- Environmental Solutions Research Centre, Unitec Institute of Technology, Auckland, New Zealand
As our global population increases, the resulting waste mountain continues to rise. It has been identified that the Construction Industry contributes to a large proportion of the waste to landfill (and cleanfill) sites. Whilst there have been a multitude of commercial ventures and research-based activities targeted to challenge waste volumes, the ambitions of a truly circular economy for this industry remain far from realised. This article will discuss industry examples of waste minimisation initiatives which have been implemented successfully to support a less linear approach and encourage sustainable waste management for industrialised nations. We also identify limitations of this decentralised approach to resource management and suggest how the creation of resource markets, on both national and international scales, could connect the waste management loop for a vastly improved environmental outcome.
Introduction
The greatest worldwide users of raw materials from natural resources are from the Construction sector (UN Environment, 2017). The industry also consumed around 50% of steel produced in 2015 and accounted for 36% of final energy-use and 39% of energy-related CO2 emissions in 2017 (World Steel Association WSA., 2015; World Economic Forum WEF., 2016; Global Alliance for Buildings Construction., 2018).
It is therefore not surprising that Construction and Demolition (C&D) waste accounts for a large share of the total municipal waste in developed countries and most landfill waste in developing countries (Ibrahim, 2016). The proportion of landfilled construction waste (by weight) compared with total amount of waste varies between 13 and 61% by country (Figure 1), with an average proportion of around 30% (Luangcharoenrat et al., 2019). C&D waste can have environmental implications when disposed into landfill, such as the release of inorganic pollutants and greenhouse gas emissions (Pimenteira et al., 2005; Roussat et al., 2008; Dubey et al., 2010). Furthermore, C&D waste takes up landfill space which is unsustainable and a scarce resource in smaller regions (Poon et al., 2003).
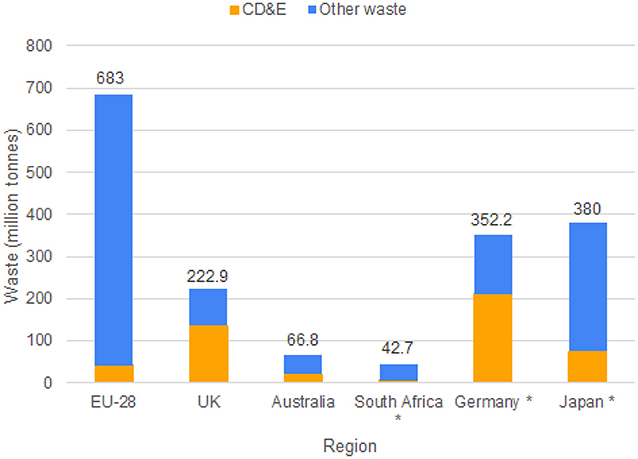
Figure 1. Total waste per year and the corresponding percentage of Construction Demolition & Excavation waste for 2016—*except for South Africa (2017), Germany (2015), and Japan (2011) (Blue Environment, 2018; Department of Environmental Affairs RSA, 2018; Federal Ministry for the Environment Nature Conservation Nuclear Safety., 2018; Yonetani, 2018; Department of Environment Food Rural Affairs, 2019; Eurostat., 2019).
The current waste pathway for buildings tends to be linear—with a mantra of “take-make-dispose” and little priority is given to waste management (Osmani et al., 2008; Andrews, 2015; Osmani and Villoria-Sáez, 2019). The hierarchy of waste management puts reduction as the top priority for waste minimisation, followed by reuse, recycle, and finally landfill disposal (Peng et al., 1997; Yuan and Shen, 2011). Reduction in the use of raw virgin materials can occur mainly during the design stage of construction and should be supported by a sustainable procurement strategy. Reuse of waste during construction and the recycling of residual waste in the final construction stages also provide opportunities for waste minimisation.
C&D waste can be classified into two groups: new-build waste and demolition waste. New-build waste comes from new materials during construction, which arises due to the way we design and build. Usually construction activities generate certain amounts of unavoidable waste—about 1–10% of purchased materials (Shen et al., 2005; Tam and Lu, 2016). During demolition (mechanical destruction of a building), most building components can only be recycled (into a new form) or disposed into landfill (Macozoma, 2001). Buildings produce waste over their lifetime through reconstitution, renovation, and partial replacements—not just at the end-of-life; therefore, demolition begins when a building is first serviced, maintained or adapted (Thomsen et al., 2011). Demolition waste can be inert and recyclable (bricks, concrete, sand, untreated wood) or include hazardous substances such as asbestos insulation, treated wood and lead-based paints (Roussat et al., 2008; United States Environmental Protection Agency USEPA, 2008; Coudert et al., 2013), thus limiting recycle potential.
Current Solutions
There are many different opportunities to reduce waste in the construction industry but none of them are sufficient to create a truly circular economy. In an ideal waste management system, the construction of a new building would first consider how much new-build waste can be designed out through careful planning for incoming materials (Poon et al., 2001; Osmani et al., 2008; Andrews, 2015). This would also consider the end of the building's life by designing it for deconstruction, so that it can be mined for future resources. In addition, buildings would be designed to minimize the use of virgin raw materials and prefer reused and recycled materials (Thomsen et al., 2011; Tingley and Davison, 2011; Andrews, 2015) A sustainable procurement strategy has to consider these reuse and recycling opportunities to decrease the use of virgin raw materials. On-site waste separation has many limitations and can only be applied in certain circumstances, however the selection of certain reusable raw materials (such as timber and sand) may be reclaimed for reuse directly on site or donated via online sharing platforms, such as Civil Share. Co-mingled waste recovery centres are the least problematic for waste separation where available. However, these centres are localised (usually in large cities) and do not help all regions of the country (or world). Finally, the loss of ~20% of waste to landfill due to construction waste associated with packaging is an issue for the suppliers rather than the waste contractor, but requires a solution.
Waste which is unavoidably produced in the C&D industry can be recovered to reuse and recycle before entering landfill. The reuse of materials is preferred as it tends to use less energy for processing and so has a lower cost than recycling (Peng et al., 1997; Yuan and Shen, 2011). There is also less generation of associated wastes. Nonetheless, recycling can process C&D waste into new materials, which helps to reduce the consumption of virgin raw materials and energy (Pimenteira et al., 2005).
In New Zealand, Green Gorilla is a waste management company that accepts and sorts co-mingled waste from the Construction Industry only for recycling. They receive around 10,000 tonnes of waste each month, over 75% of which is diverted from landfill. Their processing systems recover timber, non-ferrous metals, cardboard, plasterboard, steel, rocks, and concrete. Treated and untreated wood waste can be chipped and used in landscaping and for biofuel production, while plasterboard can be further processed and used in fertilisers. Residual wastes (typically 20% of total) or waste unsuitable for recycling (e.g., plastics) are sent to a licensed landfill (Green Gorilla Youtube., 2016; Green Gorilla Website., 2019; Sustainable Business Network website., 2019). Even with New Zealand's relatively low waste levy this is a successful commercial operation that recovers value from an otherwise problematic waste stream.
Whilst recycling (into new products) is an option for achieving significant diversion from landfill, it fails to provide resources for future construction and therefore does not complete the desired waste management loop. Other disadvantages to the use of this type of waste processing include health and safety issues associated with handling co-mingled waste (which may contain asbestos or lead-based products) and also energy requirements for recycling in contrast with reuse.
A building designed for deconstruction is dismantled to recover and reuse materials (Thomsen et al., 2011; Rios et al., 2015). This process considers buildings to be future resources for construction materials (Kibert et al., 2001); however, this end-of-life consideration is not common. Many older buildings in Germany are not designed for deconstruction (Kuehlen et al., 2014). Likewise, in Japan many buildings contain composite structures or composite materials, making them difficult to dismantle (Nakajima, 2014). While deconstruction is vital to creating a more circular economy, it can take considerably more time than demolition. This is due to the need to carefully dismantle buildings, recover and then separate the materials; as a result, there may be increased labour costs, construction delay penalties, and disincentives to implement deconstruction practices (McGrath et al., 2000; Nakajima, 2014). Despite findings that revenue generated from deconstruction can reduce overall costs to 10% less than demolition costs (Guy and McLendon, 2003), demolition remains more favourable in many countries.
Waste minimisation for this industry has often focused on the design (birth) and the deconstruction/demolition (end of life processing), however there are opportunities available both during construction and within a building's lifetime. The C&D industry needs a paradigm shift in its approach to construction materials—one that recognizes that buildings can become valuable resources throughout their lives, and consequently creates a supply of reclaimed materials. This is a “circular economy,” which calls for a new economic model where waste is the input of a continuous material loop (Ellen MacArthur Foundation EMF., 2015; Ghisellini et al., 2018). The aim is to close this loop, so that fewer virgin materials are used, waste materials become resources for the “next generation of products,” and landfill is minimised (Andrews, 2015).
This article investigates on-site waste management initiatives including social, environmental and financial barriers. We present our industry examples demonstrating successful waste sorting and waste sharing practices in New Zealand, which show the potential to evolve a resource market in other countries around the world. Moreover, we suggest that a considerable barrier to creating a circular economy for construction waste is the lack of a clear resource market.
Reuse—Waste Separation at Source
On-Site Waste Separation
On-site separation can reduce waste, increase cost savings and has the potential to increase employment on construction sites. From 2017 to 2018, we evaluated the use of source-separation (on-site waste separation) for the recovery of construction waste on an active new-build site. The site chosen was a residential development of 350 m2 floor area in Auckland (New Zealand), designed of primarily masonry blocks and internal timber framing. In this study, 2,400 kg of waste was generated and 1,750 kg diverted from landfill (a 73% diversion), which resulted in savings of about NZD$650 per skip required for landfill waste. A square of 25 m2 land with a 9 m3 skip were made available for sorting and storing waste on site. Waste was separated into timber, concrete and masonry, plasterboard, metals, and plastics and packaging. Untreated timber and concrete & masonry waste were collected by the public or reused on-site, while plasterboard was sent to be recycled, metals sold as scrap material and plastics and packaging returned to the supplier. Most treated timber was sent to landfill, and some was reused for small projects (such as on animal farms). Photos of waste were posted online on the Free Stuff and TradeMe sharing websites, where the public could arrange to pick up the materials for no charge.
Generally, this case study was found to be low risk and inexpensive. Contractors expressed that hiring staff to sort waste full-time would be costly, so they hired temporary labourers on days predicted to produce more waste (such as days for deliveries and unloading materials) or requested voluntary work from the public who wanted to take and use the waste material. For this study, about 30 h at the site over ten visits was spent, during which we facilitatedthe public collections for waste, informed new contractors of the on-site waste separation, and ensured waste was stored properly and protected from damage. Unfortunately, in other projects this may incur extra costs, effort or employment.
This study demonstrated that useful materials can be recovered and reused by sorting through waste. On-site waste separation generally requires less effort than the process of aggregating waste and then sorting it off-site (Poon et al., 2001; Krook and Eklund, 2010). Krook and Eklund (2010) discussed the difficulty in sorting municipal waste in Swedish recycling plants, where they estimated about 20% (more than 200,000 tonnes) of waste was incorrectly sorted. Direct reuse of material within one construction organisation (without entering into a marketplace) will reduce waste handling. By increasing the number of staff to sort, check and remove hazardous waste, the number of errors may decrease, but this is often impractical due to the increased labour costs.
On-Line Waste Share
Waste sharing online platforms can make it easier to upcycle materials, and so generate an interest in reclaimed C&D materials. Civil Share is an Auckland-based free website app that allows users to share construction materials, labour and equipment. It currently has over 3,100 users and experienced a 40% increase in users since November 2018. The use of Civil Share over the last 2 years has resulted in the diversion of 10,100 tonnes of waste (Civil Share., 2019). By using the Civil Share platform, an Auckland construction company (ICB Retaining and Construction) has managed to divert 281 tonnes of construction waste since 2016. During this time, ICB has saved over $120,000—~$62,000 saved from landfill bin costs and $64,000 generated by hiring out equipment and personnel between jobs (as all equipment and labour are not constantly needed, thus are underutilised).
Barriers for Reuse
Despite the relative success of both of the examples discussed, there remain multiple barriers restricting the wide scale application of these types of initiatives. Recent conversations with contractors have highlighted the following issues which still present significant barriers to certain waste management practices. These include:
• Lack of training and education; although on-site waste separation is comparatively simple, it does involve educating site workers which has been complicated by skills shortages which have plagued the construction industry for over 30 years (Dainty et al., 2004). Attracting and retaining skilled construction workers has become a major priority (Yankov and Kleiner, 2001) which has been partially addressed by recruiting foreign and migrant workers (Clark, 2003). However, the reliance of engagement of migrant workers on short or limited term contracts (McGrath-Champ et al., 2016) has made efficient on-site separation unrealistic, in part due to language barriers, high staff turnovers rates and project management logistics (Annette Day, Naylor Love Contractors, pers comm.).
• Insufficient logistics; for many contractors there is limited space available on site for effective on-site waste separation. This restricts separation for recycling and options for retaining materials on-site for future reuse are limited.
• Health and safety issues; although less of an issue for new builds, the deconstruction or demolition process during a building's lifetime may result in the production of asbestos contaminated materials which can present considerable health and safety issues for waste providers as well as site contractors. Their presence also prohibits reuse or recycling.
• Lack of incentives; Waste disposal costs and accompanying taxes and/or levies are often low, while labour is highly taxed in many industrialised economies. This encourages raw material use and discourages to employ labour for reuse and recycling efforts.
Resource Markets For The Construction Industry
These waste management initiatives have contributed considerably to the construction waste industry in New Zealand, however it is clear that a truly circular waste economy is still far from achievable. For this cycle to operate continuously, the creation of a resource market is necessary (Figure 2).
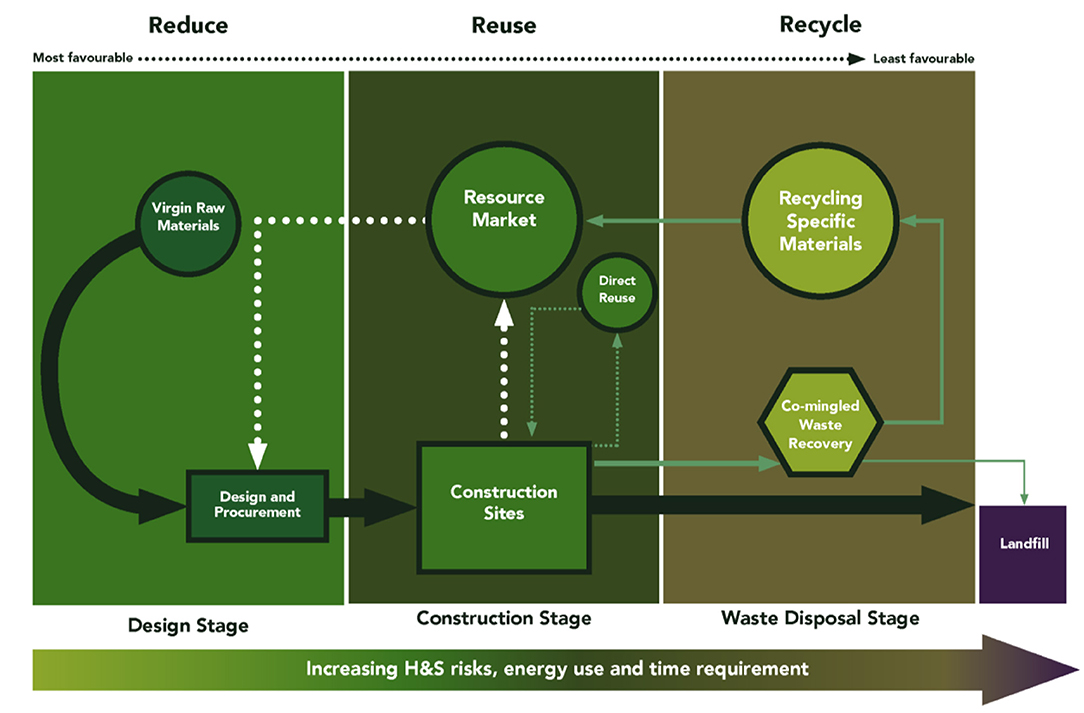
Figure 2. The cycle of input and output materials in the C&D industry split into three stages: reduce, reuse, and recycle. A circular economy is one which avoids the input of virgin raw materials, and output of waste going to landfill. Currently, the typical life cycle for a building is linear (as shown by the black arrows).
For materials to be reused, there needs to be a market—a supply and demand of second-hand materials, offcuts and recycled materials (Wang et al., 2010), which can be given away or sold to generate a profit (United States Environmental Protection Agency USEPA, 2008; Behnan and Hinkley, 2014) and where materials which cannot be reused directly are recycled. Reuse and recycling can only be sustained if there is a market demand for reclaimed materials, so that these materials can be fed back into the C&D industry and economy.
Barriers previously identified (Section Barriers for Reuse) which currently restrict the wide scale application of construction material reuse may be reduced by the creation of a resource market. This resource market can provide specialized support with waste management to reduce the pressure on non-trained site operators; solve on-site logistics of waste separation and reduce health and safety issues by externally managing construction materials. In addition, a well-functioning resource market could increase the value of waste materials through effective trading which incentivises the use of these materials rather than relying on virgin raw materials.
Such a market could be facilitated by a share centre or a large retail yard where reclaimed construction materials can be bought and sold (Kibert et al., 2001; United States Environmental Protection Agency USEPA, 2008). Online sharing platforms can also facilitate resource sharing and further increase demands for resources. Decentralised waste-sharing platforms could allow for better recirculation of materials, a wider outreach and a more secure resource market; however, these platforms would still need to conform to some centralised regulation to ensure quality and safety of the materials (Pizarro, 2017). The resource market will also need to define a role to undertake checks for structural integrity for the reuse of various construction items as well as protect consumers from any hazardous components. This has huge financial implications and it is unlikely that this type of commercial venture could profit without the right economic environment. It has been proposed that taxing virgin raw material extraction and using the proceeds to reduce taxation on labour could shift the balance to an economy employing more labour to reduce the use of raw materials by labour intensive reuse and recycling (McCarthy et al., 2018).
The inconsistency of a supply of quality reclaimed materials can be problematic. Reclaimed materials can sometimes be inferior to virgin materials; due to age of the material and how the material was reclaimed and sorted (Falk, 1999; United States Environmental Protection Agency USEPA, 2008; Ghisellini et al., 2018). This would affect both the supply of acceptable materials, and the demand, as it may encourage the perception that all reclaimed materials are of poorer quality. Theoretically, with a closed circular economy the supply of quality reclaimed materials would thin after several cycles when materials age and are inevitably damaged. However, in some instances, quality can be improved with better planning around deconstruction, design and waste sorting. Increased use of reclaimed materials over time will support reputation and the implementation of better waste management practices (around design, on-site waste separation and assessments for safety and quality) can prove the adequacy of these materials (Roussat et al., 2008; Rios et al., 2015).
Conclusion
For industrialised nations, there are a variety of potential pathways for construction waste management which maximise diversion from landfill. On-site waste separation, reuse and recycling are all viable options for construction materials, however they have limitations which may not be surmountable in some instances. The main barrier to a circular economy for the construction industry is the lack of clear resource markets across the world. Only by recognising the value of building materials both raw virgin, in-situ, and post deconstruction/demolition as future resources can a supply and demand for reclaimed materials be sustained. Quality materials need to be guaranteed through the implementation of better waste management practices and diligence around hazardous materials. This will foster the perception that reclaimed materials are valuable, and that current buildings can be future resources. Quantification of the potential benefits of a developed resource market will require extensive economic modelling which could be used to inform future taxation regimes.
Data Availability Statement
All datasets generated for this study are included in the article/supplementary material.
Author Contributions
T-AB and SW designed the work. T-AB and JL wrote the paper. GH contributed to the industry examples. IC collected the raw data. T-AB, JL, SW, and GS contributed to the structure and editing of the paper. All authors contributed to the article and approved the submitted version.
Conflict of Interest
The authors declare that the research was conducted in the absence of any commercial or financial relationships that could be construed as a potential conflict of interest.
Acknowledgments
We would like to thank Civil Share, especially Regan Burke for sharing data and industry knowledge. Sincere thanks also to Annette Day of Naylor Love Construction and Mark Roberts of Auckland Council.
References
Andrews, D. (2015). The circular economy, design thinking and education for sustainability. Local Econ. 30, 305–315. doi: 10.1177/0269094215578226
Behnan, C., and Hinkley, J. A. (2014). Lansing gets $6M to fight blight. Lansing State J. Retrieved from: http://www.lansingstatejournal.com/story/news/local/2014/12/16/lansingblight-~million/20479683/
Blue Environment (2018). National waste report 2018. Prepared for Australian Government – Department of the Environment and Energy. Retrieved from: https://www.environment.gov.au/system/files/resources/7381c1de-31d0-429b-912c-91a6dbc83af7/files/national-waste-report-2018.pdf
Civil Share. (2019). Retrieved from: http://civilshare.co.nz/ (accessed October 31, 2019).
Clark, P. (2003). Labour Agency Set to Import Workers From Romania. Retrieved from: https://www.building.co.uk/news/labour-agency-set-to-import-workers-from-romania/1026568.article (accessed November 14, 2019).
Coudert, L., Blais, J.-F., Mercier, G., Cooper, P., Gastonguay, L., Morris, P., et al. (2013). Pilot-scale investigation of the robustness and efficiency of a copper-based treated wood wastes recycling process. J. Hazard. Mater. 261, 277–285. doi: 10.1016/j.jhazmat.2013.07.035
Dainty, A. R. J., Ison, S. G., and Root, D. S. (2004). Bridging the skills gap: a regionally driven strategy for resolving the construction labour market crisis. Eng. Constr. Archit. Manag. Bradf. 11, 275–283. doi: 10.1108/09699980410547621
Department of Environment Food Rural Affairs (2019). UK Statistics on Waste. Retrieved from: https://assets.publishing.service.gov.uk/government/uploads/system/uploads/attachment_data/file/784263/UK_Statistics_on_Waste_statistical_notice_March_2019_rev_FINAL.pdf
Department of Environmental Affairs RSA (2018). South Africa State of Waste Report. Retrieved from: http://sawic.environment.gov.za/documents/8641.pdf
Dubey, B., Townsend, T., and Solo-Gabriele, H. (2010). Metal loss from treated wood products in contact with municipal solid waste landfill leachate. J. Hazard. Mater. 175, 558–568. doi: 10.1016/j.jhazmat.2009.10.042
Ellen MacArthur Foundation EMF. (2015). Towards a Circular Economy: Business Rationale for an Accelerated Transition. UK: Ellen MacArthur Foundation. Retrieved from: https://www.ellenmacarthurfoundation.org/assets/downloads/TCE_Ellen-MacArthur-Foundation_9-Dec-2015.pdf
Eurostat. (2019). Waste Statistics: Statistics Explained. Retrieved from: https://ec.europa.eu/eurostat/statistics-explained/pdfscache/1183.pdf
Falk, R. H. (1999). “The properties of lumber and timber recycled from deconstructed buildings,” in Proceedings of the Pacific Timber Engineering Conference, Rotorua New Zealand, Vol. 2 ed, eds. G. B. Walford, D. J. Gaunt Forest Research Bulletin No. 212, 255-257. Retrieved from: https://www.fpl.fs.fed.us/documnts/pdf1999/falk99b.pdf
Federal Ministry for the Environment Nature Conservation Nuclear Safety. (2018). Waste Management in Germany 2018. Retrieved from: https://www.bmu.de/fileadmin/Daten_BMU/Pools/Broschueren/abfallwirtschaft_2018_en_bf.pdf
Ghisellini, P., Ripa, M., and Ulgiati, S. (2018). Exploring environmental and economic costs and benefits of a circular economy approach to the construction and demolition sector. A literature review. J. Clean. Prod. 178, 618–643. doi: 10.1016/j.jclepro.2017.11.207
Global Alliance for Buildings Construction. (2018). 2018 Global Status Report. Retrieved from: https://wedocs.unep.org/bitstream/handle/20.500.11822/27140/Global_Status_2018.pdf?sequence=1&isAllowed=y (accessed on November 11, 2019)
Green Gorilla Website. (2019). Processing Facility. Retrieved from https://www.greengorilla.co.nz/processing-facility/ (accessed October 31, 2019).
Green Gorilla Youtube. (2016). Green Gorilla Waste Processing Facility. Retrieved from https://www.youtube.com/watch?v=53WEF2LEPsA&feature=youtu.be
Guy, B., and McLendon, S. (2003). Building Deconstruction: Reuse and Recycling of Building Materials. Retrieved from: http://www.lifecyclebuilding.org/docs/Six%20House%20Building%20Deconstruction.pdf.
Ibrahim, M. I. M. (2016). Estimating the sustainability returns of recycling construction waste from building projects. Sustain. Cities Soc. 23, 78–93. doi: 10.1016/j.scs.2016.03.005
Kibert, C. J., Chini, A., and Languell, J. (2001). “Deconstruction as an essential component of sustainable construction,” in Proceedings of the CIB World Building Congress, (Wellington, New Zealand), 1–11. Retrieved from: https://www.irbnet.de/daten/iconda/CIB3122.pdf
Krook, J., and Eklund, M. (2010). The strategic role of recycling centres for environmental performance of waste management systems. Appl. Ergon. 41, 362–367. doi: 10.1016/j.apergo.2009.06.012
Kuehlen, A., Volk, R., Stengel, J., and Schultmann, F. (2014). “Deconstruction project planning considering local environmental impacts,” in Paper presented at the meeting of the 2014 (5th) International Conference on Engineering, Project, and Production Management (EPPM), (Port Elizabeth, South Africa). Retrieved from: https://www.researchgate.net/publication/272676149_Deconstruction_Project_Planning_Consideri ng_Local_Environmental_Impacts doi: 10.32738/CEPPM.201411.0003
Luangcharoenrat, C., Intrachooto, S., Peansupap, V., and Sutthinarakorn, W. (2019). Factors influencing construction waste generation in building construction: thailand's perspective. Sustainability 11, 1–17. doi: 10.3390/su11133638
Macozoma, D. S. (2001). Building deconstruction - international report. Prepared for International Council for Research and Innovation in Building and Construction (CIB). Retrieved from: http://site.cibworld.nl/dl/publications/Pub278/05Deconstruction.pdf
McCarthy, A., Dellink, R., and Bibas, R. (2018). The Macroeconomics of the Circular Economy Transition: A Critical Review of Modelling Approaches, OECD Environment Working Papers, No. 130. Paris: OECD Publishing. doi: 10.1787/af983f9a-en
McGrath, C., Fletcher, S. L., and Bowes, H. M. (2000). “Chapter 8 – UK deconstruction report,” in Overview of Deconstruction in Selected Countries (Publication 252), eds. C. J. Kibert, and A. R. Chini (Florida, USA), 158–180. Retrieved from: https://www.iip.kit.edu/downloads/CIBpublication252.pdf
McGrath-Champ, S., Rosewarne, S., and Rittau, Y. (2016). From one skill shortage to the next: the Australian construction industry and geographies of a global labour market. J. Ind. Relat. 53, 467–485. doi: 10.1177/0022185611412897
Nakajima, S. (2014). “Barriers for deconstruction and reuse/recycling of construction materials in Japan,” in Barriers for Deconstruction and Reuse/Recycling of Construction Materials (Publication 397), ed. S. Nakajima (Rotterdam, Netherlands), 53–73. Retrieved from: https://drive.google.com/file/d/11UEZw8xJwWAcjgIbKmIhWYmu5DuHNEuC/view.
Osmani, M., Glass, J., and Price, A. (2008). Architects' perspectives on construction waste reduction by design. Waste Manag. 28, 1147–1158. doi: 10.1016/j.wasman.2007.05.011
Osmani, M., and Villoria-Sáez, P. (2019). “Chapter 19 - current and emerging construction waste management status, trends and approaches,” in Waste - A Handbook for Management 2nd Edn, eds. T. M. Letcher, D. A. Vallero (London: Academic Press), 365–380. doi: 10.1016/B978-0-12-815060-3.00019-0
Peng, C.-L., Scorpio, D. E., and Kibert, C. J. (1997). Strategies for successful construction and demolition waste recycling operations. Construct. Manag. Econ. 15, 49–58. doi: 10.1080/014461997373105
Pimenteira, C., Carpio, L., Rosa, L., and Tolmansquim, M. (2005). Solid wastes integrated management in Rio de Janeiro: input–output analysis. Waste Manag. 25, 539–553. doi: 10.1016/j.wasman.2004.08.008
Pizarro, I. O. (2017). Designing out waste - exploring barriers for material recirculation. (Ph.D. Thesis). Chalmers University of Technology. Retrieved from: https://www.mistraurbanfutures.org/files/designing_out_waste_exploring_barriers_for_material_recirculation_phd_thesis_isabel_ordonez_pizarro.pdf
Poon, C., Yu, A. T., and Ng, L. (2001). On-site sorting of construction and demolition waste in Hong Kong. Resourc. Conserv. Recycl. 32, 157–172. doi: 10.1016/S0921-3449(01)00052-0
Poon, C., Yu, A. T., and Ng, L. (2003). Comparison of low-waste building technologies adopted in public and private housing projects in Hong Kong. Eng. Construct. Archit. Manag. 10, 88–98. doi: 10.1108/09699980310466578
Rios, F. C., Chong, W. K., and Grau, D. (2015). Design for disassembly and deconstruction - challenges and opportunities. Proc. Eng. 118, 1296–1304. doi: 10.1016/j.proeng.2015.08.485
Roussat, N., Méhu, J., Abdelghafour, M., and Brula, P. (2008). Leaching behaviour of hazardous demolition waste. Waste Manag. 28, 2032–2040. doi: 10.1016/j.wasman.2007.10.019
Shen, L., Wu, Y., Chan, E., and Hao, J. (2005). Application of system dynamics for assessment of sustainable performance of construction projects. J. Zhejiang Univ. Sci. 6A, 339–349. doi: 10.1631/jzus.2005.A0339
Sustainable Business Network website. (2019). Green Gorilla. Retrieved from: https://sustainable.org.nz/members/auckland/auckland/green-gorilla/ (accessed October 31, 2019).
Tam, V. W.-Y., and Lu, W. (2016). Construction waste management profiles, practices, and performance: a cross-jurisdictional analysis in four countries. Sustainability 8:190. doi: 10.3390/su8020190
Thomsen, A., Schultmann, F., and Kohler, N. (2011). Deconstruction, demolition and destruction. Build. Res. Inform. 39, 327–332. doi: 10.1080/09613218.2011.585785
Tingley, D. D., and Davison, B. (2011). Design for deconstruction and material reuse. Proc. Inst. Civil Eng. Energy 164, 195–204. doi: 10.1680/ener.2011.164.4.195
UN Environment, and International Energy Agency. (2017). Towards a Zero-Emission, Efficient, and Resilient Buildings and Construction Sector Global Status Report 2017. Retrieved from: http://www.worldgbc.org/sites/default/files/UNEP188_GABC_en%28web%29.pdf (accessed August 25, 2018).
United States Environmental Protection Agency USEPA (2008). Lifecycle Construction Resource Guide. Retrieved from: https://www.lifecyclebuilding.org/docs/Lifecycle%20Construction%20Resource%20Guide.pdf
Wang, J., Yuan, H., Kang, X., and Lu, W. (2010). Critical success factors for on-site sorting of construction waste: a China study. Resourc. Conserv. Recyc. 54, 931–936. doi: 10.1016/j.resconrec.2010.01.012
World Economic Forum WEF. (2016). Shaping the Future of Construction: A Breakthrough in Mindset and Technology. Retrieved from: https://www.weforum.org/reports/shaping-the-future-of-construction-a-breakthrough-in-mindset-and-technology. (accessed on November 11, 2019).
World Steel Association WSA. (2015). World Steel in Figures 2015. Retrieved from: https://www.worldsteel.org/en/dam/jcr:7f5a36e2-e71e-4c58-b93f-f78d0c5933e4/WSIF_2015_vfinal.pdf (accessed on November 11, 2019).
Yankov, L., and Kleiner, B. H. (2001). Human resource issues in the construction industry. Manag. Res. News 24, 101–105. doi: 10.1108/01409170110782711
Yonetani, H. (2018). Construction and Demolition Waste Management in Japan. Retrieved from: http://www.uncrd.or.jp/content/documents/2661Parallel%20Roundtable(2)-Presentation(4)-Hideko%20Yonetani.pdf
Keywords: construction waste management, circular waste economy, on-site waste separation, recycling, reuse
Citation: Low JK, Wallis SL, Hernandez G, Cerqueira IS, Steinhorn G and Berry T-A (2020) Encouraging Circular Waste Economies for the New Zealand Construction Industry: Opportunities and Barriers. Front. Sustain. Cities 2:35. doi: 10.3389/frsc.2020.00035
Received: 05 December 2019; Accepted: 10 June 2020;
Published: 21 July 2020.
Edited by:
Elise Louise Amel, University of St. Thomas, United StatesReviewed by:
Ling Shao, China University of Geosciences, ChinaCastorina Silva Vieira, University of Porto, Portugal
Copyright © 2020 Low, Wallis, Hernandez, Cerqueira, Steinhorn and Berry. This is an open-access article distributed under the terms of the Creative Commons Attribution License (CC BY). The use, distribution or reproduction in other forums is permitted, provided the original author(s) and the copyright owner(s) are credited and that the original publication in this journal is cited, in accordance with accepted academic practice. No use, distribution or reproduction is permitted which does not comply with these terms.
*Correspondence: Shannon Lee Wallis, c3dhbGxpc0B1bml0ZWMuYWMubno=