitry of impaired emotion regulation in substance use disorders. Am. J. Psychiatr. 173, 344–361. doi: 10.1176/appi.ajp.2015.15060710
Willuhn, I., Wanat, M. J., Clark, J. J., and Phillips, P. E. M. (2010). Dopamine signaling in the nucleus accumbens of animals self-administering drugs of abuse. Curr. Top. Behav. Neurosci. 3, 29–71. doi: 10.1007/7854_2009_27
Wolf, M. E. (2016). Synaptic mechanisms underlying persistent cocaine craving. Nat. Rev. Neurosci. 17, 351–365. doi: 10.1038/nrn.2016.39
Xiao, P., Dai, Z., Zhong, J., Zhu, Y., Shi, H., and Pan, P. (2015). Regional gray matter deficits in alcohol dependence: a meta-analysis of voxel-based morphometry studies. Drug Alcohol Depend. 153, 22–28. doi: 10.1016/j.drugalcdep.2015.05.030
Yan, Q., Reith, M. E., and Yan, S. (2000). Enhanced accumbal dopamine release following 5-HT(2A) receptor stimulation in rats pretreated with intermittent cocaine. Brain Res. 863, 254–258. doi: 10.1016/s0006-8993(00)02080-1
Keywords: psychedelics, addiction, psilocybin, hallucinogen, substance, dependency, plasticity, therapy
Citation: Urban MM, Stingl MR and Meinhardt MW (2023) Mini-review: The neurobiology of treating substance use disorders with classical psychedelics. Front. Neurosci. 17:1156319. doi: 10.3389/fnins.2023.1156319
Edited by:
Erik B. Oleson, University of Colorado Denver, United StatesReviewed by:
Faizul Azam, Qassim University, Saudi ArabiaCopyright © 2023 Urban, Stingl and Meinhardt. This is an open-access article distributed under the terms of the Creative Commons Attribution License (CC BY). The use, distribution or reproduction in other forums is permitted, provided the original author(s) and the copyright owner(s) are credited and that the original publication in this journal is cited, in accordance with accepted academic practice. No use, distribution or reproduction is permitted which does not comply with these terms.
*Correspondence: Marvin M. Urban, bWFydmluLnVyYmFuQHppLW1hbm5oZWltLmRl