Antimicrobial resistance interventions in the animal sector: scoping review
- 1Department of Comparative Biomedical Sciences, School of Veterinary Medicine, University of Surrey, Guildford, United Kingdom
- 2Department of Psychological Sciences, School of Psychology, University of Surrey, Guildford, United Kingdom
Animals are considered key contributors to the development and spread of antimicrobial resistance (AMR). However, little is known about the existing AMR interventions in the animal sector. This scoping review examines the existing evidence on AMR interventions aimed at livestock, animal health professionals (AHPs), and farmers, while reviewing their impact, limitations, gaps, and lessons for future use. The scoping review was conducted following guidelines from the PRISMA-ScR checklist. The databases, Web of Science, Scopus, PubMed, and international organisations’ websites (WHO, FAO, WOAH) were searched for articles reporting interventions targeting livestock, farmers, and AHPs. Interventions were categorised based on seven pre-defined primary measures including: change in antimicrobial use (AMU) practices; change in the uptake of antimicrobial stewardship (AMS); change in development of AMR; change in knowledge of appropriate AMU practices, AMR, and AMS; change in attitudes and perceptions concerning AMU, AMR, and AMS; and surveillance strategies. In total, ninety three sources were included: 66 studies, 20 reports, and 7 webpages. The reviewed interventions focused mostly on AMU practices (22/90), AMS uptake (8/90), and reduction of bacterial or resistant strains (30/90). Changes in knowledge (14/90) and attitude (1/90) were less frequently assessed and were often implicit. Most interventions were conducted within a select country (83/90) and 7/90 were at a global level. Only 19% (16/83) of interventions were implemented in low- and middle-income countries (LMICs) and most were at herd level with many self-reporting changes. Most of the interventions that focused on surveillance strategies (30/83) were implemented in high-income countries (62/83). Only one study investigated the financial implications of the intervention. The study findings provide an overview of existing AMR interventions and insights into the gaps which can be addressed to guide future interventions and research. A focus on developing, implementing and evaluating interventions in LMICs coupled with the use of objective outcome measures (e.g., measurable outcomes vs. self-reporting) will improve our understanding of the impact of interventions in these settings. Finally, assessing the financial benefits of interventions is necessary to inform feasibility and to encourage uptake of interventions aimed at reducing AMR in the animal health sector.
1 Introduction
Antimicrobial resistance (AMR) is a critical issue for both human and animal health (O’Neill, 2016). Globally, an estimated 1.27 million human deaths in 2019 were attributed to AMR (Murray et al., 2022). This is predicted to rise to 10 million in 2050 if no action is taken (O’Neill, 2016). AMR-attributed deaths in humans have been linked to the transfer of AMR- bacteria and AMR genes from animals to humans (Rhouma et al., 2022). The antimicrobials considered of high priority and essential for humans, Highest Priority Critically Important Antimicrobials (HPCIAs), are often used to treat resistant infections in animals (World Health Organization, 2018). The continued use of critically important antimicrobials (CIAs) in animals poses the risk of developing AMR, and onward transmission of CIA-resistant bacteria to humans which can reduce the effectiveness of the available CIAs (Tang et al., 2017). Other sources of AMR bacteria are community and hospital-acquired infections that can develop after misuse or overuse of antimicrobials in humans, lack of sanitation and diagnostics (e.g., lack of sensitivity testing and toilet/hand washing facility), and failure to use appropriate infection control measures in hospitals (World Health Organization, 2019). AMR infections can also be acquired from contaminated environments (World Health Organization, 2019; Stanton et al., 2022).
Despite success in reducing antimicrobial usage (AMU) in some countries (Lam et al., 2017; DANMAP, 2022), AMU is anticipated to rise around the world. Antimicrobial consumption in food-producing animals is projected to reach over 100,000 tonnes per annum by 2030, a 67% increase since 2015, with an estimated 99,502 tonnes used in 2020 (Van Boeckel et al., 2015; Tiseo et al., 2020; Mulchandaniid et al., 2023). In the United Kingdom alone, the use of CIAs on pig farms doubled from 2015 to 2019. The use of aminoglycosides, deemed critically important, rose from 2.607 to 5.957 mg per kilogram of body weight in pigs (Mahase, 2021). In the United States of America, 54% of antimicrobials used for livestock are CIAs. After a reduction in the use of CIAs by 27% from 2009 to 2017, this rose again by 8% from 5.6 million kilograms in 2017 to 6.0 million kilograms of antibiotic active ingredient in 2020 (US Food and Drug Administration Center for Veterinary Medicine, 2020).
The problem with AMR is that it knows no country boundaries, so reducing it globally is essential (Ruckert et al., 2020). At the global and country levels, there are varying efforts and interventions to preserve the repertoire of antimicrobials available for human use and to reduce the development and spread of AMR. The quadripartite, consisting of the World Health Organisation, Food, and Agriculture Organisation of the United Nations (FAO), World Organisation for Animal Health (WOAH), and United Nations Environment Programme (UNEP) calls for a reduction in antimicrobial usage (AMU) and AMR while enhancing antimicrobial stewardship (AMS), within the human medical and animal industry (FAO UNEP WHO and WOAH, 2022). This will mean enhanced research and understanding, One Health collaboration, and implementation of action plans to ensure best practices globally (FAO UNEP WHO and WOAH, 2022).
Working across sectors to reduce AMU and the development and transmission of AMR is essential to reduce the increasing burden and mortality attributed to AMR. Within human medicine, most AMR interventions are implemented in high income countries, with only, an estimated, 1 – 2% focusing on low- and middle-income countries (LMICs) (Cox et al., 2017). A similar trend likely occurs in the animal health sector. In high income countries such as The Netherlands and Denmark, interventions at the national levels have contributed to reductions in AMU in the animal health sector. For example, The Netherlands implemented a strategy aimed primarily at farmers based on the RESET mindset (rules and regulation, education, and information, social pressure, economics, and tools) to reduce AMU and the use of HPCIAs (Lam et al., 2017). This included obligatory aspects such as transitioning from the use of HPCIAs to less critical antimicrobials, having a registered herd veterinarian to discuss herd health with, and voluntary aspects such as lectures and study groups for farmers and animal health professionals (AHPs). This intervention resulted in a reduction in AMU of 47% between 2009 to 2015 (Lam et al., 2017). In Denmark, since 1995 DANMAP, the Danish Integrated Antimicrobial Resistance Monitoring and Research Programme, has monitored both AMU (grouped by antimicrobial class) by farmers, AHPs, and human medical professionals and AMR in both animals and humans. Coupled with other initiatives, DANMAP has led to an overall reduction in prescriptions and use of HPCIAs within all livestock sectors and eradication of HPCIA use within pig production. The monitoring of AMR of indicator bacterial isolates has shown a fluctuating trend for different bacteria. Full sensitivity to antimicrobials increased in E.coli isolated from broilers during 2014 – 2019, but the upward trend was not the same for pigs and cattle (DANMAP, 2022).
The burden of AMR is unevenly distributed across the globe. In 2019, high income countries had nearly 50% fewer deaths attributed to AMR (13.0 out of 100,000 deaths) compared to Africa which had the highest rate globally (23.7 out of 100,000 deaths), nearly 1.5 times higher than the global average (16.4 out of 100,000 deaths) (Murray et al., 2022). With a rising middle class and growing population in LMICs, there is increased demand to intensify food production which can lead to higher levels of AMU to sustain the high level of production (Manyi-Loh et al., 2018). The increased intensive farming in LMICs highlights the importance of identifying viable solutions to reduce AMU in livestock production.
There is limited data on existing interventions on AMR, AMS, and AMU in the animal health sector, particularly in LMICs. A few studies attempted to explore these aspects, but the scope was narrow. The aspects investigated in previous review studies included AMS in AHPs (Gozdzielewska et al., 2020), resistance genes within broiler production (Becker et al., 2021), and levels of transmission of AMR to humans after AMU in animals (Tang et al., 2017). An understanding of existing interventions focused on reducing AMU and AMR and increasing AMS within AHPs, farmers, and livestock, globally and in LMICs, is important. To address this gap, we have undertaken a scoping review with the intent of providing a broad overview and categorisation of interventions about AMR in the animal health sector within the last decade. The scoping review provides an overview map of existing evidence on AMR interventions in the animal health sector, and the related impact, current gaps, and limitations. Findings from the review can be used to inform and shape new interventions and to tailor future research on AMR interventions.
2 Methods
2.1 Study approach
A scoping review was conducted following the PRISMA-ScR checklist (Tricco et al., 2018). The focus of the review was AMR interventions in the animal health sector, specifically interventions aimed at reducing inappropriate AMU, increasing/enhancing uptake of AMS, and/or reducing the risk of development and spread of AMR. The groups of interest to which the AMR interventions were applied included AHPs (veterinarians and para-veterinarians), farmers, and livestock (poultry, cattle, goats, sheep, swine, and aquaculture).
2.2 Data sources
The databases PUBMED, Scopus, and Web of Science were searched (Appendix 1). The websites of the World Health Organization (WHO), Food and Agriculture Organization of the United Nations (FAO), and World Organisation for Animal Health (WOAH), were also searched. Backward citation tracking was performed on articles including reviews that were otherwise excluded.
2.3 Search strategy, inclusion, and exclusion criteria
A combination of words relating to Africa, America, Animal, Antibiotic, Antimicrobial, Asia, Australia, Bacteria, Environment, Europe, Farmer, Intervention, North, Para-veterinarian, South, Surveillance, Veterinarian, and Veterinary was used for the article search (Appendix 1).
No limits were set on study design, the language was set as English and only papers published from the 1st of January 2013 through 31st of December 2022 were included. Reviews were excluded but were accessed for citations. Studies focusing on interventions at various levels were included: global, continent, country, regional (area within a country), or small-scale (multiple or singular herds or farms). Studies were excluded if they solely focused on human, environmental, or human and environmental aspects. Single cross-sectional studies, focusing on opinions or current practices only, and reports with a focus on providing singular time point surveillance data with no intervention action were excluded.
2.4 Study screening
The article search was performed in January 2023. Titles and abstracts of identified records were screened against the above inclusion and exclusion criteria. Eligible records were exported to Mendeley and then screened by the author (AJ). Articles that met the inclusion and exclusion criteria were included in the review. References from the full-text searches of articles deemed relevant were also screened against the inclusion and exclusion criteria and included in the review if relevant.
2.5 Data extraction
For each article, the following data were extracted into an excel file: author, year of publication, country where activity was implemented, level of intervention (small scale, regional, national, continental, international), study design, study population (AHPs, farmers, or livestock), results relevant to the primary intervention outcome measures (Figure 1, Appendix 1), outcomes of the intervention, impact of the interventions, strengths, and limitations. Extraction was performed by one reviewer (AJ) for all eligible articles and a second reviewer (AE) evaluated a subset of 20% of the extracted articles.
2.6 Synthesis and reporting results
A key aim of this study was to characterise the reported AMR interventions in the animal health sector, which focused on either livestock or AHPs or farmers. For the purpose of this study, interventions were grouped into seven categories based on primary outcome measures: 1) change in AMU practices of animal health professionals (AHPs) and farmers, 2) change in the uptake of AMS by AHPs and farmers, 3) change in development and/or spread/distribution of AMR, 4) change in knowledge of appropriate AMU practices, AMR and AMS, 5) change in attitudes and perceptions concerning AMU, AMR, and AMS, 6) surveillance strategies (with a focus on animals and either both or one of the following: environment, and/or humans), and 7) Other. For each of the above seven categories, primary and secondary outcome measures were defined (Figure 1). Details of defined primary and secondary measures are provided in Appendix 2. For purposes of interpretation, the reported interventions were also categorised by level of the geographical area covered as follows: small-scale (e.g., (i.e., singular or multiple herds or farms), regional (region within a country), national (country-level), continental (across an entire continent), and international (across continents) interventions. Due to this review article being a scoping review, and the variety of study designs, a light touch study design assessment was performed.
2.7 Study design appraisal
For intervention studies, a light touch quality review of the study design and subjectivity of outcome measurements was performed within the research group (AJ & JO) by asking the questions: (1) what design was used for the intervention? and (2) were the outcome measurements subjective or objective? Interventions were evaluated on a six-point scale based on the above two questions. For question 1, a maximum of four points could be obtained for study design: use of randomisation with control group (4 points), control group with no randomisation (3 points), two time points at which the intervention was measured (pre and post intervention) and with no control group (2 points), description of intervention without use of pre-and post-intervention measurement and no control group (1 point). For question 2, a maximum of two points could be obtained: including objective outcome measurements (2 points) or only subjective outcome measurements (1 point). No points were obtained if outcomes were not included. Outcome measurements were considered objective if were directly measurable (e.g., AMU, AMR genes, bacterial strains) and considered subjective if there was potential bias in reporting by participants (e.g., self-reporting of change). The interventions were split into 3 categories, high, medium, and low quality, based on the combined points (maximum of six points): high = 5 or 6 points, medium = 3 or 4 points, or low = 1 or 2 points. Surveillance reports were not included in this intervention design appraisal.
3 Findings
The database search identified 10069 articles, including duplicates, for inclusion. After title and abstract screening, 59 articles were deemed to fall within the inclusion criteria (Figure 2). Among the 59 articles duplicates were checked for and none were found. Of the 59 articles, 57 were successfully retrieved and 2 that were not available from online databases were accessed through intra-library loans. Six of the 59 articles were reviews and were therefore excluded resulting in 53 eligible articles. Through citation search, 28 additional sources were found (13 articles, 10 reports, and 5 webpages). International Organisation websites were searched for interventions that fit within the scope of the primary outcome measures and 10 reports and 2 webpages were included. In total 93 sources were included – 66 articles, 20 reports, and 7 webpages (Figure 2).
The ninety-three studies and reports resulted in 90 interventions (66 articles, 20 reports, and 7 webpages. The distribution of primary outcome measures for interventions assessed in this study was broad (Figure 3), with some overlap between measures. The reported interventions focused mostly on surveillance strategies (30/90), change in development and/or spread of AMR (30/90), change in AMU practices (22/90), change in knowledge (14/90), and change in the uptake of AMS (8/90). Studies reporting change in attitude and perceptions were nearly non-existent (1/90). Six (6/90) sources were categorised as ‘Other’.
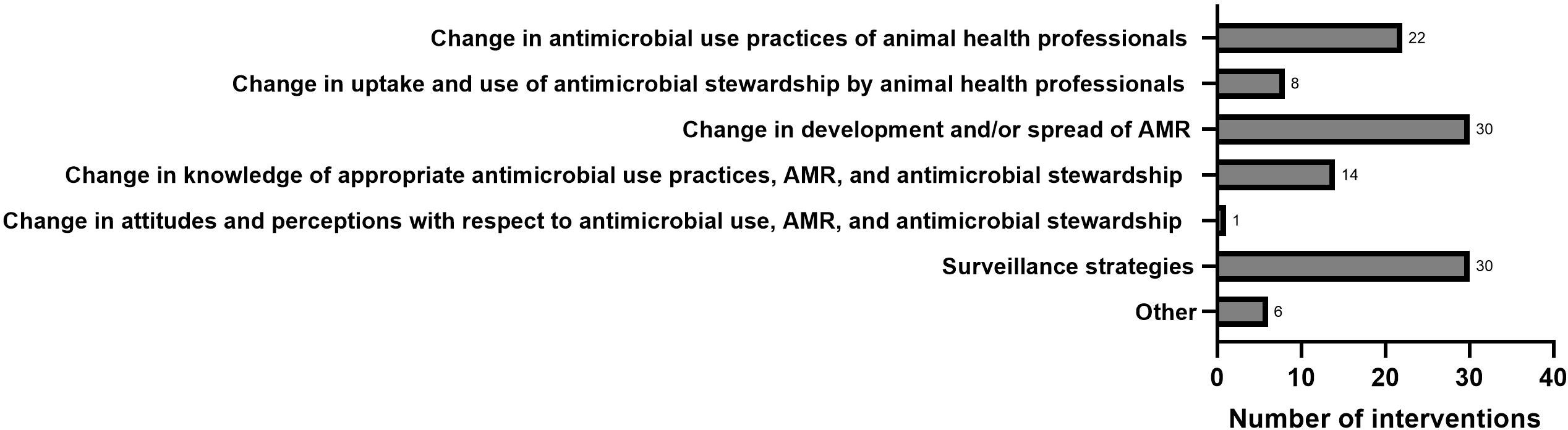
Figure 3 Interventions grouped by primary outcome measurements. Interventions are counted in more than one group if they incorporated more than one primary outcome measurement.
The interventions were implemented at various levels including small-scale (singular or multiple herds or farms), regional (region within a country), national (country-level), continental (across an entire continent), and international (across continents). Most interventions were implemented on a small scale (51/90) or national/country level (24/90), with fewer on an international (across continents) (7/90), continental (4/90), or regional level (area within a country) (4/90).
National interventions mostly took place in countries in Europe (14/24), North America (5/24), and Asia (5/24), whereas all continental interventions took place in Europe (4/4). Small-scale interventions were mostly implemented in countries in Europe (23/51), North America (14/51) and Asia (8/51). Of the country level studies, 16/83 were performed in LMICs (international studies are excluded from the denominator).
Intervention design appraisal was performed on 62/90 of the included studies. Of these, 25/62 were categorised as high quality, 32/62 were of medium, and 5/62 of low quality (Tables 1–7). The distribution of studies based on design quality in LMICs was high (2/13), medium (9/13), and low (2/13).
Throughout the key findings described in the sub-sections below, studies were highlighted as examples to illustrate the main themes of the outcomes. Further information on all the included studies can be found in Tables 1–7.
3.1 Change in AMU practices of AHPs and farmers
Change in AMU practices of AHPs was reported in 22 interventions across 24 studies (Table 1). The most frequent aspects measured were reduction of volume/weight of AMU at herd level (12/22), reduction in the volume of HPCIAs used (9/22), reduction of volume of AMU for a specific diagnosis (5/22), and reduction of volume of AMU for livestock animals at regional, national, or continental level (5/22). Nine of the twenty-two (9/22) interventions that assessed change in AMU practices were aimed at AHPs and 20/22 were aimed at farmers. Most of the interventions in this category were implemented in Europe (15/22), followed by North America (5/22) and Asia (2/22). The geographical coverage of the interventions varied from continental (1/22), national (3/22), and regional (1/22) to small scale (17/22). The quality of the intervention studies design was considered high (7/24) and medium (17/24).
The following subsections further describe the interventions in which change in AMU practices was assessed, and the related impacts, limitations, and gaps.
3.1.1 Reductions in AMU at herd level
Herd-specific interventions showed a reduction in AMU. These interventions focussed on implementation of farmers and AHP education, increased health and welfare care (e.g., stable climate, management), biosecurity (external and/or internal), and vaccine strategy (Collineau et al., 2017; Speksnijder et al., 2017; Raasch et al., 2020; Gerber et al., 2021; Gomez et al., 2021; Phu et al., 2021; Pempek et al., 2022; Schreuder et al., 2022). The targeted study groups included cattle farmers, swine farmers, broiler farmers, and AHPs (Collineau et al., 2017; Speksnijder et al., 2017; Raasch et al., 2020; Gerber et al., 2021; Gomez et al., 2021; Phu et al., 2021; Pempek et al., 2022; Schreuder et al., 2022).
As a first example of herd-specific interventions, Raasch et al. (2020) measured the reduction of AMU and HPCIAs among a swine farmer population in Belgium, Germany, France, and Sweden. A significant median reduction of AMU of 35% was reported. After the intervention was implemented, the duration for which pigs were treated reduced from 25% of their expected lifespan (200 days) to 16% (Raasch et al., 2020). The authors reported a compliance rate of 93% with the intervention plans by the target population. The strengths of this intervention were customised interventions for each herd and a broken-down assessment of AMU by diagnosis and age group. However, no control group was used on the basis that this group could change over the year (Raasch et al., 2020). This means it was not possible to adjust results for external factors that otherwise might be seen in a control group.
In a second example, reduction in AMU at the herd level was assessed for cattle farmers in Ohio, USA. A 50% reduction in AMU for calves was accomplished through didactic presentations, calf-side training, and veterinarian feedback for farmers. There was also an increased understanding of AMS and higher correct identification of cases in need of AMU (50%, 73/146) vs. the control group (14.3%, 9/63) (p=0.002) (Rojo-Gimeno et al., 2016; Pempek et al., 2022). This intervention allowed for an integrated approach looking at both AMU but also testing farmer knowledge and not relying on self-reporting. The observed weaknesses in that study were that the control and test groups were not randomly allocated, and both were presumed to have an increased interest in AMR, potentially biasing the outcomes. There was also no follow-up post-intervention measurement performed to evaluate if improvement was ongoing, and this was made difficult by veterinarians being reluctant to provide information on curative antimicrobials.
In the final example, Gerber et al. (2021) measured general AMU, diagnosis-specific usage, and HPCIAs usage amongst cattle farmers in Switzerland. Farmers picked the interventions to implement in their herds/farms from a pre-defined list of 17 udder, uterine, or calf health interventions. Udder or uterine health strategies resulted in a reduction in AMU (p < 0.04). Calf health interventions did not result in reduction in AMU. Allowing the farmers to choose herd-specific interventions from a pre-defined list allowed farmers to have partial autonomy. Observed weaknesses were the test and control groups were of different herd-sizes, breeds, and milk yields, which made comparison and interpretation of outcomes challenging. In addition, no information was collected on why the farmers chose their specific interventions (Gerber et al., 2021).
The financial benefit of AMU reduction was only explored in one intervention in two papers (Rojo-Gimeno et al., 2016; Postma et al., 2017). Increased net profit was recorded for a broad intervention that included herd management, biosecurity, and vaccination strategy customised to age groups of swine. At the same time, a decrease in treatment incidence of 52% and 32%, from birth to slaughter and for breeding animals, respectively, was reported.
3.1.2 Interventions reporting reduction in HPCIAs
Reduction in the use of HPCIAs (n = 9/22) was reported as part of broader interventions (Kuipers et al., 2016; Postma et al., 2017; Gerber et al., 2021; Morgans et al., 2021; Moura et al., 2022). Postma et al. (2017) noted a reduction of long-acting ceftiofur in sucklers by 83% and Gerber et al. (2021) reported a reduction in HPCIAs for treatment of udder related ailments (p = 0.05). However, reduction in use of HPCIAs was not always coupled with a general AMU reduction. An intervention targeting cattle-farmer-facilitated action groups assessed both general AMU and use of HPCIAs in cattle farmers in the United Kingdom and reported a reduction in use of HPCIAs of 3.484 mg/kg (p<0.001) but an overall median AMU reduction of 0.360 mg/kg (p = 0.719) (Morgans et al., 2021). In the same study, participant knowledge about AMR, AMS, and AMU at pre and post intervention was assessed qualitatively and an increase in the measured outcomes was reported. The noted study limitations were the lack of a control group and a societal push for AMR awareness at the time (Morgans et al., 2021).
3.1.3 Reduction of volume of AMU for a specific diagnosis
Five interventions (5/22) were implemented at the regional, national, or continental level and all five addressed a specific diagnosis (5/20) (Table 1). Four of the 5 interventions were at the regional, national, or continental level and measured the following: AMU in European farmers and AHPs (The European Parliament and The Council of the European Union, n.d)., reduction in ceftiofur use by AHPs and poultry farmers in Canada (Agunos et al., 2017; Agunos et al., 2018), AMU and HPCIAs reduction in AHPs and swine farmers in Denmark (Jensen et al., 2014; Dupont et al., 2017), and general AMU, AMR, diagnostic specific AMU reduction in dairy cattle farmers in the United Kingdom (Bradley et al., 2017; Breen et al., 2017), and HPCIAs reduction in dairy cattle farmers and AHPs in the Netherlands (Moura et al., 2022).
A fifth intervention, ‘Yellow Card System’, was at the national level and was aimed at reducing AMU and HPCIAs use in swine farmers and AHPs in Denmark (Jensen et al., 2014; Dupont et al., 2017). The intervention required swine farms to reduce their AMU to pre-set levels and resulted in 38.4 – 56.2% reduction of mg active ingredients/pig/day with increased use of vaccines, and decreased herd medication was reported as the biggest perceived influencing factor (Jensen et al., 2014; Dupont et al., 2017). However, these factors were only assessed in herds with > 10% reduction in AMU and self-reported by farmers and AHPs. This study included a large national sample size and excluded herds with < 10% reduction in AMU. Other national interventions overlapped with diagnostic-specific interventions. A national mastitis control scheme assessed AMU by AHPs and dairy cattle farmers in the United Kingdom (Bradley et al., 2017; Breen et al., 2017). This intervention resulted in a 40% reduction in use of intramammary medication in lactating cows and a 20% reduction in clinic mastitis rates, achieved through AHP and farmer training. However, this intervention was only noted in a letter and conference proceedings and no information was provided on strengths and limitations (Bradley et al., 2017; Breen et al., 2017).
3.1.4 Summary of change in AMU practices of AHPs and farmers
This category of interventions primarily focused on farmers and used herd-specific interventions to reduce AMU with success reported for both overall AMU reduction and reduction in use of HPCIAs. One study required farmers to select interventions from a pre-set list (Gerber et al., 2021). There was overlap of the primary outcomes measured across interventions and many studies also featured other primary outcome measures. Diagnosis-specific interventions were aimed at changing AMU in cases of mastitis and calf diarrhoea (Bradley et al., 2017; Gomez et al., 2017; Bailey et al., 2019). Studies involved pre- and post-intervention measurement of outcomes. Only a few studies reported use of control groups to account for other external influences (Kuipers et al., 2016; Becker et al., 2020).
3.2 Change in uptake and use of AMS by AHPs and farmers
Within this primary outcome, change in prescribing habits (n=4/8) was the most frequently measured, followed by increased adherence to guidelines (n=2/8) and increased use of diagnostics (n=2/8) (Table 2). There were additional aspects from ‘Other’ category of interventions reported within this primary outcome (n=6/8). Farmers (6/8) and AHPs (5/8) were almost equally targeted. Four interventions were implemented in Europe (4/8), two in Africa (2/8), and two in North America (2/8). The interventions were distributed across the levels: continental (1/8), national (1/8), regional (2/8), and small-scale (4/8). Of these interventions, two (2/8) were legislative interventions. The quality of intervention design ranged from high (1/7), medium (4/7), to low (2/7). The interventions, impact, outcome, and limitations are described in Table 2.
3.2.1 Change in prescribing habits
The studies reporting change in prescribing habits focussed on herd health plans and educational interventions. As an example, a study in Uganda used a One Health approach and focused on change in prescribing, guideline use, and diagnostic use in medical, healthcare, and AHPs (Musoke et al., 2020). Medical health professionals self-reported improved handwashing (57.3%), guideline use (52.9%), treatment based on diagnostics (44.1%), and reduction in unnecessary AMU (51.3%). Participation of AHPs was low compared to medical health professionals (Musoke et al., 2020). A disadvantage of self-reporting is perception may not translate to actual action; just because the participant says they are doing something, it does not mean they are. The other interventions surrounding prescribing habits, including the intervention of prescribing champions and herd health plans, are discussed in other sections (Raasch et al., 2020; Rees et al., 2021; Pempek et al., 2022).
3.2.2 Change of AMS through legislation
Change in AMS through legislation was reported. Two examples are the ‘California State Bill 27’ aimed at farmers in California, USA (Abdelfattah et al., 2022), and Regulation (EU) 2019/6 on veterinary medicinal products and repealing Directive 2001/82/EC aimed at both farmers and AHPs in Europe (The European Parliament and The Council of the European Union, n.d). The ‘California State Bill 27’ states that usage of antimicrobials of medical importance for humans for livestock requires a prescription. Assessment of this intervention indicated self-reported change in disease management including increased use of diagnostics (29.4%) and an increased use of alternatives to antimicrobials (26.8%) (Abdelfattah et al., 2022). This study was limited by a low response rate and possible response bias. As mentioned previously, self-reporting change may not translate to action. There was no report on AMU suggesting it was not evident whether self-reported change resulted in action (Abdelfattah et al., 2022). The latter, EU regulation, bans medication through feed or to groups for livestock use (The European Parliament and The Council of the European Union, n.d.). No data was presented within the legislation about the effect of this legislation (The European Parliament and The Council of the European Union, n.d.). In general, there were few studies evaluating legislation/bills.
3.2.3 Other reported aspects
Other aspects were reported under the primary outcome measure, change in uptake and use of AMS by AHPs and farmers. The first aspect was improving sanitation (i.e., improving hand washing and biosecurity) in both AHPs in Uganda and California (Musoke et al., 2020; Abdelfattah et al., 2022). The second aspect was improving dosage accuracy in cattle farmers in Ohio, USA (Pempek et al., 2022). These interventions were described in earlier sections and under the primary outcome measure ‘change in AMU of AHPs.’
3.2.4 Summary of change in uptake and use of AMS by AHPs and farmers
The interventions reported under this category illustrated how both voluntary programmes and legislation can create an impact on AMS. However, it is important to note the impact of many of these interventions were self-reported (Musoke et al., 2020; Abdelfattah et al., 2022). This carries the risk of response bias. Social and moral responsibility perceived by the reporting individuals may therefore influence the responses (Bradburn et al., 2004).
3.3 Change in development and/or spread of AMR
Change in development and/or spread of AMR was reported. The most frequent aspect measured was the reduced frequency of resistance genes within detected strains (21/30) followed by reduced frequency of bacterial strains (9/30), reduced frequency of resistance genes detected/isolated in food products (meat, milk, egg, etc) (5/30), and reduced frequency of resistance genes detected/isolated within the herd environment (3/30) (Table 3). The interventions were conducted in Europe (12/30), North America (10/30), Asia (4/30), South America (3/30), and Africa (1/30). The interventions were primarily small-scale (28/30) with two interventions conducted on a national level (2/30). The quality of study design was split between high (17/30) and medium (13/30) (Table 3).
3.3.1 Reduction in resistance strains
Reduced frequency of resistance genes within bacterial strains (19/26) was reported primarily at a small-scale level (17/19) and twice at a national level (2/19). Findings from the interventions at the national level are presented separately from the small-scale interventions.
3.3.1.1 National projects
Two interventions conducted at a national level focused primarily on ceftiofur resistance in broilers. The first intervention reported a voluntary reduction of ceftiofur and assessed the reduction of resistant strains in Japanese hatcheries (Hiki et al., 2015). Testing was performed on one faecal sample per farm with commercially available kits and to country standards. This longitudinal study did not have a control group or assess confounding but evaluated multiple resistance genes (Hiki et al., 2015). The second intervention, the Canadian Integrated Program for Antimicrobial Resistance Surveillance, tested for ceftiofur and other resistance genes in farms, abattoirs, and retail products (Agunos et al., 2017). All isolates were tested at national reference laboratories for continuity and to allow for comparison of results (Agunos et al., 2017). Neither intervention performed assessments of mortality or animal health. Both interventions used different reduction strategies and testing methods, but both reported reduced ceftiofur resistance in broiler production.
3.3.1.2 Small scale
Small-scale interventions were also reported and mainly focused on resistant strains in broilers (14/21), along with swine (7/21), cattle (5/21), goats (1/21), and a range of end meat products (1/21). The interventions assessed a range of parameters including biosecurity/sanitation (4/21) (Hao et al., 2013; Dorado-García et al., 2015a; Dorado-García et al., 2015b; Wanninger et al., 2016; Brusa et al., 2019), animals raised without antibiotics (6/21) (Pedroso et al., 2013; Wanninger et al., 2016; Thapaliya et al., 2017; Vikram et al., 2017; Haskell et al., 2018; Loayza-Villa et al., 2021), cessation of antimicrobials in feed (3/21) (Chinwe et al., 2014; Verrette et al., 2019; Shen et al., 2020) and competitive exposure (7/21) (Nuotio et al., 2013; Pedroso et al., 2013; Ceccarelli et al., 2017; Mourand et al., 2017; Methner et al., 2019; Dame-Korevaar et al., 2020a; Dame-Korevaar et al., 2020b). The distribution of the various interventions is summarised in Figure 4.
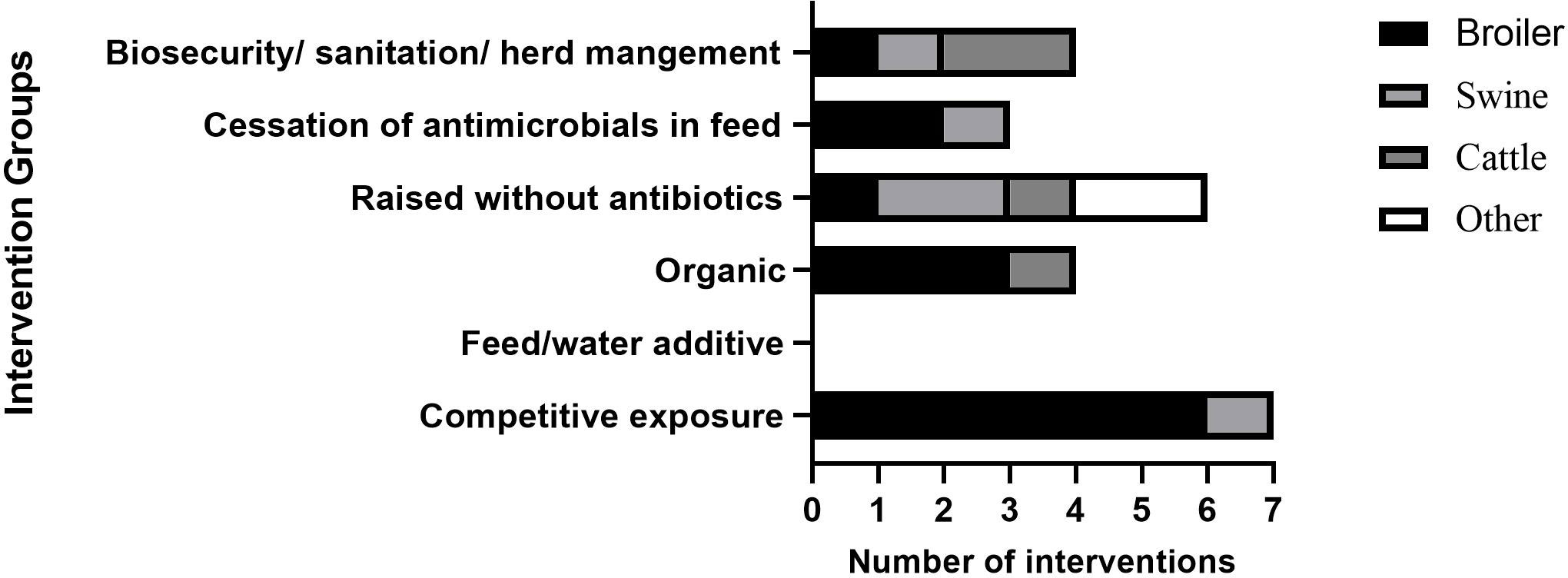
Figure 4 Distribution of interventions that assessed change in development of AMR with a focus on reducing AMR resistance genes. ‘Other’ in RWA accounts for intervention that includes broilers, swine, and cattle, and meat products.
Competitive exposure was one of the interventions used in broilers and included use of commercial products, pre-and probiotics, as well as specifically created bacterial compositions with positive effects (Nuotio et al., 2013; Pedroso et al., 2013; Ceccarelli et al., 2017; Mourand et al., 2017; Methner et al., 2019; Dame-Korevaar et al., 2020a; Dame-Korevaar et al., 2020b). Two specific examples of interventions involving competitive exposure were the use of unselected fermented intestinal bacterial and/or a selection of pre- and pro/biotics in broilers in the Netherlands (Dame-Korevaar et al., 2020a), and use of a commercial natural live intestinal flora, Aviguard, to target ESBL-E.coli in broilers in The Netherlands (Dame-Korevaar et al., 2020b). The former intervention had no effect when unselected fermented intestinal bacterial and a selection of pre- and pro/biotics were given on the same day (Day 0) as the challenge ESBL E.coli (Dame-Korevaar et al., 2020a). A reduced excretion of CTX-M-1- E.coli was seen when the challenge was given on day 5 after unselected fermented intestinal bacterial and a selection of pre- and pro/biotics. The study was limited by the short time frame (5 days) and experimental conditions. There is a gap in information on whether reduction in resistance is linked to disease or end meat contamination (Dame-Korevaar et al., 2020a).
In the latter study, Aviguard was administered to chicks right after hatching and challenged with CTX-M-1-E.coli on day 5 (Dame-Korevaar et al., 2020b). Of the test group, 0/200 broilers were CTX-M-1-E.coli positive on day 21 vs. the control with 187/200 positive. Multiple scenarios were tested and CTX-M-1-E.coli swabbing occurred every day. A potential limitation of this study was performance in semi-field conditions under stringent biosecurity means results may not translate to field conditions (Dame-Korevaar et al., 2020b). Like the previous report, disease and end meat levels were not assessed. This study suggested competitive exposure was successful within certain criteria such as high biosecurity and short time frames, but more knowledge is needed on the effect of longer timeframes and mechanism of human transmission.
A reduction in resistance levels following herd management and sanitation interventions in livestock was reported. A study in the Netherlands reported a reduction of 31 MRSA-positive herds to 29, and a 44% reduction in AMU, defined daily dosages animal per year, in swine (Dorado-García et al., 2015a). This was achieved by improving personnel and farm hygiene as well as changing animal contact structure. Having separate water pipes from medication pipes, specific rooms for deliveries, and designated sow groups, were all positively correlated with reducing MRSA. A limited intervention period (18 months) and pooling of samples may however lead to inaccuracies in measurement of outcomes (Dorado-García et al., 2015a).
3.3.2 Reduction in bacterial strains
The range of interventions focused on reducing bacterial strains (Figure 5) was similar to those for resistant strains (Figure 4). Broilers were the most frequently targeted animal group (5/9). Others were cattle (1/9) and goats (1/9). Unlike interventions focused on resistance genes, there was a larger emphasis on feed/water additives (4/9) (Hao et al., 2013; Rubio-García et al., 2015; Wanninger et al., 2016; Kannan et al., 2019), on cessation of antimicrobials (1/9) (Chinwe et al., 2014), and biosecurity/sanitation (3/9) (Bahrndorff et al., 2013; Luyckx et al., 2015; Lee et al., 2017; Kannan et al., 2019) (Figure 5).
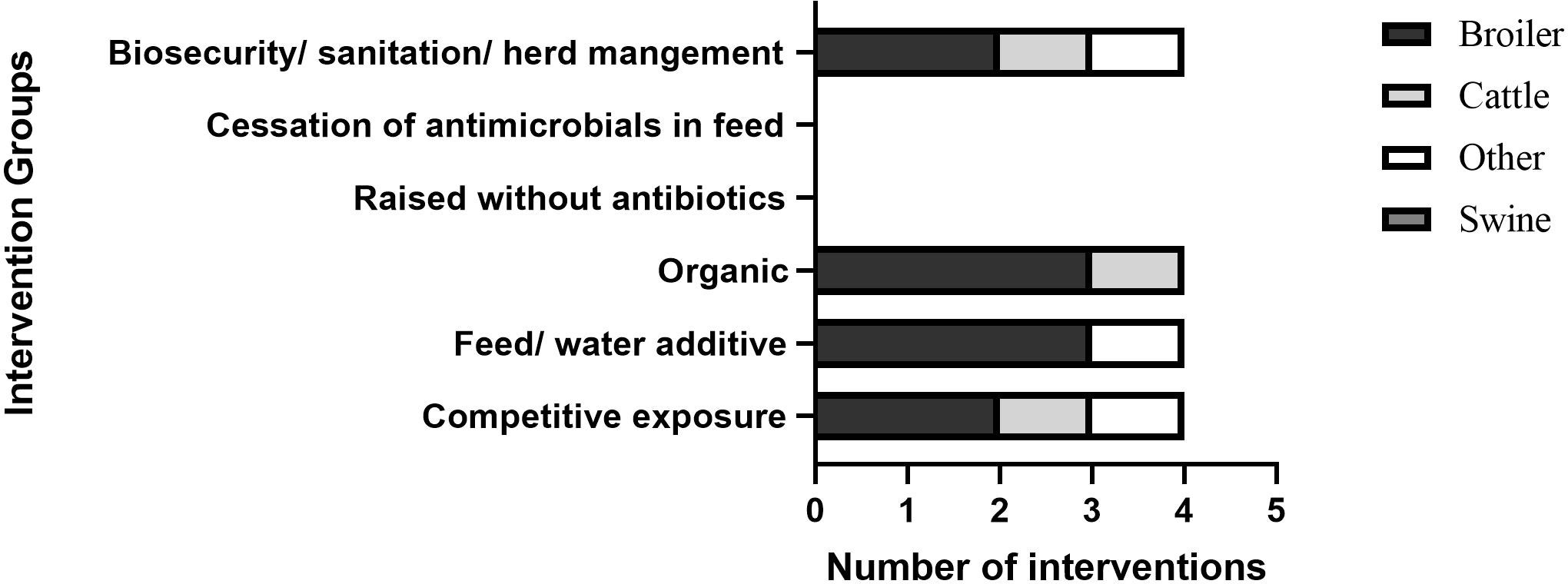
Figure 5 Distribution of interventions that assessed change in development of AMR with a focus on reducing bacterial strains. ‘Other’ in ‘biosecurity/sanitation/herd management’ and ‘feed/water additive’ is same intervention.
Two examples of feed and drink additives used in the interventions were dietary brown seaweed used to reduce rumen E.coli in goats (Kannan et al., 2019) and an allostatic modulator in drinking water and feed withdrawal from broilers to reduce coliforms (Rubio-García et al., 2015). The first intervention investigated microbiological contamination of goat carcasses in Georgia, USA (Kannan et al., 2019). To determine the effect of brown seaweed and chlorinated wash on microbiological contamination of carcasses, bucks were fed seaweed as a supplement and the feed was sprayed with 50 mg/L chlorinated water. Rumen but not skin E.coli count was reduced following feeding with seaweed (p <0.05). Skin count was reduced after chlorinated wash (p < 0.05) (Kannan et al., 2019). No information was provided on the contamination of meat in a production (abattoir) setting or transmission to humans. The second intervention aimed to reduce coliforms in broilers and end meat in Mexico (Rubio-García et al., 2015). Broilers were given an allostatic modulator in tap drinking water and a ten- or sixteen-hour feed withdrawal before slaughter shipment. The allostatic modulator contained electrolytes, acetylsalicylic acid, and ascorbic acid. Allostatic modulators aim to reduce allostasis (chronic stress). Reduction in coliforms (p = 0.014) and total aerobic mesophilic bacteria (p = 0.0001) were reported and the intervention was considered financially reasonable and accessible. A limitation of this study was it was performed under experimental conditions only (Rubio-García et al., 2015).
Two interventions on biosecurity and sanitation were reported and these included implementation of education and cleaning protocols. In the first intervention conducted in Belgium, the reduction of bacterial strains detected in broilers was assessed through on-farm cleaning protocols used by farmers (Luyckx et al., 2015). Sanitation of the broiler houses with commercial products containing sodium hydroxide resulted in 86% reduction in the number of E. coli-positive swabs (1-3% difference depending on soaking and water temperature) (Luyckx et al., 2015). The second intervention investigated the reduction in bacterial strains in fresh milk samples of cattle in Malaysia (Lee et al., 2017). The intervention was education of farmers on udder and machine sanitation and resulted in a 40% log reduction of Staphylococcus aureus in fresh milk samples (Lee et al., 2017). A limitation of this study was that statistical significance was not reported and a clear description of how the training was performed was not provided.
3.3.3 AMR in the environment and food
Articles reported on interventions focussed on the environment (n = 3) and food products (n = 4). Three studies focused on the environment and area around herds. The first study investigated bacterial strains in broilers and the environment in China (Hao et al., 2013). This intervention focused on sanitation, specifically the use of acidic water (pH 5.0 – 6.0) wash containing chlorine to reduce Salmonella spp. and E.coli in broiler houses and resulted in a 16% reduction in Salmonella spp and E.coli in broiler houses (Hao et al., 2013). A limitation of this study was the intervention was not applicable to bird housing with cages. The second intervention focused on both sanitation and reducing AMU and investigated resistant bacterial strains in veal cattle in the Netherlands (Dorado-García et al., 2015b). The intervention reported that cleaning and disinfecting negatively impacted the MRSA burden in the environment around veal cattle (Dorado-García et al., 2015b). This intervention was implemented for two production cycles with different techniques and under short time frames (12 weeks) making comparison of results difficult. A third study investigated bacterial strains on swine farms, in the surrounding farm environment, and in meat products in China (Shen et al., 2020). There were significant reductions in MCR-1-positive E. coli after the cessation of colistin as a feed additive. This was both at farm level (p < 0.0001), in food (pork) (p < 0.0001), and in the environment (soil and water around slaughterhouses) (p < 0.0001).
3.3.4 Summary of change in development and/or spread of AMR
The design of interventions under this category varied. Some interventions measured outcomes at pre and post intervention to assess change in outcomes, whereas other interventions used control herds. Experimental studies were used to evaluate outcomes within this category, more than for any other primary outcome measurement. Findings from experimental studies do not necessarily translate to or are feasible in field conditions. Replicating these findings in field conditions is an important next step to assess if the interventions work in the real-world situations. Some of the reported interventions run for a short time frame and no indication of disease level, transmission to humans, or end meat contamination was assessed (Dame-Korevaar et al., 2020a; Dame-Korevaar et al., 2020b; Shen et al., 2020).
3.4 Change in knowledge of appropriate AMU practices, AMR, and AMS
There were 14 reported interventions within the primary outcome, change in knowledge of appropriate AMU practices, AMR, and AMS. These included change in knowledge of appropriate AMU practices (n = 9), change in knowledge of AMR (n = 6), and change in knowledge of AMS (n = 6), with overlapping observed within the interventions (Figure 6). There were also interventions with aspects that did not fit within the predefined groupings (3/14). AHPs and farmers were targeted in 6/14 and 8/14 of the interventions. The interventions were conducted in high income countries in Europe (5/14) and North America (2/14) and less in LMICs within Africa (2/14), and Asia (3/14). Interventions featured across all levels; international (2/14), national (1/14), regional (2/14), and small scale (9/14) (Table 4). A description of interventions in high income countries and LMICs is provided below. The quality of design of these studies were scored as medium (7/12), low (4/12) and high (1/12).
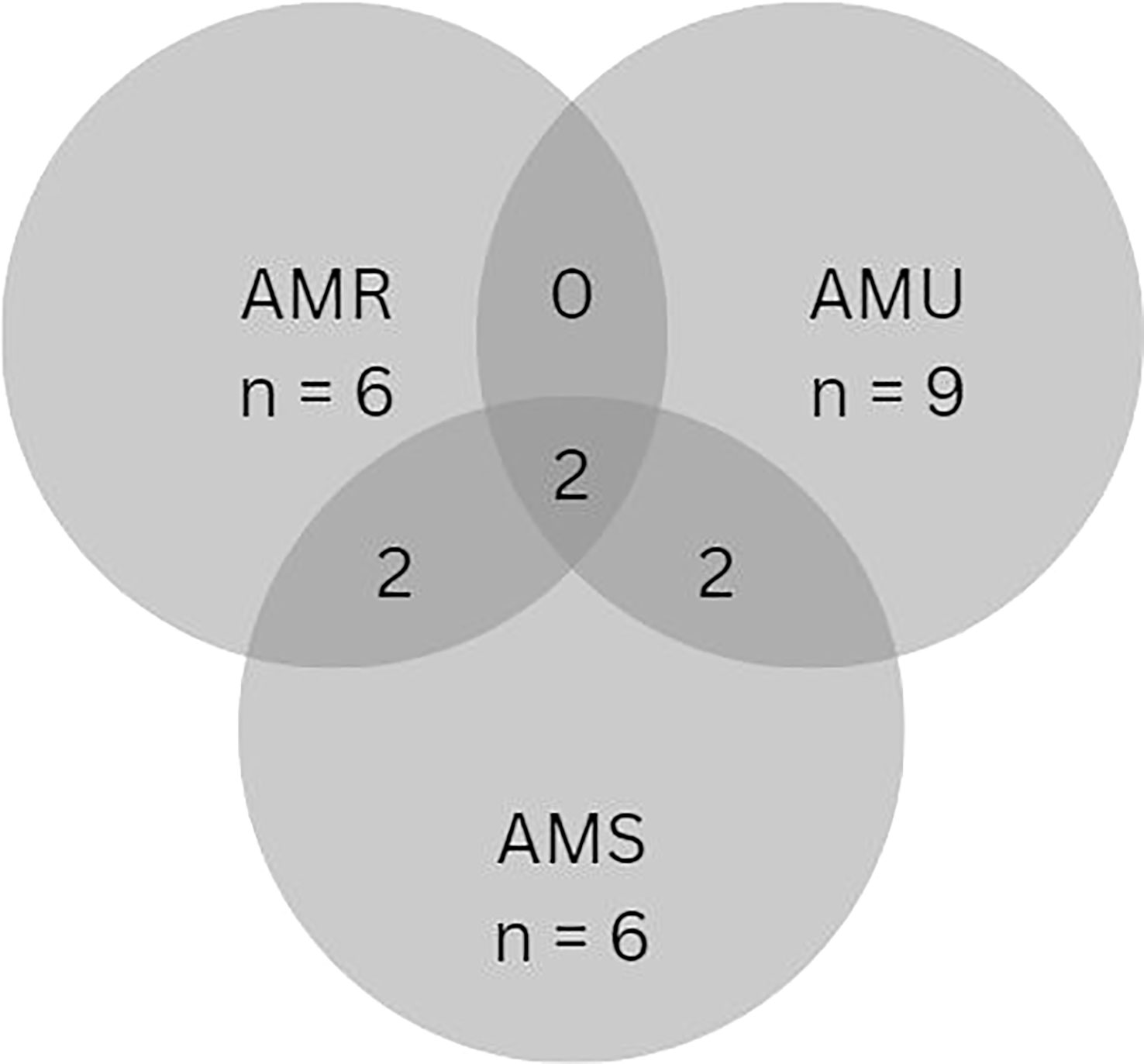
Figure 6 Distribution of interventions that assessed change in knowledge with a focus on AMU practices, AMR, and AMS within secondary outcome measurements.
3.4.1 Change in knowledge in high income countries
Interventions in this primary outcome measure were mostly in high income countries (Bradley et al., 2017; Powell et al., 2017; van Dijk et al., 2017; Feyes et al., 2021; Morgans et al., 2021; Rees et al., 2021) and overlapped with other primary outcomes. For example, the use of farmer-facilitated groups reduced AMU while increasing knowledge around AMU, AMR, and AMS for dairy farmers in the United Kingdom (Morgans et al., 2021). This intervention was considered time-consuming as required meetings every 6 – 8 weeks but allowed for conversation and discussion to create understanding (Morgans et al., 2021). In another study, the Arwain Vet Cymru Project created veterinary prescribing champions with the aim of changing the behaviour of AHPs in Wales and increasing knowledge of AMU and AMS through webinars, workshops, and discussions (Rees et al., 2021). The impact and limitations of the dissemination were not reported. This intervention was reported to be labour-intensive for the creators and participants (Rees et al., 2021). In another intervention, increased knowledge of farmers and reduction in AMU in calves in Ohio, USA was achieved through didactic presentations and calf-side training (Pempek et al., 2022). Limitations were not reported regarding knowledge acquisition, but other limitations were reported as noted earlier in the section on change in AMU practices of AHPs.
3.4.2 Change in knowledge in LMICs
Interventions focussed on knowledge acquisition were also conducted in LMICs (Roulette et al., 2017; Musoke et al., 2020; Shen et al., 2021; Sharma et al., 2022). While knowledge acquisition was part of a broad intervention in high income countries, this was conducted as a single activity in LMICs. Two interventions that focused on assessing knowledge about AMR were reported in LMICs. The first intervention assess knowledge on AMR and animal health among farmers and AHPs in India (Sharma et al., 2022). The target participants attended meetings and were given ‘knowledge packs’ on AMR and/or animal health, to raise AMR awareness. Higher knowledge scores were reported for farmers that participated in the meetings (p<0.05) and received information on animal health (p=0.03) or animal health and AMR (p=0.01). A key limitation of this study was it did not include translation of knowledge to actions or compare a pre-post intervention knowledge score (Sharma et al., 2022). In the second intervention, AMR was assessed after education on AMR was given to farmers in Tanzanian Masai communities (Roulette et al., 2017). Additionally, tape measures and antimicrobial dosage charts were given to men, and women received thermometers for milk pasteurization. At a 2-month follow-up, men retained more AMR knowledge (30%) compared to women (14%). However, 70% of women used their innovations correctly (thermometer) whereas men only performed 18% of dosage steps correctly (Roulette et al., 2017). A strength of this study was that cultural aspects and gender roles were taken into consideration. A limitation of this study was knowledge retention about AMR and innovation use were not evaluated as potential influences of each other. In general, there was limited information on interventions focussed on change in knowledge in LMICs and no demonstrated evidence of knowledge translating to action.
3.4.3 A summary of the change in knowledge of appropriate AMU practices, AMR, and AMS
The reported interventions illustrate that knowledge on AMR can be learned and retained (Roulette et al., 2017; Sharma et al., 2022) and assessed using pre- and post-intervention testing. However, there is need to gain more understanding of whether knowledge provided to farmers and AHPs translates to action and if there is sustainable change. The intervention conducted by Morgans et al. (2021) aimed to create sustained change through peer-to-peer learning and prescribing champions. However, there was no measurement of outcomes. Interventions evaluating outcome measurements are needed to understand the impact of these interventions.
3.5 Change in attitudes and perceptions to AMU, AMR, and AMS
Only one article investigated the change in attitudes and perceptions (n = 1) and did so as a small-scale qualitative assessment of farmers and AHPs in the United Kingdom (van Dijk et al., 2017) (Table 5). After participating in the creation of an AMU reduction policy, farmers and AHPs provided thoughts on their AMU practices and how these could be incorporated into their herd health plans. The responses were individual statements on an ad hoc basis (van Dijk et al., 2017). This study suggests that using stakeholders (such as AHPs and farmers) that will directly be impacted by policy to create policy could result in the stakeholders feeling more included and motivated potentially resulting in better policy outcomes.
3.6 Surveillance strategies
Interventions involving surveillance strategies (30/90) were primarily conducted on a continental (3/30) and national (23/30) level. Most of the surveillance strategies focused on both AMU and AMR (12/30), some focused solely on AMR (9/30), or AMU (4/30). Of those focussing on both AMU and AMR, some considered a One Health approach and provided human data (6/12). The largest number of surveillance strategies were reported in Europe (17/30) and less in Asia (5/30) and North America (5/30). No surveillance strategies were reported in Africa or South America. A detailed description of surveillance involving a One Health approach or focussed on AMU and AMR is provided below. Small-scale interventions (3/30) are presented separately.
3.6.1 One Health-focused strategies
Within national surveillance activities involving AMR and AMU, there was a focus on One Health. The most comprehensive surveillance strategies included AMR in zoonotic pathogens, indicator bacteria, and clinical isolates for both humans and animals along with antimicrobial sales in annual reports. These strategies were most reported in Europe and Asia. Half (6/12) of the reported strategies provided a comparison of human and veterinary isolates (JVARM, ; Federal Office of Public Health FOPH, 2015; MARAN, 2021; DANMAP, 2022; Korean Veterinary Antimicrobial Usage and Resistance Monitoring, 2022; Simonsen et al., 2022).
3.6.2 AMU-focused strategies
In other countries that did not report AMU and AMR together, surveillance strategies were split, or reported aspects on AMU or AMR or human and veterinary isolates, separately. Surveillance strategies focusing on AMU used medical sales data. As an example, on a continental level, the European Surveillance of Veterinary Antimicrobial Consumption (ESVAC) collates antimicrobial sales data from 31 European Union (EU) countries, offering an overview and accountability for usage (European Medicines Agency, 2022). These exist at country level in Europe (Danish Veterinary and Food Administration, n.d.; Finnish Food Authority, 2022).
3.6.3 AMR-focused strategies
Surveillance of AMR on its own exists in multiple forms, on both a national and continental level primarily in Europe and North America. On a continental level, the EU collates AMR data through The European Food Safety Authority (EFSA). Monitoring is conducted for zoonotic and indicator bacteria for AMR (incl. Salmonella spp., E.coli (Extended Spectrum Beta-Lactamase (ESBL)/AmpC beta-lactamases (AmpC)) with data from both human and livestock isolates from 27 EU member states (EFSA, 2022). Under creation is the European Antimicrobial Resistance Surveillance Network in veterinary medicine (EARS-Vet) which will register veterinary clinical isolates (Mader et al., 2021). Clinical isolates from livestock are currently not collected by many surveillance systems. The French surveillance network for antimicrobial resistance in bacteria from diseased animals (RESAPATH) in France offers the voluntary submission of 14 clinical isolates (Cazeau et al., 2022). Another large surveillance strategy exists in the US. Data on resistant isolates are collected through the Veterinary Laboratory Investigation and Response Network (Vet-LIRN) in partnership with The National Antimicrobial Resistance Monitoring System (NARMS) (U.S. Food and Drug Administration, 2022a; U.S. Food and Drug Administration, 2022b). NARMS monitors and publishes reports of AMR data from enteric isolates from retail meats, food animals, ill people, and companion animals.
3.6.4 Surveillance on a small scale
There were only three surveillance strategies that were considered small-scale. Two interventions were conducted in LMICs (Indonesia and Vietnam) (Yusuf et al., 2018; Ha et al., 2021) and one at a large veterinary teaching hospital in the USA (Ohio) (Feyes et al., 2021). Of the two studies in LMICs, the first study investigated AMU using sales data of veterinary antimicrobials from drug stores in Indonesia (Ha et al., 2021). An app was created for pharmacists to report sales data allowing them to monitor sales and making data available to monitor on a larger scale. The study acknowledges multiple limitations. Three weeks of sales data was extrapolated to 1 year and as such did not account for variations throughout the year. Furthermore, sales do not equate to usage, and no feed additives were accounted for (Ha et al., 2021). The second surveillance strategy measuring AMU was a self-reporting system for farmers in Vietnam (Yusuf et al., 2018). It involved reporting medical usage and disease via a tablet. This offered farmers a way to track their AMU, without a national system. The study followed farmers on the main island for 2 years, excluding rural settings (Yusuf et al., 2018). Two limitations were observed in this study; there was room for reporting inaccuracies and compliance levels were not reported.
3.6.5 Summary of surveillance strategies
Most surveillance strategies within the scope of this review were based in high income countries suggesting there is little data from LMICs – most likely due to the requirements for financial investment and infrastructure. Within the surveillance strategies that do exist, mandatory reporting at the national level appears widespread which helps ensure that isolates received reflect AMR distribution in each setting. However, reporting especially of clinical isolates is voluntary within systems (Cazeau et al., 2022) which risks a fractured picture of the clinical isolate presence and distribution. Another aspect of surveillance that operates with a margin of error is using sales data as a measure of AMU, as it does not account for off-label use and unused medication. Few surveillance interventions that are considered small-scale were reported, and with varying limitations.
3.7 Other interventions
The ‘other’ category of interventions (n = 6) included tools provided by the quadripartite to help countries with surveillance systems or self-assessment of their AMR situation (5/6) and one small-scale intervention that focused on policy related to reducing AMU by farmers and AHPs in the United Kingdom. Two examples of the tools from the quadripartite are the FAO Assessment Tool for Laboratories and AMR Surveillance Systems (FAO-ATLASS) (Food and Agriculture Organization of the United Nations, 2020) and WHO Tool for a Situation Analysis of AMR risks in the Food and Agriculture Sectors on a national level (Food and Agriculture Organisation of the United Nations, n.d).
Using the FAO Assessment Tool, either in surveillance mode or laboratory mode, a baseline level of the country’s setup can be assessed, steps for specific improvement identified, and progress made monitorable (Food and Agriculture Organization of the United Nations, 2020). There is limited information on the related impact or outcomes at national levels. The WHO Tool creates a national report on AMR risk and improvements aiming to provide a picture of the current situation and guide decisions based on One Health principles (Food and Agriculture Organisation of the United Nations, n.d). There may be a gap between receiving a report and actioning change. These tools do not have a primary effect on the target population but generally offer guidance to a country’s AMR plan.
4 Discussion
This scoping review summarises the existing evidence on interventions focussed on AMR, AMU and AMS in the animal health sector and provides insights into their impact, gaps, and limitations. Interventions targeting AHPs, farmers, and livestock were of interest. The review included 90 studies that reported interventions from around the world, with 19% of those in LMICs. Within the defined primary outcome measurements, there was a broad range of animal sector interventions. The reported interventions mainly focused on changing AMU levels and changing the development and spread of AMR. Within the primary outcome focused on reducing AMU, herd specific interventions with pre and post intervention measurements were common. Interventions aiming to reduce AMR were often experimental with few investigating the environment or end meat levels. There were few interventions focused on changing knowledge and/or attitudes and perceptions. Retention of knowledge and self-reported change was assessed in some of the reported interventions. Interventions involving surveillance were conducted at the national level and reported AMU determined from sales data and AMR based on detection of indicator bacteria.
Although interventions focusing on AMU were reported, it is important to note that a reduction in AMU does not automatically mean a reduction in AMR. Nonetheless, change in AMU is used as a measurement of impact. Evidence on the linkage between a reduction in AMU and AMR is mixed (Bennani et al., 2020). AMU is used, likely because it is more easily quantifiable and the data requires less resource to collect compared to that of resistant isolates (Bennani et al., 2020). The potential mismatch between AMU and AMR should be considered when assessing the impact of an intervention. Ideally, when assessing a reduction in AMU, the presence of AMR should also be measured.
None of the studies that investigated AMU practices assessed the duration of therapy in animals. Although there is a shortage of veterinary data, data in the human medicine sector indicates a change in therapy can have an impact on AMR without affecting treatment efficacy (Llor and Bjerrum, 2014; de Waele and Martin-Loeches, 2018; Spellberg and Rice, 2019). Furthermore, the lack of data on duration of therapy may indicate that a reduction in AMU could be due to fewer animals being treated, or the same number of animals being treated for a shorter period, unless number of animals is accounted for. This aspect requires closer examination in interventions assessing AMU practices.
Interventions assessing changes in development and/or spread of AMR were carried out in both field and experimental conditions in different livestock (cattle, swine, broilers, goats). Although experimental conditions can negate the limitations of field conditions, interventions garnering results in these conditions may not do so in field conditions. For interventions involving experimental conditions, it is important to account for these differences or to follow up the experimental studies with field studies to reflect real-world conditions.
In general, the interventions focussed on change in behaviour, knowledge, and attitude were few or lacking. Aspects on attitude, behaviour, and knowledge were often implicit parts of interventions but not outcome measurements. Interventions that investigated these aspects featured one or two time points or a set knowledge ‘bank’ without determining if the change in knowledge translated into actions or long-term change. Beyond assessing change in knowledge, it is important to investigate if increased knowledge translates to actions that reduce AMR.
In the current review, the animal health interventions in LMICs were scarce; only 19% (16/83) were performed in LMICs. This is a higher percentage compared to the estimate in human medicine of 1 – 2% (Cox et al., 2017). All the animal health related interventions in LMICs were on a small scale (at herd or farm level). The design of the interventions were mostly deemed of low quality, only one study was of high quality. The reasons for the low number of interventions in LMICs are unclear, but it is possible lack of resources is a contributing factor. The quadripartite and other key players at the global level are making efforts to lessen the gap in skills and resources between LMICs and high-income countries. For example, there are several stewardship tools and road maps that were developed and made available to LMICs to facilitate the implementation of AMR policy and interventions at a national level (World Health Organisation, 2016; Seale et al., 2017). Two examples of these are the Wellcome Trust Road Map for LMICs to participate in the global antimicrobial surveillance system (Seale et al., 2017) and the WHO manual for LMICs to implement national action plans to reduce AMR in both human and animal sectors (World Health Organisation, 2016). These tools need to be coupled with research and to focus on barriers while tailoring the AMR interventions to specific country socioeconomic needs and ensuring the output trickles down to farmers and AHPs.
Differences in the target population (farmers, AHPs, etc) and access to antimicrobials were observed in interventions performed in LMICs vs. high-income countries. Within the reviewed studies, more heterogeneity of the target population (farmers, AHP, etc) was found in LMICs. The farmers in high-income countries run mainly large farms. However, in LMICs, there were small-holdings or small-scale farmers (Phu et al., 2021) and communities that keep livestock for their own consumption (Roulette et al., 2017). In general, in high-income countries, the AHPs encompassed licensed and registered veterinarians but in LMICs, this also included unregistered practitioners prescribing antimicrobials. The access to antimicrobials varied across the targeted populations. Access to over-the-counter antimicrobials (Ha et al., 2021), which is not legal in most high-income countries, and through feed mills (Chinwe et al., 2014) exists in LMICs. This contrasts with high-income countries such as in the EU where access to over the counter is restricted and there is a ban on growth promotors (The European Parliament and The Council of the European Union, n.d.). These nuances in the target population and the access to antimicrobials make for a complex environment to promote AMS and to implement related interventions. The above findings suggest there is a need to tailor interventions aimed at restricting access to antimicrobials and promoting AMS to the local LMIC settings and to the targeted population and regulatory environment.
The AMR surveillance strategies were primarily at a national level and were reported mainly in high-income countries. AMR surveillance strategies often involve collection and testing of a range of isolates. The capacity of laboratories, along with infrastructure, technology and human resources can be a major limitation of surveillance activities at a national level. Increasing the capacity and capability of these aspects in LMICs may provide the opportunity for models applied in high-income countries to be used more globally (Fall et al., 2019; Jayatilleke, 2020). Understanding of the current resistance patterns in given settings could help focus and tailor resources to where they are most needed to reduce AMR and to guide the type of interventions needed to change AMR levels.
Cost, or perceived cost of testing and monitoring can serve as a barrier to efforts to tackle AMR. This may be more pronounced for farmers and AHPs who depend largely on livestock for their livelihood (Golding et al., 2019). Only one herd health intervention on Flemish pig farms reported financial related data with respect to interventions, specifically increased profit, and production parameters (Rojo-Gimeno et al., 2016; Postma et al., 2017). Most studies included in this review did not include financial calculations. This information is relevant for farmers, AHPs, and other key players, to assess if given interventions are financially feasible. Demonstrating that an intervention is of value or of benefit in terms of financial gain or improvement of other parameters (e.g., herd health, feed conversion), can facilitate evidence-based decision making and may encourage the uptake and implementation of interventions considered of value by AHPs and farmers. Ensuring financial and practical feasibility in real-world situations and reducing barriers to uptake within AHPs and farming communities are therefore useful targets to consider when exploring strategies to reduce AMR in the animal health sector.
The assessment of AMU in surveillance strategies was mainly performed using sales data. A limitation with use of sales data is that it does not directly translate to usage of antimicrobials. Medicine can be used for a different animal group than it was licensed and sold for. Wastage or unused medicine is not accounted for either. This means the actual usage could be vastly different from the calculated usage. Furthermore, milligram per millilitre (mg/mL) differs between antibiotics resulting in a different number of doses per mL. Some interventions focused on reducing AMU addressed this issue by using dose instead. However, there is no universal way of denoting dose amounts. The European Medicines Agency has defined daily dose based on active substance and administration route based on a mean. Other studies used other dose denominations. These do not account for discrepancies between different drugs and individual doses. Having a universal dose denomination for animal medicines would help make data comparable globally like it is in human medicine (World Health Organization, n.d).
The findings in the current study should be viewed with limitations in mind. General overview searches across multiple sources (databases and websites of organisation) were performed using defined search terms. Even with such a broad search, articles could be missed. To get an increased sensitivity, all the searches could be performed on a country basis. However, this is not feasible within a reasonable time frame considering all the countries at a global level. The quality of the data has not been evaluated in depth. A light touch review of study design has been performed to ensure some level of quality assessment. The quality of some of the literature is limited as some interventions were based on self-reported information. Self-assessment comes with a social desirability bias (Bradburn et al., 2004). For example, participants reporting that they have changed behaviour as the result of an intervention does not necessary mean that this is the case. People tend to over-report “good behaviour” (Bradburn et al., 2004). Understanding the gap between what is reportedly happening and what is happening in relation to change in AMR is critical for generating reliable outcome measurement in AMR interventions.
In addition to self-assessment and social desirability bias, volunteer bias can be a factor in intervention studies. This is especially plausible in those studies assessing knowledge and/or behaviour change with voluntary participation. Many of the studies addressed this limitation but did not correct for it. It is possible that smaller-scale studies with presumed volunteer bias may have had different outcomes than broader mandatory national/regional interventions (Salkind, 2010).
This scoping review only included interventions that reported change in measured outcomes whether successful or not. It is possible additional unsuccessful or even successful interventions were not being published and therefore not accessed or reviewed. There was a range of study designs and types, with some studies performed in field conditions while others were performed in environments created solely for the intervention. This illustrates the importance of understanding and interpreting intervention outcomes within different settings and contexts.
In conclusion, changes in AMU practices of AHPs and farmers and changes in the development and/or spread of AMR were the most frequent primary outcomes measured in the reviewed studies. Change in uptake and use of AMS, along with change in attitude and knowledge changes were measured less. Small-scale and national-level interventions were more common compared to continental or international interventions. Most interventions were performed in field conditions while some AMR interventions were conducted in experimental conditions. Only 19% of interventions took place in LMICs and were conducted primarily on a small scale. Analysis of the financial aspect of interventions was limited along with an understanding of compliance levels. Self-assessment to measure impact was commonly performed which increases the risk of volunteer bias.
Going forward, a focus on implementing and evaluating interventions in LMICs is warranted to ensure that this underrepresented group is included in the international conversation on AMR. Robust interventions that include objective outcome measures (e.g., measurable outcomes vs. self-reporting) both in LMICs and around the world can increase the understanding of the true impact of AMR interventions. Studies that investigate the benefits and financial implications of interventions are necessary to inform feasibility and the impact of interventions and to encourage uptake of AMR interventions by animal health professionals and farmers.
Author contributions
Conceptualisation, methodology, analysis, and editing: AJ and AE. Data collection, data extraction, synthesis, result reporting, and writing: AJ and AE. Supervision and reviewing: AE and JO. Methodological analysis of intervention studies: AJ and JO. All authors have read and agreed to the submitted version of the manuscript.
Funding
This work was funded through a postgraduate research studentship provided by the University of Surrey.
Acknowledgments
We thank the staff at the University of Surrey library for helping retrieve hard to find articles through intra-library loans.
Conflict of interest
The authors declare that the research was conducted in the absence of any commercial or financial relationships that could be construed as a potential conflict of interest.
Publisher’s note
All claims expressed in this article are solely those of the authors and do not necessarily represent those of their affiliated organizations, or those of the publisher, the editors and the reviewers. Any product that may be evaluated in this article, or claim that may be made by its manufacturer, is not guaranteed or endorsed by the publisher.
Supplementary material
The Supplementary Material for this article can be found online at: https://www.frontiersin.org/articles/10.3389/frabi.2023.1233698/full#supplementary-material
Abbreviations
AHP, Animal Health Professional; AmpC, AmpC Beta-lactamases; AMR, Antimicrobial Resistance; AMS, Antimicrobial Stewardship; AMU, Antimicrobial Use; CIA, Critically important antimicrobials; DANMAP, Danish Integrated Antimicrobial Resistance Monitoring and Research Programme; E. coli, Escherichia coli; EFSA, European Food Safety Authority; ESBL, Extended Spectrum Beta-Lactamase; ESVAC, European Surveillance of Veterinary Antimicrobial Consumption; EU, European Union; FAO, Food and Agriculture Organization; HPCIA, Highest Priority Critically Important Antimicrobials; LMICs, Low- and middle-income countries; NARMS, National Antimicrobial Resistance Monitoring SystemSulfa; TMP, Sulfadiazine and Trimethoprim; UNEP, United Nations Environment Programme; Vet-LIRN, Veterinary Laboratory Investigation and Response Network; WHO, World Health Organization; WOAH, World Organisation of Animal Health.
References
Abdelfattah E. M., Ekong P. S., Okello E., Williams D. R., Karle B. M., Lehenbauer T. W., et al. (2022). Factors associated with antimicrobial stewardship practices on california dairies: One year post senate bill 27. Antibiotics (Basel) 11 (2), p. 165. doi: 10.3390/ANTIBIOTICS11020165
Agunos A., Arsenault R. K., Avery B. P., Deckert A. E., Gow S. P., Janecko N., et al. (2018). Changes in antimicrobial resistance levels among Escherichia coli, Salmonella, and Campylobacter in Ontario broiler chickens between 2003 and 2015. Can. J. Veterinary Res. 82 (3), p.163–177.
Agunos A., Léger D. F., Carson C. A., Gow S. P., Bosman A., Irwin R. J., et al. (2017). Antimicrobial use surveillance in broiler chicken flocks in Canada 2013-2015. PloS One 12. doi: 10.1371/JOURNAL.PONE.0179384
AURES (2017). Resistance Report Austria AURES 2016 Summary (Vienna: Federal Ministry of Health and Women's Affairs). Available at: www.bmgf.gv.at.
Bahrndorff S., Rangstrup-Christensen L., Nordentoft S., Hald B. (2013). Foodborne disease prevention and broiler chickens with reduced campylobacter infection - volume 19, number 3—March 2013 - emerging infectious diseases journal - CDC. Emerg. Infect. Dis. 19, 425–430. doi: 10.3201/EID1903.111593
Bailey M. A., Taylor R. M., Brar J. S., Corkran S. C., Velásquez C., Novoa Rama E., et al. (2019). Prevalence and antimicrobial resistance of Campylobacterfrom antibiotic-free broilers during organic and conventional processing. Poult Sci. 98, 1447–1454. doi: 10.3382/PS/PEY486
Becker E., Projahn M., Burow E., Käsbohrer A. (2021). Are There Effective Intervention Measures in Broiler Production against the ESBL/AmpC Producer Escherichia coli? Pathogens 10 (5), 608. doi: 10.3390/PATHOGENS10050608
Becker J., Schüpbach-Regula G., Steiner A., Perreten V., Wüthrich D., Hausherr A., et al. (2020). Effects of the novel concept “outdoor veal calf” on antimicrobial use, mortality and weight gain in Switzerland. Prev. Vet. Med. 176, 104907. doi: 10.1016/J.PREVETMED.2020.104907
Bennani H., Mateus A., Mays N., Eastmure E., Stärk K. D. C., Häsler B. (2020). Overview of evidence of antimicrobial use and antimicrobial resistance in the food chain. Antibiotics 9 (2), 49. doi: 10.3390/ANTIBIOTICS9020049
Bradburn N., Sudman S., Wansink B. (2004). Asking questions: The definitive guide to questionnaire design–For market research, political polls, and social and health questionnaires San Francisco: Jossey-Bass.
Bradley A., Breen J., Leach K., Gibbons J., Armstrong D., Green M. (2017). AHDB dairy mastitis control plan. Vet. Rec 180, 154–155. doi: 10.1136/VR.J680
Breen J. E., Jones B., Jones B., Jones N., Jones M., Edwards R. (2017). “Implementation of the AHDB Dairy Mastitis Control Plan to reduce clinical mastitis rate and antibiotic use in a large dairy herd,” in 2017 British Mastitis Conference, Worcester, UK, 15 November 2017. 31–42.
Brusa V., Restovich V., Signorini M., Pugin D., Galli L., Díaz V. R., et al. (2019). Evaluation of intervention measures at different stages of the production chain in Argentinian exporting abattoirs. Food Sci. Technol. Int. (ANSES, Lyon et Ploufragan-Plouzané-Niort, France) 25, 491–496. doi: 10.1177/1082013219836326
Cazeau G., Collineau L., Haenni M., Jarrige N., Jouy E., Lupo A., et al. (2022). Resapath - French surveillance network for antimicrobial resistance in bacteria from diseased animals.
Ceccarelli D., van Essen-Zandbergen A., Smid B., Veldman K. T., Boender G. J., Fischer E. A. J., et al. (2017). Competitive exclusion reduces transmission and excretion of extended-spectrum-β-lactamase-producing Escherichia coli in broilers. Appl. Environ. Microbiol. 83 (11). doi: 10.1128/AEM.03439-16
Centers for Disease Control and Prevention (2015) National Antimicrobial Resistance Monitoring System for Enteric Bacteria (NARMS) 2015 Integrated Report. Available at: https://www.fda.gov/AnimalVeterinary/SafetyHealth/AntimicrobialResistance/NationalAntimicrobialRe (Accessed January 30, 2023).
Chinwe N., Thomas A., Ifeanyichukwu I., Chika E., Emmanuel N., Anthonia O., et al. (2014). Cloacal feacal carriage and occurrence of antibiotic resistant Escherichia coli in chicken grown with and without antibiotic supplemented feed. J. Veterinary Med. Anim. Health 6, 91–94. doi: 10.5897/JVMAH2013.0267
Cicconi-Hogan K. M., Belomestnykh N., Gamroth M., Ruegg P. L., Tikofsky L., Schukken Y. H. (2014). Short communication: Prevalence of methicillin resistance in coagulase-negative staphylococci and Staphylococcus aureus isolated from bulk milk on organic and conventional dairy farms in the United States. J. Dairy Sci. 97, 2959–2964. doi: 10.3168/JDS.2013-7523
Collineau L., Rojo-Gimeno C., Léger A., Backhans A., Loesken S., Nielsen E. O., et al. (2017). Herd-specific interventions to reduce antimicrobial usage in pig production without jeopardising technical and economic performance. Prev. Vet. Med. 144, 167–178. doi: 10.1016/J.PREVETMED.2017.05.023
Cox J. A., Vlieghe E., Mendelson M., Wertheim H., Ndegwa L., Villegas M. V., et al. (2017). Antibiotic stewardship in low- and middle-income countries: the same but different? Clin. Microbiol. Infection 23, 812–818. doi: 10.1016/J.CMI.2017.07.010
Dame-Korevaar A., Fischer E. A. J., van der Goot J., Velkers F., Ceccarelli D., Mevius D., et al. (2020a). Early life supply of competitive exclusion products reduces colonisation of extended spectrum beta-lactamase-producing Escherichia coli in broilers. Poult Sci. 99, 4052–4064. doi: 10.1016/J.PSJ.2020.04.025
Dame-Korevaar A., Kers J. G., van der Goot J., Velkers F. C., Ceccarelli D., Mevius D. J., et al. (2020b). Competitive exclusion prevents colonisation and compartmentalisation reduces transmission of ESBL-producing Escherichia coli in broilers. Front. Microbiol. 11. doi: 10.3389/FMICB.2020.566619
Danish Veterinary and Food Administration (n.d.) VetStat. Available at: https://vetstat.fvst.dk/vetstat/ (Accessed January 27, 2023).
DANMAP (2022) DANMAP 2021 - Use of antimicrobial agents and occurrence of antimicrobial resistance in bacteria from food animals, food and humans in Denmark (Copenhagen). Available at: https://www.danmap.org/reports/2021 (Accessed January 27, 2023).
de Waele J. J., Martin-Loeches I. (2018). Optimal duration of antibiotic treatment in Gram-negative infections. Curr. Opin. Infect. Dis. 31, 606–611. doi: 10.1097/QCO.0000000000000491
Dorado-García A., Dohmen W., Bos M. E. H., Verstappen K. M., Houben M., Wagenaar J. A., et al. (2015a). Dose-response relationship between antimicrobial drugs and livestock-associated MRSA in pig farming. Emerg. Infect. Dis. 21, 950. doi: 10.3201/EID2106.140706
Dorado-García A., Graveland H., Bos M. E. H., Verstappen K. M., Van Cleef B. A. G. L., Kluytmans J. A. J. W., et al. (2015b). Effects of reducing antimicrobial use and applying a cleaning and disinfection program in veal calf farming: Experiences from an intervention study to control livestock-associated MRSA. PloS One 10 (9), e0139536. doi: 10.1371/JOURNAL.PONE.0135826
Dupont N., Diness L. H., Fertner M., Kristensen C. S., Stege H. (2017). Antimicrobial reduction measures applied in Danish pig herds following the introduction of the “Yellow Card” antimicrobial scheme. Prev. Vet. Med. 138, 9–16. doi: 10.1016/J.PREVETMED.2016.12.019
EFSA (2022). The European Union Summary Report on Antimicrobial Resistance in zoonotic and indicator bacteria from humans, animals and food in 2019–2020. EFSA J. 20 (3), e07209. doi: 10.2903/J.EFSA.2022.7209
The European Parliament and The Council of the European Union (2022). Sales of veterinary antimicrobial agents in 31 European countries in 2021 - Trends from 2010 to 2021 (Twelfth ESVAC report). doi: 10.2809/291108
European Parliament Council of the European Union Regulation (EU) 2019/6 of the European Parliament and of the Council of 11 December 2018 on veterinary medicinal products and repealing Directive 2001/82/EC, (2019). Off. J. Eur. Union. L4/43. https://eur-lex.europa.eu/eli/reg/2019/6/oj (accessed August 09 2023)
Fall I. S., Rajatonirina S., Yahaya A. A., Zabulon Y., Nsubuga P., Nanyunja M., et al. (2019). Integrated Disease Surveillance and Response (IDSR) strategy: current status, challenges and perspectives for the future in Africa. BMJ Glob Health 4, e001427. doi: 10.1136/BMJGH-2019-001427
FAO UNEP WHO and WOAH (2022). Global Plan of Action on One Health. Towards a more comprehensive One Health, approach to global health threats at the human-animal-environment interface (Rome: WOAH (World Organisation for Animal Health)). doi: 10.4060/cc2289en
Federal Office of Consumer Protection and Food Safety (2020) Zoonoses Monitoring 2020 - Summary of Findings and Conclusions (Braunschweig). Available at: https://www.bvl.bund.de/SharedDocs/Downloads/01_Lebensmittel/04_Zoonosen_Monitoring/Zoonosen_Monitoring_Bericht_2020_en-summary.pdf;jsessionid=09C3B82CC0C06F572D757F22DCC3A995.1_cid372?:blob=publicationFile&v=3 (Accessed January 26, 2023).
Federal Office of Public Health FOPH (2015) Usage of Antibiotics and Occurrence of Antibiotic Resistance in Bacteria from Humans and Animals in Switzerland (Bern). Available at: www.bag.admin.ch/star (Accessed January 28, 2023).
Feyes E. E., Diaz-Campos D., Mollenkopf D. F., Horne R. L., Soltys R. C., Ballash G. A., et al. (2021). Implementation of an antimicrobial stewardship program in a veterinary medical teaching institution. J. Am. Vet. Med. Assoc. 258, 170–178. doi: 10.2460/JAVMA.258.2.170
Finnish Food Authority (2022). FINRES-Vet 2021. Finnish Veterinary Antimicrobial Resistance Monitoring and Consumption of Antimicrobial Agents (Helsinki: Finnish Food Authority).
Food and Agriculture Organisation of the United Nations AMR situation analysis tool. Available at: https://www.fao.org/antimicrobial-resistance/resources/tools/amr-situation-analysis-tool/en/ (Accessed November 22, 2022a).
Food and Agriculture Organisation of the United Nations FAO Progressive Management Pathway for Antimicrobial Resistance (FAO-PMP-AMR). Available at: https://www.fao.org/antimicrobial-resistance/resources/tools/fao-pmp-amr/en/ (Accessed November 22, 2022b).
Food and Agriculture Organization of the United Nations (2020) ATLASS. Available at: https://www.fao.org/antimicrobial-resistance/resources/tools/fao-atlass/en/ (Accessed November 22, 2022).
Gerber M., Dürr S., Bodmer M. (2021). Reducing antimicrobial use by implementing evidence-based, management-related prevention strategies in dairy cows in Switzerland. Front. Vet. Sci. 7. doi: 10.3389/FVETS.2020.611682
Golding S. E., Ogden J., Higgins H. M. (2019). Shared goals, different barriers: A qualitative study of UK veterinarians’ and farmers’ beliefs about antimicrobial resistance and stewardship. Front. Vet. Sci. 6. doi: 10.3389/FVETS.2019.00132/BIBTEX
Gomez D. E., Arroyo L. G., Poljak Z., Viel L., Weese J. S. (2017). Implementation of an algorithm for selection of antimicrobial therapy for diarrhoeic calves: Impact on antimicrobial treatment rates, health and faecal microbiota. Veterinary J. 226, 15–25. doi: 10.1016/J.TVJL.2017.06.009
Gomez D. E., Arroyo L. G., Renaud D. L., Viel L., Weese J. S. (2021). A multidisciplinary approach to reduce and refine antimicrobial drugs use for diarrhoea in dairy calves. Veterinary J. 274, , 105713. doi: 10.1016/J.TVJL.2021.105713
Gozdzielewska L., King C., Flowers P., Mellor D., Dunlop P., Price L. (2020). Scoping review of approaches for improving antimicrobial stewardship in livestock farmers and veterinarians. Prev. Vet. Med. 180, 105025. doi: 10.1016/j.prevetmed.2020.105025
Ha L. T. T., RueanghIran C., Giang N. T. H., Thuy D. P., Phu D. H., Tuan Kiet B., et al. (2021). Antimicrobial usage surveillance through sales at veterinary drug shops intended for livestock in Vietnam. Front. Sustain Food Syst. 5. doi: 10.3389/FSUFS.2021.784500
Hao X. X., Li B. M., Wang C. Y., Zhang Q., Cao W. (2013). Application of slightly acidic electrolyzed water for inactivating microbes in a layer breeding house. Poult Sci. 92, 2560–2566. doi: 10.3382/PS.2013-03117
Haskell K. J., Schriever S. R., Fonoimoana K. D., Haws B., Hair B. B., Wienclaw T. M., et al. (2018). Antibiotic resistance is lower in Staphylococcus aureus isolated from antibiotic-free raw meat as compared to conventional raw meat. PloS One 13(12), e0206712. doi: 10.1371/JOURNAL.PONE.0206712
Hiki M., Kawanishi M., Abo H., Kojima A., Koike R., Hamamoto S., et al. (2015). Decreased Resistance to Broad-Spectrum Cephalosporin in Escherichia coli from Healthy Broilers at Farms in Japan After Voluntary Withdrawal of Ceftiofur. Foodborne Pathog. Dis. 12, 639–643. doi: 10.1089/FPD.2015.1960
INFAAR (2020) INFAAR-a research platform for accelerating laboratory-based surveillance of antimicrobial resistance in fisheries and aquaculture in India. Available at: https://amr-review (Accessed January 26, 2023).
Jayatilleke K. (2020). Challenges in implementing surveillance tools of high-income countries (HICs) in low middle income countries (LMICs). Curr. Treat Options Infect. Dis. 12, 191. doi: 10.1007/S40506-020-00229-2
Jensen V. F., de Knegt L. V., Andersen V. D., Wingstrand A. (2014). Temporal relationship between decrease in antimicrobial prescription for Danish pigs and the “Yellow Card” legal intervention directed at reduction of antimicrobial use. Prev. Vet. Med. 117, 554–564. doi: 10.1016/J.PREVETMED.2014.08.006
JVARM The Japanese Veterinary Antimicrobial Resistance Monitoring System:動物医薬品検査所. Available at: https://www.maff.go.jp/nval/tyosa_kenkyu/taiseiki/monitor/e_index.html (Accessed February 7, 2023).
Kannan G., Lee J. H., Kouakou B., Terrill T. H. (2019). Reduction of microbial contamination of goat meat using dietary brown seaweed (Ascophyllum nodosum) supplementation and chlorinated wash. Can. J. Anim. Sci. 99, 570–577. doi: 10.1139/CJAS-2018-0156/ASSET/IMAGES/LARGE/CJAS-2018-0156F3.JPEG
Kassem I. I., Kehinde O., Kumar A., Rajashekara G. (2017). Antimicrobial-resistant campylobacter in organically and conventionally raised layer chickens. Foodborne Pathog. Dis. 14, 29–34. doi: 10.1089/FPD.2016.2161
Kempf I., Kerouanton A., Bougeard S., Nagard B., Rose V., Mourand G., et al. (2017). Campylobacter coli in organic and conventional pig production in France and Sweden: Prevalence and antimicrobial resistance. Front. Microbiol. 8. doi: 10.3389/FMICB.2017.00955
Korean Veterinary Antimicrobial Usage and Resistance Monitoring (2022) National Antimicrobial Usage and Resistance Monitoring - Livestock and fishery. Available at: https://www.kdca.go.kr/nohas/en/common/main.do (Accessed January 28, 2023).
Kuipers A., Koops W. J., Wemmenhove H. (2016). Antibiotic use in dairy herds in the Netherlands from 2005 to 2012. J. Dairy Sci. 99, 1632–1648. doi: 10.3168/jds.2014-8428
Lam T. J. G. M., Jansen J., Wessels R. J. (2017). The RESET Mindset Model applied on decreasing antibiotic usage in dairy cattle in the Netherlands. Ir Vet. J. 70 (5). doi: 10.1186/s13620-017-0085-x
Lee H. Y., Loong S. K., Khoo J. J., Lim F. S., Chai L. C., Suntharalingam C., et al. (2017). Impact of hygiene intervention practices on microbial load in raw milk. J. Pure Appl. Microbiol. 11, 1281–1286. doi: 10.22207/JPAM.11.3.07
Llor C., Bjerrum L. (2014). Antimicrobial resistance: risk associated with antibiotic overuse and initiatives to reduce the problem. Ther. Adv. Drug Saf. 5, 229. doi: 10.1177/2042098614554919
Loayza-Villa F., Torres A., Zhang L., Trueba G., De Robles D., Pampite Y., et al. (2021). Removal of antimicrobial prophylaxis and its effect on swine carriage of antimicrobial-resistant coliforms. Sci. Prog. 104, 1–17. doi: 10.1177/00368504211050279
Luyckx K. Y., Van Weyenberg S., Dewulf J., Herman L., Zoons J., Vervaet E., et al. (2015). On-farm comparisons of different cleaning protocols in broiler houses. Poult Sci. 94, 1986–1993. doi: 10.3382/PS/PEV143
Mader R., behalf of EU-JAMRAI, on, Bourély C., Amat J.-P., Broens E. M., Busani L., et al. (2022). Defining the scope of the European Antimicrobial Resistance Surveillance network in Veterinary medicine (EARS-Vet): a bottom-up and One Health approach. J. Antimicrobial Chemotherapy 77, 816–826. doi: 10.1093/jac/dkab462
Mader R., Damborg P., Amat J. P., Bengtsson B., Bourély C., Broens E. M., et al. (2021). Building the European Antimicrobial Resistance Surveillance network in veterinary medicine (EARS-Vet). Eurosurveillance 26 (4). doi: 10.2807/1560-7917.ES.2021.26.4.2001359
Mahase E. (2021). Use of critically important antibiotics class has more than doubled on UK pig farms. BMJ 373, n1572. doi: 10.1136/BMJ.N1572
Manyi-Loh C., Mamphweli S., Meyer E., Okoh A. (2018). Antibiotic use in agriculture and its consequential resistance in environmental sources: Potential public health implications. Molecules : A J. Synthetic Chem. Natural Product Chem. 23 (4). doi: 10.3390/MOLECULES23040795
MARAN (2021) Monitoring of Antimicrobial Resistance and Antibiotic Usage in Animals in the Netherlands. Available at: https://www.wur.nl/en/Research-Results/Research-Institutes/Bioveterinary-Research/In-the-spotlight/Antibiotic-resistance/MARAN-reports.htm (Accessed January 26, 2023).
Methner U., Friese A., Rösler U. (2019). Competitive exclusion: A tool to combat extended-spectrum β-lactamase-producing Escherichia coli strains in chickens. Res. Vet. Sci. 123, 124–128. doi: 10.1016/J.RVSC.2019.01.003
Millar N., Aenishaenslin C., Lardé H., Roy J. P., Fourichon C., Francoz D., et al. (2022). Evidence of a decrease in sales of antimicrobials of very high importance for humans in dairy herds after a new regulation restricting their use in Quebec, Canada. Zoonoses Public Health 69, 370–381. doi: 10.1111/ZPH.12929
Morgans L. C., Bolt S., Bruno-McClung E., van Dijk L., Escobar M. P., Buller H. J., et al. (2021). A participatory, farmer-led approach to changing practices around antimicrobial use on UK farms. J. Dairy Sci. 104, 2212–2230. doi: 10.3168/JDS.2020-18874
Moura P., Sanders P., Heederik D., Van Geijlswijk I. M., Niza-Ribeiro J. (2022). Use of a new antimicrobial consumption monitoring system (Vet-AMNet): Application to Dutch dairy sector over a 9-year period. Front. Vet. Sci. 9. doi: 10.3389/fvets.2022.984771
Mourand G., Paboeuf F., Fleury M. A., Jouy E., Bougeard S., Denamur E., et al. (2017). Escherichia coli Probiotic Strain ED1a in Pigs Has a Limited Impact on the Gut Carriage of Extended-Spectrum-β-Lactamase-Producing E. coli. Antimicrob. Agents Chemother. 61 (1). doi: 10.1128/AAC.01293-16
Mulchandaniid R., Wangid Y., Gilbert M., Van Boeckelid T. P. (2023). Global trends in antimicrobial use in food-producing animals: 2020 to 2030. PloS Global Public Health 3, e0001305. doi: 10.1371/JOURNAL.PGPH.0001305
Murray C. J., Ikuta K. S., Sharara F., Swetschinski L., Robles Aguilar G., Gray A., et al. (2022). Global burden of bacterial antimicrobial resistance in 2019: a systematic analysis. Lancet 399, 629–655. doi: 10.1016/S0140-6736(21)02724-0
Musoke D., Kitutu F. E., Mugisha L., Amir S., Brandish C., Ikhile D., et al. (2020). A one health approach to strengthening antimicrobial stewardship in wakiso district, Uganda. Antibiotics 9, 1–16. doi: 10.3390/ANTIBIOTICS9110764
Nuotio L., Schneitz C., Nilsson O. (2013). Effect of competitive exclusion in reducing the occurrence of Escherichia coli producing extended-spectrum β-lactamases in the ceca of broiler chicks. Poult Sci. 92, 250–254. doi: 10.3382/PS.2012-02575
O’Neill J. (2016) Tackling Drug-Resistant Infections Globally: Final Report And Recommendations The Review On Antimicrobial Resistance Chaired By Jim O’Neill. Available at: https://amr-review.org/sites/default/files/160518_Final%20paper_with%20cover.pdf (Accessed November 16, 2022).
Pedroso A. A., Hurley-Bacon A. L., Zedek A. S., Kwan T. W., Jordan A. P. O., Avellaneda G., et al. (2013). Can probiotics improve the environmental microbiome and resistome of commercial poultry production? Int. J. Environ. Res. Public Health 10, 4534–4559. doi: 10.3390/IJERPH10104534
Pempek J., Masterson M., Portillo-Gonzalez R., Creutzinger K., Cheng T. Y., Habing G. (2022). The impact of antimicrobial stewardship training on calf producers’ Knowledge, treatment behaviors and quantified antimicrobial use. Microorganisms 10 (8). doi: 10.3390/MICROORGANISMS10081525
Phu D. H., Van, Cuong N., Truong D. B., Kiet B. T., Hien V. B., Thu H. T. V., et al. (2021). Reducing antimicrobial usage in small-scale chicken farms in Vietnam: A 3-year intervention study. Front. Vet. Sci. 7. doi: 10.3389/FVETS.2020.612993
Postma M., Vanderhaeghen W., Sarrazin S., Maes D., Dewulf J. (2017). Reducing antimicrobial usage in pig production without jeopardizing production parameters. Zoonoses Public Health 64, 63–74. doi: 10.1111/ZPH.12283
Powell N., Davidson I., Yelling P., Collinson A., Pollard A., Johnson L., et al. (2017). Developing a local antimicrobial resistance action plan: the Cornwall One Health Antimicrobial Resistance Group. J. Antimicrobial Chemotherapy 72, 2661–2665. doi: 10.1093/JAC/DKX164
Public Health Agency of Sweden and National Veterinary Institute (2022) Swedres-Svarm 2021. Sales of antibiotics and occurrence of resistance in Sweden (Solna/Uppsala). Available at: www.folkhalsomyndigheten.sewww.sva.sewww.folkhalsomyndigheten.se/publicerat-material/oratwww.sva.se/swedres-svarm/ (Accessed January 27, 2023).
Raasch S., Collineau L., Postma M., Backhans A., Sjölund M., Belloc C., et al. (2020). Effectiveness of alternative measures to reduce antimicrobial usage in pig production in four European countries. Porcine Health Manag 6 (6). doi: 10.1186/S40813-020-0145-6
Rees G. M., Bard A., Reyher K. K. (2021). Designing a national veterinary prescribing champion programme for welsh veterinary practices: The arwain vet cymru project. Antibiotics 10, 1–18. doi: 10.3390/ANTIBIOTICS10030253
Rhouma M., Soufi L., Cenatus S., Archambault M., Butaye P. (2022). Current insights regarding the role of farm animals in the spread of antimicrobial resistance from a one health perspective. Vet. Sci. 9 (9), 480. doi: 10.3390/vetsci9090480
Rojo-Gimeno C., Postma M., Dewulf J., Hogeveen H., Lauwers L., Wauters E. (2016). Farm-economic analysis of reducing antimicrobial use whilst adopting improved management strategies on farrow-to-finish pig farms. Prev. Vet. Med. 129, 74–87. doi: 10.1016/J.PREVETMED.2016.05.001
Roulette C. J., Caudell M. A., Roulette J. W., Quinlan R. J., Quinlan M. B., Subbiah M., et al. (2017). A two-month follow-up evaluation testing interventions to limit the emergence and spread of antimicrobial resistant bacteria among Maasai of northern Tanzania. BMC Infect. Dis. 17. doi: 10.1186/S12879-017-2857-Z
Rubio-García M. E., Rubio-Lozano M. S., Ponce-Alquicira E., Rosario-Cortes C., Nava G. M., Castañeda-Serrano M. P. (2015). Improving appearance and microbiologic quality of broiler carcasses with an allostatic modulator. Poult Sci. 94, 1957–1963. doi: 10.3382/PS/PEV144
Ruckert A., Fafard P., Hindmarch S., Morris A., Packer C., Patrick D., et al. (2020). Governing antimicrobial resistance: a narrative review of global governance mechanisms. J. Public Health Policy 41, 515–528. doi: 10.1057/S41271-020-00248-9
Salkind N. (2010). Encyclopedia of Research Design. (2455 Teller Road, Thousand Oaks California 91320 United States: SAGE Publications, Inc). doi: 10.4135/9781412961288
Sapkota A. R., Kinney E. L., George A., Hulet R. M., Cruz-Cano R., Schwab K. J., et al. (2014). Lower prevalence of antibiotic-resistant Salmonella on large-scale U.S. conventional poultry farms that transitioned to organic practices. Sci. Total Environ. 476–477, 387–392. doi: 10.1016/J.SCITOTENV.2013.12.005
Simonsen G.S., Blix H.S., Grave K., Urdahl A.M., Akselsen P.E., Andersen C.T., et al (2022). NORM/NORM-VET 2021 Usage of Antimicrobial Agents and Occurrence of Antimicrobial Resistance in Norway (Veterinærinstituttet og Folkehelseinstituttet. 164. NORM/NORM-VET(1) Veterinærinstituttet.
Schreuder J., Simitopoulou M., Angastiniotis K., Ferrari P., Wolthuis-Fillerup M., Kefalas G., et al. (2022). Development and implementation of a risk assessment tool for broiler farm biosecurity and a health intervention plan in the Netherlands, Greece, and Cyprus. Poult Sci. 102 (2), 102394. doi: 10.1016/J.PSJ.2022.102394
Seale A. C., Gordon N. C., Islam J., Peacock S. J., Scott J. A. G. (2017). AMR surveillance in low and middle-income settings - A roadmap for participation in the Global Antimicrobial Surveillance System (GLASS). Wellcome Open Res. 2 (92). doi: 10.12688/WELLCOMEOPENRES.12527.1
Sharma G., Mutua F., Deka R. P., Shome R., Bandyopadhyay S., Shome B. R., et al. (2022). Comparing the effectiveness of different approaches to raise awareness about antimicrobial resistance in farmers and veterinarians of India. Front. Public Health 10. doi: 10.3389/FPUBH.2022.837594
Shen L., Dyar O. J., Sun Q., Wei X., Yang D., Sun C., et al. (2021). The effectiveness of an educational intervention on knowledge, attitudes and reported practices on antibiotic use in humans and pigs: A quasi-experimental study in twelve villages in Shandong Province, China. Int. J. Environ. Res. Public Health 18, 1–12. doi: 10.3390/IJERPH18041940
Shen C., Zhong L. L., Yang Y., Doi Y., Paterson D. L., Stoesser N., et al. (2020). Dynamics of mcr-1 prevalence and mcr-1-positive Escherichia coli after the cessation of colistin use as a feed additive for animals in China: a prospective cross-sectional and whole genome sequencing-based molecular epidemiological study. Lancet Microbe 1, e34–e43. doi: 10.1016/S2666-5247(20)30005-7
Speksnijder D. C., Graveland H., Eijck I. A. J. M., Schepers R. W. M., Heederik D. J. J., Verheij T. J. M., et al. (2017). Effect of structural animal health planning on antimicrobial use and animal health variables in conventional dairy farming in the Netherlands. J. Dairy Sci. 100, 4903–4913. doi: 10.3168/JDS.2016-11924
Spellberg B., Rice L. B. (2019). Duration of antibiotic therapy: Shorter is better. Ann. Intern. Med. 171, 210. doi: 10.7326/M19-1509
Stanton I. C., Bethel A., Leonard A. F. C., Gaze W. H., Garside R. (2022). Existing evidence on antibiotic resistance exposure and transmission to humans from the environment: a systematic map. Environ. Evid 11, 1–24. doi: 10.1186/S13750-022-00262-2/FIGURES/21
Tang K. L., Caffrey N. P., Nóbrega D. B., Cork S. C., Ronksley P. E., Barkema H. W., et al. (2017). Restricting the use of antibiotics in food-producing animals and its associations with antibiotic resistance in food-producing animals and human beings: a systematic review and meta-analysis. Lancet Planet Health 1, e316–e327. doi: 10.1016/S2542-5196(17)30141-9
Teng K. T., Aerts M., Jaspers S., Ugarte-Ruiz M., Moreno M. A., Saez J. L., et al. (2022). Patterns of antimicrobial resistance in Salmonella isolates from fattening pigs in Spain. BMC Vet. Res. 18, 1–17. doi: 10.1186/S12917-022-03377-3/FIGURES/12
Thapaliya D., Forshey B. M., Kadariya J., Quick M. K., Farina S., O’ Brien A., et al. (2017). Prevalence and molecular characterisation of Staphylococcus aureus in commercially available meat over a one-year period in Iowa, USA. Food Microbiol. 65, 122–129. doi: 10.1016/J.FM.2017.01.015
Tiseo K., Huber L., Gilbert M., Robinson T. P., Van Boeckel T. P. (2020). Global trends in antimicrobial use in food animals from 2017 to 2030. Antibiotics (Basel) 9, 1–14. doi: 10.3390/ANTIBIOTICS9120918
Toya R., Sasaki Y., Uemura R., Sueyoshi M. (2022). Optimizing antimicrobial use by improving medication adherence among pig producers. Anim. Sci. J. 93, e13713. doi: 10.1111/asj.13713
Tricco A. C., Lillie E., Zarin W., O’Brien K. K., Colquhoun H., Levac D., et al. (2018). PRISMA extension for scoping reviews (PRISMA-scR): checklist and explanation. Ann. Intern. Med. 169, 467–473. doi: 10.7326/M18-0850
Turner A., Tisdall D., Barrett D. C., Wood S., Dowsey A., Reyher K. K. (2018). Ceasing the use of the highest priority critically important antimicrobials does not adversely affect production, health or welfare parameters in dairy cows. Vet. Rec 183, 67. doi: 10.1136/VR.104702
U.S. Department of Agriculture Animal and Plant Health Inspection Service (2022) National Animal Health Monitoring System. Available at: https://www.aphis.usda.gov/aphis/ourfocus/animalhealth/monitoring-and-surveillance/nahms (Accessed January 28, 2023).
U.S. Food and Drug Administration (2022a) The National Antimicrobial Resistance Monitoring System. Available at: https://www.fda.gov/animal-veterinary/national-antimicrobial-resistance-monitoring-system/2019-narms-update-integrated-report-summary-interactive-version (Accessed January 28, 2023).
U.S. Food and Drug Administration (2022b) Veterinary Laboratory Investigation and Response Network. Available at: https://www.fda.gov/animal-veterinary/science-research/veterinary-laboratory-investigation-and-response-network (Accessed February 3, 2023).
US Food and Drug Administration (2021). 2020 Summary Report On Antimicrobials Sold or Distributed for Use in Food-Producing Animals US Food and Drug Administration, Silver Springs, MD.
Van Boeckel T. P., Brower C., Gilbert M., Grenfell B. T., Levin S. A., Robinson T. P., et al. (2015). Global trends in antimicrobial use in food animals. Proc. Natl. Acad. Sci. U.S.A. 112, 5649–5654. doi: 10.1073/PNAS.1503141112/SUPPL_FILE/PNAS.201503141SI.PDF
van Dijk L., Hayton A., Main D. C. J., Booth A., King A., Barrett D. C., et al. (2017). Participatory policy making by dairy producers to reduce anti-Microbial use on farms. Zoonoses Public Health 64, 476–484. doi: 10.1111/ZPH.12329
Verrette L., Fairbrother J. M., Boulianne M. (2019). Effect of cessation of ceftiofur and substitution with lincomycin-spectinomycin on extended-spectrum-β-lactamase/ampC genes and multidrug resistance in escherichia coli from a Canadian broiler production pyramid. Appl. Environ. Microbiol. 85 (13). doi: 10.1128/AEM.00037-19
Veterinary Medicines Directorate Veterinary Antimicrobial Resistance and Sales Surveillance - UK VARSS. Available at: https://www.gov.uk/government/publications/veterinary-antimicrobial-resistance-and-sales-surveillance-2021 (Accessed January 26, 2023).
Vikram A., Rovira P., Agga G. E., Arthur T. M., Bosilevac J. M., Wheeler T. L., et al. (2017). Impact of “Raised without antibiotics” Beef cattle production practices on occurrences of antimicrobial resistance. Appl. Environ. Microbiol. 83. doi: 10.1128/AEM.01682-17
Wanninger S., Donati M., Di Francesco A., Hässig M., Hoffmann K., Seth-Smith H. M. B., et al. (2016). Selective pressure promotes tetracycline resistance of Chlamydia suis in fattening pigs. PloS One 11 (11), e0166917. doi: 10.1371/JOURNAL.PONE.0166917
WHO (2022) Global Database for Tracking Antimicrobial Resistance (AMR) Country Self- Assessment Survey (TrACSS) (WHO). Available at: https://amrcountryprogress.org/#/visualization-view (Accessed February 3, 2023).
World Health Organization Defined Daily Dose (DDD). Available at: https://www.who.int/tools/atc-ddd-toolkit/about-ddd (Accessed March 8, 2023).
World Health Organisation (2016) Antimicrobial Resistance A manual for Developing National Action Plans (Geneva). Available at: https://www.who.int/publications/i/item/antimicrobial-resistance-a-manual-for-developing-national-action-plans (Accessed November 25, 2022).
World Health Organization (2018) Critically Important Antimicrobials for Human Medicine (Switzerland). Available at: https://apps.who.int/iris/bitstream/handle/10665/312266/9789241515528-eng.pdf (Accessed December 16, 2022).
World Health Organization (2019) Antimicrobial resistance (World Health Organisation). Available at: https://www.who.int/news-room/fact-sheets/detail/antimicrobial-resistance (Accessed April 5, 2023).
World Organisation for Animal Health Veterinary Legislation Support. Available at: https://www.woah.org/en/what-we-offer/improving-veterinary-services/pvs-pathway/targeted-support/veterinary-legislation-support/ (Accessed November 22, 2022).
World Organisation for Animal Health (2019) OIE Tool for the Evaluation of the Performance of Veterinary Services (Paris). Available at: https://www.woah.org/app/uploads/2021/03/2019-pvs-tool-final.pdf (Accessed November 22, 2022).
World Organisation for Animal Health (2021) OIE Annual Report on Antimicrobial Agents Intended for Use in Animals (Paris). Available at: https://www.woah.org/app/uploads/2021/05/a-fifth-annual-report-amr.pdf (Accessed November 21, 2022).
Yusuf H., Idris S., Paul M. (2018). Antimicrobial usage surveillance of cattle in Indonesia to address antimicrobial resistance. Proceedings of the 1st International Conference Postgraduate School Universitas Airlangga : "Implementation of Climate Change Agreement to Meet Sustainable Development Goals" (ICPSUAS 2017). doi: 10.2991/icpsuas-17.2018.77
Keywords: antimicrobial resistance, interventions, antimicrobial stewardship, antimicrobial usage, behavioural science, One Health, animal
Citation: Jacobsen ABJE, Ogden J and Ekiri AB (2023) Antimicrobial resistance interventions in the animal sector: scoping review. Front. Antibiot. 2:1233698. doi: 10.3389/frabi.2023.1233698
Received: 02 June 2023; Accepted: 27 July 2023;
Published: 31 August 2023.
Edited by:
Kebede Amenu, Addis Ababa University, EthiopiaReviewed by:
Laura Montoro-Dasi, Universidad Cardenal Herrera-CEU, CEU Universities, SpainIndranil Samanta, West Bengal University of Animal and Fishery Sciences, India
Copyright © 2023 Jacobsen, Ogden and Ekiri. This is an open-access article distributed under the terms of the Creative Commons Attribution License (CC BY). The use, distribution or reproduction in other forums is permitted, provided the original author(s) and the copyright owner(s) are credited and that the original publication in this journal is cited, in accordance with accepted academic practice. No use, distribution or reproduction is permitted which does not comply with these terms.
*Correspondence: Abel B. Ekiri, ab.ekiri@surrey.ac.uk