- 1CSIR-Central Institute of Mining and Fuel Research, Dhanbad, Jharkhand, India
- 2Department of Life Sciences, School of Natural Sciences, Central University of Jharkhand, Ranchi, Jharkhand, India
Urban ecosystems play a crucial role in providing a wide range of services to their inhabitants, and their functioning is deeply intertwined with the effects of climate change. The present review explores the dynamic interplay between urban ecosystem services and climate change, highlighting the reciprocal relationships, impacts, and adaptation strategies associated with these phenomena. The urban environment, with its built infrastructure, green spaces, and diverse human activities, offers various ecosystem services that enhance the wellbeing and resilience of urban dwellers. Urban ecosystems offer regulatory services like temperature control, air quality upkeep, and stormwater management, plus provisioning like food and water. They also provide cultural benefits, promoting recreation and community unity. However, climate change poses significant challenges to urban ecosystem services. Rising temperatures, altered precipitation patterns, and increased frequency of extreme weather events can disrupt the functioning of urban ecosystems, impacting the provision of services. Heatwaves and urban heat island effects can compromise human health and energy demands, while changes in rainfall patterns can strain stormwater management systems and lead to flooding. Moreover, climate change can disrupt biodiversity and ecological processes, affecting the overall resilience and sustainability of urban ecosystems. To address these challenges, cities are adopting various adaptation strategies that recognize the interdependence between urban ecosystems and climate change. Green infrastructure interventions, such as the creation of urban parks, green roofs, and community gardens, aim to mitigate the impacts of climate change by enhancing the regulation of temperature, improving air quality, and reducing stormwater runoff. Additionally, urban planning and design approaches prioritize compact and walkable neighborhoods, promoting public transportation and reducing reliance on fossil fuels. Furthermore, engaging communities in the management of urban ecosystems and climate change adaptation measures is crucial for ensuring equitable distribution of ecosystem services and building social resilience. Therefore, the review article highlights a comprehensive understanding of the dynamic interrelationship between urban ecosystem services and climate change and their implications. By recognizing and integrating the contributions of urban ecosystems, cities can develop sustainable and resilient strategies to mitigate and adapt to climate change, ensuring the wellbeing and habitability of urban environments for present and future generations.
1. Introduction
Currently, 56% (4.4 billion) world population reside in urban areas, projected to reach nearly seven out of 10 by 2050 (World Bank, 2023). Urbanization's potential for sustainable growth lies in cities contributing over 80% of global GDP, fostering productivity and innovation when managed effectively (Zhang, 2016). In such an era marked by rapid urbanization and escalating climate change, the intricate relationship between urban ecosystems and the changing climate has come to the forefront of global environmental discourse. Cities, as vibrant hubs of human activity and economic development, rely heavily on the services provided by their surrounding ecosystems to sustain and enhance human wellbeing. These services, known as urban ecosystem services, encompass a wide range of benefits, including clean air and water, climate regulation, food production, cultural and recreational opportunities, and support for biodiversity. By adopting the ecosystem services approach, researchers, advocates, and policymakers can effectively showcase the advantages that urban trees and other elements of ecological infrastructure provide to human societies. This framework allows for a comprehensive evaluation of the positive impacts, such as improved wellbeing, enhanced environmental quality, and social values, while also considering potential drawbacks and costs (Salmond et al., 2016). By precisely identifying, measuring, and modeling these benefits, informed decisions can be made, priorities can be set, and resources can be allocated efficiently for the planning and management of urban green infrastructure.
In mainstream concept, ecosystem services have been merely divided into four categories according to the classification offered by the Millennium Ecosystem Assessment (2005) regulatory, provisioning, cultural, and supporting services. Several researchers have proposed more detailed classifications of ecosystem services specifically tailored to urban environments. In a pioneering study by Bolund and Hunhammar (1999), they introduced a straightforward categorization of ecosystem services that are distinct to urban ecosystems and environments. Their research emphasized the numerous benefits that urban green infrastructure offers to human health. These benefits include micro-climatic control, air purification, acoustic attenuation, rainwater harvesting, effluent treatment, and aesthetic values. However, the delicate balance between these urban ecosystem services is being disrupted by the far-reaching impacts of climate change. Rising temperatures, altered precipitation patterns, sea-level rise, and more frequent extreme weather events pose significant challenges to the functioning and resilience of urban ecosystems. Moreover, there have been noticeable changes over time including the rapid reduction of ozone in the stratosphere, the expansion of desert areas, the loss of forests, excessive deposition of nitrogen in the atmosphere, a reduction in freshwater resources, shifts in land use patterns, and biotic interchanges (Thuiller, 2007).
The review highlights the aspects involving two Sustainable Development Goal, i.e., 13 and 15. The interaction between Sustainable Development Goal 13 (SDG 13), which focuses on climate action, and Sustainable Development Goal 15 (SDG 15), which emphasizes life on land, is integral to addressing the complex challenges of environmental sustainability which is being emphasized in this review. Climate change, a central concern of SDG 13, directly impacts terrestrial ecosystems and biodiversity, as highlighted in SDG 15. Mitigating greenhouse gas emissions and adapting to climate change (SDG 13) can enhance the resilience of land-based ecosystems and contribute to the prevention of land degradation, deforestation, and desertification (SDG 15). Conversely, the conservation and sustainable management of forests, wetlands, and other land resources (SDG 15) play a critical role in sequestering carbon and mitigating climate change, aligning with the goals of SDG 13. This interconnectedness underscores the importance of a holistic approach that recognizes the symbiotic relationship between climate action and the preservation of terrestrial ecosystems to achieve sustainable development.
The interplay between urban ecosystem services and climate change is a complex and dynamic relationship (Figure 1). On one hand, climate change poses threats to the provision of ecosystem services, making cities more vulnerable to environmental risks and undermining their capacity to support thriving and sustainable communities (Frumkin et al., 2008). On the other hand, urban ecosystems have the potential to act as critical tools for climate change mitigation and adaptation, contributing to efforts to reduce greenhouse gas emissions, enhance urban resilience, and promote sustainability. For example, Urban areas possess a dual role in relation to greenhouse gas (GHG) emissions, serving as both a significant source and a potential reservoir of carbon (Strohbach et al., 2012; Schröder et al., 2013). In urban environments, the source-sink feedback system functions by cities releasing greenhouse gases through activities such as transportation and energy consumption (acting as a source) and simultaneously offering potential for carbon sequestration through urban green spaces and infrastructure (functioning as a sink). Furthermore, urban soils, containing 3–5 times more carbon than natural soils in various climates, have the potential to sequester significant carbon, although the effectiveness of this sequestration remains debated due to the loss of a substantial portion of new carbon inputs through soil respiration (Upadhyay et al., 2021). Consequently, it is crucial to include cities in global initiatives aimed at mitigating and adapting to climate change (Bulkeley and Betsill, 2013; Masson et al., 2014). Urban forests, consisting of trees and shrubs within urban environments, offer valuable services for climate regulation by sequestering and storing carbon (Nowak and Crane, 2002; Strohbach and Haase, 2012; Brandt et al., 2016; Upadhyay et al., 2021). Considering their capacity to capture and retain atmospheric carbon, urban forests present a valuable tool for climate change mitigation on various scales. For instance, at micro level, it can influence the microclimatic conditions of the urban buildings and structures by providing shade, lessen up the energy consumption, and subsequently lowering carbon emissions. At meso level or at the city level, urban forests have the potential to influence solar radiation reaching the ground surface, the atmospheric humidity, and other local climatic variables (Gill et al., 2007; Redon et al., 2020). On the other hand, at macro level or at a global scale, it can serve as magnificent carbon sink (Jo and McPherson, 2001; Chen, 2015). Therefore, understanding and harnessing the interconnections between urban ecosystems and climate change is crucial for creating more sustainable and resilient cities. Nevertheless, these forests are under threat from the impacts of climate change and air pollution. The decline of forests has primarily been observed in regions spanning from 27° to 56°N latitude and 103° to 145°E longitude, encompassing subtropical, temperate, and cool temperate climates. The most significant decline has been noted in Western Japan and Korea. Across Asia, this decline can largely be attributed to localized emission sources, including traffic, thermal power plants, electric power plants, other industrial activities, and commercial areas (Sonwani et al., 2022). This review delves into the intricate dynamics between urban ecosystem services and climate change, exploring the impacts of climate change on urban ecosystems and the reciprocal role of urban ecosystems in mitigating and adapting to climate change. It examines the various components of urban ecosystem services and their vulnerability to climate change, while also highlighting the potential for urban ecosystems to contribute to climate change mitigation and adaptation efforts. This review also underscores the significance of the interplay between two pivotal Sustainable Development Goals, namely SDG 13, which centers on climate action, and SDG 15, with its focus on terrestrial ecosystems and biodiversity. This interaction is pivotal in tackling the intricate issues associated with environmental sustainability, a central theme emphasized throughout the review.
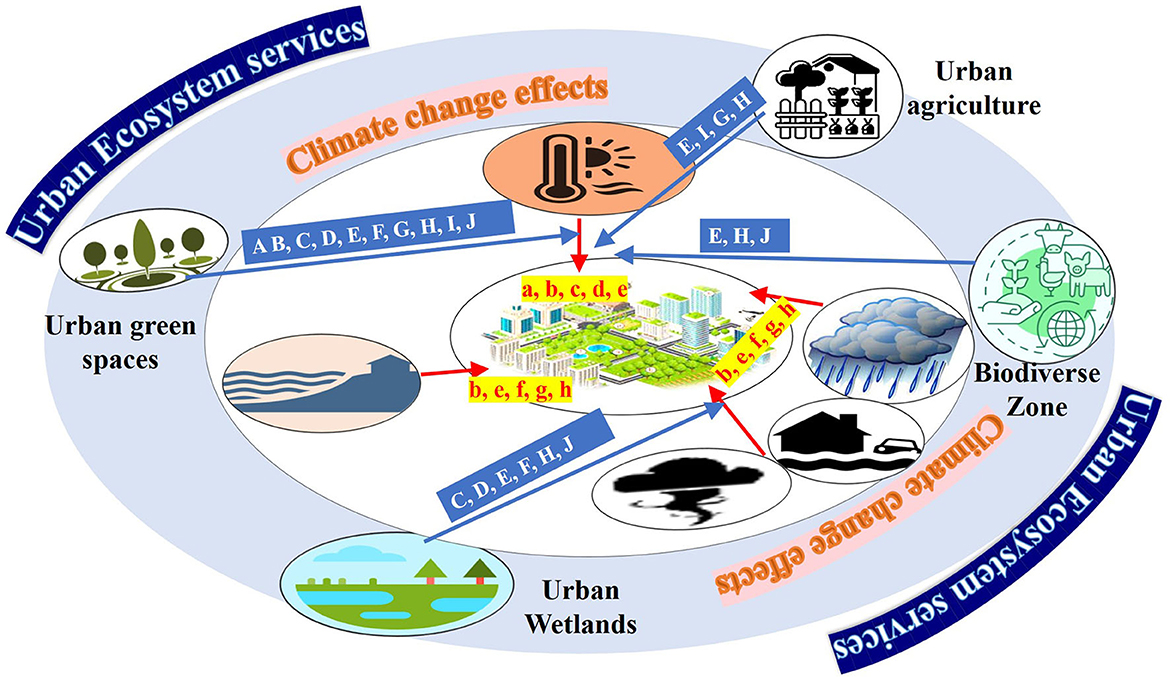
Figure 1. An illustrative portrayal showcasing the dynamic interplay between urban ecosystem services and climate change which serves as a powerful tool for communicating intricate connections. Within this representation, blue arrows signify the ways in which urban ecosystem services act as a buffer, alleviating the effects of climate change. Conversely, red arrows depict how climate change acts as a stressor, imposing strains on the delicate balance of the urban ecosystem. A, temperature regulation; B, air quality control; C, stormwater management/flood water absorption; D, flood mitigation; E, biodiversity support; F, carbon sequestration; G, pollination services; H, cultural services; I, food security; J, climate resilience; a, urban heat island/global warming; b, loss of cultural services; c, air pollution; d, biodiversity loss; e, less carbon sequestration; f, flooding; g, affected pollination; h, food insecurity.
Thus, by examining the current knowledge and emerging trends in the field, this review seeks to shed light on the complex and nuanced relationship between urban ecosystems and climate change. It aims to inform policymakers, urban planners, researchers, and other stakeholders about the importance of recognizing and valuing urban ecosystem services in the context of climate change, while also emphasizing the need for integrated and sustainable approaches that can maximize the benefits of urban ecosystems and enhance the resilience of cities in a rapidly changing world.
2. Material and methodology
The selection procedure for scientific papers for a review article followed a systematic and rigorous approach to ensure the quality and relevance of the included literature. Literature published between 2000 and 2023 in relevance to the specified sub-topics focusing on urban ecosystem services, climate change impacts on urban areas, and nature-based solutions for climate change adaptation has been the defined inclusion criteria. The following standards were used to select, appraise, and eliminate articles: (1) presenting findings from an original scientific study or a review and summary of reports of such studies, (2) determining ecosystem services under supporting, provisioning, cultural and regulating services category (3) representing studies urban ecology and global climate change. A comprehensive literature search was conducted using academic databases, such as PubMed, Google Scholar, Scopus, or Web of Science. Use a combination of keywords related to the sub-topics, such as “urban ecosystem services,” “urban structures” “climate change adaptation,” “synergies and trade-offs,” “urban development,” “policy and planning,” and “challenges.” Those papers that do not meet the inclusion criteria or are not directly related to the specified sub-topics has been excluded.
3. Navigating climate change effects on ecosystem services and sustainability
Urban Ecosystem Services encompass a wide array of services directly or indirectly influencing human wellbeing and urban functionality. As climate change accelerates, urban areas confront escalating pressures on their ecosystems and the indispensable services they provide (Weiskopf et al., 2020). For instance, in coastal cities, rising sea levels pose threats to wetland ecosystems (Plane et al., 2019) that offer flood protection, carbon sequestration (Speak et al., 2020), and habitat for biodiversity (Williams and Newbold, 2020). Urban heat islands intensify due to extreme temperatures, impacting green spaces and compromising their capacity for cooling, air quality improvement, and carbon dioxide absorption (Moser et al., 2017; Moser-Reischl et al., 2018). Changes in precipitation patterns disrupt urban water systems, affecting water availability and stormwater management, jeopardizing the crucial role of urban green infrastructure in mitigating floods and enhancing water quality (Park et al., 2010; Nelson et al., 2013; Molina-Navarro et al., 2014; Wang et al., 2017). Moreover, urbanization has triggered significant ecological and societal transformations, underscoring the need to understand the diverse benefits provided by urban ecosystems and effect of climate change on ecosystem services (Bai et al., 2017; Wang et al., 2021).
3.1. Provisioning services in urban areas
Provisioning services in urban areas refer to the tangible goods obtained from urban ecosystems, such as food, water, and raw materials (Rodríguez et al., 2006; Kettunen and D'Amato, 2013). Urban provisioning ecosystem services encompass a wide range of benefits in urban areas which is being listed in Table 1. While cities may not seem like primary providers of these services, urban agriculture, green infrastructure, and water management play vital roles in meeting the demands of their inhabitants (Mell, 2009; Cilliers et al., 2013; Artmann and Sartison, 2018).
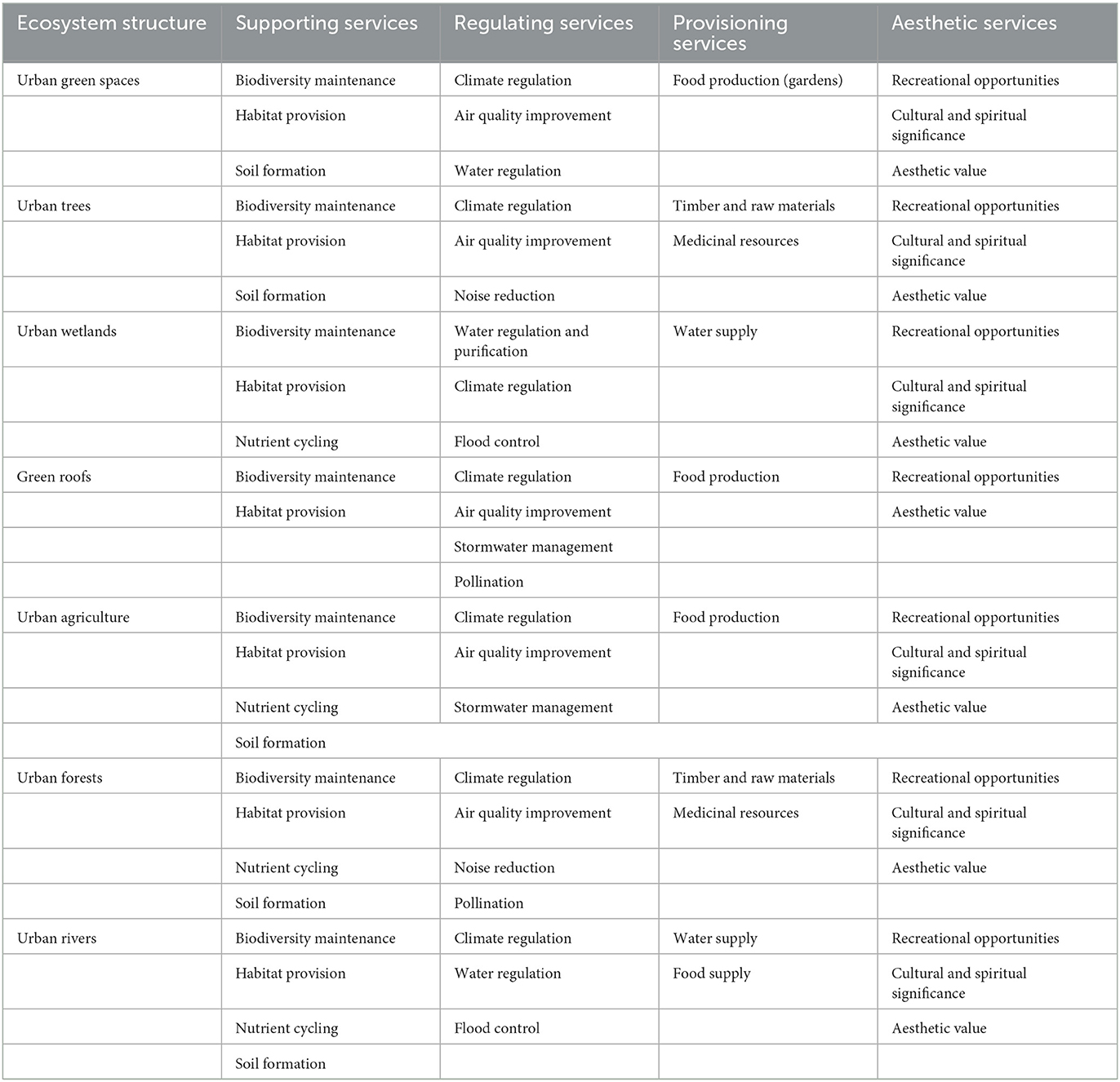
Table 1. A general overview of the ecosystem services associated with different urban ecosystem structures.
3.1.1. Food production
Climate change significantly impacts urban provisioning services, including food production, distribution, and access. Altered temperature and precipitation patterns influence urban vegetation yields, leading to heat stress and reduced productivity (Cârlan et al., 2020; Kabisch et al., 2021). This stress affects fish and aquatic plants in urban ponds, impacting aquaculture yields and water-related food production (Hill et al., 2017; Pagliaro and Knouft, 2020; Krivtsov et al., 2022). Changes in precipitation cause drought or excessive rainfall, affecting urban and peri-urban food production and water resources (Forkuor and Cofie, 2011; Sabiiti et al., 2014). Water scarcity can lower peri-urban agricultural productivity, potentially causing food shortages and higher prices (Wolf et al., 2003). Extreme weather events, like droughts, floods, and heatwaves, stress urban trees, hinder nutrient absorption, and damage roots, leading to lower yields (Czaja et al., 2020; Haase and Hellwig, 2022). Higher temperatures deplete food nutrients, reducing their nutritional value, while floods and storms cause spoilage and food waste (Wagstaff, 2016; Sehgal et al., 2018; Blekking et al., 2022). Disruptions in supply chains and transportation lead to food losses, impacting urban populations. Climate-induced changes increase food prices, affecting affordability, particularly for low-income households. Farmers may adapt to alternative crops, influencing food choices in urban areas (Blekking et al., 2022). Furthermore, the susceptibility of crops to environmental stress induced by the emission of greenhouse gases is a matter of significant concern, as highlighted by Uprety and Saxena (2021). Climate change is making its presence felt in India with noticeable shifts like shorter winters and an earlier onset of summer than usual. This change is particularly significant due to India's proximity to the equator. Additionally, the delayed sowing of wheat, caused by late rice harvesting, places the wheat crop at risk of high-temperature stress during the crucial grain-filling stage (Ghosh et al., 2020), ultimately resulting in terminal heat stress and reduced crop yields. According to simulations conducted by Dubey et al. (2020), based on field experimental trials (2011–2012 and 2012–2013) the projected outcome for the year 2050 indicates that this terminal heat stress is expected to lead to an 11.1% reduction in wheat yield. Furthermore, another Indian researcher Guntukula (2020) uses annual time-series data from 1961 to 2017 to examine how rainfall, maximum, and minimum temperatures affect crop yields of rice, wheat, pulses, sugarcane, and groundnut in India. The study revealed that a spike in rainfall could negatively affected food crops except pulses, whereas the average maximum temperature helps food and non-food crops except rice. Furthermore, food crops tend to benefit from average minimum temperature.
Climate change poses diverse and interconnected challenges to urban livestock and dairy (Beneberu and Wondifraw, 2020; Chari and Ngcamu, 2022). Rising temperatures induce heat stress (Polsky and von Keyserlingk, 2017), reducing productivity (Babinszky et al., 2011), impairing reproduction (Boni, 2019), and increasing mortality in animals (Crescio et al., 2010), affecting milk quality (de Felicio Porcionato et al., 2009). Altered precipitation patterns limit water availability, impacting hydration, sanitation, and causing waterborne diseases. Climate shifts influence feed crop growth, affecting nutrition and animal health, and alter disease and pest distribution, requiring additional resources (Bett et al., 2017; Murray-Tortarolo and Jaramillo, 2019, 2020).
3.1.2. Timber quality
Climate change also significantly impacts urban timber provision services (Sevianu et al., 2021). Changes in temperature, precipitation patterns, and extreme weather events can have profound effects on the quality and quantity of timber resources within urban ecosystems. Higher temperatures and prolonged heatwaves can stress urban trees, altering their growth, wood quality, and suitability for construction (Depietri et al., 2012; Esperon-Rodriguez et al., 2021; Marchin et al., 2022). Increased temperatures can also impact timber's chemical composition, affecting durability and resistance to decay, pests, and fungi (Ayanleye et al., 2022). Changes in precipitation patterns influence urban tree growth, with droughts reducing timber availability due to water stress (Savi et al., 2015) and excessive rainfall lowering yields by affecting root health and nutrient uptake (Sieghardt et al., 2005; Brune, 2016). Additionally, changes in the distribution of timber-yielding tree species have been documented as climate change induced alteration. A recent study by Patasaraiya et al. (2023) investigated how climate change is affecting the distribution of important timber-yielding tree species in India, particularly Sal and Teak, which were identified as crucial species by Kaul et al. (2010). The study focused on central India and used high-resolution modeling for two future time periods (2050 and 2070) under two climate change scenarios (RCP 2.6 and 8.5). The study's findings indicated that there will likely be an expansion of suitable habitat for teak in the future, while the habitat for sal is expected to decrease. Temperature was found to be the primary influencing factor for teak, whereas precipitation played a critical role in the distribution of sal. Climate change also affects pest and disease prevalence, favoring certain pests and leading to timber losses (Volney and Fleming, 2000; Holmes et al., 2009). Extreme weather events like storms and hurricanes damage urban forests, causing tree mortality and limiting timber availability (Escobedo et al., 2009; Landry et al., 2021).
3.1.3. Water quality and replenishing groundwater
Soil compaction from human activities hinders water infiltration, increasing stormwater volume and flood risks. Urban forests influence watershed hydrology by intercepting, evapotranspiring, and storing rainfall (Oke, 1989; Wang et al., 2008; Berland et al., 2017). Climate change exerts selective pressures on urban tree species, leading to shifts in their distribution and abundance. Some species exhibit enhanced resilience, while others struggle to adapt, impacting their capacity for rainfall interception and water retention (Jones et al., 2020). Climate-induced shifts in rainfall patterns challenge urban trees' ability to intercept and store intense rainfall events, leading to increased runoff and reduced water infiltration (Riedel and Weber, 2020; Hendricks and Dowtin, 2023). Drought stress associated with climate change decreases canopy cover and evapotranspiration, limiting the capacity of urban trees to intercept and store rainfall (Baptista et al., 2018; Carlyle-Moses et al., 2020). Extreme rainfall overwhelms canopy interception capacity, saturates soil, and leads to increased runoff (Qin, 2020). Soil erosion and compaction compromise tree root systems, hindering water uptake. Warmer temperatures from climate change elevate evapotranspiration rates, impacting groundwater recharge and soil moisture maintenance (Chan et al., 2019). Additionally, climate-induced pest and pathogen activity reduce canopy interception and transpiration, while altered tree-microorganism interactions compromise tree defenses (O'Neil-Dunne et al., 2014). Climate change-induced disruptions exacerbate urban tree illnesses, affecting their capacity to manage rainfall water.
3.2. Regulating services for urban resilience
Regulating services, integral to urban resilience, encompass imperceptible yet crucial functions like maintaining the quality of air and soil, providing flood and disease control, and plant pollination (Haase et al., 2014; McPhearson et al., 2014) (Table 1). Despite their inconspicuous nature, detrimental perturbations of ecosystem regulating services can lead to substantial, intricate losses that pose challenges in restoration (Baró and Gómez-Baggethun, 2017; Palliwoda and Priess, 2021; Morris et al., 2022).
3.2.1. Air quality
Reducing air pollution poses a critical issue in numerous major urban centers across various income-level nations. Scientific studies (Jalaludin et al., 2004; Karagulian et al., 2015) consistently reinforce the detrimental effects of ambient air pollution on human health. In the latest Global Burden of Diseases Study, air pollution ranked as the fourth primary contributor to premature mortality worldwide in 2019, accounting for 6.67 million untimely deaths (Murray et al., 2020). The urban air quality of many cities is jeopardized by local emissions from transportation, industries, and other origins, while climate change also significantly influences these conditions. This interaction not only modifies local microclimates and influences building energy consumption but also involves the release of pollen, impacting allergies (D'Amato, 2011), and volatile organic compounds (VOCs), which contribute to the formation of O3 and PM2.5 (Wakamatsu et al., 2017; Pandey and Ghosh, 2022).
Besides, acting as a sink to various air pollutants, urban vegetation emits biogenic volatile compounds (BVOC), contributing to ground-level ozone (O3) and carbon monoxide formation (Davoren and Shackleton, 2021) and such problem could be exacerbated under present scenario of climate change (Ghosh et al., 2018). Conversely, research suggests that trees, especially low volatile organic compound (VOCs) emitters, can effectively decrease urban O3 levels (Taha, 1996; Nowak et al., 2000; Ghosh et al., 2018; Sicard et al., 2018; Fitzky et al., 2019). Ground-level ozone concentrations can surge significantly due to the oxidation of isoprene (BVOC) by NOx, as noted by Lerdau et al. (1997). The presence of substantial NOx, stemming largely from automobile exhaust within urban locales, contributes to elevated urban O3 levels when combined with VOCs emitted by nearby vegetation. The findings of Saxena and Ghosh (2011) unveiled those locales in Delhi, characterized by dense vegetation and significant vehicular emissions exhibited the most pronounced O3 formation which was linked to the release of precursor gases (NOx and VOCs) responsible for ozone production, emanating from traffic sources, as well as VOCs discharged by vegetation like isoprene and terpenes.
In addition to the inherent VOCs emission capacity of trees, the atmospheric conditions of a given region play a pivotal role in O3 formation. This phenomenon is particularly notable in Mediterranean and tropical countries, as explored by Calfapietra et al. (2013). Consequently, a meticulous approach to urban plantation strategies is imperative to manage the burden of VOCs emissions. The selection of high VOC-emitting species for urban environments could exacerbate VOCs emissions and, by extension, O3 production—a concern, especially in light of the already elevated global O3 levels. Moreover, the situation could worsen due to global warming, as high temperatures and extended periods of sunshine tend to promote the formation of O3.
3.2.2. Climate regulation
United Nations (2018) attributes 60% of anthropogenic greenhouse gas emissions to urban activities. Urban vegetation, particularly trees, directly counteract greenhouse gas emissions by sequestering carbon dioxide through photosynthesis and biomass storage (Nowak et al., 2013a; Agbelade and Onyekwelu, 2020; Klein et al., 2021). Most of the mentioned studies focus solely on CO2 flux from urban trees and vegetation, neglecting soil contribution. Urban soils, particularly those rich in organic matter (histosols or peat soils), can serve as substantial carbon sinks (Malone et al., 2023). However, shifting climate conditions might influence the growth and survival of trees and plants. Rising temperatures can dehydrate and damaged roots, thus reducing their ability to absorb water, affecting soil microbial communities and impacting nutrient uptake by plants (Pandey et al., 2019). Climate change can pose a threat to the availability of nutrients (Lotze-Campen, 2011; Elbasiouny et al., 2022). Nonetheless, the process of global warming has the potential to alter the yearly and seasonal patterns of nutrient availability and cycling (Koller and Phoenix, 2017). Specifically, changes in the availability of carbon (C), nitrogen (N), and phosphorus (P) can have significant consequences for plant life, as these nutrients are vital for proper plant growth and development. Insufficient nutrient availability can lead to stunted growth of the urban trees, their instability, and vulnerability to other stressors. Thus, elevated temperature due to climate change, diminished the capacity of the urban green spaces to counteract local warming. Higher temperatures could potentially undermine the cooling effect provided by vegetation, reducing their ability to create cooler microclimates within urban areas.
Additionally, extreme weather events such as flooding, prolonged droughts can compromise the health of urban vegetation, reducing its carbon sequestration potential. These events can even cause soil erosion and disrupt the carbon stored in the soil. Warmer temperatures might accelerate microbial activity in the soil, potentially leading to increased decomposition of organic matter (Elbasiouny et al., 2022; Shahzad et al., 2022). This could lead to an accelerated release of carbon into the atmosphere in the form of carbon dioxide (Dotaniya et al., 2016), contributing to a positive feedback loop that amplifies the effects of climate change. Additionally, changes in soil moisture levels can affect the balance between carbon uptake and release, potentially leading to reduced carbon sequestration. However, soil respiration can also emit carbon (Velasco et al., 2016; Upadhyay and Raghubanshi, 2020), adding complexity to urban carbon budget assessments under ongoing climate change scenario.
3.2.3. Pollination and flowering
Pollination, typically classified as a regulating service (Smith et al., 2011; Crossman et al., 2013), occurs through abiotic (primarily wind) or biotic (pollinating animals) means (Ollerton et al., 2011). Flowering plants, as complex biological entities, intricately intertwine with the survival and reproductive success of a myriad of pollinators, comprising bees, butterflies, and a plethora of other insect species (Dorin et al., 2022). The symbiotic relationship between these plants and pollinators is an elemental cornerstone of ecosystem functionality (Stevenson et al., 2020). Through this dynamic interaction, they inadvertently transfer pollen grains, laden with genetic material, from one flower to another, orchestrating the fertilization process vital for plant reproduction (Carper et al., 2022). The consequences of this mutualistic alliance extend far beyond the realm of botany, as numerous plant species integral to human sustenance and wellbeing heavily depend on successful pollination events (Oliveira et al., 2019).
Amid the various facets of climate change, one aspect that stands out is the impact of heat stress. This stress not only affects pollination services, foraging behavior, and the overall growth of pollinators but also triggers observable changes in these areas (Zhao et al., 2021). These negative effects are projected to be even more pronounced as climate change progresses, with significant shifts expected in tropical, cold, and mountainous regions, as previously noted by Medina et al. (2018), Alqarni (2020), and Soroye et al. (2020). The primary driving force behind these changes is the heightened frequency and duration of heat waves, which have become a prominent characteristic of climate change (Xu et al., 2018). Of particular concern is the impact on tropical regions, which are already accustomed to elevated temperatures. With the anticipated temperature increases brought about by climate change, these tropical regions are likely to experience a disproportionately negative impact compared to temperate areas (Mora et al., 2013). The rising temperatures have far-reaching consequences on the reproductive processes of plants, potentially leading to decreased fertility in numerous species and subsequent effects on pollination. The repercussions of heat stress extend to changes in pollen quantity, morphology, cell wall structure, and notably, pollen metabolism (Hedhly, 2011; Chaturvedi et al., 2021).
Climate change has sparked significant interest due to its considerable impact on pollination. Two key effects have garnered attention: (a) disruptions in the timing of plant-pollinator interactions, known as phenological decoupling, and (b) shifts in the geographical distribution of these interactions, referred to as spatial decoupling (Høye et al., 2013; Herrera et al., 2014; Sirois-Delisle and Kerr, 2018). The influence of heat on this delicate balance is noteworthy, as it can lead to reduced pollen viability and alter the timing of flower initiation and opening, ultimately impacting successful pollination. When flowering times shift while pollinator schedules remain unchanged, mismatches between flowers and pollinators arise. This can instigate phenological decoupling in plant-pollinator interactions, with temperature changes causing distinct shifts in developmental stages among plants (Settele et al., 2016). Notably, some butterflies have exhibited 3-fold faster spring advancement in phenology compared to their host plants, highlighting an increasing asynchrony between these two groups (Settele et al., 2016). Such phenological mismatches can have cascading effects. They may lead to diminished food availability for insect pollinators while also reducing pollinator visits to the very plants they serve. This misalignment further disrupts pollen transfer, resulting in decreased fertilization rates and reproductive success. These repercussions, in turn, reverberate through plant fitness and offspring viability. The cumulative impact of heat-induced disruptions during flowering and fruiting can impede genetic diversity and jeopardize population persistence. Additionally, evidence points to the ongoing shift in the spatial distribution of insect pollinators because of climate change. This phenomenon is projected to persist, particularly affecting bumblebees and butterflies (Settele et al., 2008; Rasmont et al., 2015). Heat stress further amplifies these challenges by diminishing the production of secondary metabolites like phenolic compounds, which act as natural defenses against pests and pathogens. While heat-induced stomatal closure limits water loss, it also reduces the emission of volatile organic compounds (VOCs) that contribute to pest deterrence. The alteration in VOC composition under heat stress modifies the pattern of attraction or repulsion of pests, adding another layer of complexity to the evolving dynamics of plant-insect interactions.
3.3. Supporting services for urban ecosystem functioning
Supporting services provided by urban ecosystem services are the invisible but essential foundation that sustains the functionality and health of cities (Table 1). These services encompass processes like nutrient cycling, soil formation, and biodiversity maintenance, working behind the scenes to ensure the vitality of urban environments. They contribute to the productivity of urban green spaces, facilitate water purification, and foster habitat for diverse species. As urban areas grapple with the challenges posed by rapid development and climate change, recognizing and nurturing these supporting services becomes paramount. Climate change has exerted noticeable impacts on urban supporting ecosystem services, altering the foundational processes that sustain urban environments. Rising temperatures and altered precipitation patterns influence soil health and composition, affecting nutrient cycling and soil fertility (Scholes, 2016; Weiskopf et al., 2020; Bakure et al., 2022). Rising temperatures lead to heightened soil organic matter decomposition, potentially elevating soil carbon losses and causing shifts in C:N balances (Davidson and Janssens, 2006). These alterations are influenced by various biotic interactions, including secondary changes to the composition of soil microbial communities (Crowther et al., 2011). Concurrently, human activities linked to climate change, such as the combustion of fossil fuels, lead to heightened nitrogen deposition. This deposition carries significant consequences for both terrestrial and aquatic ecosystems, notably contributing to issues like eutrophication (Galloway et al., 2008). The urban environment, in particular, contends with heightened vulnerabilities to more intense and erratic rainfall, leading to elevated risks of flooding and erosion that directly impact water regulation and purification services. The essential role of biodiversity in enhancing ecosystem resilience faces jeopardy, with many species struggling to acclimate to the evolving environmental conditions. Notably, recent research by Habibullah et al. (2022) underscores that the impact of biodiversity loss is notably influenced by shifts in precipitation and temperature, surpassing even the effects of alterations in the frequency of natural disaster events. Urban heat islands, exacerbated by climate change, strain energy systems and human health while also disrupting natural processes. The interplay of these factors has led to a decline in the capacity of urban ecosystems to provide essential supporting services, challenging the ability of cities to maintain their functionality and the wellbeing of their inhabitants. Adaptation and mitigation strategies are crucial to restore and enhance these services, ensuring the resilience and sustainability of urban areas in the face of a changing climate.
3.4. Cultural services and human wellbeing
Cultural ecosystem services can be defined as the non-material benefits that people obtain from nature, including spiritual, aesthetical, educational and recreational values (Schaich et al., 2010; Plieninger et al., 2015; Ament et al., 2017). Parks, green spaces, and vibrant urban landscapes offer places of relaxation, leisure, and social interaction, enhancing the overall quality of life in cities (Gotham and Brumley, 2002; Kazmierczak, 2013; Tsunetsugu et al., 2013; Cox et al., 2017; Verma et al., 2020). These spaces serve as venues for cultural events, festivals, and artistic expressions, fostering community identity and connection (Ulrich, 1981; Kaplan, 1985; Van den Berg et al., 2010; Russell et al., 2013). Additionally, urban ecosystems offer settings for education and learning, enabling people, especially children, to connect with nature and gain insights into ecological processes (Tidball and Krasny, 2011).
Climate change is affecting some of the critical services that ecosystems provide to society (Lipton et al., 2018). The cultural ecosystem in terms of recreation and tourism provides valuable services which play a crucial role in generating substantial economic benefits (Reyers et al., 2013). Most recreational services exhibit a high degree of vulnerability to the impacts of global warming, with a particular emphasis on outdoor winter sports and tropical holidays (Khan et al., 2013). Many urban cultural events and festivals take place in outdoor settings, drawing residents and tourists together to celebrate diversity and community spirit. However, climate change can disrupt these events by increasing the risk of extreme weather events such as storms, heavy rainfall, or heatwaves. These weather patterns could lead to cancellations, rescheduling, or diminished attendance, impacting the vibrancy and social cohesion associated with such gatherings. In a recent investigation conducted by Dhavale et al. (2022), findings reveal a notable 52% surge in cyclone occurrences within the Arabian Sea from 2001 to 2019. Moreover, the study reports a staggering 150% increase in the incidence of “very severe” cyclones during the same period. These climatic shifts have consequently impacted coastal tourism in states like Goa and Kerala.
The global phenomenon of climate change has been observed to have a significant impact on these cultural ecosystem services on a worldwide scale (Tuvendal and Elmqvist, 2011). Climate change can alter the availability and functionality of the green spaces. Increased temperatures, heatwaves, and shifting precipitation patterns, rising air pollution might limit the comfortable use of outdoor areas, reducing people's ability to engage in outdoor activities like picnicking, jogging, or sports (Evans, 2019). Moreover, droughts and water scarcity can negatively impact the vitality of green spaces, affecting the visual appeal and recreational value of these areas. These effects are not limited to specific regions, but rather have implications that extend beyond geographical boundaries. Debnath et al. (2023) conducted a recent study highlighting the vulnerability of the entire Delhi region, India, to severe heat wave impacts. Surprisingly, the current state action plan for climate change does not acknowledge this vulnerability. Through an analytical assessment of the Heat Index (HI) combined with the Climate Vulnerability Index (CVI), the study reveals that over 90% of the country faces an alarmingly high risk level, with potential adverse effects on livelihood adaptability, food grain yields, the spread of vector-borne diseases, and urban sustainability. These conditions not only have the potential to deter tourists but also pose health risks, including heat-related illnesses. Furthermore, urban ecosystems often historical sites, monuments, and culturally significant landmarks that connect residents to their heritage and identity could be affected by climate change-related factors which can damage or threaten these sites, potentially erasing important aspects of a city's cultural history and identity (Quesada-Ganuza et al., 2021).
4. Enhancing urban ecosystem services for climate change adaptation
4.1. Green infrastructure and ecological design
Green infrastructure and ecological design play crucial roles in climate change adaptation by promoting sustainable and resilient solutions. They encompass a range of practices and systems that harness the power of nature to mitigate the impacts of climate change and enhance ecosystem services. Green infrastructure refers to strategically planned and managed networks of natural and semi-natural areas, as well as green spaces within urban environments (Bendict and McMahon, 2006; Wright, 2011; Matthews et al., 2015). It includes features such as urban forests, wetlands, green roofs, green buildings, green walls, permeable pavements, and bioswales, vegetated surfaces green streets and alleys, community parks and gardens (Gill et al., 2007; Douglas, 2010; Klemm et al., 2015). These elements work together to provide multiple benefits, including stormwater management, heat island reduction, lowering of wind speed, reduction in energy consumption, air quality improvement, biodiversity conservation, carbon sequestration and maintenance of wellbeing of the urban societies, nevertheless, the full measure of these advantages is still up for debate (Pataki et al., 2011). Table 2 presents a compilation of noteworthy case studies covering these diverse ecosystem structures spanning the years 2013 to 2023.
In the context of climate change adaptation, green infrastructure helps cities and communities cope with the challenges posed by changing climate patterns. By capturing and storing rainfall, green infrastructure reduces the risk of flooding and enhances water resource management. It also helps mitigate urban heat islands by providing shade, evaporative cooling, and natural ventilation, thereby reducing energy consumption for cooling purposes. For example, the city of Portland, located in the northwestern United States, serves as an illustrative case of how adopting a green infrastructure design approach for managing flood risks can yield a wide range of benefits for the local community, both at the site and neighborhood level. In response to significant strain on Portland's drainage system, leading to around 50 combined wastewater overflows into the Willamette River in 1990, Hoyer et al. (2011) emphasize the proactivmeasures taken by the city's municipal government. They implemented a diverse range of green infrastructure initiatives to alleviate the burden on the sewer system and minimize adverse impacts on urban waterways. These measures include providing monetary incentives for disconnecting downpipes, redirecting stormwater to lawns, gardens, and ground infiltration, constructing green roofs to boost local biodiversity, and establishing a network of green spaces for recreational purposes, which also helps mitigate rainwater runoff into the Willamette. By implementing such programs, there is an increase in local biodiversity, as well as an enhancement in the attractiveness of the streetscape (Hoyer et al., 2011). In a similar vein, Lancaster (2006) analyze the comprehensive and community-based drainage programs in Vancouver, Canada. The municipal government has actively encouraged the Green Streets program, which enables residents to engage in city gardening through the funding of projects aimed at improving roadside areas (Lancaster, 2006).
Ecological design focuses on integrating ecological principles and processes into the planning and design of human-built environments. It seeks to create sustainable and regenerative systems that harmonize with nature. Ecological design approaches consider the ecological, social, and economic aspects of a project to foster resilience and adaptability. In the face of climate change, ecological design emphasizes strategies such as using native plant species, restoring and enhancing natural habitats, promoting biodiversity, and conserving natural resources. As an illustration, the plans for Ho Chi Minh City in Vietnam encompass various strategies such as reservoir management, constructing a ring dike, and preserving the riparian area, all with the primary objective of flood prevention. One potential advantage of safeguarding the riparian zone is the preservation of habitat integrity and species diversity, as demonstrated in Lagos, Nigeria. Furthermore, the Ho Chi Minh City plan includes a target to reduce river pollution, which presents additional opportunities for biodiversity benefits (Butt et al., 2018). Chennai and Kochi are showing initial progress in embarking on a journey toward urban climate transition. These Indian cities actively participated in normative adaptation integration, leveraging a diverse array of actions and projects. They prioritized the restoration and enhancement of Blue-green infrastructures as a foundational strategy to bolster urban resilience in the face of climate change challenges. Notably, international cooperation organizations played a pivotal role in assisting the municipalities in these case studies, particularly in the revitalization of the Buckingham Canal in Chennai and the Mullassery Canal in Kochi, marking a significant milestone in the evolution of a novel regulatory framework (Sánchez and Govindarajulu, 2023). By integrating green infrastructures into urban development, there is also the potential to enhance landscape connectivity, provide supplementary or improved habitat, and in certain cases, facilitate the establishment of controlled populations of threatened species (Butt et al., 2018). These strategies not only enhance ecosystem resilience but also provide numerous co-benefits, such as improved air and water quality, enhanced wildlife habitats, and enhanced community wellbeing. Therefore, such integration of nature-based solutions into urban planning and design, cities can better prepare for the future while promoting ecological health and human wellbeing.
The global consciousness surrounding sustainability in the construction sector has been steadily increasing, driven by factors such as climate change, population growth, and rapid urbanization. This growing awareness has led to a heightened demand for green buildings as a means to achieve sustainable development (Gou and Xie, 2017). Green buildings, as defined by The Energy and Resource Institute, a non-profit organization specializing in sustainable development, are structures meticulously designed, constructed, and operated to minimize their overall environmental impact while simultaneously enhancing user comfort and productivity (GRIHA Manual, 2010). Evidence supporting the economic advantages and reduced energy consumption associated with green buildings has emerged from various studies. For instance, research conducted in Malaysia demonstrated that green buildings can save ~71.1% of energy compared to industry baseline standards, underscoring their economic benefits and energy efficiency (Dwaikat and Ali, 2018). The imperative to reduce carbon emissions from buildings and construction aligns with the broader goal of mitigating the global impact of climate change. Moreover, the environmental, social, and economic benefits of green infrastructure, such as green roofs and walls, extend beyond the building itself. A simulation study conducted by Pragati et al. (2023) on multi-storied commercial buildings in Chennai, situated in India's hot and humid tropical climate, revealed significant positive impacts of greening systems on the urban environment. These systems led to reductions in air temperature, radiant temperature, humidity, and solar gain. Furthermore, they lowered the total energy consumption of buildings with green roofs and walls by 10.5% and reduced district cooling demand by 13%. In addition to these energy-related benefits, greening systems also substantially improved air quality and overall building energy efficiency.
4.2. Urban agriculture and food security
There is a growing global focus on recognizing the significance of cities in fostering resilient and ecologically sound food systems. These systems play a crucial role in withstanding and recovering from various crises, including natural disasters like storms, droughts floods, as well as anthropogenic and socioeconomic emergencies. The emergence of the COVID-19 pandemic has shed light on the importance of food resilience within cities, prompting a fresh consideration of the desired level of food self-sufficiency achievable through urban agriculture. This socio-economic crisis has prompted us to reevaluate the role of urban agriculture in ensuring a reliable and resilient food supply within cities. As a result, international policy efforts are increasingly directed toward understanding and addressing the pivotal role, cities play in ensuring food security and adaptability in the face of challenges.
The Sustainable Development Goal 11 of the UN 2030 Agenda for Sustainable Development acknowledges the imperative of creating inclusive, secure, resilient, and eco-friendly cities and human settlements. Within this agenda, there are additional goals related to sustainable agriculture that aim to alleviate poverty (SDG 1), enhance nutrition and diminish hunger (SDG 2), promote sustainable supply and demand patterns (SDG 12), and mitigate the effects of climate change (SDG 13). While agriculture has traditionally been practiced in and around cities, it is only recently that urban agriculture has gained formal recognition in international agendas. What distinguishes urban and peri-urban agriculture is not merely its urban location, but rather its integral role within the urban socioecological and economical system (Mougeot, 2000). The dependency of urban and peri-urban agriculture on urban resources such as land, workforce, and organic waste from the treated wastewater and municipal solid wastes to be used in the form of organic fertilizer, cannot be ignored. The urban agricultural system is also profoundly influenced by urban conditions such as governmental policies, regulations, land competition, urban markets demand patterns, and prices. Moreover, it in turn could impacts the urban system by affecting food security, poverty, ecology, and public health. Thus, urban agriculture plays a vital role in climate change adaptation by contributing to food security and enhancing the resilience of urban communities.
Over the past decade, urban agriculture has experienced a significant shift in perception, moving from being considered a niche interest to garnering substantial attention from policymakers and urban planners, both in developed and developing nations. The urgency of providing sustenance to a rapidly urbanizing global population has become a critical priority for cities (FAO, 2012, 2014). The incorporation of productive landscapes into urban design and development planning has gained widespread acceptance (Bohn and Viljoen, 2011), recognizing the need for integrating agricultural practices within urban environments. In the context of climate change, urban agriculture offers several benefits for adaptation strategies when integrated with proper policy framework, resulting to smart climate agriculture (Figure 2). The expansion of urban and peri-urban agriculture offers a valuable solution in mitigating the consequences of increased rainfall by preserving low-lying areas free from construction. This approach helps to minimize the impact of floods, reduce runoff, and facilitate the storage and infiltration of excess water. Notable examples include the cities of Kesbewa in the Western Province of Sri Lanka and Rosario in Argentina, which actively promote the preservation and safeguarding of green and productive spaces along stream banks as a means to mitigate flood risks (Dubbeling, 2015). Within the framework of its Urban Master Plan (2005–2020), Beijing, China, has set forth a range of initiatives to preserve agricultural land and green spaces. These include the establishment of permanent green areas in peripheral zones and corridors, the promotion of wastewater recycling and rainwater/floodwater harvesting, the protection of forested areas and parks, and the certification and subsidization of energy-saving production practices.
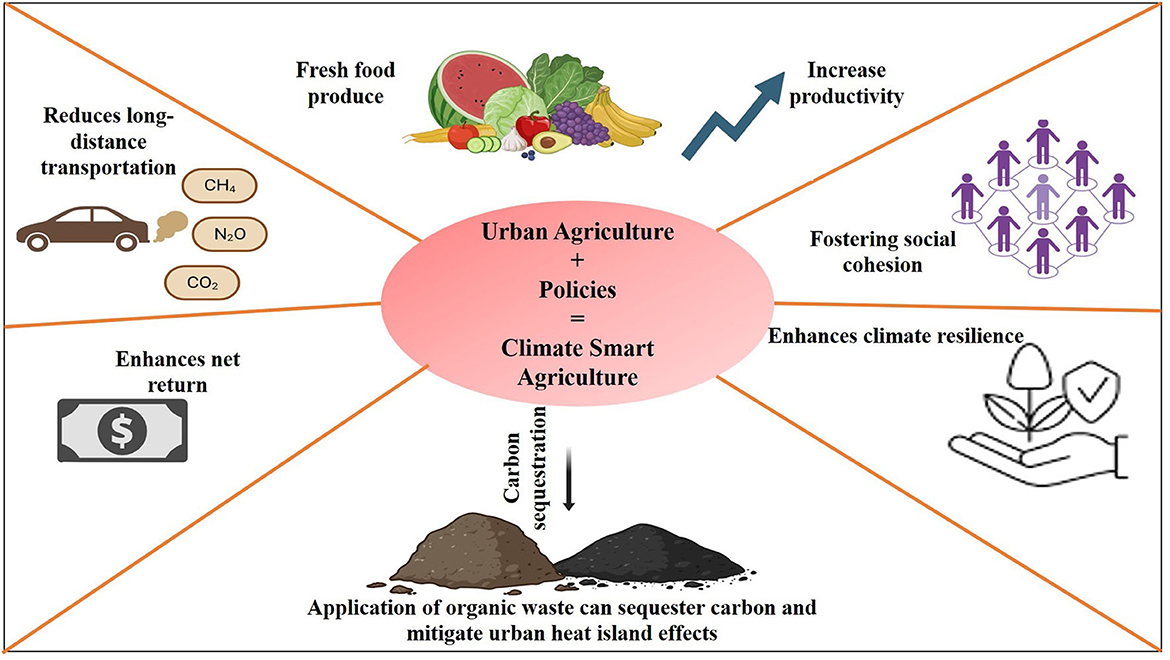
Figure 2. Cultivating sustainability: How urban agriculture nurtures communities and the environment. The picture depicts how urban agriculture along with policies could enhance food security by providing local and fresh produce, reducing reliance on distant and vulnerable food supply chains. This localized food production reduces the risks associated with disruptions in transportation and distribution caused by extreme weather events or other climate-related factors. Secondly, urban agriculture can contribute to climate change mitigation by reducing greenhouse gas emissions (Sonwani and Saxena, 2022). By producing food closer to consumers, it reduces the carbon footprint associated with long-distance transportation and refrigeration. Moreover, urban farming practices such as composting organic waste and promoting soil health can sequester carbon and mitigate urban heat island effects. Thirdly, urban agriculture enhances the resilience of urban communities by fostering social cohesion and building community networks. It provides opportunities for community engagement, skill-building, and knowledge sharing, creating a sense of ownership and empowerment among residents. In times of climate-related crises, such as extreme heatwaves or flooding, urban agriculture initiatives can serve as resilience hubs, providing access to fresh food, social support, and disaster response mechanisms.
In densely populated urban areas where space is limited, cities can encourage the development of rooftop gardens to enhance the thermal comfort of apartments situated beneath them. Such agricultural rooftops not only provide food for households but also have the potential to generate income through sales. An illustrative scenario developed for Vancouver, Canada, indicates that utilizing half of the city's available rooftop space for urban agriculture could meet ~4% of the food requirements for 10,000 people. By incorporating hydroponic greenhouses into the equation, this percentage could be further increased to 60%. Prominent examples of rooftop projects in North America include Brooklyn Grange, renowned for its soil-based rooftop gardens in New York City where they cultivate over 45,000 kg of organic produce annually.1 Another notable venture is Gotham Greens, which operates hydroponic greenhouses across five states in the United States.2 These innovative initiatives showcase the potential of utilizing rooftops for urban agriculture, demonstrating successful models of sustainable food production in urban environments. Urban agriculture has indeed gained momentum in India, with various factors influencing its prospects within the Indian context. Despite its potential, urban agriculture faces notable challenges in the country. A study conducted in Bengaluru by Camille (2018) sheds light on the class-specific nature of involvement in organic terrace gardening, primarily limited to the middle class. These terrace gardens are primarily driven by concerns about declining food quality and safety. Additionally, they serve as a means to create green spaces that counteract environmental degradation in the city. However, the study points out both the possibilities and limitations of urban gardening as a middle-class intervention into unsafe food systems and deteriorating urban ecologies.
Notably, in recent times, several Indian celebrities have taken proactive steps to promote and support urban organic farming. This movement has gained popularity due to its emphasis on sustainable and chemical-free agricultural practices. These celebrities leverage their influence and resources to raise awareness about organic farming and encourage its adoption. For instance, former Indian cricket captain Mahendra Singh Dhoni operates an agricultural farm spanning 43 acres in Ranchi, Jharkhand. His farm cultivates a variety of crops, including strawberries, capsicums, dragon fruit, watermelons, muskmelons, peas, and other vegetables. Such influential initiatives have the potential to significantly raise awareness among local communities, highlighting the importance of urban agriculture and its positive impact on sustainable food production and urban environments.
4.3. Biodiversity conservation and habitat restoration
As cities continue to expand and face the challenges posed by climate change, it becomes increasingly important to prioritize the preservation and restoration of biodiversity and habitats within urban areas. Urban areas often suffer from habitat loss and fragmentation due to urbanization, leading to a decline in biodiversity. However, preserving and restoring biodiversity within cities can provide numerous benefits for climate change adaptation and the overall wellbeing of urban populations. One of the primary benefits of biodiversity conservation in urban areas is the enhancement of ecosystem services such as clean air and water, pollination, and climate regulation. For example, urban green spaces, which are part of the green infrastructure, are facing growing expectations to serve multiple ecosystem services, including provisioning (gardening), regulating (such as thermoregulation, air quality control, flood mitigation, pollination), and cultural purposes (such as aesthetic and education) (Oberndorfer, 2007; Jerome et al., 2019; Keeler et al., 2019).
According to the survey conducted by Williams et al. (2014), the most frequently mentioned conservation benefit in green roof project descriptions was the creation of biodiversity habitat through facilitating plant growth. Various taxa were reported to benefit from these habitats, including native plants, birds, reptiles, mammals, bees, butterflies, moths, spiders, beetles, grasshoppers, and flies. Numerous studies have confirmed the presence of insects on green roofs (Kadas, 2006; Colla et al., 2009; MacIvor and Lundholm, 2011; Schindler et al., 2011; Tonietto et al., 2011), which are also readily colonized by spiders and soil-dwelling arthropods (Buttschardt, 2005; Schrader and Böning, 2006). Sedum-based roofs, in particular, have been noted for supporting high densities of collembolan species, as the fleshy vegetation retains water in the substrate for longer periods, creating a suitable habitat even during the warmest and driest times of the year (Schrader and Böning, 2006). Urban gardens also emerge as vital elements in pollinator habitat networks (Hall et al., 2017), benefiting species like bumblebees (Andersson et al., 2007; Jansson and Polasky, 2010; Conflitti et al., 2022). Surprisingly, urban areas harbor diverse pollinator species, with single gardens supporting 35% of UK hoverflies (Owen, 2010) and Berlin sustaining half of Germany's bee species (Saure, 1996). Urbanization influences pollinator communities, introducing new species and favoring adaptable ones (McIntyre et al., 2001; Angold et al., 2006; Geslin et al., 2013). Urban spaces provide consistent pollinator communities, benefiting agricultural productivity both within cities and neighboring rural areas (Baldock et al., 2015). Functional response diversity varies among pollinator types in response to biosphere changes, underscoring urban gardens' significance (Jansson and Polasky, 2010). The efficacy of this intricate process is inherently linked to the diversity and abundance of flowering plants available to pollinators. The ramifications of a thriving pollinator population are not confined to the immediate floral realm but reverberate throughout the entire urban ecosystem.
Bird visitation has also been observed in many studies (Millett, 2004; Baumann and Kasten, 2010; Fernández Cañero and González Redondo, 2010), and Pearce and Walters (2012) discovered increased bat activity over green roofs. A study conducted in Dehradun, India emphasized the significant impact of tree species diversity on enhancing bird communities within urban green spaces (Kaushik et al., 2022). The study identified the size of the urban green space at the broader landscape level and the diversity of tree species at the local scale as key factors that influenced bird species richness, density, and the presence of vulnerable insectivorous bird groups across different seasons. Another study conducted in Pune, India, highlighted that vegetation composition remains the primary determinant for birds in their habitat selection, regardless of the size or location of urban green spaces (Choudaj and Wankhade, 2023). Furthermore, biodiverse habitats in urban areas play a vital role in supporting pollinators like bees and butterflies, which are essential for the reproduction of numerous plant species (MacIvor and Lundholm, 2011; O'Brien et al., 2012; Ksiazek et al., 2014; Zhang et al., 2022). Similar to managed residential gardens, green roofs can provide floral and nesting opportunities for bees in urban settings, contributing to both ecological and socio-economic benefits (Loder, 2014; Makinson et al., 2017; Baldock, 2020). However, a recent study by Dusza et al. (2020) emphasizes the importance of plant community composition in modulating plant-pollinator interactions on the green roofs. It suggests that combining plant species with diverse flowering morphologies and phenologies can enhance pollinator diversity. Therefore, urban gardens and green spaces that offer suitable habitats for pollinators not only contribute to the preservation of plant diversity but also enhance the production of fruits and vegetables. This, in turn, can enhance food security, particularly in densely populated urban areas.
Biodiversity conservation and habitat restoration also contribute to the resilience of urban ecosystems in the face of climate change impacts. To enhance urban ecosystem services for climate change adaptation, several strategies can be employed. The analysis of city adaptation plans revealed that “Physical infrastructure” and “Green infrastructure” were the predominant types of actions identified (Butt et al., 2018). The plans frequently included measures to enhance urban spaces by increasing vegetation cover through initiatives such as tree planting, expanding canopy cover, and restoring native vegetation in parks, wetlands, forests, and other natural areas within cities. This can be achieved through land-use planning and zoning regulations that prioritize the preservation of green infrastructure. Amsterdam, as a notable example, has developed a dedicated biodiversity climate adaptation plan that aims to enhance resilience against extreme events by increasing and expanding protected areas. This plan also focuses on improving connectivity to facilitate species dispersal and migration. By utilizing natural processes such as recruitment and succession, the plan aims to enhance landscape heterogeneity, thereby promoting habitat integrity and persistence, which in turn benefits threatened species (McKinney, 2002). This case study exemplifies the alignment and mutual reinforcement of climate adaptation and biodiversity protection.
Another noteworthy initiative is seen in the Brazilian NDC (Nationally Determined Contribution), which includes a target to restore and reforest 12 million hectares of forests by 2030 (Bustamante et al., 2019). The restoration of native vegetation is considered a fundamental pillar for sustainable rural development in Brazil, and it should encompass multiple objectives, ranging from the conservation of biodiversity and ecosystem services to fostering social and economic advancement. By integrating these various purposes, the restoration efforts can effectively contribute to the holistic development of the region. Another notable achievement in the restoration and conservation of urban biodiversity can be found in WIPRO's efforts in Bangalore, India.
The implementation of watershed protection activities can also play a crucial role in promoting biodiversity by effectively reducing polluted runoff and enhancing water flow within these areas. This has the potential to yield significant improvements for a total river length of 5,872 km, benefiting numerous threatened freshwater fish species identified within the cities (Butt et al., 2018). Considering the inherent dryness and higher temperatures of urban areas compared to the surrounding landscapes (Moriwaki et al., 2013), coupled with the high-water demand in cities (where 60% of urban water use is concentrated; Grimm et al., 2008), water management emerges as a key focus in many city adaptation plans. Prominent examples include cities such as Copenhagen, Rotterdam, and Lagos, which have prioritized water management strategies to address the challenges posed by climate change and urbanization.
4.4. Urban forests: carbon sequestration, air quality improvement and climate regulation
Urban forests play a crucial role in climate change adaptation, providing numerous environmental, social, and economic benefits to cities and their inhabitants. By strategically managing and expanding urban forest cover, cities can effectively address climate-related challenges and build more resilient communities. Investments in urban forests have witnessed an upsurge in numerous cities across the United States. Studies highlight the importance of expanding urban forests as they offer a desirable solution to mitigate pollution-related issues (Manning, 2008; McPherson et al., 2013). Consequently, urban tree planting initiatives are actively advocated as a planning tool to facilitate climate change adaptation, promote urban sustainability, and enhance human health and wellbeing.
Urban areas are often characterized by the “urban heat island” effect, where temperatures are significantly higher than surrounding rural areas. Urban forests can mitigate this effect by providing shade and evaporative cooling, thereby reducing ambient temperatures. The dense foliage of trees and vegetation can act as natural air conditioners, creating a cooler microclimate and reducing energy demand for cooling. Trees excel in these mechanisms compared to other vegetation types like shrubs or grass due to their higher evapotranspiration capacity. Shashua-Bar and Hoffman (2000) highlights the significance of the road-side trees in the urban ecosystem, as they could modify indoor temperatures by providing shade to buildings. This shading effect greatly induces indoor insulation (Mavrogianni et al., 2014). Furthermore, urban trees mitigate greenhouse gas emissions tied to building energy usage by regulating microclimates via shading and evapotranspiration effects (McPherson et al., 2013; Hsieh et al., 2018). This is particularly beneficial for human health in situations where financial resources are limited for cooling buildings, as it can alleviate the need for artificial cooling methods and potentially reduce energy consumption. A study by Berry et al. (2013) demonstrated that tree shade can lower wall temperatures by as much as 9°C and air temperatures by up to 1°C.
Moreover, trees contribute to increased humidity levels in their vicinity, serving as conduits for water loss to the atmosphere (Salmond et al., 2016). Their roots draw moisture from deeper soil layers, which is especially advantageous in urban areas facing water availability challenges. Implementing water-sensitive urban design, stormwater harvesting, and recycled water systems can effectively enhance soil moisture levels in cities. Water stored on urban tree canopies may either evaporate or be transmitted to the ground for root absorption (Seitz and Escobedo, 2008; Berland et al., 2017). Trees utilize some absorbed rainfall and transpire the rest. Unabsorbed canopy water drips to the ground, while stemflow mitigates raindrop impact, preventing soil erosion (Livesley et al., 2016; Qin, 2020). Leaf litter aids water absorption, stabilizes soil, and curbs erosion.
Urban forests also contribute to the sequestration of carbon. For instance, studies estimate that the urban forest in the Chicago region alone provides ~$14 million per year in carbon sequestration benefits (Nowak et al., 2013c). A recent interpretive analysis conducted by Bherwani et al. (2022) focused on five cities in India—Nagpur, Navi Mumbai, Bengaluru, Delhi, and Leh. The study utilized the normalized difference vegetation index (NDVI) and allometric relationships to evaluate the fiscal importance of urban greeneries in terms of carbon sequestration and their role in mitigating severe climatic impacts. The valuation of carbon sequestration in these cities ranged from $19.04 to $6,537.15 million, highlighting the substantial economic value associated with the carbon sequestration potential of urban green spaces. Many studies evaluating urban vegetation's carbon storage and sequestration utilize tree biomass and growth equations, often integrating field surveys and remote sensing data (Nowak et al., 2008; Liu and Li, 2012; He et al., 2017; Li et al., 2020). Some meta-analyses in the USA and China underscore significant carbon sequestration potential in urban green infrastructure (Nowak et al., 2013a; Chen, 2015). Assessments estimating urban carbon budgets generally demonstrate modest impact on offsetting through urban vegetation (Escobedo et al., 2010; Liu and Li, 2012; Vaccari et al., 2013; Baró et al., 2015; Zhao and Sander, 2015; Baró and Gómez-Baggethun, 2017). However, these studies predominantly consider direct carbon sequestration, overlooking urban vegetation's indirect contributions to reducing city energy use (Escobedo et al., 2010; McPherson et al., 2013).
Urban vegetation, particularly trees, plays a pivotal role in removing pollutants from the air through processes like pollutant uptake via leaf stomata and airborne particle interception (Linden et al., 2023). This vegetation also acts as a physical barrier, restricting pollutant entry into specific zones (Prigioniero et al., 2023). Greenery-based strategies are proposed for reducing air pollution (Nowak et al., 2006). However, the extent of vegetation's impact on urban air quality and health is debated due to predictive uncertainties and limited empirical data (Pataki et al., 2011). Through various mechanisms, such as leaf surfaces and stomata, trees absorb harmful substances, preventing them from lingering in the air and reducing their negative impacts on human health and the environment. The ability of urban trees to act as natural air purifiers highlights their immense value in combating urban air pollution and underscores the importance of preserving and expanding urban forest areas (Garcia, 2017; Kim, 2017). Dry deposition is the primary mechanism by which urban trees filter out harmful gases from the air (Cabaraban et al., 2013). With help of the stomata in their leaves, they can absorb gaseous pollutants, while capturing or trapping particulate matter in the air by other processes such as sedimentation or impaction (Gómez-Baggethun et al., 2013; Sharma and Saxena, 2022). The foliar surface serves as a resting place for particles while other surfaces, including as bark, hair, moisture, and stickiness, help to adsorb the particles on such organs (Grote et al., 2016). Studies assessing urban vegetation's air pollution mitigation capacity often employ dry deposition models. These models (Yang et al., 2005; Nowak et al., 2006, 2013b; Escobedo and Nowak, 2009; Cabaraban et al., 2013; Selmi et al., 2016; Yin et al., 2022) operate at the city scale, incorporating parameters such as leaf area index, pollution concentrations, and meteorological data. This modeling showcases substantial pollution removal by urban vegetation, though it has limited efficacy in addressing city-wide pollution challenges (Baró et al., 2015).
Air pollutants (such as O3, SO2, and NO2) that can be efficiently metabolized inside the leaf tissues are taken up through the stomata (Rai et al., 2011; Ghosh et al., 2021). The cuticle is a porous barrier that allows some pollutants (such as NO and NO2) access to the intercellular space of the plants (Bytnerowicz, 1996) where moisture or the chemicals inside the apoplast alter the gaseous pollutants and dispersed into the intercellular spaces (Omasa et al., 2002; Nowak et al., 2006). Once pollutants enter cells, they are met with defensive mechanisms and initial and subsequent metabolic reactions (Bytnerowicz, 1996). Different metabolic mechanisms in tree leaves biochemically convert each pollutant (Omasa et al., 2002). Remarkably, localized greening strategies like green roofs and walls, proposed by Pugh et al. (2012), exhibit notable reductions in NO2 (up to 43%) and PM10 (up to 62%) due to enhanced deposition without impeding ventilation. Experimental studies consistently affirm that green spaces are linked to reduced site-scale pollution, especially particulate matter (Irga et al., 2015; Yao et al., 2023). Plants can ozonolyze O3 in the sub-stomatal cavity and apoplast after it has been taken up through the stomata (Ghosh et al., 2018). Most of the carbon dioxide (CO2) that trees take in is converted into serine and then, via the serine pathway, into sucrose. Bidwell and Fraser (1972) and Nowak (1994) both suggest that photosynthesis might fix some CO as CO2.
A broad variety of plant and animal species rely on the habitats and resources provided by urban forests in cities (Stagoll et al., 2012; Wood and Esaian, 2020). Native trees are excellent in sustaining native animal populations and communities. Recent research, however, suggests that non-native trees can nevertheless help to preserve native biodiversity in urban areas (Liu and Slik, 2022). To help wildlife cope with the effects of climate change on ecosystems and migration patterns, urban woods provide safe havens. Urban areas can help the cause of biodiversity conservation on a regional and global scale by protecting and extending their green spaces.
Apart from their ecological significance, urban forests offer numerous societal benefits and wellbeings to city dwellers. Having easy access to green areas and natural habitats has been associated with better psychological wellbeing, lower anxiety levels, and more time spent being physically active. Community resilience and cohesion can be boosted by efforts to improve urban woods, which in turn increase options for leisure pursuits, civic engagement, and individual wellbeing. In this way, urban forests not only connect people with nature but also cultivate a sense of place—an intricate process where individuals interact with urban forests, have unique experiences (both favorable and unfovorable), and develop their own ways of valuing and appreciating nature (Phillips and Atchison, 2020; Bolleter and Hooper, 2021).
5. Synergies and trade-offs: balancing urban development and ecosystem services
Achieving a balance between urban development and the provision of ecosystem services is a complex challenge faced by policymakers, urban planners, and communities. A pictorial diagram has been provided illustrating the interplay between urban ecosystem services and climate change factors, depicting the environmental health of urban areas (Figure 3). While urban development is necessary for meeting the needs of the growing populations, it often leads to the loss or degradation of natural ecosystems, resulting in a reduction of essential ecosystem services. Synergies and trade-offs exist between urban development and ecosystem services, requiring careful consideration to strike a sustainable balance. Synergies refer to situations where urban development and the maintenance or enhancement of ecosystem services can reinforce one another. For example, incorporating green infrastructure, such as parks, urban forests, and wetlands, into urban designs can provide multiple benefits. These green spaces not only contribute to the aesthetic appeal of cities but also enhance air quality, regulate temperatures, support biodiversity, and offer recreational opportunities. Thus, urban development that integrates and enhances ecosystem services can create positive synergies, improving both the livability and sustainability of cities (Salmond et al., 2016). However, trade-offs can also arise when urban development compromises ecosystem services. For instance, converting natural habitats into built environments can result in the loss of biodiversity, reduced water infiltration, increased runoff, and diminished climate regulation. This can have negative consequences such as increased flood risk, reduced water quality, and decreased resilience to climate change. Trade-offs may also arise when land is allocated to urban infrastructure rather than preserving or restoring natural areas.
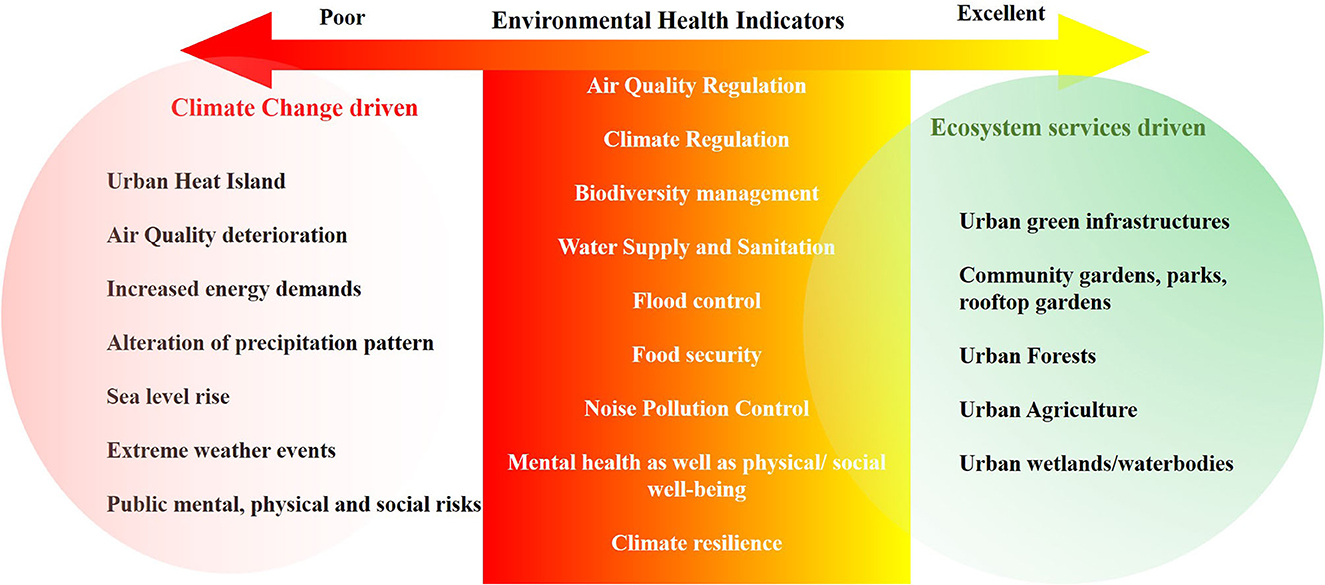
Figure 3. A visual diagram depicts how urban ecosystem services and climate change factors interact, offering insight into the overall environmental heath of urban regions.
5.1. Urbanization and service provision
Urbanization and land use change have profound implications for the provision of ecosystem services and pose challenges in achieving a balance between urban development and the preservation of these services. As cities expand and develop, natural ecosystems are often converted into built environments, leading to the loss or degradation of valuable ecosystem services. Balancing urban development and ecosystem services requires understanding the synergies and trade-offs associated with these processes. Urbanization can result in both positive and negative synergies with ecosystem services. Land use changes associated with urban development often involve the conversion of natural habitats, agricultural lands, or wetlands into built-up areas. This loss of natural ecosystems results in the degradation or reduction of critical services. For instance, the loss of forests or wetlands can diminish water filtration and purification, increase stormwater runoff, and reduce habitat availability for biodiversity. Such trade-offs can have negative consequences for water quality, flood risk, climate resilience, and overall ecological functioning. Contrary, if well-planned urban development can incorporate green infrastructure, such as parks, urban forests, and green spaces, which provide multiple benefits. By integrating ecosystem services into urban design, cities can enhance livability and sustainability while providing important social, environmental, and economic benefits (Elmqvist et al., 2015). For example, India strives to achieve a remarkable urbanization rate of 60% by 2050, the Government of India is actively working to match this rapid urban transition. A key initiative in this endeavor is the ambitious Smart Cities Mission, which aims to overhaul 100 cities by modernizing their infrastructure. This transformation involves the application of cutting-edge technology to enhance essential urban services and improve living conditions. The Smart Cities Mission encompasses various aspects, including the development of eco-friendly infrastructure, the construction of energy-efficient buildings, and a strong emphasis on renewable energy sources. Ultimately, the mission's strategy revolves around the creation of well-integrated, livable, and sustainable cities that offer a high quality of life for all their residents.
Furthermore, green infrastructure and built infrastructure are two contrasting approaches to urban development, each with its own set of synergies and trade-offs when it comes to balancing urban development and ecosystem services. While the benefits of urban forests are widely acknowledged, understanding the specific contributions of trees in kerbside locations to human wellbeing and the local environment requires further exploration (Salmond et al., 2016). Developing comprehensive methodologies and frameworks for assessing their impact at this scale remains a challenge. Nonetheless, continued research and innovation in evaluating the net impact of trees in urban areas will be essential to inform effective decision-making and maximize the benefits derived from urban tree planting initiatives. Most studies on the benefits of street trees for human health and the urban environment have been conducted in isolated locations, and those that have been conducted have used overly simplistic methods. Street trees have far-reaching and interrelated effects on urban ecosystems, but these effects are typically overlooked by techniques that take a more general view of trees or focus primarily on individual benefits or drawbacks. Consequently, urban planners and policymakers may make decisions based on optimizing a single parameter, which can be problematic when a single action produces a wide range of outcomes, has many effects, and presents potential trade-offs in diverse settings (Salmond et al., 2016).
It is widely recognized that incorporating green infrastructure and urban greening practices into urban design can effectively mitigate the urban heat island effect. Nonetheless, to harness the full potential of urban forests and tree covers and optimize the benefits they offer, strategic planning becomes imperative. It is important to weigh the benefits of street trees during the day against their drawbacks at night and in the winter, as stated by Coutts et al. (2016). While trees do help mitigate nighttime heat loss, they also act as a radiation barrier within the urban canyon, making it harder for air to circulate and preventing sensible heat from building up during the day. A broad tree canopy can be beneficial during the day, but it may prevent the building from receiving sufficient longwave radiation at night, leading to warmer and uncomfortable conditions inside.
It is also important to remember that trees change the aerodynamic resistance to heat diffusion, which can prevent cool breezes from penetrating open windows on hot summer evenings (Salmond et al., 2016). Trees and the weather in an area interact in a mutually beneficial way. Maintaining tree canopies in good health and transpirational activity requires a steady supply of water. But unlike their forest cousins in the countryside, street trees in cities face a variety of hazards (Ferrini et al., 2014) which includes pollution, vehicular damage, vandalism, inadequate watering, soil compaction, pest attack, etc. There is a significant evaporative requirement because of the high temperatures, low humidity, and low soil moisture found in cities (Montague et al., 2000). The health and ability of street trees to cool metropolitan areas may be jeopardized if they are not provided with adequate irrigation to keep soil moist, support trees, and absorb excessive heat loads. Given the anticipated patterns of climate change in many urban locations, this scenario is of particular concern.
5.2. Socioeconomic equity and access to services
Socioeconomic equity and access to services are critical considerations in the context of balancing urban development and ecosystem services. Achieving a balance between these factors involves understanding the synergies and trade-offs associated with urban development and ensuring that the benefits of ecosystem services are accessible to all segments of society. Urban development can have both positive and negative impacts on socioeconomic equity and access to services. On one hand, well-planned urban development can lead to improved infrastructure, enhanced service provision, and increased opportunities for economic growth and social development. This includes access to basic services such as clean water, sanitation, healthcare, education, and transportation, which are essential for improving the quality of life for urban residents.
However, urban development can also create trade-offs and challenges in achieving equitable access to services. Rapid urbanization and land use changes often result in the displacement of marginalized communities, who may be forced to relocate to areas with limited access to essential services (Forbes, 2016). Forced urban displacements, especially slum removals, are often justified under the pretext of aesthetic reasons or the necessity to accommodate major events like international games, fairs, conferences, etc., according to research by the Habitat International Coalition, a non-governmental organization focused on housing policy matters. These measures, however, only benefit special interests rather than the general public and do little to solve the underlying housing crisis (Forbes, 2016). Furthermore, the development of certain urban areas may prioritize high-income neighborhoods, leaving lower-income communities with inadequate infrastructure and limited access to ecosystem services such as green spaces, clean air, and recreational opportunities. According to research conducted by Lara-Valencia and García-Pérez (2015), residents of low-income areas in the Mexican city of Hermosillo are disproportionately affected by the unequal distribution of public park space. Based on the findings, it appears that the struggle between globalized places and local public locations is becoming more pronounced as the city's economy gets more interwoven with global networks. As a result, this exacerbates the marginalization of communities that have unequal access to urban parks to begin with. In another instance, Guzman and Bocarejo (2017) conducted a case study in Bogota, Colombia, focusing on accessibility analysis for various income levels to the urban infrastructure. The study revealed that more than 80% of the low-income population faced significantly low accessibility indexes concerning their commute to work. These disparities in access likely play a pivotal role in the substantial variations in mobility observed among different socioeconomic groups. In particular, the study found that high-income groups made ~150% more trips compared to their low-income counterparts.
While the idea of the Smart Cities mission is widely embraced in India, there remains a notable lack of clarity in the concept note regarding its implications for the most marginalized sectors of society. It is of paramount importance to take into account urban ecosystem services, especially the affordable and publicly accessible provisioning services originating from urban commons, when formulating strategic plans for Indian cities. This approach can serve as a vital means to bolster the social and ecological resilience of the ever-expanding population of disempowered and underprivileged individuals residing in cities across India (Mundoli et al., 2017). The research conducted by Mundoli et al. (2017) highlights several crucial principles that should be integrated into the vision of smart cities. One such principle emphasizes the importance of preserving urban commons and the ecosystem services they offer as shared resources accessible to all members of society. Regrettably, there appears to be a growing trend among urban planners to redefine urban ecosystem services as economic commodities, thereby restricting their use to those with the financial means to afford these services. This shift in perspective is concerning. To address this challenge, it is imperative that our country embarks on a thoughtful and contextually aware reimagining of the concept and representation of urban commons. These areas should be considered as assets owned collectively by and for all citizens. To address these challenges, it is important to integrate principles of social equity into urban planning and decision-making processes. This involves prioritizing inclusive development, affordable housing, and mixed-income neighborhoods to ensure that all residents have access to essential services. Proactive measures should be taken to prevent the displacement of vulnerable communities and provide them with adequate support and opportunities for upward mobility. Promoting the equitable distribution of ecosystem services is also crucial. Green infrastructure and urban greening projects should be strategically implemented to benefit all communities, including those in socioeconomically disadvantaged areas. This can help reduce environmental inequalities and provide equal access to the multiple benefits that ecosystem services offer, such as improved health and wellbeing, enhanced resilience to climate change, and increased social cohesion. Community engagement and participation play a vital role in achieving socioeconomic equity and ensuring access to services. Inclusive decision-making processes that involve the voices of diverse stakeholders, including marginalized communities, can lead to more equitable outcomes. This can help identify specific needs, preferences, and priorities of different groups and guide the development of policies and interventions that address their unique challenges and aspirations. Therefore, by integrating principles of social equity into urban planning, preventing displacement, promoting mixed-income neighborhoods, and prioritizing the equitable distribution of ecosystem services, cities can create inclusive and sustainable environments that benefit all residents. A comprehensive and participatory approach is essential to address trade-offs and foster synergies between urban development, ecosystem services, and socioeconomic equity, leading to more resilient and thriving communities.
6. Policy and planning for sustainable urban ecosystem services and the existing challenges
Incorporating ecosystem services into urban planning is a crucial approach in policy and planning for sustainable urban ecosystem services since last 100 years. Policy and planning for sustainable urban ecosystem services involves several key aspects. Firstly, it requires recognizing and valuing the contributions of ecosystems to urban areas. This involves understanding the various ecosystem services provided by natural systems and quantifying their economic and social value. By incorporating this information into decision-making processes, policymakers and planners can prioritize the preservation and restoration of ecosystems within urban environments. Secondly, sustainable urban ecosystem services planning emphasizes the integration of green infrastructure into urban design (McPhearson et al., 2014). Additionally, policy and planning for sustainable urban ecosystem services necessitates collaboration among various stakeholders, including government agencies, community organizations, and private entities (Hansen et al., 2015). This collaborative approach ensures that diverse perspectives are considered and integrated into decision-making processes. It also fosters partnerships for implementing and maintaining green infrastructure, promoting shared responsibility for the sustainable management of urban ecosystems. Furthermore, monitoring and evaluation play a vital role in policy and planning for sustainable urban ecosystem services. Continuous monitoring of ecosystem conditions and the assessment of the effectiveness of interventions and policies help refine strategies and ensure that desired outcomes are achieved. By monitoring indicators related to ecosystem health, biodiversity, and the provision of ecosystem services, policymakers and planners can make informed decisions and adapt their strategies as needed.
Governance and stakeholder engagement are critical components of policy and planning for sustainable urban ecosystem services. Effective governance ensures that decision-making processes are transparent, inclusive, and participatory, while stakeholder engagement involves actively involving various stakeholders in the planning and implementation of policies related to urban ecosystem services. For instance, the study of Olsson et al. (2020) highlights the importance of involving various stakeholders in the decision-making process to achieve a just and honest urban ecosystem services governance that benefits the entire community and fosters long-term sustainable development in the Sofielund district in Malmö, Sweden. They conducted interviews with 16 stakeholders, including property owners or managers, businesses, and representatives from the Swedish Union of Tenants. The findings stress the need for inclusive and accountable governance of urban ecological services. Failure to take into account the interests of all parties involved could have resulted to one group's voice affecting urban development at the expense of others. The lack of a formal system for property owners to have input and communicate with the city government is a major obstacle. A different instance in Bogota, Colombia, highlights the susceptibility of local stakeholder involvement and cooperative management when faced with unstable government regulations concerning urban wetland management. In response to this precarious situation, local stakeholders have found legal actions to be the most effective strategy. These legal actions not only facilitate collaborative management but also safeguard their participation in the development and continuity of this management approach (Remolina, 2015). Thus, the governance and policy frameworks related to urban ecosystem services and climate change adaptation are often fragmented or inadequate. This highlights the fact that there is often a lack of coordination and collaboration among different sectors and stakeholders involved in urban ecosystem management. Climate change adaptation and mitigation strategies need to be better integrated into urban planning frameworks to ensure the effective management and enhancement of ecosystem services.
In another assessment, Kabisch (2015) uncovered how the ecosystem service framework is structured and implemented inside Berlin's existing urban green planning structure. This evaluation not only highlighted considerable difficulties in urban green governance, but also indicated the level of integration of the ecosystem service framework into the planning system. Although stakeholders are aware with the phrase, the examination of planning documents revealed that only recently established informal strategies expressly use the ecosystem service framework. Increasing development pressure due to population growth and municipal budget constraints, the loss of expertise, and a lack of communication leading to a lack of awareness of the benefits of green spaces among various stakeholders are the primary challenges facing urban green governance in Berlin. Such examples set out for the need of more coherent and integrated policies that explicitly address the linkages between climate change, urban development, and ecosystem services. Additionally, stronger coordination and collaboration between different levels of government and stakeholders are required to effectively address climate change impacts on urban ecosystems.
Furthermore, urban resilience and adaptation strategies in the context of policy and planning are crucial for cities to address and prepare for the challenges posed by various stressors and shocks. These strategies aim to enhance the ability of cities to withstand and recover from adverse events of climate change while maintaining essential functions and quality of life for their residents. Despite its great popularity, resilience has been shown in studies to occasionally lead to the maintenance of the current situation at the expense of social justice (Ziervogel et al., 2017). Further, it has the potential to accidentally lock in unsustainable growth patterns, leading to complex and underappreciated trade-offs at many spatial and temporal scales (Chelleri et al., 2015). This results in a governance gap (Wagenaar and Wilkinson, 2015) between the aspirational goal of resilience and the practical capacity to effectively control resilience at the urban level. When thinking about governance, the most effective method for bolstering urban resilience is to create a network of public institutions, government agencies, and private citizens working together to achieve common goals as part of a coordinated plan of action (Coaffee et al., 2009). However, municipal officials face substantial obstacles in accomplishing such objective. Furthermore, effective monitoring and evaluation systems are also lacking to assess the effectiveness of climate change adaptation measures and the provision of ecosystem services in urban areas. Thus, there is a need to develop standardized indicators and monitoring protocols to track changes in urban ecosystems and evaluate the success of climate change adaptation strategies. Public backing appears essential, particularly for consistent monitoring of habitat alterations and for their sustained engagement in the preservation and administration efforts. Furukawa (2013) research on the revitalized ecosystems of Shibaura Island and Yokoyama in Tokyo provides a prime illustration of the active participation of local residents from both urban and rural areas in the planning, implementation, and maintenance of these spaces. Kolkata's urban wetlands in India provide another example of success, primarily attributed to three factors: (1) diverse utilization of the ecosystem, (2) the harmony of urban ecological interactions, and (3) the powerful collaborative efforts of the community. This demonstrates how effective environmental and ecosystem governance relies on collective action and informal institutions as much as formal governance mechanisms (Hettiarachchi and Morrison, 2017). An additional instance of active involvement from the public, particularly students, can be observed in the upkeep of Hanul Madang, a rooftop garden located in Seoul city, South Korea as indicated in Table 2 (Son, 2018). Despite presenting positive case studies, it does not alter the harsh reality of ongoing social inequity in ecosystem service management and access which varies with regions and countries. However, ensuring that all communities, especially those most vulnerable to the impacts of climate change, have access to the benefits of ecosystem services can help alleviate some of the existing societal challenges in this context.
Another crucial aspect is the financing mechanisms that can play a significant role in supporting the enhancement of ecosystem services in urban areas. These mechanisms provide the necessary financial resources to implement projects and initiatives aimed at improving and maintaining the provision of ecosystem services. However, the deployment of green infrastructure faces extra hurdles due to the presence of financial constraints in many cities (Pataki et al., 2011). Cities can hardly afford to keep their current infrastructure running, let alone invest in researching, designing, building, and testing greener alternatives. Tree planting, stream daylighting (raising underground streams to the surface), and biofiltration project building are just a few examples of the ecosystem-services infrastructure efforts that many communities have turned to non-profits to implement in order to deal with this problem. This strategy, however, may result in complex public-private partnerships that are not sustainable over the long run due to grant cycles, inadequate nonprofit capacity, and other structural challenges (Svendsen and Campbell, 2008). Green bonds have emerged as a viable and attractive financing option that India, alongside other nations like China, the United States, Brazil, and many more across the globe, has actively embraced. India has been steadily advancing its commitment to environmentally sustainable project financing for an extended period. The demand for “green” financial products is on the rise, accompanied by a growing trend of investors declining to invest in companies that do not meet the evolving sustainability criteria. Consequently, financial service providers find themselves facing mounting pressure from both investors and rating agencies to establish effective strategies for sustainable management and conduct. Additionally, they now contend with new competitors who prioritize sustainability as a central element of their business models. In the context of the Indian banking sector, the government has introduced several laws and initiatives aimed at incentivizing lending to carbon-neutral enterprises and promoting environmentally friendly loans. These measures reflect a broader effort to align the financial sector with ecologically responsible practices (Bhatnagar et al., 2022).
7. Conclusion
In conclusion, the review highlights the importance of urban ecosystem services in the context of climate change and emphasizes the need for enhancing these services for effective adaptation. Urban ecosystems provide a range of benefits, including improved air and water quality, climate regulation, biodiversity conservation, and enhanced wellbeing for urban residents. However, climate change poses significant challenges to urban ecosystem services, including increased heat stress, flooding, and habitat loss. These impacts require proactive measures to enhance the resilience of urban ecosystems and ensure their continued provision of services. By incorporating nature-based solutions, such as green and blue infrastructure, cities can mitigate climate change effects, reduce vulnerabilities, and promote adaptation. It is essential to strike a balance between urban development and the preservation of ecosystem services. Synergies and trade-offs need to be carefully considered to avoid compromising the long-term sustainability and functionality of urban ecosystems. Integrating ecosystem services into policy and planning frameworks is crucial for sustainable urban development and effective climate change adaptation. Balancing urban development and ecosystem services requires an integrated approach that considers the long-term impacts on both human wellbeing and environmental health. This involves incorporating principles of sustainable development, such as compact city planning, mixed land-use zoning, and the protection and restoration of natural habitats. It also necessitates engaging stakeholders, including local communities, in decision-making processes to ensure their needs and perspectives are considered. Innovative planning and design strategies can help maximize synergies and minimize trade-offs. Nevertheless, challenges and gaps exist in the field of urban ecosystem services. Knowledge gaps, limited financial resources, governance and policy deficiencies, social equity considerations, and the need for robust monitoring and evaluation systems are significant challenges that must be addressed. These gaps call for increased research, innovative financing mechanisms, improved governance structures, and greater inclusivity and equity in decision-making processes. However, addressing these challenges and filling the gaps requires a multi-dimensional approach that combines scientific research, policy development, financial mechanisms, and community engagement. It calls for stronger collaboration among stakeholders, increased investment in urban ecosystem services, and the integration of climate change considerations into urban planning and governance processes.
Future research in the realm of urban ecosystem services and climate change should explore innovative approaches for enhancing urban resilience. This includes investigating the effectiveness of nature-based solutions in mitigating climate impacts, such as urban green infrastructure, green roofs, and sustainable urban planning strategies. Additionally, understanding the socioeconomic and equity implications of these solutions, as well as developing integrated assessment tools and models, will be critical for informed decision-making by urban policymakers. Furthermore, research should address emerging challenges related to climate-induced migration, urban heat island effects, and the preservation of biodiversity within cities. Ultimately, a holistic approach that combines ecological, social, and technological perspectives will be essential to create sustainable and climate-resilient urban environments.
Author contributions
BP: Methodology, Writing—original draft. AG: Conceptualization, Supervision, Writing—review and editing.
Funding
The author(s) declare no financial support was received for the research, authorship, and/or publication of this article.
Acknowledgments
BP expresses gratitude to the Director of CSIR-CIMFR for the provision of essential resources. AG extends appreciation to the Head of the Department of Life Sciences at Central University of Jharkhand, Ranchi, India, for facilitating necessary facilities. AG acknowledges the Department of Science and Technology (DST) INSPIRE division for their generous financial support in the form of the DST INSPIRE Faculty Fellowship (IFA22-LSPA 154).
Conflict of interest
The authors declare that the research was conducted in the absence of any commercial or financial relationships that could be construed as a potential conflict of interest.
Publisher's note
All claims expressed in this article are solely those of the authors and do not necessarily represent those of their affiliated organizations, or those of the publisher, the editors and the reviewers. Any product that may be evaluated in this article, or claim that may be made by its manufacturer, is not guaranteed or endorsed by the publisher.
Footnotes
1. ^Brooklyn Grange Farm. Brooklyn Grange. Available online at: https://www.brooklyngrangefarm.com (accessed August 6, 2023).
2. ^Gotham Greens. Available online at: https://www.gothamgreens.com/ (accessed August 17, 2023).
References
Agbelade, A. D., and Onyekwelu, J. C. (2020). Tree species diversity, volume yield, biomass and carbon sequestration in urban forests in two Nigerian cities. Urban Ecosyst. 23, 957–970. doi: 10.1007/s11252-020-00994-4
Alexandra, J., and Norman, B. (2020). The city as forest-integrating living infrastructure, climate conditioning and urban forestry in Canberra, Australia. Sustain. Earth 3, 1–11. doi: 10.1186/s42055-020-00032-3
Alqarni, A. S. (2020). Differential foraging of indigenous and exotic honeybee (Apis mellifera L.) races on nectar-rich flow in a subtropical ecosystem. Insects 11, 254. doi: 10.3390/insects11040254
Ament, J. M., Moore, C. A., Herbst, M., and Cumming, G. S. (2017). Cultural ecosystem services in protected areas: understanding bundles, trade-offs, and synergies. Conserv. Lett. 10, 440–450. doi: 10.1111/conl.12283
Andersson, E., Barthel, S., and Ahrné, K. (2007). Measuring social–ecological dynamics behind the generation of ecosystem services. Ecol. Appl. 17, 1267–1278. doi: 10.1890/06-1116.1
Angold, P. G., Sadler, J. P., Hill, M. O., Pullin, A., Rushton, S., Austin, K., et al. (2006). Biodiversity in urban habitat patches. Sci. Total Environ. 360, 196–204. doi: 10.1016/j.scitotenv.2005.08.035
Armson, D., Stringer, P., and Ennos, A. R. (2013). The effect of street trees and amenity grass on urban surface water runoff in Manchester, UK. Urban Forest. Urban Green. 12, 282–286. doi: 10.1016/j.ufug.2013.04.001
Artmann, M., and Sartison, K. (2018). The role of urban agriculture as a nature-based solution: a review for developing a systemic assessment framework. Sustainability 10, 1937. doi: 10.3390/su10061937
Aryal, K., Ojha, B. R., and Maraseni, T. (2021). Perceived importance and economic valuation of ecosystem services in Ghodaghodi wetland of Nepal. Land Use Policy 106, 105450. doi: 10.1016/j.landusepol.2021.105450
Ayanleye, S., Udele, K., Nasir, V., Zhang, X., and Militz, H. (2022). Durability and protection of mass timber structures: a review. J. Build. Eng. 46, 103731. doi: 10.1016/j.jobe.2021.103731
Babinszky, L., Halas, V., and Verstegen, M. W. (2011). Impacts of Climate Change on Animal Production and Quality of Animal Food Products. Climate Change Socioeconomic Effects. Rijeka: InTech, 165–190.
Bai, X., McPhearson, T., Cleugh, H., Nagendra, H., Tong, X., Zhu, T., et al. (2017). Linking urbanization and the environment: conceptual and empirical advances. Annu. Rev. Environ. Resour. 42, 215–240. doi: 10.1146/annurev-environ-102016-061128
Bakure, B. Z., Hundera, K., and Abara, M. (2022). “Review on the effect of climate change on ecosystem services,” in IOP Conference Series: Earth and Environmental Science, Vol. 1016 (IOP Publishing), 012055 p.
Baldock, K. C. (2020). Opportunities and threats for pollinator conservation in global towns and cities. Curr. Opin. Insect Sci. 38, 63–71. doi: 10.1016/j.cois.2020.01.006
Baldock, K. C., Goddard, M. A., Hicks, D. M., Kunin, W. E., Mitschunas, N., Osgathorpe, L. M., et al. (2015). Where is the UK's pollinator biodiversity? The importance of urban areas for flower-visiting insects. Proc. R. Soc. B Biol. Sci. 282, 20142849. doi: 10.1098/rspb.2014.2849
Baptista, M. D., Livesley, S. J., Parmehr, E. G., Neave, M., and Amati, M. (2018). Variation in leaf area density drives the rainfall storage capacity of individual urban tree species. Hydrol. Process. 32, 3729–3740. doi: 10.1002/hyp.13255
Baró, F., Chaparro, L., Gómez-Baggethun, E., Langemeyer, J., Nowak, D. J., and Terradas, J. (2014). Contribution of ecosystem services to air quality and climate change mitigation policies: the case of urban forests in Barcelona, Spain. Ambio 43, 466–479. doi: 10.1007/s13280-014-0507-x
Baró, F., and Gómez-Baggethun, E. (2017). “Assessing the potential of regulating ecosystem services as nature-based solutions in urban area,” in Nature-Based Solutions To Climate Change Adaptation in Urban Areas: Linkages Between Science, Policy Practices, eds N. Kabisch, H. Korn, and J. Stadler (Bonn), 139–158. doi: 10.1007/978-3-319-56091-5_9
Baró, F., Haase, D., Gómez-Baggethun, E., and Frantzeskaki, N. (2015). Mismatches between ecosystem services supply and demand in urban areas: a quantitative assessment in five European cities. Ecol. Indic. 55, 146–158. doi: 10.1016/j.ecolind.2015.03.013
Baumann, N., and Kasten, F. (2010). “Green roof-urban habitat for ground nesting bird,” in Urban Biodiversity and Design, eds N. Muller, P. Werner, and G. Kelcey (John Wiley & Sons), 348–362.
Bendict, M. A., and McMahon, E. T. (2006). Green Infrastructure: Linking Landscapes and Communities. Washington, DC: Island Press.
Beneberu, N., and Wondifraw, Z. (2020). Challenges and opportunities of milk production in urban dairy farms in ethiopia: a review. Food Sci. Qual. Manage. 102, 12–20.
Bera, B., Shit, P. K., Saha, S., and Bhattacharjee, S. (2021). Exploratory analysis of cooling effect of urban wetlands on Kolkata metropolitan city region, eastern India. Curr. Res. Environ. Sustain. 3, 100066. doi: 10.1016/j.crsust.2021.100066
Berland, A., Shiflett, S. A., Shuster, W. D., Garmestani, A. S., Goddard, H. C., Herrmann, D. L., et al. (2017). The role of trees in urban stormwater management. Landsc. Urban Plan. 162, 167–177. doi: 10.1016/j.landurbplan.2017.02.017
Berry, R., Livesley, S. J., and Aye, L. (2013). Tree canopy shade impacts on solar irradiance received by building walls and their surface temperature. Build. Environ. 69, 91–100. doi: 10.1016/j.buildenv.2013.07.009
Bett, B., Kiunga, P., Gachohi, J., Sindato, C., Mbotha, D., Robinson, T., et al. (2017). Effects of climate change on the occurrence and distribution of livestock diseases. Prev. Vet. Med. 137, 119–129. doi: 10.1016/j.prevetmed.2016.11.019
Bhatnagar, M., Taneja, S., and Özen, E. (2022). A wave of green start-ups in India—The study of green finance as a support system for sustainable entrepreneurship. Green Finan. 4, 253–273. doi: 10.3934/GF.2022012
Bherwani, H., Banerji, T., and Menon, R. (2022). Role and value of urban forests in carbon sequestration: review and assessment in Indian context. Environ. Dev. Sustain. 1–24. doi: 10.1007/s10668-022-02725-5
Bidwell, R. G. S., and Fraser, D. E. (1972). Carbon monoxide uptake and metabolism by leaves. Can. J. Bot. 50, 1435–1439. doi: 10.1139/b72-174
Blekking, J., Giroux, S., Waldman, K., Battersby, J., Tuholske, C., Robeson, S. M., et al. (2022). The impacts of climate change and urbanization on food retailers in urban sub-Saharan Africa. Curr. Opin. Environ. Sustain. 55, 101169. doi: 10.1016/j.cosust.2022.101169
Bohn, K., and Viljoen, A. (2011). The edible city: envisioning the continuous productive urban landscape (CPUL). Field J. 4, 149–161.
Bolleter, J., and Hooper, P. (2021). The importance of place-based narrative in suburban forest planning. J. Urban Des. 26, 209–234. doi: 10.1080/13574809.2020.1851594
Bolund, P., and Hunhammar, S. (1999). Ecosystem services in urban areas. Ecol. Econ. 29, 293–301. doi: 10.1016/S0921-8009(99)00013-0
Boni, R. (2019). Heat stress, a serious threat to reproductive function in animals and humans. Mol. Reprod. Dev. 86, 1307–1323. doi: 10.1002/mrd.23123
Brandt, L., Lewis, A. D., Fahey, R., Scott, L., Darling, L., and Swanston, C. (2016). A framework for adapting urban forests to climate change. Environ. Sci. Policy 66, 393–402. doi: 10.1016/j.envsci.2016.06.005
Brune, M. (2016). Urban Trees Under Climate Change: Potential Impacts of Dry Spells and Heat Waves in Three German Regions in the 2050s (Doctoral dissertation). Hamburg: Climate Service Center Germany (GERICS).
Bulkeley, H., and Betsill, M. M. (2013). Revisiting the urban politics of climate change. Environ. Polit. 22, 136–154. doi: 10.1080/09644016.2013.755797
Bustamante, M. M., Silva, J. S., Scariot, A., Sampaio, A. B., Mascia, D. L., Garcia, E., et al. (2019). Ecological restoration as a strategy for mitigating and adapting to climate change: lessons and challenges from Brazil. Mitigat. Adapt. Strat. Global Change 24, 1249–1270. doi: 10.1007/s11027-018-9837-5
Butt, N., Shanahan, D. F., Shumway, N., Bekessy, S. A., Fuller, R. A., Watson, J. E., et al. (2018). Opportunities for biodiversity conservation as cities adapt to climate change. Geogr. Environ. 5, e00052. doi: 10.1002/geo2.52
Buttschardt, T. (2005). “Long-term development of extensive green roofs: Are they suitable as permanent habitat for soil meso-and macrofauna,” in International Green Roof Congress (Berlin: International Green Roof Association), 189–198.
Bytnerowicz, A. (1996). Physiological aspects of air pollution stress in forests. Phyton Horn 36, 15–22.
Cabaraban, M. T. I., Kroll, C. N., Hirabayashi, S., and Nowak, D. J. (2013). Modeling of air pollutant removal by dry deposition to urban trees using a WRF/CMAQ/i-Tree Eco coupled system. Environ. Pollut. 176, 123–133. doi: 10.1016/j.envpol.2013.01.006
Calfapietra, C., Fares, S., Manes, F., Morani, A., Sgrigna, G., and Loreto, F. (2013). Role of Biogenic Volatile Organic Compounds (BVOC) emitted by urban trees on ozone concentration in cities: a review. Environ. Pollut. 183, 71–80. doi: 10.1016/j.envpol.2013.03.012
Camille, F. (2018). Grow what you eat eat what you grow: urban agriculture as middle class intervention in India. J. Political. Ecol. 25, 222–238.
Cârlan, I., Haase, D., Große-Stoltenberg, A., and Sandric, I. (2020). Mapping heat and traffic stress of urban park vegetation based on satellite imagery-A comparison of Bucharest, Romania and Leipzig, Germany. Urban Ecosyst. 23, 363–377. doi: 10.1007/s11252-019-00916-z
Carlyle-Moses, D. E., Livesley, S., Baptista, M. D., Thom, J., and Szota, C. (2020). Urban trees as green infrastructure for stormwater mitigation and use. For. Water Interact. 397–432. doi: 10.1007/978-3-030-26086-6_17
Carper, A. L., Warren, P. S., Adler, L. S., and Irwin, R. E. (2022). Pollen limitation of native plant reproduction in an urban landscape. Am. J. Bot. 109, 1969–1980. doi: 10.1002/ajb2.16080
Chan, N. W., Tan, M. L., Ghani, A. A., and Zakaria, N. A. (2019). “Sustainable urban drainage as a viable measure of coping with heat and floods due to climate change,” in IOP Conference Series: Earth and Environmental Science, Vol. 257 (IOP Publishing), 012013.
Chari, F., and Ngcamu, B. S. (2022). Climate change-related hazards and livestock industry performance in (peri-) urban areas: a case of the City of Masvingo, Zimbabwe. Climate 10, 187. doi: 10.3390/cli10120187
Chaturvedi, P., Wiese, A. J., Ghatak, A., Zaveska Drabkova, L., Weckwerth, W., and Honys, D. (2021). Heat stress response mechanisms in pollen development. New Phytol. 231, 571–585. doi: 10.1111/nph.17380
Chelleri, L., Waters, J. J., Olazabal, M., and Minucci, G. (2015). Resilience trade-offs: addressing multiple scales and temporal aspects of urban resilience. Environ. Urban. 27, 181–198. doi: 10.1177/0956247814550780
Chen, W. Y. (2015). The role of urban green infrastructure in offsetting carbon emissions in 35 major Chinese cities: a nationwide estimate. Cities 44, 112–120. doi: 10.1016/j.cities.2015.01.005
Choudaj, K., and Wankhade, V. (2023). Study of the interrelationship between woody plants and birds in Pune urban area, insights on negative impacts of exotic plants. Trop. Ecol. 64, 264–275. doi: 10.1007/s42965-022-00269-3
Cilliers, S., Cilliers, J., Lubbe, R., and Siebert, S. (2013). Ecosystem services of urban green spaces in African countries—perspectives and challenges. Urban Ecosyst. 16, 681–702. doi: 10.1007/s11252-012-0254-3
Coaffee, J., Wood, D. M., and Rogers, P. (2009). The Everyday Resilience of the City. Basingstoke: Palgrave Macmillan, 9780230583337.
Colla, S. R., Willis, E., and Packer, L. (2009). Can green roofs provide habitat for urban bees (Hymenoptera: Apidae)?. Cities Environ. 2, 4. doi: 10.15365/cate.2142009
Conflitti, I. M., Arshad Imrit, M., Morrison, B., Sharma, S., Colla, S. R., and Zayed, A. (2022). Bees in the six: determinants of bumblebee habitat quality in urban landscapes. Ecol. Evol. 12, e8667. doi: 10.1002/ece3.8667
Coutts, A. M., White, E. C., Tapper, N. J., Beringer, J., and Livesley, S. J. (2016). Temperature and human thermal comfort effects of street trees across three contrasting street canyon environments. Theoret. Appl. Climatol. 124, 55–68. doi: 10.1007/s00704-015-1409-y
Cox, D. T., Shanahan, D. F., Hudson, H. L., Plummer, K. E., Siriwardena, G. M., Fuller, R. A., et al. (2017). Doses of neighborhood nature: the benefits for mental health of living with nature. AIBS Bull. 67, 147–155. doi: 10.1093/biosci/biw173
Crescio, M. I., Forastiere, F., Maurella, C., Ingravalle, F., and Ru, G. (2010). Heat-related mortality in dairy cattle: a case crossover study. Prev. Vet. Med. 97, 191–197. doi: 10.1016/j.prevetmed.2010.09.004
Crossman, N. D., Burkhard, B., Nedkov, S., Willemen, L., Petz, K., Palomo, I., et al. (2013). A blueprint for mapping and modelling ecosystem services. Ecosyst. Serv. 4, 4–14. doi: 10.1016/j.ecoser.2013.02.001
Crowther, T. W., Boddy, L., and Jones, T. H. (2011). Outcomes of fungal interactions are determined by soil invertebrate grazers. Ecol. Lett. 14, 1134–1142. doi: 10.1111/j.1461-0248.2011.01682.x
Czaja, M., Kołton, A., and Muras, P. (2020). The complex issue of urban trees—Stress factor accumulation and ecological service possibilities. Forests 11, 932. doi: 10.3390/f11090932
Dai, P., Zhang, S., Gong, Y., Zhou, Y., and Hou, H. (2022). A crowd-sourced valuation of recreational ecosystem services using mobile signal data applied to a restored wetland in China. Ecol. Econ. 192, 107249. doi: 10.1016/j.ecolecon.2021.107249
D'Amato, G. (2011). Effects of climatic changes and urban air pollution on the rising trends of respiratory allergy and asthma. Multidiscip. Respir. Med. 6, 1–10. doi: 10.1186/2049-6958-6-1-28
Davidson, E. A., and Janssens, I. A. (2006). Temperature sensitivity of soil carbon decomposition and feedbacks to climate change. Nature 440, 165–173. doi: 10.1038/nature04514
Davoren, E., and Shackleton, C. M. (2021). Urban ecosystem disservices in the Global South. Urban Ecol. Global South 265–292. doi: 10.1007/978-3-030-67650-6_11
de Felicio Porcionato, M. A., Fernandes, A. M., Netto, A. S., and dos Santos, M. V. (2009). Influence of heat stress on milk yield and quality. Rev. Acad. Ciênc. Agrár. Ambient 7, 483–490. doi: 10.7213/cienciaanimal.v7i4.10292
Debnath, R., Bardhan, R., and Bell, M. L. (2023). Lethal heatwaves are challenging India's sustainable development. PLOS Clim. 2, e0000156. doi: 10.1371/journal.pclm.0000156
Depietri, Y., Renaud, F. G., and Kallis, G. (2012). Heat waves and floods in urban areas: a policy-oriented review of ecosystem services. Sustain. Sci. 7, 95–107. doi: 10.1007/s11625-011-0142-4
Dhavale, S., Mujumdar, M., Roxy, M. K., and Singh, V. K. (2022). Tropical cyclones over the Arabian Sea during the monsoon onset phase. Int. J. Climatol. 42, 2996–3006. doi: 10.1002/joc.7403
Dorin, A., Taylor, T., and Dyer, A. G. (2022). Goldilocks' quarter-hectare urban farm: an agent-based model for improved pollination of community gardens and small-holder farms. PLOS Sustain. Transform. 1, e0000021. doi: 10.1371/journal.pstr.0000021
Dotaniya, M. L., Rajendiran, S., Meena, B. P., Meena, A. L., Dotaniya, C. K., Meena, B. L., et al. (2016). “Elevated carbon dioxide (CO2) and temperature vis-a-vis carbon sequestration potential of global terrestrial ecosystem,” in Conservation Agriculture, eds J. Bisht, V. Meena, P. Mishra, and A. Pattanayak, 225–256. doi: 10.1007/978-981-10-2558-7_9
Douglas, I. (2010). “The role of green infrastructure in adapting cities to climate change,” in The Routledge Handbook of Urban Ecology (Routledge), 607–612.
Dubbeling, M. (2015). Integrating Urban Agriculture and Forestry Into Climate Change Action Plans: Lessons from Western Province, Sri Lanka and Rosario, Argentina. Green Ink: Resource Center on Urban Agriculture and Food Safety, 1–17.
Dubey, R., Pathak, H., Chakrabarti, B., Singh, S., Gupta, D. K., and Harit, R. C. (2020). Impact of terminal heat stress on wheat yield in India and options for adaptation. Agric. Syst. 181, 102826. doi: 10.1016/j.agsy.2020.102826
Dusza, Y., Kraepiel, Y., Abbadie, L., Barot, S., Carmignac, D., Dajoz, I., et al. (2020). Plant-pollinator interactions on green roofs are mediated by substrate characteristics and plant community composition. Acta Oecol. 105, 103559. doi: 10.1016/j.actao.2020.103559
Dwaikat, L. N., and Ali, K. N. (2018). The economic benefits of a green building–evidence from Malaysia. J. Build. Eng. 18, 448–453. doi: 10.1016/j.jobe.2018.04.017
Elbasiouny, H., El-Ramady, H., Elbehiry, F., Rajput, V. D., Minkina, T., and Mandzhieva, S. (2022). Plant nutrition under climate change and soil carbon sequestration. Sustainability 14, 914. doi: 10.3390/su14020914
Elmqvist, T., Setäl,ä, H., Handel, S. N., van der Ploeg, S., Aronson, J., Blignaut, J. N., et al. (2015). Benefits of restoring ecosystem services in urban areas. Curr. Opin. Environ. Sustain. 14, 101–108. doi: 10.1016/j.cosust.2015.05.001
Escobedo, F., Varela, S., Zhao, M., Wagner, J. E., and Zipperer, W. (2010). Analyzing the efficacy of subtropical urban forests in offsetting carbon emissions from cities. Environ. Sci. Policy 13, 362–372. doi: 10.1016/j.envsci.2010.03.009
Escobedo, F. J., Luley, C. J., Bond, J., Staudhammer, C., and Bartel, C. (2009). Hurricane debris and damage assessment for Florida urban forests. J. Arboricult. 35, 100. doi: 10.48044/jauf.2009.018
Escobedo, F. J., and Nowak, D. J. (2009). Spatial heterogeneity and air pollution removal by an urban forest. Landsc. Urban Plan. 90, 102–110. doi: 10.1016/j.landurbplan.2008.10.021
Esperon-Rodriguez, M., Power, S. A., Tjoelker, M. G., Marchin, R. M., and Rymer, P. D. (2021). Contrasting heat tolerance of urban trees to extreme temperatures during heatwaves. Urban Forest. Urban Green. 66, 127387. doi: 10.1016/j.ufug.2021.127387
Estoque, R. C., Murayama, Y., and Myint, S. W. (2017). Effects of landscape composition and pattern on land surface temperature: an urban heat island study in the megacities of Southeast Asia. Sci. Total Environ. 577, 349–359. doi: 10.1016/j.scitotenv.2016.10.195
Evans, G. W. (2019). Projected behavioral impacts of global climate change. Annu. Rev. Psychol. 70, 449–474. doi: 10.1146/annurev-psych-010418-103023
FAO (2012). The State of Food Insecurity in the World. Food and Agriculture Organization of the United Nations. Available online at: https://www.fao.org/3/i2845e/i2845e00.pdf (accessed August 17, 2023).
FAO (2014). The State of Food and Agriculture. Food and Agriculture Organization of the United Nations. Available online at: https://www.fao.org/3/i4040e/i4040e.pdf (accessed August 17, 2023).
Fernández Cañero, R., and González Redondo, P. (2010). Green roofs as a habitat for birds: a review. J. Anim. Vet. Adv. 9, 2041–2052. doi: 10.3923/javaa.2010.2041.2052
Ferrini, F., Bussotti, F., Tattini, M., and Fini, A. (2014). Trees in the Urban Environment: Response Mechanisms and Benefits for the Ecosystem Should Guide Plant Selection for Future Plantings. Agrochimica, Pisa University Press. Italy 3, 234–246.
Fitzky, A. C., Sandén, H., Karl, T., Fares, S., Calfapietra, C., Grote, R., et al. (2019). The interplay between ozone and urban vegetation—BVOC emissions, ozone deposition, and tree ecophysiology. Front. For. Global Change 2, 50. doi: 10.3389/ffgc.2019.00050
Forbes, E. I. (2016). On the frontier of urbanization: informal settlements in Yangon, Myanmar. Ind. J. Burmese Scholar. 1, 197–238.
Forkuor, G., and Cofie, O. (2011). Dynamics of land-use and land-cover change in Freetown, Sierra Leone and its effects on urban and peri-urban agriculture–a remote sensing approach. Int. J. Remote Sens. 32, 1017–1037. doi: 10.1080/01431160903505302
Frumkin, H., McMichael, A. J., and Hess, J. J. (2008). Climate change and the health of the public. Am. J. Prev. Med. 35, 401–402. doi: 10.1016/j.amepre.2008.08.031
Furukawa, K. (2013). Case studies for urban wetlands restoration and management in Japan. Ocean Coast. Manag. 81, 97–102. doi: 10.1016/j.ocecoaman.2012.07.012
Galloway, J. N., Townsend, A. R., Erisman, J. W., Bekunda, M., Cai, Z., Freney, J. R., et al. (2008). Transformation of the nitrogen cycle: recent trends, questions, and potential solutions. Science 320, 889–892. doi: 10.1126/science.1136674
Garcia, D. A. (2017). Green areas management and bioengineering techniques for improving urban ecological sustainability. Sustain. Cities Soc. 30, 108–117. doi: 10.1016/j.scs.2017.01.008
Geslin, B., Gauzens, B., Thébault, E., and Dajoz, I. (2013). Plant pollinator networks along a gradient of urbanisation. PLoS ONE 8, e63421. doi: 10.1371/journal.pone.0063421
Ghosh, A., Agrawal, M., and Agrawal, S. B. (2020). Effect of water deficit stress on an Indian wheat cultivar (Triticum aestivum L. HD 2967) under ambient and elevated level of ozone. Sci. Total Environ. 714, 136837. doi: 10.1016/j.scitotenv.2020.136837
Ghosh, A., Agrawal, M., and Agrawal, S. B. (2021). Examining the effectiveness of biomass-derived biochar for the amelioration of tropospheric ozone-induced phytotoxicity in the Indian wheat cultivar HD 2967. J. Hazard. Mater. 408, 124968. doi: 10.1016/j.jhazmat.2020.124968
Ghosh, A., Singh, A. A., Agrawal, M., and Agrawal, S. B. (2018). Ozone toxicity and remediation in crop plants. Sustain. Agric. Rev. 27, 129–169. doi: 10.1007/978-3-319-75190-0_5
Gill, S. E., Handley, J. F., Ennos, A. R., and Pauleit, S. (2007). Adapting cities for climate change: the role of the green infrastructure. Built Environ. 33, 115–133. doi: 10.2148/benv.33.1.115
Gómez-Baggethun, E., Gren, Å., Barton, D. N., Langemeyer, J., McPhearson, T., O'farrell, P., et al. (2013). Urban ecosystem services,” in Urbanization, Biodiversity and Ecosystem Services: Challenges and Opportunities, eds T. Elmqvist, M. Fragkias, J. Goodness, B. Güneralp, P. J. Marcotullio, R. I. McDonald, S. Parnell, M. Schewenius, M. Sendstad, K. C. Seto, and C. Wilkinson, 175–251. doi: 10.1007/978-94-007-7088-1_11
Gotham, K. F., and Brumley, K. (2002). Using space: agency and identity in a public–housing development. City Commun. 1, 267–289. doi: 10.1111/1540-6040.00023
Gou, Z., and Xie, X. (2017). Evolving green building: triple bottom line or regenerative design?. J. Clean. Prod. 153, 600–607. doi: 10.1016/j.jclepro.2016.02.077
GRIHA Manual (2010). Introduction to National Rating System – GRIHA An Evaluation Tool to Help Design, Build, Operate, and Maintain a Resource-Efficient Built Environment. Ministry of New and Renewable Energy, Government of India, and The Energy and Resources Institute. Available online at: http://www.grihaindia.org/files/Manual_VolI.pdf/ (accessed September 24, 2023).
Grimm, N. B., Foster, D., Groffman, P., Grove, J. M., Hopkinson, C. S., Nadelhoffer, K. J., et al. (2008). The changing landscape: ecosystem responses to urbanization and pollution across climatic and societal gradients. Front. Ecol. Environ. 6, 264–272. doi: 10.1890/070147
Grote, R., Samson, R., Alonso, R., Amorim, J. H., Cariñanos, P., Churkina, G., et al. (2016). Functional traits of urban trees: air pollution mitigation potential. Front. Ecol. Environ. 14, 543–550. doi: 10.1002/fee.1426
Guntukula, R. (2020). Assessing the impact of climate change on Indian agriculture: evidence from major crop yields. J. Public Aff. 20, e2040. doi: 10.1002/pa.2040
Guzman, L. A., and Bocarejo, J. P. (2017). Urban form and spatial urban equity in Bogota, Colombia. Transport. Res. Proc. 25, 4491–4506. doi: 10.1016/j.trpro.2017.05.345
Haase, D., Frantzeskaki, N., and Elmqvist, T. (2014). Ecosystem services in urban landscapes: practical applications and governance implications. Ambio 43, 407–412. doi: 10.1007/s13280-014-0503-1
Haase, D., and Hellwig, R. (2022). Effects of heat and drought stress on the health status of six urban street tree species in Leipzig, Germany. Trees For. People 8, 100252. doi: 10.1016/j.tfp.2022.100252
Haberman, D., Gillies, L., Canter, A., Rinner, V., Pancrazi, L., and Martellozzo, F. (2014). The potential of urban agriculture in Montréal: a quantitative assessment. ISPRS Int. J. Geo Inf. 3, 1101–1117. doi: 10.3390/ijgi3031101
Habibullah, M. S., Din, B. H., Tan, S. H., and Zahid, H. (2022). Impact of climate change on biodiversity loss: global evidence. Environ. Sci. Pollut. Res. 29, 1073–1086. doi: 10.1007/s11356-021-15702-8
Hall, D. M., Camilo, G. R., Tonietto, R. K., Ollerton, J., Ahrné, K., Arduser, M., et al. (2017). The city as a refuge for insect pollinators. Conserv. Biol. 31, 24–29. doi: 10.1111/cobi.12840
Hansen, R., Frantzeskaki, N., McPhearson, T., Rall, E., Kabisch, N., Kaczorowska, A., et al. (2015). The uptake of the ecosystem services concept in planning discourses of European and American cities. Ecosyst. Serv. 12, 228–246. doi: 10.1016/j.ecoser.2014.11.013
Hara, Y., McPhearson, T., Sampei, Y., and McGrath, B. (2018). Assessing urban agriculture potential: a comparative study of Osaka, Japan and New York city, United States. Sustain. Sci. 13, 937–952. doi: 10.1007/s11625-018-0535-8
He, N., Wen, D., Zhu, J., Tang, X., Xu, L., Zhang, L., et al. (2017). Vegetation carbon sequestration in Chinese forests from 2010 to 2050. Glob. Chang. Biol. 23, 1575–1584. doi: 10.1111/gcb.13479
Hedhly, A. (2011). Sensitivity of flowering plant gametophytes to temperature fluctuations. Environ. Exp. Bot. 74, 9–16. doi: 10.1016/j.envexpbot.2011.03.016
Hendricks, M. D., and Dowtin, A. L. (2023). Come hybrid or high water: making the case for a Green–Gray approach toward resilient urban stormwater management. J. Am. Water Resour. Assoc. 59, 885–893. doi: 10.1111/1752-1688.13112
Herrera, J. M., Ploquin, E. F., Rodríguez-Pérez, J., and Obeso, J. R. (2014). Determining habitat suitability for bumblebees in a mountain system: a baseline approach for testing the impact of climate change on the occurrence and abundance of species. J. Biogeogr. 41, 700–712. doi: 10.1111/jbi.12236
Hettiarachchi, M., and Morrison, T. H. (2017). A Tale of Two Cities: Similar Ecologies and Diverging Governance of Urban Fisheries in Kolkata and Colombo. St. John's, NF: Intersectoral Governance of Inland Fisheries. Too Big To Ignore-WorldFish, 58–70.
Hill, M. J., Biggs, J., Thornhill, I., Briers, R. A., Gledhill, D. G., White, J. C., et al. (2017). Urban ponds as an aquatic biodiversity resource in modified landscapes. Glob. Change Biol. 23, 986–999. doi: 10.1111/gcb.13401
Holmes, T. P., Aukema, J. E., Von Holle, B., Liebhold, A., and Sills, E. (2009). Economic impacts of invasive species in forests: past, present, and future. Ann. N. Y. Acad. Sci. 1162, 18–38. doi: 10.1111/j.1749-6632.2009.04446.x
Høye, T. T., Post, E., Schmidt, N. M., Trøjelsgaard, K., and Forchhammer, M. C. (2013). Shorter flowering seasons and declining abundance of flower visitors in a warmer Arctic. Nat. Clim. Chang. 3, 759–763. doi: 10.1038/nclimate1909
Hoyer, J., Dickhaut, W., Kronawitter, L., and Weber, B. (2011). Water Sensitive Urban Design: Principles and Inspiration for Sustainable Stormwater Management in the City of the Future. Berlin: Jovis, 18–20.
Hsieh, C. M., Li, J. J., Zhang, L., and Schwegler, B. (2018). Effects of tree shading and transpiration on building cooling energy use. Energy Build. 159, 382–397. doi: 10.1016/j.enbuild.2017.10.045
Irga, P. J., Burchett, M. D., and Torpy, F. R. (2015). Does urban forestry have a quantitative effect on ambient air quality in an urban environment? Atmos. Environ. 120, 173–181. doi: 10.1016/j.atmosenv.2015.08.050
Islam, M., Al Nayeem, A., Majumder, A. K., and Elahi, K. T. (2019). Study on the status of roof top gardening in selected residential areas of Dhaka City, Bangladesh. Malays. J. Sustain. Agric 3, 31–34. doi: 10.26480/mjsa.02.2019.31.34
Jalaludin, B. B., O'Toole, B. I., and Leeder, S. R. (2004). Acute effects of urban ambient air pollution on respiratory symptoms, asthma medication use, and doctor visits for asthma in a cohort of Australian children. Environ. Res. 95, 32–42. doi: 10.1016/S0013-9351(03)00038-0
Jansson, Å., and Polasky, S. (2010). Quantifying biodiversity for building resilience for food security in urban landscapes: getting down to business. Ecol. Soc. 15, 20. doi: 10.5751/ES-03520-150320
Jeanjean, A. P., Buccolieri, R., Eddy, J., Monks, P. S., and Leigh, R. J. (2017). Air quality affected by trees in real street canyons: the case of Marylebone neighbourhood in central London. Urban Forest. Urban Green. 22, 41–53. doi: 10.1016/j.ufug.2017.01.009
Jerome, G., Sinnett, D., Burgess, S., Calvert, T., and Mortlock, R. (2019). A framework for assessing the quality of green infrastructure in the built environment in the UK. Urban Forest. Urban Green. 40, 174–182. doi: 10.1016/j.ufug.2019.04.001
Jo, H. K., and McPherson, E. G. (2001). Indirect carbon reduction by residential vegetation and planting strategies in Chicago, USA. J. Environ. Manage. 61, 165–177. doi: 10.1006/jema.2000.0393
Jones, J. A., Wei, X., Archer, E., Bishop, K., Blanco, J. A., Ellison, D., et al. (2020). “Forest-water interactions under global change,” in Forest-Water Interaction. Ecological Studies, eds D. F. Levia, D. E. Carlyle-Moses, S. Iida, B. Michalzik, K. Nanko, and A. Tischer (Cham: Springer), 240, 589–624. doi: 10.1007/978-3-030-26086-6_24
Kabisch, N. (2015). Ecosystem service implementation and governance challenges in urban green space planning—The case of Berlin, Germany. Land Use Policy 42, 557–567. doi: 10.1016/j.landusepol.2014.09.005
Kabisch, N., Kraemer, R., Brenck, M. E., Haase, D., Lausch, A., Luttkus, M. L., et al. (2021). A methodological framework for the assessment of regulating and recreational ecosystem services in urban parks under heat and drought conditions. Ecosyst. People 17, 464–475. doi: 10.1080/26395916.2021.1958062
Kabisch, N., Strohbach, M., Haase, D., and Kronenberg, J. (2016). Urban green space availability in European cities. Ecol. Indic. 70, 586–596. doi: 10.1016/j.ecolind.2016.02.029
Kaplan, R. (1985). The analysis of perception via preference: a strategy for studying how the environment is experienced. Landsc. Plann. 12, 161–176. doi: 10.1016/0304-3924(85)90058-9
Karagulian, F., Belis, C. A., Dora, C. F. C., Prüss-Ustün, A. M., Bonjour, S., Adair-Rohani, H., et al. (2015). Contributions to cities' ambient particulate matter (PM): a systematic review of local source contributions at global level. Atmos. Environ. 120, 475–483. doi: 10.1016/j.atmosenv.2015.08.087
Kaul, M., Mohren, G. M. J., and Dadhwal, V. K. (2010). Carbon storage and sequestration potential of selected tree species in India. Mitigat. Adapt. Strat. Glob. Change 15, 489–510. doi: 10.1007/s11027-010-9230-5
Kaushik, M., Tiwari, S., and Manisha, K. (2022). Habitat patch size and tree species richness shape the bird community in urban green spaces of rapidly urbanizing Himalayan foothill region of India. Urban Ecosyst. 25, 423–436. doi: 10.1007/s11252-021-01165-9
Kazmierczak, A. (2013). The contribution of local parks to neighbourhood social ties. Landsc. Urban Plan. 109, 31–44. doi: 10.1016/j.landurbplan.2012.05.007
Keeler, B. L., Dalzell, B. J., Gourevitch, J. D., Hawthorne, P. L., Johnson, K. A., and Noe, R. R. (2019). Putting people on the map improves the prioritization of ecosystem services. Front. Ecol. Environ. 17, 151–156. doi: 10.1002/fee.2004
Kettunen, M., and D'Amato, D. (2013). “Provisioning services and related goods,” in The Social and Economic Benefits of Protected areas: An Assessment Guide (Earthscan).
Khan, S. M., Page, S., Ahmad, H., Shaheen, H., Ullah, Z., Ahmad, M., et al. (2013). Medicinal flora and ethnoecological knowledge in the Naran Valley, Western Himalaya, Pakistan. J. Ethnobiol. Ethnomed. 9, 1–13. doi: 10.1186/1746-4269-9-4
Kim, G. (2017). An integrated system of urban green infrastructure on different types of vacant land to provide multiple benefits for local communities. Sustain. Cities Soc. 36, 116–130. doi: 10.1016/j.scs.2017.10.022
Kiss, M., Takács, Á., Pogácsás, R., and Gulyás, Á. (2015). The role of ecosystem services in climate and air quality in urban areas: evaluating carbon sequestration and air pollution removal by street and park trees in Szeged (Hungary). Moravian Geogr. Rep. 23, 36–46. doi: 10.1515/mgr-2015-0016
Klein, L. J., Zhou, W., and Albrecht, C. M. (2021). Quantification of carbon sequestration in urban forests. arXiv [Preprint]. arXiv:2106.00182. doi: 10.48550/arXiv.2106.00182
Klemm, W., Heusinkveld, B. G., Lenzholzer, S., Jacobs, M. H., and Van Hove, B. (2015). Psychological and physical impact of urban green spaces on outdoor thermal comfort during summertime in The Netherlands. Build. Environ. 83, 120–128. doi: 10.1016/j.buildenv.2014.05.013
Koller, E. K., and Phoenix, G. K. (2017). Seasonal dynamics of soil and plant nutrients at three environmentally contrasting sites along a sub-Arctic catchment sequence. Polar Biol. 40, 1821–1834. doi: 10.1007/s00300-017-2105-4
Konarska, J., Uddling, J., Holmer, B., Lutz, M., Lindberg, F., Pleijel, H., et al. (2016). Transpiration of urban trees and its cooling effect in a high latitude city. Int. J. Biometeorol. 60, 159–172. doi: 10.1007/s00484-015-1014-x
Krivtsov, V., Forbes, H., Birkinshaw, S., Olive, V., Chamberlain, D., Buckman, J., et al. (2022). Ecosystem services provided by urban ponds and green spaces: a detailed study of a semi-natural site with global importance for research. Blue Green Syst. 4, 1–23. doi: 10.2166/bgs.2022.021
Ksiazek, K., Tonietto, R., and Ascher, J. S. (2014). Ten bee species new to green roofs in the Chicago area. Great Lakes Entomol. 47, 13. doi: 10.22543/0090-0222.2295
Lancaster, T. (2006). Planning and Implementation of Sustainable Stormwater Management Systems in the City of Vancouver: The Green Roof Example (Doctoral dissertation). University of British Columbia, Canada.
Landry, S. M., Koeser, A. K., Kane, B., Hilbert, D. R., McLean, D. C., Andreu, M., et al. (2021). Urban forest response to Hurricane Irma: the role of landscape characteristics and sociodemographic context. Urban For. Urban Green. 61, 127093. doi: 10.1016/j.ufug.2021.127093
Lara-Valencia, F., and García-Pérez, H. (2015). Space for equity: socioeconomic variations in the provision of public parks in Hermosillo, Mexico. Local Environ. 20, 350–368. doi: 10.1080/13549839.2013.857647
Lee, H., Mayer, H., and Chen, L. (2016). Contribution of trees and grasslands to the mitigation of human heat stress in a residential district of Freiburg, Southwest Germany. Landsc. Urban Plan. 148, 37–50. doi: 10.1016/j.landurbplan.2015.12.004
Lerdau, M., Guenther, A., and Monson, R. (1997). Plant production and emission of volatile organic compounds. Bioscience 47, 373–383. doi: 10.2307/1313152
Li, L., Zhou, X., Chen, L., Chen, L., Zhang, Y., and Liu, Y. (2020). Estimating urban vegetation biomass from Sentinel-2A image data. Forests 11, 125. doi: 10.3390/f11020125
Li, X., Ratti, C., and Seiferling, I. (2018). Quantifying the shade provision of street trees in urban landscape: a case study in Boston, USA, using Google Street View. Landsc. Urban Plan. 169, 81–91. doi: 10.1016/j.landurbplan.2017.08.011
Lin, P., Lau, S. S. Y., Qin, H., and Gou, Z. (2017). Effects of urban planning indicators on urban heat island: a case study of pocket parks in high-rise high-density environment. Landsc. Urban Plan. 168, 48–60. doi: 10.1016/j.landurbplan.2017.09.024
Linden, J., Gustafsson, M., Uddling, J., and Pleijel, H. (2023). Air pollution removal through deposition on urban vegetation: the importance of vegetation characteristics. Urban For. Urban Green. 81, 127843. doi: 10.1016/j.ufug.2023.127843
Lipton, D., Rubenstein, M. A., Weiskopf, S. R., Carter, S. L., Peterson, J., Crozier, L., et al. (2018). Ecosystems, Ecosystem Services, and Biodiversity. Washington, DC: US Global Change Research Program, 268–321.
Liu, C., and Li, X. (2012). Carbon storage and sequestration by urban forests in Shenyang, China. Urban For. Urban Green. 11, 121–128. doi: 10.1016/j.ufug.2011.03.002
Liu, J., and Slik, F. (2022). Are street trees friendly to biodiversity?. Landsc. Urban Plan. 218, 104304. doi: 10.1016/j.landurbplan.2021.104304
Livesley, S. J., McPherson, E. G., and Calfapietra, C. (2016). The urban forest and ecosystem services: impacts on urban water, heat, and pollution cycles at the tree, street, and city scale. J. Environ. Qual. 45, 119–124. doi: 10.2134/jeq2015.11.0567
Loder, A. (2014). ‘There's a meadow outside my workplace': a phenomenological exploration of aesthetics and green roofs in Chicago and Toronto. Landsc. Urban Plan. 126, 94–106. doi: 10.1016/j.landurbplan.2014.01.008
Lotze-Campen, H. (2011). “Climate change, population growth, and crop production: an overview,” in Crop Adaption to Climate Change, eds S. S. Yadav, R. J. Redden, J. L. Hatfield, H. Lotze-Campen, and A. E. Hall, 1–11. doi: 10.1002/9780470960929.ch1
MacIvor, J. S., and Lundholm, J. (2011). Insect species composition and diversity on intensive green roofs and adjacent level-ground habitats. Urban Ecosyst. 14, 225–241. doi: 10.1007/s11252-010-0149-0
Makinson, J. C., Threlfall, C. G., and Latty, T. (2017). Bee-friendly community gardens: impact of environmental variables on the richness and abundance of exotic and native bees. Urban Ecosyst. 20, 463–476. doi: 10.1007/s11252-016-0607-4
Malone, Z., Berhe, A. A., and Ryals, R. (2023). Impacts of organic matter amendments on urban soil carbon and soil quality: a meta-analysis. J. Clean. Prod. 419, 138148. doi: 10.1016/j.jclepro.2023.138148
Manning, W. J. (2008). Plants in urban ecosystems: essential role of urban forests in urban metabolism and succession toward sustainability. Int. J. Sustain. Dev. World Ecol. 15, 362–370. doi: 10.3843/SusDev.15.4:12
Marchin, R. M., Esperon-Rodriguez, M., Tjoelker, M. G., and Ellsworth, D. S. (2022). Crown dieback and mortality of urban trees linked to heatwaves during extreme drought. Sci. Total Environ. 850, 157915. doi: 10.1016/j.scitotenv.2022.157915
Masson, V., Marchadier, C., Adolphe, L., Aguejdad, R., Avner, P., Bonhomme, M., et al. (2014). Adapting cities to climate change: a systemic modelling approach. Urban Clim. 10, 407–429. doi: 10.1016/j.uclim.2014.03.004
Matthews, T., Lo, A. Y., and Byrne, J. A. (2015). Reconceptualizing green infrastructure for climate change adaptation: barriers to adoption and drivers for uptake by spatial planners. Landsc. Urban Plan. 138, 155–163. doi: 10.1016/j.landurbplan.2015.02.010
Mavrogianni, A., Davies, M., Taylor, J., Chalabi, Z., Biddulph, P., Oikonomou, E., et al. (2014). The impact of occupancy patterns, occupant-controlled ventilation and shading on indoor overheating risk in domestic environments. Build. Environ. 78, 183–198. doi: 10.1016/j.buildenv.2014.04.008
McInnes, R. J., and Everard, M. (2017). Rapid assessment of wetland ecosystem services (RAWES): an example from Colombo, Sri Lanka. Ecosyst. Serv. 25, 89–105. doi: 10.1016/j.ecoser.2017.03.024
McIntyre, N. E., Rango, J., Fagan, W. F., and Faeth, S. H. (2001). Ground arthropod community structure in a heterogeneous urban environment. Landsc. Urban Plan. 52, 257–274. doi: 10.1016/S0169-2046(00)00122-5
McKinney, M. L. (2002). Urbanization, biodiversity, and conservation: the impacts of urbanization on native species are poorly studied, but educating a highly urbanized human population about these impacts can greatly improve species conservation in all ecosystems. Bioscience 52, 883–890. doi: 10.1641/0006-3568(2002)052[0883:UBAC]2.0.CO;2
McPhearson, T., Hamstead, Z. A., and Kremer, P. (2014). Urban ecosystem services for resilience planning and management in New York City. Ambio 43, 502–515. doi: 10.1007/s13280-014-0509-8
McPherson, E. G., Xiao, Q., and Aguaron, E. (2013). A new approach to quantify and map carbon stored, sequestered and emissions avoided by urban forests. Landsc. Urban Plan. 120, 70–84. doi: 10.1016/j.landurbplan.2013.08.005
Medina, R. G., Paxton, R. J., De Luna, E., Fleites-Ayil, F. A., Medina, L. A. M., and Quezada-Euán, J. J. G. (2018). Developmental stability, age at onset of foraging and longevity of Africanized honey bees (Apis mellifera L.) under heat stress (Hymenoptera: Apidae). J. Therm. Biol. 74, 214–225. doi: 10.1016/j.jtherbio.2018.04.003
Mell, I. C. (2009). “Can green infrastructure promote urban sustainability?,” in Proceedings of the Institution of Civil Engineers-Engineering Sustainability, Vol. 162 (Thomas Telford Ltd.), 23–34.
Middel, A., Chhetri, N., and Quay, R. (2015). Urban forestry and cool roofs: assessment of heat mitigation strategies in Phoenix residential neighborhoods. Urban For. Urban Green. 14, 178–186. doi: 10.1016/j.ufug.2014.09.010
Millennium Ecosystem Assessment (2005). Ecosystems and Human Well-being, Vol. 5. Washington, DC: Island Press. 563 p.
Moges, A., Beyene, A., Triest, L., Ambelu, A., and Kelbessa, E. (2018). Imbalance of ecosystem services of wetlands and the perception of the local community towards their restoration and management in Jimma Highlands, Southwestern Ethiopia. Wetlands 38, 1081–1095. doi: 10.1007/s13157-016-0743-x
Molina-Navarro, E., Trolle, D., Martínez-Pérez, S., Sastre-Merlín, A., and Jeppesen, E. (2014). Hydrological and water quality impact assessment of a Mediterranean limno-reservoir under climate change and land use management scenarios. J. Hydrol. 509, 354–366. doi: 10.1016/j.jhydrol.2013.11.053
Montague, T., Kjelgren, R., and Rupp, L. (2000). Surface energy balance affects gas exchange and growth of two irrigated landscape tree species in an arid climate. J. Am. Soc. Hortic. Sci. 125, 299–309. doi: 10.21273/JASHS.125.3.299
Mora, C., Frazier, A. G., Longman, R. J., Dacks, R. S., Walton, M. M., Tong, E. J., et al. (2013). The projected timing of climate departure from recent variability. Nature 502, 183–187. doi: 10.1038/nature12540
Moriwaki, R., Watanabe, K., and Morimoto, K. (2013). Urban dry island phenomenon and its impact on cloud base level. J. JSCE 1, 521–529. doi: 10.2208/journalofjsce.1.1_521
Morris, R., Davis, S., Grelet, G. A., and Gregorini, P. (2022). Multiscapes and urbanisation: the case for spatial agroecology. Sustainability 14, 1352. doi: 10.3390/su14031352
Moser, A., Uhl, E., Rötzer, T., Biber, P., Dahlhausen, J., Lefer, B., et al. (2017). Effects of climate and the urban heat island effect on urban tree growth in Houston. Open J. For. 7, 428–445. doi: 10.4236/ojf.2017.74026
Moser-Reischl, A., Uhl, E., Rötzer, T., Biber, P., Con, T. V., Tan, N. T., et al. (2018). Effects of the urban heat island and climate change on the growth of Khaya senegalensis in Hanoi, Vietnam. Forest Ecosyst. 5, 1–14. doi: 10.1186/s40663-018-0155-x
Mougeot, L. J. (2000). Urban Agriculture: Definition, Presence, Potentials and Risks, and Policy Challenges. Cities Feeding People Series; Rept. 31. International Development Research Centre.
Mundoli, S., Unnikrishnan, H., and Nagendra, H. (2017). The “Sustainable” in smart cities: ignoring the importance of urban ecosystems. Decision 44, 103–120. doi: 10.1007/s40622-017-0152-x
Murray, C. J., Aravkin, A. Y., Zheng, P., Abbafati, C., Abbas, K. M., Abbasi-Kangevari, M., et al. (2020). Global burden of 87 risk factors in 204 countries and territories, 1990–2019: a systematic analysis for the Global Burden of Disease Study 2019. Lancet 396, 1223–1249. doi: 10.1016/S0140-6736(20)30752-2
Murray-Tortarolo, G. N., and Jaramillo, V. J. (2019). The impact of extreme weather events on livestock populations: the case of the 2011 drought in Mexico. Clim. Change 153, 79–89. doi: 10.1007/s10584-019-02373-1
Murray-Tortarolo, G. N., and Jaramillo, V. J. (2020). Precipitation extremes in recent decades impact cattle populations at the global and national scales. Sci. Total Environ. 736, 139557. doi: 10.1016/j.scitotenv.2020.139557
Nelson, E. J., Kareiva, P., Ruckelshaus, M., Arkema, K., Geller, G., Girvetz, E., et al. (2013). Climate change's impact on key ecosystem services and the human well-being they support in the US. Front. Ecol. Environ. 11, 483–893. doi: 10.1890/120312
Nowak, D. J. (1994). “Air pollution removal by Chicago's Urban Forest,” in Chicago's Urban Forest Ecosystem: Results of the Chicago Urban Forest Climate Project, eds E. G. McPherson, D. J. Nowak, and R. A. Rowntree (Radnor, PA: U.S. Department of Agriculture, Forest Service, Northeastern Forest Experiment Station), 63–81.
Nowak, D. J., Civerolo, K. L., Rao, S. T., Sistla, G., Luley, C. J., and Crane, D. E. (2000). A modeling study of the impact of urban trees on ozone. Atmos. Environ. 34, 1601–1613. doi: 10.1016/S1352-2310(99)00394-5
Nowak, D. J., and Crane, D. E. (2002). Carbon storage and sequestration by urban trees in the USA. Environ. Pollut. 116, 381–389. doi: 10.1016/S0269-7491(01)00214-7
Nowak, D. J., Crane, D. E., and Stevens, J. C. (2006). Air pollution removal by urban trees and shrubs in the United States. Urban Forest. Urban Green. 4, 115–123. doi: 10.1016/j.ufug.2006.01.007
Nowak, D. J., Crane, D. E., Stevens, J. C., Hoehn, R. E., Walton, J. T., and Bond, J. (2008). A ground-based method of assessing urban forest structure and ecosystem services. Arboricult. Urban For. 34, 347–358. doi: 10.48044/jauf.2008.048
Nowak, D. J., Greenfield, E. J., Hoehn, R. E., and Lapoint, E. (2013a). Carbon storage and sequestration by trees in urban and community areas of the United States. Environ. Pollut. 178, 229–236. doi: 10.1016/j.envpol.2013.03.019
Nowak, D. J., Hirabayashi, S., Bodine, A., and Hoehn, R. (2013b). Modeled PM2. 5 removal by trees in ten US cities and associated health effects. Environ. Pollut. 178, 395–402. doi: 10.1016/j.envpol.2013.03.050
Nowak, D. J., Hoehn, R. E., Bodine, A. R., Crane, D. E., Dwyer, J. F., Bonnewell, V., et al. (2013c). Urban Trees and Forests of the Chicago Region. United States Department of Agriculture, Forest Service, Northern Research Station.
Oberndorfer, E. (2007). Green Roofs: Ecosystem Services and Research Frontiers. Available online at: https://scitizen.com/future-energies/green-roofs-ecosystem-services-and-research-frontiers_a-14-1225.html (accessed September 14, 2023).
O'Brien, M. F., Swanson, D. R., and Monsma, J. (2012). Anthidium oblongatum (Apoidea: Megachilidae) confirmed as a Michigan resident, with notes on other Michigan Anthidium species. Great Lakes Entomol. 45, 9. doi: 10.22543/0090-0222.2242
Oh, R. R., Richards, D. R., and Yee, A. T. (2018). Community-driven skyrise greenery in a dense tropical city provides biodiversity and ecosystem service benefits. Landsc. Urban Plan. 169, 115–123. doi: 10.1016/j.landurbplan.2017.08.014
Oke, T. R. (1989). The micrometeorology of the urban forest. Philos. Transact. R. Soc. London B Biol. Sci. 324, 335–349. doi: 10.1098/rstb.1989.0051
Oliveira, W., e Silva, J. L. S., de Oliveira, M. T. P., Cruz-Neto, O., da Silva, L. A. P., Borges, L. A., et al. (2019). Reduced reproductive success of the endangered tree brazilwood (Paubrasilia echinata, Leguminosae) in urban ecosystem compared to Atlantic Forest remnant: lessons for tropical urban ecology. Urban For. Urban Green. 41, 303–312. doi: 10.1016/j.ufug.2019.04.020
Ollerton, J., Winfree, R., and Tarrant, S. (2011). How many flowering plants are pollinated by animals? Oikos 120, 321–326. doi: 10.1111/j.1600-0706.2010.18644.x
Olsson, J. A., Brunner, J., Nordin, A., and Hanson, H. I. (2020). A just urban ecosystem service governance at the neighbourhood level-perspectives from Sofielund, Malmö, Sweden. Environ. Sci. Policy 112, 305–313. doi: 10.1016/j.envsci.2020.06.025
Omasa, K., Tobe, K., and Kondo, T. (2002). “Absorption of organic and inorganic air pollutants by plants,” in Air Pollution and Plant Biotechnology: Prospects for Phytomonitoring and Phytoremediation, eds K. Omas, H. Saji, S. Youssefia, and N. Kondo (Tokyo: Springer), 155–178.
O'Neil-Dunne, J., MacFaden, S., and Royar, A. (2014). A versatile, production-oriented approach to high-resolution tree-canopy mapping in urban and suburban landscapes using GEOBIA and data fusion. Remote Sens. 6, 12837–12865. doi: 10.3390/rs61212837
Orsini, F., Gasperi, D., Marchetti, L., Piovene, C., Draghetti, S., Ramazzotti, S., et al. (2014). Exploring the production capacity of rooftop gardens (RTGs) in urban agriculture: the potential impact on food and nutrition security, biodiversity and other ecosystem services in the city of Bologna. Food Sec. 6, 781–792. doi: 10.1007/s12571-014-0389-6
Owen, J. (2010). Wildlife of a Garden: A Thirty-Year Study. Peterborough: Royal Horticultural Society, 261.
Pagliaro, M. D., and Knouft, J. H. (2020). Differential effects of the urban heat island on thermal responses of freshwater fishes from unmanaged and managed systems. Sci. Total Environ. 723, 138084. doi: 10.1016/j.scitotenv.2020.138084
Palliwoda, J., and Priess, J. (2021). What do people value in urban green? Linking characteristics of urban green spaces to users' perceptions of nature benefits, disturbances, and disservices. Ecol. Soc. 26. doi: 10.5751/ES-12204-260128
Pandey, A. K., Ghosh, A., Rai, K., Fatima, A., Agrawal, M., and Agrawal, S. B. (2019). “Abiotic stress in plants: a general outline,” in Approaches for Enhancing Abiotic Stress Tolerance in Plants, eds M. Hasanuzzaman, K. Nahar, M. F. H. Oku, and T. Islam (CRC Press, Taylor & Francis Group), 1–46.
Pandey, B., and Ghosh, A. (2022). “Toxicological implications of fine particulates: sources, chemical composition, and possible underlying mechanism,” in Airborne Particulate Matter: Source, Chemistry and Health, eds A. Shukla and S. Sonwani (Singapore: Springer Nature Singapore), 131–166.
Panuccio, M., Foschi, F., Audinet, J. P., Calò, C. M., and Bologna, M. A. (2017). Urban wetlands: wastelands or hotspots for conservation? Two case studies from Rome, Italy. Avocetta 41, 13–18.
Park, J. H., Duan, L., Kim, B., Mitchell, M. J., and Shibata, H. (2010). Potential effects of climate change and variability on watershed biogeochemical processes and water quality in Northeast Asia. Environ. Int. 36, 212–225. doi: 10.1016/j.envint.2009.10.008
Pataki, D. E., Carreiro, M. M., Cherrier, J., Grulke, N. E., Jennings, V., Pincetl, S., et al. (2011). Coupling biogeochemical cycles in urban environments: ecosystem services, green solutions, and misconceptions. Front. Ecol. Environ. 9, 27–36. doi: 10.1890/090220
Patasaraiya, M. K., Devi, R. M., Sinha, B., and Bisaria, J. (2023). Predicting impacts of climate change on teak and sal forests in central India using maximum entropy modeling: an approach for future conservation and silvicultural strategies. For. Sci. 69, 463–472. doi: 10.1093/forsci/fxad014
Pearce, H., and Walters, C. L. (2012). Do green roofs provide habitat for bats in urban areas? Acta chiropterologica 14, 469–478. doi: 10.3161/150811012X661774
Peng, J., Liu, Z., Liu, Y., Hu, X., and Wang, A. (2015). Multifunctionality assessment of urban agriculture in Beijing City, China. Sci. Total Environ. 537, 343–351. doi: 10.1016/j.scitotenv.2015.07.136
Peng, L., Zhang, L., Li, X., Wang, P., Zhao, W., Wang, Z., et al. (2022). Spatio-temporal patterns of ecosystem services provided by urban green spaces and their equity along urban–rural gradients in the Xi'an metropolitan area, China. Remote Sens. 14, 4299. doi: 10.3390/rs14174299
Phillips, C., and Atchison, J. (2020). Seeing the trees for the (urban) forest: more-than-human geographies and urban greening. Aust. Geogr. 51, 155–168. doi: 10.1080/00049182.2018.1505285
Plane, E., Hill, K., and May, C. (2019). A rapid assessment method to identify potential groundwater flooding hotspots as sea levels rise in coastal cities. Water 11, 2228. doi: 10.3390/w11112228
Plieninger, T., Bieling, C., Fagerholm, N., Byg, A., Hartel, T., Hurley, P., et al. (2015). The role of cultural ecosystem services in landscape management and planning. Curr. Opin. Environ. Sustain. 14, 28–33. doi: 10.1016/j.cosust.2015.02.006
Polsky, L., and von Keyserlingk, M. A. (2017). Invited review: effects of heat stress on dairy cattle welfare. J. Dairy Sci. 100, 8645–8657. doi: 10.3168/jds.2017-12651
Pragati, S., Shanthi Priya, R., Pradeepa, C., and Senthil, R. (2023). Simulation of the energy performance of a building with green roofs and green walls in a tropical climate. Sustainability 15:2006. doi: 10.3390/su15032006
Prigioniero, A., Postiglione, A., Zuzolo, D., Niinemets, Ü., Tartaglia, M., Scarano, P., et al. (2023). Leaf surface functional traits influence particulate matter and polycyclic aromatic hydrocarbons air pollution mitigation: insights from Mediterranean urban forests. J. Clean. Prod. 418, 138158. doi: 10.1016/j.jclepro.2023.138158
Pugh, T. A., MacKenzie, A. R., Whyatt, J. D., and Hewitt, C. N. (2012). Effectiveness of green infrastructure for improvement of air quality in urban street canyons. Environ. Sci. Technol. 46, 7692–7699. doi: 10.1021/es300826w
Qin, Y. (2020). Urban flooding mitigation techniques: a systematic review and future studies. Water 12, 3579. doi: 10.3390/w12123579
Quesada-Ganuza, L., Garmendia, L., Roji, E., and Gandini, A. (2021). Do we know how urban heritage is being endangered by climate change? A systematic and critical review. Int. J. Disast. Risk Reduct. 65, 102551. doi: 10.1016/j.ijdrr.2021.102551
Rai, R., Rajput, M., Agrawal, M., and Agrawal, S. B. (2011). Gaseous air pollutants: a review on current and future trends of emissions and impact on agriculture. J. Sci. Res. 55, 1.
Rasmont, P., Franzen, M., Lecocq, T., Harpke, A., Roberts, S. P., Biesmeijer, J. C., et al. (2015). Climatic Risk and Distribution Atlas of European Bumblebees, Vol. 10. Bulgaria: Pensoft Publishers, 1–236.
Redon, E., Lemonsu, A., and Masson, V. (2020). An urban trees parameterization for modeling microclimatic variables and thermal comfort conditions at street level with the Town Energy Balance model (TEB-SURFEX v8. 0). Geosci. Model Dev. 13, 385–399. doi: 10.5194/gmd-13-385-2020
Remolina, F. (2015). Role of Local Stakeholders in Reaching, Developing, and Sustaining Collaborative Management: Case Study of Urban Wetlands of Bogotá, Colombia (Master's Thesis). 60 ECTS Social-Ecological Resilience for Sustainable Development, Stockholm Resilience Centre, Stockholm, Sweden.
Reyers, B., Biggs, R., Cumming, G. S., Elmqvist, T., Hejnowicz, A. P., and Polasky, S. (2013). Getting the measure of ecosystem services: a social–ecological approach. Front. Ecol. Environ. 11, 268–273. doi: 10.1890/120144
Riedel, T., and Weber, T. K. (2020). The influence of global change on Europe's water cycle and groundwater recharge. Hydrogeol. J. 28, 1939–1959. doi: 10.1007/s10040-020-02165-3
Rodríguez, J. P., Beard Jr, T. D., Bennett, E. M., Cumming, G. S., Cork, S. J., Agard, J., et al. (2006). Trade-offs across space, time, and ecosystem services. Ecol. Soc. 11. doi: 10.5751/ES-01667-110128
Russell, R., Guerry, A. D., Balvanera, P., Gould, R. K., Basurto, X., Chan, K. M., et al. (2013). Humans and nature: how knowing and experiencing nature affect well-being. Annu. Rev. Environ. Resour. 38, 473–502. doi: 10.1146/annurev-environ-012312-110838
Sabiiti, E. N., Katongole, C. B., Katuromunda, S., Sengendo, H., Basalirwa, C. P. K., Atukunda, G., et al. (2014). Building Urban Resilience: Assessing Urban and Peri-urban Agriculture in Kampala, Uganda. Nairobi: United Nations Environment Programme (UNEP).
Salmond, J. A., Tadaki, M., Vardoulakis, S., Arbuthnott, K., Coutts, A., Demuzere, M., et al. (2016). Health and climate related ecosystem services provided by street trees in the urban environment. Environ. Health 15, 95–111. doi: 10.1186/s12940-016-0103-6
Sánchez, F. G., and Govindarajulu, D. (2023). Integrating blue-green infrastructure in urban planning for climate adaptation: Lessons from Chennai and Kochi, India. Land Use Policy 124, 106455. doi: 10.1016/j.landusepol.2022.106455
Sanyé-Mengual, E., Specht, K., Krikser, T., Vanni, C., Pennisi, G., Orsini, F., et al. (2018). Social acceptance and perceived ecosystem services of urban agriculture in Southern Europe: the case of Bologna, Italy. PLoS ONE 13, e0200993. doi: 10.1371/journal.pone.0200993
Savi, T., Bertuzzi, S., Branca, S., Tretiach, M., and Nardini, A. (2015). Drought-induced xylem cavitation and hydraulic deterioration: risk factors for urban trees under climate change?. New Phytol. 205, 1106–1116. doi: 10.1111/nph.13112
Saxena, P., and Ghosh, C. (2011). Variation in the concentration of ground level ozone at selected sites in Delhi. Int. J. Environ. Sci. 1, 1899–1911.
Schaich, H., Bieling, C., and Plieninger, T. (2010). Linking ecosystem services with cultural landscape research. Gaia Ecol. Perspect. Sci. Soc. 19, 269–277. doi: 10.14512/gaia.19.4.9
Schindler, B. Y., Griffith, A. B., and Jones, K. N. (2011). Factors influencing arthropod diversity on green roofs. Cities Environ. 4, 5. doi: 10.15365/cate.4152011
Scholes, R. J. (2016). Climate change and ecosystem services. Wiley Interdiscip. Rev. 7, 537–550. doi: 10.1002/wcc.404
Schrader, S., and Böning, M. (2006). Soil formation on green roofs and its contribution to urban biodiversity with emphasis on Collembolans. Pedobiologia 50, 347–356. doi: 10.1016/j.pedobi.2006.06.003
Schröder, C., Mancosu, E., and Roerink, G. J. (2013). Methodology Proposal for Estimation of Carbon Storage in Urban Green Areas. European Environment Agency.
Sehgal, A., Sita, K., Siddique, K. H., Kumar, R., Bhogireddy, S., Varshney, R. K., et al. (2018). Drought or/and heat-stress effects on seed filling in food crops: impacts on functional biochemistry, seed yields, and nutritional quality. Front. Plant Sci. 9, 1705. doi: 10.3389/fpls.2018.01705
Seitz, J., and Escobedo, F. (2008). Urban Forests in Florida: Trees Control Stormwater Runoff and Improve Water Quality: FOR184/FR239, 5/2008. University of Florida, Edis 2008.
Selmi, W., Weber, C., Rivière, E., Blond, N., Mehdi, L., and Nowak, D. (2016). Air pollution removal by trees in public green spaces in Strasbourg city, France. Urban Forest. Urban Green. 17, 192–201. doi: 10.1016/j.ufug.2016.04.010
Settele, J., Bishop, J., and Potts, S. G. (2016). Climate change impacts on pollination. Nat. Plants 2, 1–3. doi: 10.1038/nplants.2016.92
Settele, J., Kudrna, O., Harpke, A., Kühn, I., Van Swaay, C., Verovnik, R., et al. (2008). Climatic Risk Atlas of European Butterflies. Sofia: Pensoft, 392–393.
Sevianu, E., Maloş, C. V., Arghiuş, V., Brişan, N., Bǎdǎrǎu, A. S., Moga, M. C., et al. (2021). Mainstreaming ecosystem services and biodiversity in peri-urban forest park creation: experience from eastern Europe. Front. Environ. Sci. 9, 618217. doi: 10.3389/fenvs.2021.618217
Shahzad, K., Sintim, H., Ahmad, F., Abid, M., and Nasim, W. (2022). “Importance of carbon sequestration in the context of climate change,” in Building Climate Resilience in Agriculture, eds M. A. Cheema, Z. Lin, and M. Z. Hashmi, 385–401. doi: 10.1007/978-3-030-79408-8_23
Sharma, P., and Saxena, P. (2022). “Impacts and responses of particulate matter pollution on vegetation,” in Airborne Particulate Matter, eds S. Sonwani and A. Shukla, 229–264. doi: 10.1007/978-981-16-5387-2_10
Shashua-Bar, L., and Hoffman, M. E. (2000). Vegetation as a climatic component in the design of an urban street: an empirical model for predicting the cooling effect of urban green areas with trees. Energy Build. 31, 221–235. doi: 10.1016/S0378-7788(99)00018-3
Sicard, P., Agathokleous, E., Araminiene, V., Carrari, E., Hoshika, Y., De Marco, A., et al. (2018). Should we see urban trees as effective solutions to reduce increasing ozone levels in cities? Environ. Pollut. 243, 163–176. doi: 10.1016/j.envpol.2018.08.049
Sieghardt, M., Mursch-Radlgruber, E., Paoletti, E., Couenberg, E., Dimitrakopoulus, A., Rego, F., et al. (2005). “The abiotic urban environment: impact of urban growing conditions on urban vegetation,” in Urban Forest Trees, eds C. Konijnendijk, K. Nilsson, T. Randrup, and J. Schipperijn, 281–323. doi: 10.1007/3-540-27684-X_12
Sirois-Delisle, C., and Kerr, J. T. (2018). Climate change-driven range losses among bumblebee species are poised to accelerate. Sci. Rep. 8, 14464. doi: 10.1038/s41598-018-32665-y
Smith, P., Ashmore, M., Black, H., Burgess, P., Evans, C., Hails, R., et al. (2011). “Regulating Services,” in UK National Ecosystem Assessment: Technical Report (UK NEA Publications), 535–596. Available online at: http://centaur.reading.ac.uk/25219
Son, Y. H. (2018). “Creation of green culture and values in the Hanul Madang rooftop garden at Seoul National University,” in Green City Planning and Practices in Asian Cities, Strategies for Sustainability, eds Z. Shen, L. Huang, K. Peng, and J. Pai (Cham: Springer), 227–239. doi: 10.1007/978-3-319-70025-0_11
Sonwani, S., Hussain, S., and Saxena, P. (2022). Air pollution and climate change impact on forest ecosystems in Asian region–a review. Ecosyst. Health Sustain. 8, 2090448. doi: 10.1080/20964129.2022.2090448
Sonwani, S., and Saxena, P. eds. (2022). Greenhouse Gases: Sources, Sinks and Mitigation. Singapore: Springer.
Soroye, P., Newbold, T., and Kerr, J. (2020). Climate change contributes to widespread declines among bumble bees across continents. Science 367, 685–688. doi: 10.1126/science.aax8591
Soydan, O. (2020). Effects of landscape composition and patterns on land surface temperature: urban heat island case study for Nigde, Turkey. Urban Clim. 34, 100688. doi: 10.1016/j.uclim.2020.100688
Speak, A., Escobedo, F. J., Russo, A., and Zerbe, S. (2020). Total urban tree carbon storage and waste management emissions estimated using a combination of LiDAR, field measurements and an end-of-life wood approach. J. Clean. Prod. 256, 120420. doi: 10.1016/j.jclepro.2020.120420
Stagoll, K., Lindenmayer, D. B., Knight, E., Fischer, J., and Manning, A. D. (2012). Large trees are keystone structures in urban parks. Conserv. Lett. 5, 115–122. doi: 10.1111/j.1755-263X.2011.00216.x
Stevenson, P. C., Bidartondo, M. I., Blackhall-Miles, R., Cavagnaro, T. R., Cooper, A., Geslin, B., et al. (2020). The state of the world's urban ecosystems: what can we learn from trees, fungi, and bees? Plants People Planet 2, 482–498. doi: 10.1002/ppp3.10143
Strohbach, M. W., Arnold, E., and Haase, D. (2012). The carbon footprint of urban green space—A life cycle approach. Landsc. Urban Plan. 104, 220–229. doi: 10.1016/j.landurbplan.2011.10.013
Strohbach, M. W., and Haase, D. (2012). Above-ground carbon storage by urban trees in Leipzig, Germany: analysis of patterns in a European city. Landsc. Urban Plan. 104, 95–104. doi: 10.1016/j.landurbplan.2011.10.001
Svendsen, E., and Campbell, L. K. (2008). Urban ecological stewardship: understanding the structure, function and network of community-based urban land management. Cities Environ. 1, 4. doi: 10.15365/cate.1142008
Taha, H. (1996). Modeling impacts of increased urban vegetation on ozone air quality in the South Coast Air Basin. Atmos. Environ. 30, 3423–3430. doi: 10.1016/1352-2310(96)00035-0
Thapa, S., Nainabasti, A., and Bharati, S. (2021). Assessment of the linkage of urban green roofs, nutritional supply, and diversity status in Nepal. Cogent. Food Agric. 7, 1911908. doi: 10.1080/23311932.2021.1911908
Tidball, K. G., and Krasny, M. E. (2011). Urban environmental education from a social-ecological perspective: conceptual framework for civic ecology education. Cities Environ. 3, 11. doi: 10.15365/cate.31112010
Tonietto, R., Fant, J., Ascher, J., Ellis, K., and Larkin, D. (2011). A comparison of bee communities of Chicago green roofs, parks and prairies. Landsc. Urban Plan. 103, 102–108. doi: 10.1016/j.landurbplan.2011.07.004
Tsunetsugu, Y., Lee, J., Park, B. J., Tyrväinen, L., Kagawa, T., and Miyazaki, Y. (2013). Physiological and psychological effects of viewing urban forest landscapes assessed by multiple measurements. Landsc. Urban Plan. 113, 90–93. doi: 10.1016/j.landurbplan.2013.01.014
Tuvendal, M., and Elmqvist, T. (2011). Ecosystem services linking social and ecological systems: river brownification and the response of downstream stakeholders. Ecol. Soc. 16, 21. doi: 10.5751/ES-04456-160421
Ulrich, R. S. (1981). Natural versus urban scenes: Some psychophysiological effects. Environ. Behav. 13, 523–556. doi: 10.1177/0013916581135001
United Nations (2018). Cities and Pollution Contribute to Climate Change. Available online at: https://www.un.org/en/climatechange/cities-pollution.shtml (accessed July 17, 2023).
Upadhyay, S., and Raghubanshi, A. S. (2020). “Determinants of soil carbon dynamics in urban ecosystems,” in Urban Ecology, eds A. S. Raghubanshi, P. Singh, P. Verma, and R. Singh (Elsevier) 299–314.
Upadhyay, S., Singh, R., Verma, P., and Raghubanshi, A. S. (2021). Spatio-temporal variability in soil CO2 efflux and regulatory physicochemical parameters from the tropical urban natural and anthropogenic land use classes. J. Environ. Manage. 295, 113141. doi: 10.1016/j.jenvman.2021.113141
Uprety, D. C., and Saxena, P. (2021). Technologies for Green House Gas Assessment in Crop Studies, Vol. 1. Singapore: Springer.
Vaccari, F. P., Gioli, B., Toscano, P., and Perrone, C. (2013). Carbon dioxide balance assessment of the city of Florence (Italy), and implications for urban planning. Landsc. Urban Plan. 120, 138–146. doi: 10.1016/j.landurbplan.2013.08.004
Van den Berg, A. E., Maas, J., Verheij, R. A., and Groenewegen, P. P. (2010). Green space as a buffer between stressful life events and health. Soc. Sci. Med. 70, 1203–1210. doi: 10.1016/j.socscimed.2010.01.002
Velasco, E., Roth, M., Norford, L., and Molina, L. T. (2016). Does urban vegetation enhance carbon sequestration? Landsc. Urban Plan 148, 99–107. doi: 10.1016/j.landurbplan.2015.12.003
Venter, Z. S., Barton, D. N., Gundersen, V., Figari, H., and Nowell, M. (2020). Urban nature in a time of crisis: recreational use of green space increases during the COVID-19 outbreak in Oslo, Norway. Environ. Res. Lett. 15, 104075. doi: 10.1088/1748-9326/abb396
Verma, P., Singh, R., Bryant, C., and Raghubanshi, A. S. (2020). Green space indicators in a social-ecological system: a case study of Varanasi, India. Sustain. Cities Soc. 60, 102261. doi: 10.1016/j.scs.2020.102261
Volney, W. J. A., and Fleming, R. A. (2000). Climate change and impacts of boreal forest insects. Agric. Ecosyst. Environ. 82, 283–294. doi: 10.1016/S0167-8809(00)00232-2
Wagenaar, H., and Wilkinson, C. (2015). Enacting resilience: a performative account of governing for urban resilience. Urban Stud. 52, 1265–1284. doi: 10.1177/0042098013505655
Wagstaff, R. K. (2016). Farming in the City: How the Urban Environment Affects Vegetable Crop Production, Soil Heavy Metal Contamination and Nutrient Dynamics, Produce Nutritive Quality, and Insect Dynamics in Urban Gardens (Doctoral dissertation). University of Illinois at Urbana-Champaign.
Wakamatsu, S., Kanda, I., Okazako, Y., Saito, M., Yamamoto, M., Watanabe, T., et al. (2017). A Comparative Study of Urban Air Quality in Megacities in Mexico and Japan: Based on Japan-Mexico Joint Research Project on Formation Mechanism of Ozone, VOCs and PM2. 5, and Proposal of Countermeasure Scenario (No. 145). Tokyo: JICA Research Institute.
Wang, J., Endreny, T. A., and Nowak, D. J. (2008). Mechanistic simulation of tree effects in an urban water balance model 1. J. Am. Water Resour. Assoc. 44, 75–85. doi: 10.1111/j.1752-1688.2007.00139.x
Wang, M., Zhang, D. Q., Su, J., Trzcinski, A. P., Dong, J. W., and Tan, S. K. (2017). Future scenarios modeling of urban stormwater management response to impacts of climate change and urbanization. Clean Soil Air Water 45, 1700111. doi: 10.1002/clen.201700111
Wang, R., Bai, Y., Alatalo, J. M., Yang, Z., Yang, Z., Yang, W., et al. (2021). Impacts of rapid urbanization on ecosystem services under different scenarios–A case study in Dianchi Lake Basin, China. Ecol. Indic. 130, 108102. doi: 10.1016/j.ecolind.2021.108102
Wang, Z. H., Zhao, X., Yang, J., and Song, J. (2016). Cooling and energy saving potentials of shade trees and urban lawns in a desert city. Appl. Energy 161, 437–444. doi: 10.1016/j.apenergy.2015.10.047
Weiskopf, S. R., Rubenstein, M. A., Crozier, L. G., Gaichas, S., Griffis, R., Halofsky, J. E., et al. (2020). Climate change effects on biodiversity, ecosystems, ecosystem services, and natural resource management in the United States. Sci. Total Environ. 733, 137782. doi: 10.1016/j.scitotenv.2020.137782
Williams, J. J., and Newbold, T. (2020). Local climatic changes affect biodiversity responses to land use: a review. Divers. Distribut. 26, 76–92. doi: 10.1111/ddi.12999
Williams, N. S., Lundholm, J., and Scott MacIvor, J. (2014). Do green roofs help urban biodiversity conservation? J. Appl. Ecol. 51, 1643–1649. doi: 10.1111/1365-2664.12333
Wolf, J., Van Wijk, M. S., Cheng, X., Hu, Y., Van Diepen, C. A., Jongbloed, A. W., et al. (2003). Urban and peri-urban agricultural production in Beijing municipality and its impact on water quality. Environ. Urban. 15, 141–156. doi: 10.1177/095624780301500205
Wood, E. M., and Esaian, S. (2020). The importance of street trees to urban avifauna. Ecol. Appl. 30, e02149. doi: 10.1002/eap.2149
World Bank (2023). World Development Indicators. Available online at: https://data.worldbank.org/data-catalog/world-development-indicators (accessed July 12, 2023).
Wright, H. (2011). Understanding green infrastructure: the development of a contested concept in England. Local Environ. 16, 1003–1019. doi: 10.1080/13549839.2011.631993
Xu, Y., Ramanathan, V., and Victor, D. G. (2018). Global warming will happen faster than we think. Nature 564, 30–32.
Yang, J., McBride, J., Zhou, J., and Sun, Z. (2005). The urban forest in Beijing and its role in air pollution reduction. Urban For. Urban Green. 3, 65–78. doi: 10.1016/j.ufug.2004.09.001
Yao, J., Wu, S., Cao, Y., Wei, J., Tang, X., Hu, L., et al. (2023). Dry deposition effect of urban green spaces on ambient particulate matter pollution in China. Sci. Total Environ. 900:165830. doi: 10.1016/j.scitotenv.2023.165830
Yin, Z., Zhang, Y., and Ma, K. (2022). Evaluation of PM2. 5 retention capacity and structural optimization of urban park green spaces in Beijing. Forests 13, 415. doi: 10.3390/f13030415
Zhang, B., Shi, Y. T., Liu, J. H., and Xu, J. (2017). Economic values and dominant providers of key ecosystem services of wetlands in Beijing, China. Ecol. Indic. 77, 48–58. doi: 10.1016/j.ecolind.2017.02.005
Zhang, X., Zhang, L., Wang, Y., Shao, Y., Daniels, B., Roß-Nickoll, M., et al. (2022). Pollinators and urban riparian vegetation: important contributors to urban diversity conservation. Environ. Sci. Europe 34, 78. doi: 10.1186/s12302-022-00661-9
Zhang, X. Q. (2016). The trends, promises and challenges of urbanisation in the world. Habitat Int. 54, 241–252. doi: 10.1016/j.habitatint.2015.11.018
Zhao, C., and Sander, H. A. (2015). Quantifying and mapping the supply of and demand for carbon storage and sequestration service from urban trees. PLoS ONE 10, e0136392. doi: 10.1371/journal.pone.0136392
Zhao, H., Li, G., Guo, D., Li, H., Liu, Q., Xu, B., et al. (2021). Response mechanisms to heat stress in bees. Apidologie 52, 388–399. doi: 10.1007/s13592-020-00830-w
Ziervogel, G., Pelling, M., Cartwright, A., Chu, E., Deshpande, T., Harris, L., et al. (2017). Inserting rights and justice into urban resilience: a focus on everyday risk. Environ. Urban 29, 123–138. doi: 10.1177/0956247816686905
Keywords: climate change, ecosystem services, resilience, sustainability, urban ecosystem
Citation: Pandey B and Ghosh A (2023) Urban ecosystem services and climate change: a dynamic interplay. Front. Sustain. Cities 5:1281430. doi: 10.3389/frsc.2023.1281430
Received: 22 August 2023; Accepted: 03 October 2023;
Published: 30 October 2023.
Edited by:
Saurabh Sonwani, University of Delhi, IndiaCopyright © 2023 Pandey and Ghosh. This is an open-access article distributed under the terms of the Creative Commons Attribution License (CC BY). The use, distribution or reproduction in other forums is permitted, provided the original author(s) and the copyright owner(s) are credited and that the original publication in this journal is cited, in accordance with accepted academic practice. No use, distribution or reproduction is permitted which does not comply with these terms.
*Correspondence: Annesha Ghosh, YW5uZTE5OTAxOTAwQGdtYWlsLmNvbQ==