- 1Department of Experimental and Clinical Medicine, University of Florence, Florence, Italy
- 2Department of Industrial Engineering, University of Florence, Florence, Italy
- 3School of Occupational Medicine, University of Florence, Florence, Italy
Objective: Work-related musculoskeletal disorders (WRMSDs) are considered nowadays the most serious issue in the Occupational Health and Safety field and industrial exoskeletons appear to be a new approach to addressing this medical burden. A systematic review has been carried out to analyze the real-life data of the application of exoskeletons in work settings considering the subjective responses of workers.
Methods: The review was registered on PROSPERO. The literature search and its report have been performed following the PRISMA guidelines. A comprehensive literature search was performed in PubMed, EMBASE, Web of Science, and Scopus.
Results: Twenty-four original studies were included in the literature review; 42% of the papers retrieved included automobilist industry workers, 17% of the studies evaluated the use of exoskeletons in logistic facilities, and 17% of articles involved healthcare. The remaining six papers recruited farmers, plasterers, wasting collectors, construction workers, and other workmen. All the papers selected tested the use of passive exoskeletons, supporting upper arms or back. Usability, perceived comfort, perceived exertion and fatigue, acceptability and intention to use, occupational safety and health, and job performance and productivity were the main topic analyzed.
Conclusion: Exoskeletons are not a fix-all technology, neither for workers nor for job tasks; they tend to show more of their potential in static activities, while in dynamic tasks, they can obstacle regular job performance. Comfort and easiness of use are the key factors influencing the user's experience. More research is needed to determine the most effective and safe ways to implement exoskeleton use in occupational settings.
Systematic review registration: https://www.crd.york.ac.uk/prospero/display_record.php?RecordID=275728, identifier CRD42021275728.
Introduction
The European Agency for Safety and Health at Work (EU-OSHA) has recently reported that around 60% of all workers with a work-related health problem, identify work-related musculoskeletal disorders (WRMSDs) as their most serious issue, representing also one of the most common accidents. According to EU-OSHA 2019 report (1), roughly three out of every five workers in the European Union report WRMSD complaints. The most common types of WRMSDs reported by workers are backache and muscular pains in the upper limbs, especially in the construction, water supply, agriculture, forestry, and fishing sectors. Women report slightly more WRMSDs than men. WRMSDs prevalence is higher among older workers while decreasing with educational level (2), considering also that a higher level of education almost always corresponds to a lower chance of carrying out physically demanding jobs (3, 4). WRMSDs affect the general health of workers, that tend to be absent from work more often than others, resulting in a very high impact of WRMSDs in economic terms (5). Analysis of the phenomenon identified a significant relationship between self-reported WRMSDs and some physical risk factors; the advent of industry 4.0 meant a slight decrease in the prevalence of most physical risks, except for working with computers, laptops, and smartphones, while the back of the coin has resulted in a significant relationship between self-reported WRMSDs and organizational and psychological risk factors since more than half of workers complain about work-related stress (4). Occupational Health and Safety Management Systems (OHSMS) have forward-looking sustainable solutions to preserve employees' health and physical wellbeing (6–9). In this sense, workplace ergonomics can be considered a major point to achieve this goal (10). Improving workplace ergonomics should be treated as a continuous process, which systematically identifies and effectively reduces the level of workers' exposure to the risk factors known to cause WRMSDs. Until today, ergonomics improvement processes have been based on a continuous improvement model such as the quality (ISO 9001), environmental (ISO 14001), and safety (OHSAS 18001) models, without forgetting the ISO 12100, regarding the safety of machinery, and including general principles for design, aimed at reducing risk. Each of these management system models provides a common and familiar set of steps for managing environmental and safety risks, including WRMSDs risks. The recently introduced ISO 45001 (Safety Management System standard) provides a new, and soon-to-be common, model that can be used as an effective system for managing ergonomics. As result, most employers have implemented one or several preventive measures: rotation of tasks to reduce repetitive movements or physical strain, encouraging regular breaks for people in uncomfortable or static postures including prolonged sitting/provision of ergonomic equipment or equipment to help with lifting or moving, promoting healthy lifestyles (11). Newly developed Information Technology systems for ergonomics assessment help companies to evaluate how ergonomic their workstations are (12–16). At the same time, they enable qualification profiles to be drawn up for the assignment of physically impaired employees in line with their abilities and minding principles based also on the WHO International Classification of Functioning, Disability and Health (ICF) (17). Also, innovative solutions are provided by Human-Robot Cooperation (HRC) and the possibility to equip employees with wearable computing systems and devices in production facilities (18–24). In this scenario, the exoskeletons undoubtedly represent the state-of-the-art technological evolution made available to health and safety, providing useful opportunities also in the framework of Industry 4.0 and human-machine cooperation (25). The first research on exoskeletons dates to the early 1970s with projects undertaken for military purposes, aiming to produce armors that would enhance the user's strength, but colliding with technological limits in terms of sensors, structures, and actuators, resulting in systems that were too heavy and inefficient (26). Subsequently, the patent designs for exoskeletons have taken off in the past decade in the industrial sector with improved designs and materials, much more efficient and resistant to mechanical stress thanks to research and development (R&D). In addition, exoskeletons have been used in medical rehabilitation or occupational therapy, for example, to enable victims of spinal injuries to walk again (27–30). In recent decades, research has focused considerably on the development of this technology and exoskeletons have entered various fields and sectors, from military (31) to industrial and more generally manual work (32–34). Exoskeletons appear to be a new approach to addressing the issue of WRMSDs (35, 36), especially when other organizational interventions in workplaces are not feasible. Industrial exoskeletons are designed to mechanically assist workers during strenuous and physically demanding tasks, which include the lifting of loads or overhead work. Exoskeletons may light the load or enhance human strength, and this is of particular interest when it is not possible to improve workplace design and layout, as in temporary workplaces.
Industrial exoskeletons can be classified by kinematic structure and type of actuation. Concerning kinematics, rigid-structure devices can be classified as anthropomorphic and nonanthropomorphic devices, whereas soft exosuits do not present any kinematic structure. Moreover, the structure can range from full-body devices to devices that assist only one joint, thus making them potentially tailorable. Actuation types include passive, semi-active, and active systems. The passive exoskeletons do not have actuators or electronic components, such as transducers or controllers, but the force necessary to assist the actions performed by the user is released by exploiting the elasticity of the materials (i.e., torsion springs or pistons). The advantages offered by this type are in the lightness, economy, and simplicity of redesign, unlike the powered (semi-active and active) ones. Due to those peculiar characteristics, exoskeletons work in tandem with the wearer, lightening the load and increasing the physical capabilities of the wearer, as well as solving potential ergonomic issues of holding and working with tools.
Several experimental studies and literature reviews have been conducted to analyze the biomechanical effects of the available exoskeletons on users. Three recent systematic reviews analyzed the effects of industrial exoskeletons on exertion, muscle activity, and partially on user experience (37–39). They found a significant reduction in the user's acute physical stress and strain in the target area (40) and mean values of metabolic and cardiorespiratory parameters (41); also user endurance seemed to improve with the exoskeleton but performance declined in agility tasks (37). Only the review of Kermavnar et al. (37) included in the analysis aspects linked to user experience and satisfaction, which was moderate, and efficacy was rated from low to modest. However, most of the included studies recruit healthy and young volunteers, with no previous working experience and test the exoskeletons in controlled environments performing standardized and simple movements. This kind of lab environment is actually very far from real working settings, where job dynamics are complex, and workers are a heterogenous population. The redistribution of stress to different parts of the body can have effects on workers' health indeed and can play a huge role in the overall acceptance of exoskeletons in the workplace. Designing equipment that is user/worker centered is fundamental to make exoskeletons accepted, according to the idea that machines should adapt to workers and that a wearable product must not only be safe, comfortable, useful, and usable but also just as importantly, must be desirable to the end user (40, 41). Recently, data on the subjective experience of workers wearing the exoskeletons in working environments or performing real job tasks are slowly emerging. Nevertheless, evidence of the effectiveness of industrial exoskeletons in real occupational settings is missing.
Our review aimed at filling the gap in the literature about the worker's experience using the exoskeleton in real working tasks, since studies performed in the lab may have a limited value, not considering the complexity of a work environment as well as the experience of workers, who are the final users of industrial exoskeletons. The purpose of this systematic review is to analyze real-life data on the use of exoskeletons in work settings. In particular, this paper aims to outline a picture of the possible impact, benefits, and criticalities of exoskeletons in the workplace by reviewing and analyzing the subjective responses of workers.
Materials and methods
Literature research and data collection
This systematic review was registered on the PROSPERO register at number CRD42021275728 on September 30th 2021. The literature search and its report have been performed following the PRISMA guidelines (42). A comprehensive literature search was performed in PubMed, EMBASE, Web of Science, and Scopus. The search strategy combined free text and controlled vocabulary, using the keywords “exoskeleton” and “worker”. No limits were applied to the search regarding the period of publication and the study design; only papers written in English were deemed eligible for the selection. The details of the search strategy for each database are listed in Supplementary material. Moreover, manual research was performed to screen the bibliographic references of the most relevant papers selected. The research was based on the modified PICO scheme, the SPICE framework (43):
Setting
Working environment or lab setting in which working tasks are performed.
Perspective
Workers of all types.
Intervention
Use of any kind of exoskeleton in an occupational setting (field study/laboratory study reproducing working tasks).
Comparison
When present, the use of no exoskeleton for the performance of the same tasks.
Evaluation metrics
User's subjective experience/perception/compliance/usability of the device.
A first screening of the results was performed by two independent reviewers (LGL and AM) through titles and abstracts of the reports identified. A further selection was made by analyzing the full text of the articles. The judgment about the inclusion of each paper was performed separately by the investigators; disagreements were solved by the discussion with a third reviewer (AB).
Data were manually extracted in a chart jointly developed by the authors, including relevant data from the papers retrieved.
Inclusion criteria
The inclusion criteria followed the SPICE scheme mentioned above; we included articles focusing on the application of exoskeletons in the occupational field. We selected papers considering the use of exoskeletons by workers acting in real occupational tasks, both in the field and in the lab. The studies selected took into account the subjective perspectives of workers wearing the exoskeletons regarding comfort, usability, and personal experience.
Exclusion criteria
Articles written in languages other than English were excluded. Studies analyzing stereotyped tasks which did not reproduce a real working task, or not considering the subjective experience of the participants were also excluded. We also excluded articles that did not conduct experiments on real workers. Also, review articles were not included in the literature synthesis but were discussed in other paragraphs.
Quality assessment
The quality assessment was performed using the Mixed Methods Appraisal Tool (MMAT) (44, 45) which is designed for the appraisal stage of systematic mixed studies reviews, i.e., reviews that include qualitative, quantitative, and mixed methods studies, thus, it was suitable for our review. The full presentation of the ratings is reported in Supplementary Table S1.
Results
The online search retrieved a total of 600 papers. The selection process is shown in Figure 1.
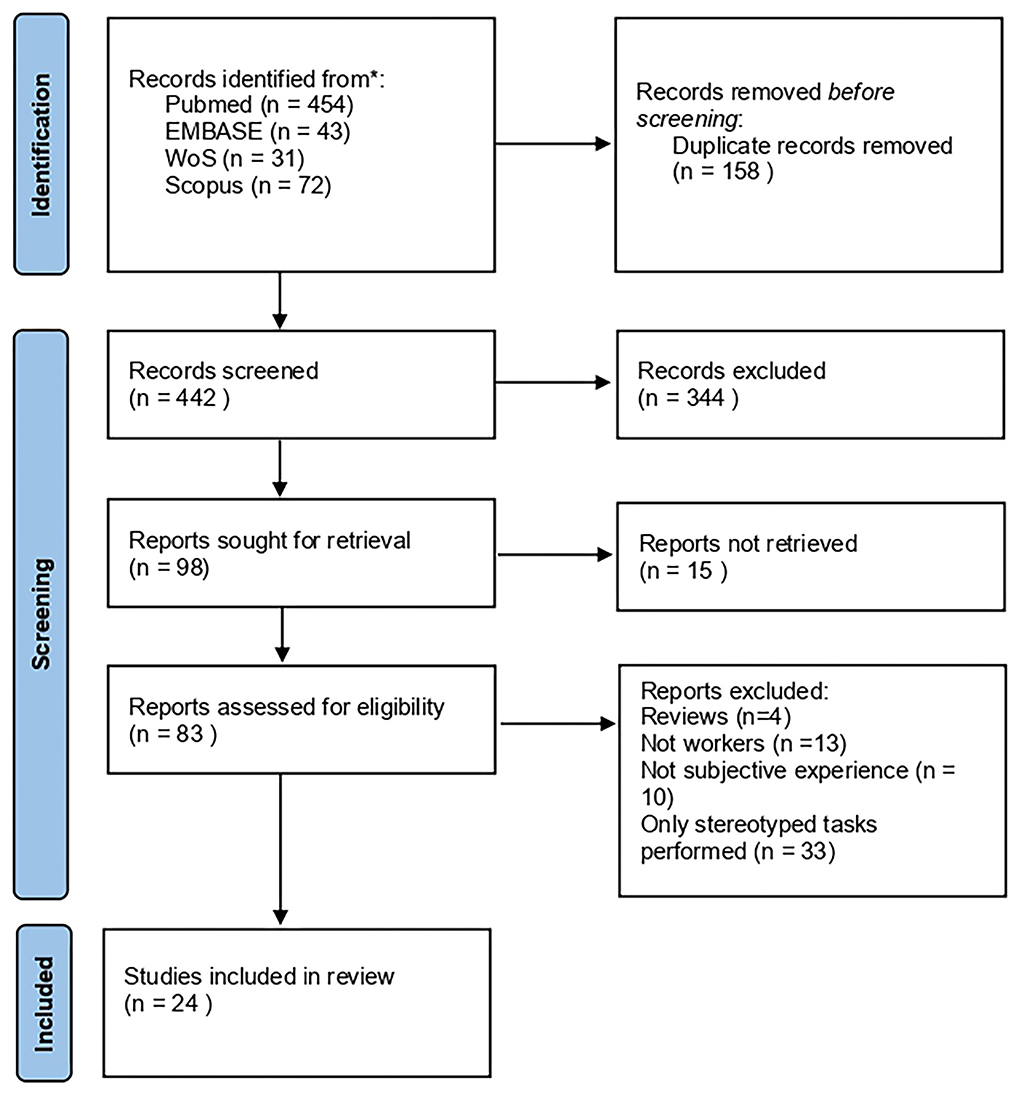
Figure 1. PRISMA 2020 flow-chart (42).
At the end of the selection, 24 original studies were included in the literature review. Most (sixteen) of the selected articles have a cross-sectional design, administering validated and not validated questionnaires at the end of an experimental period in which the workers perform their job wearing the exoskeleton. Four studies adopted a mixed-method approach and 1 was purely qualitative; the experiment performed is similar to that of a cross-over study: the workers wear the exoskeleton for a period and then evaluate their experience. Three articles described prospective cohort studies of two working populations studied for several months. Fourteen studies were published in Europe, 8 in North America, 1 in Iran, and 1 in Korea. The overall quality of the studies, evaluated with the MMAT tool, ranges from 20 to 80%: 5 studies scored 80%, 13 scored 60%, and 6 scored 40%. The details of the quality appraisal are included in Supplementary material S2.
42% (n = 10) of the selected articles included automobile industry workers, who work in the assembly lines of vehicles; 17% (n = 4) of the studies evaluated the use of exoskeletons in logistic facilities, 17% (n = 4) of the articles involved healthcare workers such as surgery team members and professional caregivers. The remaining 6 papers (25%) recruited farmers, plasterers, wasting collectors, construction workers, and other workmen. Table 1 includes the selected studies of the review and Figure 2 summarizes the main characteristics of the selected articles.
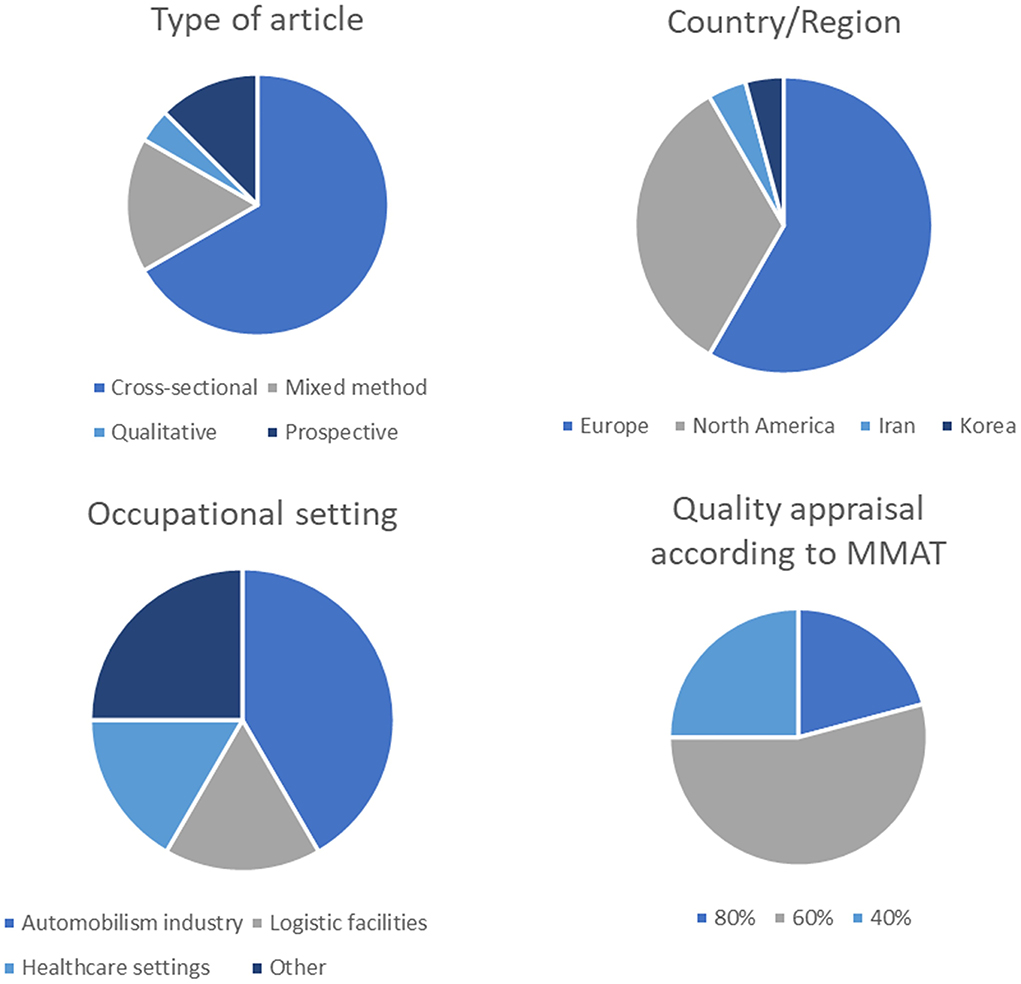
Figure 2. Summary of the selected studies in terms of type of study, country/region, occupational setting and quality appraisal.
Models of exoskeletons tested
All the papers selected tested the use of passive exoskeletons. Two main types of exoskeletons were worn by the workers: exoskeletons supporting the upper limbs and exoskeletons supporting the back. The types of exoskeletons were chosen according to the job performed. Only one study provided results of usability on lower limbs supporting exoskeleton (Hyundai CEX chair exoskeleton) (49).
Table 2 includes the models of the upper limb exoskeleton used in the selected studies. The weight of the exoskeletons ranges from about 5 kg (ShoulderX) to 2 kg (Levitate AirFrame). The exoskeletons are designed to reduce the load on the shoulders during work at shoulder height or higher and, as a result, prevent shoulder injuries. Different models can provide support when the arms are forward flexed or adducted. The exoskeleton is worn as an upper body suit, and it is connected to the trunk. Most exoskeletons are available in two different sizes and can be further adjustable. Also, the support provided in some models can be regulated by the user.
Table 3 shows the exoskeleton models for back support tested in the selected papers. The weight of the back support exoskeleton ranges from 0.9 kg (ErgoVest) to over 2 kg (Laevo). Nevertheless, the Laevo model was the most used. The exoskeletons came in different sizes and can be further adjusted. The back support exoskeletons are supposed to support lifting and forward bending activities and heavy workload manipulation at the workplace. The devices act by transferring a portion of the force from the spinal column to the shoulders, pelvis, and legs. In a study, the exoskeleton tested was a novel model aimed to reduce lumbar back loadings during awkward postures (i.e., stoop and squat postures) and during lifting/lowering/carrying in repetitive manual handling activities (47).
Scale used
The selected studies used several validated tools, ad hoc- not validated - questionnaires and qualitative approaches to investigate the worker's experience wearing the exoskeleton. Usability was measured with the SUS – System Usability Scale (71), in its original form and other version adapted by authors to the exoskeleton experience. Usability and user experience were also measured with the Post-Study System Usability Questionnaire (PSSUQ) (72), with the Usability metrics questionnaire (73), and with the scale by Laugwitz et al. (74). Global satisfaction was measured with the tool by Nielsen (75). The Technology Acceptance Model (TAM) questionnaire was used to assess the perceived efficacy, usability, and acceptance of the device (76). The German Technology Usage Inventory assessed the Intention to Use and usefulness (77). Other validated tools adopted in the studies are the Usability Metric for User Experience lite (UMUX) (78) and the scale to evaluate Task-Specific Self-Efficacy (TSSE) (79, 80). Exertion, physical load, and comfort were analyzed with NASA-TLX (task load index) questionnaire (81), Borg's Rating Perceived Exertion (RPE) scale (82), Local Perceived Pressure (LPP) scale (83), and Cornell Musculoskeletal Discomfort Questionnaire (CMDQ) (84). Qualitative approaches included focus groups and semistructured interviews (48, 49, 54, 58, 62, 66, 67).
Usability
Eighteen papers (75%) investigated the concept of usability of the exoskeleton worn by workers. The scale used to evaluate usability was heterogenous, the most used was SUS (System Usability Scale), which was adopted by 4 studies. The scales used by each study are reported in Table 1. For all the exoskeletons tested, the usability was judged from medium to high. Key features of the usability of an assistive wearable device are the easiness of use, simple design, and minimal effort to learn (56). Also, the device should be effective, flexible, intuitive, and easy to use (54). The usability is strictly connected to lightweightness and easiness to wear (47, 50, 67). Usability decreases as body discomfort and frustration intervene in the task (51). In surgery settings (59), worker role and specific operation were key factors identified for successful implementation and improved usability: the constant movement and the need to take on and off the device to speak with the patients required by the attending surgeon prevent this figure to benefit the most from the device. However, the surgical team confirmed that this technology can be relevant to reduce physical load and identified the time needed for donning/doffing the exoskeleton as a key term for usability. In the automotive tasks, the study by Chae et al. (49) underlined the importance of wearability, in the part of fastening and adjusting the harness and finding the right position. Stability and convenience were the most important factors related to overall wearing satisfaction. In the study by Hensel and Keil (55), workers reported moderate-high levels of usability, which suggests a good level of support to reduce physical demands, though this aspect of usability decreased from the start to the end of the trial.
Perceived comfort
Thirteen studies (54%) addressed the perceived comfort and discomfort of the workers wearing the exoskeletons. There was no homogeneity in the scale used to measure physical comfort or discomfort. The most used scale was a Likert scale measuring discomfort, adopted by four studies. The physical comfort of the device is mainly expressed in the direct interface between the exoskeleton and the body. In logistic workers, participants reported the highest discomfort in the shoulder regions where the rigid frame of the exoskeleton and the user's body interacted, or body parts onto which the exoskeletons were attached. These aspects influenced the score of usability (51). In the automotive plants, the physical discomfort on the lower back perceived by workers wearing back support exoskeletons and working in static positions, decreases significantly over time, thus suggesting a potential for the devices to reduce physical demands on the lower back. On the contrary, this improvement in comfort was not found for the dynamic workstation, where workers tended to report increasing uneasiness during that time (55). Similar findings were found in the construction setting, where workers working with increased lifting loads rated a reduced level of perceived discomfort in their lower back while using a back support exoskeleton system (47). In automotive settings, the body region that was found more involved in the perceived discomfort was the chest. The discomfort was attributable to the chest pad of the back support exoskeleton, which caused pain in particular in dynamic workload situations (55). Other body regions involved were the upper back, chest, hips, and thighs due to friction, pressure, and heath. For certain tasks that involved trunk rotation, the rigid bars of the back support exoskeleton made pressure on the thoracic region causing pain (53).
Thermic comfort was recognized as a critical issue for the comfort of the exoskeleton, both for those supporting the back (60) and those supporting the upper arms (57, 58, 65), to the point that it could inhibit the use of the device (65). In an automotive assembly, after 3 months of regular use of an upper limb supporting exoskeleton, participants reported a substantial decrease in the amount of discomfort experienced in their neck and arms and a slight reduction in back discomfort (65). For press and shear workers, higher significant discomfort scores wearing the back support exoskeleton were found located at the chest and thighs, compared to those not wearing the exoskeleton and the discomfort scores were much higher as experienced by study participants in a lab situation (46). In order for picking activities and logistic activities, the support at the legs and the hip belt was two determinants of the comfort of the back support exoskeleton; workers reported that it would be not possible to wear the exoskeleton during the whole day (60). Carrying heavy loads across the facilities was associated with perceived discomfort in the chest and thighs, showing the need for optimization of the back support exoskeleton (68). In care settings, several nurses complained about poor fit, referring that wearing the back support exoskeleton made them stiffer and unable to react to sudden situations (67). Finally, perceived comfort may widely vary between men and women: in Hwang et al. study (56), most participants are women and the different tropism and muscle reserves could impact the perception of comfort. Also, the interface between the back support exoskeleton and the chest in female workers can be a noticeable issue in terms of not only comfort but also usability and intention to use (48, 56).
Perceived exertion and fatigue
Nine studies (39%) analyzed the exertion and fatigue perceived by workers wearing the device. The most used scale was the RPE scale, adopted by three studies. Overall, as for usability and comfort, it was found great heterogeneity in the methods used to measure exertion and fatigue. In plastering workers, the perceived exertion was reduced using the upper limb supporting exoskeleton, in particular for activities at the ceiling, while during tasks involving various movements passive exoskeletons were found not as effective as during less varied tasks (52). In the automotive assembly setting, using an exoskeleton had little impact on perceived work intensity or musculoskeletal disturbs. The beneficial effects did not occur immediately and were observed after a 6-month period of continuous use. However, the reduction of physical demand on the shoulder, neck, and back was perceived positively by workers wearing the upper limbs exoskeletons (57, 58). Nevertheless, a portion of the reduced exertion perceived in the shoulders and the arms could be attributed to a sort of placebo effect elicited by users' positive expectations (63). Upper limbs exoskeletons were judged effective in increasing endurance time and reducing perceived effort while holding demanding postures with raised arms and/or having to lift and hold small work tools (66). Reducing workload, however, may not be always linked to good usability, as proved by logistic workers wearing shoulders supporting exoskeletons who experienced a reduced temporal workload, but still scored the usability moderate (51). Testing the upper limbs exoskeletons in the surgical room, the assistive device is perceived by workers to be able to address the high perceived physical workload among residents, who often maintain static posture, such as when holding instruments (e.g., scopes or retractors) (48). In general, subjects experienced significantly less pain in their shoulders after 1 day of operating with the exosuit compared to 1 day of operating without the exosuit. Subjects also reported not only decreased neck, upper arm, wrist, and knee pain but also a small increase in lower back pain after wearing the device (59). In an enclosure production line, participants reported higher exertion scores in the in-field session compared to the simulated session (63). In wasting collecting activity, results showed that the perceived exertion of the workers using the Ergo-Vest was significantly lower than those who did not use the device; the work difficulty perceived wearing the exoskeleton was one level lower than without the device (69).
Occupational safety and health
Only three studies addressed the issue of improvement of the participant's health and prevention of injuries, and one study considered the possible harmfulness of the exoskeleton regarding safety in the workplace. In an 18-month experiment, automotive operators reported an equivalent or slightly better perceived safety and slightly better perceived performance. Those who used the ASE were roughly half as likely to make a medical visit that involved an injury to or pain in the upper extremity (58). In the three participants of the study of Smets (65) who had varying levels of musculoskeletal discomfort (shoulder, neck, and back), a decreasing trend was observed in the total musculoskeletal disturbs and at the end of the 3 months of regular exoskeleton use, the scores were zero for all three. In terms of self-perception, the perception of safety and health varies across the workers: some participants described the benefits of exoskeleton use in terms of feeling more support or facilitating better posture; on the contrary, others who described having a strong or healthy back did not perceive these long-term health benefits (62). Finally, in particular working environments such as nurse homes, workers were concerned about their safety because the patients, mostly affected by dementia, could grab onto the device possibly causing injuries (67).
Job performance and productivity
Eight (33%) studies considered the topic of job performance and productivity. The efficacy of the exoskeleton widely varies according to the task performed. In the automotive setting, tasks involving heavy material handling were reported to be easier to perform with the exoskeleton, while more dynamic tasks which involved various postures were classified as harder to perform, and sometimes the exoskeleton might disturb rather than help. Also, dynamic tasks, which require adjustments in the exoskeleton support, were difficult to manage due to time pressure (53). Exoskeletons were reported to improve endurance and accuracy execution of precision tasks (66). In a 3-month experience, a slight gain in self-reported task performance was observed; participants reported that they received the most benefit from the exoskeleton when performing tasks that were overhead, while they reported continued difficulties with tasks that required even moderate nonneutral trunk postures (65). Plasterer workers reported the risk of hindrance and slowness of the productivity process (52), and a similar finding was retrieved in the study by Omoniyi et al. (62), in which some farmers reported being able to accomplish more shoveling work faster with less energy, while others felt encumbered by tension in leg pads while walking. Using the exoskeleton while driving or operating farm machinery was often described as an encumbrance and did not provide appropriate support. Also in a logistic facility, some workers felt constrained rather than relieved by the exoskeleton, or that its functions did not correspond well enough with their work tasks to be considered useful (64). While the exoskeleton provided a positive effect in lifting and lowering loads, when carrying the load and walking without it, the suitability of the exoskeleton is greatly reduced (63). On the contrary, the exoskeleton model tested in the waste-collecting activities did not create a restriction on workers' motion (69).
Acceptability and intention to use
Twelve studies (50%) dealt with the acceptability and the intention to use exoskeletons by workers. Fit and comfort are in general key determinants of the possible adoption of the exoskeleton by workers (57, 58, 65), along with perceived performance (58). Also, a perceived decrease in physical demands when using the exoskeleton was positively associated with reported intention-to-use. Nevertheless, intention-to-use was strictly connected to usability and negatively impacted by discomfort, to the extent that even a minimal level of discomfort might hinder a user's acceptance (55). At the same time, it was pointed out that workers tended to judge more positively the exoskeleton per se for its characteristics and potential than its use in a real field social aspects come into play, linked to how others may judge an individual who decides to use the device or how the person perceives the decision and the imposition of the exoskeleton (54). In a study involving enclosure workers, although the exoskeleton obtained good levels of usability and acceptability, lower scores were observed on aspects related to image and output quality. Workers also identified major improvements to perform before considering to use constantly the device at work (52, 61). Among surgical team members, workers answered positively when asked whether they would be frequently using this device (48) and most of them would consider incorporating the exosuit into their daily practice (59). The aspects of attractiveness, perspicuity, efficiency, dependability, and stimulation were judged neutral by shear workers, while the exoskeleton was judged positively about the novelty (46). In the automotive assembly setting, user acceptance was found to decrease significantly by the end of the period of exoskeleton use (4 weeks), after being rated very high at the beginning (55). The exoskeleton acceptance was found associated with technology-induced self-efficacy beliefs, which are in turn moderated by exertion relief capacity and usefulness attributed to the device (64). In a geriatric care setting (67), the intention to use the exoskeleton was associated with its perceived usefulness and how enjoyable it was to use. Besides, the exoskeleton's trustworthiness, inked to personal and procedural characteristics of technological use, was a possible determinant of the intention to use.
Discussion
This systematic review included 24 studies, in which the exoskeletons were tested on workers performing real occupational tasks, both in the lab setting and directly in the field. Despite the online research being performed without any date restriction, all the studies were published in the last five years, mostly in 2020 and 2021. This testifies to the novelty of the introduction of industrial exoskeletons in real working settings.
Models of exoskeletons tested in the selected studies
All the papers included in our review used passive exoskeletons which use the restoring forces of springs, dampers, or other materials to support the human movement. The movements of the user generate the energy stored in a passive exoskeleton, and forces are redistributed to protect specific body regions. The improvement of the performance of the exoskeleton user is not given by additional physical strength (as it would be for active devices) but by the ability provided by passive exoskeletons to maintain exhausting positions over a longer period of time (70). Passive exoskeletons are relatively light (from 1 to 5 kg approximately in our selection) and have an affordable cost. Also, there are several models already available on the market and overall require acceptable maintenance costs. The exoskeletons tested in the selected studies showed good usability and acceptable comfort; almost all the models are already in the market, so they can be considered in an advanced development phase. Although a lack of intervention effectiveness studies in the field, industrial exoskeletons are already being used in several work settings, as also testified by the heterogeneity of the job included in our review. Active exoskeletons instead are still very limited on market, due to the higher costs and the need for further technological developments. With the purpose of collecting evidence only on exoskeletons used in real occupational fields, this review excluded studies addressing active devices. Indeed, we found only one study providing an on-field experience of an active device (85), which was tested among military personnel and determined increased workload perceptions among participants. Also in lab experiments, often the active exoskeleton is tested only on very few participants, limiting the quality of evidence (70). According to Toxiri et al. (86), active exoskeletons offer greater versatility and are potentially suited to provide stronger assistance. Active devices may, therefore, be more appropriate for demanding and dynamic tasks, such as handling heavier loads. This was also found by a recent study comparing active and passive back exoskeletons in the lab, showing that in dynamic conditions, the active device had better scoring (87). Active devices may have a better potential in reducing physical load than passive ones, but they are much heavier, with the lighter ones weighing from 6 to 9 kg (88, 89), and can provide higher pressure at the body–machine interface (90).
Exoskeletons and WMSDs
Previous studies and reviews have focused on the effects of exertion and reduction in muscle activity provided by industrial exoskeletons, mainly through lab experiments. Only recently, since the beginning of the use of exoskeletons in working settings, user experience has gained relevance in research. Currently, prospective studies analyzing the intervention of using exoskeletons to reduce the incidence of WMDS are still missing. Industrial exoskeletons were born and marketed with the aim of reducing WMSDs, assuming that the reduction in exertion, which is the outcome most analyzed in the current literature, corresponds to a reduction in musculoskeletal disease risk factors. WRMSDs have a complex pathogenesis (91), and such an assumption may appear too simplistic. It is nevertheless true that if exoskeletons achieved reductions in the mechanical stressors associated with manual handling tasks, they have the potential to reduce the incidence of WMSDs and the related burden of disease, including the economic cost of management of working days loss and disability (92, 93). In our review, only the study of Kim et al. (57) provided some evidence of the reduction of medical visits requested by workers wearing the exoskeleton for a long period of time. Certainly, further research, involving a long period of observation and medical records, is needed in this field. As pointed out by the consensus guidelines by Steinhilber et al. (94), the use of exoskeletons should be monitored medically by the occupational physician, with regular interviews and medical examinations. Longitudinal studies and case-control studies are needed to evaluate the effects of industrial exoskeletons on workers' health.
Safety and risk assessment for exoskeletons in occupational settings
There are several different opinions on how industrial exoskeletons might be considered. They can be defined as Personal Protective Equipment (95), or as performance and amplification devices (PAADs). As PPE, they should be considered for the health protection of workers only when all other organizational measures to reduce occupational risks are taken and certified according to the Regulation (EU) 2016/425 on personal protective equipment (96). However, there are many workplaces that are not tied to a specific location (e.g., agriculture), where ergonomic design measures cannot be implemented because of the changing environmental requirements and where heavy manual material handling is common and injury risk is high (97). As PAADs should be considered during the workplace risk assessment, the potential risks of exoskeletons in working environments, for example, during a slip or a fall accident and perhaps the massive introduction of exoskeletons in industrial settings should pass through a redesign of workplaces (98). According to our findings, in some cases, workers have recognized exoskeletons as a hindrance and that can represent an issue for security, for example, in case of workplace evacuation during an emergency. For this reason, the donning and the doffing of the device are crucial points when considering usability, and therefore, the intention to use the exoskeleton in real settings. Moreover, some authors suggested that exoskeletons should not be used to motivate increased work demands or duration, since the levels of activity observed in secondary muscle groups other than the target area are largely unchanged (99), and no change in the overall physical demand of the task is observed (100).
As the introduction of exoskeletons is becoming more and more frequent in industrial settings, studies designed to study the potential risks linked to exoskeletons are needed (93). In our selection, there was no evidence of any specific risks to the health and safety of workers, but the findings underlined that the exoskeleton is not useful in dynamic tasks but is considered by workers as a hindrance. This consideration leads to the need to accurately select the task in which wearing the exoskeleton, as pointed out also by lab studies (101).
The difference between lab and field studies
In the field, studies show overall less brilliant results than lab studies, in terms of user satisfaction. The work tasks are far more complex than the experimental tests and exoskeletons are the most useful during static postures, for example, when it is necessary to keep the arms above the shoulders for a long period of time (e.g., assembly line overhead). The differences in the performed tasks, with those in real working environments far more complex than those studied in the lab, is the first great difference between lab and field studies. Recent research has also pointed out how wearing an exoskeleton and performing complex tasks impose greater motor adaptation and neurocognitive efforts, which may almost offset the biomechanical advantages of exoskeletons (102). Industrial exoskeletons can be efficient in physical exertion reduction but not for all tasks and not for all workers, because they cannot be considered one-size technology. In our review, the working population tested was mainly constituted of healthy male subjects and for the most part, one criterion of exclusion from the experiment was having any musculoskeletal disturbs. Only a study included a very restricted sample of workers with some low back disturbs (65) who definitely benefit from wearing the exoskeleton during work. In this regard, even if the studies tested a working population, they could not be considered fully representative, due to the high prevalence of WRMSDs in Europe (1). The two studies including female workers – whose number is currently growing in the workplace – evidenced issues about the adaptation of the device to the shape of the female body. Differences in kinematics wearing exoskeletons between men and women were already found, but it is still unknown if they can have a relevant meaning (99).
Usability and acceptance by real workers
Comfort and fit indeed are the main factor influencing the usability and acceptance of the device by workers. Workers may accept the exoskeleton only if the benefits perceived will overcome the possible negative residual drawbacks, like the overall discomfort (heath, human-machine interface, time to don and doff) (103). Despite the heterogeneity of the jobs analyzed and the exoskeletons tested, the wearability and the easiness of taking on and off the exoskeleton remain key factors for the usability and intention-to-use of the device. An interesting point is that when the measure of subjective experience was repeated over time, it decreased after a first enthusiastic evaluation. This was consistent with a previous finding in a lab test where subjective ratings of perceived discomfort and usability worsened during the time (104). The novelty and the enthusiasm of wearing a novel device can be a driving force for workers, which are obviously destined to decrease over time so longitudinal evaluations are necessary to discriminate it and to obtain a not-biased evaluation that can also provide a suggestion for further development. Overall, the findings of our review underline the need for a human-centered design (103) that aims at making systems usable and useful by focusing on the users, their needs, and their requirements, and by applying human factors/ergonomics, and usability knowledge and techniques. In this optic, the development and the large-scale adoption of industrial exoskeletons can be contextualized in the framework of Industry 5.0 whose main concern is the synergy between humans and machines (105).
Limitations and strengths of the evidence retrieved
This review has several limitations and points of strength. First, the evidence retrieved is subject to the quality of the articles included in the review. The selected papers have a cross-sectional design for the most part, and in some cases adopted a qualitative approach, and this impacts the level of confidence of the evidence. The lack of standards in evaluating the outcomes and the use of not-validated questionnaires makes it hard to compare studies and generalize the findings. Second, as mentioned above, the participants of the studies and the occupational settings involved may not be fully representative of the general working population and working environments in general. Regarding the methodology adopted in this systematic review, although performed according to PRISMA guidelines and with a rigorous search and selection strategy, it is possible relevant studies may have been missed. However, to the best of our knowledge, this is the first review that systematically addressed on-field studies involving real workers wearing exoskeletons and analyzing the depth of their user experience. It identified major points to consider when introducing exoskeletons on the field, such as comfort, job performance, acceptance from workers, task specificity, safety, and health issues.
Conclusion
The use of exoskeletons in occupational settings is a relatively new phenomenon with a limited amount of research available on the topic. A systematic review of the literature was conducted in order to identify and evaluate the available evidence on the field use of exoskeletons from an occupational safety and health perspective. Twenty-four scientific articles were identified that met the inclusion criteria for the review. The findings of the review suggest that exoskeletons have the potential to reduce the risk of musculoskeletal injuries in a variety of occupational settings. However, there is a lack of evidence on the long-term safety and efficacy of exoskeleton use in the workplace. Additionally, there are several potential concerns that need to be addressed, such as safety issues and ergonomics, considering that is not clear if the exoskeleton itself can cause the discomfort, or if the discomfort should be due to the lifting tasks that the workers are called to perform. More research is needed to determine the most effective and safe ways to implement exoskeleton use in occupational settings.
Our review explored the experience of real workers wearing industrial exoskeletons. The lack of longitudinal studies is the core limitation when analyzing such data and medical data regarding the possible prevention of WMDs using exoskeletons are currently missing. On-field studies, which addressed workers' experience wearing exoskeletons, showed overall less brilliant results than lab experiments, due to the higher complexity of work tasks compared to stereotyped exercises. Exoskeletons are not a fix-all technology, neither for workers nor for job tasks; they tend to show more of their potential in static activities while in dynamic tasks they can obstacle regular job performance. Comfort and easiness of use are the key factors influencing the user's experience.
The existing literature on the field use of exoskeletons is mostly anecdotal, consisting of case reports and small case series. There is a need for larger, well-designed studies on the field use of exoskeletons in order to better understand the potential safety and health risks associated with their use.
Author contributions
The first draft, conceptualization, and the original idea were provided by AB and LGL. The literature search was performed by LGL and AM. The selection of articles was performed by LGL, AM, and AB. The study was critically revised and reviewed by NM, GA, FC, and LF. All authors contributed to the article and approved the submitted version.
Conflict of interest
The authors declare that the research was conducted in the absence of any commercial or financial relationships that could be construed as a potential conflict of interest.
Publisher's note
All claims expressed in this article are solely those of the authors and do not necessarily represent those of their affiliated organizations, or those of the publisher, the editors and the reviewers. Any product that may be evaluated in this article, or claim that may be made by its manufacturer, is not guaranteed or endorsed by the publisher.
Supplementary material
The Supplementary Material for this article can be found online at: https://www.frontiersin.org/articles/10.3389/fpubh.2022.1039680/full#supplementary-material
References
1. De Kok J, Vroonhof P, Snijders J, Roullis G, Clarke M, Peereboom K, Van Dorst P, Isusi I. Work-related musculoskeletal disorders: prevalence, costs and demographics in the EU. European Risk Observatory Report (2019). Available online at: https://osha.europa.eu/it/publications/msds-facts-and-figures-overview-prevalence-costs-and-demographics-msds-europe (accessed May 11, 2022).
2. EU Labour Force Survey - modules - Statistics Explained. (2022). Available online at: https://ec.europa.eu/eurostat/statistics-explained/index.php?title=EU_labour_force_survey_-_modules&oldid=543984 (accessed September 8, 2022).
3. Eurostat Labour Force Survey ad hoc module 2013 Eurostat Labour Force Survey ad hoc module “Accidents at work and other work-related health problems” (2013). Available online at: https://ec.europa.eu/eurostat/web/products-eurostat-news/-/ESS_LFS_2013_AHM (accessed July 18, 2022).
4. Sixth European Working Conditions Survey. 2015. Eurofound (2015). Available online at: https://www.eurofound.europa.eu/surveys/european-working-conditions-surveys/sixth-european-working-conditions-survey-2015 (accessed July 18, 2022).
5. Work-Related Musculoskeletal Disorders and Ergonomics. Workplace Health Strategies by Condition. Workplace Health Promotion. CDC. (2022). Available online at: https://www.cdc.gov/workplacehealthpromotion/health-strategies/musculoskeletal-disorders/index.html (accessed October 18, 2022).
6. Kavouras S, Vardopoulos I, Mitoula R, Zorpas AA, Kaldis P. occupational health and safety scope significance in achieving sustainability. Sustainability. (2022) 14:2424. doi: 10.3390/su14042424
7. Schulte PA, Iavicoli I, Fontana L, Leka S, Dollard MF, Salmen-Navarro A, et al. Occupational safety and health staging framework for decent work. Int J Environ Res Public Health. (2022) 19:10842. doi: 10.3390/ijerph191710842
8. Alli BO. Fundamental Principles of Occupational Health and Safety 2nd Edn. Geneva: International Labour Organization (2008).
9. Safety and Health at Work. A Vision for Sustainable Prevention : XX World Congress on Safety and Health at Work 2014 : Global Forum for Prevention, 24–27 August 2014, Frankfurt, Germany. 2014. Geneva: ILO (2022). Available online at: https://labordoc.ilo.org/discovery/fulldisplay/alma994857923402676/41ILO_INST:41ILO_V2 (accessed October 18, 2022).
10. Nastasia I, Coutu MF, Tcaciuc R. Topics and trends in research on non-clinical interventions aimed at preventing prolonged work disability in workers compensated for work-related musculoskeletal disorders (WRMSDs): a systematic, comprehensive literature review. Disabil Rehabil. (2014) 36:1841–56. doi: 10.3109/09638288.2014.882418
11. European Survey of Enterprises on New and Emerging Risks (ESENER). Safety and health at work EU-OSHA (2022). Available online at: https://osha.europa.eu/en/facts-and-figures/esener (accessed July 18, 2022).
12. Schwerha D, Casey A, Loree N. Development of a system to integrate safety, productivity, and quality metrics for improved communication and solutions. Saf Sci. (2020) 129:104765. doi: 10.1016/j.ssci.2020.104765
13. Turk M, Šimic M, Pipan M, Herakovič N. Multi-criterial algorithm for the efficient and ergonomic manual assembly process. Int J Environ Res Public Health. (2022) 19:3496. doi: 10.3390/ijerph19063496
14. Ghasemi F, Mahdavi N. A new scoring system for the rapid entire body assessment (REBA) based on fuzzy sets and Bayesian networks. Int J Ind Ergon. (2020) 80:103058. doi: 10.1016/j.ergon.2020.103058
15. Caporaso T, Grazioso S, Di Gironimo G. Development of an integrated virtual reality system with wearable sensors for ergonomic evaluation of human–robot cooperative workplaces. Sensors. (2022) 22:2413. doi: 10.3390/s22062413
16. Klussmann A, Liebers F, Brandstädt F, Schust M, Serafin P, Schäfer A, et al. Validation of newly developed and redesigned key indicator methods for assessment of different working conditions with physical workloads based on mixed-methods design: a study protocol. BMJ Open. (2017) 7:e015412. doi: 10.1136/bmjopen-2016-015412
17. World Health Organization. International classification of functioning, disability and health: ICF. World Health Organization (2001). Available online at: https://apps.who.int/iris/handle/10665/42407 (accessed October 18, 2022).
18. Ajoudani A. Transferring Human Impedance Regulation Skills to Robots. Berlin: Springer International Publishing (2016).
19. Ciullo AS, Catalano MG, Bicchi A, Ajoudani A. A supernumerary soft robotic hand-arm system for improving worker ergonomics. In:Carrozza MC, Micera S, Pons JL, , editors. Wearable Robotics: Challenges and Trends. Cham: Springer International Publishing (2019) p. 520–4.
20. Lorenzini M. Toward a synergistic framework for human-robot coexistence and collaboration (hrc2). in Institute for Robotics and Intelligent Machines Conference (I-RIM) Proceedings. (2019).
21. Fortini L, Lorenzini M, Kim W, De Momi E, Ajoudani A. A real-time tool for human ergonomics assessment based on joint compressive forces. In: 2020 29th IEEE International Conference on Robot and Human Interactive Communication (RO-MAN). IEEE (2020) p. 1164–70.
22. Fortini L, Lorenzini M, Kim W, De Momi E, Ajoudani A. A framework for real-time and personalisable human ergonomics monitoring. In: 2020 IEEE/RSJ International Conference on Intelligent Robots and Systems (IROS). IEEE (2020) p. 11101–7.
23. Lamon E, De Franco A, Peternel L, Ajoudani A, A. capability-aware role allocation approach to industrial assembly tasks. IEEE Robot Autom Lett. (2019) 4:3378–85. doi: 10.1109/LRA.2019.2926963
24. Peternel L, Fang C, Tsagarakis N, Ajoudani A. Online human muscle force estimation for fatigue management in human-robot co-manipulation. In: 2018 IEEE/RSJ International Conference on Intelligent Robots and Systems (IROS). IEEE (2018). p. 1340–6.
25. Crea S, Beckerle P, Looze MD, Pauw KD, Grazi L, Kermavnar T, et al. Occupational exoskeletons: a roadmap toward large-scale adoption methodology and challenges of bringing exoskeletons to workplaces. Wearable Technol. (2021) 2:E11. doi: 10.1017/wtc.2021.11
26. Makinson BJ. Research Development Prototype for Machine Augmentation of Human Strength Endurance Hardiman I Project. (1971). Available online at: https://apps.dtic.mil/sti/pdfs/AD0724797.pdf
27. Tan K, Koyama S, Sakurai H, Teranishi T, Kanada Y, Tanabe S. Wearable robotic exoskeleton for gait reconstruction in patients with spinal cord injury: a literature review. J Orthop Transl. (2021) 28:55–64. doi: 10.1016/j.jot.2021.01.001
28. Angerhöfer C, Colucci A, Vermehren M, Hömberg V, Soekadar SR. Post-stroke rehabilitation of severe upper limb paresis in germany-towards long-term treatment with personalized neurotechnology. Front Neurol. (2021) 2166: 772199. doi: 10.3389/fneur.2021.772199
29. Alguacil-Diego IM, Cuesta-Gómez A, Contreras-González AF, Pont-Esteban D, Cantalejo-Escobar D, Sánchez-Urán MÁ, et al. Validation of a hybrid exoskeleton for upper limb rehabilitation a preliminary study. Sensors. (2021) 21:7342. doi: 10.3390/s21217342
30. Lee SH, Park G, Cho DY, Kim HY, Lee JY, Kim S, et al. Comparisons between end-effector and exoskeleton rehabilitation robots regarding upper extremity function among chronic stroke patients with moderate-to-severe upper limb impairment. Sci Rep. (2020) 10:1806. doi: 10.1038/s41598-020-58630-2
31. Proud JK, Lai DTH, Mudie KL, Carstairs GL, Billing DC, Garofolini A, et al. Exoskeleton application to military manual handling tasks. Hum Factors. (2022) 64:527–54. doi: 10.1177/0018720820957467
32. Riccò M, Ranzieri S, Vezzosi L, Balzarini F, Bragazzi NL. Wearable exoskeletons on the workplaces: knowledge, attitudes and perspectives of health and safety managers on the implementation of exoskeleton technology in Northern Italy. Acta Bio-Medica Atenei Parm. (2022) 92:e2021310.
33. Pesenti M, Antonietti A, Gandolla M, Pedrocchi A. Towards a functional performance validation standard for industrial low-back exoskeletons: state of the art review. Sensors. (2021) 21:808. doi: 10.3390/s21030808
34. Gillette JC, Saadat S, Butler T. Electromyography-based fatigue assessment of an upper body exoskeleton during automotive assembly. Wearable Technol. (2022) 3:e23. doi: 10.1017/wtc.2022.20
35. Golabchi A, Chao A, Tavakoli M. A systematic review of industrial exoskeletons for injury prevention: efficacy evaluation metrics, target tasks, and supported body postures. Sensors. (2022) 22:2714. doi: 10.3390/s22072714
36. Theurel J, Desbrosses K. Occupational exoskeletons: overview of their benefits and limitations in preventing work-related musculoskeletal disorders. IISE Trans Occup Ergon Hum Factors. (2019) 7:264–80. doi: 10.1080/24725838.2019.1638331
37. Kermavnar T, De Vries AW, De Looze MP, O'Sullivan LW. Effects of industrial back-support exoskeletons on body loading and user experience: an updated systematic review. Ergonomics. (2021) 64:685–711. doi: 10.1080/00140139.2020.1870162
38. Bär M, Steinhilber B, Rieger MA, Luger T. The influence of using exoskeletons during occupational tasks on acute physical stress and strain compared to no exoskeleton – A systematic review and meta-analysis. Appl Ergon. (2021) 94:103385. doi: 10.1016/j.apergo.2021.103385
39. Del Ferraro S, Falcone T, Ranavolo A, Molinaro V. The effects of upper-body exoskeletons on human metabolic cost and thermal response during work tasks—a systematic review. Int J Environ Res Public Health. (2020) 17:7374. doi: 10.3390/ijerph17207374
40. Roveda L, Pesenti M, Rossi M, Covarrubias Rodriguez M, Pedrocchi A, Braghin F, et al. User-centered back-support exoskeleton: design and prototyping. Procedia CIRP. (2022) 107:522–7. doi: 10.1016/j.procir.2022.05.019
41. Davis KG, Reid CR, Rempel DD, Treaster D. Introduction to the human factors special issue on user-centered design for exoskeleton. Hum Factors. (2020) 62:333–6. doi: 10.1177/0018720820914312
42. Page MJ, McKenzie JE, Bossuyt PM, Boutron I, Hoffmann TC, Mulrow CD, et al. The PRISMA 2020 statement: an updated guideline for reporting systematic reviews. BMJ. (2021) 372:n71. doi: 10.1136/bmj.n71
43. Booth A. Clear and present questions: formulating questions for evidence based practice. Libr Hi Tech. (2006) 24:355–68. doi: 10.1108/07378830610692127
44. Hong QN, Pluye P, Fàbregues S, Bartlett G, Boardman F, Cargo M, et al. Improving the content validity of the mixed methods appraisal tool: a modified e-Delphi study. J Clin Epidemiol. (2019) 111:49–59. doi: 10.1016/j.jclinepi.2019.03.008
45. Hong QN, Fàbregues S, Bartlett G, Boardman F, Cargo M, Dagenais P, et al. The mixed methods appraisal tool (MMAT) version 2018 for information professionals and researchers. Educ Inf. (2018) 34:285–91. doi: 10.3233/EFI-180221
46. Amandels S., Op het Eyndt H, Daenen L, Hermans V. Introduction and testing of a passive exoskeleton in an industrial working environment. Adv Intell Syst Comput. (2018) 820:387–92. doi: 10.1007/978-3-319-96083-8_51
47. Antwi-Afari MF Li H, Anwer S, Li D, Yu Y, Mi HY, et al. Assessment of a passive exoskeleton system on spinal biomechanics and subjective responses during manual repetitive handling tasks among construction workers. Saf Sci. (2021) 142:105382. doi: 10.1016/j.ssci.2021.105382
48. Cha JS, Monfared S, Stefanidis D, Nussbaum MA Yu D. Supporting surgical teams: identifying needs and barriers for exoskeleton implementation in the operating room. Hum Factors. (2020) 62:377–90. doi: 10.1177/0018720819879271
49. Chae UR, Kim K, Choi J, Hyun DJ, Yun J, Lee GH, et al. Systematic usability evaluation on two harnesses for a wearable chairless exoskeleton. Int J Ind Ergon. (2021) 84:103162. doi: 10.1016/j.ergon.2021.103162
50. Daratany C, Taveira A. Quasi-experimental study of exertion, recovery, and worker perceptions related to passive upper-body exoskeleton use during overhead, low force work. In:Ahram T, Taiar R, Gremeaux-Bader V, Aminian K, , editors. Human Interaction, Emerging Technologies and Future Applications II. Cham: Springer International Publishing (2020) p. 369–73.
51. De Bock S, Ghillebert J, Govaerts R, Elprama SA, Marusic U, Serrien B, et al. Passive shoulder exoskeletons: more effective in the lab than in the field? IEEE Trans Neural Syst Rehabil Eng Publ IEEE Eng Med Biol Soc. (2021) 29:173–83. doi: 10.1109/TNSRE.2020.3041906
52. De Vries AW, Krause F, de Looze MP. The effectivity of a passive arm support exoskeleton in reducing muscle activation and perceived exertion during plastering activities. Ergonomics. (2021) 64:712–21. doi: 10.1080/00140139.2020.1868581
53. Flor R, Gaspar J, Fujão C, Nunes IL. How workers perceive LAEVO exoskeleton use in non-cyclic tasks. In:Nunes IL, , editor. Advances in Human Factors and System Interactions. Cham: Springer International Publishing (2021) p. 147–54.
54. Gilotta S, Spada S, Ghibaudo L, Isoardi M, Mosso CO. Acceptability beyond usability: a manufacturing case study. In:Bagnara S, Tartaglia R, Albolino S, Alexander T, Fujita Y, , editors. Proceedings of the 20th Congress of the International Ergonomics Association (IEA 2018). Cham: Springer International Publishing (2019) p. 922–34. doi: 10.1007/978-3-319-96071-5_95
55. Hensel R, Keil M. Subjective evaluation of a passive industrial exoskeleton for lower-back support: a field study in the automotive sector. IISE Trans Occup Ergon Hum Factors. (2019) 7:213–21. doi: 10.1080/24725838.2019.1573770
56. Hwang J, Kumar Yerriboina VN, Ari H, Kim JH. Effects of passive back-support exoskeletons on physical demands and usability during patient transfer tasks. Appl Ergon. (2021) 93:103373. doi: 10.1016/j.apergo.2021.103373
57. Kim S, Nussbaum MA, Smets M, Ranganathan S. Effects of an arm-support exoskeleton on perceived work intensity and musculoskeletal discomfort: An 18-month field study in automotive assembly. Am J Ind Med. (2021) 64:905–14. doi: 10.1002/ajim.23282
58. Kim S, Nussbaum MA, Smets M. Usability, user acceptance, and health outcomes of arm-support exoskeleton use in automotive assembly: an 18-month field study. J Occup Environ Med. (2022) 64:202–11. doi: 10.1097/JOM.0000000000002438
59. Liu S, Hemming D, Luo RB, Reynolds J, Delong JC, Sandler BJ, et al. Solving the surgeon ergonomic crisis with surgical exosuit. Surg Endosc. (2018) 32:236–44. doi: 10.1007/s00464-017-5667-x
60. Motmans R, Debaets T, Chrispeels S. Effect of a passive exoskeleton on muscle activity and posture during order picking. In:Bagnara S, Tartaglia R, Albolino S, Alexander T, Fujita Y, , editors. Proceedings of the 20th Congress of the International Ergonomics Association (IEA 2018). Cham: Springer International Publishing (2019) p. 338–46.
61. Moyon A, Poirson E, Petiot JF. Experimental study of the physical impact of a passive exoskeleton on manual sanding operations. Procedia CIRP. (2018) 70:284–9. doi: 10.1016/j.procir.2018.04.028
62. Omoniyi A, Trask C, Milosavljevic S, Thamsuwan O. Farmers' perceptions of exoskeleton use on farms: finding the right tool for the work(er). Int J Ind Ergon. (2020) 80:103036. doi: 10.1016/j.ergon.2020.103036
63. Pacifico I, Parri A, Taglione S, Sabatini AM, Violante FS, Molteni F, et al. Exoskeletons for workers: a case series study in an enclosures production line. Appl Ergon. (2022) 101:103679. doi: 10.1016/j.apergo.2022.103679
64. Siedl SM, Mara M. Exoskeleton acceptance and its relationship to self-efficacy enhancement, perceived usefulness, and physical relief: A field study among logistics workers. Wearable Technol. (2021) 2:e10. doi: 10.1017/wtc.2021.10
65. Smets M, A. field evaluation of arm-support exoskeletons for overhead work applications in automotive assembly. IISE Trans Occup Ergon Hum Factors. (2019) 7:1–7. doi: 10.1080/24725838.2018.1563010
66. Spada S, Ghibaudo L, Carnazzo C, Gastaldi L, Cavatorta MP. Passive upper limb exoskeletons: an experimental campaign with workers. In:Bagnara S, Tartaglia R, Albolino S, Alexander T, Fujita Y, , editors. Proceedings of the 20th Congress of the International Ergonomics Association (IEA 2018). Cham: Springer International Publishing (2019) p. 230–9. doi: 10.1007/978-3-319-96068-5_26
67. Turja T, Saurio R, Katila J, Hennala L, Pekkarinen S, Melkas H. Intention to use exoskeletons in geriatric care work: need for ergonomic and social design. Ergon Des. (2022) 30:13–6. doi: 10.1177/1064804620961577
68. Winter G, Felten C, Hedtmann J. Testing of exoskeletons in the context of logistics - application and limits of use. Stephanidis C, editor. In: HCI International 2019 - Posters. Cham: Springer International Publishing; 2019. p. 265–70.
69. Ziaei M, Choobineh A, Ghaem H, Abdoli-Eramaki M. Evaluation of a passive low-back support exoskeleton (Ergo-Vest) for manual waste collection. Ergonomics. (2021) 64:1255–70. doi: 10.1080/00140139.2021.1915502
70. De Looze MP, Bosch T, Krause F, Stadler KS, O'Sullivan LW. Exoskeletons for industrial application and their potential effects on physical work load. Ergonomics. (2016) 59:671–81. doi: 10.1080/00140139.2015.1081988
71. Brooke J. SUS: A “Quick and Dirty” Usability Scale Usability Evaluation In Industry. Boca Raton, FL: CRC Press (1996).
72. Sauro J, James RL. Quantifying the User Experience: Practical Statistics for User Research. Burlington, MA: Morgan Kaufmann (2016).
73. Han SH, Yun MH, Kwahk J, Hong SW. Usability of consumer electronic products. Int J Ind Ergon. (2001) 28:143–51. doi: 10.1016/S0169-8141(01)00025-7
74. Laugwitz B, Held T, Schrepp M. Construction and evaluation of a user experience questionnaire. In:Holzinger A, , editor. HCI and Usability for Education and Work. Berlin; Heidelberg: Springer (2008). p. 63–76.
76. Venkatesh V, Davis F. A theoretical extension of the technology acceptance model: four longitudinal field studies. Manag Sci. (2000) 46:186–204.
77. Kothgassner, OD. “TUI.” Technology Usage Inventory. (2012). Available online at: https://www.ffg.at/sites/default/files/allgemeine_downloads/thematische%20programme/programmdokumente/tui_manual.pdf (accessed September 8, 2022).
78. Finstad K. The usability metric for user experience. Interact Comput. (2010) 22:323–7. doi: 10.1016/j.intcom.2010.04.004
79. Abele A, Stief M. Andrä MS. Zur ökonomischen Erfassung beruflicher Selbstwirksamkeitserwartungen-Neukonstruktion einer BSW-Skala. Zeitschrift für Arbeits-und Organisationspsychologi. (2000) 44:145–51. doi: 10.1026//0932-4089.44.3.145
80. Rosen M. Zum Einfluss beruflicher Selbstwirksamkeitserwartungen und persönlicher Ziele auf beruflichen Erfolg: eine empirische Untersuchung bei Arbeitnehmerinnen und Arbeitnehmern in einem mittelständischen Unternehmen. Berlin: Freie Universität Berlin, FB Erziehungswissenschaft und Psychologie. (2004).
81. Hart SG, Staveland LE. Development of NASA-TLX (Task Load Index): results of empirical and theoretical research. In: Hancock PA, Meshkati N, editors Advances in Psychology. (1988) 11:139–83. doi: 10.1016/S0166-4115(08)62386-9
82. Williams N. The Borg Rating of Perceived Exertion (RPE) scale. Occup Med. (2017) 67:404–5. doi: 10.1093/occmed/kqx063
83. Van der Grinten, Maarten P., and P. Smitt. “Development of a practical method for measuring body part discomfort.” Advances in industrial ergonomics and safety. (1992) 4635:311-18.
84. Hedge A, Morimoto S, McCrobie D. Effects of keyboard tray geometry on upper body posture and comfort. Ergonomics. (1999) 42:1333–49. doi: 10.1080/001401399184983
85. Bequette B. Physical and cognitive load effects due to a powered lower-body exoskeleton. Hum Factors. (2020) 62:411–23. doi: 10.1177/0018720820907450
86. Toxiri S, Näf MB, Lazzaroni M, Fernández J, Sposito M, Poliero T, et al. Back-support exoskeletons for occupational use: an overview of technological advances and trends. IISE Trans Occup Ergon Hum Factors. (2019) 7:237–49. doi: 10.1080/24725838.2019.1626303
87. Poliero T, Fanti V, Sposito M, Caldwell DG, Natali CD. Active and passive back-support exoskeletons: a comparison in static and dynamic tasks. IEEE Robot Autom Lett. (2022) 7:8463–70. doi: 10.1109/LRA.2022.3188439
88. Muramatsu Y, Kobayashi H, Sato Y, Jiaou H, Hashimoto T, Kobayashi H. Quantitative performance analysis of exoskeleton augmenting devices - muscle suit - for manual worker. Int J Aut Technol. (2011) 5:559–67. doi: 10.20965/ijat.2011.p0559
89. Lazzaroni M, Tabasi A, Toxiri S, Caldwell D, De Momi E, Van Dijk W, et al. Evaluation of an acceleration-based assistive strategy to control a back-support exoskeleton for manual material handling. Wearable Technol. (2020) 1:E9. doi: 10.1017/wtc.2020.8
90. Huysamen K, De Looze M, Bosch T, Ortiz J, Toxiri S, O'Sullivan LW. Assessment of an active industrial exoskeleton to aid dynamic lifting and lowering manual handling tasks. Appl Ergon. (2018) 68:125–31. doi: 10.1016/j.apergo.2017.11.004
91. Da Costa BR, Vieira ER. Risk factors for work-related musculoskeletal disorders: a systematic review of recent longitudinal studies. Am J Ind Med. (2010) 53:285–323. doi: 10.1002/ajim.20750
92. Wearable Exoskeletons to Reduce Physical Load at Work. Blogs. CDC (2022). Available online at: https://blogs.cdc.gov/niosh-science-blog/2016/03/04/exoskeletons/ (accessed October 17, 2022).
93. Howard J, Murashov V, Lowe B, Lu ML. Industrial exoskeletons: Need for intervention effectiveness research. Am J Ind Med. (2019) 1:63. doi: 10.1002/ajim.23080
94. Steinhilber B, Luger T, Schwenkreis P, Middeldorf S, Bork H, Mann B, et al. The use of exoskeletons in the occupational context for primary, secondary, and tertiary prevention of work-related musculoskeletal complaints. IISE Trans Occup Ergon Hum Factors. (2020) 8:132–44. doi: 10.1080/24725838.2020.1844344
95. Butler T, Jason CG. Exoskeletons Used as a PPE for Injury Prevention. Prof Saf. (2019) 64:32–7. doi: 10.1024/1012-5302/a000659
96. Peters M, Wischniewski S. The impact of using exoskeletons on occupational safety and health. Safety and health at work EU-OSHA (2022). Available online at: https://osha.europa.eu/it/publications/impact-using-exoskeletons-occupational-safety-and-health (accessed September 8, 2022).
97. Mucci N, Traversini V, Lulli LG, Baldassarre A, Galea RP, Arcangeli G. Upper limb's injuries in agriculture: a systematic review. Int J Environ Res Public Health. (2020) 17:E4501. doi: 10.3390/ijerph17124501
98. Constantinescu C, Muresan PC, Simon GM. JackEx: the new digital manufacturing resource for optimization of exoskeleton-based factory environments. Procedia CIRP. (2016) 50:508–11. doi: 10.1016/j.procir.2016.05.048
99. Kim S, Madinei S, Alemi MM, Srinivasan D, Nussbaum MA. Assessing the potential for “undesired” effects of passive back-support exoskeleton use during a simulated manual assembly task: Muscle activity, posture, balance, discomfort, and usability. Appl Ergon. (2020) 89:103194. doi: 10.1016/j.apergo.2020.103194
100. Whitfield BH, Costigan PA, Stevenson JM, Smallman CL. Effect of an on-body ergonomic aid on oxygen consumption during a repetitive lifting task. Int J Ind Ergon. (2014) 1:39–44. doi: 10.1016/j.ergon.2013.10.002
101. Zhu Z, Dutta A, Dai F. Exoskeletons for manual material handling – A review and implication for construction applications. Autom Constr. (2021) 122:103493. doi: 10.1016/j.autcon.2020.103493
102. Zhu Y, Weston EB, Mehta RK, Marras WS. Neural and biomechanical tradeoffs associated with human-exoskeleton interactions. Appl Ergon. (2021) 96:103494. doi: 10.1016/j.apergo.2021.103494
103. Salvadore G. Industrial Wearable Robots: A Human Facturing Approach. Mediterranean Conference on Medical and Biological Engineering and Computing Cham: Springer (2019).
104. Bosch T, Van Eck J, Knitel K, de Looze M. The effects of a passive exoskeleton on muscle activity, discomfort and endurance time in forward bending work. Appl Ergon. (2016) 54:212–7. doi: 10.1016/j.apergo.2015.12.003
Keywords: industrial exoskeleton, occupational medicine, work-related musculoskeletal disorders, user experience, Human-Robot Cooperation, ergonomics, occupational safety and health (OSH), personal protection equipment (PPE)
Citation: Baldassarre A, Lulli LG, Cavallo F, Fiorini L, Mariniello A, Mucci N and Arcangeli G (2022) Industrial exoskeletons from bench to field: Human-machine interface and user experience in occupational settings and tasks. Front. Public Health 10:1039680. doi: 10.3389/fpubh.2022.1039680
Received: 08 September 2022; Accepted: 26 October 2022;
Published: 21 November 2022.
Edited by:
Mario Fargnoli, Mercatorum University, ItalyReviewed by:
Maria Lazzaroni, Italian Institute of Technology (IIT), ItalyFederica Caffaro, Roma Tre University, Italy
Davide Gattamelata, National Institute for Insurance Against Accidents at Work (INAIL), Italy
Copyright © 2022 Baldassarre, Lulli, Cavallo, Fiorini, Mariniello, Mucci and Arcangeli. This is an open-access article distributed under the terms of the Creative Commons Attribution License (CC BY). The use, distribution or reproduction in other forums is permitted, provided the original author(s) and the copyright owner(s) are credited and that the original publication in this journal is cited, in accordance with accepted academic practice. No use, distribution or reproduction is permitted which does not comply with these terms.
*Correspondence: Lucrezia Ginevra Lulli, lucreziaginevra.lulli@unifi.it
†These authors have contributed equally to this work and share first authorship