- 1Department of Neurological Surgery, University of Miami Miller School of Medicine, Miami, FL, United States
- 2The Miami Project to Cure Paralysis, Miami, FL, United States
- 3Department of Neurological Surgery, Washington University School of Medicine, St. Louis, MO, United States
Introduction: Peripheral nerve injuries are common neurologic injuries that are challenging to treat with current therapies. Electrical stimulation has been shown to accelerate reinnervation and enhance functional recovery. This study aims to review the literature on clinical application of electrical stimulation for peripheral nerve injury.
Methods: PubMed and Embase were sourced from 1995 to August 2022. Selection was based on predetermined inclusion/exclusion criteria. Eight hundred and thirty-five articles were screened with seven being included in this review.
Results: Two hundred and twenty-nine patients with peripheral nerve injuries were represented. Six of the studies were randomized controlled trials. A variety of nerve injuries were represented with all being in the upper extremity and supraclavicular region. Electrical stimulation protocols and evaluation varied. Electrodes were implanted in four studies with one also implanting the stimulator. Length of stimulation per session was either 20 mins or 1 h. Median stimulation frequency was 20 Hz. Stimulation intensity varied from 3 to 30V; pulse width ranged from 0.1 to 1.007 ms. Three protocols were conducted immediately after surgery. Patients were followed for an average of 13.5 months and were evaluated using electrophysiology and combinations of motor, sensory, and functional criteria.
Discussion: Patients who received electrical stimulation consistently demonstrated better recovery compared to their respective controls. Electrical stimulation for peripheral nerve injury is a novel treatment that has not been well-studied in humans. Our review illustrates the potential benefit in implementing this approach into everyday practice. Future research should aim to optimize protocol for clinical use.
Introduction
Peripheral nerve injuries are common neurologic injures and are challenging to treat adequately with current surgical techniques. Although peripheral nerve axons have the potential to regenerate, functional recovery after nerves injuries remains challenging particularly when longer distances are required for motor and sensory reinnervation. One method of interest to aid in regeneration is the use of electrical stimulation (ES), which has been shown to both accelerate reinnervation and enhance functional recovery (Gordon, 2016; Chu et al., 2022). Prior research has demonstrated that ES may address the complex pathophysiology involved in the inhibition of synaptic stripping and the excessive excitability of the dorsal root ganglion, while alleviating neuropathic pain, improving neurologic function, and accelerating nerve regeneration (Chu et al., 2022). Investigation of ES for peripheral nerve injury began in the mid-twentieth century when Hoffman (1952) demonstrated accelerated axon sprouting at various frequencies. Following this initial study, ES has been shown to be most promising when applied immediately following nerve injury with brief stimulation for 1 h at 20 Hz (Al-Majed et al., 2000a; Brushart et al., 2005; Ahlborn et al., 2007; Lal et al., 2008; Sharma et al., 2010; Singh et al., 2012; Witzel et al., 2016). In addition to peripheral nerve regeneration, ES has also been investigated for treatment of a variety of neurologic injuries or chronic conditions, including spinal injuries, traumatic brain injuries, and neuropathic pain (Peri et al., 2001; Johnson and Burchiel, 2004; Cheing and Luk, 2005; Jarrett et al., 2005; Shields and Dudley-Javoroski, 2006; Oosterhof et al., 2008; Deer et al., 2010; Lee et al., 2012; Lairamore et al., 2014; Oo, 2014; Chen et al., 2015; Gall et al., 2016; Wu et al., 2017; Redshaw et al., 2018; Stampas et al., 2019; Liechti et al., 2020; Johnson et al., 2021; Kamboonlert et al., 2021). Although a relatively new therapy, ES has been demonstrated to be a safe and effective adjunctive therapy with established treatments including surgical repair, pharmacologic treatment, and cell-based therapies (Chu et al., 2022).
The preclinical data supporting electrical stimulation following peripheral nerve injury is robust and shows an overwhelming support for its use. After peripheral nerve injury, nerve growth factor (NGF) production in Schwann cells declines, limiting neural repair (Huang et al., 2010a). However, animal studies have shown that ES may be able to counteract this through stimulating dorsal root ganglions and Schwann cells to increase production of cyclic adenosine monophosphate (cAMP) and NGF respectively (Udina et al., 2008; Huang et al., 2010b). Because cAMP counteracts myelin inhibition and allows axon regeneration, increased levels after ES is proposed to enhance neurite outgrowth and extension (Aglah et al., 2008). ES stimulates peripheral nerve regeneration-associated genes within the cell body, namely Talpha1-tubulin and growth associated protein 43 (GAP-43) expression, while also reducing medium-molecular-weight neurofilament mRNA (Al-Majed et al., 2004; Chu et al., 2022). This change in the neurofilament/tubulin expression ratio is thought to allow more tubulin to be transported at a faster rate there by accelerating elongation (Al-Majed et al., 2004). Brief electrical stimulation also stimulates brain derived neurotrophic factor (BDNF) and its receptor, tyrosine receptor kinase B (trkB), expression in regenerating motoneurons which is believed to promote axonal regeneration through an autocrine and/or paracrine function (Al-Majed et al., 2000b; English et al., 2007).
Further, it has been noted in the literature that ES proximal to the site of peripheral nerve injury leads to therapeutic benefits (Chu et al., 2022). In one study, researchers examined continuous 20 Hz ES applied at variable durations (1 h to 2 weeks) in a rat, femoral nerve transection model to assess motor axonal regeneration (Al-Majed et al., 2000a). Although it was established that the processes associated with preferential motor reinnervation take 10 weeks to occur, ES dramatically reduced this period to 3 weeks, demonstrating its ability to substantially augment recovery (Al-Majed et al., 2000a). English and colleagues have also shown convincing data demonstrating the benefits of ES when applied to a sciatic injury model (English et al., 2007). In their study, they were able to show accelerated axonal growth in common fibular nerves that had been subjected to ES proximally compared to those that had received sham ES (English et al., 2007). Taken together, these studies and several others on the subject demonstrate the clear efficacy of ES in animal models with recovery after injury.
While there is extremely promising data on ES in animal models (Al-Majed et al., 2000b; Geremia et al., 2007; Gordon et al., 2009; Xu et al., 2014; Elzinga et al., 2015; Willand et al., 2016; Shapira et al., 2019), the applicability of this approach to humans is still under investigation. Relatively few clinical trials have been completed in human populations leaving optimal protocol, including electrode arrangements, and application methods unknown. Regarding the frequency and duration of ES, some hypothesize that any stimulation beyond the initial aforementioned 1-h brief stimulation may be dose dependent (Javeed et al., 2021).
For ES to be implemented in a clinical setting, a multifaceted approach targeting the different aspects of regeneration is essential. ES in conjunction with other rehabilitation methods, such as exercise, must be investigated before confidently transitioning to clinical application (Javeed et al., 2021). In this systematic review, we examine the current literature surrounding clinical application of electrical stimulation for peripheral nerve injuries to consolidate current findings and provide next steps for implementation into everyday practice.
Methods
Following PRISMA guidelines, PubMed and Embase were sourced for clinical trials between 1995 and August 2022 (Page et al., 2021). The search terms (peripheral nerve stimulation) AND (nerve injury) were used to retrieve articles of relevance. Inclusion criteria included clinical application on human subjects, sustainment of a peripheral nerve injury, and specification of nerve injured. Additionally, included studies must have outlined stimulation protocol and evaluation criteria used during follow-up. Case reports, studies involving central nervous system injuries, and studies for neuropathic pain control were excluded. Two reviewers independently screened articles for inclusion to reduce the risk of bias. One reviewer extracted data which was later verified by a second reviewer to ensure accuracy and also reduce bias. Given the small number of relevant studies, a meta-analysis was foregone (Cheung and Vijayakumar, 2016). Study type, size, design, and results were collected from each of the included studies. Additionally, stimulation protocols and evaluation criteria were also collected.
Results
Eight hundred thirty-five articles were initially generated and were screened using predetermined inclusion and exclusion criteria. A total of seven studies ultimately met the review criteria and were included. Figure 1 outlines the process of study selection. A significant number of sourced studies were excluded from analysis; however, the majority were excluded because they investigated ES for purposes outside the scope of this study. Between the seven included studies, a total of 229 patients with peripheral nerve injuries were represented. All included studies were a variation of a randomized controlled trial except for the study by Williams (1996) which was a pilot trial. A variety of nerve injuries were studied with all being in the upper extremity or supraclavicular region. Four studies included injuries from chronic compression of the median or ulnar nerves, one study involved injury following surgical repair for digital transection, and one study investigated injury after surgical retraction and devascularization of the spinal accessory nerve. Williams (1996) included a variety of upper extremity nerve injuries and did not report mechanism of injury. An overview of the clinical trials is outlined Table 1 (Williams, 1996; Naeser et al., 2002; Gordon et al., 2010; Koca et al., 2014; Wong et al., 2015; Barber et al., 2018; Power et al., 2020).
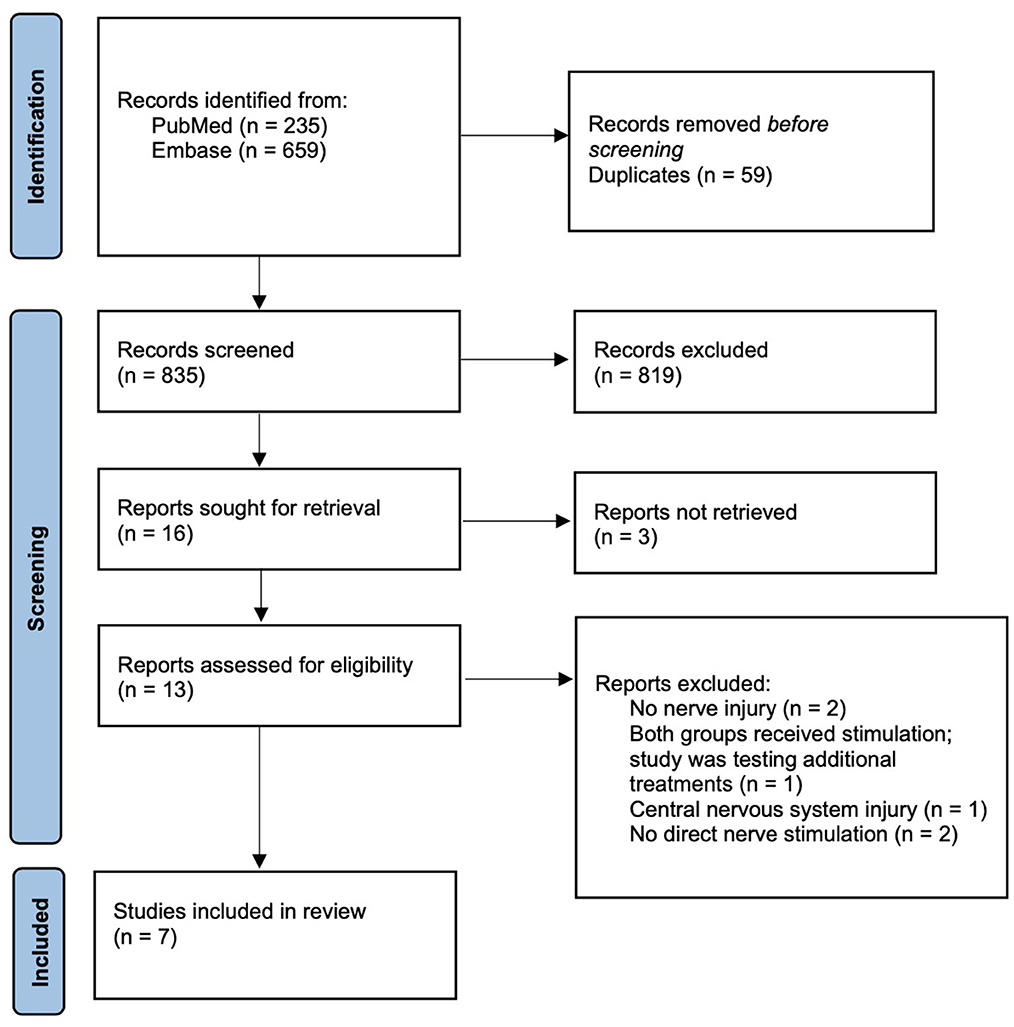
Figure 1. PRISMA diagram outlining study selection process (Page et al., 2021).
Electrical stimulation protocols and evaluation criteria were compared from all studies. Although protocol specifics varied, similar components were demonstrated throughout. The majority of protocols (n = 5) used bipolar stimulation. Electrodes were implanted in four studies with one also implanting the electrical stimulator. Four studies included only one session of stimulation and two studies explored the use of multiple stimulation sessions over the course of a few weeks. Williams (1996) explored the use of continuous stimulation to denervated muscle until nerve regeneration was determined to be complete upon assessment. The length of stimulation per session was either 20 mins or 1 h (excluding). The median stimulation frequency for six of the studies was 20 Hz (range 20–130 Hz). Naeser et al. (2002) explored the use of multiple frequencies during their protocol. Stimulation intensity varied from 3 to 30 V with most studies reporting a range used. Stimulation pulse width was also reported and ranged from 0.1 to 1.007 ms. Three protocols were conducted immediately after surgery, either in the operating room or in the post-anesthesia care unit. Three were conducted in a laboratory setting with Gordon et al. (2010) utilizing local anesthesia (1% lidocaine) at the site of surgical incision (Williams, 1996). While lidocaine is a voltage-gated sodium channel blocker, which could prevent action potential propagation, when injected into the epidermis it should have very little effect on ES. Given that Williams (1996) implemented continuous stimulation for months at a time using implantable stimulators, patients were able to complete their daily activities while receiving electrical stimulation. Differences in electrode placement can necessitate adjustment to stimulation parameters. Tables 1–3 outline the differences in the included studies that require variations in protocol. Across all studies, patients were followed for an average of 13.5 months and were evaluated using electrophysiology as well as combinations of motor, sensory, and functional criteria. Tables 2, 3 detail the electrical stimulation protocols and evaluation components for each study (Williams, 1996; Naeser et al., 2002; Gordon et al., 2010; Koca et al., 2014; Wong et al., 2015; Barber et al., 2018; Power et al., 2020).
Patients who received electrical stimulation consistently demonstrated better recovery when compared to their respective controls. Power et al. (2020) found that after three years of follow-up, electrically stimulated patients had more than double the number of motor units and higher McGown-Goldberg grades than the control group. Barber et al. (2018) demonstrated significantly higher functional recovery among patients receiving electrical stimulation. Similarly, Wong et al. (2015) also demonstrated improved functional recovery in addition to a greater sensory recovery than the respective control group. Like Gordon et al. (2010) also demonstrated a significant increase in motor units as well as improved axonal regeneration, terminal motor latency, and earlier sensory nerve conduction values. Significant improvements in sensation and symptoms of nerve injury were also demonstrated by Naeser et al. (2002). Although Williams (1996) did not have a control for comparison, all patients demonstrated satisfactory to excellent recovery based on evaluation components.
Discussion
Electrical stimulation for peripheral nerve injury is a relatively new approach to treatment that has not been well studied in human populations. Our review highlights the potential value and utility of adopting this treatment modality into clinical practice. Each of the studies included in this review conclude that ES may aid in nerve recovery and function. Patients receiving ES outperformed their control counterparts in motor, sensory, and/or functional recovery. To our knowledge, this is the first comprehensive systematic review of clinical trials investigating electrical stimulation for peripheral nerve injuries. Despite common themes being present throughout the ES protocols highlighted in this review, variations in type of stimulation, duration, frequency, intensity, pulse width, along with sex and timing of surgical nerve repair are difficult to account for given the relative scarcity of literature. Although an ample amount of research has been done using animal models, human clinical trials are needed to further elucidate their impacts on patient outcomes. Further investigation into the applicability, usefulness, and feasibility of this approach in human populations is needed.
While all studies were grouped together for the purposes of this review, there are differences worth mentioning among the studies across various factors. While one of the seven included studies was a transection injury, four of the others were chronic compression injuries. The remaining two studies included one study that involved a surgical retraction and devascularization and another study that did not report the type of injury. Overall, all the included studies examined injured nerves from the upper extremity; however, one study involved the spinal accessory nerve, one involved the digital nerves, one involved the ulnar nerve, three examined the median nerve, and the final study examined a combination of median and ulnar nerves. Although all included studies utilized ES, the protocol for ES treatment was different across the seven studies. For instance, two studies that involved surgical intervention utilized ES in two different ways, with one of the studies using ES during the repair and the other study using ES after the surgery had taken place. Interestingly, even though these and other differences were present among the seven studies, outcomes were generally the same. Specifically, those that were treated with ES showed improvement compared to those that were treated without ES, helping to demonstrate the efficacy of ES as a potential treatment after peripheral nerve injury (for overview of studies, see Table 1).
Although there are few studies pertaining to ES for peripheral nerve injury, this treatment modality has the potential to substantially change the management of these injuries. There has been no other pharmacological or interventional option that has provided the robust improvement in clinical outcomes like ES. As demonstrated by this review, ES has been shown to aid in functional recovery of peripheral nerve injuries. Significantly, this functional recovery was seen across a variety of peripheral nerves located in the upper extremities with similar success; however, it would be pertinent to assess the ability of ES to aid in functional recovery after lower extremity injury. The next step in advancing this treatment is further application in the clinical setting to possibly generate more data supporting its use in injuries beyond the scope of this review. By utilizing the work already put forward (Viv et al., 2008; Gordon et al., 2010; Wong et al., 2015; Gordon and English, 2016; Barber et al., 2018; Power et al., 2020) as a guide, future research into clinical outcomes may change current guidelines for addressing these types of injuries.
In this review, we summarized the available literature surrounding clinical applications of electrical stimulation for peripheral nerve injuries. Using the seven included studies, we were able to review the implementation of this treatment on a relatively large patient population. Our review also included studies that investigated implementation for multiple peripheral nerves and mechanisms of injury. The studies included had adequate follow-up time, allowing evaluation of nerve recovery over an average of more than one year. This, coupled with the ability to assess nerve injuries using multiple methods, provided a thorough and adequate assessment of the utility of this approach.
Use of electrical stimulation for the treatment of peripheral nerve injures is a relatively new approach to care that has not yet been well studied. However, despite the scarcity of literature detailing its use, initial reports appear promising and highlight the benefit of this approach to potentially enhance and accelerate functional recovery. Importantly, based on these studies, the advantages and disadvantages of both bipolar and monopolar ES should be considered prior to their use. Studies have shown that there are unique differences between the two electrode configurations, including differences in threshold amplitude, relative gain, and selectivity (Grandjean and Mortimer, 1986). Specifically, although the threshold amplitude is lower with monopolar configurations, the relative gain is decreased in bipolar configurations (Grandjean and Mortimer, 1986). While preclinical and clinical models have utilized monopolar ES with much success, others have argued that bipolar ES is preferred as it allows for the cathode and anode to be placed directly on the nerve, thereby leading to better focused treatment (López, 2010). Clinicians should consider both options of stimulation prior to selection. Further, before wide-scale implementation into clinical settings, future research is needed and should be aimed to resolve such translational concerns.
Limitations
This study is not without limitations. Given the relatively small number of relevant studies, a meta-analysis was foregone (Cheung and Vijayakumar, 2016; Muka et al., 2020). Without this analysis, definitive conclusions could not be drawn regarding clinical trial variations. However, systematic reviews, even those not including a meta-analysis, are vital for linking research to practice (Cook et al., 1997; Mulrow et al., 1997). Additionally, many of the measures used, such as MUNE, are only currently available in the laboratory setting limiting their use in the clinical environment. Cost effectiveness and technical limitations also impede ES adaptation.
Conclusions
Peripheral nerve injuries are common, but complete recovery is hard to achieve with current practices. Electrical stimulation for nerve injuries is a relatively new therapy that shows promising outcomes when used in adjunct with known treatment options. This review demonstrates that patients who received electrical stimulation consistently experienced better recovery when compared to their respective controls. Guidelines for use of electrical stimulation for peripheral nerve injury have not been established. Future work should focus on determining how to best incorporate this treatment for a variety of peripheral nerve injuries into the clinical environment.
Data availability statement
The original contributions presented in the study are included in the article/supplementary material, further inquiries can be directed to the corresponding author.
Author contributions
MC, EE, and SB contributed to idea conception and study design. MC organized the database. MC wrote the manuscript with assistance of TS and EE. SB, AL, and WR provided edits and assisted in manuscript revision. All authors have read and approved the submitted version.
Conflict of interest
The authors declare that the research was conducted in the absence of any commercial or financial relationships that could be construed as a potential conflict of interest.
Publisher's note
All claims expressed in this article are solely those of the authors and do not necessarily represent those of their affiliated organizations, or those of the publisher, the editors and the reviewers. Any product that may be evaluated in this article, or claim that may be made by its manufacturer, is not guaranteed or endorsed by the publisher.
References
Aglah, C., Gordon, T., and Posse de Chaves, E. I. (2008). cAMP promotes neurite outgrowth and extension through protein kinase A but independently of Erk activation in cultured rat motoneurons. Neuropharmacology. 55, 8–17. doi: 10.1016/j.neuropharm.2008.04.005
Ahlborn, P., Schachner, M., and Irintchev, A. (2007). 1 h electrical stimulation accelerates functional recovery after femoral nerve repair. Exp. Neurol. 208, 137–144. doi: 10.1016/j.expneurol.2007.08.005
Al-Majed, A. A., Brushart, T. M., and Gordon, T. (2000b). Electrical stimulation accelerates and increases expression of BDNF and trkB mRNA in regenerating rat femoral motoneurons. Eur. J. Neurosci. 12, 4381–4390. doi: 10.1046/j.1460-9568.2000.01341.x
Al-Majed, A. A., Neumann, C. M., Brushart, T. M., and Gordon, T. (2000a). Brief electrical stimulation promotes the speed and accuracy of motor axonal regeneration. J. Neurosci. 20, 2602–2608. doi: 10.1523/JNEUROSCI.20-07-02602.2000
Al-Majed, A. A., Tam, S. L., and Gordon, T. (2004). Electrical stimulation accelerates and enhances expression of regeneration-associated genes in regenerating rat femoral motoneurons. Cell Mol. Neurobiol. 24, 379–402. doi: 10.1023/B:CEMN.0000022770.66463.f7
Barber, B., Seikaly, H., Ming Chan, K., Beaudry, R., Rychlik, S., Olson, J., et al. (2018). Intraoperative Brief Electrical Stimulation of the Spinal Accessory Nerve (BEST SPIN) for prevention of shoulder dysfunction after oncologic neck dissection: a double-blinded, randomized controlled trial. J. Otolaryngol. Head Neck Surg. 47, 7. doi: 10.1186/s40463-017-0244-9
Brushart, T. M., Jari, R., Verge, V., Rohde, C., and Gordon, T. (2005). Electrical stimulation restores the specificity of sensory axon regeneration. Exp. Neurol. 194, 221–229. doi: 10.1016/j.expneurol.2005.02.007
Cheing, G. L., and Luk, M. L. (2005). Transcutaneous electrical nerve stimulation for neuropathic pain. J. Hand Surg. Br. 30, 50–55. doi: 10.1016/J.JHSB.2004.08.007
Chen, G., Liao, L., and Li, Y. (2015). The possible role of percutaneous tibial nerve stimulation using adhesive skin surface electrodes in patients with neurogenic detrusor overactivity secondary to spinal cord injury. Int. Urol. Nephrol. 47, 451–455. doi: 10.1007/s11255-015-0911-6
Cheung, M. W., and Vijayakumar, R. (2016). A guide to conducting a meta-analysis. Neuropsychol. Rev. 26, 121–128. doi: 10.1007/s11065-016-9319-z
Chu, X. L., Song, X. Z., Li, Q., Li, Y. R., He, F., Gu, X. S., et al. (2022). Basic mechanisms of peripheral nerve injury and treatment via electrical stimulation. Neural. Regen. Res. 17, 2185–2193. doi: 10.4103/1673-5374.335823
Cook, D. J., Mulrow, C. D., and Haynes, R. B. (1997). Systematic reviews: synthesis of best evidence for clinical decisions. Ann. Intern. Med. 126, 376–380. doi: 10.7326/0003-4819-126-5-199703010-00006
Deer, T. R., Levy, R. M., and Rosenfeld, E. L. (2010). Prospective clinical study of a new implantable peripheral nerve stimulation device to treat chronic pain. Clin. J. Pain. 26, 359–372. doi: 10.1097/AJP.0b013e3181d4d646
Elzinga, K., Tyreman, N., Ladak, A., Savaryn, B., Olson, J., and Gordon, T. (2015). Brief electrical stimulation improves nerve regeneration after delayed repair in Sprague Dawley rats. Exp. Neurol. 269, 142–153. doi: 10.1016/j.expneurol.2015.03.022
English, A. W., Schwartz, G., Meador, W., Sabatier, M. J., and Mulligan, A. (2007). Electrical stimulation promotes peripheral axon regeneration by enhanced neuronal neurotrophin signaling. Dev. Neurobiol. 67, 158–172. doi: 10.1002/dneu.20339
Gall, C., Schmidt, S., Schittkowski, M. P., Antal, A., Ambrus, G. G., Paulus, W., et al. (2016). Alternating current stimulation for vision restoration after optic nerve damage: a randomized clinical trial. PLoS One. 11, e0156134. doi: 10.1371/journal.pone.0156134
Geremia, N. M., Gordon, T., Brushart, T. M., Al-Majed, A. A., and Verge, V. M. (2007). Electrical stimulation promotes sensory neuron regeneration and growth-associated gene expression. Exp. Neurol. 205, 347–359. doi: 10.1016/j.expneurol.2007.01.040
Gordon, T. (2016). Electrical stimulation to enhance axon regeneration after peripheral nerve injuries in animal models and humans. Neurotherapeutics. 13, 295–310. doi: 10.1007/s13311-015-0415-1
Gordon, T., Amirjani, N., Edwards, D. C., and Chan, K. M. (2010). Brief post-surgical electrical stimulation accelerates axon regeneration and muscle reinnervation without affecting the functional measures in carpal tunnel syndrome patients. Exp. Neurol. 223, 192–202. doi: 10.1016/j.expneurol.2009.09.020
Gordon, T., Chan, K. M., Sulaiman, O. A., Udina, E., Amirjani, N., and Brushart, T. M. (2009). Accelerating axon growth to overcome limitations in functional recovery after peripheral nerve injury. Neurosurgery. 65, A132–A144. doi: 10.1227/01.NEU.0000335650.09473.D3
Gordon, T., and English, A. W. (2016). Strategies to promote peripheral nerve regeneration: electrical stimulation and/or exercise. Eur. J. Neurosci. 43, 336–350. doi: 10.1111/ejn.13005
Grandjean, P. A., and Mortimer, J. T. (1986). Recruitment properties of monopolar and bipolar epimysial electrodes. Ann. Biomed. Eng. 14, 53–66. doi: 10.1007/BF02364648
Hoffman, H. (1952). Acceleration and retardation of the process of axon-sprouting in partially devervated muscles. Aust. J. Exp. Biol. Med. Sci. 30, 541–566. doi: 10.1038/icb.1952.52
Huang, J., Lu, L., Hu, X., Ye, Z., Peng, Y., Yan, X., et al. (2010b). Electrical stimulation accelerates motor functional recovery in the rat model of 15-mm sciatic nerve gap bridged by scaffolds with longitudinally oriented microchannels. Neurorehabil. Neural Repair. 24, 736–745. doi: 10.1177/1545968310368686
Huang, J., Ye, Z., Hu, X., Lu, L., and Luo, Z. (2010a). Electrical stimulation induces calcium-dependent release of NGF from cultured Schwann cells. Glia. 58, 622–631. doi: 10.1002/glia.20951
Jarrett, M. E., Matzel, K. E., Christiansen, J., Baeten, C. G., Rosen, H., Bittorf, B., et al. (2005). Sacral nerve stimulation for faecal incontinence in patients with previous partial spinal injury including disc prolapse. Br. J. Surg. 92, 734–739. doi: 10.1002/bjs.4859
Javeed, F., Rehman, L., Afzal, A., and Abbas, A. (2021). Outcome of diffuse axonal injury in moderate and severe traumatic brain injury. Surg. Neurol. Int. 12, 384. doi: 10.25259/SNI_573_2020
Johnson, M. D., and Burchiel, K. J. (2004). Peripheral stimulation for treatment of trigeminal postherpetic neuralgia and trigeminal posttraumatic neuropathic pain: a pilot study. Neurosurgery. 55, 135-41. doi: 10.1227/01.NEU.0000126874.08468.89
Johnson, S., Marshall, A., Hughes, D., Holmes, E., Henrich, F., Nurmikko, T., et al. (2021). Mechanistically informed non-invasive peripheral nerve stimulation for peripheral neuropathic pain: a randomised double-blind sham-controlled trial. J. Transl. Med. 19, 458. doi: 10.1186/s12967-021-03128-2
Kamboonlert, K., Panyasriwanit, S., Tantisiriwat, N., and Kitisomprayoonkul, W. (2021). Effects of bilateral transcutaneous tibial nerve stimulation on neurogenic detrusor overactivity in spinal cord injury: a urodynamic study. Arch. Phys. Med. Rehabil. 102, 1165–1169. doi: 10.1016/j.apmr.2020.10.130
Koca, I., Boyaci, A., Tutoglu, A., Ucar, M., and Kocaturk, O. (2014). Assessment of the effectiveness of interferential current therapy and TENS in the management of carpal tunnel syndrome: a randomized controlled study. Rheumatol. Int. 34, 1639–1645. doi: 10.1007/s00296-014-3005-3
Lairamore, C. I., Garrison, M. K., Bourgeon, L., and Mennemeier, M. (2014). Effects of functional electrical stimulation on gait recovery post-neurological injury during inpatient rehabilitation. Percept. Mot. Skills. 119, 591–608. doi: 10.2466/15.25.PMS.119c19z5
Lal, D., Hetzler, L. T., Sharma, N., Wurster, R. D., Marzo, S. J., Jones, K. J., et al. (2008). Electrical stimulation facilitates rat facial nerve recovery from a crush injury. Otolaryngol. Head Neck. Surg. 139, 68–73. doi: 10.1016/j.otohns.2008.04.030
Lee, Y. H., Kim, S. H., Kim, J. M., Im, H. T., Choi, I. S., and Lee, K. W. (2012). The effect of semiconditional dorsal penile nerve electrical stimulation on capacity and compliance of the bladder with deformity in spinal cord injury patients: a pilot study. Spinal Cord. 50, 289–293. doi: 10.1038/sc.2011.141
Liechti, M. D., van der Lely, S., Stalder, S. A., Anderson, C. E., Birkhäuser, V., Bachmann, L. M., et al. (2020). Update from TASCI, a nationwide, randomized, sham-controlled, double-blind clinical trial on transcutaneous tibial nerve stimulation in patients with acute spinal cord injury to prevent neurogenic detrusor overactivity. Eur. Urol. Focus. 6, 877–879. doi: 10.1016/j.euf.2019.09.019
López, J. R. (2010). “Peripheral nerve monitoring:: basics and indications,” in Intraoperative Neurophysiologic Monitoring, eds Galloway GM, Lopez JR, Zamel KM, Nuwer MR (Cambridge: Cambridge University Press), p. 142–162.
Muka, T., Glisic, M., Milic, J., Verhoog, S., Bohlius, J., Bramer, W., et al. (2020). A 24-step guide on how to design, conduct, and successfully publish a systematic review and meta-analysis in medical research. Euro. J. Epidemiol. 35, 49–60. doi: 10.1007/s10654-019-00576-5
Mulrow, C. D., Cook, D. J., and Davidoff, F. (1997). Systematic reviews: critical links in the great chain of evidence. Ann. Intern. Med. 126, 389–391. doi: 10.7326/0003-4819-126-5-199703010-00008
Naeser, M. A., Hahn, K. A., Lieberman, B. E., and Branco, K. F. (2002). Carpal tunnel syndrome pain treated with low-level laser and microamperes transcutaneous electric nerve stimulation: a controlled study. Arch. Phys. Med. Rehabil. 83, 978–988. doi: 10.1053/apmr.2002.33096
Oo, W. M. (2014). Efficacy of addition of transcutaneous electrical nerve stimulation to standardized physical therapy in subacute spinal spasticity: a randomized controlled trial. Arch. Phys. Med. Rehabil. 95, 2013–2020. doi: 10.1016/j.apmr.2014.06.001
Oosterhof, J., Samwel, H. J., de Boo, T. M., Wilder-Smith, O. H., Oostendorp, R. A., and Crul, B. J. (2008). Predicting outcome of TENS in chronic pain: a prospective, randomized, placebo controlled trial. Pain. 136, 11–20. doi: 10.1016/j.pain.2007.06.009
Page, M. J., Moher, D., Bossuyt, P. M., Boutron, I., Hoffmann, T. C., Mulrow, C. D., et al. (2021). The PRISMA 2020 statement: an updated guideline for reporting systematic reviews. Bmj. 372:n71. doi: 10.1136/bmj.n71
Peri, C. V., Shaffrey, M. E., Farace, E., Cooper, E., Alves, W. M., Cooper, J. B., et al. (2001). Pilot study of electrical stimulation on median nerve in comatose severe brain injured patients: 3-month outcome. Brain Inj. 15, 903–910. doi: 10.1080/02699050110065709
Power, H. A., Morhart, M. J., Olson, J. L., and Chan, K. M. (2020). Postsurgical electrical stimulation enhances recovery following surgery for severe cubital tunnel syndrome: a double-blind randomized controlled trial. Neurosurgery. 86, 769–777. doi: 10.1093/neuros/nyz322
Redshaw, J. D., Lenherr, S. M., Elliott, S. P., Stoffel, J. T., Rosenbluth, J. P., Presson, A. P., et al. (2018). Protocol for a randomized clinical trial investigating early sacral nerve stimulation as an adjunct to standard neurogenic bladder management following acute spinal cord injury. BMC Urol. 18, 72. doi: 10.1186/s12894-018-0383-y
Shapira, Y., Sammons, V., Forden, J., Guo, G. F., Kipp, A., Girgulis, J., et al. (2019). Brief electrical stimulation promotes nerve regeneration following experimental in-continuity nerve injury. Neurosurgery. 85, 156–163. doi: 10.1093/neuros/nyy221
Sharma, N., Marzo, S. J., Jones, K. J., and Foecking, E. M. (2010). Electrical stimulation and testosterone differentially enhance expression of regeneration-associated genes. Exp. Neurol. 223, 183–191. doi: 10.1016/j.expneurol.2009.04.031
Shields, R. K., and Dudley-Javoroski, S. (2006). Musculoskeletal plasticity after acute spinal cord injury: effects of long-term neuromuscular electrical stimulation training. J. Neurophysiol. 95, 2380–2390. doi: 10.1152/jn.01181.2005
Singh, B., Xu, Q. G., Franz, C. K., Zhang, R., Dalton, C., Gordon, T., et al. (2012). Accelerated axon outgrowth, guidance, and target reinnervation across nerve transection gaps following a brief electrical stimulation paradigm. J. Neurosurg. 116, 498–512. doi: 10.3171/2011.10.JNS11612
Stampas, A., Korupolu, R., Zhu, L., Smith, C. P., and Gustafson, K. (2019). Safety, Feasibility, and efficacy of transcutaneous tibial nerve stimulation in acute spinal cord injury neurogenic bladder: a randomized control pilot trial. Neuromodulation. 22, 716–722. doi: 10.1111/ner.12855
Udina, E., Furey, M., Busch, S., Silver, J., Gordon, T., and Fouad, K. (2008). Electrical stimulation of intact peripheral sensory axons in rats promotes outgrowth of their central projections. Exp. Neurol. 210, 238–247. doi: 10.1016/j.expneurol.2007.11.007
Puigdemasa, A., Casals, L., Asensio, E., Udina, E., and Navarro, X. (2008). Immediate electrical stimulation enhances regeneration and reinnervation and modulates spinal plastic changes after sciatic nerve injury and repair. Exp. Neurol. 211, 180–193. doi: 10.1016/j.expneurol.2008.01.020
Willand, M. P., Nguyen, M. A., Borschel, G. H., and Gordon, T. (2016). Electrical stimulation to promote peripheral nerve regeneration. Neurorehabil. Neural Rep. 30, 490–496. doi: 10.1177/1545968315604399
Williams, H. B. (1996). A clinical pilot study to assess functional return following continuous muscle stimulation after nerve injury and repair in the upper extremity using a completely implantable electrical system. Microsurgery. 17, 597–605. doi: 10.1002/(SICI)1098-2752(1996)17:11<597::AID-MICR6>3.0.CO;2-M
Witzel, C., Brushart, T. M., Koulaxouzidis, G., and Infanger, M. (2016). Electrical nerve stimulation enhances perilesional branching after nerve grafting but fails to increase regeneration speed in a murine model. J. Reconstr. Microsurg. 32, 491–497. doi: 10.1055/s-0036-1579540
Wong, J. N., Olson, J. L., Morhart, M. J., and Chan, K. M. (2015). Electrical stimulation enhances sensory recovery: a randomized controlled trial. Ann. Neurol. 77, 996–1006. doi: 10.1002/ana.24397
Wu, X., Zhang, C., Feng, J., Mao, Q., Gao, G., and Jiang, J. (2017). Right median nerve electrical stimulation for acute traumatic coma (the Asia Coma Electrical Stimulation trial): study protocol for a randomised controlled trial. Trials. 18, 311. doi: 10.1186/s13063-017-2045-x
Keywords: peripheral nerve injury, electrical stimulation, clinical application, recovery, reinnervation
Citation: Costello MC, Errante EL, Smartz T, Ray WZ, Levi AD and Burks SS (2023) Clinical applications of electrical stimulation for peripheral nerve injury: a systematic review. Front. Neurosci. 17:1162851. doi: 10.3389/fnins.2023.1162851
Received: 10 February 2023; Accepted: 26 April 2023;
Published: 03 August 2023.
Edited by:
Lin Li, Capital Medical University, ChinaReviewed by:
Hongkai Wang, Northwestern University, United StatesBrendan MacKay, Texas Tech University Health Sciences Center, United States
Copyright © 2023 Costello, Errante, Smartz, Ray, Levi and Burks. This is an open-access article distributed under the terms of the Creative Commons Attribution License (CC BY). The use, distribution or reproduction in other forums is permitted, provided the original author(s) and the copyright owner(s) are credited and that the original publication in this journal is cited, in accordance with accepted academic practice. No use, distribution or reproduction is permitted which does not comply with these terms.
*Correspondence: Stephen Shelby Burks, c2J1cmtzQG1lZC5taWFtaS5lZHU=