- 1Department of Neurosurgery, Charité – Universitätsmedizin, corporate member of Freie Universität Berlin and Humboldt-Universität zu Berlin, Berlin, Germany
- 2Einstein Center for Neurosciences, Charité – Universitätsmedizin, corporate member of Freie Universität Berlin and Humboldt-Universität zu Berlin, Berlin, Germany
- 3International Graduate Program Medical Neurosciences, Charité – Universitätsmedizin, corporate member of Freie Universität Berlin and Humboldt-Universität zu Berlin, Berlin, Germany
- 4Department of Physiology, University of Eastern Finland, Kuopio, Finland
- 5Cluster of Excellence Matters of Activity, Image Space Material, Humboldt-Universität zu Berlin, Berlin, Germany
Background: Damage to the supplementary motor area (SMA) can lead to impairments of motor and language function. A detailed preoperative mapping of functional boarders of the SMA could therefore aid preoperative diagnostics in these patients.
Objective: The aim of this study was the development of a repetitive nTMS protocol for non-invasive functional mapping of the SMA while assuring effects are caused by SMA rather than M1 activation.
Methods: The SMA in the dominant hemisphere of 12 healthy subjects (28.2 ± 7.7 years, 6 females) was mapped using repetitive nTMS at 20 Hz (120% RMT), while subjects performed a finger tapping task. Reductions in finger taps were classified in three error categories (≤15% = no errors, 15–30% = mild, >30% significant). The location and category of induced errors was marked in each subject’s individual MRI. Effects of SMA stimulation were then directly compared to effects of M1 stimulation in four different tasks (finger tapping, writing, line tracing, targeting circles).
Results: Mapping of the SMA was possible for all subjects, yet effect sizes varied. Stimulation of the SMA led to a significant reduction of finger taps compared to baseline (BL: 45taps, SMA: 35.5taps; p < 0.01). Line tracing, writing and targeting of circles was less accurate during SMA compared to M1 stimulation.
Conclusion: Mapping of the SMA using repetitive nTMS is feasible. While errors induced in the SMA are not entirely independent of M1, disruption of the SMA induces functionally distinct errors. These error maps can aid preoperative diagnostics in patients with SMA related lesions.
1. Introduction
Lesions of the supplementary motor area (SMA) can lenad to widespread impairments such as unilateral motor deficits up to hemiplegia and mutism (Zentner et al., 1996; Nachev et al., 2008; Sjöberg et al., 2019). This so-called SMA syndrome is often temporary with patients recovering over the course of days to months. Yet, some patients report persisting deficits in complex motor functions several months after the lesion (Zentner et al., 1996; Rajshekhar, 2000; Acioly et al., 2015). It has been suggested that functional reorganisation of the SMA mainly via recruitment of the contralateral SMA facilitates recovery of deficits (Acioly et al., 2015; Vassal et al., 2017; Chivukula et al., 2018; Tuncer et al., 2022). However, these mechanisms are still poorly understood, and prediction of the individual extent and time course of recovery is limited.
Similarly, in case of surgical lesions to the SMA, preoperative assessment of the risk of a SMA syndrome is limited. Determining the exact location of functionally relevant portions of the SMA can be crucial to avoid damaging these regions during surgery, specifically since the SMA is not limited by exact anatomical boundaries. Previous studies (Zeharia et al., 2012; Hiroshima et al., 2014; Bathla et al., 2019) used functional MRI to localize the exact location of the SMA on the individual patient’s brain. Yet, task-based fMRI can be difficult to integrate into the clinical routine due to limitations of scanning time and available equipment. Recently, repetitive TMS (rTMS) has been used to disrupt SMA function in healthy subjects (Schramm et al., 2019, 2020). While the spatial resolution of the reported protocol was still limited, it provided evidence that the SMA can be functionally mapped similarly to language and motor relevant areas (Tarapore et al., 2016; Krieg et al., 2017). It further showed the possibility to map different SMA functionalities, thus enabling adaptation of the mapping to individual patients’ impairments. Further, while single-neuron responses are similar between SMA and the primary motor cortex (M1), both areas show distinct population dynamics during execution of motor tasks (Lara et al., 2018). Thus, disturbance of these dynamics using rTMS should lead to differential effects on task performance that can be used to separate SMA and M1 effects despite the vicinity of both areas.
The purpose of the present study was to develop a neuronavigated TMS (nTMS) -based protocol to localize portions of the SMA relevant for motor function on the individual brain. Such a protocol could then be used in preoperative planning to preserve functional SMA areas, to assess the risk for postoperative SMA syndrome or to quantify the extent of postoperative reorganisation. Due to the proximity of SMA and M1 a specific focus of this study was to validate that induced effects are indeed caused by SMA stimulation rather than activation of M1.
2. Materials and methods
2.1. Subjects
Twelve subjects (mean age 28.2 years, SD 7.7 years, 6 females) without any history of neurological or psychiatric illness provided their written informed consent to participate in this study. All subjects met the criteria for receiving an MRI scan and the TMS assessment. Exclusion criteria were history of epilepsy (also within the family), migraine, tinnitus, pregnancy, intake of prescription drugs within the past 14 days, permanent make-up, tattoos or metallic implants including any form of intrauterine devices. The study was conducted in accordance with the Declaration of Helsinki and approved by the local ethics committee.
2.2. MRI
All subjects received a T1-weighted MPRAGE sequence (TR = 2.530 ms, TE = 4.94 ms, TI = 1.100 ms, flip angle = 7, voxel size = 1 mm × 1 mm × 1 mm, 176 slices) measured on a Siemens 3-T Magnetom Trio MRI scanner (Siemens AG, Erlangen, Germany). The scan took approximately 10 min for each subject.
2.3. Neuronavigated TMS
The neuronavigated TMS (nTMS) assessment was divided into three parts (Figure 1): First the primary motor cortex was examined using single-pulse TMS. Next, the SMA was stimulated with repetitive TMS (rTMS) while subjects performed a motor task. In the final part, effects of stimulation of both areas were compared using different motor tasks. This protocol took roughly 2.5 h for each subject. NTMS was applied using a Nexstim NBS 5 stimulator (Nexstim, Helsinki, Finland) with a biphasic figure-of-eight coil (outer diameter: 70 mm). The previously acquired structural MRI was used as a subject-specific navigational dataset. Subjects were seated in a comfortable chair with a headrest and facing a black monitor in front of a white wall. The monitor contained the systems inbuilt camera used to record all tasks.
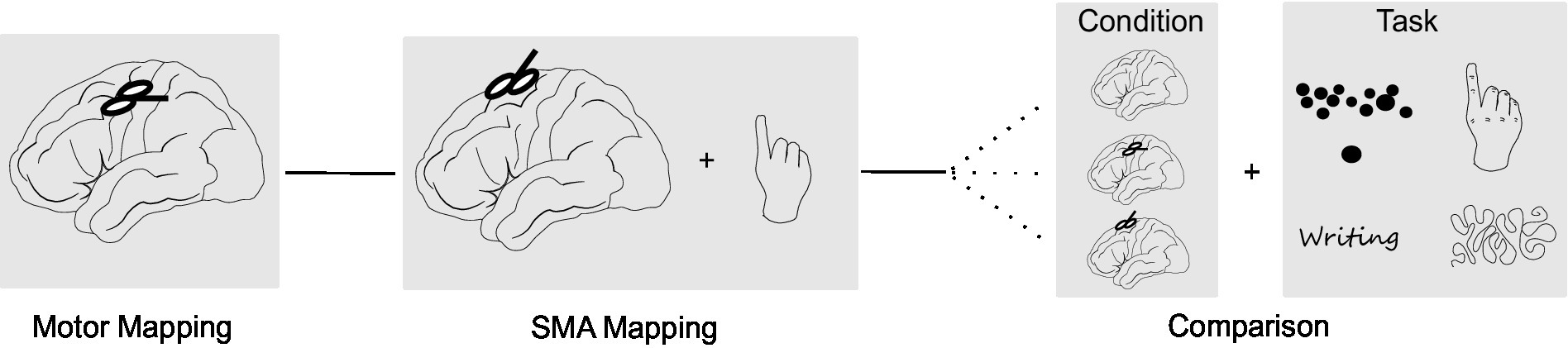
Figure 1. Study design. The study consisted of three parts: (A) Mapping of the primary motor cortex using single-pulse TMS. (B) SMA mapping with repetitive TMS (rTMS) while subjects performed a finger tapping task. (C) Comparison of task performance without stimulation, stimulation of the M1 hotspot and stimulation of the SMA hotspot. In this part, four different motor tasks (finger tapping, writing, line tracing, targeting circles) were applied in randomized order.
2.3.1. Motor assessment
Motor evoked potentials were recorded from the first dorsal interosseous muscle of the dominant hand. To this purpose, disposable Ag/ AgCl surface electrodes (Neuroline 700; Ambu, Ballerup, Denmark) were attached in a belly-tendon fashion with the ground electrode on the left palmar wrist. Subjects were instructed to relax their hand muscles and muscle activity was monitored to assure relaxation of the muscle below a threshold of 10 μV. The motor hotspot was defined as stimulation site, electric field direction and angulation consistently eliciting the largest motor evoked potentials in the target muscle. For this point, the RMT was measured using the system’s inbuilt automated threshold hunting method (Engelhardt et al., 2019). The RMT was recorded as percentage of the stimulator output as well as the intensity of the induced electric field. To determine the size of the cortical representation of the target muscle, an area mapping with an intensity of 105% of the RMT was performed concentrically (Engelhardt and Picht, 2020). This area was then compared with the SMA area to identify potential overlapping portions and ensure SMA mapping is not confounded by direct activation of motor responses.
2.3.2. SMA mapping
Consequently, anatomical boarders of the SMA region were estimated based on the structural MRI (Vorobiev et al., 1998). SMA was estimated as portion of the superior frontal gyrus until the point where a vertical line traversing the anterior commissure crosses the cortex. The suspected SMA region was then stimulated with rTMS (20 Hz, 120% RMT, 5 s bursts, ITI 5 s) while subjects performed a finger tapping task (Hiroshima et al., 2014; Schramm et al., 2019). For stimulation, the coil was placed perpendicular to the interhemispheric cleft. We chose 20 Hz as stimulation frequency as this was tolerated well and induced reliable disruptions of task performance in pilot subjects. There was no delay between the onset of stimulation and onset of the task, that is subjects were instructed to start immediately when they heard the rTMS. For the finger tapping, subjects were instructed to tap with their dominant index finger as fast as possible. Each subject performed two rounds of finger tapping without stimulation as baseline. Next, the anatomically estimated SMA region was stimulated in variable order with rTMS. After 5 stimulations, subjects rested their hand for roughly 1 minute to prevent fatigue of the target muscles. Due to anatomical differences and different stages of protocol development, the amount of stimulation points and order of stimulation varied between subjects. While we recommend around 20 stimulation points per hemisphere with each point being stimulated twice to assess replicability of the induced effects, SMA maps with fewer points are present in this study. The location of induced errors was marked in each subject’s individual MRI. Each session was further recorded on video to allow for offline analysis of induced errors using the nTMS systems inbuilt camera. The number of finger taps was recorded for each trial and converted to measure the reduction in finger taps compared to the baseline (in %).
2.3.3. Comparison between SMA and M1 stimulation
To exclude SMA effects due to stimulation of M1 via the peripheral magnetic field, effects of SMA stimulation were directly compared to effects of M1 stimulation. To this purpose, a SMA hotspot was defined as the point eliciting the largest disruptions of task performance upon stimulation. For this point, the intensity of the induced electric field was recorded. Further, the electric field induced at the M1 hotspot when stimulating the SMA hotspot was estimated (residual SMA intensity). In the Nexstim system this intensity can be identified by selecting a stimulation point over the SMA hotspot and placing a crosshair over the M1 hotspot. The system then displays the electric field based on a spherical head model for the position of the crosshair. Consequently, the SMA hotspot was stimulated with the SMA mapping intensity (120% RMT) and the M1 hotspot was stimulated with the residual SMA intensity. Each subject performed two rounds without stimulation (baseline), with SMA stimulation and with M1 stimulation while executing 1 of 4 different tasks (Figure 1). Duration of stimulation was increased to last for the whole duration of the task. The order of the stimulation conditions and tasks applied were randomized between subjects.
Task 1 consisted of a finger tapping for 10 s (n = 9 subjects). The number of finger taps was recorded as well as any noticeable deviations in the movement pattern, for example arrhythmicity of tapping, changes in movement ranges or a time-dependency of these effects. For task 2, subjects had to write a short sentence on a piece of paper. For analysis, the time to write the sentence (n = 7 subjects) and deviations in the writing pattern (legible, non-legible; n = 8 subjects) were documented. An example categorization is presented in Figure 2B. Task 3 required tracing a curved line with a pencil as fast and accurately as possible (Supplementary Figure S1; n = 9 subjects). The task was stopped if subjects did not reach the end of the line after 20 s. Deviations from the line were analysed qualitatively (line traceable without problems, line traceable with strong deviations, line not traceable). An example categorization is presented in Figure 2A. For the fourth task, subjects (n = 8) had to point a pencil to small circles on a paper as fast and accurately as possible for 20 s (Supplementary Figure S2). The number of circles targeted and number of circles missed (i.e., pencil marks outside a circle) were recorded. Tasks 2–4 were chosen as they represent more complex, coordinated movements, which are often impaired after surgical resection of the SMA according to the experience of neurosurgeons in our department.
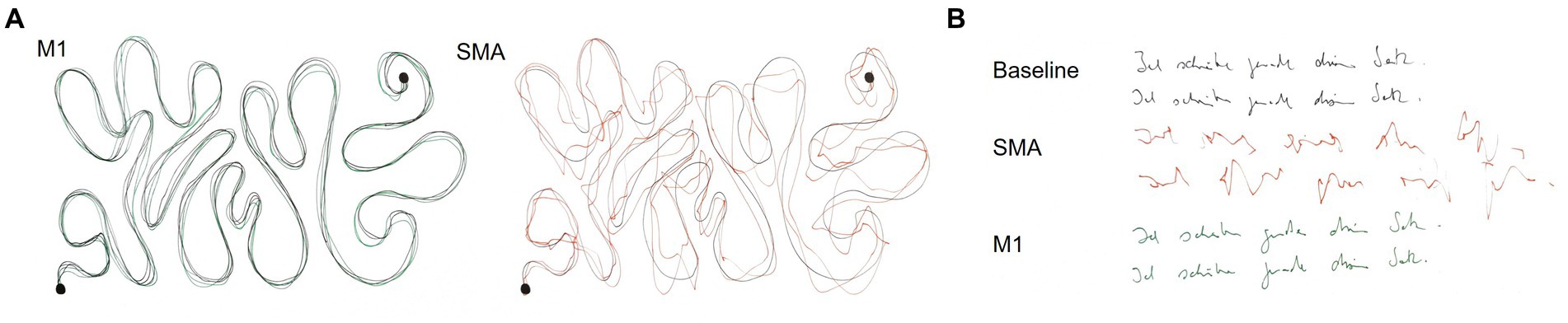
Figure 2. Example results of the line tracing (A) and writing task (B) for one subject. (A) The black line corresponds to the reference line during the tracing task. M1 stimulation was rated as traceable without problems and SMA stimulation as traceable with strong deviations. (B) Both baseline and M1 stimulation were rated as legible, whereas SMA stimulation was categorized as non-legible.
2.4. Data analysis
The reduction in finger taps compared to baseline for each stimulation was categorized into three groups. Reductions ≤15% were counted as no errors, between 15% and 30% as mild errors, and >30% as significant errors. These errors were marked using the nTMS system inbuilt analysis program and imported into the NBS software to create coloured SMA error maps.
Locations of SMA and M1 areas were assessed for potential overlaps in all subjects. We further compared the individual RMTs with the electric field induced at the M1 hotspot during SMA stimulation for all stimulation points. SMA stimulation points inducing an electric field above the RMT at M1 were highlighted and removed from SMA error maps.
For comparison of M1 and SMA stimulation, we first calculated the mean of the two trials per subject, task and stimulation condition. Next, values of baseline, M1 and SMA stimulation were compared using one-sided Wilcoxon signed-rank tests. Median values for each stimulation condition and task are reported. p-values <0.05 were considered significant, p-values ≤0.1 are additionally reported as tendencies due to the small sample size. Further, qualitative deviations between both stimulations were analysed using the recorded videos and handwritten tests. These results are presented as incidence rates.
All statistical analyses were performed in RStudio (Version 1.3.1073) using the packages base (R Core Team, 2021) and tidyverse (Wickham et al., 2019).1
3. Results
3.1. SMA error maps
Errors in task performance could be induced in all 12 subjects. However, there was considerable variability in the size and location of SMA error maps as well as intensity of induced errors between subjects. Across subjects, a median of 34 points were stimulated (minimum 24, maximum 42 points). In 5 subjects, significant errors >30% reductions could be induced over a median of 3 stimulation points. Accordingly, error incidence for significant errors varied between 3% and 26% between subjects with a median incidence of 7%. Mild errors (15%–30% reduction) were recorded in all 12 subjects over a median of 12 stimulation points. Error incidence for mild errors varied between 3% and 79% between subjects with a median incidence of 36%. In two subjects (Figures 3D,G), only one error could be induced after exclusion of errors likely due to M1 stimulation. SMA error maps of all subjects are presented in Figure 3.
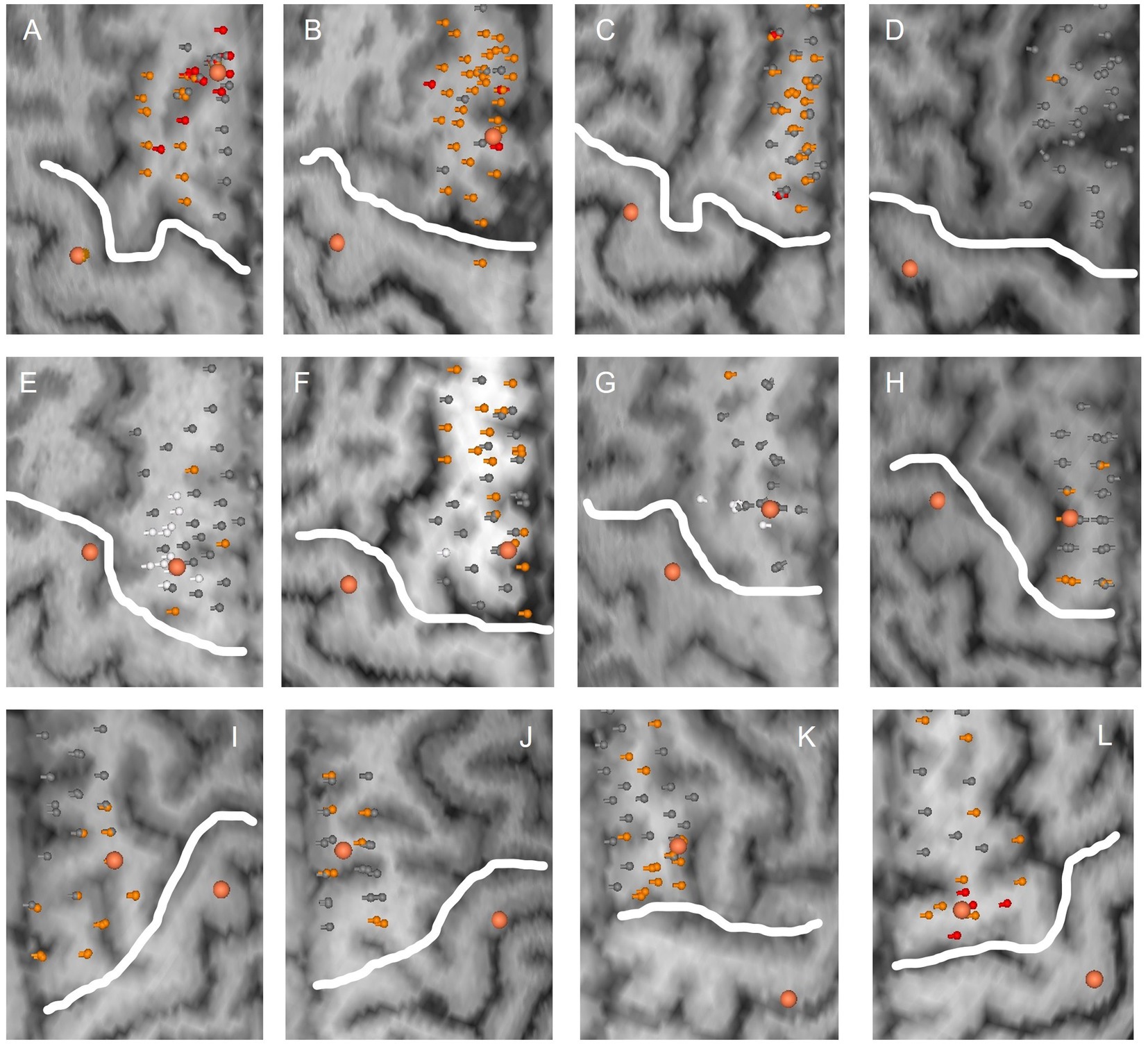
Figure 3. SMA error maps for all subjects. Each subplot (A–L) corresponds to the stimulated hemisphere of one subject. Stimulation points are visualized with their respective error category in grey (no error), orange (mild errors) and red (significant errors). Stimulation points inducing an electric field above the RMT at M1 are marked in white and were excluded from SMA areas. Larger orange dots correspond to SMA and M1 hotspots, respectively. The white line delineates M1 from the presumed SMA area.
3.2. Comparison of SMA error maps and M1 areas
There was no overlap of stimulation points in SMA and M1 areas. However, in three subjects the electric field recoded at the motor hotspot was above the RMT for some of the functional points of the SMA area. In one subject, this included most of the functional SMA points as well as the SMA hotspot (Figure 3E). The subject was therefore removed from further analysis. For the other two subjects, these points were highlighted and removed from the SMA error maps (Figures 3F,G).
3.3. Comparison of SMA and M1 stimulation
Definition of an SMA hotspot was possible in 9 out of 12 subjects. One subject had to be excluded as the electric field recorded at the motor hotspot was above the RMT for this location (Figure 3E). In two other subjects, no clear hotspot could be detected during the SMA mapping (Figures 3C,D). Generally, the strongest task disruptions and thus SMA hotspots were located in the posterior or medial SMA. One of the remaining 9 subjects did not perform the writing task due to time constraints of the measurement. Finally, in one subject only the written result of the line tracing and writing could be analysed due to problems with the video recording. Examples of induced disruptions in task performance are presented as videos (Supplementary Video 1 Finger tapping; Supplementary Video 2 Writing; Supplementary Video 3 Line tracing; Supplementary Video 4 Targeting circles). Note that these results always refer to task disruptions during hotspot stimulation and not mapping of a larger area.
A significant reduction of finger taps compared to baseline during SMA (BL: 45 taps, SMA: 35.5 taps; p < 0.01) and M1 stimulation (M1: 41.5 taps; p = 0.02) was observed. This effect was stronger during SMA then M1 stimulation (p = 0.04; Figure 4A). Further, there was a tendency for a reduction in the number of circles targeted during SMA stimulation compared to baseline (BL: 18 circles, SMA: 15 circles; p = 0.09; Figure 4C) as well as an increase in the error rate (BL: 5.2%, SMA: 9.7%; p = 0.07; Figure 4D). No effects were observed during M1 stimulation for either the number of circles (M1: 16 circles; p = 0.24; Figure 4C) or the error rate (M1: 8.2%; p = 0.32; Figure 4D). Fewer circles were targeted during SMA compared to M1 stimulation (p < 0.01) and a tendency for a higher error rate was observed (p = 0.1). No differences between stimulations were observed in the time to complete the writing task (Figure 4B).
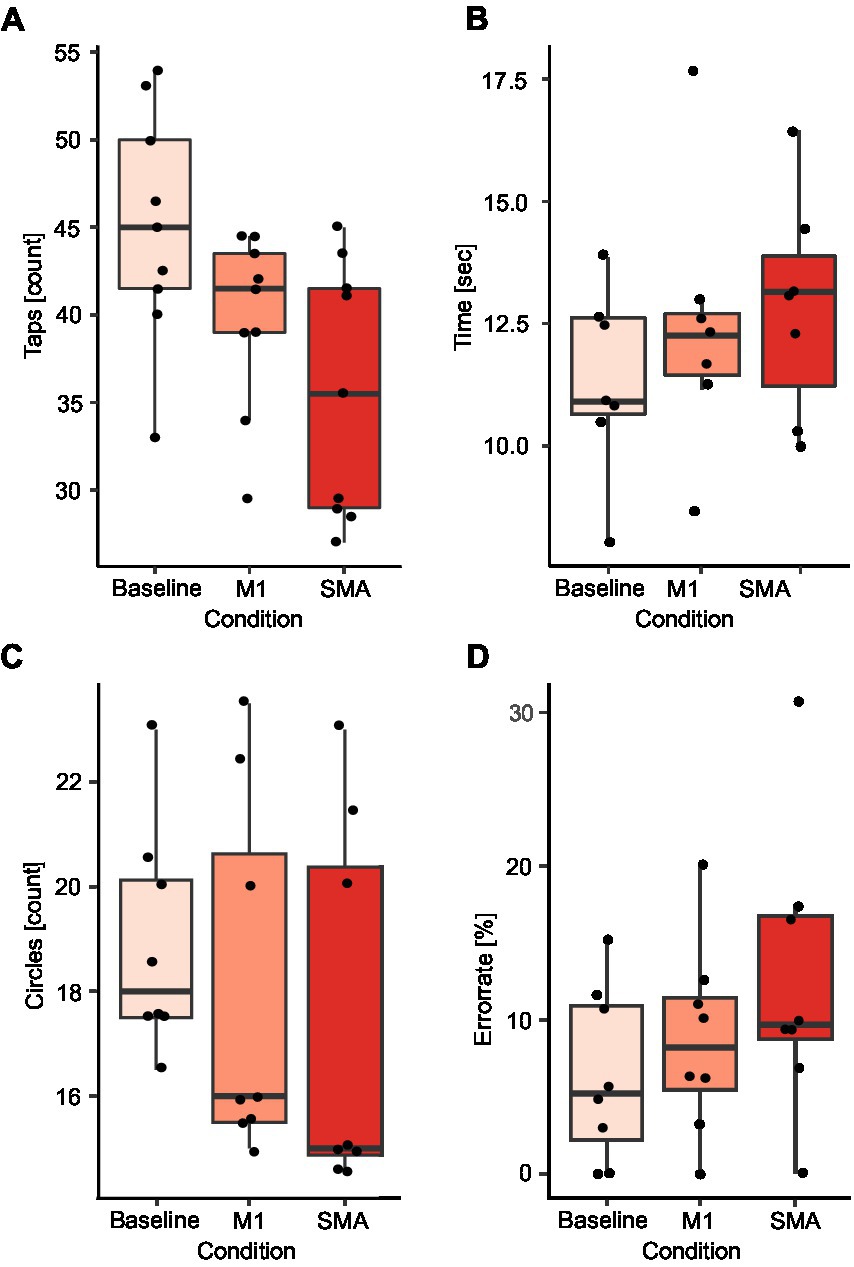
Figure 4. Comparison of SMA and M1 stimulation. Results are presented for the finger tapping task (A), the writing task (B), the number of circles targeted (C), and the error rate during the circle targeting task (D). Black dots correspond to average values for single subjects in each condition and task.
Line tracing and writing was less accurate during SMA compared to M1 stimulation. In the line tracing task, 4 subjects (44%) were able to trace the line without deviations during stimulation of the SMA. 4 subjects (44%) showed strong deviations from the reference line and one subject (11%) was not able to trace the line at all. In contrast, during M1 stimulation only one subject showed slight deviations (11%) from the line. In the writing task, SMA stimulation led to a non-legible result in 3 subjects (38%), whereas only one subject produced non-legible writing during M1 stimulation. The error categories as well as results of both tasks for one example subject are presented in Figure 2. In two subjects (40%) with visible disruptions in the line tracing task and one subject (33%) with disruptions in the writing task, effects of SMA disruption increased with stimulation time, while effects of M1 stimulation were present from the beginning on. Four subjects (44%) show a modulation of task performance during SMA stimulation in all executed tasks, while five (56%) subjects show a modulation in some but not all tasks.
4. Discussion
The present study developed a nTMS-based SMA mapping protocol with a high spatial resolution. In this way, we were able to localize functionally relevant subregions of the SMA within a larger anatomically predefined area. It was further possible to define a hotspot where the strongest errors could be induced, analogous to motor nTMS assessments.
The proposed protocol follows a virtual lesion paradigm as it is commonly used for the assessment of language function using nTMS (Krieg et al., 2017; Burke et al., 2019). Previously, two studies (Schramm et al., 2019, 2020) in healthy subjects have shown that the SMA is susceptible to this kind of stimulation. In these studies, 10 Hz stimulation could disrupt performance of different sub modalities of the Jebsen Taylor Hand function test when applied to the SMA. While these results are promising regarding the capabilities of repetitive nTMS, stimulation was only applied to six predefined targets of the SMA. Therefore, it remained unclear if this stimulation paradigm can be extended to a detailed mapping of the SMA. The present study used a short 5 s finger tapping task which can be repeated over multiple stimulation points to assess SMA function. In favour of this task, the SMA has been suggested to play a role in the encoding of movement sequences (Nachev et al., 2008; Solopchuk et al., 2017) and fMRI studies have shown an activation of the SMA during finger tapping (Hiroshima et al., 2014). Further, rTMS has been used to modulate inter-tap intervals during finger tapping (Schramm et al., 2019). While the present study focused on the number of taps as mapping outcome, further studies should certainly look into a more detailed analysis of movement kinematics to make mapping more specific to different functional aspects. This could include quantifying arrhythmicity of tapping, changes in movement ranges or a time-dependency of these effects.
Using this stimulation protocol, it is possible to achieve a high-resolution mapping in roughly 10 min for one hemisphere. The proposed protocol includes short breaks after a maximum of five stimulation targets to avoid fatigue of hand muscles. Further, to reduce the impact of false positives on the accuracy of the functional assessment, stimulation targets could be stimulated multiple times. Classification of errors could then be restricted to points with replicable reductions in finger tapping performance, assuming that stimulation of functional points should consistently disrupt task execution. Future studies should also investigate the proposed limits for error categories to quantify when a reduction in finger taps is sufficiently large. This could be aided by studies in neurosurgical patients, where resection of functional points can be compared with occurrence and severity of functional impairments.
In the present study, a disruption of performance was also visible in other tasks involving more complex movements such as writing or targeting circles. Thus, our findings highlight the possibility to study functional organisation and somatotopy of the SMA using repetitive nTMS, when paired for example with toe tapping to study lower extremity function or targeting small circles to examine coordinated movements. Previous studies have suggested that the SMA has a somatotopic organisation with lower extremities being represented in the posterior SMA, upper extremities in the medial SMA and the face in the anterior part of the SMA (Zeharia et al., 2012). While the present study was not designed to investigate SMA somatotopy, most subjects showed the strongest disruptions in task performance in medial to posterior portions of the SMA thus supporting this notion. Since additional tasks in this study were only tested on the SMA hotspot, no further effects of somatotopy could be investigated. However, these effects should be studied more systematically by including lower extremity movements to assess somatotopy. Additionally, it could be investigated whether hemispheric differences are present in the functional organisation of the SMA or how disruptions in task performance differ when stimulating the pre-SMA.
Importantly, there was a considerable variation in the strengths of the induced stimulation effect between subjects. We hypothesize that in some subjects the stimulation intensity might not have been sufficient to disrupt the SMA region. As the SMA is located in the posterior portion of the superior frontal gyrus extending into the interhemispheric cleft (Vorobiev et al., 1998; Nachev et al., 2008), some subregions might be more difficult to stimulate as they are further away from the coil. In the present study, we refrained from increasing the stimulation intensity beyond 120% of the RMT due to the novelty of the protocol, to reduce the risk for any side effects and to reduce the risk of M1 contamination of the effects. However, since the stimulation was tolerated well, it seems that these limits can be exceeded in future studies and thereby the responder rate might be increased if effects are controlled for M1 contamination.
Finally, we aimed to distinguish effects of SMA and M1 stimulation to ensure recorded stimulation effects are not due to an indirect activation of M1 (Mirbagheri et al., 2020). In support of our protocol, errors induced during stimulation of the SMA hotspot were stronger than during M1 stimulation with the residual SMA targeting electric field. Further, at least in some subjects effects during SMA stimulation were qualitatively different from M1. Errors built up over time, while effects of M1 stimulation were present from the beginning on. However, an induced electric field larger than the RMT was recorded over the motor hotspot when stimulating some of the functionally positive points over the SMA. This is not surprising given the proximity of both regions and intensity of stimulation but warrants caution when interpreting any SMA mapping results. Consequently, we argue that the electric field induced at M1 should always be controlled for when stimulating the SMA. Future studies in patients could further assess whether stimulated points are functionally essential by comparing resection of positive nTMS mapping points to occurrence and type of postoperative deficits (Moser et al., 2017).
Our results are limited by the fact that the present protocol was only tested in a young, healthy population. While we are confident that the protocol can also be used in elderly, factors such as an increased coil to cortex distance following brain atrophy or a reduced attention span during the task execution might impact the mapping. Further, the included calculations of the electric field are based on the systems inbuilt modelling which uses a multi-sphere model with locally chosen spherical conductors. A recent study (Nieminen et al., 2022) has shown that electric field models based on more realistic head models provide slightly different and more accurate electric field estimates. Therefore, we cannot exclude that our results, specifically points of the SMA area excluded due to M1 activation, would be different with a more realistic electric field model. It can also not be excluded that sub threshold stimulation of other cortical areas contributed to induced effects, since there is no consensus on the minimally required electric field magnitude. We tried to address this to some extent by directly stimulating M1 with the residual SMA intensity and comparing effects to direct SMA stimulation but did not control for other non-primary motor areas. Finally, a different coil type might lead to a more focal stimulation and hence reduce any potential impact of other brain areas on the effects given that required stimulation intensities can be reached in the SMA.
In conclusion, mapping of the SMA using repetitive nTMS is possible as stimulation of the SMA can disrupt hand movements analogous to a virtual lesion paradigm. Due to the proximity to M1, stimulation intensities as well as the electric field induced at M1 need to be monitored during SMA mapping so assure validity of the induced errors. Finally, this protocol could also be integrated in preoperative planning to assess the risk for developing a postoperative SMA syndrome or to quantify the extent of postoperative reorganisation.
Data availability statement
The original contributions presented in the study are included in the article/Supplementary material, further inquiries can be directed to the corresponding author.
Ethics statement
The studies involving human participants were reviewed and approved by Ethics Committee of Charité – Universitätsmedizin Berlin, Campus Mitte. The participants provided their written informed consent to participate in this study.
Author contributions
ME: conceptualization, methodology, formal analysis, investigation, data curation, writing – original draft, visualization, and project administration. GK: formal analysis, data curation, and writing – review and editing. JK: conceptualization, methodology, and writing – review and editing. TP: conceptualization, methodology, writing – review and editing, and supervision. All authors contributed to the article and approved the submitted version.
Funding
The authors acknowledge the support of the Cluster of Excellence Matters of Activity. Image Space Material funded by the Deutsche Forschungsgemeinschaft (DFG, German Research Foundation) under Germany’s Excellence Strategy – EXC 2025-390648296.
Acknowledgments
MR-imaging for this study was performed at the Berlin Center for Advanced Neuroimaging (BCAN).
Conflict of interest
The authors declare that the research was conducted in the absence of any commercial or financial relationships that could be construed as a potential conflict of interest.
Publisher’s note
All claims expressed in this article are solely those of the authors and do not necessarily represent those of their affiliated organizations, or those of the publisher, the editors and the reviewers. Any product that may be evaluated in this article, or claim that may be made by its manufacturer, is not guaranteed or endorsed by the publisher.
Supplementary material
The Supplementary material for this article can be found online at: https://www.frontiersin.org/articles/10.3389/fnins.2023.1185483/full#supplementary-material
Footnotes
References
Acioly, M. A., Cunha, A. M., Parise, M., Rodrigues, E., and Tovar-Moll, F. (2015). Recruitment of contralateral supplementary motor area in functional recovery following medial frontal lobe surgery: an fMRI case study. J Neurol Surg A Cent Eur Neurosurg 76, 508–512. doi: 10.1055/s-0035-1558408
Bathla, G., Gene, M. N., Peck, K. K., Jenabi, M., Tabar, V., and Holodny, A. I. (2019). Resting state functional connectivity of the supplementary motor area to motor and language networks in patients with brain tumors. J. Neuroimaging 29, 521–526. doi: 10.1111/jon.12624
Burke, M. J., Fried, P. J., and Pascual-Leone, A. (2019). Transcranial magnetic stimulation: neurophysiological and clinical applications. Handb. Clin. Neurol. 163, 73–92. doi: 10.1016/B978-0-12-804281-6.00005-7
Chivukula, S., Pikul, B. K., Black, K. L., Pouratian, N., and Bookheimer, S. Y. (2018). Contralateral functional reorganization of the speech supplementary motor area following neurosurgical tumor resection. Brain Lang. 183, 41–46. doi: 10.1016/j.bandl.2018.05.006
Engelhardt, M., and Picht, T. (2020). 1 Hz repetitive transcranial magnetic stimulation of the primary motor cortex: impact on excitability and task performance in healthy subjects. J Neurol Surg A Cent Eur Neurosurg 81, 147–154. doi: 10.1055/s-0040-1701624
Engelhardt, M., Schneider, H., Gast, T., and Picht, T. (2019). Estimation of the resting motor threshold (RMT) in transcranial magnetic stimulation using relative-frequency and threshold-hunting methods in brain tumor patients. Acta Neurochir. 161, 1845–1851. doi: 10.1007/s00701-019-03997-z
Hiroshima, S., Anei, R., Murakami, N., and Kamada, K. (2014). Functional localization of the supplementary motor area. Neurol. Med. Chir. (Tokyo) 54, 511–520. doi: 10.2176/nmc.oa2012-0321
Krieg, S. M., Lioumis, P., Mäkelä, J. P., Wilenius, J., Karhu, J., Hannula, H., et al. (2017). Protocol for motor and language mapping by navigated TMS in patients and healthy volunteers; workshop report. Acta Neurochir. 159, 1187–1195. doi: 10.1007/s00701-017-3187-z
Lara, A. H., Cunningham, J. P., and Churchland, M. M. (2018). Different population dynamics in the supplementary motor area and motor cortex during reaching. Nat. Commun. 9:2754. doi: 10.1038/s41467-018-05146-z
Mirbagheri, A., Schneider, H., Zdunczyk, A., Vajkoczy, P., and Picht, T. (2020). NTMS mapping of non-primary motor areas in brain tumour patients and healthy volunteers. Acta Neurochir. 162, 407–416. doi: 10.1007/s00701-019-04086-x
Moser, T., Bulubas, L., Sabih, J., Conway, N., Wildschutz, N., Sollmann, N., et al. (2017). Resection of navigated transcranial magnetic stimulation-positive Prerolandic motor areas causes permanent impairment of motor function. Neurosurgery 81, 99–110. doi: 10.1093/neuros/nyw169
Nachev, P., Kennard, C., and Husain, M. (2008). Functional role of the supplementary and pre-supplementary motor areas. Nat. Rev. Neurosci. 9, 856–869. doi: 10.1038/nrn2478
Nieminen, A. E., Nieminen, J. O., Stenroos, M., Novikov, P., Nazarova, M., Vaalto, S., et al. (2022). Accuracy and precision of navigated transcranial magnetic stimulation. J. Neural Eng. 19:066037. doi: 10.1088/1741-2552/aca71a
Rajshekhar, U. B. V. (2000). Post operative supplementary motor area syndrome: clinical features and outcome. Br. J. Neurosurg. 14, 204–210. doi: 10.1080/026886900408379
Schramm, S., Albers, L., Ille, S., Schröder, A., Meyer, B., Sollmann, N., et al. (2019). Navigated transcranial magnetic stimulation of the supplementary motor cortex disrupts fine motor skills in healthy adults. Sci. Rep. 9:17744. doi: 10.1038/s41598-019-54302-y
Schramm, S., Sollmann, N., Ille, S., Meyer, B., and Krieg, S. M. (2020). Application of navigated transcranial magnetic stimulation to map the supplementary motor area in healthy subjects. J. Clin. Neurophysiol. 37, 140–149. doi: 10.1097/WNP.0000000000000530
Sjöberg, R. L., Stålnacke, M., Andersson, M., and Eriksson, J. (2019). The supplementary motor area syndrome and cognitive control. Neuropsychologia 129, 141–145. doi: 10.1016/j.neuropsychologia.2019.03.013
Solopchuk, O., Alamia, A., Dricot, L., Duque, J., and Zénon, A. (2017). cTBS disruption of the supplementary motor area perturbs cortical sequence representation but not behavioural performance. NeuroImage 163, 34–40. doi: 10.1016/j.neuroimage.2017.09.013
Tarapore, P. E., Picht, T., Bulubas, L., Shin, Y., Kulchytska, N., Meyer, B., et al. (2016). Safety and tolerability of navigated TMS for preoperative mapping in neurosurgical patients. Clin. Neurophysiol. 127, 1895–1900. doi: 10.1016/j.clinph.2015.11.042
Tuncer, M. S., Fekonja, L. S., Ott, S., Pfnür, A., Karbe, A.-G., Engelhardt, M., et al. (2022). Role of interhemispheric connectivity in recovery from postoperative supplementary motor area syndrome in glioma patients. J. Neurosurg. 1–10, 1–10. doi: 10.3171/2022.10.JNS221303
Vassal, M., Charroud, C., Deverdun, J., le Bars, E., Molino, F., Bonnetblanc, F., et al. (2017). Recovery of functional connectivity of the sensorimotor network after surgery for diffuse low-grade gliomas involving the supplementary motor area. J. Neurosurg. 126, 1181–1190. doi: 10.3171/2016.4.JNS152484
Vorobiev, V., Govoni, P., Rizzolatti, G., Matelli, M., and Luppino, G. (1998). Parcellation of human mesial area 6: cytoarchitectonic evidence for three separate areas. Eur. J. Neurosci. 10, 2199–2203. doi: 10.1046/j.1460-9568.1998.00236.x
Wickham, H., Averick, M., Bryan, J., Chang, W., McGowan, L., François, R., et al. (2019). Welcome to the Tidyverse. J Open Source Softw. 4:1686. doi: 10.21105/joss.01686
Zeharia, N., Hertz, U., Flash, T., and Amedi, A. (2012). Negative blood oxygenation level dependent homunculus and somatotopic information in primary motor cortex and supplementary motor area. Proc. Natl. Acad. Sci. 109, 18565–18570. doi: 10.1073/pnas.1119125109
Keywords: rTMS, supplementary motor area, brain mapping, preoperative planning, somatotopy
Citation: Engelhardt M, Kern G, Karhu J and Picht T (2023) Protocol for mapping of the supplementary motor area using repetitive navigated transcranial magnetic stimulation. Front. Neurosci. 17:1185483. doi: 10.3389/fnins.2023.1185483
Edited by:
Samu Taulu, University of Washington, United StatesReviewed by:
Koen Cuypers, University of Hasselt, BelgiumMaria Nazarova, Massachusetts General Hospital, Harvard Medical School, United States
Copyright © 2023 Engelhardt, Kern, Karhu and Picht. This is an open-access article distributed under the terms of the Creative Commons Attribution License (CC BY). The use, distribution or reproduction in other forums is permitted, provided the original author(s) and the copyright owner(s) are credited and that the original publication in this journal is cited, in accordance with accepted academic practice. No use, distribution or reproduction is permitted which does not comply with these terms.
*Correspondence: Melina Engelhardt, bWVsaW5hLmVuZ2VsaGFyZHRAY2hhcml0ZS5kZQ==