- 1Department of Public Health, Faculty of Medicine, Qinghai University, Xining, China
- 2Xining Centre for Disease Control and Prevention, Xining, China
- 3Qinghai Institute of Health Sciences, Xining, China
- 4Qinghai Province Cardio Cerebrovascular Disease Specialist Hospital, Xining, China
Background: With global climate change, the health impacts of cold spells and air pollution caused by PM2.5 are increasingly aggravated, especially in high-altitude areas, which are particularly sensitive. Exploring their interactions is crucial for public health.
Methods: We collected time-series data on meteorology, air pollution, and various causes of death in Xining. This study employed a time-stratified case-crossover design and conditional logistic regression models to explore the association between cold spells, PM2.5 exposure, and various causes of death, and to assess their interaction. We quantitatively analyzed the interaction using the relative excess odds due to interaction (REOI), attributable proportion due to interaction (AP), and synergy index (S). Moreover, we conducted stratified analyses by average altitude, sex, age, and educational level to identify potential vulnerable groups.
Results: We found significant associations between cold spells, PM2.5, and various causes of death, with noticeable effects on respiratory disease mortality and COPD mortality. We identified significant synergistic effects (REOI>0, AP > 0, S > 1) between cold spells and PM2.5 on various causes of death, which generally weakened with a stricter definition of cold spells and longer duration. It was estimated that up to 9.56% of non-accidental deaths could be attributed to concurrent exposure to cold spells and high-level PM2.5. High-altitude areas, males, the older adults, and individuals with lower educational levels were more sensitive. The interaction mainly varied among age groups, indicating significant impacts and a synergistic action that increased mortality risk.
Conclusion: Our study found that in high-altitude areas, exposure to cold spells and PM2.5 significantly increased the mortality risk from specific diseases among the older adults, males, and those with lower educational levels, and there was an interaction between cold spells and PM2.5. The results underscore the importance of reducing these exposures to protect public health.
1 Introduction
In the current context of global climate change, the frequency and intensity of extreme weather events are showing an upward trend, posing significant threats to human health and society (1). Cold spells, characterized by sudden and severe drops in temperature, together with the ongoing issue of air pollution, exacerbate the risks to public health (2, 3). Several studies have revealed the association between cold environments and PM2.5 pollution with a range of health problems, including cardiovascular diseases, respiratory diseases, and diabetes (4, 5). In high-altitude areas, thin air, strong ultraviolet radiation, and increased human activities may have exacerbated the problem of air pollution in the environment (6–8). Studies have shown that air pollution in high-altitude regions has a significant impact on human health (9, 10). For example, a time-series analysis revealed a correlation between air pollution and respiratory health issues in children in the Xining area (11). Moreover, the impact of indoor air pollution in these areas on residents’ health is even more severe (12, 13). Research on the health impacts of cold spells and air pollution in high-altitude areas is relatively scarce due to their unique geographical and climatic conditions and the specificity of population distribution, limiting our comprehensive understanding of the health impacts of extreme climate and air pollution in these regions. Additionally, the low oxygen and low pressure environment of high-altitude areas may negatively impact health through various physiological mechanisms, such as triggering the activation of hypoxia-inducible factors, enhancing inflammatory responses, and damaging mitochondrial function (14–17). Under extreme weather conditions, such as cold spells, these health effects may be further amplified. Therefore, in high-altitude areas, it is crucial to fully elucidate the correlation between cold spells, air pollution, and health outcomes for a thorough understanding and targeted prevention and adaptation.
Several studies have shown that the combination of extreme weather events and air pollution can have negative effects on human health (18, 19). However, most of these studies have focused on the impact of extreme high temperatures, with less attention given to the potential health impacts of different intensities, frequencies, and durations of extreme weather events such as cold spells. Furthermore, these studies assess the interaction between the two factors by calculating the relative excess risk due to interaction (RERI), but this single indicator does not take into account the possible complex synergistic effects between them. Xining City, being a high-altitude area, may exacerbate the negative health effects of air pollution due to its unique geographical and climatic conditions. The city experiences a six-month heating period during the winter and longer cold periods. This scenario highlights the need for a more comprehensive and detailed assessment method when considering the combined effects of extreme weather events and air pollution in high-altitude areas, in order to fully understand and evaluate the potential health impacts of these environmental factors’ synergistic effects.
To complement existing research, this study aims to quantitatively assess the interactive effects of cold spells and PM2.5 exposure on the risk of various causes of death in high-altitude areas and to calculate the corresponding excess mortality rates and numbers. In addition, this study will conduct stratified analyses to identify potentially vulnerable groups, in particular through stratification by average altitude, to further explore the effects of cold spells in high-altitude areas.
2 Materials and methods
2.1 Data collection and definition of cold spell
We collected mortality data from January 1, 2016, to December 31, 2021, from the Xining City Center for Disease Control and Prevention, including age, sex, level of education, disease diagnosis, and codes according to the International Classification of Diseases, Tenth Revision (ICD-10). The data were categorized by average altitude (2,500 m and 3,000 m), sex (male and female), age (0–64 years and ≥ 65 years), level of education (junior high school and below, high school and above), and specific causes of death: non-accidental (ICD-10:A00-R99), cardiovascular disease (ICD-10:I00-I99), ischemic heart disease (IHD, ICD-10:I20-I25), stroke (ICD-10:I60-I69), respiratory disease (ICD-10:J00-J99), chronic obstructive pulmonary disease (COPD, ICD-10:J40-J47), and diabetes (ICD-10:E10-E14). For altitude classification, we referred to the categories proposed by Bärtsch et al. in 2008 (near sea level 0–500 meters, low altitude 500–2,000 meters, mid altitude 2000–3,000 meters, high altitude 3,000–5,500 meters, extreme altitude above 5,500 meters) (20, 21). Meteorological data were obtained from the Qinghai Provincial Meteorological Bureau, including daily average temperature and humidity, with no missing data. Air pollutant data were sourced from the China Air Quality Online Monitoring and Analysis Platform,1 supplemented by five national control monitoring stations in the urban area of Xining. The overall missing rate of air pollutant data was about 1.76%, including daily average concentrations of PM2.5, PM10, O3, SO2, NO2, and CO. For missing air pollution data, we used the median imputation method for data filling. We used Spearman’s correlation analysis of meteorological factors and pollutants.
Based on previous studies, cold spells were defined as daily average temperatures falling below specific percentiles (2.5, 5, 7.5, or 10th) and persisting for a minimum of 2 to 4 consecutive days (22, 23).
2.2 Study sites
Xining City is located in the northwest of China and the northeast of the Tibetan Plateau, with an altitude range of 2091–4,857 meters, making it one of the world’s high-altitude cities. The terrain is higher in the southwest and lower in the northeast, with a total population of about 2.4756 million people (in 2021), accounting for approximately 42% of Qinghai Province (Figure 1). The climate is characterized by a high mountain plateau climate, with cold and prolonged winters and pleasant summers.
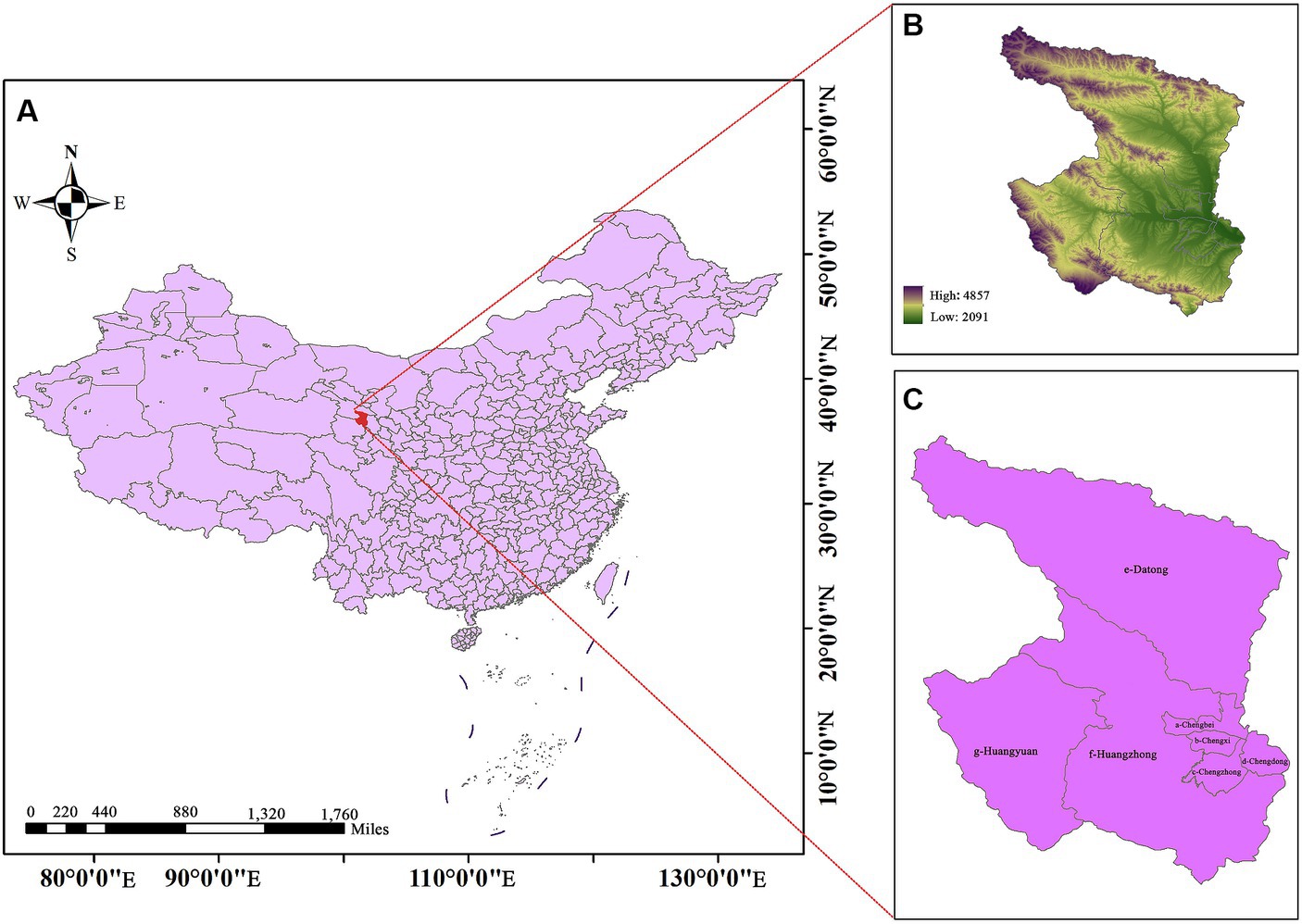
Figure 1. Location, altitude range and topography of Xining, China (C: a is Chengbei District, b is Chengxi District, c is Chengzhong District, d is Chengdong District, e is Datong County, f is Huangzhong District, and g is Huangyuan County). The average altitude of a-d is about 2500 m, and the average altitude of e-g is about 3000 m.
2.3 Statistical analysis
We used a time-stratified case-crossover design and conditional logistic regression to quantitatively analyze the association between cold spells, PM2.5, and mortality (24). The case-crossover design considers each study subject as its own control, with the visit date defined as the case day, and other dates in the same year, month, and week as the case day defined as control days. Each case period is matched with three or four control periods before or after the case period to control for long-term trends, seasonal trends, and the effects of the day of the week.
A distributed lag non-linear model (DLNM) was applied to fit separate exposure-response and lag-response relationships for cold spells, PM2.5, and mortality (25). Based on previous studies, the exposure-response relationship was modeled using a linear function (26), while the lag-response relationship was fitted using a natural spline function with 3 degrees of freedom (df) (23). Previous studies have shown that the lag effect for cold spells was typically 21 days (4), while for PM2.5 it was 7 days (27). A natural spline with 3 df was used to control for the confounding effects of relative humidity (28). The formula was as follows:
where E(Y) is the expected daily number of deaths; α is the intercept; cb(CS), cb(pm) are the cross-basis functions for cold spells and PM2.5, respectively, used to examine lag effects; stratum is the time stratification variable, used to control for the impact of time factors such as long-term trends and seasonal changes; ns(rh, 3) is the natural cubic spline for relative humidity with 3 df; holiday is a binary variable used to control for Chinese holidays.
To further evaluate the interactive effects of exposure to cold spells and PM2.5 on mortality, we classified PM2.5 exposure into a binary variable (low concentration: ≤37.5 μg/m3, high concentration: >37.5 μg/m3) according to the interim target 3 for PM2.5 in the World Health Organization’s 2021 air quality guidelines (29, 30). We created a new variable with four levels representing the combinations of exposure to cold spells and PM2.5, including: (1) non-cold spell and low-level PM2.5 (Level 1); (2) cold spell and low-level PM2.5 (Level 2); (3) non-cold spell and high-level PM2.5 (Level 3); (4) cold spell and high-level PM2.5 (Level 4), with Level 1 serving as the reference group. By incorporating this variable into the conditional logistic regression model, we used three measures to assess this impact, including the relative excess odds due to interaction (REOI), the proportion attributable to interaction (AP), and the synergy index (S), which represents the part of the effect due to interaction (31, 32). The proportion of the joint effect due to interaction, as well as the ratio of the joint effect to the independent effects, were calculated using the following formulas:
where OR10, OR01, OR11 are the OR values of Levels 2, 3, and 4 relative to Level 1 (OR00 = 1), respectively. REOI = 0, AP = 0, S = 1 indicates that there is no interaction between cold spells and PM2.5 on mortality; REOI>0, AP > 0, S > 1 indicates that the combined effect of cold spells and PM2.5 on mortality is greater than the sum of the effects of exposure alone (synergistic effect); whereas REOI<0, AP < 0, S < 1 indicates that the combined effect is less than the sum of the effects of each exposure alone. The 95% confidence intervals (CI) for the three indicators are calculated using the delta method (33).
To estimate the excess mortality attributable to simultaneous exposure to cold spells and high levels of PM2.5, this study used the calculation method [exp(β)-1], where β represents the coefficient of the fourth level of exposure in the conditional logistic regression model (34).
To identify potential vulnerable groups, we classified them by altitude, sex, age, and level of education to assess the independent impacts of cold spells and PM2.5 on non-accidental deaths and their interaction. We used a two-sample z-test to examine the differences in the effects estimated for each stratified variable (35–37).
where β represents the specific point estimate in the conditional logistic regression model; SE represents the standard error corresponding to each β.
2.4 Sensitivity analysis
Several sensitivity analyses were performed to test the robustness of our results. The lag days for cold spells were adjusted from 0–21 days to 0–27 days, and for PM2.5 from 0–7 days to 0–10 days, while the degrees of freedom for relative humidity in the model were adjusted from 3 to 6. Simultaneously, we incorporated individual air pollutants (NO2, CO, SO2, and O3) and a combination of air pollutants (NO2 & SO2 & CO) into the model. A new variable of different levels was created using the value of 39.5 μg/m3 for PM2.5 categorization to observe changes in the synergistic effect. Moreover, to observe potential disturbances brought by the COVID-19 pandemic, we divided the study data into two periods: 2016–2019 as the pre-pandemic control period and 2020–2021 as the pandemic period. This study primarily utilized R software for statistical analysis (version 4.3.1). p < 0.05 (two-sided) were considered statistically significant.
3 Results
Supplementary Table S1 presents a descriptive analysis of meteorological elements, atmospheric pollutants, and the number of specific causes of death. During the study period, the average daily temperature and relative humidity in Xining City were 6.46 ± 9.15 (°C) and 56.69 ± 16.20 (%), respectively. The daily average concentrations of PM2.5, SO2, NO2, CO, and O3 were 40.20 ± 27.90 μg/m3, 20.03 ± 13.12 μg/m3, 39.31 ± 16.11 μg/m3, 1.36 ± 0.78 mg/m3, and 93.31 ± 33.47 μg/m3, respectively. From 2016 to 2021, there were a total of 64,128 non-accidental deaths, 29,906 deaths from circulatory disease, 8,553 from respiratory disease, 11,617 from IHD, 12,237 from stroke, 7,074 from COPD, and 2,560 from diabetes. Figure 2 reveals the trend of PM2.5 versus temperature over time, showing the temporal synchronization of high PM2.5 levels with the occurrence of low temperatures. A low to moderate correlation existed between the average daily temperature and other variables (p < 0.05; Supplementary Figure S1). Among these variables, the correlation between PM2.5 and PM10 was relatively strong (p < 0.05), with a correlation coefficient greater than 0.8. However, the correlation between O3 and relative humidity was minimal (p > 0.05).
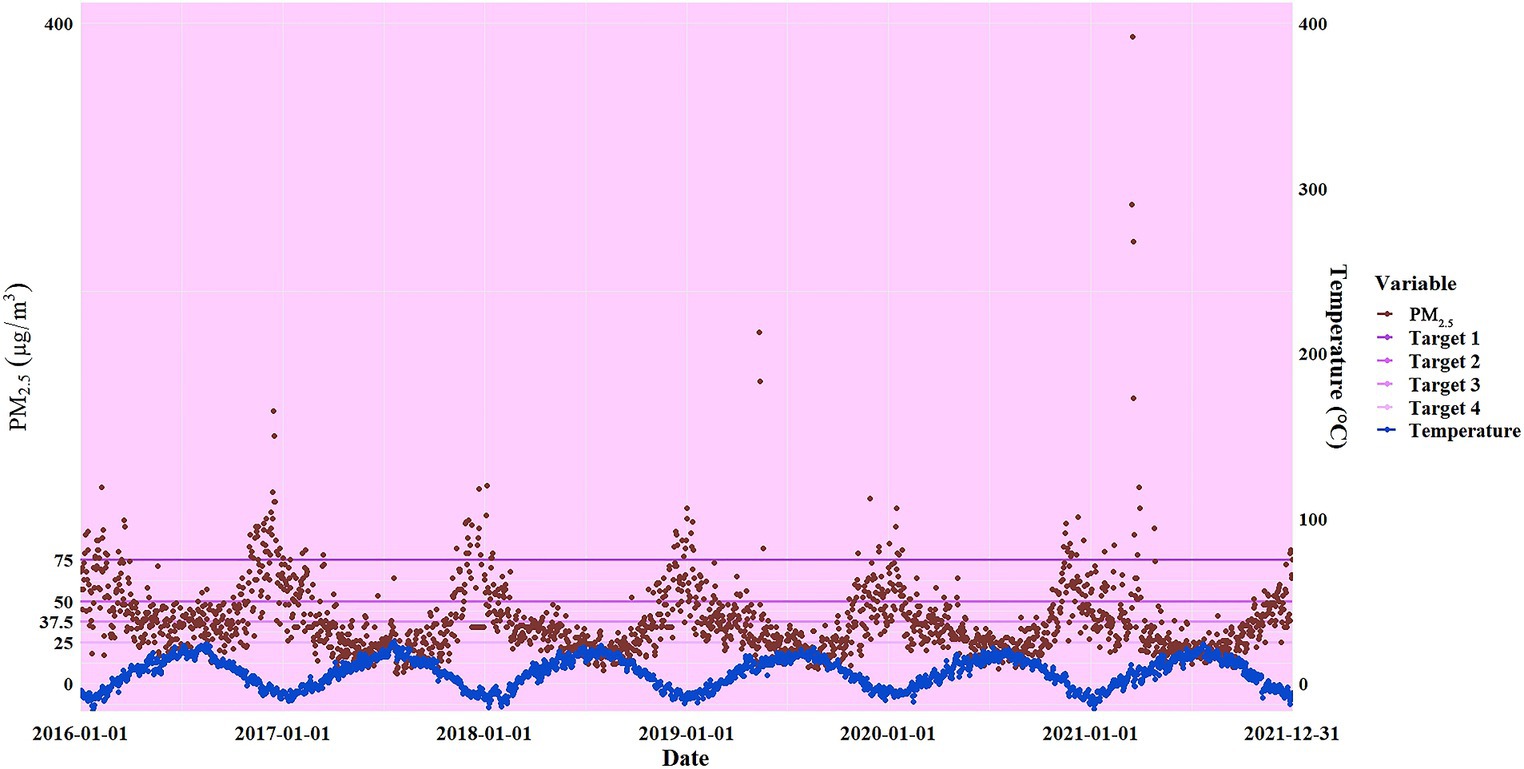
Figure 2. Trends in temperature and PM2.5 over time and interim targets for daily average PM2.5 concentrations according to the World Health Organization’s 2021 Air Quality Guidelines (interim target 4 is 25 μg/m3, 3 is 37.5 μg/m3, 2 is 50 μg/m3, 4 is 75 μg/m3, and HIGH is greater than 75 μg/m3).
Table 1 shows the number of non-accidental deaths at different exposure levels in Xining City from 2016 to 2021. According to the definition of a cold spell by 7th4D, there were 142 cold spell days, with 4,102 cases (6.4%) of deaths occurring on cold spell days, and the majority of deaths (60,026 cases) occurred on non-cold spell days. Among these, 87.9% (3,605) of the deaths occurred under the condition of simultaneous exposure to cold spells and high levels of PM2.5, while 12.1% (497) occurred under simultaneous exposure to cold spells and low levels of PM2.5. Overall, the number of non-accidental deaths during cold spell days decreased with lower temperature thresholds and longer durations.
Figures 3A–C and Supplementary Figures S2A–D show the relationship between exposure to cold spells and various causes of death. We observed a significant increase in the risk of death from all causes associated with exposure to cold spells. According to the 5th2D definition of cold spells, the odds ratios (OR) for total non-accidental deaths, deaths from circulatory disease, and respiratory disease were 1.168 (95%CI: 1.085, 1.258), 1.182 (1.061, 1.317), and 1.556 (1.294, 1.871), respectively, indicating an increase in death risk of 16.8% (8.5, 25.8%), 18.2% (6.1, 31.7%), and 55.6% (29.4, 87.1%). Overall, the risk of death decreased with stricter definitions of cold spells, and the confidence intervals of effect estimates became wider, with a higher risk of death from respiratory disease.
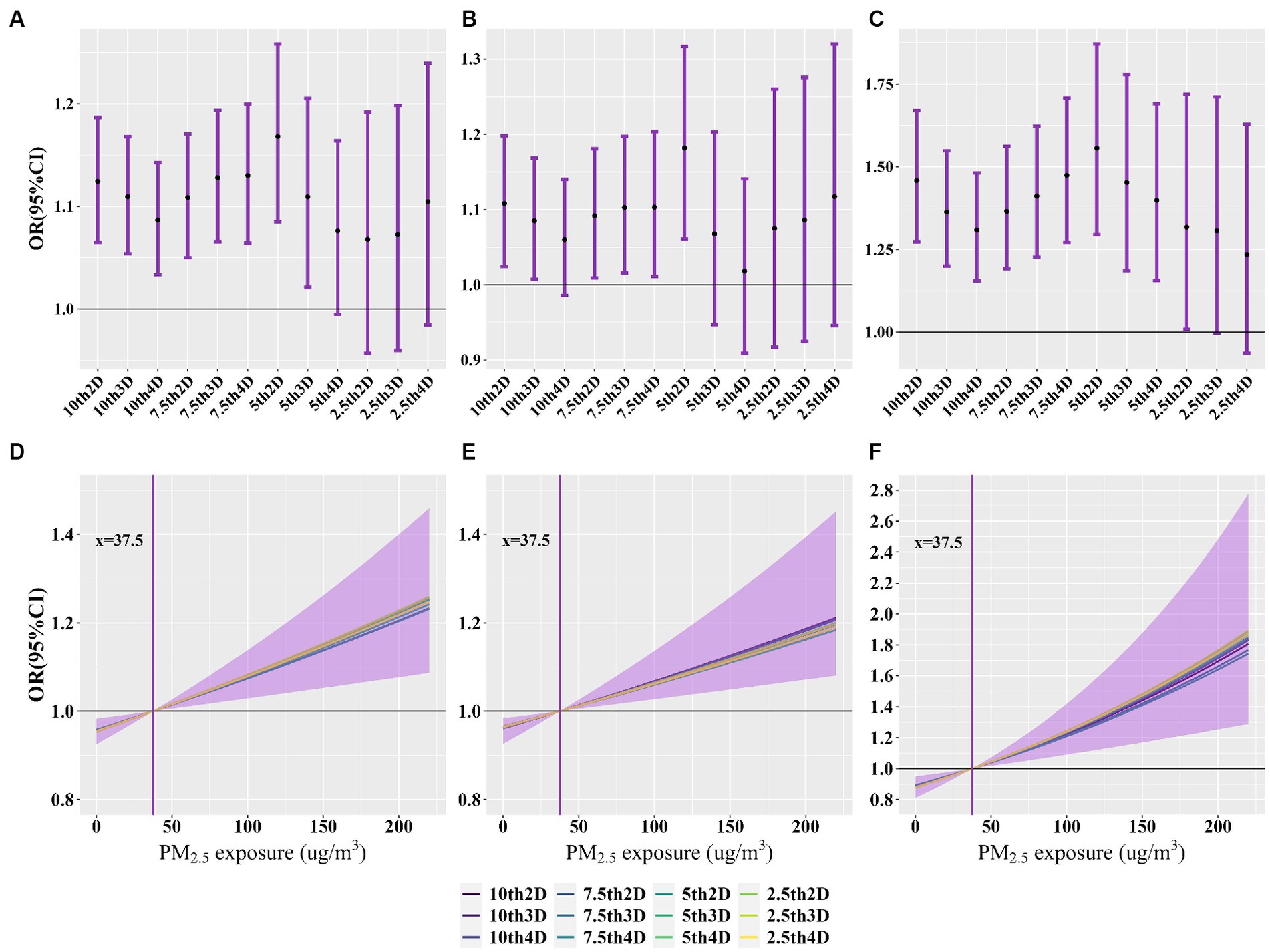
Figure 3. Association of exposure to cold spells and PM2.5 with non-accidental, cardiovascular and respiratory disease deaths. (A–C) OR (95%CI) for nonaccidental, cardiovascular, and respiratory disease deaths with exposure to cold spells, respectively. (D–F) Exposure-response curves for the association between exposure to ambient PM2.5 and non-accidental, cardiovascular and respiratory disease deaths, respectively.
Figures 3D–F and Supplementary Figures S2E–H show the association between exposure to PM2.5 and various causes of death. After adjusting for different definitions of cold spells in the model, the OR for total non-accidental deaths, deaths from circulatory disease, and respiratory disease monotonically increased with higher exposure to PM2.5, with the highest risk for respiratory disease.
Figure 4 and Supplementary Figure S3 show the additive interactive effects on specific causes of death due to exposure to cold spells and PM2.5. According to the 7th4D definition of cold spells, the REOI for total non-accidental deaths, deaths from circulatory disease, respiratory disease, IHD, stroke, COPD, and diabetes were 0.159 (95%CI: 0.045, 0.272), 0.173 (0.012, 0.334), 0.501 (0.199, 0.802), 0.353 (0.077, 0.628), 0.431 (0.189, 0.673), 0.354 (0.009, 0.699), and 1.109 (0.621, 1.598), respectively. Except for IHD, COPD, and diabetes, other causes indicated a significant synergistic effect of exposure to cold spells and PM2.5 on mortality (indicated by REOI >0, AP >0, and S > 1; all p < 0.05). Overall, the REOI, AP, and S decreased with lower temperature thresholds and longer durations of cold spells, and the confidence intervals also became wider.
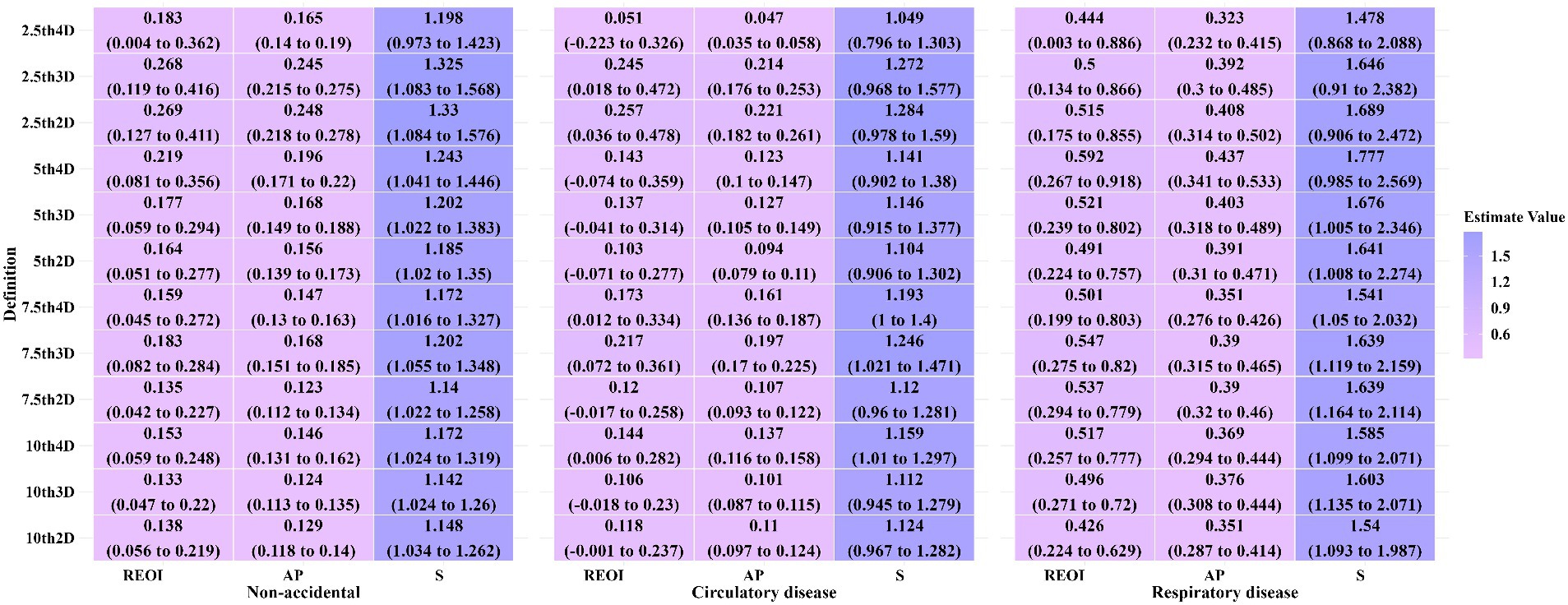
Figure 4. Additive interaction effects of exposure to cold periods and PM2.5 on non-accidental, cardiovascular and respiratory disease mortality.
Figure 5 and Supplementary Figures S4, S5 show the excess fraction and number of excess deaths due to exposure to cold spells and high-levels of PM2.5. According to the 7th3D definition of cold spells, the excess mortality rates for total non-accidental deaths, deaths from circulatory disease, respiratory disease, IHD, stroke, COPD, and diabetes were 0.083 (95%CI: 0.007, 0.166), 0.070 (−0.038, 0.191), 0.428 (0.188, 0.716), 0.330 (0.129, 0.567), 0.216 (0.033, 0.430), 0.421 (0.183, 0.708), and 0.607 (0.179, 1.191), respectively. Overall, significant findings were mainly concentrated between 7th2D to 7th4D, with lower excess mortality rates associated with lower temperature thresholds and longer durations of cold spells.
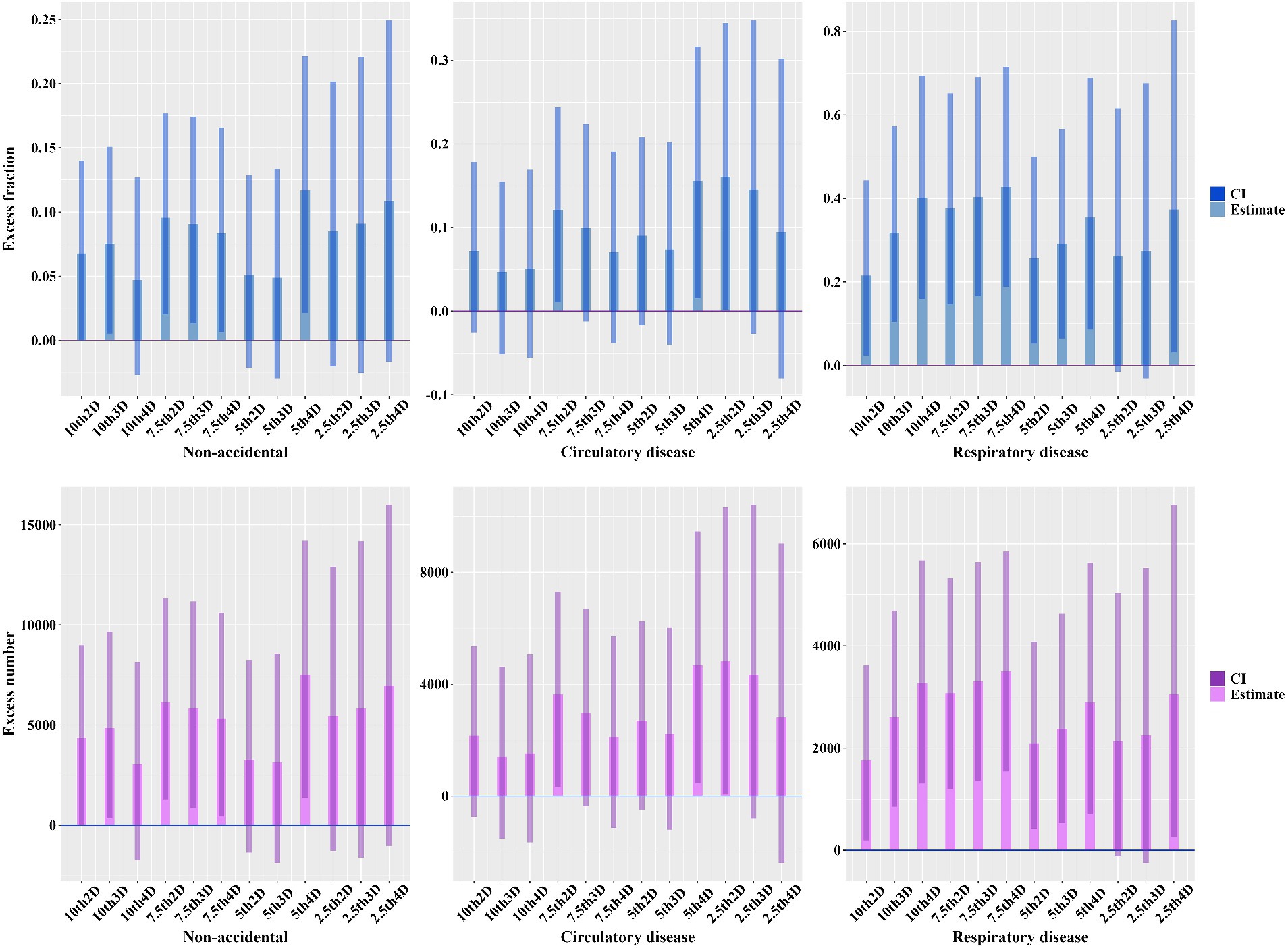
Figure 5. Excess fraction and number of excess deaths due to exposure to cold spells and high-level PM2.5.
Table 2 and Supplementary Figures S6, S7 show the OR and additive interactive effects for non-accidental deaths under different definitions of cold spells, stratified by average altitude, sex, age, and education level. We observed that, in the independent effects of exposure to cold spells on non-accidental deaths, the effect was slightly higher in populations living at an average altitude of 3,000 m compared to those at 2,500 m, slightly higher in males compared to females, lower in the 0–64 age group compared to those aged ≥65, and higher in individuals with lower educational levels compared to those with higher educational levels; however, no statistically significant differences were observed between them (p > 0.05; Supplementary Figure S6). In the independent effects of exposure to PM2.5 on non-accidental deaths, the effect was slightly lower in populations living at an average altitude of 3,000 m compared to those at 2,500 m, similar between males and females, lower in the 0–64 age group compared to those aged ≥65, and higher in individuals with lower educational levels compared to those with higher educational levels, but no significant differences were observed across the groups (p > 0.05; Supplementary Figure S7). We observed a significant synergistic effect (p < 0.05; Table 2) in the interaction between exposure to cold spells and PM2.5 on non-accidental deaths for the age group ≥65 compared with the age group 0–64 in the 7th3D and 7th4D definitions, indicating a significant synergistic effect. No significant differences in the synergistic effect (meeting the conditions of REOI >0, AP >0, and S > 1) were observed between average altitude, sex, or education level (p > 0.05; Supplementary Tables S2, S4).
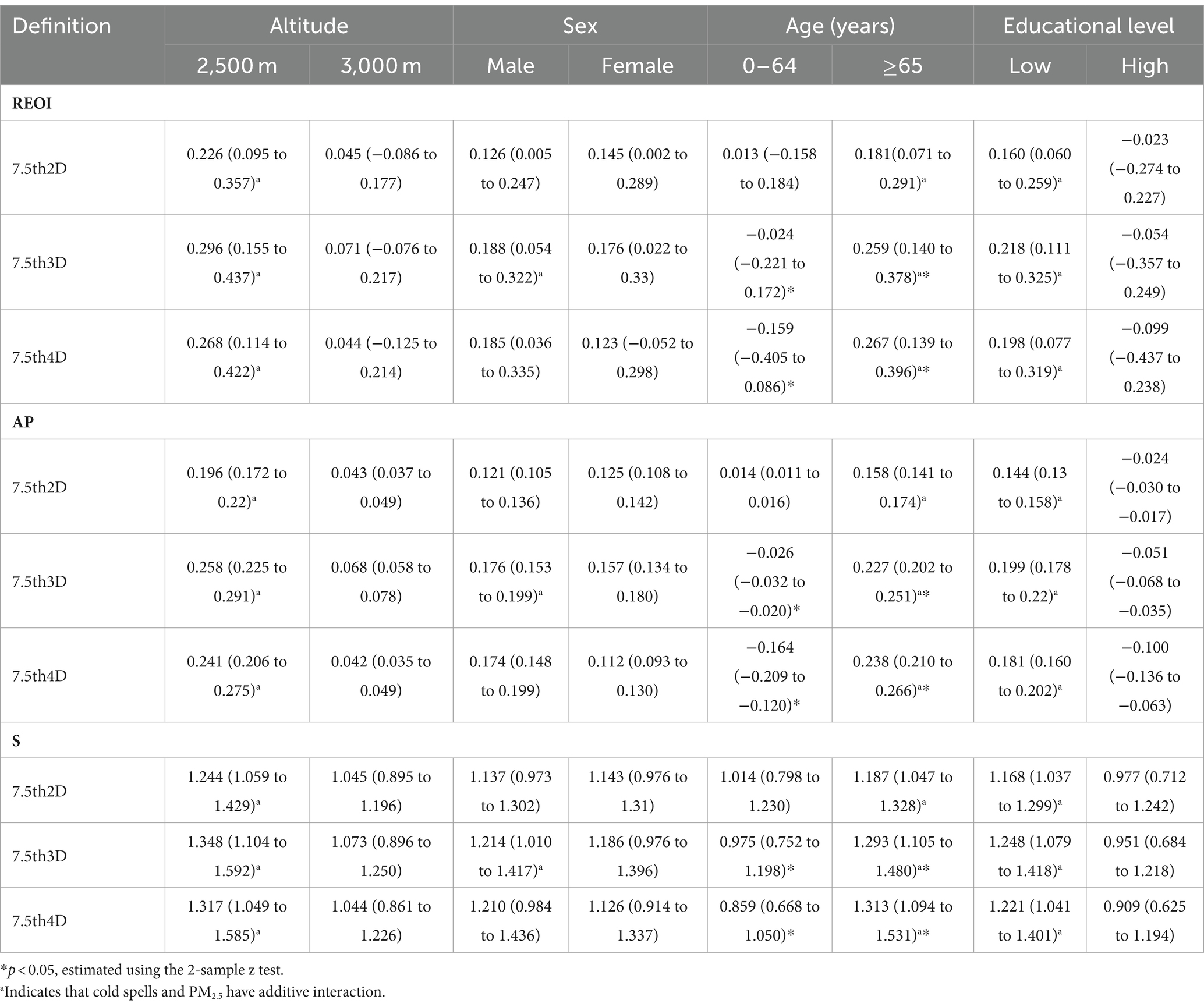
Table 2. The REOI, AP and S of exposure to cold spells and PM2.5 on non-accidental mortality, by average altitude, sex, age and education level.
By adjusting the lag days for cold spells from 0–21 days to 0–27 days, the lag days for PM2.5 from 0–7 days to 0–10 days, modifying the degrees of freedom for relative humidity in the model, and including both individual air pollutants (SO2, NO2, CO,and O3) and combined air pollutants (NO2&SO2&CO) in a sensitivity analysis, the estimated values for both independent and interactive effects were similar, showing minor variations (Supplementary Figures S8–S10). When using a PM2.5 categorization value of 39.5 μg/m3, the association between cold spells and PM2.5 with non-accidental deaths was stable (Supplementary Figures S11, S12), but the estimated value for the interaction effect between exposure to cold spells and PM2.5 on non-accidental deaths slightly decreased. Furthermore, the study results from different periods suggested that, during 2020–2021, the individual effects of cold spells and PM2.5 slightly decreased compared to previous years (Supplementary Figure S13), with the COVID-19 pandemic potentially acting as a confounding factor but also possibly related to the reduced time span and global warming. This indicated that the impact of large-scale public health events on assessing the health effects of environmental factors needs to be considered.
4 Discussion
We studied the association between cold spells and PM2.5 with mortality in high-altitude areas (Xining) and quantified their interaction. In this case-crossover study, we found a significant correlation between exposure to cold spells and PM2.5 and an increase in various causes of death. Cold spells could synergistically interact with PM2.5 to increase the risk of death, especially the risk of respiratory disease and COPD deaths. The individual effects of cold spells and PM2.5, as well as their interaction on various causes of death, decreased with lower temperature thresholds and longer durations. Populations that lived at an altitude of 3,000 m, males, the older adults, and those with lower educational levels seemed to be more susceptible to cold spells. The interaction between cold spells and PM2.5 mainly varied across age groups (p < 0.05).
This study has identified a significant correlation between cold spells and various causes of death, providing an important supplement to the field of research on the impact of extreme climate conditions on human health. This study found results similar to those in Ningbo (OR 95%CI: 1.156, 1.095–1.221), Tianjin (1.26:1.15–1.39), and Jinan (1.08:1.06–1.11) regarding non-accidental deaths (22, 38, 39). This finding not only confirms the consistency of the increased risk of non-accidental deaths due to cold spells but also highlights the importance of conducting research in different geographical locations. Cold spells significantly impact public health in high-altitude areas. Research data indicate that during the cold period, there is a noticeable increase in the mortality risk in highland areas such as Tibet, Xining, and Yuxi (40–42). For instance, a study focusing on provincial capitals in China shows that during cold waves, the mortality risk in Xining is higher than in other western cities and similar latitude plain areas (22). In Tibet, studies also demonstrate that as temperatures continue to drop, the risk of death increases (42). These findings emphasize that in high-altitude regions, geographic and climatic characteristics play a crucial role in assessing health risks, which is particularly important for developing effective response strategies. In terms of specific disease categories, the risk of death from circulatory disease in this study was close to that in Jinan (1.06:1.03–1.10) (38). For the risk of respiratory disease, this study was close to those in Ningbo (1.444: 1.173–1.777) and Nanjing (1.54:1.16–2.04) (39, 43). These findings further emphasize the importance of protecting the cardiovascular and respiratory systems during cold spell events. Studies in southern China (such as Shenzhen, Guangzhou, and Wuhan) show that the risk of non-accidental deaths, cardiovascular disease, and respiratory disease due to cold spells was higher than the findings of this study (22, 44, 45), which could be related to different climate adaptabilities and cold adaptabilities of populations across regions, highlighting the role of environmental conditions in affecting the health effects of cold spells (46–48). Xining, being exposed to lower temperatures for longer periods, has a stronger cold adaptation. At the same time, significant differences between indoor and outdoor temperatures may exacerbate the risk of death from circulatory and respiratory disease (49, 50). Notably, the heating period in Xining lasts up to half a year, which may further amplify the impact of temperature differences on diseases. Additionally, the heterogeneity of this study’s results may stem from inconsistencies in the definition of cold spells, the diversity of research methods, and population size differences. In terms of the risk of diabetes mortality, this study’s results were higher than those in Harbin (1.223:1.054–1.418), Chongqing (1.201:1.006–1.434), and Korea (2.02: 1.37–2.99) (51, 52), which may be related to a higher comorbidity rate of diabetes patients in this region and other chronic diseases such as hypertension, cerebrovascular disease, kidney disease, etc.
This study reveals that cold spells significantly increase the risk of circulatory and respiratory disease, associated with a series of physiological changes triggered by cold environments. Conditions of cold increase platelet and red blood cell counts, blood viscosity, and arterial pressure, collectively promoting thrombosis (53), and increasing the risk of heart attacks or strokes. Additionally, peripheral vasoconstriction leads to elevated blood pressure (54), further increasing the probability of cardiovascular diseases (55), such as hypertension (56). Regarding the respiratory system, cold environments may increase the number of granulocytes and macrophages in the respiratory tract (57), causing the respiratory mucosa to produce more mucus (58), leading to airway obstruction and inflammation (59). Additionally, it can cause reflexive constriction of the airways (60), increasing the risk of asthma and chronic cough. Furthermore, the cold affects the local immune defense of the respiratory tract (61), increasing the possibility of respiratory infections (62), such as pneumonia. This study further confirms the widespread consensus on the significant association between PM2.5 and various causes of death (63, 64). Numerous experimental studies show that PM2.5 can increase the risk of cardiovascular diseases by triggering inflammatory responses and oxidative stress (65), affecting vascular function, and promoting arteriosclerosis (66), thereby causing blood pressure to rise and cardiovascular events (67). Inhaling PM2.5 also acts directly on the respiratory tract, inducing inflammation and airway allergic reactions, exacerbating diseases like asthma and COPD (65, 68). These findings highlight the importance of reducing PM2.5 exposure to lower the risk of related diseases.
Despite increasing studies focusing on the synergistic effects of extreme weather events and PM2.5 on human health, a comprehensive assessment of the synergistic effects of cold spells and PM2.5 on specific causes of death is still lacking. This study, for the first time, uses the REOI, AP, and S to comprehensively assess the health impacts of cold spells and PM2.5 interactions of varying intensities and durations, providing a new perspective for research in this field. This study confirms the synergistic effect of combined exposure to cold spells and PM2.5 on specific causes of death, especially in increasing the risk of respiratory deaths, aligning with previous research results from the Shanghai region (69). As the intensity and duration of cold spells increased, the independent impact of the cold spells and their interaction with PM2.5 on health appeared to diminish. This phenomenon is partly due to the strict definition of cold waves, which not only reduces the frequency of such events but also integrates with a more efficient early warning system. Therefore, the public is able to receive alerts in a timely manner and take corresponding preventive measures, such as enhancing warmth and limiting outdoor activities, effectively reducing health risks. However, some studies have not observed significant interactions between cold spells and PM2.5 (19), even suggesting a possible antagonistic effect between the two (18, 70). These differences may be due to geographical variations, including climate characteristics, residents’ adaptability, and differences in assessment methods. Other factors such as socioeconomic status, individual health conditions, and regional public health policies may also influence the results. Additionally, this study reveals that combined exposure to cold spells and high levels of PM2.5 can lead to an excess mortality rate of up to 9.56% (95%CI: 2.02, 17.66%; non-accidental deaths). Therefore, optimizing early warning services for extreme weather events by reducing PM2.5 exposure can significantly enhance public health protection. This conclusion highlights the importance of integrating air quality management when developing strategies to respond to extreme weather events and taking corresponding measures to reduce PM2.5 exposure to mitigate its health impacts.
Our stratified analysis shows that the impact of cold spells varies among different populations, with those living at an average altitude of 3,000 m, males, the older adults, and individuals with lower educational levels being more sensitive. Populations residing at altitudes above 3,000 meters appear to be more sensitive to cold spells, possibly due to the combined effects of low temperatures and low oxygen environments at high altitudes on human physiological mechanisms (71, 72). These conditions may lead to increased blood pressure, and chronic residence can also cause chronic mountain sickness and endothelial dysfunction of the cardiovascular system (73). With increased altitude, the health risks individuals face also increase, including the risks of cardiovascular and respiratory disease (17, 74). From a social perspective, due to economic constraints, regions above 3,000 meters have insufficient heating and insulation facilities compared to lower altitudes. Conversely, the city center, at an altitude of 2,500 meters and with more frequent economic activities, faces the main challenge of higher PM2.5 pollution. This also demonstrates the environmental challenges faced by different areas. In cold environments, males show more sensitive physiological responses than females, especially in terms of elevated blood pressure, cardiac responses, and metabolic reactions (75, 76). Meanwhile, short-term cold exposure increases central aortic pressure and cardiac load, further increasing cardiovascular health risks (77). Additionally, males are more likely to engage in outdoor work, experience greater indoor and outdoor temperature differences, and have smoking habits, all of which may diminish their adaptability to extreme environments. With the degradation of physiological processes and the immune system (78, 79), the older adults are more susceptible to extreme weather events and atmospheric pollutants, potentially leading to a higher incidence of pre-existing conditions. Individuals with lower educational levels are more susceptible to cold spells and PM2.5, possibly related to factors such as low income, household sanitation conditions, insufficient heating facilities, shallow protective awareness, and demographic structure.
Limitations existed in this study. Meteorological and pollution data came from monitoring stations, not individual exposure data, which might have contributed to exposure inaccuracies. Our meteorological and PM2.5 data were outdoor data, and most individuals spent more time indoors in cold weather, ignoring indoor-outdoor temperature differences. Third, the study area was high-altitude, had a peculiar environment, low average temperatures, and a tiny population, limiting generalization.
5 Conclusion
We discovered that cold spells and PM2.5 increased particular disease deaths, especially in people who resided at an average altitude above 3,000 meters, males, the older adults, and those with lesser education. Cold spells could synergistically interact with PM2.5 to increase the risk of death. Increased cold spell intensity and duration reduced the independent and interaction effects of cold spells and PM2.5 on several causes of death. Our study shows that reducing cold spells and PM2.5 in high-altitude areas can safeguard public health, with major public health consequences.
Data availability statement
The data analyzed in this study is subject to the following licenses/restrictions: the mortality data in this paper cannot be published as they contain a great deal of personal information about the deceased and their families. However, they can be obtained from the corresponding author on reasonable request. Requests to access these datasets should be directed to SH, hehe3991391@126.com.
Ethics statement
The research protocol for this study has been approved by the Ethics Review Committee of the Xining Center for Disease Control and Prevention in Qinghai Province, China (Approval No.: qhxncdcllsc-2024004). All procedures were conducted in accordance with relevant guidelines and regulations. Daily death data were summarized at the city level, were retrospective, and were low-risk studies. All personal information involved in this article was conducted under the supervision of the relevant researchers at the Xining Municipal Center for Disease Control and Prevention, and only secondary aggregated data were used in the analysis, which did not involve participants’ names, identifying information, telephone numbers, or residential addresses; therefore, the Ethics Review Committee of the Xining Center for Disease Control and Prevention waived written informed consent.
Author contributions
ZN: Conceptualization, Formal analysis, Investigation, Methodology, Software, Visualization, Writing – original draft. SH: Conceptualization, Funding acquisition, Resources, Supervision, Writing – review & editing. QL: Writing – original draft. HM: Data curation, Investigation, Writing – original draft. CM: Resources, Supervision, Writing – review & editing. JW: Resources, Supervision, Writing – review & editing. YM: Data curation, Investigation, Writing – review & editing. YZ: Data curation, Investigation, Writing – review & editing.
Funding
The author(s) declare financial support was received for the research, authorship, and/or publication of this article. This study was supported by a grant from the Science and Technology Project of Xining (2022-M-29), but there was no financial support.
Acknowledgments
We appreciate the support and guidance from Yu Wang at the Chinese Center for Disease Control and Prevention (China CDC).
Conflict of interest
The authors declare that the research was conducted in the absence of any commercial or financial relationships that could be construed as a potential conflict of interest.
Publisher’s note
All claims expressed in this article are solely those of the authors and do not necessarily represent those of their affiliated organizations, or those of the publisher, the editors and the reviewers. Any product that may be evaluated in this article, or claim that may be made by its manufacturer, is not guaranteed or endorsed by the publisher.
Supplementary material
The Supplementary material for this article can be found online at: https://www.frontiersin.org/articles/10.3389/fpubh.2024.1414945/full#supplementary-material
Footnotes
References
1. Newman, R, and Noy, I. The global costs of extreme weather that are attributable to climate change. Nat Commun. (2023) 14:6103. doi: 10.1038/s41467-023-41888-1
2. Alahmad, B, Khraishah, H, Althalji, K, Borchert, W, al-Mulla, F, and Koutrakis, P. Connections between air pollution, climate change, and cardiovascular health. Can J Cardiol. (2023) 39:1182–90. doi: 10.1016/j.cjca.2023.03.025
3. Michetti, M, Gualtieri, M, Anav, A, Adani, M, Benassi, B, Dalmastri, C, et al. Climate change and air pollution: translating their interplay into present and future mortality risk for Rome and Milan municipalities. Sci Total Environ. (2022) 830:154680. doi: 10.1016/j.scitotenv.2022.154680
4. Gasparrini, A, Guo, Y, Hashizume, M, Lavigne, E, Zanobetti, A, Schwartz, J, et al. Mortality risk attributable to high and low ambient temperature: a multicountry observational study. Lancet. (2015) 386:369–75. doi: 10.1016/s0140-6736(14)62114-0
5. Stafoggia, M, Michelozzi, P, Schneider, A, Armstrong, B, Scortichini, M, Rai, M, et al. Joint effect of heat and air pollution on mortality in 620 cities of 36 countries. Environ Int. (2023) 181:108258. doi: 10.1016/j.envint.2023.108258
6. Aslam, MY, Mukherjee, S, Kumar, VA, Patil, RD, Patil, SS, Dudhambe, SD, et al. Seasonal characteristics of boundary layer over a high-altitude rural site in Western India: implications on dispersal of particulate matter. Environ Sci Pollut Res Int. (2021) 28:35266–77. doi: 10.1007/s11356-021-13163-7
7. Jia, Z, Chen, Q, Na, H, Yang, Y, and Zhao, J. Impacts of industrial agglomeration on industrial pollutant emissions: evidence found in the Lanzhou-Xining urban agglomeration in western China. Front Public Health. (2022) 10:1109139. doi: 10.3389/fpubh.2022.1109139
8. Piovani, D, Brunetta, E, and Bonovas, S. UV radiation and air pollution as drivers of major autoimmune conditions. Environ Res. (2023) 224:115449. doi: 10.1016/j.envres.2023.115449
9. Ha, H . Geographic variation in mentally unhealthy days: air pollution and altitude perspectives. High Alt Med Biol. (2017) 18:258–66. doi: 10.1089/ham.2016.0137
10. Xing, Z, Yang, T, Shi, S, Meng, X, Chen, R, Long, H, et al. Ambient particulate matter associates with asthma in high altitude region: a population-based study. World Allergy Organ J. (2023) 16:100774. doi: 10.1016/j.waojou.2023.100774
11. Yang, H, Yan, C, Li, M, Zhao, L, Long, Z, Fan, Y, et al. Short term effects of air pollutants on hospital admissions for respiratory diseases among children: a multi-city time-series study in China. Int J Hyg Environ Health. (2021) 231:113638. doi: 10.1016/j.ijheh.2020.113638
12. Pratali, L, Marinoni, A, Cogo, A, Ujka, K, Gilardoni, S, Bernardi, E, et al. Indoor air pollution exposure effects on lung and cardiovascular health in the high Himalayas, Nepal: an observational study. Eur J Intern Med. (2019) 61:81–7. doi: 10.1016/j.ejim.2018.10.023
13. Rosati, JA, Yoneda, KY, Yasmeen, S, Wood, S, and Eldridge, MW. Respiratory health and indoor air pollution at high elevation. Arch Environ Occup Health. (2005) 60:96–105. doi: 10.3200/aeoh.60.2.96-105
14. Chun, H, Yue, Y, Wang, Y, Dawa, Z, Zhen, P, la, Q, et al. High prevalence of congenital heart disease at high altitudes in Tibet. Eur J Prev Cardiol. (2019) 26:756–9. doi: 10.1177/2047487318812502
15. Richalet, JP . Altitude and the cardiovascular system. Presse Med. (2012) 41:638–43. doi: 10.1016/j.lpm.2012.02.003
16. Rimoldi, SF, Sartori, C, Seiler, C, Delacrétaz, E, Mattle, HP, Scherrer, U, et al. High-altitude exposure in patients with cardiovascular disease: risk assessment and practical recommendations. Prog Cardiovasc Dis. (2010) 52:512–24. doi: 10.1016/j.pcad.2010.03.005
17. Seys, SF, Daenen, M, Dilissen, E, van Thienen, R, Bullens, DMA, Hespel, P, et al. Effects of high altitude and cold air exposure on airway inflammation in patients with asthma. Thorax. (2013) 68:906–13. doi: 10.1136/thoraxjnl-2013-203280
18. Cheng, C, Liu, Y, Han, C, Fang, Q, Cui, F, and Li, X. Effects of extreme temperature events on deaths and its interaction with air pollution. Sci Total Environ. (2024) 915:170212. doi: 10.1016/j.scitotenv.2024.170212
19. Zhou, L, Wang, Y, Wang, Q, Ding, Z, Jin, H, Zhang, T, et al. The interactive effects of extreme temperatures and PM(2.5) pollution on mortalities in Jiangsu Province, China. Sci Rep. (2023) 13:9479. doi: 10.1038/s41598-023-36635-x
20. Levine, BD, Stray-Gundersen, J, and Mehta, RD. Effect of altitude on football performance. Scand J Med Sci Sports. (2008) 18:76–84. doi: 10.1111/j.1600-0838.2008.00835.x
21. Bärtsch, P, Dvorak, J, and Saltin, B. Football at high altitude. Scand J Med Sci Sports. (2008) 18:iii–v. doi: 10.1111/j.1600-0838.2008.00826.x
22. Chen, J, Yang, J, Zhou, M, Yin, P, Wang, B, Liu, J, et al. Cold spell and mortality in 31 Chinese capital cities: definitions, vulnerability and implications. Environ Int. (2019) 128:271–8. doi: 10.1016/j.envint.2019.04.049
23. Feng, J, Cao, D, Zheng, D, Qian, Z(M), Huang, C, Shen, H, et al. Cold spells linked with respiratory disease hospitalization, length of hospital stay, and hospital expenses: exploring cumulative and harvesting effects. Sci Total Environ. (2023) 863:160726. doi: 10.1016/j.scitotenv.2022.160726
24. Rahman, MM, McConnell, R, Schlaerth, H, Ko, J, Silva, S, Lurmann, FW, et al. The effects of Coexposure to extremes of heat and particulate air pollution on mortality in California: implications for climate change. Am J Respir Crit Care Med. (2022) 206:1117–27. doi: 10.1164/rccm.202204-0657OC
25. Tobías, A, Madaniyazi, L, Gasparrini, A, and Armstrong, B. High summer temperatures and heat stroke mortality in Spain. Epidemiology. (2023) 34:892–6. doi: 10.1097/ede.0000000000001661
26. Lei, J, Chen, R, Yin, P, Meng, X, Zhang, L, Liu, C, et al. Association between cold spells and mortality risk and burden: a Nationwide study in China. Environ Health Perspect. (2022) 130:027006. doi: 10.1289/EHP9284
27. Wang, C, Feng, L, and Chen, K. The impact of ambient particulate matter on hospital outpatient visits for respiratory and circulatory system disease in an urban Chinese population. Sci Total Environ. (2019) 666:672–9. doi: 10.1016/j.scitotenv.2019.02.256
28. Jiang, Y, Yi, S, Gao, C, Chen, Y, Chen, J, Fu, X, et al. Cold spells and the onset of acute myocardial infarction: a Nationwide case-crossover study in 323 Chinese cities. Environ Health Perspect. (2023) 131:87016. doi: 10.1289/ehp11841
29. Kan, H . World Health Organization air quality guidelines 2021: implication for air pollution control and climate goal in China. Chin Med J. (2022) 135:513–5. doi: 10.1097/cm9.0000000000002014
30. Organization, W.H. WHO global air quality guidelines: particulate matter (PM2. 5 and PM10), ozone, nitrogen dioxide, sulfur dioxide and carbon monoxide. Geneva: World Health Organization (2021).
31. Nie, Y, Lu, Y, Wang, C, Yang, Z, Sun, Y, Zhang, Y, et al. Effects and interaction of meteorological factors on pulmonary tuberculosis in Urumqi, China, 2013-2019. Front Public Health. (2022) 10:951578. doi: 10.3389/fpubh.2022.951578
32. Liu, Z, Wang, S, Zhang, Y, Xiang, J, Tong, MX, Gao, Q, et al. Effect of temperature and its interactions with relative humidity and rainfall on malaria in a temperate city Suzhou. China Environ Sci Pollution Res. (2021) 28:16830–42. doi: 10.1007/s11356-020-12138-4
33. Knol, MJ, and VanderWeele, TJ. Recommendations for presenting analyses of effect modification and interaction. Int J Epidemiol. (2012) 41:514–20. doi: 10.1093/ije/dyr218
34. Gasparrini, A, and Leone, M. Attributable risk from distributed lag models. BMC Med Res Methodol. (2014) 14:55. doi: 10.1186/1471-2288-14-55
35. Altman, DG, and Bland, JM. Interaction revisited: the difference between two estimates. BMJ. (2003) 326:219. doi: 10.1136/bmj.326.7382.219
36. Xu, R, Sun, H, Zhong, Z, Zheng, Y, Liu, T, Li, Y, et al. Ozone, heat wave, and cardiovascular disease mortality: a population-based case-crossover study. Environ Sci Technol. (2024) 58:171–81. doi: 10.1021/acs.est.3c06889
37. Xu, R, Huang, S, Shi, C, Wang, R, Liu, T, Li, Y, et al. Extreme temperature events, fine particulate matter, and myocardial infarction mortality. Circulation. (2023) 148:312–23. doi: 10.1161/circulationaha.122.063504
38. Han, J, Liu, S, Zhang, J, Zhou, L, Fang, Q, Zhang, J, et al. The impact of temperature extremes on mortality: a time-series study in Jinan, China. BMJ Open. (2017) 7:e014741. doi: 10.1136/bmjopen-2016-014741
39. Song, H, Li, Y, Cheng, Y, Huang, Y, Zhang, R, Yao, X, et al. Effects of cold spells on mortality - Ningbo City, Zhejiang Province, China, 2014-2018. China CDC Wkly. (2022) 4:342–6. doi: 10.46234/ccdcw2022.079
40. Deng, C, Ding, Z, Li, L, Wang, Y, Guo, P, Yang, S, et al. Burden of non-accidental mortality attributable to ambient temperatures: a time series study in a high plateau area of Southwest China. BMJ Open. (2019) 9:e024708. doi: 10.1136/bmjopen-2018-024708
41. Ning, Z, He, S, Liao, X, Ma, C, and Wu, J. Health impacts of a cold wave and its economic loss assessment in China's high-altitude city, Xining. Arch Public Health. (2024) 82:52. doi: 10.1186/s13690-024-01284-7
42. Guoxia, B, Heng, S, Zhuoma, P, Yajie, L, Cangjue, G, Jianxiong, H, et al. Case-crossover study on association between temperature and non-accidental mortality in Tibet plateau, China. J Environ Occup Med. (2022) 39:261–7. doi: 10.11836/JEOM21462
43. Hu, X, Tao, J, Zheng, H, Ding, Z, Cheng, J, and Shen, T. Impact of cold spells on COPD mortality in Jiangsu Province. China Environ Sci Pollut Res Int. (2023) 30:6048–54. doi: 10.1007/s11356-022-22387-0
44. Chen, J, Dong, H, Yang, J, Li, L, Jin, J, Yang, Z, et al. The impact of cold spells on mortality from a wide spectrum of diseases in Guangzhou, China. Environ Res Lett. (2021) 16:015009. doi: 10.1088/1748-9326/abd26f
45. Meng, C, Ke, F, Xiao, Y, Huang, S, Duan, Y, Liu, G, et al. Effect of cold spells and their different definitions on mortality in Shenzhen. China Front Public Health. (2021) 9:817079. doi: 10.3389/fpubh.2021.817079
46. Sun, QH, Sun, Y, Yan, ML, Cao, J, and Li, TT. The north-south difference of the relationship between cold spells and mortality risk of cardiovascular diseases in China. Zhonghua Yu Fang Yi Xue Za Zhi. (2022) 56:1435–40. doi: 10.3760/cma.j.cn112150-20220504-00436
47. Ma, W, Wang, L, Lin, H, Liu, T, Zhang, Y, Rutherford, S, et al. The temperature-mortality relationship in China: an analysis from 66 Chinese communities. Environ Res. (2015) 137:72–7. doi: 10.1016/j.envres.2014.11.016
48. Masselot, P, Mistry, M, Vanoli, J, Schneider, R, Iungman, T, Garcia-Leon, D, et al. Excess mortality attributed to heat and cold: a health impact assessment study in 854 cities in Europe. Lancet Planet Health. (2023) 7:e271–81. doi: 10.1016/s2542-5196(23)00023-2
49. D’Amato, M, Molino, A, Calabrese, G, Cecchi, L, Annesi-Maesano, I, and D’Amato, G. The impact of cold on the respiratory tract and its consequences to respiratory health. Clin Transl Allergy. (2018) 8:20. doi: 10.1186/s13601-018-0208-9
50. Vardoulakis, S, Dimitroulopoulou, C, Thornes, J, Lai, KM, Taylor, J, Myers, I, et al. Impact of climate change on the domestic indoor environment and associated health risks in the UK. Environ Int. (2015) 85:299–313. doi: 10.1016/j.envint.2015.09.010
51. Kim, KN, Lim, YH, Bae, S, Kim, JH, Hwang, SS, Kim, MJ, et al. Associations between cold spells and hospital admission and mortality due to diabetes: a nationwide multi-region time-series study in Korea. Sci Total Environ. (2022) 838:156464. doi: 10.1016/j.scitotenv.2022.156464
52. Li, Y, Lan, L, Wang, Y, Yang, C, Tang, W, Cui, G, et al. Extremely cold and hot temperatures increase the risk of diabetes mortality in metropolitan areas of two Chinese cities. Environ Res. (2014) 134:91–7. doi: 10.1016/j.envres.2014.06.022
53. Keatinge, WR, Coleshaw, SR, Cotter, F, Mattock, M, Murphy, M, and Chelliah, R. Increases in platelet and red cell counts, blood viscosity, and arterial pressure during mild surface cooling: factors in mortality from coronary and cerebral thrombosis in winter. Br Med J (Clin Res Ed). (1984) 289:1405–8. doi: 10.1136/bmj.289.6456.1405
54. Madsen, C, and Nafstad, P. Associations between environmental exposure and blood pressure among participants in the Oslo health study (HUBRO). Eur J Epidemiol. (2006) 21:485–91. doi: 10.1007/s10654-006-9025-x
55. Cheng, X, and Su, H. Effects of climatic temperature stress on cardiovascular diseases. Eur J Intern Med. (2010) 21:164–7. doi: 10.1016/j.ejim.2010.03.001
56. Ikaheimo, TM, Lehtinen, T, Antikainen, R, Jokelainen, J, Nayha, S, Hassi, J, et al. Cold-related cardiorespiratory symptoms among subjects with and without hypertension: the national FINRISK study 2002. Eur J Pub Health. (2014) 24:237–43. doi: 10.1093/eurpub/ckt078
57. Larsson, K, Tornling, G, Gavhed, D, Muller-Suur, C, and Palmberg, L. Inhalation of cold air increases the number of inflammatory cells in the lungs in healthy subjects. Eur Respir J. (1998) 12:825–30. doi: 10.1183/09031936.98.12040825
58. Koskela, HO . Cold air-provoked respiratory symptoms: the mechanisms and management. Int J Circumpolar Health. (2007) 66:91–100. doi: 10.3402/ijch.v66i2.18237
59. Hyrkäs, H, Jaakkola, MS, Ikäheimo, TM, Hugg, TT, and Jaakkola, JJK. Asthma and allergic rhinitis increase respiratory symptoms in cold weather among young adults. Respir Med. (2014) 108:63–70. doi: 10.1016/j.rmed.2013.10.019
60. Eccles, R . An explanation for the seasonality of acute upper respiratory tract viral infections. Acta Otolaryngol. (2002) 122:183–91. doi: 10.1080/00016480252814207
61. Janský, L, Pospíšilová, D, Honzová, S, Uličný, B, Šrámek, P, Zeman, V, et al. Immune system of cold-exposed and cold-adapted humans. Eur J Appl Physiol Occup Physiol. (1996) 72-72:445–50. doi: 10.1007/bf00242274
62. Mourtzoukou, EG, and Falagas, ME. Exposure to cold and respiratory tract infections. Int J Tuberc Lung Dis. (2007) 11:938–43.
63. Brunekreef, B, Strak, M, Chen, J, Andersen, ZJ, Atkinson, R, Bauwelinck, M, et al. Mortality and morbidity effects of Long-term exposure to low-level PM(2.5), BC, NO(2), and O(3): an analysis of European cohorts in the ELAPSE project. Res Rep Health Eff Inst. (2021) 2021:1–127.
64. Gutiérrez-Avila, I, Riojas-Rodríguez, H, Colicino, E, Rush, J, Tamayo-Ortiz, M, Borja-Aburto, VH, et al. Short-term exposure to PM(2.5) and 1.5 million deaths: a time-stratified case-crossover analysis in the Mexico City metropolitan area. Environ Health. (2023) 22:70. doi: 10.1186/s12940-023-01024-4
65. Li, N, Hao, M, Phalen, RF, Hinds, WC, and Nel, AE. Particulate air pollutants and asthma. A paradigm for the role of oxidative stress in PM-induced adverse health effects. Clin Immunol. (2003) 109:250–65. doi: 10.1016/j.clim.2003.08.006
66. Liang, S, Sun, Q, du, Z, Ren, X, Xu, Q, Sun, Z, et al. PM(2.5) induce the defective efferocytosis and promote atherosclerosis via HIF-1α activation in macrophage. Nanotoxicology. (2022) 16:290–309. doi: 10.1080/17435390.2022.2083995
67. Li, J, Tang, W, Li, S, He, C, Dai, Y, Feng, S, et al. Ambient PM2.5 and its components associated with 10-year atherosclerotic cardiovascular disease risk in Chinese adults. Ecotoxicol Environ Saf. (2023) 263:115371. doi: 10.1016/j.ecoenv.2023.115371
68. Nel, AE, Diaz-Sanchez, D, and Li, N. The role of particulate pollutants in pulmonary inflammation and asthma: evidence for the involvement of organic chemicals and oxidative stress. Curr Opin Pulm Med. (2001) 7:20–6. doi: 10.1097/00063198-200101000-00004
69. Huang, Y, Wang, Y, Zhang, T, Wang, P, Huang, L, and Guo, Y. Exploring health effects under specific causes of mortality based on 90 definitions of PM(2.5) and cold spell combined exposure in Shanghai, China. Environ Sci Technol. (2023) 57:2423–34. doi: 10.1021/acs.est.2c06461
70. Tian, Q, Li, M, Montgomery, S, Fang, B, Wang, C, Xia, T, et al. Short-term associations of fine particulate matter and synoptic weather types with cardiovascular mortality: an ecological time-series study in Shanghai, China. Int J Environ Res Public Health. (2020) 17:1111. doi: 10.3390/ijerph17031111
72. Marugg, D . Lung problems in acute to subacute exposure to medium altitudes. Praxis. (1995) 84:1101–7.
73. Whayne, TF Jr . Altitude and cold weather: are they vascular risks? Curr Opin Cardiol. (2014) 29:396–402. doi: 10.1097/hco.0000000000000064
74. Jayaswal, R, Sivadas, P, and Mishra, SS. Health and performance of military personnel in cold climatic environment of the western HIMALAYAS. Med J Armed Forces India. (2001) 57:322–5. doi: 10.1016/s0377-1237(01)80014-9
75. Graham, TE . Thermal, metabolic, and cardiovascular changes in men and women during cold stress. Med Sci Sports Exerc. (1988) 20:S185–92. doi: 10.1249/00005768-198810001-00017
76. Korhonen, I . Blood pressure and heart rate responses in men exposed to arm and leg cold pressor tests and whole-body cold exposure. Int J Circumpolar Health. (2006) 65:178–84. doi: 10.3402/ijch.v65i2.18090
77. Hintsala, H, Kandelberg, A, Herzig, KH, Rintamaki, H, Mantysaari, M, Rantala, A, et al. Central aortic blood pressure of hypertensive men during short-term cold exposure. Am J Hypertens. (2014) 27:656–64. doi: 10.1093/ajh/hpt136
78. Jones, E, Sheng, J, Carlson, J, and Wang, S. Aging-induced fragility of the immune system. J Theor Biol. (2021) 510:110473. doi: 10.1016/j.jtbi.2020.110473
Keywords: cold spell, fine particulate matter, mortality, air pollution, climate change
Citation: Ning Z, He S, Liu Q, Ma H, Ma C, Wu J, Ma Y and Zhang Y (2024) Effects of the interaction between cold spells and fine particulate matter on mortality risk in Xining: a case-crossover study at high altitude. Front. Public Health. 12:1414945. doi: 10.3389/fpubh.2024.1414945
Edited by:
Yanlin Niu, Beijing Center for Disease Prevention and Control (Beijing CDC), ChinaReviewed by:
Mirmajid Farhadi, Consultant, Khorramabad, IranYujia Huang, Monash University, Australia
Copyright © 2024 Ning, He, Liu, Ma, Ma, Wu, Ma and Zhang. This is an open-access article distributed under the terms of the Creative Commons Attribution License (CC BY). The use, distribution or reproduction in other forums is permitted, provided the original author(s) and the copyright owner(s) are credited and that the original publication in this journal is cited, in accordance with accepted academic practice. No use, distribution or reproduction is permitted which does not comply with these terms.
*Correspondence: Shuzhen He, hehe3991391@126.com
†These authors have contributed equally to this work and share first authorship