- 1School of Rehabilitation Medicine, Nanjing Medical University, Nanjing, Jiangsu, China
- 2Department of Rehabilitation Medicine, The First Affiliated Hospital of Nanjing Medical University, Nanjing, Jiangsu, China
- 3Department of Rehabilitation Medicine, Sir Run Run Hospital, Nanjing Medical University, Nanjing, Jiangsu, China
Background: Transcutaneous auricular vagus nerve stimulation (taVNS) has emerged as a promising brain stimulation modality in poststroke upper extremity rehabilitation. Although several studies have examined the safety and reliability of taVNS, the mechanisms underlying motor recovery in stroke patients remain unclear.
Objectives: This study aimed to investigate the effects of taVNS paired with task-oriented training (TOT) on upper extremity function in patients with subacute stroke and explore the potential underlying mechanisms.
Methods: In this double-blinded, randomized, controlled pilot trial, 40 patients with subacute stroke were randomly assigned to two groups: the VNS group (VG), receiving taVNS during TOT, and the Sham group (SG), receiving sham taVNS during TOT. The intervention was delivered 5 days per week for 4 weeks. Upper extremity function was measured using the Fugl-Meyer Assessment-Upper Extremity (FMA-UE), the Action Research Arm Test (ARAT). Activities of daily living were measured by the modified Barthel Index (MBI). Motor-evoked potentials (MEPs) were measured to evaluate cortical excitability. Assessments were administered at baseline and post-intervention. Additionally, the immediate effect of taVNS was detected using functional near-infrared spectroscopy (fNIRS) and heart rate variability (HRV) before intervention.
Results: The VG showed significant improvements in upper extremity function (FMA-UE, ARAT) and activities of daily living (MBI) compared to the SG at post-intervention. Furthermore, the VG demonstrated a higher rate of elicited ipsilesional MEPs and a shorter latency of MEPs in the contralesional M1. In the VG, improvements in FMA-UE were significantly associated with reduced latency of contralesional MEPs. Additionally, fNIRS revealed increased activation in the contralesional prefrontal cortex and ipsilesional sensorimotor cortex in the VG in contrast to the SG. However, no significant between-group differences were found in HRV.
Conclusion: The combination of taVNS with TOT effectively improves upper extremity function in patients with subacute stroke, potentially through modulating the bilateral cortex excitability to facilitate task-specific functional recovery.
1 Introduction
A cerebrovascular accident (CVA), often referred to as a stroke, arises from an interruption of blood flow or bleeding in a region of the brain, resulting in impaired brain function. According to the Global Burden of Disease (GBD) study, stroke is the primary cause of mortality among Chinese adults and the second leading cause of death worldwide (Zhou et al., 2019; GBD 2019 Stroke Collaborators, 2021). Reports indicate that 55–75% of stroke patients continue to experience upper extremity motor dysfunction within 3–6 months of onset (Kwakkel et al., 2003). Upper extremity motor dysfunction markedly influences the prognosis of patients, impacting their mobility, daily activities, and overall quality of life (Lai et al., 2002; Nichols-Larsen et al., 2005). For poststroke upper extremity rehabilitation, the task-oriented training (TOT) involves structured movement training based on daily activities to engage patients actively, thereby promoting motor function during the targeted task practice (Yoo and Park, 2015). Motor priming, a type of implicit learning wherein external stimulation prompts changes in the motor cortex and behavior, has been reported in motor skill learning recently (Jin et al., 2019). Previous research demonstrated that stimulation-based priming combined with TOT could facilitate brain reorganization and enhance upper extremity dexterity (Higgins et al., 2013; Alsubiheen et al., 2022). The most commonly used non-invasive brain stimulation techniques include transcranial magnetic stimulation (TMS) and transcranial direct current stimulation (tDCS) (Chhatbar et al., 2017; Bai et al., 2022; Zhang et al., 2022). However, the application of TMS and tDCS is limited by the need for high precision requirements and complex operations.
Vagus nerve stimulation (VNS) acts as a promising brain stimulation-based priming technique involving various forms of stimulation applied to the vagus nerve network. By regulating the balance of the autonomic nervous system and targeting neuroprotective and neuroplasticity pathways, VNS holds potential as a therapeutic tool in various neurological and psychiatric conditions (Ma et al., 2019). A multicenter, randomized, double-blind trial (VNS-REHAB) conducted by Dawson et al. (2021) confirmed the effectiveness of implanted VNS (iVNS) paired with upper extremity training in patients with ischemic stroke. However, the application process of iVNS may carry a high rate of potential complications due to its invasiveness (Ma et al., 2019). Recently, transcutaneous auricular VNS (taVNS), as a safe and easy-to-use neuromodulation technique, has been proposed, which utilizes transcutaneous stimulation of the cymba conchae and tragus innervated by fibers of the auricular branch of the vagus nerve (ABVN). Neuroimaging studies have demonstrated that taVNS can elicit brain activation effects similar to iVNS (Badran et al., 2018). Several studies (Capone et al., 2017; Redgrave et al., 2018; Wu et al., 2020; Chang et al., 2021; Li et al., 2022) have combined taVNS with regular rehabilitation training or robot-assisted arm training to improve upper extremity function in hemiplegic patients. To ensure consistency in taVNS research, an international consensus has been established for minimum reporting standards, which reported that a signal with a pulse width between 200 and 300 μs at 25 Hz, and a duty cycle of 30 s on, 30 s off has often been adopted in studies (Farmer et al., 2021). Nonetheless, the mechanism by which taVNS achieves task-specific benefits in upper extremity function remains unclear. In this study, we hypothesized that combining taVNS with TOT simultaneously would present a novel approach to enhance upper extremity function recovery in stroke patients. To assess the immediate and long-term effects of taVNS on hemodynamics and cortical excitability, we employed functional near-infrared spectroscopy (fNIRS) and single-pulse transcranial magnetic stimulation (TMS), respectively. The primary objective of this study was to investigate the effects and potential mechanisms of taVNS paired with TOT in facilitating the recovery of upper extremity function in patients with subacute stroke.
2 Materials and methods
2.1 Participants
The stroke patients with upper extremity motor dysfunction were recruited from the Department of Rehabilitation Medicine at Sir Run Run Hospital of Nanjing Medical University between February 2020 and December 2022. The inclusion criteria were as follows: (1) first-time occurrence of a unilateral supratentorial stroke confirmed by computed tomography (CT) or magnetic resonance imaging (MRI) within 6 months of onset; (2) presence of unilateral hemiplegia of the upper extremity; (3) upper extremity impairment ≥ third level in the Functional Test for the Hemiparetic Upper Extremity (FTHUE), and FMA-UE scores ranged from 20 ~ 50; and (4) normal cognitive function with the ability to follow instructions and complete the study. The exclusion criteria were as follows: (1) presence of implanted electronic devices, intracerebral vascular clips, or other electrically activated or sensitive support systems; (2) presence of abnormal skin conditions that may interfere with the stimulation or the stimulation device, such as scar tissue, broken skin; (3) presence of severe cardiovascular, pulmonary, or advanced diseases affecting other systems; (4) previous impairment of the vagus nerve; (5) upper extremity dysfunction caused by reasons other than stroke; (6) use of neuropsychotropic drugs such as antidepressants or benzodiazepines; (7) Botox injection within the past 3 months; (8) resting heart rate below 60 beats per minute; and (9) presence of asthma, tumors, or severe dysphagia. The trial was performed in accordance with the principles of the Declaration of Helsinki and received approval from the Ethics Committee of Sir Run Run Hospital, Nanjing Medical University (No. 2020-SR-001). All participants signed informed consent. Further details regarding the subjects can be found in Table 1.
2.2 Study design
This pilot study was designed as a randomized, double-blind, sham-controlled clinical trial, which followed the CONSORT checklist (Supplementary material S1). The patients were randomly assigned (1:1) to either the VNS group (VG) or the Sham group (SG) using a random number table. The patients in the VG received real taVNS during 1 h of task-oriented training, while the patients in the SG received sham taVNS during task-oriented training. The treatment was delivered 5 ays per week for 4 weeks. To minimize subjective bias, different individuals performed the roles of the TOT therapist, taVNS operator, and outcome assessor. To explore the immediate effect of taVNS, an fNIRS examination with HRV assessment was conducted before the first treatment session. The study flow is shown in Figure 1.
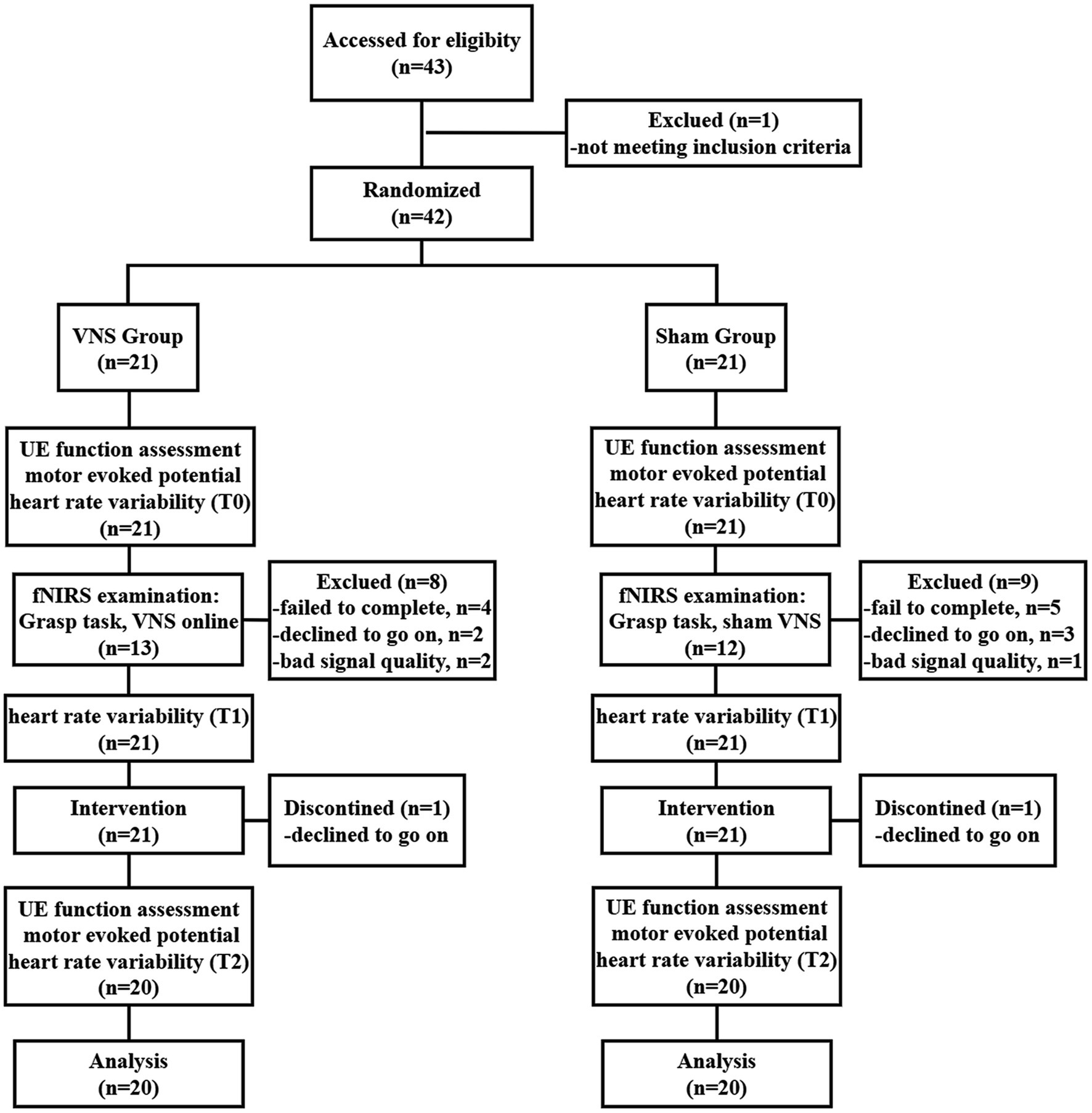
Figure 1. Study flow diagram. VNS: vagus nerve stimulation; UE, Upper extremity; fNIRS, Functional near-infrared spectroscopy.
2.3 Intervention
2.3.1 Transcutaneous auricular vagus nerve stimulation (taVNS)
The taVNS treatment was delivered simultaneously with TOT for 1 h in accordance with Zhang et al. (2023), using the Auricular Vagus Nerve Stimulator (tVNS501, RISHENA Co., Ltd., Changzhou, China). A specialized earphone with two dot-like electrodes delivered electric stimulation to the left auricular cymba concha of patients in the VG. Conversely, patients in the SG wore the same earphones without electrodes. Although they could observe the current reading on the stimulator, no actual current was delivered, resulting in ineffective stimulation. Before stimulation, the left auricular concha of each patient was cleaned using alcohol wipes. According to the international consensus for minimum reporting standards (Farmer et al., 2021), the stimulation parameters were configured as follows: 500 μs square pulses at 25 Hz for 30 s, with a duty cycle of 1:1. The current intensity was individually adjusted to a tolerable level for each patient, ensuring that it did not exceed 10 mA (with an average of 6.55 ± 1.57 mA in the VG) (Capone et al., 2017; Redgrave et al., 2018; Wu et al., 2020; Li et al., 2022).
2.3.2 Task-oriented training (TOT)
Both groups of patients underwent TOT practice, which was supervised or assisted by a well-trained occupational therapist. The TOT practice involved eight exercises, requiring patients to perform as many trials as possible in each session. Each session of task-oriented training lasted for 1 h. The occupational therapist was allowed to adjust the intensity of each exercise based on the patient’s training objectives and functional performance. The detailed steps of the TOT were as follows (Kim et al., 2013; Tang et al., 2014): (1) instruct patients to place their hand on a table with adjustable height; (2) instruct patients to touch the tip of their nose; (3) instruct patients to rotate their forearm and alternate the palm upwards and downwards; (4) instruct patients to bend the elbow to a 90-degree angle, position the forearm neutrally, and extend the wrist joint to touch a designated object on the table as far as possible; (5) while maintaining the same position as in step (4), instruct patients to grip and release a water bottle placed on the table; (6) instruct patients to pick up a cup from the table, simulating a drinking motion, then return it to its original position; (7) instruct patients to pour peanuts from a cup onto a plate on the table without using compensatory trunk movements; (8) instruct patients to manipulate objects with the assistant of a hand-function robot (Gloreha Professional 2, Idrogenet, Italy).
2.4 Outcome measurements
The long-term outcomes were assessed using clinical scales and motor-evoked potentials (MEPs) elicited by single-pulse TMS. The Fugl-Meyer Assessment-Upper Extremity (FMA-UE) and the Action Research Arm Test (ARAT) were employed to evaluate the function of the hemiplegic upper extremity, and the modified Barthel Index (MBI) was used to evaluate the activity of daily living (Chen et al., 2021). The MEPs were applied to evaluate the cortico-spinal excitability. The primary outcome measure was the change score of the Upper Extremity Fugl-Meyer Assessment (UE-FMA). All outcomes were evaluated before the first treatment and after the final treatment. Additionally, in order to explore the feasibility of the current study and assess the immediate effect of taVNS during grasp tasks with the hemiplegic hand, an fNIRS examination was conducted before the first treatment session. HRV data were collected before and after the fNIRS examination (T0, T1) and after the last treatment (T2) to assess the effectiveness of taVNS on the vagus nerve network.
2.4.1 Upper extremity function assessment
The Fugl-Meyer Assessment-Upper Extremity (FMA-UE) is a widely used tool for evaluating upper extremity impairment and coordination/speed in stroke patients. The FMA-UE comprises 33 items, each scored on a scale ranging from 0 to 2, culminating in a total score of 66 points (Gladstone et al., 2002; Rech et al., 2020).
The Action Research Arm Test (ARAT) is a standardized observational scale extensively used to assess the functional abilities of the upper extremity in stroke survivors, closely reflecting their daily activities. The ARAT consists of 19 items categorized into four subtests: grasping, gripping, pinching, and gross movement. Each item is scored on a scale from 0 to 3, with a maximum possible score of 57 (Hsieh et al., 1998; Zhao et al., 2019a, b).
The Modified Barthel Index (MBI) is a frequently used outcome measure to evaluate performance in activities of daily living (ADL) among stroke patients. The MBI comprises 10 items, with a total score of 100 (Shah et al., 1989).
2.4.2 Motor-evoked potentials examination
Motor-evoked potentials (MEPs) refer to the action potentials elicited by single-pulse TMS of the primary motor cortex (M1), providing insight into cortico-spinal excitability. MEP latency refers to the time taken for the motor response to occur after the stimulation of the motor cortex. It is calculated by measuring the time interval between the onset of stimulation and the onset of the MEP waveform (Rossi et al., 2021; Vucic et al., 2023). In certain research, the MEPs have been considered as indicators of motor cortical excitability in stroke patients (Mäkelä et al., 2015; Jo et al., 2016). Therefore, we utilized MEPs to evaluate the long-term effects of the intervention on motor cortical excitability. The examination procedure in this study adhered to established practice guidelines (Fried et al., 2021). A figure-eight coil (Xiangyu Medical Co., Ltd., Henan, China) was placed over the M1 to elicit the MEPs, while surface electromyography (sEMG) was recorded from the first dorsal interosseous (FDI) muscle. The initial intensity was set at 30% of the maximum stimulator output (MSO). Then the intensity was increased by 5% until the minimum stimulus produced minimal motor-evoked responses (≥50 μV in at least 5 out of 10 trials) in the FDI. The average latency and amplitude of the bilateral MEPs were recorded. The detailed information can be found in Supplementary material S2. If MEPs could not be elicited even at 100% MSO, it was recorded as “NA.” The examination was well tolerated by the patient without any adverse events.
2.4.3 Heart rate variability examination
HRV refers to the fluctuation in the time intervals between adjacent heartbeats. The link between vagus nerve activity and HRV has been established, as the heart is innervated by the vagus motor fibers (Shaffer and Ginsberg, 2017; Capilupi et al., 2020). In our study, we conducted HRV examination to determine whether taVNS effectively targeted the vagus nerve. The average heart rate (HR), the standard deviation of the normal-to-normal (NN) intervals (SDNN), and the square root of the mean squared differences of successive NN intervals (RMSSD) were recorded as time-domain measures of HRV. Additionally, the ratio of low-frequency to high-frequency power (LF/HF ratio) was recorded as a frequency-domain measure of HRV. The detailed processing pipelines for HRV can be found in Supplementary material S2. An increase in parasympathetic activity induced by taVNS correlates with increases in SDNN and RMSSD, and decreases in HR and LF/HF ratio (Marek, 1996). We compared the data collected at T0 with that at T1 and T2 to examine the immediate and long-term effectiveness of taVNS. All the patients completed the examination.
2.4.4 Functional near-infrared spectroscopy (fNIRS) examination
fNIRS is a non-invasive brain imaging technique that detects variations in oxyhemoglobin (HbO2) and deoxyhemoglobin (HbR) within the regional cortex. Increased cortical neural activity leads to escalated metabolic demand, resulting in augmented blood flow in the surrounding vasculature, consequently elevating HbO2 concentrations and reducing HbR concentrations (Huppert et al., 2006; Buxton, 2009). Applying specific near-infrared light, fNIRS can measure brain metabolic alterations associated with neuronal activity (Buxton, 2013; Pinti et al., 2020). Studies have shown strong consistency between fNIRS and functional magnetic resonance imaging (fMRI) (Sasai et al., 2012).
This study used a continuous-wave near-infrared imaging device (NIRSmart II-3000A, Huichuang Medical Co., Ltd., China) consisting of 14 light sources (λ1|2 = 730|850 nm) with an average power of <1 mW and 14 avalanche photodiode detectors operating at 11 Hz sampling rate. The sources and detectors were distributed over the bilateral prefrontal cortex (PFC) and sensorimotor cortex (SMC) according to the 10–20 international standard electrode placement system, constituting 35 channels. The montage of the probes and channels is detailed in Figure 2A. The Patriot localization system was employed to determine the Montreal Neurological Institute (MNI) spatial coordinates for each channel and to annotate the corresponding Brodmann areas. The corresponding brain areas for each fNIRS channel are shown in Figure 2B, Supplementary material S3.
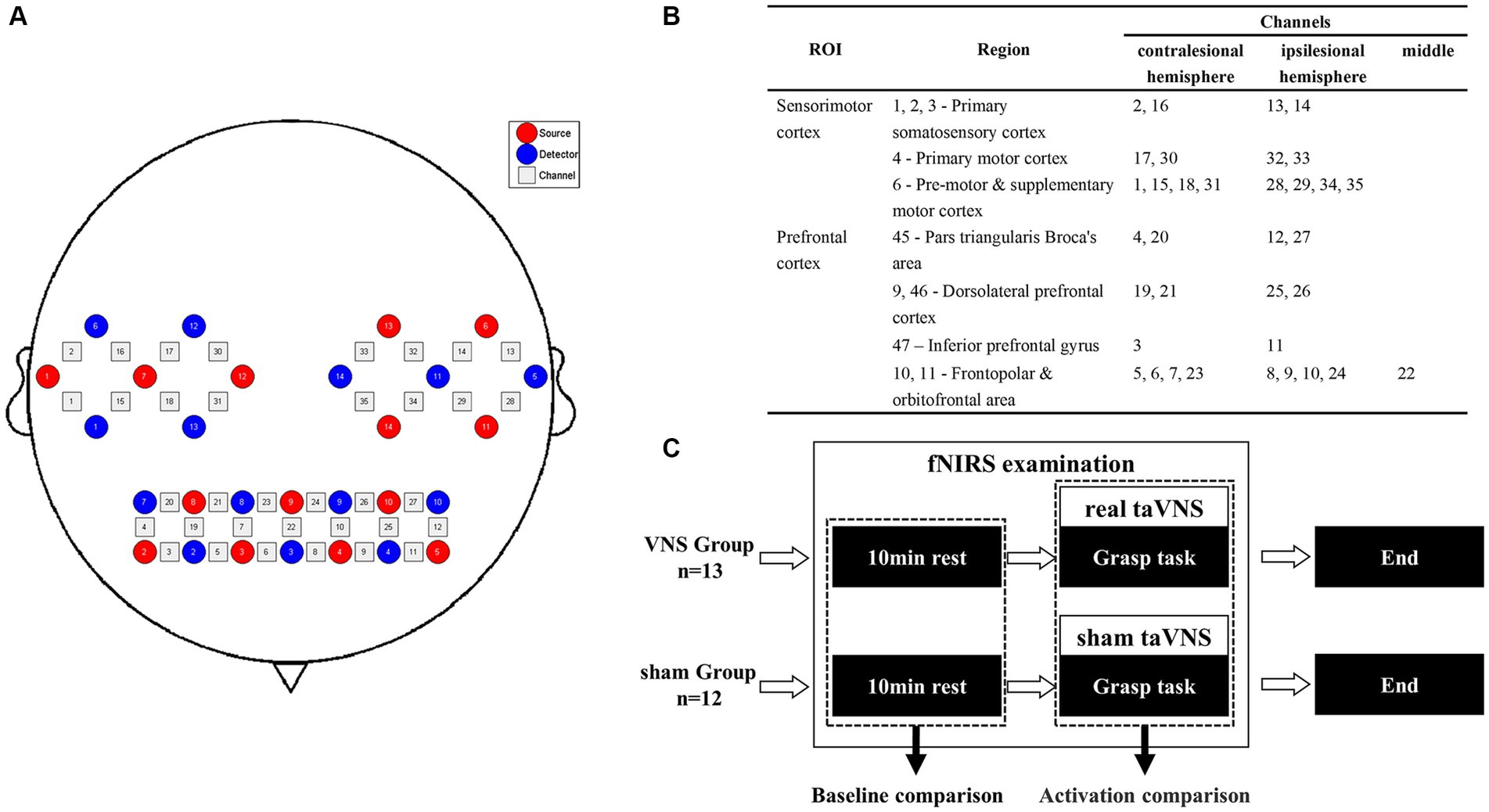
Figure 2. Procedure of the fNIRS examination. (A) Channel arrangements with numbers marked on a 2D brain template. (B) The regions of interest based on the Brodmann area of each channel. (C) Pipeline of the fNIRS examination.
The fNIRS examination was conducted in a quiet room with subdued lighting. To investigate the immediate effect on task-related cortical responses in the patients during the treatment, we simulated a scenario in which patients received taVNS while performing TOT. Initially, a 10-min resting state was recorded, during which patients were instructed to keep their eyes closed, maintain restfulness, and minimize head movements. Subsequently, patients were instructed to perform the grasp task using their hemiplegic hands during the examination, which was practicable and related to the procedure of TOT. The task adopted a block design comprising 20 blocks, each consisting of 15 s of repetitive grasp trials followed by 20 s of rest. Computer-generated auditory cues were provided to guide the patients during the task performance. Patients in the VG received concurrent taVNS, whereas patients in the SG received sham taVNS (Figure 2C).
2.5 Data analysis
2.5.1 fNIRS signal processing
The fNIRS signal was processed using MATLAB R2013b (MathWorks, USA), and the Homer2 package was employed for preprocessing the raw data. The following steps were performed: (1) conversion of light intensity to optical density; (2) detection and correction of motion artifacts; (3) application of a bandpass filter (0.01–0.08 Hz) to the signal; (4) conversion of optical density to concentrations of (de)oxygenated hemoglobin based on the modified Beer–Lambert law. Subsequently, the mean concentration of HbO2 during the resting state was calculated to conduct inter-group baseline comparisons. Additionally, the MATLAB-based NIRS-SPM toolkit was utilized to detect brain area activation. To align the data, patients’ hemispheres were flipped, considering the left hemisphere as the ipsilesional hemisphere. Beta values (β) for each channel were calculated using the general linear model (GLM) analysis. Group-level comparison was conducted using an independent t test with a false discovery rate (FDR) correction. Detailed procedures for data processing were shown in Supplementary material S2. BrainNet Viewer (Xia et al., 2013) was employed for 3D visualization of brain activation, and space registration was performed using the NFRI method to convert the channel space into Montreal Neurological Institute (MNI) space.
2.5.2 Clinical data analysis
The statistical analysis was conducted using the open-source statistical software Jamovi (Version 2.4.8) (JAMOVI, 2023). The Shapiro-Wilks test was performed to assess the normality of the data. For baseline demographic and clinical characteristics, the Chi-square test or Fisher’s exact test was used for categorical variables. The two-sample t-test and the Mann–Whitney test were used for continuous variables. A 2 × 2 analysis of covariance (ANCOVA) was used to determine the effects of the treatment on the FMA-UE, ARAT, MBI, and contralesional MEPs parameters, considering time (baseline, post-treatment) as the within-subject factor, group (VNS, Sham) as the between-subjects factor, and baseline data as a covariate. Due to the impairment of cortico-spinal tract integrity after stroke (Yarossi et al., 2019), the statistical analysis for ipsilesional MEP was determined based on the number of MEPs collected. A 3 × 2 repeated measures analysis of variance (RMANOVA) was conducted to determine the effects on the HRV, considering time (T0, T1, T2) as the between-subjects factor and group (VNS, Sham) as the between-subjects factor. A linear regression analysis was used to analyze the correlation between the recovery of upper extremity function and changes in MEPs. A significance level of p < 0.05 was considered statistically significant for all tests.
3 Results
Forty-three patients were recruited, with 21 allocated to the VG and 21 to the SG. The flow chart can be found in Figure 1. Two participants (one from the VG and another from the SG) dropped out of the study due to unwillingness. In addition, eight participants in the VG and nine participants in the SG were excluded from the fNIRS data analysis. Detailed reasons for participants’ exclusion are shown in Figure 1.
3.1 Demographics and clinical characteristics
Table 1 presents a summary of the demographics and clinical characteristics of the patients. There were no statistically significant differences in any of the variables between the two groups. Furthermore, no adverse events were reported throughout the entire duration of the study.
3.2 Outcomes for upper extremity function
The ANCOVA revealed that patients in both groups showed significant improvements in FMA-UE, ARAT, MBI compared to baseline. Regarding the inter-group comparison, there were statistically greater improvements in FMA-UE, ARAT, and MBI in the VG (Figure 3, Table 2).
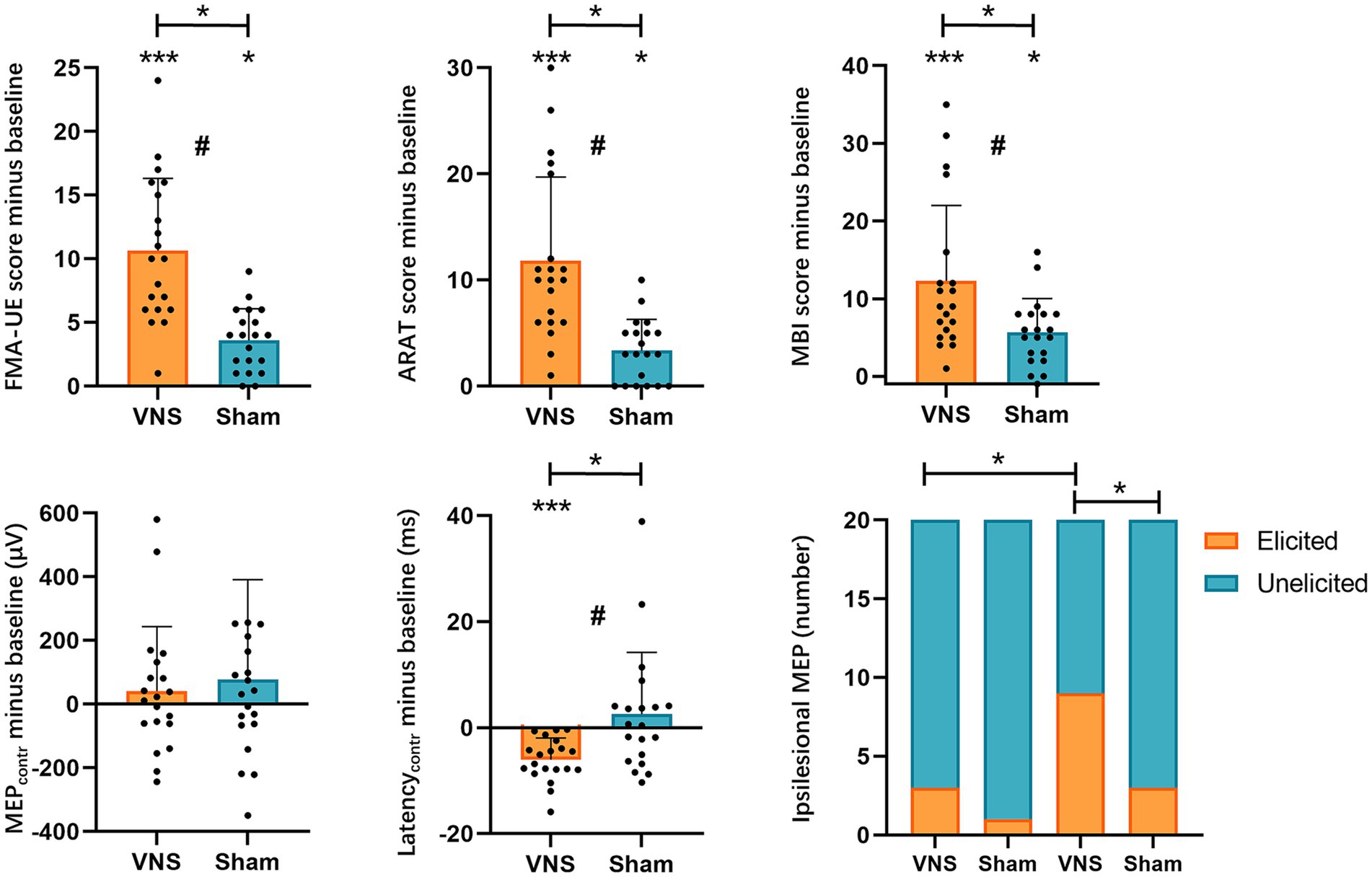
Figure 3. Effects of taVNS on upper extremity function and MEPs. FMA-UE, Fugl-Meyer Assessment-Upper Extremity; ARAT, Action Research Arm Test; MBI, modified Barthel Index; MEPs, motor-evoked potentials; VNS, taVNS group; Sham: Sham group; *, p < 0.05; **, p < 0.01; ***, p < 0.001; #, a significant time × intervention interaction in ANCOVA.
3.3 Outcomes for MEPs
In our study, only 4 patients were able to elicit ipsilesional MEPs at baseline (VG, n = 3; SG, n = 1). After 20 sessions of treatment, the number of patients who could elicit ipsilesional MEPs increased to 12 cases (VG, n = 9; SG, n = 3). To determine the effects on the elicitation rate of ipsilesional MEPs, we employed the Chi-square test or Fisher’s exact test for between-group comparison and the McNemar test for within-group comparison. The Fisher’s exact test indicated that the baseline elicited ipsilesional MEPs were comparable across groups. After treatment, the VG showed significantly higher elicitation rates of ipsilesional MEPs than baseline and SG (Figure 3, Table 3).
The contralesional MEPs were collected from all included patients. The ANCOVA found that the contralesional latency of MEPs in the VG significantly shortened compared with baseline. While no significant change in the SG was detected. There was a statistically significant reduction in contralesional latency of MEPs in the VG (Figure 3, Table 4). However, no significant difference was found in contralesional amplitude of MEPs within or between groups (Figure 3, Table 4). Additionally, the univariable regression revealed that there were significant correlations between the improvements in FMA-UE and the changes in the contralesional latency of MEPs (Figure 4).
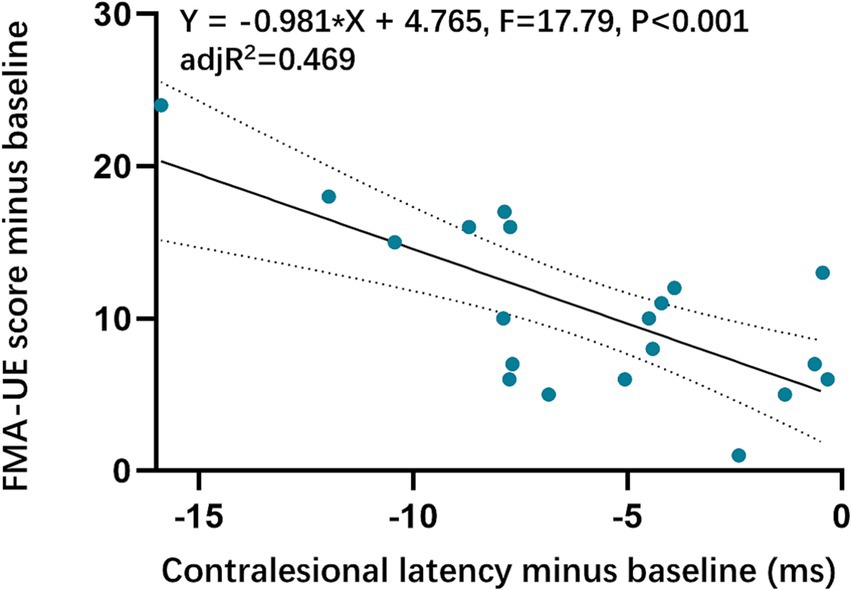
Figure 4. Correlations between Upper extremity function and contralesional MEPs latency. FMA-UE, the Fugl-Meyer Assessment-Upper Extremity.
3.4 Outcomes for fNIRS
The resting state fNIRS data showed there were no significant differences in baseline. As for task fNIRS data, group-level comparison revealed that the channels in the ipsilesional postcentral gyrus (PoCGipsi, CH14), precentral gyrus (PreCGipsi, CH32), supplementary motor area (SMAipsi, CH34), middle frontal gyrus orbital part (ORBmidipsi, CH9), contralesional orbital middle frontal gyrus (ORBmidcontr, CH5, CH7, CH23), and dorsolateral superior frontal gyrus (SFGdorcontr, CH21) showed significantly larger activation in the VG compared to the SG (pFDR < 0.05). The specific coordination of activated channels is shown in Figure 5.
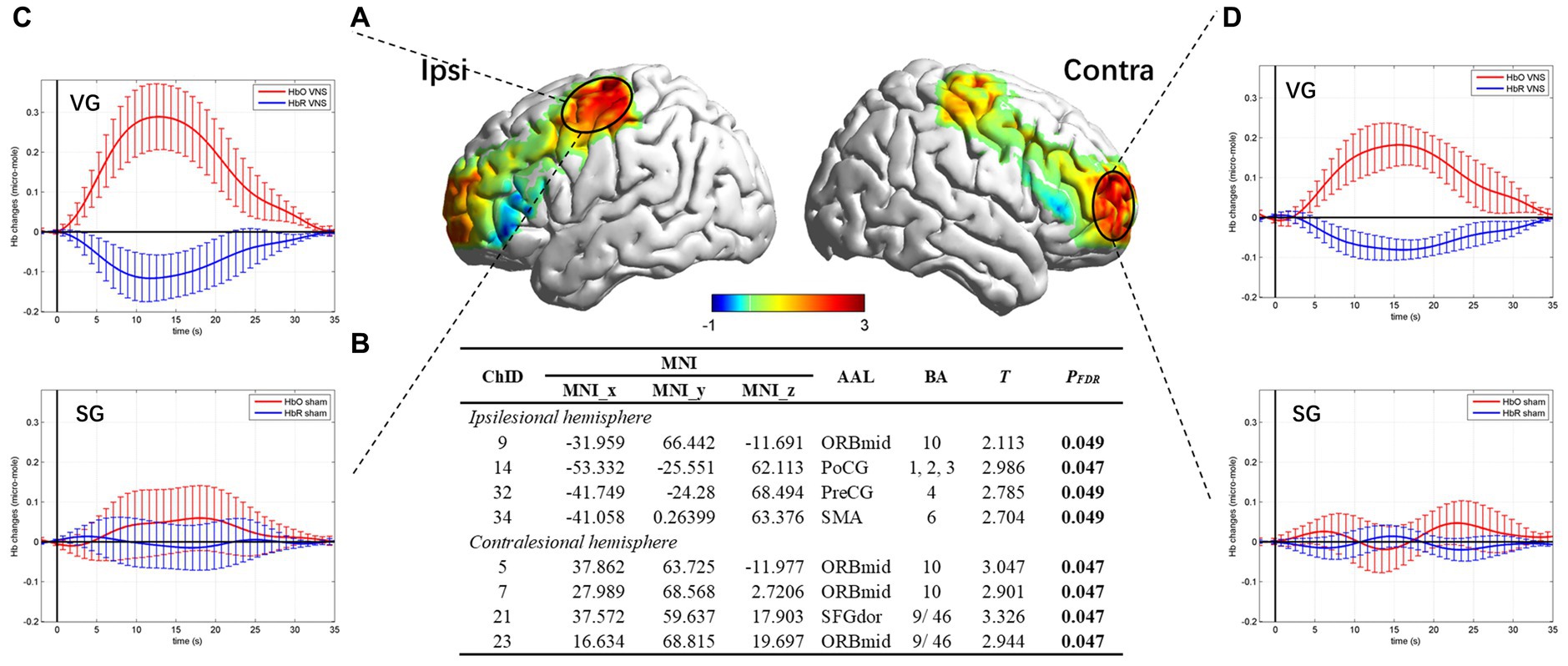
Figure 5. Brain activation map and hemodynamics response for task state. (A) Cortical activation map marked on a three-dimensional template (Colin 27 version 2019, using the interpolated mapping algorithm) in ipsilesional and contralesional vision. (B) Results from the independent t-test of beta values between the VG and the SG. Channels with a significant difference were listed. (C) Hemodynamic curve in PoCGipsi. (D) Hemodynamic curve in MFGcontr.
3.5 Outcomes for HRV
The 3 × 2 RMANOVA revealed significant group*time interactions for HR, SDNN, and LF/HF, but not for RMSSD. We also found significant group main effects in HR and LF/HF. For the interaction effect, Bonferroni post hoc tests showed that patients in the VG presented significantly decreased HR at T1, T2 compared with T0, increased SDNN at T2 compared with T0, and decreased LF/HF at T1, T2 compared with T0. However, no between-group difference was found after Bonferroni correction (Figure 6, Table 5).
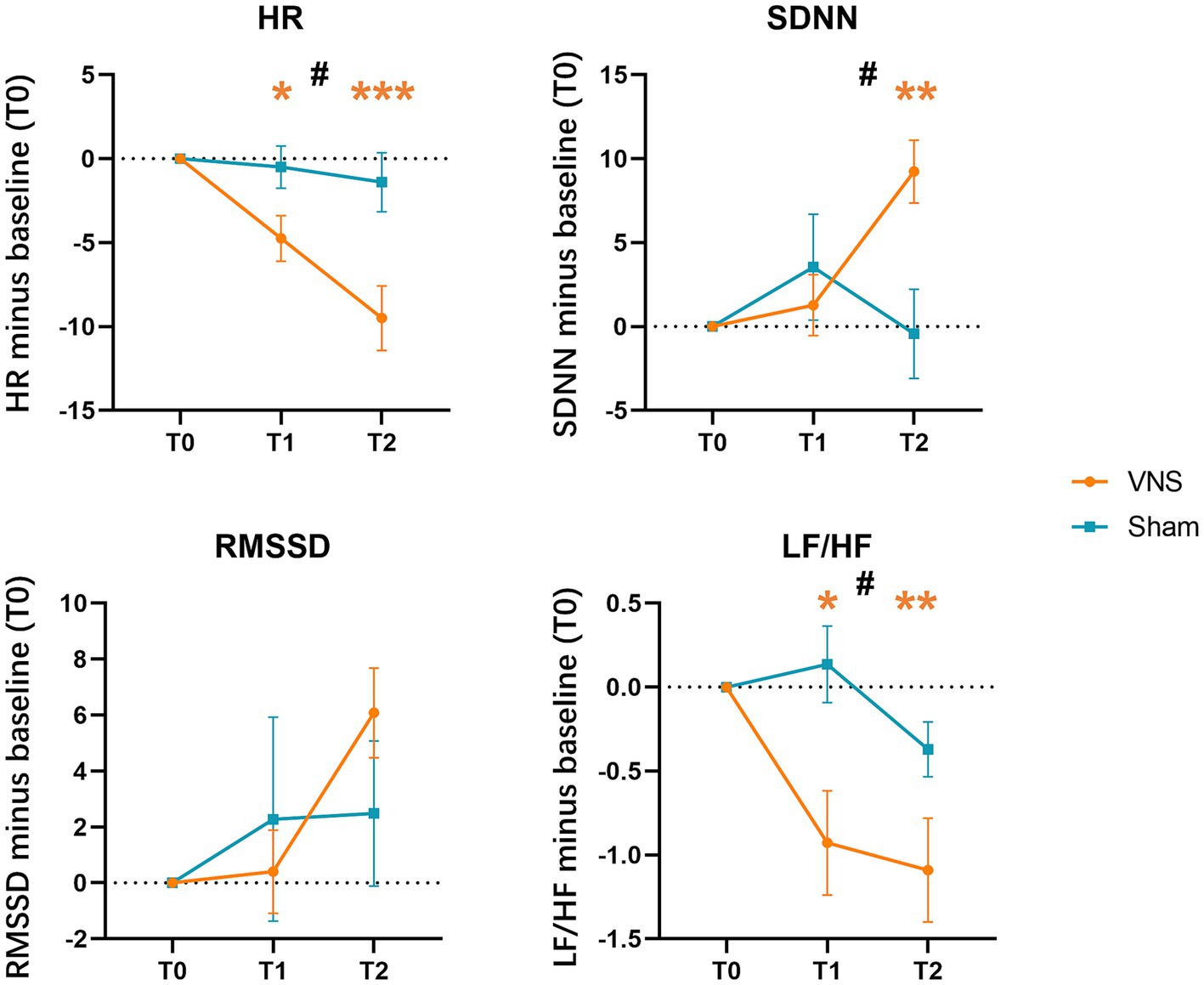
Figure 6. Effects of taVNS on heart rate variability. HR, averaged heart rate; SDNN, the standard deviation of the normal-to-normal intervals; RMSSD, the square root of the mean squared differences of successive normal-to-normal intervals; LF/HF, the ratio of low-frequency to high-frequency power; * in orange color: p < 0.05 compared with T0 in VNS group; ** in orange color: p < 0.01 compared with T0 in VNS group; *** in orange color: p < 0.001 compared with T0 in VNS group; #: a significant time × intervention interaction in repeated-measures ANOVA.
4 Discussion
In recent years, non-invasive VNS has garnered increasing attention due to its potential to improve upper extremity motor function in stroke patients, although its underlying neural mechanisms are not fully understood. In order to explore the feasibility of the current study, we conducted HRV and fNIRS examinations prior to the initiation of the treatment. The effectiveness of taVNS was assessed using HRV examination. Our current results demonstrated significant decreases in HR, LF/HF ratio, and a marked increase in SDNN in the VG, whereas no changes were observed in the SG. These results indicated that taVNS indeed modulated the vagus nerve network. Subsequently, using fNIRS, we observed greater hemodynamic responses in the bilateral PFC and the ipsilesional SMC during the grasp task with taVNS, suggesting an enhancement of cortical activation. In the fNIRS study conducted by Kunii et al. (2021), the effects of iVNS on cerebral blood flow (CBF) during a resting state and a verbal fluency task were investigated. They found that no changes in CBF were observed during the resting state, while the verbal fluency task led to a significant increase in CBF. Wang et al. (2023) found that a single session of taVNS could significantly bolster the activation within damaged cerebral territories in stroke patients without destabilizing cerebral lateralization. These findings provide evidence that taVNS can effectively activate the task-specific cortex during motor task performance, supporting the immediate effect of taVNS.
The TOT approach aims to teach stroke patients specific task strategies and improve their ability to adapt to the environment through functional tasks related to daily life. Previous fMRI studies (Jang et al., 2003; McCombe Waller et al., 2014) have reported that TOT could improve upper extremity function in post-stroke patients. These studies found that activity in the related motor cortex increased with the recovery of upper extremity motor function. In our study, we observed improvements in the motor capacity of the upper extremity (FMA-UE), arm-hand capacity (ARAT), and activities of daily living (MBI) in both groups. However, patients in the taVNS group demonstrated greater improvements in upper extremity function, which is consistent with previous research (Hoonhorst et al., 2015; Capone et al., 2017; Redgrave et al., 2018; Chang et al., 2021; Li et al., 2022). Notably, the improvement observed in the VG was clinically significant, surpassing the minimal clinically important difference (MCID) of 9–10 points for the FMA-UE in individuals with subacute stroke (Singer and Garcia-Vega, 2017). Khodaparast et al. (2016) observed that the rate of forelimb strength recovery (86%) in ischemic stroke rats was significantly higher after receiving VNS with rehabilitation training compared to the simple rehabilitation group (47%) and the delayed VNS group (42%). Morrison et al. (2019, 2020) reported that intracortical microstimulation with VNS can improve motor cortical plasticity in mice. In this study, significant improvements were observed in upper extremity motor function, indicating that taVNS paired with task-specific activities may promote neuroplastic changes.
Neuroanatomy research has provided insights into the mechanisms underlying the activation of the locus ceruleus-noradrenaline (LC-NE) release system by taVNS. Norepinephrine (NE) is an excitatory neurotransmitter, while the locus coeruleus (LC) is the primary source of the norepinephrine-producing neurons in the brain. Studies indicated that both short-term and long-term VNS can increase neuronal firing rates of the LC, leading to increased NE concentrations in the amygdala, hippocampus, and prefrontal cortex. The VNS-induced NE could maintain long-term activity (Groves et al., 2005; Hulsey et al., 2017). NE can enhance cortical excitability and plasticity, which are associated with daytime vigilance, attention, and motor learning (Duffau, 2006; Ciampa et al., 2022). The fMRI studies by Kraus et al. (2007) and Dietrich et al. (2008) have detected blood oxygenation level-dependent (BOLD) signal activations in the bilateral sensorimotor cortex and prefrontal cortex during taVNS. Furthermore, Frangos and Komisaruk (2017) observed sustained activation of the bilateral precentral gyrus for nearly 10 min (9 min, in fact) following taVNS. Combining these findings with the results of fNIRS in our trial, it is plausible to speculate that taVNS can induce bilateral hemisphere activation during the treatment, which may be attributable to the related neurotransmitter release.
In this study, we utilized single-pulse TMS to assess long-term changes in motor cortex excitability by measuring MEPs in both hemispheres. The study found a relatively low elicitation rate of ipsilesional MEPs in patients at baseline. However, the elicitation rate of ipsilesional MEPs increased significantly from 15% before treatment to 45% after treatment in the VG, which is consistent with previous studies (Escudero et al., 1998; Powell et al., 2019; Yarossi et al., 2019). Moreover, the average latency of ipsilesional MEPs in the VG decreased from 63.53 ms to 55.36 ms, while the amplitude increased from 87.60 μV to 140.18 μV, suggesting a trend toward increased cortical excitability in the ipsilesional M1. Cakar et al. (2016) reported a positive correlation between shorter MEP latency, increased amplitude, and improved motor function in stroke patients. Additionally, we observed a reduction in the latency of contralesional MEPs, indicating the modulation of the contralesional M1. Increased intracortical excitability of contralesional M1 has been observed in subacute and chronic stroke patients (Bütefisch, 2003; Bütefisch et al., 2008), with suggestions that the increased excitability is associated with better recovery of upper extremity function (Bütefisch, 2015). Bi-hemispheric transcranial direct current stimulation (tDCS) studies using fMRI have supported this notion, proposing a cooperative role of contralesional M1 rather than competition (Waters et al., 2017). Furthermore, a randomized controlled trial conducted by Hsu et al. (2023) demonstrated that improvements in FMA-UE scores in subacute stroke patients correlated with changes in functional connectivity within the bilateral intra-hemisphere networks. In our study, we found a significant correlation between the decrease in latency of contralesional MEPs and improvements in FMA-UE scores, which is consistent with previous literature (Wang et al., 2020). Based on these findings, the long-term combined intervention may modulate bilateral motor cortical excitability and facilitate training-specific motor performance.
5 Limitations
This study has several limitations that need to be addressed in future research. First, the small sample size and the high dropout rate of the fNIRS examination in this study might result in insufficient statistical power, potentially increasing the chance of a type I error. A larger sample size is needed to confirm these findings and achieve more reliable results. Second, it is worth noting that, due to the limited number of positive events (elicited ipsilesional MEPs), the significance and generalizability of this result should be carefully considered. Finally, follow-up assessments were not conducted to investigate the sustained effects of taVNS in this study, which should be considered in future studies.
6 Conclusion
In conclusion, our study provides evidence that the combined intervention of taVNS with TOT is effective in enhancing upper extremity motor function in patients with post-stroke hemiplegia. This improvement can be attributed to the modulation of cortical excitability in both hemispheres, which facilitates the remodeling of motor function.
Data availability statement
The raw data supporting the conclusions of this article will be made available by the authors, without undue reservation.
Ethics statement
The studies involving humans were approved by the Ethics Committee of Sir Run Run Hospital, Nanjing Medical University (No. 2020-SR-001). The studies were conducted in accordance with the local legislation and institutional requirements. The participants provided their written informed consent to participate in this study.
Author contributions
M-HW: Conceptualization, Data curation, Formal analysis, Software, Visualization, Writing – original draft. Y-XW Conceptualization, Writing – review & editing. MX: Investigation, Methodology, Validation, Writing – review & editing. L-YC: Investigation, Methodology, Writing – review & editing. M-FH: Investigation, Methodology, Writing – review & editing. FL: Conceptualization, Project administration, Supervision, Writing – review & editing. Z-LJ: Conceptualization, Funding acquisition, Project administration, Resources, Supervision, Writing – review & editing.
Funding
The author(s) declare that financial support was received for the research, authorship, and/or publication of this article. This work was supported by the National Key R&D Program of China (grant numbers No.2020YFC2008505).
Acknowledgments
The authors thank all the individuals who participated in this study.
Conflict of interest
The authors declare that the research was conducted in the absence of any commercial or financial relationships that could be construed as a potential conflict of interest.
Publisher’s note
All claims expressed in this article are solely those of the authors and do not necessarily represent those of their affiliated organizations, or those of the publisher, the editors and the reviewers. Any product that may be evaluated in this article, or claim that may be made by its manufacturer, is not guaranteed or endorsed by the publisher.
Supplementary material
The Supplementary material for this article can be found online at: https://www.frontiersin.org/articles/10.3389/fnins.2024.1346634/full#supplementary-material
References
Alsubiheen, A. M., Choi, W., Yu, W., and Lee, H. (2022). The effect of task-oriented activities training on upper-limb function, daily activities, and quality of life in chronic stroke patients: a randomized controlled trial. Int. J. Environ. Res. Public Health 19:14125. doi: 10.3390/ijerph192114125
Badran, B. W., Dowdle, L. T., Mithoefer, O. J., LaBate, N. T., Coatsworth, J., Brown, J. C., et al. (2018). Neurophysiologic effects of transcutaneous auricular vagus nerve stimulation (taVNS) via electrical stimulation of the tragus: a concurrent taVNS/fMRI study and review. Brain Stimul. 11, 492–500. doi: 10.1016/j.brs.2017.12.009
Bai, Z., Zhang, J., and Fong, K. N. K. (2022). Effects of transcranial magnetic stimulation in modulating cortical excitability in patients with stroke: a systematic review and meta-analysis. J. Neuroeng. Rehabil. 19:24. doi: 10.1186/s12984-022-00999-4
Bütefisch, C. M. (2003). Remote changes in cortical excitability after stroke. Brain 126, 470–481. doi: 10.1093/brain/awg044
Bütefisch, C. M. (2015). Role of the Contralesional hemisphere in post-stroke recovery of upper extremity motor function. Front. Neurol. 6:214. doi: 10.3389/fneur.2015.00214
Bütefisch, C. M., Wessling, M., Netz, J., Seitz, R. J., and Hömberg, V. (2008). Relationship between interhemispheric inhibition and motor cortex excitability in subacute stroke patients. Neurorehabil. Neural Repair 22, 4–21. doi: 10.1177/1545968307301769
Buxton, R. B.. Introduction to functional magnetic resonance imaging: principles and techniques. Cambridge; New York: Cambridge University Press (2009).
Buxton, R. B. (2013). The physics of functional magnetic resonance imaging (fMRI). Rep. Prog. Phys. 76:096601. doi: 10.1088/0034-4885/76/9/096601
Cakar, E., Akyuz, G., Durmus, O., Bayman, L., Yagci, I., Karadag-Saygi, E., et al. (2016). The relationships of motor-evoked potentials to hand dexterity, motor function, and spasticity in chronic stroke patients: a transcranial magnetic stimulation study. Acta Neurol. Belg. 116, 481–487. doi: 10.1007/s13760-016-0633-2
Capilupi, M. J., Kerath, S. M., and Becker, L. B. (2020). Vagus nerve stimulation and the cardiovascular system. Cold Spring Harb. Perspect. Med. 10:a034173. doi: 10.1101/cshperspect.a034173
Capone, F., Miccinilli, S., Pellegrino, G., Zollo, L., Simonetti, D., Bressi, F., et al. (2017). Transcutaneous Vagus nerve stimulation combined with robotic rehabilitation improves upper limb function after stroke. Neural Plast. 2017, 1–6. doi: 10.1155/2017/7876507
Chang, J. L., Coggins, A. N., Saul, M., Paget-Blanc, A., Straka, M., Wright, J., et al. (2021). Transcutaneous auricular Vagus nerve stimulation (tAVNS) delivered during upper limb interactive robotic training demonstrates novel antagonist control for reaching movements following stroke. Front. Neurosci. 15:767302. doi: 10.3389/fnins.2021.767302
Chen, Z. J., He, C., Gu, M. H., Xu, J., and Huang, X. L. (2021). Kinematic evaluation via inertial measurement unit associated with upper extremity motor function in subacute stroke: a cross-sectional study. J. Healthc. Eng. 2021, 1–7. doi: 10.1155/2021/4071645
Chhatbar, P. Y., Chen, R., Deardorff, R., Dellenbach, B., Kautz, S. A., George, M. S., et al. (2017). Safety and tolerability of transcranial direct current stimulation to stroke patients -a phase I current escalation study. Brain Stimul. 10, 553–559. doi: 10.1016/j.brs.2017.02.007
Ciampa, C. J., Parent, J. H., Harrison, T. M., Fain, R. M., Betts, M. J., Maass, A., et al. (2022). Associations among locus coeruleus catecholamines, tau pathology, and memory in aging. Neuropsychopharmacology 47, 1106–1113. doi: 10.1038/s41386-022-01269-6
Dawson, J., Liu, C. Y., Francisco, G. E., Cramer, S. C., Wolf, S. L., Dixit, A., et al. (2021). Vagus nerve stimulation paired with rehabilitation for upper limb motor function after ischaemic stroke (VNS-REHAB): a randomised, blinded, pivotal, device trial. Lancet 397, 1545–1553. doi: 10.1016/S0140-6736(21)00475-X
Dietrich, S., Smith, J., Scherzinger, C., Hofmann-Preiß, K., Freitag, T., Eisenkolb, A., et al. (2008). A novel transcutaneous vagus nerve stimulation leads to brainstem and cerebral activations measured by functional MRI. Biomed. Tech. 53, 104–111. doi: 10.1515/BMT.2008.022
Duffau, H. (2006). Brain plasticity: from pathophysiological mechanisms to therapeutic applications. J. Clin. Neurosci. 13, 885–897. doi: 10.1016/j.jocn.2005.11.045
Escudero, J. V., Sancho, J., Bautista, D., Escudero, M., and López-Trigo, J. (1998). Prognostic value of motor evoked potential obtained by transcranial magnetic brain stimulation in motor function recovery in patients with acute ischemic stroke. Stroke 29, 1854–1859. doi: 10.1161/01.STR.29.9.1854
Farmer, A. D., Strzelczyk, A., Finisguerra, A., Gourine, A. V., Gharabaghi, A., Hasan, A., et al. (2021). International consensus based review and recommendations for minimum reporting standards in research on transcutaneous Vagus nerve stimulation (version 2020). Front. Hum. Neurosci. 14:568051. doi: 10.3389/fnhum.2020.568051
Frangos, E., and Komisaruk, B. R. (2017). Access to vagal projections via cutaneous electrical stimulation of the neck: fMRI evidence in healthy humans. Brain Stimul. 10, 19–27. doi: 10.1016/j.brs.2016.10.008
Fried, P. J., Santarnecchi, E., Antal, A., Bartres-Faz, D., Bestmann, S., Carpenter, L. L., et al. (2021). Training in the practice of noninvasive brain stimulation: recommendations from an IFCN committee. Clin. Neurophysiol. 132, 819–837. doi: 10.1016/j.clinph.2020.11.018
GBD 2019 Stroke Collaborators (2021). Global, regional, and national burden of stroke and its risk factors, 1990-2019: a systematic analysis for the global burden of disease study 2019. Lancet Neurol. 20, 795–820. doi: 10.1016/S1474-4422(21)00252-0
Gladstone, D. J., Danells, C. J., and Black, S. E. (2002). The fugl-meyer assessment of motor recovery after stroke: a critical review of its measurement properties. Neurorehabil. Neural Repair 16, 232–240. doi: 10.1177/154596802401105171
Groves, D. A., Bowman, E. M., and Brown, V. J. (2005). Recordings from the rat locus coeruleus during acute vagal nerve stimulation in the anaesthetised rat. Neurosci. Lett. 379, 174–179. doi: 10.1016/j.neulet.2004.12.055
Higgins, J., Koski, L., and Xie, H. (2013). Combining rTMS and task-oriented training in the rehabilitation of the arm after stroke: a pilot randomized controlled trial. Stroke Res. Treat. 2013:539146, 1–8. doi: 10.1155/2013/539146
Hoonhorst, M. H., Nijland, R. H., van den Berg, J. S., Emmelot, C. H., Kollen, B. J., and Kwakkel, G. (2015). How do Fugl-Meyer arm motor scores relate to dexterity according to the action research arm test at 6 months Poststroke? Arch. Phys. Med. Rehabil. 96, 1845–1849. doi: 10.1016/j.apmr.2015.06.009
Hsieh, C. L., Hsueh, I. P., Chiang, F. M., and Lin, P. H. (1998). Inter-rater reliability and validity of the action research arm test in stroke patients. Age Ageing 27, 107–113. doi: 10.1093/ageing/27.2.107
Hsu, S.-P., Lu, C.-F., Lin, B.-F., Tang, C.-W., Kuo, I.-J., Tsai, Y.-A., et al. (2023). Effects of bihemispheric transcranial direct current stimulation on motor recovery in subacute stroke patients: a double-blind, randomized sham-controlled trial. J. Neuroeng. Rehabil. 20:27. doi: 10.1186/s12984-023-01153-4
Hulsey, D. R., Riley, J. R., Loerwald, K. W., Rennaker, R. L., Kilgard, M. P., and Hays, S. A. (2017). Parametric characterization of neural activity in the locus coeruleus in response to vagus nerve stimulation. Exp. Neurol. 289, 21–30. doi: 10.1016/j.expneurol.2016.12.005
Huppert, T. J., Hoge, R. D., Diamond, S. G., Franceschini, M. A., and Boas, D. A. (2006). A temporal comparison of BOLD, ASL, and NIRS hemodynamic responses to motor stimuli in adult humans. NeuroImage 29, 368–382. doi: 10.1016/j.neuroimage.2005.08.065
JAMOVI. Open statistical software for the desktop and cloud. (2023) Available at:https://www.jamovi.org/.
Jang, S. H., Kim, Y.-H., Cho, S.-H., Lee, J.-H., Park, J.-W., and Kwon, Y.-H. (2003). Cortical reorganization induced by task-oriented training in chronic hemiplegic stroke patients. Neuroreport 14, 137–141. doi: 10.1097/00001756-200301200-00025
Jin, M., Zhang, Z., Bai, Z., and Fong, K. N. K. (2019). Timing-dependent interaction effects of tDCS with mirror therapy on upper extremity motor recovery in patients with chronic stroke: a randomized controlled pilot study. J. Neurol. Sci. 405:116436. doi: 10.1016/j.jns.2019.116436
Jo, J. Y., Lee, A., Kim, M. S., Park, E., Chang, W. H., Shin, Y. I., et al. (2016). Prediction of motor recovery using quantitative parameters of motor evoked potential in patients with stroke. Ann. Rehabil. Med. 40, 806–815. doi: 10.5535/arm.2016.40.5.806
Khodaparast, N., Kilgard, M. P., Casavant, R., Ruiz, A., Qureshi, I., Ganzer, P. D., et al. (2016). Vagus nerve stimulation during rehabilitative training improves forelimb recovery after chronic ischemic stroke in rats. Neurorehabil. Neural Repair 30, 676–684. doi: 10.1177/1545968315616494
Kim, T. H., In, T. S., and Cho, H. (2013). Task-related training combined with transcutaneous electrical nerve stimulation promotes upper limb functions in patients with chronic stroke. Tohoku J. Exp. Med. 231, 93–100. doi: 10.1620/tjem.231.93
Kraus, T., Hösl, K., Kiess, O., Schanze, A., Kornhuber, J., and Forster, C. (2007). BOLD fMRI deactivation of limbic and temporal brain structures and mood enhancing effect by transcutaneous vagus nerve stimulation. J. Neural Transm. (Vienna) 114, 1485–1493. doi: 10.1007/s00702-007-0755-z
Kunii, N., Koizumi, T., Kawai, K., Shimada, S., and Saito, N. (2021). Vagus nerve stimulation amplifies task-induced cerebral blood flow increase. Front. Hum. Neurosci. 15:726087. doi: 10.3389/fnhum.2021.726087
Kwakkel, G., Kollen, B. J., van der Grond, J., and Prevo, A. J. H. (2003). Probability of regaining dexterity in the flaccid upper limb: impact of severity of paresis and time since onset in acute stroke. Stroke 34, 2181–2186. doi: 10.1161/01.STR.0000087172.16305.CD
Lai, S.-M., Studenski, S., Duncan, P. W., and Perera, S. (2002). Persisting consequences of stroke measured by the stroke impact scale. Stroke 33, 1840–1844. doi: 10.1161/01.str.0000019289.15440.f2
Li, J.-N., Xie, C.-C., Li, C.-Q., Zhang, G.-F., Tang, H., Jin, C.-N., et al. (2022). Efficacy and safety of transcutaneous auricular vagus nerve stimulation combined with conventional rehabilitation training in acute stroke patients: a randomized controlled trial conducted for 1 year involving 60 patients. Neural Regen. Res. 17, 1809–1813. doi: 10.4103/1673-5374.332155
Ma, J., Qiao, P., Li, Q., Wang, Y., Zhang, L., Yan, L.-J., et al. (2019). Vagus nerve stimulation as a promising adjunctive treatment for ischemic stroke. Neurochem. Int. 131:104539. doi: 10.1016/j.neuint.2019.104539
Mäkelä, J. P., Lioumis, P., Laaksonen, K., Forss, N., Tatlisumak, T., Kaste, M., et al. (2015). Cortical excitability measured with nTMS and MEG during stroke recovery. Neural Plast. 2015, 1–8. doi: 10.1155/2015/309546
Marek, M. (1996). Heart rate variability: standards of measurement, physiological interpretation, and clinical use. Task force of the European Society of Cardiology and the north American Society of Pacing and Electrophysiology. Circulation 17, 354–381.
McCombe Waller, S., Whitall, J., Jenkins, T., Magder, L. S., Hanley, D. F., Goldberg, A., et al. (2014). Sequencing bilateral and unilateral task-oriented training versus task oriented training alone to improve arm function in individuals with chronic stroke. BMC Neurol. 14:236. doi: 10.1186/s12883-014-0236-6
Morrison, R. A., Danaphongse, T. T., Pruitt, D. T., Adcock, K. S., Mathew, J. K., Abe, S. T., et al. (2020). A limited range of vagus nerve stimulation intensities produce motor cortex reorganization when delivered during training. Behav. Brain Res. 391:112705. doi: 10.1016/j.bbr.2020.112705
Morrison, R. A., Hulsey, D. R., Adcock, K. S., Rennaker, R. L., Kilgard, M. P., and Hays, S. A. (2019). Vagus nerve stimulation intensity influences motor cortex plasticity. Brain Stimul. 12, 256–262. doi: 10.1016/j.brs.2018.10.017
Nichols-Larsen, D. S., Clark, P. C., Zeringue, A., Greenspan, A., and Blanton, S. (2005). Factors influencing stroke survivors’ quality of life during subacute recovery. Stroke 36, 1480–1484. doi: 10.1161/01.STR.0000170706.13595.4f
Pinti, P., Tachtsidis, I., Hamilton, A., Hirsch, J., Aichelburg, C., Gilbert, S., et al. (2020). The present and future use of functional near-infrared spectroscopy (fNIRS) for cognitive neuroscience. Ann. N. Y. Acad. Sci. 1464, 5–29. doi: 10.1111/nyas.13948
Powell, E. S., Westgate, P. M., Goldstein, L. B., and Sawaki, L. (2019). Absence of motor-evoked potentials does not predict poor recovery in patients with severe-moderate stroke: an exploratory analysis. Arch. Rehabil. Res. Clin. Transl. 1:100023. doi: 10.1016/j.arrct.2019.100023
Rech, K. D., Salazar, A. P., Marchese, R. R., Schifino, G., Cimolin, V., and Pagnussat, A. S. (2020). Fugl-Meyer assessment scores are related with kinematic measures in people with chronic hemiparesis after stroke. J. Stroke Cerebrovasc. Dis. 29:104463. doi: 10.1016/j.jstrokecerebrovasdis.2019.104463
Redgrave, J. N., Moore, L., Oyekunle, T., Ebrahim, M., Falidas, K., Snowdon, N., et al. (2018). Transcutaneous auricular Vagus nerve stimulation with concurrent upper limb repetitive task practice for Poststroke motor recovery: a pilot study. J. Stroke Cerebrovasc. Dis. 27, 1998–2005. doi: 10.1016/j.jstrokecerebrovasdis.2018.02.056
Rossi, S., Antal, A., Bestmann, S., Bikson, M., Brewer, C., Brockmöller, J., et al. (2021). Safety and recommendations for TMS use in healthy subjects and patient populations, with updates on training, ethical and regulatory issues: expert guidelines. Clin. Neurophysiol. 132, 269–306. doi: 10.1016/j.clinph.2020.10.003
Sasai, S., Homae, F., Watanabe, H., Sasaki, A. T., Tanabe, H. C., Sadato, N., et al. (2012). A NIRS-fMRI study of resting state network. NeuroImage 63, 179–193. doi: 10.1016/j.neuroimage.2012.06.011
Shaffer, F., and Ginsberg, J. P. (2017). An overview of heart rate variability metrics and norms. Front. Public Health 5:258. doi: 10.3389/fpubh.2017.00258
Shah, S., Vanclay, F., and Cooper, B. (1989). Improving the sensitivity of the Barthel index for stroke rehabilitation. J. Clin. Epidemiol. 42, 703–709. doi: 10.1016/0895-4356(89)90065-6
Singer, B., and Garcia-Vega, J. (2017). The Fugl-Meyer upper extremity scale. J. Physiother. 63:53. doi: 10.1016/j.jphys.2016.08.010
Tang, C., Ding, Z., Li, C., Chen, C., Ding, L., Zhang, X., et al. (2014). Effects of motor imagery combined with task-oriented training on the recovery of upper extremity function after stroke: a randomized controlled trial. Chin. J. Phys. Med. Rehabil. 36, 832–837. doi: 10.3760/cma.j.issn.0254-1424.2014.011.004
Vucic, S., Stanley Chen, K.-H., Kiernan, M. C., Hallett, M., Benninger, D. H., Di Lazzaro, V., et al. (2023). Clinical diagnostic utility of transcranial magnetic stimulation in neurological disorders. Updated report of an IFCN committee. Clin. Neurophysiol. 150, 131–175. doi: 10.1016/j.clinph.2023.03.010
Wang, L., Gao, F., Dai, Y., Wang, Z., Liang, F., Wu, J., et al. (2023). Transcutaneous auricular vagus nerve stimulation on upper limb motor function with stroke: a functional near-infrared spectroscopy pilot study. Front. Neurosci. 17:1297887. doi: 10.3389/fnins.2023.1297887
Wang, Q., Zhang, D., Zhao, Y.-Y., Hai, H., and Ma, Y.-W. (2020). Effects of high-frequency repetitive transcranial magnetic stimulation over the contralesional motor cortex on motor recovery in severe hemiplegic stroke: a randomized clinical trial. Brain Stimul. 13, 979–986. doi: 10.1016/j.brs.2020.03.020
Waters, S., Wiestler, T., and Diedrichsen, J. (2017). Cooperation not competition: Bihemispheric tDCS and fMRI show role for ipsilateral hemisphere in motor learning. J. Neurosci. 37, 7500–7512. doi: 10.1523/JNEUROSCI.3414-16.2017
Wu, D., Ma, J., Zhang, L., Wang, S., Tan, B., and Jia, G. (2020). Effect and safety of transcutaneous auricular Vagus nerve stimulation on recovery of upper limb motor function in subacute ischemic stroke patients: a randomized pilot study. Neural Plast. 2020, 1–9. doi: 10.1155/2020/8841752
Xia, M., Wang, J., and He, Y. (2013). BrainNet viewer: a network visualization tool for human brain connectomics. PLoS One 8:e68910. doi: 10.1371/journal.pone.0068910
Yarossi, M., Patel, J., Qiu, Q., Massood, S., Fluet, G., Merians, A., et al. (2019). The association between reorganization of bilateral M1 topography and function in response to early intensive hand focused upper limb rehabilitation following stroke is dependent on Ipsilesional corticospinal tract integrity. Front. Neurol. 10:258. doi: 10.3389/fneur.2019.00258
Yoo, C., and Park, J. (2015). Impact of task-oriented training on hand function and activities of daily living after stroke. J. Phys. Ther. Sci. 27, 2529–2531. doi: 10.1589/jpts.27.2529
Zhang, J. J., Bai, Z., and Fong, K. N. K. (2022). Priming intermittent Theta burst stimulation for Hemiparetic upper limb after stroke: a randomized controlled trial. Stroke 53, 2171–2181. doi: 10.1161/STROKEAHA.121.037870
Zhang, H., Cao, X., Wang, L., Tong, Q., Sun, H., Gan, C., et al. (2023). Transcutaneous auricular vagus nerve stimulation improves gait and cortical activity in Parkinson’s disease: a pilot randomized study. CNS Neurosci. Ther. 29, 3889–3900. doi: 10.1111/cns.14309
Zhao, J.-L., Chen, P.-M., Li, W.-F., Bian, R.-H., Ding, M.-H., Li, H., et al. (2019a). Translation and initial validation of the Chinese version of the action research arm test in people with stroke. Biomed. Res. Int. 2019, 5416560–5416569. doi: 10.1155/2019/5416560
Zhao, J.-L., Chen, P.-M., Zhang, T., Li, H., Lin, Q., Mao, Y.-R., et al. (2019b). Inter-rater and intra-rater reliability of the Chinese version of the action research arm test in people with stroke. Front. Neurol. 10:540. doi: 10.3389/fneur.2019.00540
Keywords: transcutaneous auricular vagus nerve stimulation, task-oriented training, motor evoked potentials, functional near-infrared spectroscopy, stroke, upper extremity rehabilitation
Citation: Wang M-H, Wang Y-X, Xie M, Chen L-Y, He M-F, Lin F and Jiang Z-L (2024) Transcutaneous auricular vagus nerve stimulation with task-oriented training improves upper extremity function in patients with subacute stroke: a randomized clinical trial. Front. Neurosci. 18:1346634. doi: 10.3389/fnins.2024.1346634
Edited by:
Vitor Engracia Valenti, São Paulo State University, BrazilReviewed by:
Jack Jiaqi Zhang, Hong Kong Polytechnic University, Hong Kong SAR, ChinaJitka Veldema, Bielefeld University, Germany
Copyright © 2024 Wang, Wang, Xie, Chen, He, Lin and Jiang. This is an open-access article distributed under the terms of the Creative Commons Attribution License (CC BY). The use, distribution or reproduction in other forums is permitted, provided the original author(s) and the copyright owner(s) are credited and that the original publication in this journal is cited, in accordance with accepted academic practice. No use, distribution or reproduction is permitted which does not comply with these terms.
*Correspondence: Zhong-Li Jiang, amlhbmd6aG9uZ2xpQG5qbXUuZWR1LmNu
†These authors have contributed equally to this work and share first authorship