- 1Sustainability Expert, Instituto de Desarrollo Urbano, Bogotá, Colombia
- 2Instituto Javeriano del Agua, Pontificia Universidad Javeriana, Bogotá, Colombia
Introduction: Nature-based solutions are increasingly recognized as vital components of urban resilience strategies, particularly within the framework of green infrastructure. This study aims to propose an approach that fosters symbiosis between green and gray infrastructure to address the challenges posed by climate change in urban environments.
Methods: We conducted a comprehensive review of guidelines and scientific literature to inform the selection of species and the design of root containers for urban tree planting. Additionally, we performed a multicriteria analysis and assessed water comfort to guide decision-making regarding species selection in specific city areas.
Results: The methodology was applied to a case study in Bogotá, yielding insights applicable to any city with basic knowledge of suitable species for planting in built public spaces. Crucial criteria for selecting local species for sidewalks were identified, including size, permeability, soil compaction characteristics, and climatic adaptability. A list of desirable species adapted to all humidity zones of the case study city was generated. Hydrological sizing methods proposed are contingent upon both the species to be planted and the geometry of the streets.
Discussion: The approach and findings presented in this study promote the development of trees and their ecosystem services while mitigating potential damage to surrounding infrastructure.
Conclusion: Implementing strategies that facilitate symbiosis between green and gray infrastructure contributes to urban resilience and aids in climate change adaptation efforts.
1 Introduction
Green infrastructure (GI), made up of natural, semi-natural, and artificial networks located in and around urban areas (Tzoulas et al., 2007), is a mitigation and adaptation strategy to climate change that promotes the improvement of ecosystems and urban resilience. It is also a multiscale territorial planning tool that serves ecological, productive, and cultural functions while contributing to the resilience of the territories (Calaza Martínez, 2019). In the case of urban street trees (ST), GI configures a hybrid system in which natural and built elements interact (Alberti, 2008). Then, ecosystem services of provision, regulation, and culture are generated (Scholz et al., 2018), along with disservices associated with their location (Döhren and Haasen, 2019) (Figure 1).
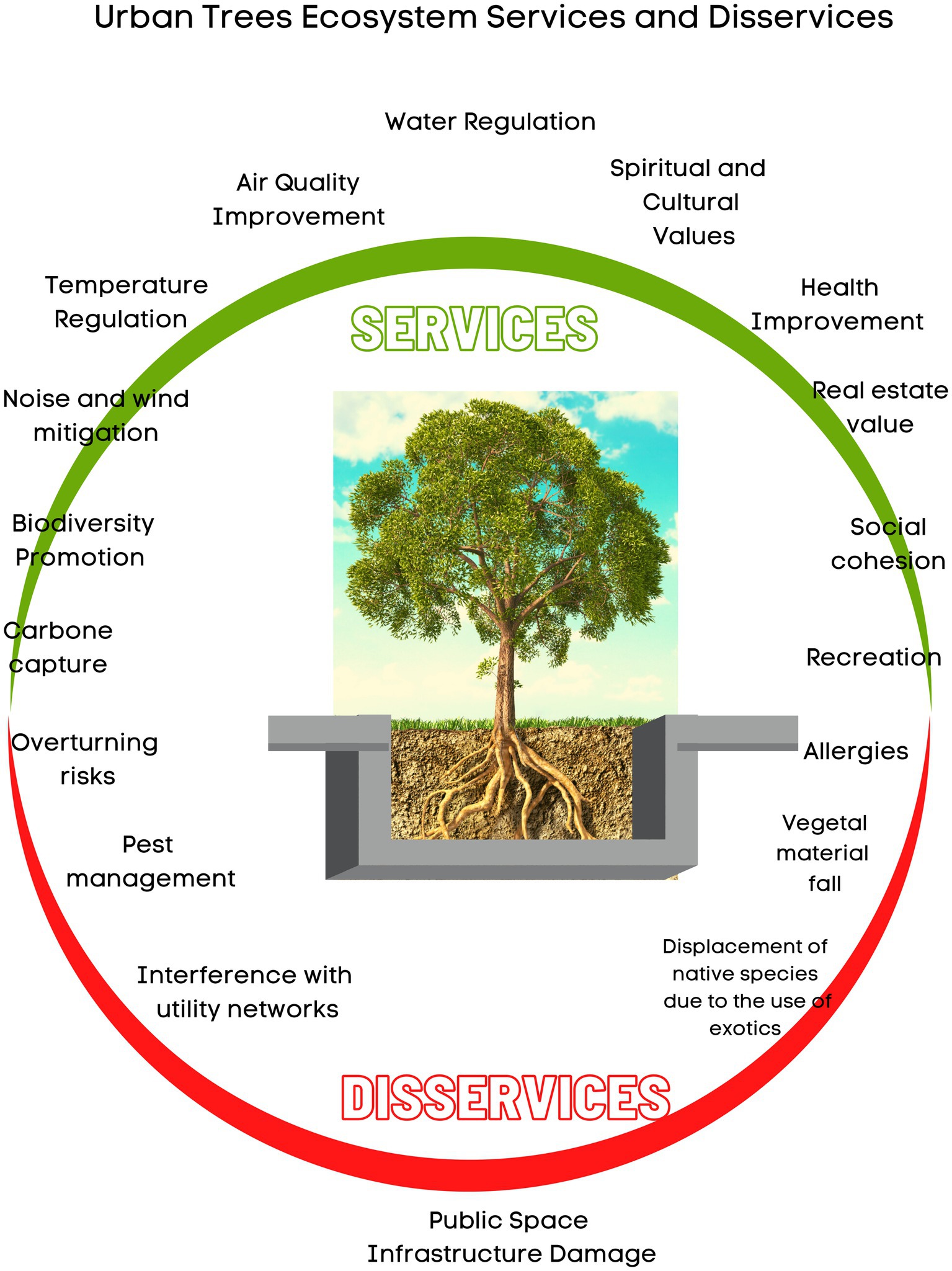
Figure 1. Urban street tree services and disservices. Authors based on (Otero-Durán 2021a).
As urbanization increases, the management of urban ecosystem disservices is more critical: It is essential to identify which aspects of ecosystem disservices are global and which are to be understood within local contexts to prevent them (Lyytimäki and Sipilä, 2009). Recent studies suggest that services and disservices can be better integrated into decision-making by evaluating tradeoffs and synergies (Roman et al., 2021). In Bogotá, the local authority also recommends that the landscape design should consider the urban climatic zone and the specific place in which the trees will be located to avoid interference with public services (Álvarez et al., 2020). Nevertheless, the infrastructure protection approach has not been considered yet. In recent years, there has been a growing interest in developing methodologies applicable to tropical cities to select suitable tree species that enhance ecosystem services. In particular, methodologies have been developed to reduce high temperatures in urban environments (Núñez-Florez et al., 2019; Morakinyo et al., 2020; Meili et al., 2021) or mitigate the negative effects of urban runoff (Carlyle-Moses et al., 2020). However, most developed methodologies overlook the water comfort conditions that species must have to provide the expected ecosystem services without conflicting with built infrastructure. Additionally, in general, developed methodologies are used to select species based on a few indicators of environmental or financial order, overlooking other relevant selection criteria, including ecosystem disservices. Finally, there are few studies that focus on the selection of native species as recommended by Arcos-LeBert et al. (2021) and provide specific recommendations for urban design.
Hence, the objective of this study is to propose a methodological approach for reducing damage to public space caused by tree roots and obtain benefits in ecosystem services and public space infrastructure (Figure 2). This includes a review of local guidelines and scientific papers for species selection and the design of root containers, as well as multicriteria and water comfort analysis for decision-making regarding species selection in specific areas of the city so that trees’ roots minimize damage to the built infrastructure. Although we used the methodological tools for a specific case study (tree pits on roads in Bogotá), it can be applied in different case studies that involve the selection and management of urban tree species considering local conditions.
2 Materials and methods
This study introduces a novel approach aimed at safeguarding urban public space infrastructure. The primary focus is strategically selecting native tree species and determining optimal dimensions for tree pits. The proposed methodology comprises three essential steps: first, an analysis of root containers based on guidelines and expert surveys; second, a multicriteria analysis for the selection of tree species; and third, a water comfort analysis specifically tailored to the chosen species. Additionally, the study identifies key interaction factors crucial for ensuring a harmonious relationship between public spaces and trees.
2.1 Assessment of tree boxes according to the guidelines
The research was conducted in the urban area of Bogotá, D.C., framed between 2000 and 2020. The main normative documents consulted are listed in Table 1, like the Urban Forestry Handbook for Bogotá (UFHB) that recommends species for sidewalks and details their physical and functional characteristics, such as resistance to pollution and adaptability to Bogotá’s humidity zones. Moreover, for the root containers and floodable tree pits, the District Planning Secretary’s sidewalk guidelines (SG) and the technical standard for sustainable drainage systems (SUDS) were consulted. Furthermore, the daily rainfall information was taken from the District Institute for Risk Management and Climate Change (IDIGER) and the proposed method for calculating the daily water requirement (Devia and Torres, 2019).
We identified the criteria for species selection and the design of root containers that reduce damage to public space from a bibliographic review (Mcpherson and Muchnick, 2005; Mullaney et al., 2015a; Elliott et al., 2018; Escobedo et al., 2019). To place the right tree in the right place (Vogt et al., 2017), we considered: (i) checking the humidity zone of the city and selecting species with the highest adaptability; (ii) promoting the species heterogeneity and their ecological functionality [i.e., 10/20/30 rule 10% of any particular species, 20% of any one genus or 30% of any single family (Santamour, 1990)]; (iii) estimating the interference with public service networks, considering the dimensions of the mature tree; and (iv) allowing tree pits enlargement to guarantee the proper development of the tree, according to the trunk’s diameter at maturity.
To determine the adaptability of the species, the local handbook UFHB comprises an analysis of the climatic conditions in the city, resulting in four humidity zones depending on the mean annual precipitation (MAP). Then, the UFHB classifies the tree species’ adaptability according to its response in each zone from zero (0) to five (5), where 5 is the best value for the tree’s health, and 0 means a not suitable zone for that species.
With these criteria (Table 2), we applied a survey to an interdisciplinary group of experts in urban trees. The survey results classified the species according to each characteristic and their score of least interference with the public space. The study consulted 17 local experts from the disciplines of architecture, landscape, biology, environmental engineering, civil engineering, pavement engineering, and forestry engineering. It was held using the Microsoft Forms tool and quantitative and qualitative questions about the criteria for species selection to be implanted in root containers in the urban public space were discussed.
Furthermore, we scored the characteristics of the species to establish their importance (Table 3), using the description for each one given by the UFHB. In that way, the root system is considered “shallow” up to 30 cm depth, “medium” between 31 and 100 cm, and “deep” for more than 101 cm depth. Hereby, the roots’ intrusiveness corresponds to the observed behavior of trees in built environments from “low” when there is no damage to the infrastructure to “remarkably” when the roots break the infrastructure, generating risk to the users. Similarly, the rusticity and resistance to treatments vary from “low” to “high” according to the tree’s health in response to the treatment and the urban conditions. Finally, for the crown diameter, “low,” “medium,” and “wide” go for less than 4 m, 4–6 m, and more than 6 m.
2.2 Multicriteria methods for species selection
For this study, we used expert surveys, including qualitative and numeric information for selecting the most appropriate tree species based on a significant number of criteria. Thus, the multicriteria analysis was conducted using the ELECTRE I method (Figueira et al., 2005), which is a practical and well-known method as presented in Galarza Molina et al. (2014). For this method, the number of parameters required (thresholds for concordance and discordance indexes) is limited, increasing the result’s confidence. In this analysis, each species obtained represents an alternative, and each characteristic represents a decision criterion. To begin, we reviewed the species proposed in the UFHB and selected 18 of native origin, following the recommendation of Arcos-LeBert et al. (2021). Subsequently, we evaluated each species based on the chosen criteria, as outlined in Table 4.
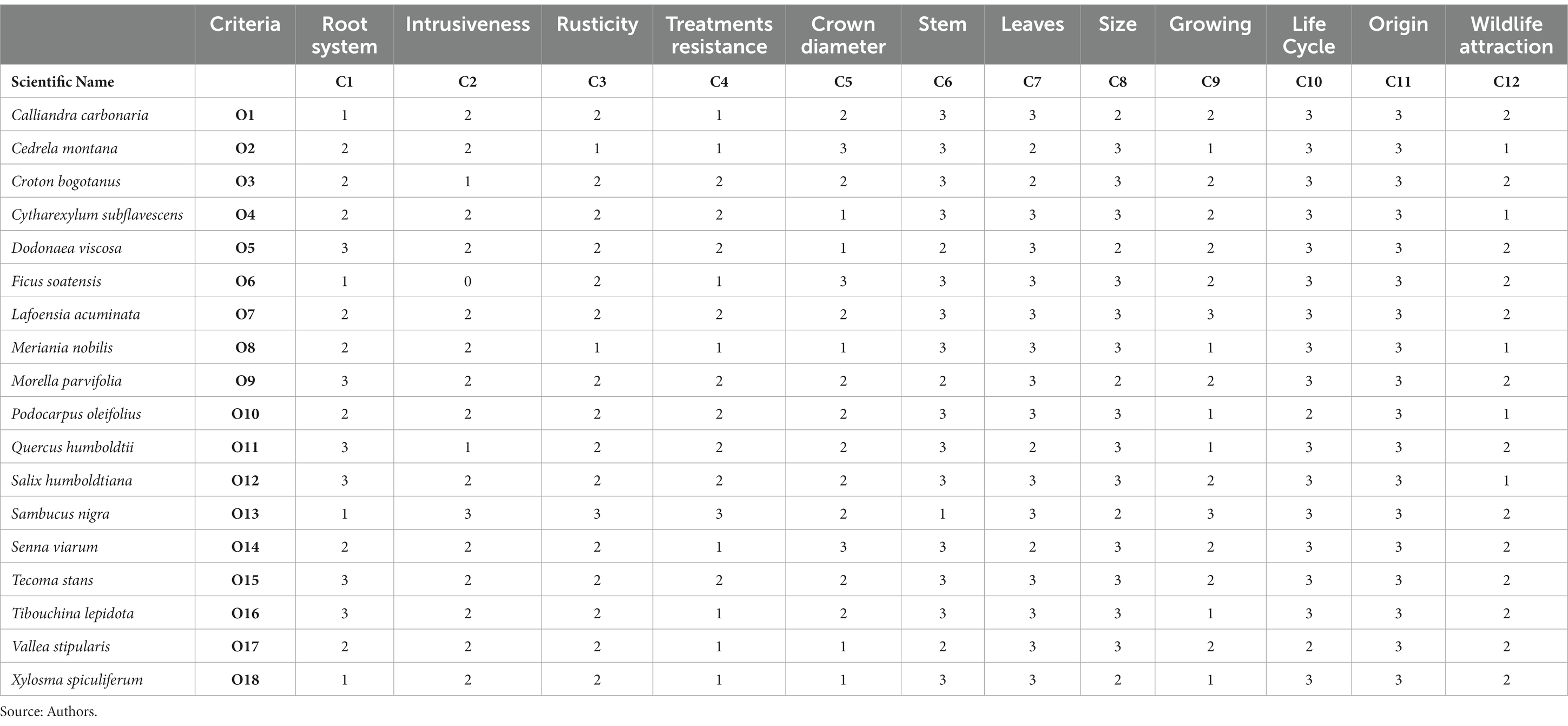
Table 4. Species for multicriteria analysis according to characteristics and value for low interference with public space.
We calculated the concordance and discordance matrices and the exceedance ratio to identify the best species in terms of ecosystem services and less interference with public space. We defined that alternative “a” overcomes “O” alternative “b” if the concordance index is greater than or equal to 65%, and if the discordance index is less than or equal to 35%, that is, a O b if C (a,b) ≥ 0.65 and d (a,b) ≤ 0.35 (see equations for C and d in Figueira et al., 2005).
Finally, we propose overcoming ratio reading horizontally, and those that surpass one or more species are selected. The obtained species list is submitted to a local forestry expert as a validation procedure, bringing a definitive list (Table 5). For the selected species, their root container sizing is verified according to the sidewalk guidelines, depending on the space from the diameter of the trunk and the height (H) of the species: shrubs (H < 3 m), short trees (3 m ≤ H ≤ 5 m), medium-sized trees (5 < H < 10 m), and tall trees (H ≥ 10 m). It is noted that the sidewalk guidelines establish the landscaping and furniture strip (LFS), in which the trees are located, with widths ranging between 0.6 and 4.4 m, depending on the type of road. We excluded the street types with a landscape and furniture stripe less than 0.7 m since their dimensions are scarce for root containers.
2.3 Water comfort analysis
For each IDIGER station, we calculated the MAP between January 2011 and April 2021. Each station is classified into humidity zones as follows: humid (MAP >1,000 mm), subhumid (851 mm ≤ MAP ≤1,000 mm), semi-dry (700 mm ≤ MAP ≤850 mm), and dry (MAP<700 mm). The number of consecutive days of typical dry weather “ds” is obtained for each zone. The daily water requirement of each tree is calculated with Equation 1 with the adjustment factor for soil waterproofing “k” of 1 for a crown diameter (CD) < 4 m, 1.5 for 4 m ≤ CD ≤ 6 m y 2 for CD ≥ 6 m, and the infiltration factor “i” of 0.4 for floodable tree pits. It is noticed that in Equation 1, the variable “r” denotes the radius of the tree crown.
Equation 1: Tree water requirement. Source: adapted from Devia and Torres (2019).
The reserve volume during the dry days, “Vres,” is calculated as the daily water requirement multiplied by the characteristic “ds” of each zone, under the assumption that during the rainy days, the daily water requirements of the tree are supplied, and a reserve volume is captured for the following dry days. Tree pits are sized with the values of “Vres,” and the volume occupied by the tree trunk is added in the depth of ponding of 15 cm according to the technical standard NS-166 (Empresa de Acueducto y Alcantarillado de Bogotá, 2018), and the design volume is obtained Vd = Vres + 0.15*π* R2, where R is the radius of the tree trunk (at DBH of 1.3 m according to the local forestry guideline). Thus, the surface area occupied by the tree pit is determined from As = Vd /0.15.
We propose two criteria for dimensioning the tree pits according to As: (i) the minimum side depends on the tree’s height H as follows: 1.2 m if H < 5 m, 1.6 m if 5 m ≤ H ≤ 15 m, and 2.0 m if H > 15 m and (ii) the minimum side as the diameter of the trunk plus the living space of 1 m if H < 5 m, 2 m if 5 m ≤ H ≤ 15 m and 3 m if H > 15 m. For both criteria, once side 1 is defined, side 2 is calculated as the highest value between side 1 and that obtained from As/side 1.
In addition, we propose finding the minimum distance between tree pits located on the road as follows: For each draining afferent area A (varying between 1 m2 and 300 m2 with steps of 0.5 m2), the daily water supply is calculated as A multiplied by each daily precipitation value and runoff coefficient of 1. The number of days in which the water requirement exceeds the water supply is counted and divided by the total number of days, obtaining the probability that the tree does not have a proper water supply. For each area A, one specific value of said probability is obtained for each humidity zone.
The adaptability of the tree to each humidity zone is considered, from 0 to 5, to select the maximum limiting probability that each tree does not have an adequate water supply, using values of 2, 5, 10, 15, 20, and 30%. It allows the selection of the minimal design afferent area Ad for each species and each zone. For each street profile from the sidewalk guidelines, the distance between street-facing dp is obtained. The minimum distance between tree pits is calculated as Ad/(dp/2), if all streets have a canopy composed of linear trees located on both sides, generating a runoff from the center to the sides.
3 Results
3.1 Key interaction factors between street trees and public space
The criteria for selecting street trees with minimum damage to the public space, according to the bibliographic review, are outlined in Table 2, which can be enhanced with the data obtained from the survey, where very high intrusiveness corresponds to a rating of 0. The root system and the level of intrusiveness represent a weight of 4.9 on a scale of 1–5 (Table 3). Additionally, the criteria for root containers and their level of importance, including humidity conditions of the site, dimensions according to size, infiltration area, soil compaction, and container enclosure, are shown in Table 6.
3.2 Species selection
From the 43 species suggested by UFHB, we kept the native origin ones with maximum adaptability to specific humidity areas and less affected by air pollution (medium PM10 concentration between 81 mg/m3 and 135 mg/m3). We scored each species under the criteria of least interference with the public space with values from 0 to 3 for each characteristic analyzed, such as root system, intrusiveness, rusticity, forestry treatments resistance, crown diameter, stem, leaves, size, growing, life cycle, origin, and wildlife attraction.
It is noticed that the values given to each species in Table 5 indicate their level of adaptability to each humidity zone from Bogotá related to MAP, ranging from 0 (null) to 5 (optimal) (JBB and De, 2010). Species with values between 4 and 5 are recommended depending on the location area to guarantee the tree’s health without irrigation. Finally, the definitive list includes species with reported benefits for avifauna as Calliandra carbonaria and Tibouchina lepidota (Corzo, 2019), suitable in high-traffic avenues as Croton bogotanus (Ramos-Montaño, 2020), and for atmospheric particle adsorption as Dodonaea viscosa (Khalilimoghadam et al., 2021) and Tecoma stans in Sapkota and Devkota (2021).
3.3 Root container evaluation
According to the sidewalk guidelines, Table 7 shows the root container dimensioning for the selected species. When calculating the relationship between the length of the container and the dimension for living space for container type B22 (medium), an average value of 72% is obtained, and for container type B23 (large), an average value of 55%. On the other hand, for the root’s container type B24 in the recommended species, the relationship between the length of the container and the dimension for living space reaches an average of 90% for medium-sized species and 77% for species of tall size. It indicates that to prevent damage to the public space infrastructure as the tree grows in type B22 and B23 containers, it is necessary to increase the dimensions for medium and tall trees. The B24 container of rectangular geometry and variable depth dimensions, depending on the size, offers more space for developing medium and tall trees, without being optimal.
To cope with this and in order to maximize the expansion potential of uncompacted soil, we advocate for the utilization of staggered root containers. These containers have a surface dimension that can expand according to the growth of the trunk, up to the limit of the landscaping strip and with a second level that leads to the expansion of the roots to greater depth. The proposed solution is shown in Figure 3, with detailed surface widths provided in Table 8. The proposed variable depths consider the first step to be approximately 50% of the total depth and then widen along the length according to the diameter of the crown between 1.2 m and 4 m. Furthermore, to enhance underground water recharge, we propose incorporating a granular material at the container’s base. This facilitates infiltration into the natural terrain wherever feasible.
3.4 Water comfort analysis
Figure 4 (top) illustrates the MAP data for the 50 stations studied and their distribution across different zones: 11 in humid, 13 in sub-humid, 10 in semi-dry, and 16 in dry zones. Figure 4 (bottom) depicts the number of consecutive dry days ds, which is 6 days for humid and sub-humid zones and 8 days for semi-dry and dry zones. In Table 9, the daily water requirement and reserve volume for dry weather are listed based on the location area for each species. Additionally, Table 10 provides the dimensions of each tree pit for different zones, with a ponding depth of 15 cm. It is observed that species with maximum adaptability to dry and semi-dry zones have lower space requirements, while taller species that are better suited for humid and sub-humid areas require more space. Notably, the water requirement only influences the sizing of the tree pit based on criterion 1, specifically when Senna viarum is employed.
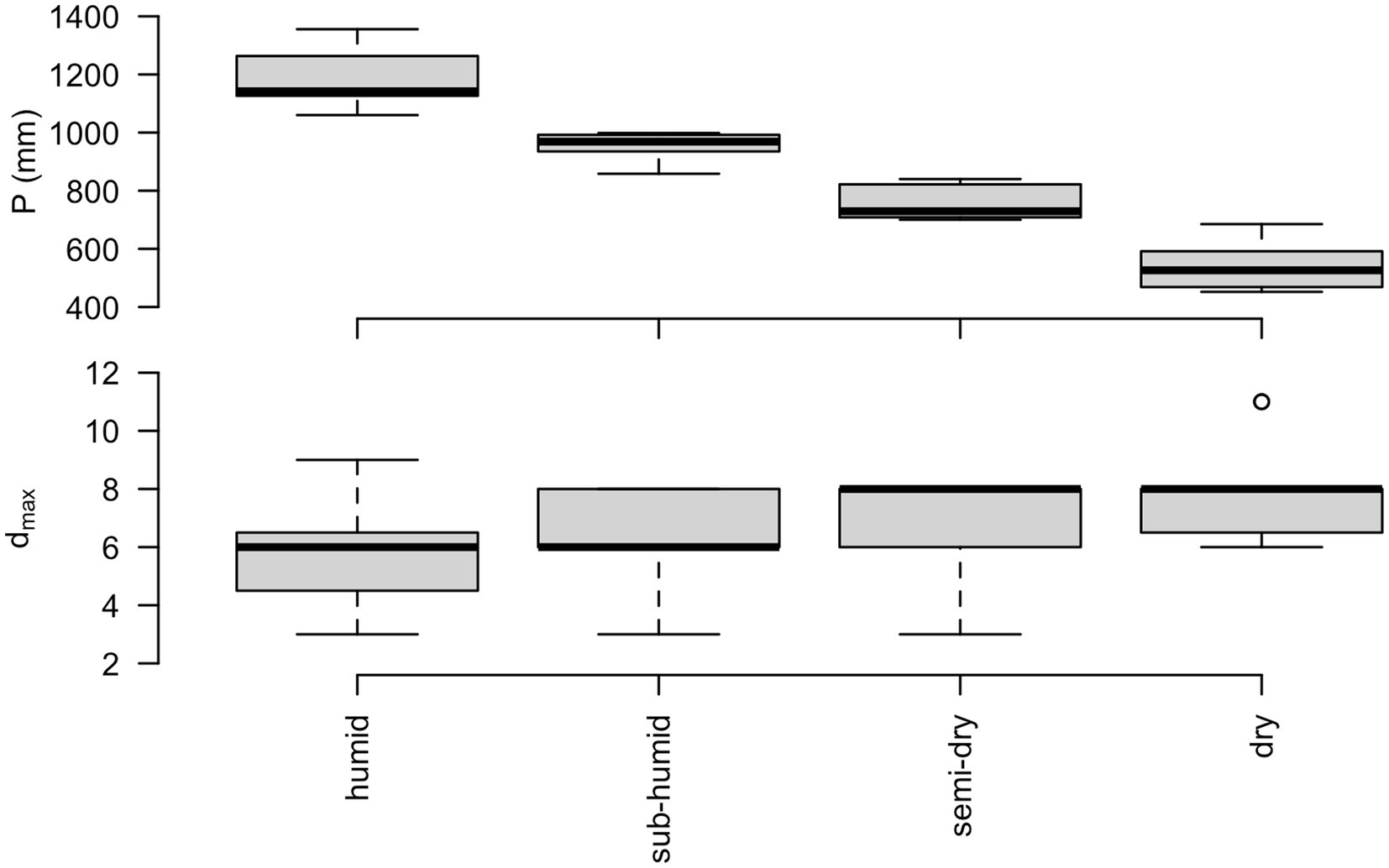
Figure 4. Boxplots showing the distribution of mean annual precipitation in the stations of each humidity zone (top) and the maximum number of consecutive days of dry weather in each humidity zone (bottom). Box limits denote the 25th (Q1) and 75th (Q3) percentiles, with medians indicated by central lines. Minimum and maximum values exclude outliers, defined as observations falling below Q1–1.5 × IQR or exceeding Q3 + 1.5 × IQR, where IQR is the interquartile range. Source: Authors.
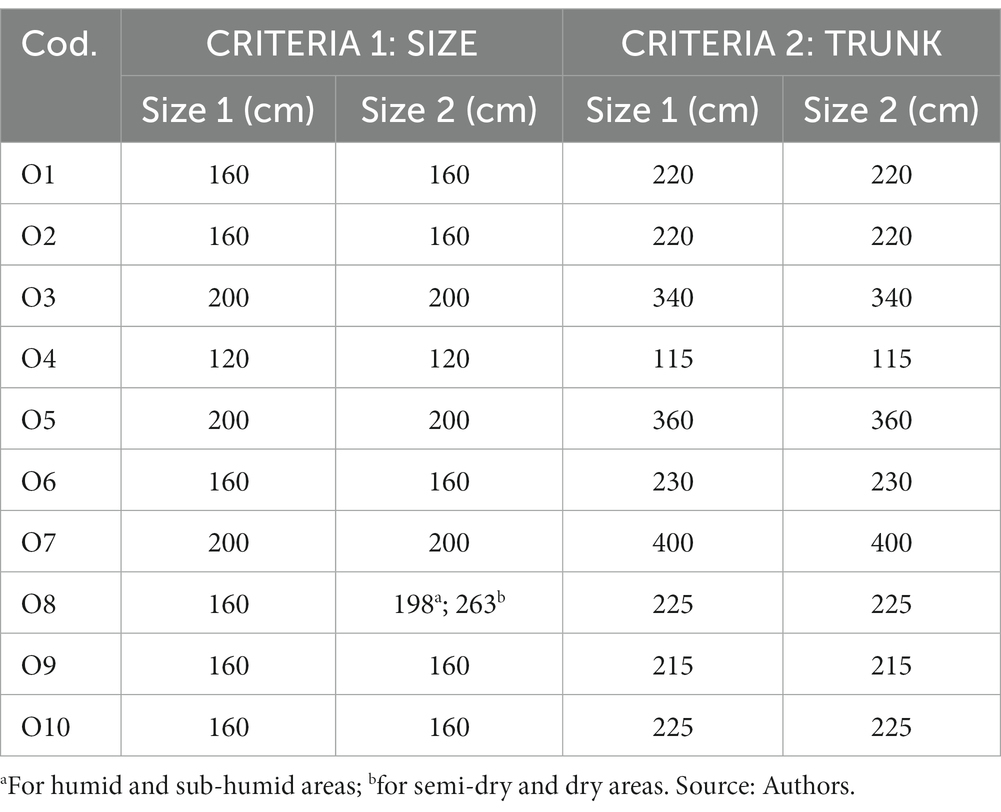
Table 10. Plant dimensions of tree pits in humid, sub-humid, semi-dry, and dry zones for 15-cm ponding depths.
According to the zone, the hydric comfort of each species considers the minimum design afferent area. In addition, the minimum distance between tree pits is calculated according to their location in the street profiles proposed in the sidewalk guide, ranging from 8 m for V-9 pedestrian paths to 100 m for V-0 integral ways, and in Table 11, there is an example for the V-0 and V-1 routes. Similarly, minimum distances between tree pits of less than 10 m are obtained on routes V-0 to V-7 (13 m) for the recommended species Croton bogotanus, Calliandra carbonaria, and Tibouchina lepidota in sub-humid zones, Tecoma stans and Cytharexylum subflavescens in sub-humid and semi-dry areas and Morella parvifolia in semi-dry and dry areas. These results respond to that indicated by Caplan et al. (2019) in that it is necessary to select the tree species adapted to the extremes of the hydrological regime in the green stormwater infrastructures.
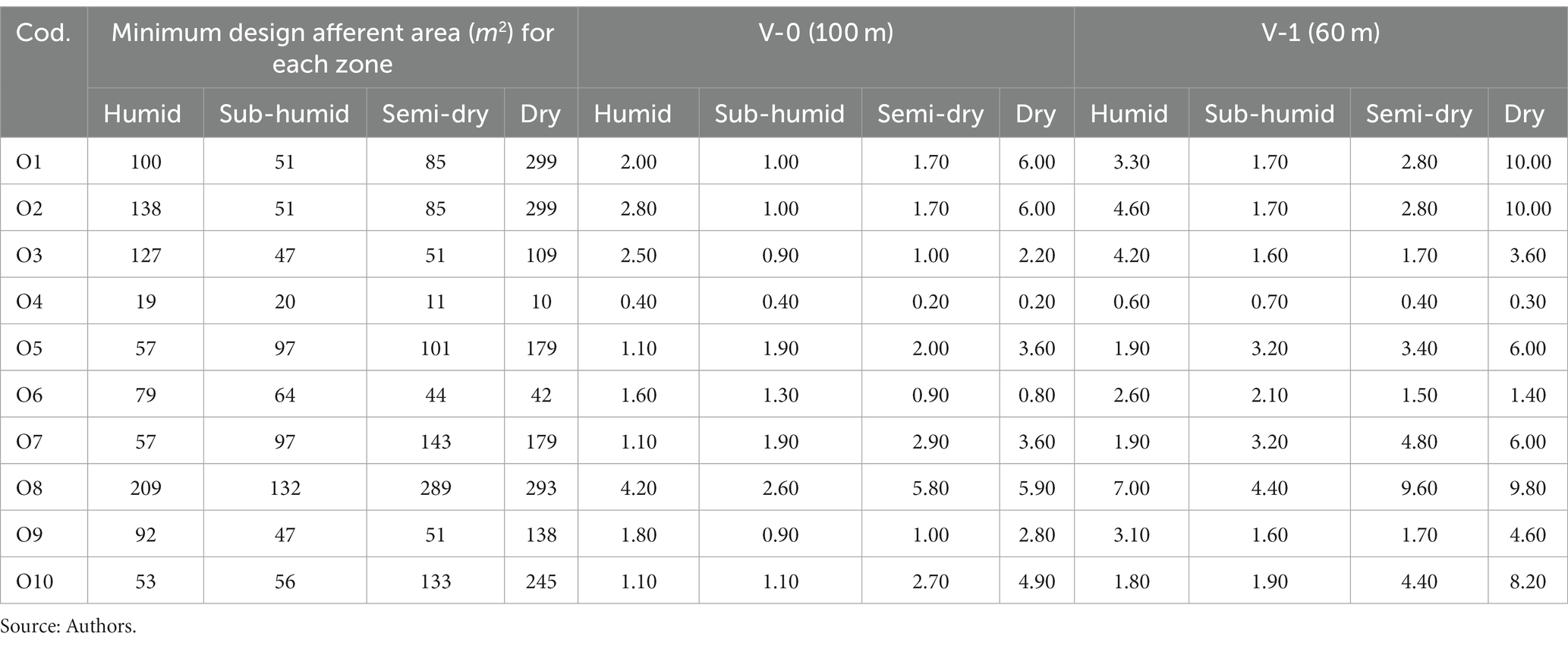
Table 11. Minimum design afferent area, for each species depending on its location area and minimum distance between tree pits (m) for routes V-0 and V-1, for hydric comfort.
4 Discussion
The key interaction factors results vary with previously proposed species selection criteria, including species characteristics such as growth, site factors, costs, aesthetics, management, and maintenance issues (Roy et al., 2017; Ghafari et al., 2020; Pham and Van Nguyen, 2021) since these studies did not seek to reduce the damage to the built infrastructure. By setting up the importance of each characteristic, secondary source information is complemented with the multicriteria analysis to propose a list of species compatible with the public space. Similarly, the criteria of multifunctionality are adopted as the ability to solve various environmental and social challenges of the urban context through the increase of space for nature, designed and managed strategically to favor human well-being and biodiversity (Figueroa Arango, 2020).
For the present case study, the final list is extremely limited due to the initial shortage of species included in the Urban Forestry Handbook of Bogotá, which contains only 18 native species resistant to air pollution and highly adaptable to humidity zones in Bogotá. Hence, we confirm a need for research on local flora to incorporate it into the city’s GI. This is particularly crucial given that a substantial portion of prevailing urban forestry research originates from developed countries, and its applicability to urban areas in developing countries may be limited (Roman et al., 2021). As outlined in the review article by Barona et al. (2020), research on urban forestry in Latin America and the Caribbean has been somewhat limited. The predominant focus of most articles has been on ecological studies utilizing field surveys to investigate the diversity of urban vegetation. However, a significant oversight has been the minimal integration of social or management considerations in these studies. A smaller proportion of research delved into spatiotemporal dynamics, and an even more limited fraction explored the direct opinions of stakeholders.
Furthermore, these findings have practical implications for urban planning in Bogotá and other cities, enhancing local guidelines by introducing greater flexibility in side dimensions for tree pits. This involves incorporating area and volume parameters into the boxes, with a specific focus on safeguarding infrastructure and promoting tree development. By reconceptualizing urban trees as dynamic systems interacting with infrastructure, we can mitigate public space damage, empowering municipal authorities to plant more trees and foster the resilience of cities. It is imperative to ensure that the root container for each tree is generously proportioned in all dimensions to facilitate optimal tree growth (Álvarez et al., 2020). A similar analytical approach, utilizing appropriate species and reassessing local parameters for urban planning, can be applied to other cities.
On the other hand, from the multicriteria analysis with the ELECTRE I method and the expert survey results, we obtained a list of eight species suitable for planting in Bogotá sidewalks, according to their low interference with public space. The local forestry expert indicated that Salix humboldtiana is unsuitable because its roots extend to meet the water in lower strata and affect the infrastructure (Hernández-Leal et al., 2019). Three or more species with optimal adaptability to the humid, sub-humid, and semi-dry zones are observed in the list, while only one species is adapted to the dry zone. Therefore, to promote heterogeneity, the species Dodonaea viscosa was included, which meets the desirable characteristics due to low interference.
However, this method is proposed as an aid to decision-making, which implies not neglecting the criteria of experts or decision-makers to confirm the proposed list of species: In our case, a local expert finally excluded one of the species initially included from the multicriteria analysis method. Furthermore, to deepen the analysis, it is necessary to review the state of the road corridors with damage to the infrastructure caused by the trees and cross it with the data of the species planted there (Otero-Durán, 2021b).
The volume of the recommended tree pits by local guidelines does not comply with the NACTO recommendation (NACTO, 2017), and for the medium and tall species (underlined values), the required living space of the tree exceeds the dimension of type B22, B23, and B24 containers. Similarly, the dimensions proposed are lower than those that would have been obtained using the methods presented by Jim (2019), possibly because our study’s methodology is adapted both to the hydrological conditions of Bogotá and to the native species contemplated. Nevertheless, our sizing method for the root containers yielded comparable dimensions to those recommended by NACTO. This may be because the method proposed in the present study is more adapted to the local species and climate. In addition, we recommend rethinking the dimensions techniques of the root containers to optimize the provision of ecosystem services associated with trees and reduce interference with gray infrastructure, especially for tropical cities (Table 6).
To integrate all the discussed results from the infrastructure protection approach, we propose staggered root containers with a surface that allows enlargement according to the growth of the trunk and, at most up, to the limit of the landscape and furniture stripe and with a lower level that leads to the expansion of the roots. In the lower part of the pit, a material for infiltration where possible. With these types of containers, an increase in the soil volume of 40% is achieved, allowing better conditions for tree development and ecosystem services and reducing the infrastructure’s damage (Table 8 and Figure 3).
The hydrological sizing methods proposed here for tree pits depend both on the species to be planted and the characteristics of the streets. It implies that the design of tree pits cannot be thoroughly homogenized. For example, when introducing a new species, it is necessary to perform specific calculations for that species concerning its intended path. Additionally, we note that the results obtained may represent an additional decision-making aid to select species according to the humidity zones of the city and the road profile because (i) the species with maximum adaptability to the driest zones have lower space requirements than the taller species and adapted to the more humid zones and (ii) there are species with greater versatility than others in terms of distance between tree pits.
However, for future studies, we recommend considering the effects of climate change on dry/rainy periods (i.e., David et al., 2018) since our hydrological design method relies on estimating days of dry weather in specific city areas. Similarly, it is necessary to account for more detailed trees’ physiological responses to these conditions (Gebert et al., 2019). Note that in addition to hydrological criteria, the definitive distance between tree pits must include landscape criteria and interactions with urban furniture, depending on the size of each tree. Based on all the findings, design guidelines could be formulated with suitable root containers for the selected species that positively interact with the public space and provide the water regulation service.
5 Conclusion
This study highlights the importance of carefully selecting tree species to achieve specific ecosystem goals based on the case study. The results obtained for the proposed case study (Bogotá) further underscore the significance of considering the climatic dynamics of large cities, which has substantial implications for the appropriate selection of tree species. In other words, it is not feasible to rely solely on a list of species and randomly choose them for any area within the city. Similarly, it was demonstrated that standardized tree pit dimensions and distances between them cannot be universally applied, as these factors depend on the pursued ecosystem goal, the city’s zone, and the tree species.
The analysis conducted led to specific recommendations for the case study, which could streamline urban planning and construction activities. For the proposed case study, a limited list of species was identified, facilitating decision-making in tree management within the city. This study underscores the ongoing need for research on key aspects of urban forestry in cities in developing countries.
Data availability statement
The raw data supporting the conclusions of this article will be made available by the authors, without undue reservation.
Author contributions
LO-D: Conceptualization, Data curation, Formal analysis, Investigation, Methodology, Project administration, Resources, Visualization, Writing – original draft, Writing – review & editing. AT: Conceptualization, Data curation, Formal analysis, Funding acquisition, Methodology, Software, Supervision, Writing – review & editing.
Funding
The author(s) declare that no financial support was received for the research, authorship, and/or publication of this article.
Acknowledgments
The authors want to thank Pedro Calaza for encouraging them to publish the results of the research carried out at Javeriana University between the engineering faculty and the environmental and rural studies faculty. The authors also appreciate the contribution of the experts with the answers to the survey carried out on the interaction of urban trees and public space.
Conflict of interest
The authors declare that the research was conducted in the absence of any commercial or financial relationships that could be construed as a potential conflict of interest.
Publisher’s note
All claims expressed in this article are solely those of the authors and do not necessarily represent those of their affiliated organizations, or those of the publisher, the editors and the reviewers. Any product that may be evaluated in this article, or claim that may be made by its manufacturer, is not guaranteed or endorsed by the publisher.
References
Alberti, M. (2008). Advances in urban ecology: Integrating humans and ecological processes in urban ecosystems.
Álvarez, G., Serrano, C., Duque, R., Guzmán, D., Carvajal, L., Arteaga, S., et al. (2020). Manual de coberturas vegetales para Bogotá, D. C. Available at: https://arqdis.uniandes.edu.co/publicaciones/manual-de-coberturas-vegetales/
Arcos-LeBert, G., Aravena-Hidalgo, T., Figueroa, J. A., Jaksic, F. M., and Castro, S. A. (2021). Native trees provide more benefits than exotic trees when ecosystem services are weighted in Santiago, Chile. Trees 35, 1663–1672. doi: 10.1007/s00468-021-02144-5
Barona, C. O., Devisscher, T., Dobbs, C., Aguilar, L. O., Baptista, M. D., Navarro, N. M., et al. (2020). Trends in urban forestry research in Latin America & the Caribbean: a systematic literature review and synthesis. Urban For. Urban Green. 47:126544. doi: 10.1016/j.ufug.2019.126544
Calaza Martínez, P. (2019). Guía de la Infraestructura verde municipal. Available at: https://www.aepjp.es/wp-content/uploads/2019/07/AEPJP-Guia-Biodiversidad.pdf
Caplan, J. S., Galanti, R. C., Olshevski, S., and Eisenman, S. W. (2019). Water relations of street trees in green infrastructure tree trench systems. Urban For. Urban Green. 41, 170–178. doi: 10.1016/j.ufug.2019.03.016
Carlyle-Moses, D. E., Livesley, S., Baptista, M. D., Thom, J., and Szota, C. (2020). Urban trees as green infrastructure for stormwater mitigation and use. Forest Water Interact. 240, 397–432. doi: 10.12804/revistas.urosario.edu.co/territorios/a.6253
Corzo, G. T. (2019). Handling of avifauna as part of the Management of Urban Trees in Bogotá, DC. Territorios. 40, 83–117. doi: 10.1007/978-3-030-26086-6_17
Dahlhausen, J., Biber, P., Rötzer, T., Uhl, E., and Pretzsch, H. (2016). Tree species and their space requirements in six urban environments worldwide. Forests 7, 111. doi: 10.3390/f7060111
David, A. A., Boura, A., Lata, J. C., Rankovic, A., Kraepiel, Y., Charlot, C., et al. (2018). Street trees in Paris are sensitive to spring and autumn precipitation and recent climate changes. Urban Ecosyst. 21, 133–145. doi: 10.1007/s11252-017-0704-z
Day, S. D., and Dickinson, S. B. (2008). Managing Stormwater for urban sustainability using trees and structural soils. Virginia Polytechnic Institute and State University: Blacksburg, VA, 63
de Acueducto, Empresa y de Bogotá, Alcantarillado . (2018) Norma Técnica NS-166. Criterios para Diseño y Construcción de Sistemas Urbanos de Drenaje Sostenible. Available at: https://www.acueducto.com.co/wps/portal/EAB2/Home/acueducto-y-alcantarillado/normalizacion-tecnica
Devia, C., and Torres, A. (2019). Atenuación de la temperatura y radiación UV de la vegetación en entornos urbanos de ciudades y su demanda hídrica. Revista Luna Azul 49, 200–219. doi: 10.17151/luaz.219.49.12
Döhren,, and Haasen, (2019). Risk assessment concerning urban ecosystem disservices: The example of street trees in Berlin, Germany. Ecosystem Services. 40.
Elliott, R. M., Adkins, E. R., Culligan, P. J., and Palmer, M. I. (2018). Stormwater infiltration capacity of street tree pits: quantifying the influence of different design and management strategies in new York City. Ecol. Eng. 111, 157–166. doi: 10.1016/j.ecoleng.2017.12.003
Empresa de Acueducto y Alcantarillado de Bogotá (2018). Norma Técnica NS-166. Criterios para Diseño y Construcción de Sistemas Urbanos de Drenaje Sostenible. Available at: https://www.acueducto.com.co/wps/portal/EAB2/Home/acueducto-y-alcantarillado/normalizacion-tecnica
Escobedo, F. J., Clerici, N., Staudhammer, C. L., and Corzo, G. T. (2015). Socio-ecological dynamics and inequality in Bogotá, Colombia’s public urban forests and their ecosystem services. Urban Forestry Urban Greening 14, 1040–1053. doi: 10.1016/j.ufug.2015.09.011
Escobedo, F. J., Giannico, V., Jim, C. Y., Sanesi, G., and Lafortezza, R. (2019). Urban forests, ecosystem services, green infrastructure and nature-based solutions: Nexus or evolving metaphors? Urban Forestry Urban Greening 37, 3–12. doi: 10.1016/j.ufug.2018.02.011
Figueira, J., Mousseau, V, and Roy, B. (Eds.) (2005). ELECTRE methods. In Multiple criteria decision analysis: State of the art surveys. Boston, MA: Springer Science + Business Media. 133–153.
Figueroa Arango, C. (2020). Guía para la integración de las Soluciones Basadas en la Naturaleza en la planificación urbana. Primera aproximación para Colombia. Available at: http://hdl.handle.net/20.500.11761/35585
Galarza Molina, S., Torres, A., and Mendez, S. (2014). Herramientas multi-criterio para la toma de decisiones en proyectos de carácter social. Available at: https://www.researchgate.net/publication/262039475_Herramientas_multi-criterio_para_la_toma_de_decisiones_en_proyectos_de_caracter_social
Gebert, L. L., Coutts, A. M., and Tapper, N. J. (2019). The influence of urban canyon microclimate and contrasting photoperiod on the physiological response of street trees and the potential benefits of water sensitive urban design. Urban For. Urban Green. 40, 152–164. doi: 10.1016/j.ufug.2018.07.017
Ghafari, S., Kaviani, B., Sedaghathoor, S., and Allahyari, M. S. (2020). Ecological potentials of trees, shrubs and hedge species for urban green spaces by multi criteria decision making. Urban For. Urban Green. 55:126824. doi: 10.1016/j.ufug.2020.126824
Hernández-Leal, M., Suárez-Atilano, M., Piñero, D., and González-Rodríguez, A. (2019). Regional patterns of genetic structure and environmental differentiation in willow populations (Salix humboldtiana Willd). Ecol. Evol. 9, 9564–9579. doi: 10.1002/ece3.5475
JBB, J. B, and De, B. J. C. M. (2010). Manual de Silvicultura Urbana de Bogotá. Available at: https://www.ambientebogota.gov.co/documentos-especificos-silvicultura
Jim, C. Y. (2019). Soil volume restrictions and urban soil design for trees in confined planting sites. J. Landscape Architect. 14, 84–91. doi: 10.1080/18626033.2019.1623552
Khalilimoghadam, B., Siadat, S. A., Yusefi, A., and Negaresh, K. (2021). Atmospheric particle adsorption rates of plants in an industrial city of Southwest Iran. Aeolian Res. 53:100752. doi: 10.1016/j.aeolia.2021.100752
Lyytimäki, J., and Sipilä, M. (2009). Hopping on one leg – the challenge of ecosystem disservices for urban green management. Urban For. Urban Green. 8, 309–315. doi: 10.1016/j.ufug.2009.09.003
Mcpherson, B. E. G., and Muchnick, J. (2005). Effects of street tree shade on asphalt concrete pavement performance 31, 303–310. doi: 10.48044/jauf.2005.039,
Meili, N., Acero, J. A., Peleg, N., Manoli, G., Burlando, P., and Fatichi, S. (2021). Vegetation cover and plant-trait effects on outdoor thermal comfort in a tropical city. Build. Environ. 195:107733. doi: 10.1016/j.buildenv.2021.107733
Morakinyo, T. E., Ouyang, W., Lau, K. K. L., Ren, C., and Ng, E. (2020). Right tree, right place (urban canyon): tree species selection approach for optimum urban heat mitigation-development and evaluation. Sci. Total Environ. 719:137461. doi: 10.1016/j.scitotenv.2020.137461
Mullaney, J., Lucke, T., and Trueman, S. J. (2015a). A review of benefits and challenges in growing street trees in paved urban environments. Landsc. Urban Plan. 134, 157–166. doi: 10.1016/j.landurbplan.2014.10.013
Mullaney, J., Lucke, T., and Trueman, S. J. (2015b). The effect of permeable pavements with an underlying base layer on the growth and nutrient status of urban trees. Urban Forestry Urban Greening 14, 19–29. doi: 10.1016/j.ufug.2014.11.007
NACTO . (2017). Urban Street Stormwater Guide. Island Press. Available at: http://ezproxy.javeriana.edu.co:2048/login?url=https://search.ebscohost.com/login.aspx?direct=true&db=nlebk&AN=1843207&lang=es&site=eds-live
Núñez-Florez, R., Pérez-Gómez, U., and Fernández-Méndez, F. (2019). Functional diversity criteria for selecting urban trees. Urban For. Urban Green. 38, 251–266. doi: 10.1016/j.ufug.2019.01.005
Otero-Durán, L. P. (2021a). El arbolado urbano protector de la infraestructura de espacio público en Bogotá. Available at: http://hdl.handle.net/10554/53173
Otero-Durán, L. P. (2021b). “Urban forestry in sidewalks of Bogotá, Colombia” in The Palgrave encyclopedia of urban and regional futures. ed. R. Brears (Cham: Palgrave Macmillan)
Pham, T. A., and Van Nguyen, M. (2021). Assessment of plant species for the roadside at Vung Tau City of Vietnam using multi-criteria analysis In Proceedings of the international conference on innovations for sustainable and responsible mining (pp. 124–148). Cham
Ramos-Montaño, C. (2020). Effects of vehicle emissions on physiology and health of five urban tree species in Bogotá, Colombia. Revista Biol. Trop. 68, 1001–1015. doi: 10.15517/rbt.v68i3.40248
Roman, L. A., Conway, T. M., Eisenman, T. S., Koeser, A. K., Ordóñez Barona, C., Locke, D. H., et al. (2021). Beyond ‘trees are good’: disservices, management costs, and tradeoffs in urban forestry. Ambio 50, 615–630. doi: 10.1007/s13280-020-01396-8
Roy, S., Davison, A., and Östberg, J. (2017). Pragmatic factors outweigh ecosystem service goals in street tree selection and planting in south-East Queensland cities. Urban For. Urban Green. 21, 166–174. doi: 10.1016/j.ufug.2016.12.003
Santamour, F. S.Jr. (1990). Trees for urban planting: diversity, uniformity, and common sense. Proc. 7th Conf. Metropolitan Tree Improvement Alliance (METRIA). Available at: https://agroforestry.org/the-overstory/144-overstory-126-trees-for-urban-planting-diversity-uniformity-and-common-sense
Sapkota, S., and Devkota, A. (2021). Comparative assessment of biochemical characteristics of plants from polluted and non-polluted regions of urban areas. Oct. J. Env. Res. 9, 1–12.
Scholz, T., Hof, A., and Schmitt, T. (2018). Cooling effects and regulating ecosystem services provided by urban trees—novel analysis approaches using urban tree cadastre data. Sustain. 10, 172.
Tzoulas, K., Korpela, K., Venn, S., Yli-Pelkonen, V., Kaźmierczak, A., Niemela, J., et al. (2007). Promoting ecosystem and human health in urban areas using green infrastructure: a literature review. Landsc. Urban Plan. 81, 167–178. doi: 10.1016/j.landurbplan.2007.02.001
Keywords: nature-based solutions, ecosystem services and disservices, green infrastructure, tree hydric comfort, landscaping
Citation: Otero-Durán L and Torres A (2024) Trees and sidewalks: toward an infrastructure protection approach. Front. Sustain. Cities. 6:1336472. doi: 10.3389/frsc.2024.1336472
Edited by:
Graziano Salvalai, Polytechnic University of Milan, ItalyReviewed by:
Juan Diego Blanco Cadena, Polytechnic University of Milan, ItalySalvador García-Ayllón Veintimilla, Polytechnic University of Cartagena, Spain
Copyright © 2024 Otero-Durán and Torres. This is an open-access article distributed under the terms of the Creative Commons Attribution License (CC BY). The use, distribution or reproduction in other forums is permitted, provided the original author(s) and the copyright owner(s) are credited and that the original publication in this journal is cited, in accordance with accepted academic practice. No use, distribution or reproduction is permitted which does not comply with these terms.
*Correspondence: Laura Otero-Durán, bGF1cmEub3Rlcm9AaWR1Lmdvdi5jbw==