- Department of Psychiatry and Psychotherapy, University of Bonn, Bonn, Germany
Although body size misperceptions are known to occur under various neurological and psychiatric conditions, their neurocognitive underpinnings are not yet resolved. Accordingly, it would be beneficial to have an experimental paradigm, by which aberrant body misperceptions could be induced and systematically investigated. Expanding upon the “Virtual Hand Illusion” (VHI), this study aimed to design such a paradigm. Using a body-motion tracking system, we translated the participant's real hand position and movements to an embodiable, but resizable virtual hand model that we presented to the participants via a head-mounted display. The virtual hand's size was then systematically shrunk and enlarged in five different conditions (i.e., −50%, −25%, 0%, +25% or +50% rescaling). Applying this VHI derivate on n = 35 healthy participants, we investigated (1) if participants experience Sense of Ownership (SoO) and Sense of Agency (SoA) over a virtual hand that significantly deviates in size from their own hand, and (2) if by such size-deviant VHI induction, a change in their own hand size perception is also induced. Virtual hand embodiment was explicitly and implicitly assessed by means of self-report and EDA analysis. Questionnaire results revealed a stable SoA across all hand size conditions, while SoO parametrically decreased according to the hand scaling factor in either direction. Hand size perception, in turn, adapted according to the hand-scaling factor. In conclusion, the present study provides an important step toward an experimental paradigm that can induce and investigate aberrant body-size misperceptions.
Introduction
Usually, we do not reflect upon our body sensations, but pre-reflectively take them for granted and as veridical. Several clinical and non-clinical conditions exist, however, that expose a great malleability in how we perceive our body and what we perceive as our body (Dieguez and Lopez, 2017). Patients suffering from the “Alice in Wonderland” syndrome (AIWS), for instance, may perceive their whole body, or parts of it, as disproportionally small (microsomatognosia) or enlarged (macrosomatognosia) as two of their most common symptoms (Weissenstein et al., 2014; Blom, 2016). One middle-aged man, suffering from an anxiety disorder, reported feelings of full-body micro- and macrosomatognosia that varied from feeling eight feet to three feet tall (Todd, 1955). Another patient with migraine headaches, in turn, reported her whole body to be experientially shrinking, yet at the same time perceiving her hands to be “ginormous” (Kew et al., 1998). While AIWS has been particularly observed in the context of paroxysmal neurological disorders and infectious diseases (Blom, 2016), further clinical cases of micro- and macrosomatognosia have been documented after stroke (Rode et al., 2012; Weijers et al., 2013), after epileptic seizures (Dieguez and Lopez, 2017) or in association with depressive disorders (Bui et al., 2010). Moreover, body size misperceptions have been reported in healthy persons, for instance during hypnagogic hallucinations (Podoll et al., 1998) or during local anaesthesia (Paqueron et al., 2003).
Although some evidence exists that body size misperceptions are accompanied by neural activity changes in the somatosensory areas (Ehrsson et al., 2005b), the neurocognitive mechanisms underlying body size misperceptions remain largely unknown. In part, this lack of knowledge might be due to the fact that body size misperceptions occur rather spontaneously and as a concomitant symptom, which hampers their systematic investigation. Hence, what appears missing is an experimental paradigm by which aberrant body size perceptions can also be induced in healthy participants. Even if such a paradigm failed to completely imitate the complex phenomenology of AIWS in all its possible manifestations, the isolated experimental inducibility of body size misperceptions as a key symptom would be a valuable starting point to unravel the core mechanisms underlying AIWS. A promising approach to developing such a new paradigm could lie in a further development of existing body transfer illusion (BTI) paradigms. BTIs are a class of perceptual illusions that share the induction of an illusory sense of ownership (SoO), and sometimes also sense of agency (SoA), over an artificial or virtual limb (Kilteni et al., 2015; Dieguez and Lopez, 2017; Braun et al., 2018). That is, participants undergoing a BTI perceive the illusion that a presented artificial or virtual limb forms part of their own biological body (SoO) and that they can control the artificial or virtual limb's movements and actions (SoA).
One popular BTI is mirror visual feedback (MVF) (Ramachandran and Altschuler, 2009; Wittkopf et al., 2017). In this paradigm, one hand of a participant is positioned behind a mirror and the other hand in front of the mirror. The exposed hand is thereby positioned in such a way that its reflection is superimposed on the position of the hidden hand behind the mirror. If participants then move their exposed hand and concomitantly look into the mirror, they typically begin to experience SoO and SoA for the virtual hand displayed in the mirror (Wittkopf et al., 2017). This embodiment effect is evident in participants' subjective reports as well as implicitly demonstrated by a “proprioceptive drift” toward the mirror reflection. That is, participants blindly locate their hidden hand to be closer to their body and the mirror than it actually was.
Another prominent BTI is the “rubber hand illusion” (RHI) (Botvinick and Cohen, 1998), in which an artificial hand is positioned in an anatomically-plausible position in front of the participant, while the participant's equilateral real hand becomes occluded from view. If both the artificial and the participant's own hidden hand are then simultaneously stroked (Botvinick and Cohen, 1998), or moved (Kalckert and Ehrsson, 2012; Braun et al., 2014), a majority of participants begin to experience SoO toward the artificial hand (Braun et al., 2018). This effect can be explicitly inferred from subjective ratings, as well as implicitly concluded (e.g., from physiological responses toward an apparently painful manipulation to the artificial hand) (Braun et al., 2018).
A third way to induce BTIs is by means of virtual reality (VR). A virtual realisation of the RHI is the “virtual hand illusion” (VHI) (Slater et al., 2008, 2009; Sanchez-Vives et al., 2010). In this BTI variant, a virtual hand is presented to the participant via a head-mounted display (HMD) or similar device, such that the virtual hand appears to originate from the participant's own right shoulder. If the virtual hand and the participant's real hand are then synchronously stroked (Slater et al., 2008) or moved (Sanchez-Vives et al., 2010), a comparable illusory SoO over the virtual hand may be induced as with the classical RHI. One advantage of VR-based BTI settings over mechanical BTI settings is that they allow for a broader and more flexible range of experimental manipulations, such as the dynamical rescaling of the body parts presented, or the online changing of their appearance. Moreover, VR-based BTIs often allow a more fine-grained and reliable movement animation of the virtual hand.
Assuming that body size perception depends on bottom-up sensory stimulation, a BTI-based induction of aberrant body size perceptions could potentially be realised by presenting the artificial or virtual hand in a size that strongly deviates from a participant's own hand size. Given that the artificial or virtual hand presented remains embodiable, the working assumption would be that under such stimulus situations, the inner body size perception will adapt to the size of the embodied virtual or artificial hand, and thus an experience of micro- or macrosomatognosia will occur.
While the authors are not aware of any study that has specifically tested the occurrence of micro- or macrosomatognosia during BTIs, several studies have investigated the extent to which the inducibility of a BTI depends on the size of the artificial or virtual limb presented. Wittkopf et al. (2017), for instance, conducted a MVF study, in which the mirror hand reflection was magnified, minified, or non-disruptively presented. The result was that while SoO and SoA declined for the magnified and miniaturised mirror reflections as compared to the non-disruptive mirror reflection, no proprioceptive drift changes were observable between the three conditions. In line with these findings were also the RHI results by Haggard and Jundi (2009) in which the artificial hand presented was either smaller or larger than the participant's own hand. Here, likewise, a proprioceptive drift was clearly observable for both hand size conditions, as long as the hand strokes were applied synchronously. Pavani and Zampini (2007), in turn, mirrored in real-time the participant's own hand via video technology and found that a proprioceptive drift was only observable if the displayed hand size was veridical or enlarged, but not when it was smaller than the participant's real hand size. Finally, a VHI study of Lin et al. (2019), explicitly assessed SoO and SoA (besides other usability measures) under three different hand size conditions (normal, −25%, +25%) and neither found a SoO nor SoA difference between the three conditions.
Whereas the studies reviewed above provide first indications that a BTI-based induction of aberrant body size perceptions might be possible, many research questions still remain unaddressed. First, while embodiment effects toward the virtual hand have been investigated, it has not been examined how a participant's hand size perception is altered by the applied hand size manipulations. Second, all studies used either the proprioceptive drift (Pavani and Zampini, 2007; Haggard and Jundi, 2009; Wittkopf et al., 2017) or no implicit embodiment measure at all (Lin et al., 2019). The proprioceptive drift measure should, however, be interpreted with caution, given that it dissociates from SoO and also appears in experimental situations where no virtual hand embodiment is expected (Holle et al., 2011; Rohde et al., 2011). Third, all previous studies focused on rather small hand size deviations (at maximum 30%). The way SoO, SoA and micro- and macrosomatognosia evolve under larger hand size deviations, or dynamically changing hand sizes, has therefore not yet been clarified.
To address these unresolved research questions, we conducted a VHI study, in which the participant's real hand movements were translated to a realistic looking and resizable hand model and presented to the participant via a HMD. Using this general setup, we examined the VHI under five fixed hand-size conditions (i.e., −50%, −25%, 0%, +25% or +50% rescaling) as well as under two dynamically-changing hand-size conditions (shrinking condition, growing condition). We thereby focused on three major research questions: First, whether participants can experience SoO and SoA over a virtual hand that significantly deviates in size from their own hand. Second, whether such a size-deviant VHI induction can also induce a change in one's own hand size perception. And third, up to which hand-scaling factor a VHI can still be induced. To systematically assess these three questions, we relied on self-reports as well as on an implicit electrodermal embodiment measure. By answering these questions, our study might pave the ground for the experimental inducibility of aberrant body size misperceptions, such as micro- and macrosomatognosia, which could then be used to systematically examine the neurocognitive underpinnings of these syndromes.
Methods
Participants
Thirty-five participants between the ages of 18 and 32 (14 females; M = 21.685; SD = 3.056) were recruited for the study. All participants were required to have normal or correct-to-normal vision and no known history of a psychiatric or neurological disorder. Moreover, they had to give written informed consent into the study and were naive to the purpose of the study. The experiment was approved by the University of Bonn's medical ethics committee and the participants were paid 20€for their participation.
Overview
The experiment took place in the VR laboratory of the University Hospital of Bonn and lasted about 2 h, including preparation and post-processing time. Upon arrival, participants were first informed about the study's procedure and eligibility requirements and subsequently gave written informed consent for the study. Next, they filled out a demographic questionnaire that was developed by our research group and were prepared for the actual VR experiment and concomitant electrodermal recording. After that, the participants were immersed in the virtual testing environment (details below), in which they first underwent an instruction phase and then the actual experiment. The experiment itself took around 35 min and consisted of two experimental blocks. Upon completion, participants left the virtual environment and filled out a Virtual Reality Sickness Questionnaire (Kim et al., 2018) as well as an expense allowance.
Apparatus and Virtual Environment Implementation
The virtual environment and experimental apparatus are displayed in Figure 1. Participants sat in the middle of a 3.70 × 2.65 m VR-play area in front of a 1 × 1 m table and placed both of their arms onto the table. While the left hand was equipped with multiple neurophysiological sensors (details below), the right hand remained cable-free and was involved in the VHI manipulations. By means of the HMD HTC VIVE Business (HTC Corporation, Taoyuan City, Taiwan) participants were immersed in the virtual testing environment. This HMD has a 110-degree field of view, a 90 Hz screen refresh rate and a 1,080 × 1,200 image resolution per eye. Wearing the HMD, participants were immersed in a virtual imitation of our experiment room which contained the same furnishings as our real experiment room. Both the physical and virtual room were spatially mapped by positional tracking, such that the perspective of the HMD in virtual space always corresponded to the participant's perspective in real space. As in the real world, the participants were thus immersed in a virtual space position, at which they were sitting in front of a virtual table.
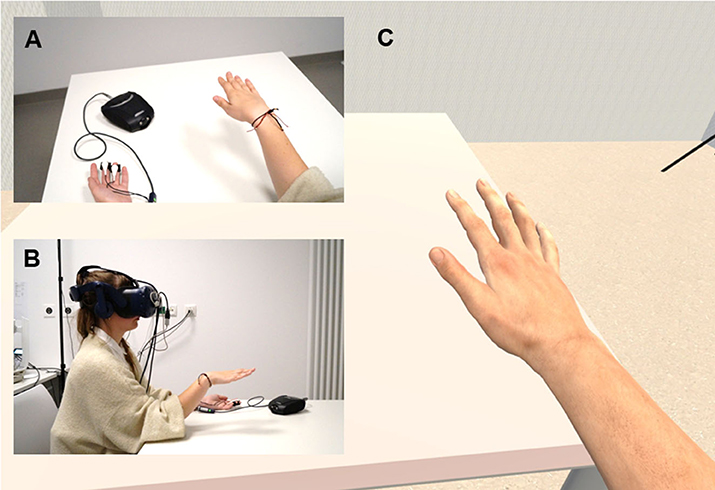
Figure 1. Experimental apparatus. (A) First person perspective of the participant in the real world. Participants were seated at a table and performed motor movements with their right hand. (B) Lateral view onto the participant in the real world. While participants were conducting their hand movements, they wore a head-mounted display (HMD) through which the virtual scenery was presented. (C) First person perspective of the participant in the virtual world (HMD view). Participants saw a right virtual hand that mimicked their own right hand's movements and which changed its size, depending on the current experimental condition.
The virtual environment was created by means of Unity 3D 2018.3.4f1 (Unity Technologies, San Francisco, CA, USA) and its interaction logic was scripted in C#. The 3D models used for recreating our experiment room and implementing the VHI were partly self-designed by means of Blender 2.79b (Stichting Blender Foundation, Amsterdam, Netherlands), and partly bought from 3D object stores (for details, see Supplementary Material). For motion-tracking the participants' real right hand, the Leap Motion system (Leap Motion Incorporation, San Francisco, CA, USA) was used. This system consists of a small USB device mounted onto the HMD's top and enables hand movements to be tracked in real-time, based on optical sensors and infrared light (Bachmann et al., 2018). Access to the functionalities of the Leap Motion system is provided as a ready-to-use software development kit (SDK) for Unity 3D (https://developer.leapmotion.com/unity; accessed 07.01.21). Using this SDK, the participants' right-hand movements were real-time tracked and immediately translated onto a rigged human hand model throughout the experiment. That is, whenever the participant moved his or her right biological hand in real space, the virtual hand always moved correspondingly and immediately in virtual space. The 3D hand model shown therein, was taken from the “Leap Motion Realistic Hands” collection (downloadable over Unity's asset store) and represented a white-coloured, average-sized, right-sided human hand. The participant's left hand was not motion-tracked and thus not visible throughout the experiment.
Instruction Phase
Before the actual experiment began, the participants underwent an instruction phase, during which they became familiarised with the VR experiment. First, the participants were instructed on how to conduct hand movements that could be reliably tracked by the Leap Motion system. To this end, participants were given prototypical examples of what limb movements typically work well with the Leap Motion system, such as moving single fingers, turning one's hand, or grab gestures. Likewise, participants were given examples of situations which oftentimes cannot be interpreted accurately. These examples included moving the hand out of sensor range or fingers occluding each other. Next, after the participants had practised for 2 min and had learnt to conduct artefact-free limb movements, they were trained in how to use the user interface (UI) that we used for the self-report measures (details in section Self-Report).
Experimental Block 1: Comparison of Different Virtual Hand Sizes
In the first experimental block (see Figure 2, upper panels), five different conditions were compared. These conditions only differed from each other in respect of the size of the virtual hand presented. More specifically, there was a baseline condition in which the virtual hand was normally scaled according to the Leap Motion's default hand size settings, and four rescaling conditions, where the virtual hand was rescaled by either +25%, +50%, −25% or −50% in volume. Each condition thereby consisted of three trials, whereby the inner trial structure always followed the same procedure: First, participants had to carry out movements with the virtual hand in accordance with how they previously practised it in the instruction phase. While conducting these movements, they had to introspectively assess as to how far the virtual hand presented felt like part of their own body and as to what extent the real hand felt being changed in size. This presentation phase of the virtual hand lasted 60 s and was immediately superseded by an assessment phase, during which the participants' phenomenal experiences were either explicitly or implicitly assessed. In two of the three trials, an explicit phenomenological assessment was carried out by an online questionnaire (see section Self-Report), whereas in the remaining trial, virtual limb embodiment was implicitly assessed via the application of a virtual syringe (see section Virtual Syringe Application and EDA Analysis). To this end, a virtual syringe appeared on the left-hand or right-hand side from behind the participant's point of view and then slowly pierced the virtual hand (see Figure 3B). All 15 trials were presented in a randomised order, whereby each trial could occur exactly once during the experiment. After ten trials, a pause of 1 min was implemented, during which the participants could relax and rest their hands.
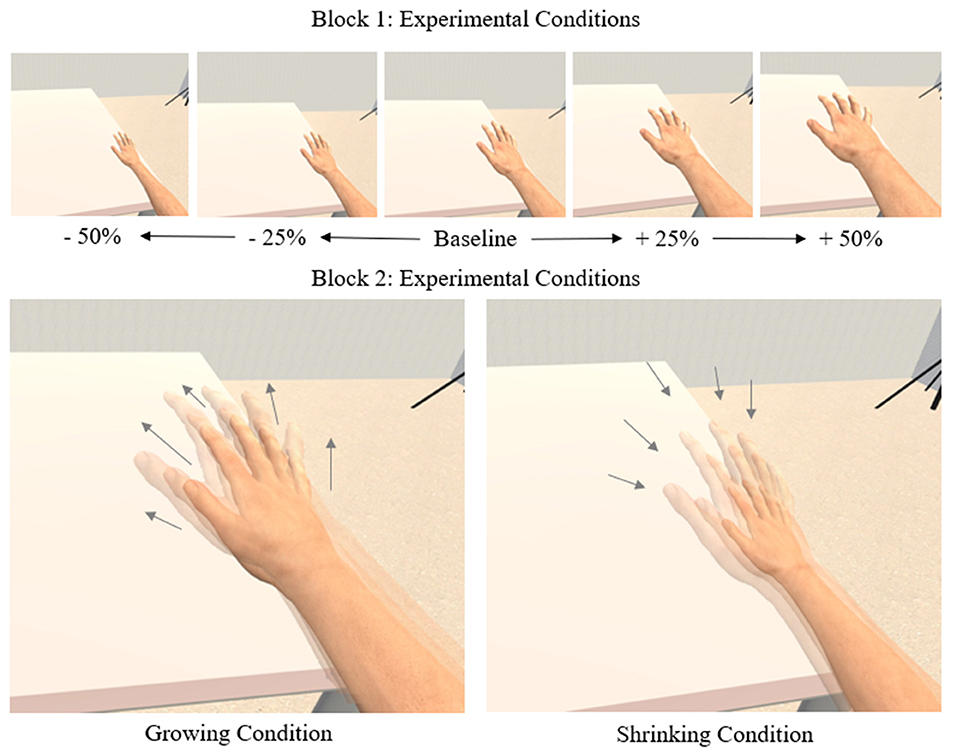
Figure 2. Experimental design. (Upper) Experimental conditions of the first experimental block. Five experimental conditions were compared that differed from each other in the displayed hand size. A veridical hand size was displayed for the baseline-condition, while the other hand sizes were rescaled by −50%, −25%, +25%, and +50%. (Lower) Experimental conditions of the second experimental block. There were two experimental conditions in block 2. At the start of a trial, the hand size started normally and then either continuously shrank (shrinking condition) or grew (growing condition) up to the point where participants indicated that they no longer perceived the virtual hand as their own.
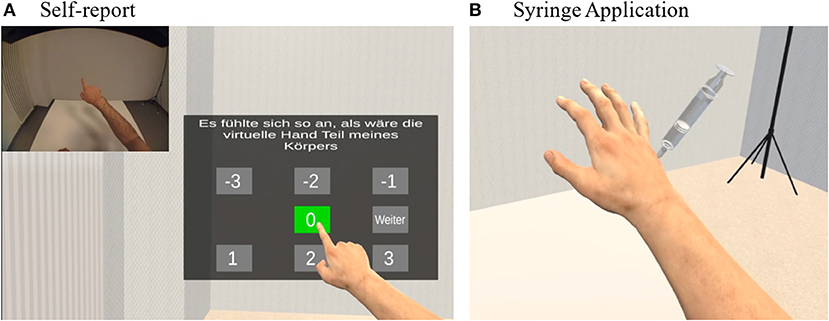
Figure 3. Explicit and implicit assessment of virtual hand embodiment. (A) Self-report. To assess participants' experiences, a virtual questionnaire system was implemented, by which participants could give their answer without having to leave the virtual environment. (B) Syringe Application. To also implicitly assess participants' SoO experience toward the virtual hand, a virtual syringe was injected to the virtual right hand. In the case of a successful illusion this should evoke a fear response measurable in the phasic parts of electrodermal activity. Figure adapted from Braun et al. (2020).
Experimental Block 2: Assessment of Individual Illusion Breakdowns
The second experimental block (see Figure 2, lower panels) investigated the perceptual threshold at which a steadily growing or shrinking virtual hand is no longer perceived as part of one's own body. That is, we explored to which rescaling factor participants still perceived the virtual hand as part of their own body. To this end, the experimental block compared two conditions, in which the virtual hand was initially presented unmodified and then was either continuously increased (growing condition) or continuously reduced (shrinking condition) in size. Each condition was tested by three trials, whereby each trial was identically structured, except for the rescaling direction: First, a 10 s countdown was blended in to indicate the beginning of the new trial. Next, the virtual hand showed up in the normal hand size and either immediately started to increase (growing condition) or to decrease (shrinking condition) in size. For each trial, participants were instructed to verbally signal as soon as they lost the feeling of ownership over the virtual hand. As soon as they gave this signal, the current trial ended, and the current virtual hand volume was automatically logged. A questionnaire was not implemented for the second block, given that we expected the VHI to have vanished. As in the first experimental block, all trials were presented in a randomised order.
Self-Report
As stated, in two thirds of the trials of the first experimental block, participants were instructed to fill in a questionnaire directly after the presentation phase. To this end, a UI was directly displayed within the virtual environment as a semi-transparent overlay (see Figure 3A). The UI thereby appeared directly within arm's reach in front of the participant and could be easily controlled by mere gesture control. To answer a question, the participant held his or her virtual index finger onto the desired answer button until this button turned green after one second and confirmed the answer by holding the virtual index finger on a confirm button for another second. The UI guided the participants through a 6-item questionnaire that was adapted from previous studies (Kalckert and Ehrsson, 2012; Braun et al., 2014). The English version of the questionnaire is depicted in Table 1. Three phenomenal target properties were assessed by the questionnaire: SoO, SoA and micro-/macrosomatognosia. SoO was defined as the experiential level of “mineness” toward the virtual hand (e.g., “It felt as if the virtual hand was part of my body”), SoA as the subjective amount of authorship over the virtual hand's movements (e.g., “It felt as if I was causing the movements of the virtual hand”), and micro-/macrosomatognosia as how size-distorted one's own biological right hand was experienced (e.g., “It felt as if my own hand was different in size”). Two items were used for each phenomenal target property and later averaged to obtain a single value for each condition and participant. The remaining three items were control items. The control items were included to cheque for potential response biases (e.g., acquisition bias, extreme response bias, social desirability bias) and to prove the illusion-specificity of our experimental manipulations. One control item related to micro-/macropsia (“It felt as if the virtual room was different in size”), which we defined as how size-distorted the virtual environment itself was perceived. The purpose of inserting this control item was to validate the specifity of micro- or macrosomatognostic feelings, that is, to confirm that the aberrant body size perceptions induced are not caused by a general size misperception. The other two control items related to SoO and SoA but did not specifically capture the phenomenal experiences of virtual hand embodiment. For each item, participants had to express their level of agreement on a 7-point Likert-scale ranging from −3 (“totally disagree”) to +3 (“totally agree”). All questions were presented in a randomised order. In line with former studies (Kalckert and Ehrsson, 2012; Braun et al., 2014), the illusion criterion for a successful induction of a phenomenal target property was set to 1. That is, an average value of ≥1 for a particular phenomenal target property was interpreted as confirmation of the respective SoO or SoA experience.
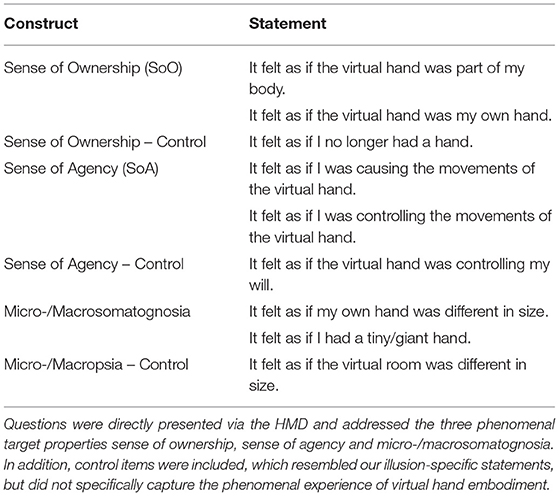
Table 1. Questionnaire (translated from German) used for the assessment of the participant's phenomenal experiences.
Virtual Syringe Application and EDA Analysis
In accordance with previous studies (Armel and Ramachandran, 2003; Alimardani et al., 2013; Braun et al., 2020; Möller et al., 2020), EDA was recorded during the syringe applications to implicitly assess the participants' level of embodiment over the virtual hand. Following the previous studies, we expected that in the case of a successful integration of the virtual hand into one's own body scheme, a fear-evoking stimulus such as a syringe would trigger a physiologically-measurable fear response.
To record the participants' EDA, two skin conductance sensors were attached to the middle and ring finger of the participants' left hand and recorded using the NEXUS-10 device (Mind Media BV, Herten, Netherlands), which was placed near the left hand on top of the table. The device itself was interfaced with the computer via Bluetooth, and digitised the EDA data with a sampling frequency of 256 Hz, using a self-adapted SDK provided by the manufacturer that allows to record psychophysiological data via LabStreamingLayer (LSL; https://github.com/sccn/labstreamingplayer; accessed 07.01.21). To prevent the recording of artefacts, participants were instructed to rest their left hand on the table throughout the experiment.
For the offline analysis, the recorded EDA data were first imported by means of EEGLAB 14_1_2b (Delorme and Makeig, 2004) into Matlab R2019a (Mathworks, Natick, MA, USA) and then analysed by LEDALAB v3.4.9. This Matlab toolbox enables a continuous decomposition analysis (CDA) (Benedek and Kaernbach, 2010a,b) to be performed, by which the EDA signal becomes deconvolved into its tonic and phasic parts. The phasic EDA part was retained for the −3 to +12 s time interval, relative to the onset of the syringe application. Next, these segments were baseline-corrected by calculating the percent amplitude change, relative to the average EDA of the −3 to −1 s time interval immediately preceding the syringe application. For the statistical analysis, the peak between +4 and +11 s relative to the syringe application was separately extracted from these EDA segments for each condition. Due to data corruption, three EDA data files had to be excluded from the statistical analysis.
Statistical Analyses
There were four main experimental variables (perceived SoO, perceived SoA, perceived micro-/macrosomatognosia, phasic EDA) and three control variables (SoO control scores, SoA control scores and perceived micro-/macropsia) for the first experimental block. Using the Anderson-Darling test, all variables were checked for normality before any further statistical data analyses. Since every variable showed at least one non-normal distribution in at least one condition, all main experimental variables were analysed by a one-way Friedman-test with the factor hand size. Significant effects were followed up by pairwise Bonferroni-Holm corrected Wilcoxon signed-rank tests between the baseline condition and every other condition. Moreover, to validate that our experimental manipulations only affected SoO, SoA and micro- or macrosomatognosia, and not induced some general suggestibility effect, pairwise comparisons between each phenomenal target property and each belonging control values were separately carried out for each condition.
For the second experimental block, we logged the maximally reached change in virtual hand volume (in %) relative to the baseline hand volume that was measured immediately before the participants indicated the VHI to be broken down. Next, we conducted a paired t-test between the maximally reached volume changes in the growing condition and decreasing condition. This was done in order to investigate whether there was a higher body size plasticity for macrosomatognosia than microsomatognosia, as indicated by Pavani and Zampini's study (2007).
Furthermore, to explore the relationship between our operationalized phenomenal target properties, Pearson correlation coefficients were calculated for the first experimental block between perceived SoO, SoA, micro-/macrosomatognosia and micro-/macropsia. P-values were Bonferroni-Holm corrected.
All statistical analyses were carried out using MATLAB2019a (Mathworks, Natick, MA, USA), IBM SPSS Statistics 25.0 and the Multiple Testing Toolbox (https://www.mathworks.com/matlabcentral/fileexchange/70604-multiple-testing-toolbox, retrieved 07.01.2021).
Results
Sense of Ownership and Illusion Breakdowns
Questionnaire data for SoO are depicted in Figure 4A. SoO above the a priori defined illusion criterion (+1) was reported in 62.86% of the participants for the baseline-condition (Mdn = 1.500, M = 1.343, SD = 1.288), 34.29% for the −50% condition (Mdn = 0.000, M = −0.185, SD = 1.715), 54.29% for the −25%-condition (Mdn = 1.000, M = 0.714, SD = 1.703), 57.14% for the +25% condition (Mdn = 1.000, M = 0.671, SD = 1.553) and 34.29% for the +50% condition (Mdn = 0.000, M = 0.029, SD = 1.761). Planned Bonferroni-Holm corrected pairwise Wilcoxon signed-rank test between SoO scores and SoO control scores were significant for all five conditions (see Table 2), confirming the illusion-specificity in our experiment. A Friedman test revealed a significant main effect for factor hand size (chi-square = 35.58, df = 4, p < 0.001), which was followed up by Bonferroni-Holm corrected Wilcoxon signed-rank tests between the baseline condition and all other conditions. These tests revealed stronger SoO for the baseline condition as compared to each other condition (baseline vs. −50% condition: Z = −4.342, p = 0.001; baseline vs. −25% condition: Z = −2.658, p = 0.008; baseline vs. +25% condition: Z = −2.680, p = 0.015; baseline vs. +50% condition: Z = −3.965, p = 0.001).
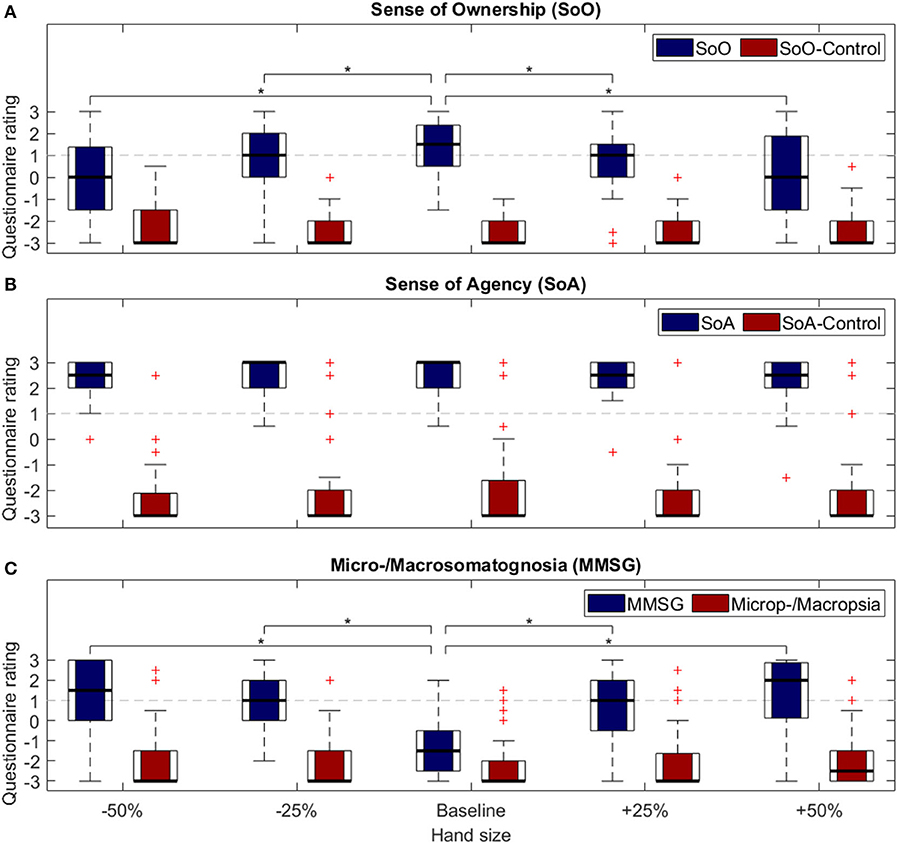
Figure 4. Questionnaire ratings. Questionnaire results for block 1. Control questions were asked for (A) Sense of Ownership and (B) Sense of Agency. Questions related to Micro-/Macropsia served as control items for (C) Micro-/Macrosomatognosia. *p < 0.05.
As regards the second experimental block, participants indicated the virtual hand still to be part of their own body up to a +19.05% (SD = 0.116) volume increase in the growing condition and up to a 19.32% (SD = 0.101) volume decrease in the shrinking condition. A t-test comparing the volume values of the growing condition and shrinking condition, revealed no significant condition differences [t(68) = 0.102; p < 0.918].
Sense of Agency
SoA questionnaire data are presented in Figure 4B. For all conditions, the a priori defined illusion criterion (+1) was reached. More specifically, 91.43% of the participants met the criterion for the baseline-condition (Mdn = 3.000, M = 2.500, SD = 0.767), 94.29% for the −50% condition (Mdn = 2.500, M = 2.414, SD = 0.691), 97.14% for the −25%-condition (Mdn = 3.000, M = 2.543, SD = 0.623), 97.14% for the +25% condition (Mdn = 2.500, M = 2.414, SD = 0.733) and 97.14% for the +50% condition (Mdn = 2.500, M = 2.229, SD = 0.942). Planned Bonferroni-Holm corrected pairwise Wilcoxon signed-rank test revealed significantly stronger effects for the experimental SoA variables than their control counterparts for every combination (see Table 2), confirming the illusion-specificity of SoA in our experiment. A Friedman test revealed a significant main effect for factor hand size (chi-square = 12.15, df = 4, p < 0.016, n = 35), which was followed up by Bonferroni-Holm corrected, pairwise Wilcoxon signed-rank tests between the baseline condition and every other condition. None of these comparisons was, however, significant (baseline vs. −50% condition: Z = −1.255, p = 0.629 baseline vs. −25% condition: Z = 0.263, p = 0.793; baseline vs. +25% condition: Z = −0.649, p = 0.999; baseline vs. +50% condition: Z = −2.211, p = 0.108).
Micro- and Macrosomatognosia
Reported experiences of micro- and macrosomatognosia are depicted in Figure 4C. As expected, micro- and macrosomatognostic experiences increased as a function of our rescaling factor. That is, the highest micro-/macrosomatognostic values were reported for the −50% condition (Mdn = 1.500, M = 1.286, SD = 1.729) and +50% condition (Mdn = 2.000, M = 1.443, SD = 1.697), while for the baseline condition, any micro- or macrosomatognostic experiences were clearly denied (Mdn = −1.500, M = −1.171, SD = 1.440). For the −25% condition and +25% condition, some micro- or macrosomatognostic experiences were reported (−25% condition: Mdn = 1.000, M = 0.9714, SD = 1.323; +25% condition: Mdn = 1.000, M = 0.857, SD = 1.556) that, were, however, descriptively lower than for the −50% condition and +50% condition. Planned Bonferroni-Holm corrected pairwise Wilcoxon signed-rank test between the micro-/macrosomatognostic values and micro-/macropsic control values were significant for all conditions, except for the baseline condition. Hence, the body size misperceptions induced in our participants were not just due to some generally induced, non-body related size misperception. A Friedman test on the factor hand size was significant (chi-square = 55.34, df = 4, p = 0.001, n = 35) and followed up by the planned Bonferroni-Holm corrected pairwise Wilcoxon signed-rank tests. These tests revealed a significant effect for the baseline vs. −25% comparison (Z = 4.933, p < 0.001), the baseline vs. −50% comparison (Z = 4.881, p < 0.001), the baseline vs. +25% comparison (Z = 4.639, p < 0.001) and the baseline vs. +50% comparison (Z = 4.871, p < 0.001).
Electrodermal Results
Phasic EDA responses relative to the virtual syringe injections are illustrated in Figure 5. As can be seen, a phasic EDA increase shortly after the injection of the syringe was present in all five conditions. The ensuing Friedman test, however, revealed no significant effect for hand size (chi-square = 4.28, df = 4, p = 0.370, n = 32).
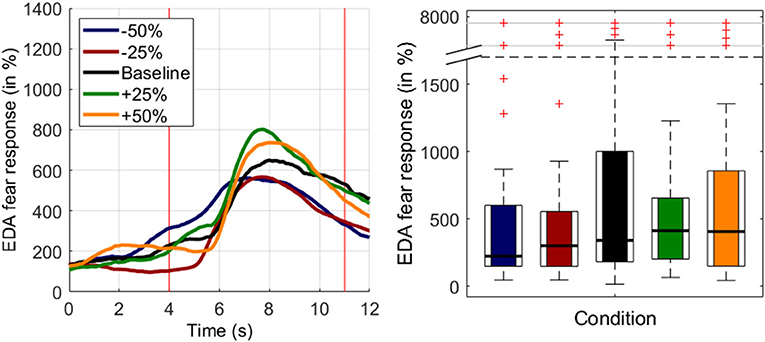
Figure 5. Electrodermal results for experimental block 1. (Left) Baseline-corrected EDA responses (±SEMs) across participants during syringe injections. Time point zero indicates the beginning of the syringe application. (Right) Mean phasic EDA responses for the extracted time interval between 4 and 11 s.
Correlation Analysis
The results of the correlation analysis are depicted in Table 3. Significant correlations were found across conditions as well as within conditions. While across conditions, a significant correlation was found between SoO and SoA (r = 0.318, p = 0.001) as well as between micro-/macrosomatognosia and micro-/macropsia (r = 0.255, p = 0.023), for the −50% condition, a significant correlation was found between micro-/macrosomatognosia and micro-/macropsia (r = 0.540, p = 0.028). Moreover, descriptively, we also found moderately high correlations for many of the remaining variable pairs. For these correlations, however, the p-values did not remain significant after Bonferroni-Holm adjustment.
Discussion
The aims of the present study were to investigate if participants can experience SoO and SoA over a virtual hand that significantly deviates in size from their own hand and if by such size-deviant VHI induction, a change in their own hand size perception can also be induced.
Regarding SoO, we find that it was strongest for the baseline condition, while for the other experimental conditions, it steadily decreased in either direction according to the scaling factors. That is, the more strongly the presented virtual hand deviated in size from the participant's real hand, the stronger also was the decrease in SoO over the virtual hand. While this result is in line with Wittkopf et al. (2017) finding of lower SoO toward size-skewed limb mirror reflections than non-skewed limb mirror reflections, it contradicts the RHI results of Pavani and Zampini (2007). There, the visuotactile stimulation of a rubber hand yielded stronger SoO toward veridical- and enlarged rubber hands than toward shrunken rubber hands. Moreover, the present SoO result also contradicts Lin et al.'s (2019) VHI study in which the reported SoO levels remained unaffected by virtual hand size manipulations. However, it should be recalled that in this former study, the virtual hands were only rescaled by −25% and +25% and the sampling size (n = 20) was lower than in the current study (n = 35). Given that the illusion was still inducible for over half of our participants in these two conditions and given the fact that it broke down at a rescaling-factor of around 19% in either direction, it might be speculated that statistical power was just too low to find statistical effects between the different hand size conditions in Lin et al.'s study (2019).
Regardless of what is the exact rescaling factor at which SoO collapses, our study clearly indicates that the inducibility of SoO over a virtual hand is subject to spatial constraints. Only if the virtual hand presented is approximately the same size as the participant's real hand can full-blown SoO be established. This spatial constraint falls in line with several other identified top-down constraints that a perceptual object has to fulfil to become embodiable and identified as “mine” (Braun et al., 2018). A RHI is typically not inducible, for example, if the artificial hand is placed in an anatomically-implausible position (Ehrsson et al., 2005a; Kalckert and Ehrsson, 2012; Braun et al., 2014; Möller et al., 2020), or if a non-hand object is used instead of an artificial hand (Haans et al., 2008; Tsakiris et al., 2010). Following Tsakiris (2010) classification, according to which most SoO theories can be situated in a continuum ranging from bottom–up to top–down accounts, the present results rather support top-down theories of SoO. Whereas bottom–up theories regard multisensory integration as the major enabling mechanism underlying SoO, top–down theories proclaim a strong involvement of pre-existing internal body maps in the SoO constitution (Tsakiris, 2010; Braun et al., 2018). If SoO was completely bottom-up driven, SoO would not decrease toward size-deviant virtual hands, as long as the spatiotemporal correlation between virtual and real hand persisted.
For the EDA analysis, we found phasic EDA increases relative to the virtual syringe applications for most of our participants and in all five conditions. In accordance with the literature (Armel and Ramachandran, 2003; Yuan and Steed, 2010; Braun et al., 2016), we interpret these EDA increases as transient fear responses that occurred due to the threatening of the virtual hand. If this interpretation is right, the present EDA finding indicates that at least on the functional level, the virtual hand presented became successfully embodied into the participant's own body scheme in most of the cases. Notably, however, we found no differences in the EDA increases between our five conditions. While this null finding is in line with Wittkopf et al.'s (2017) and Lin et al.'s (2019) proprioceptive drift null findings, it was against expectations and contradicts the phenomenological SoO results. One possible reason for this could be that our implicit embodiment test measures a different kind of embodiment than our SoO measure, and that this kind of embodiment was induced to a similar extent in all five conditions. Another reason, however, could be that we lacked enough statistical power to detect such a condition difference, given the following two circumstances: First, presumably due to habituation, transient EDA increases are known to decline with the number of stimulus repetitions (Dawson et al., 2016) – a fact that might be of relevance for the present study, given that here the syringe application was repeated five times (albeit from different vision angles). Second, it is known that around 5% (Dawson et al., 2016) to 25% (Venables and Mitchell, 1996) of healthy participants do not produce transient EDA increases.
Pertaining to SoA, a vivid SoA experience over the virtual hand's movement behaviour could be induced in most of our participants. Notably, in line with Lin et al. (2019), our statistical analysis revealed no SoA condition differences. That is, regardless of the virtual hand's size, the participants experienced a similarly strong feeling of authorship over the virtual hand's movements. In some sense, this finding contradicts previous RHI studies (Kalckert and Ehrsson, 2012; Braun et al., 2014, 2016) which reported increased SoA under SoO-enabling conditions. The experimental manipulations in these studies were, however, different, as artificial hand embodiment was either completely enabled or completely shut off, by either positioning the artificial hand in, respectively, an anatomically plausible or anatomically implausible position. Thus, it might be speculated that the residual SoO that participants felt toward the rescaled virtual hands in the present study might have modulated SoA as well. Furthermore, experimental SoA investigations in RHI-settings underlie rather restrictive constraints, given that typically only single fingers of the rubber hand can be moved through a mechanical connexion to the participants' real hands. As argued by Ma and Hommel (2015) this may lead to an underestimation of the influences of bottom-up factors in the development of SoA, given that less freedom of movement leads to a sparser database of sensory information to base one's SoA judgements on. When comparing the current study to a similar experimental setup, our results replicate Wittkopf et al. (2017) null findings of high and similar SoA ratings for rescaled, virtual hands. Notably, SoA has also been found to be similarly high for abstract, iconic and realistic representations of virtual hands (Argelaguet et al., 2016). Taken together, these findings might indicate that SoA is rather modulated through the capabilities to control (virtual) effectors efficiently than through their actual appearance.
Regarding hand size perception, we found that micro- and macrosomatognosia parametrically increased according to the hand scaling factor in either direction. That is, participants perceived their own hand to have altered in size, when the virtually-presented hand was rescaled to be either smaller or larger, and a greater rescaling-factor induced a more pronounced aberrant hand size perception. This finding replicates other studies (Normand et al., 2011; Piryankova et al., 2014; Preston and Ehrsson, 2014; Serino et al., 2016) in that size perception of one's own body can be altered through means of multisensory stimulation in a VR-illusion.
Notably, in the present study, the induced experiences of micro- and macrosomatognosia do not seem to be caused by a distorted size perception of objects in general: Although a weak correlation (r = 0.255) existed between micro-/macrosomatognostic and micro-/macropsia ratings across conditions, strong aversive ratings for micro- and macropsia were given for each condition. That is, our participants strongly disagreed that the virtual room and its furniture changed in size throughout the experiment. The strong negation of micro- and macropsia contrasts with former studies (van der Hoort et al., 2011; Linkenauger et al., 2013; Ogawa et al., 2018), which found that the perception of spatial dimensions of a virtual environment can be altered through embodying a rescaled virtual body or hand. Thus, it could have also been expected for our study that the virtual hand might be used as an approximate reference to determine the size of the virtual environment, leading to a perception of objects to be smaller when being presented with a larger virtual hand, or larger when being presented with a smaller virtual hand. As per previous studies, such body-based scaling effects appear to be strongly linked to SoO over a virtual body, as the effect was significantly stronger for SoO-enabling conditions (van der Hoort et al., 2011) and specific to the rescaling of participants' “own” virtual hand rather than rescaling of any body part in the virtual environment (Linkenauger et al., 2013). Therefore, the lack of such a body-based scaling effect might potentially be explained by the attenuated feelings of embodiment over the rescaled, virtual hand.
Summarising the SoO and micro-/macrosomatognosia results, SoO parametrically decreased according to the hand scaling factor, while micro- or macrosomatognosia parametrically increased according to the hand-scaling factor. Hence, it appears that the induction of micro- or macrosomatognosia over a limb does not necessarily require the co-existence of full-blown SoO over that limb. Instead, our results suggest that micro- or macrosomatognosia over a virtual limb also arises in experimental situations (−50%, +50%) where full-blown SoO cannot (yet) be established. This suggests that, besides SoO, further forms of embodiment exist and that perceptual objects may be embodied into one's own body schema (and thereby alter it), regardless of whether SoO is also established toward them. This interpretation of the present results concurs with several previous studies that indicate that tool use (which typically does not induce “tool-ownership”) may modulate body size perception (for a review, see Schettler et al., 2019).
Regarding our correlation analysis, we found a weak positive correlation (r = 0.318) between SoO and SoA across conditions. This finding is in line with previous studies (Kalckert and Ehrsson, 2012; Braun et al., 2014; Möller et al., 2020; Spychala et al., 2020) and suggests that both subjective measures do not assess completely distinct, but rather overlapping aspects of self- experience, or that SoO and SoA promote each other (Braun et al., 2018).
Limitations and Future Directions
A limitation of the present study is that due to technical reasons, the size of the virtual hand model was not precisely matched with the participants' real hand size, but instead an average human hand was presented in the baseline condition. One reason for this was that we were not aware of a simple solution for exactly matching the virtual hand size and shape in the baseline condition with each participant's actual hand size and shape. Consequently, participants were presented with a virtual hand that, to a small extent, was already smaller or larger than their actual hand in the baseline condition. This difference in hand size was subsequently propagated to the other conditions as well and thus might have biased results or reduced statistical power. That this suboptimal implementation resulted in a systematic measurement error, appears, however, unlikely, given that, across subjects, the size deviations caused between the virtual and participant's real hand likely occurred in both directions. Hence, on the group level, these size deviations likely cancelled each other out. Also, our major finding that SoO parametrically decreased according to the hand scaling factor in either direction, while micro- or macrosomatognosia parametrically increased according to the hand-scaling factor in either direction, does not indicate any obvious systematic bias. Rather, it suggests that the fixed hand size used was actually quite representative for most of our participants.
Similarly, we did not take the handedness of participants into account, as for every participant a virtual right hand was displayed. However, since other studies in RHI-settings found no influence of handedness on the strength of the illusion (Ocklenburg et al., 2011), it seems unlikely that it impacted the present study.
Another potential caveat is that that the dynamic resizing of the virtual hand in block 2 started as soon as the trial began. This is in contrast to experimental block 1, that was preceded by a VHI-induction phase. In other studies as well as in our first experimental block, such a VHI-induction phase ranged from 30 s (e.g., Choi et al., 2016) to 90 s (e.g., Ma and Hommel, 2015). Hence, it might be speculated that participants may not have had enough time to develop a sufficient illusory SoO over the virtual hand from the beginning. This, in turn, could have affected their perception as to the extent they were able to incorporate the resizing, virtual hand into their own body scheme. Our anecdotal observation, however, is that VHI embodiment is much quicker than classical RHI embodiment and that most participants start to experience SoO quite quickly.
To assess the research question as to the hand-scaling factor up to which a VHI can still be induced, we started at baseline and then dynamically resized the virtual hand into either a growing or a shrinking direction. To further investigate this question in the future, it could also be interesting to include trials that start from the opposite direction. That is, that start from a drastically modified hand size and then dynamically rescale the hand up to normal hand size, in order to study the onset of when participants experience SoO and SoA.
Another limitation of the present study is that we operationalised body size misperception without explicitly assessing the direction of perceived body size change (“I felt as if I had a tiny/giant hand”; “It felt as if my own hand was different in size”). Interestingly, however, we found an equally parametrical reduction of SoO in both the growing and the shrinking condition, which indicates that the direction of size-deviancy is not that influential. Still, in future studies, the direction of perceived body size change should be assessed more distinctively.
While the current study suggests that a change in one's body size perception may be induced by visuomotor synchrony, previous studies were also able to induce changes in body size perception by visuotactile synchrony (Keizer et al., 2016) or by a combination of visuotactile and visuomotor synchrony (Normand et al., 2011). Unfortunately, the differences in experimental setups as well as the ambiguity of reported SoO over the resized virtual limbs impede a reasonable conclusion based on the existing literature. Future studies are therefore necessary to disentangle the influences of different sensory modalities involved.
It should be noted that the assessment of perceived hand size was merely based on questionnaire data and as such lacked the potential to also behaviourally demonstrate the participants' perceptual changes as we did not conduct any size measurements before and after the illusion. In retrospect, it would have been interesting to include an estimation task into our VHI study. One potentially interesting option for future study might be to implement a virtual calliper to indicate length and width of perceived hand size, as a similar measurement method has already been used for the estimation of perceptual hand size within a RHI-setting (Keizer et al., 2014). During the size-estimation tasks the virtual hands would not be visible to participants, to ensure their perceptual body image was not confounded by current visual information. Then, a virtual calliper could be displayed, such that the two points of it could be adjusted to the distance, which participants would deem as fitting to their currently perceived hand size. In so doing, pre- and post-measurements of perceptual hand size could reveal if and to what degree the different rescaling-factors of a virtual hand influence one's own hand size perception.
While our study paves the ground for experimentally inducing body size misperceptions, the question remains of how close our experimentally-induced body size misperceptions actually resemble the clinical cases of micro- and macrosomatognosia. To answer this question, one would have to directly compare both forms of body size misperception. A future VHI study could, for instance, be conducted, in which participants that have already experienced clinical manifestations of micro- or macrosomatognosia would be surveyed about the phenomenological similarities or differences between their pathologically-induced and experimentally-induced aberrant body size perceptions. Our suspicion is that the negative affective stance and fear of body size misperception that often accompanies the clinical cases of micro- and macrosomatognosia (Tunç and Başbug, 2017; Palacios-Sánchez et al., 2018) was not induced by our experiment. Instead, our participants were not worried, but rather curious about their altered body experiences.
Conclusion
The current study suggests that an aberrant hand size perception can be induced by means of the VHI. This induced change in hand-size perception occurs regardless of whether participants experience SoO over the rescaled, virtual hand, or not. While further research appears necessary to examine the causal factors influencing body size perception, our study provides a first important step toward an experimental paradigm with which body-size misperceptions can be investigated. Future studies could complement our experimental paradigm with fNIRS or EEG methodologies to gain further knowledge about the neurocognitive underpinnings of body misperceptions.
Data Availability Statement
The datasets and analyses presented in this study can be accessed under the following data repository: https://doi.org/10.17605/OSF.IO/BPCMT.
Ethics Statement
The studies involving human participants were reviewed and approved by the local medical ethics committee of the University of Bonn. The patients/participants provided their written informed consent to participate in this study.
Author Contributions
DA and AB designed the experiment under the supervision of NB. DA collected the data. DA and NB analysed the data. DA, NB, and LA wrote major parts of the manuscript. AP, BS, LA, and JT contributed to, reviewed, and edited the manuscript. All authors contributed to the article and approved the submitted version.
Conflict of Interest
The authors declare that the research was conducted in the absence of any commercial or financial relationships that could be construed as a potential conflict of interest.
Acknowledgments
This research article is a reworked excerpt from a non-published bachelor thesis by DA. We acknowledge the support of Prof. Dr. Reinhard Klein.
Supplementary Material
The Supplementary Material for this article can be found online at: https://www.frontiersin.org/articles/10.3389/frvir.2021.656788/full#supplementary-material
References
Alimardani, M., Nishio, S., and Ishiguro, H. (2013). Humanlike robot hands controlled by brain activity arouse illusion of ownership in operators. Sci. Rep. 3, 1–5. doi: 10.1038/srep02396
Argelaguet, F., Hoyet, L., Trico, M., and Lécuyer, A. (2016). “The role of interaction in virtual embodiment: effects of the virtual hand representation,” in Proceedings - IEEE Virtual Reality, 3–10.
Armel, K. C., and Ramachandran, V. S. (2003). Projecting sensations to external objects: evidence from skin conductance response. Proc. R. Soc. B Biol. Sci. 270, 1499–1506. doi: 10.1098/rspb.2003.2364
Bachmann, D., Weichert, F., and Rinkenauer, G. (2018). Review of three-dimensional human-computer interaction with focus on the leap motion controller. Sensors 18, 1–39. doi: 10.3390/s18072194
Benedek, M., and Kaernbach, C. (2010a). A continuous measure of phasic electrodermal activity. J. Neurosci. Methods 190, 80–91. doi: 10.1016/j.jneumeth.2010.04.028
Benedek, M., and Kaernbach, C. (2010b). Decomposition of skin conductance data by means of nonnegative deconvolution. Psychophysiology 47, 647–658. doi: 10.1111/j.1469-8986.2009.00972.x
Blom, J. D. (2016). Alice in Wonderland syndrome: a systematic review. Neurol. Clin. Pract. 6, 259–270. doi: 10.1212/CPJ.0000000000000251
Botvinick, M., and Cohen, J. (1998). Rubber hands “feel” touch that eyes see. Nature 391:756. doi: 10.1038/35784
Braun, N., Berisha, A., Anders, D., Kannen, K., Lux, S., and Philipsen, A. (2020). Experimental inducibility of supernumerary phantom limbs: a series of virtual reality experiments. Front. Virt. Real. 1, 1–18. doi: 10.3389/frvir.2020.00012
Braun, N., Debener, S., Spychala, N., Bongartz, E., Sörös, P., Müller, H. H. O., et al. (2018). The senses of agency and ownership: a review. Front. Psychol. 9, 1–17. doi: 10.3389/fpsyg.2018.00535
Braun, N., Emkes, R., Thorne, J. D., and Debener, S. (2016). Embodied neurofeedback with an anthropomorphic robotic hand. Sci. Rep. 6, 1–13. doi: 10.1038/srep37696
Braun, N., Thorne, J. D., Hildebrandt, H., and Debener, S. (2014). Interplay of agency and ownership: the intentional binding and rubber hand illusion paradigm combined. PLoS ONE 9:e111967. doi: 10.1371/journal.pone.0111967
Bui, E., Chatagner, A., and Schmitt, L. (2010). Alice in Wonderland syndrome in major depressive disorder. J. Neuropsychiatry Clin. Neurosci. 22:352.e16. doi: 10.1176/jnp.2010.22.3.352.e16
Choi, W., Li, L., Satoh, S., and Hachimura, K. (2016). Multisensory integration in the virtual hand illusion with active movement. BioMed Res. Int. 2016, 1–9. doi: 10.1155/2016/9195219
Dawson, M. E., Schell, A. M., and Filion, D. L. (2016). “The electrodermal system,” in Handbook of Psychophysiology, eds J. T. Cacioppo, L. G. Tassinary, and G. G. Berntson (Cambridge: Cambridge University Press), 217–243.
Delorme, A., and Makeig, S. (2004). EEGLAB: An open source toolbox for analysis of single-trial EEG dynamics including independent component analysis. J. Neurosci. Methods 134, 9–21. doi: 10.1016/j.jneumeth.2003.10.009
Dieguez, S., and Lopez, C. (2017). The bodily self: Insights from clinical and experimental research. Ann. Phys. Rehabil. Med. 60, 198–207. doi: 10.1016/j.rehab.2016.04.007
Ehrsson, H. H., Holmes, N. P., and Passingham, R. E. (2005a). Touching a rubber hand: feeling of body ownership is associated with activity in multisensory brain areas. J. Neurosci. 25, 10564–10573. doi: 10.1523/JNEUROSCI.0800-05.2005
Ehrsson, H. H., Kito, T., Sadato, N., Passingham, R. E., and Naito, E. (2005b). Neural substrate of body size: illusory feeling of shrinking of the waist. PLoS Biol. 3:e412. doi: 10.1371/journal.pbio.0030412
Haans, A., IJsselsteijn, W. A., and de Kort, Y. A. W. (2008). The effect of similarities in skin texture and hand shape on perceived ownership of a fake limb. Body Image 5, 389–394. doi: 10.1016/j.bodyim.2008.04.003
Haggard, P., and Jundi, S. (2009). Rubber hand illusions and size-weight illusions: self-representation modulates representation of external objects. Perception 38, 1796–1803. doi: 10.1068/p6399
Holle, H., McLatchie, N., Maurer, S., and Ward, J. (2011). Proprioceptive drift without illusions of ownership for rotated hands in the “rubber hand illusion” paradigm. Cogn. Neurosci. 2, 171–178. doi: 10.1080/17588928.2011.603828
Kalckert, A., and Ehrsson, H. H. (2012). Moving a rubber hand that feels like your own: a dissociation of ownership and agency. Front. Hum. Neurosci. 6, 1–14. doi: 10.3389/fnhum.2012.00040
Keizer, A., Smeets, M. A. M., Postma, A., van Elburg, A., and Dijkerman, H. C. (2014). Does the experience of ownership over a rubber hand change body size perception in anorexia nervosa patients? Neuropsychologia 62, 26–37. doi: 10.1016/j.neuropsychologia.2014.07.003
Keizer, A., Van Elburg, A., Helms, R., and Dijkerman, H. C. (2016). A virtual reality full body illusion improves body image disturbance in anorexia nervosa. PLoS ONE 11:e0163921. doi: 10.1371/journal.pone.0163921
Kew, J., Wright, A., and Halligan, P. W. (1998). Somesthetic aura: the experience of “Alice in Wonderland.” Lancet 351:1934. doi: 10.1016/S0140-6736(05)78619-0
Kilteni, K., Maselli, A., Kording, K. P., and Slater, M. (2015). Over my fake body: body ownership illusions for studying the multisensory basis of own-body perception. Front. Hum. Neurosci. 9, 1–20. doi: 10.3389/fnhum.2015.00141
Kim, H. K., Park, J., Choi, Y., and Choe, M. (2018). Virtual reality sickness questionnaire (VRSQ): motion sickness measurement index in a virtual reality environment. Appl. Ergonom. 69, 66–73. doi: 10.1016/j.apergo.2017.12.016
Lin, L., Normovle, A., Adkins, A., Sun, Y., Robb, A., Ye, Y., et al. (2019). “The effect of hand size and interaction modality on the virtual hand illusion,” in IEEE Conference on Virtual Reality and 3D User Interfaces (VR), 510–518. doi: 10.1109/VR.2019.8797787
Linkenauger, S. A., Leyrer, M., Bülthoff, H. H., and Mohler, B. J. (2013). Welcome to Wonderland: the influence of the size and shape of a virtual hand on the perceived size and shape of virtual objects. PLoS ONE 8:e68594. doi: 10.1371/journal.pone.0068594
Ma, K., and Hommel, B. (2015). The role of agency for perceived ownership in the virtual hand illusion. Conscious. Cogn. 36, 277–288. doi: 10.1016/j.concog.2015.07.008
Möller, T. J., Braun, N., Thöne, A.-K., Herrmann, C. S., and Philipsen, A. (2020). The senses of agency and ownership in patients with borderline personality disorder. Front. Psychiatry 11, 1–13. doi: 10.3389/fpsyt.2020.00474
Normand, J. M., Giannopoulos, E., Spanlang, B., and Slater, M. (2011). Multisensory stimulation can induce an illusion of larger belly size in immersive virtual reality. PLoS ONE 6:e16128. doi: 10.1371/journal.pone.0016128
Ocklenburg, S., Rüther, N., Peterburs, J., Pinnow, M., and Güntürkün, O. (2011). Laterality in the rubber hand illusion. Laterality 16, 174–187. doi: 10.1080/13576500903483515
Ogawa, N., Narumi, T., and Hirose, M. (2018). “Object Size Perception in Immersive Virtual Reality: Avatar Realism Affects the Way We Perceive,” in 25th IEEE Conference on Virtual Reality and 3D User Interfaces, VR 2018 - Proceedings, 647–648.
Palacios-Sánchez, L., Botero-Meneses, J. S., Mora-Muñoz, L., Guerrero-Naranjo, A., Moreno-Matson, M. C., Pachón, N., et al. (2018). Alice in Wonderland syndrome (AIWS). A reflection. Colomb. J. Anesthesiol. 46, 143–147. doi: 10.1097/CJ9.0000000000000026
Paqueron, X., Leguen, M., Rosenthal, D., Coriat, P., Willer, J. C., and Danziger, N. (2003). The phenomenology of body image distortions induced by regional anaesthesia. Brain 126, 702–712. doi: 10.1093/brain/awg063
Pavani, F., and Zampini, M. (2007). The role of hand size in the fake-hand illusion paradigm. Perception 36, 1547–1554. doi: 10.1068/p5853
Piryankova, I. V., Wong, H. Y., Linkenauger, S. A., Stinson, C., Longo, M. R., Bülthoff, H. H., et al. (2014). Owning an overweight or underweight body: distinguishing the physical, experienced and virtual body. PLoS ONE 9:e103428. doi: 10.1371/journal.pone.0103428
Podoll, K., Mühlbauer, V., Houben, I., and Ebel, H. (1998). Hypnagogic hallucinations presenting as macrosomatognosia and microsomatognosia. Fortschritte Der Neurologie Psychiatrie 66, 338–344. doi: 10.1055/s-2007-995271
Preston, C., and Ehrsson, H. H. (2014). Illusory changes in body size modulate body satisfaction in a way that is related to non-clinical eating disorder psychopathology. PLoS ONE 9:e85773. doi: 10.1371/journal.pone.0085773
Ramachandran, V. S., and Altschuler, E. L. (2009). The use of visual feedback, in particular mirror visual feedback, in restoring brain function. Brain 132, 1693–1710. doi: 10.1093/brain/awp135
Rode, G., Vallar, G., Revol, P., Tilikete, C., Jacquin-Courtois, S., Rossetti, Y., et al. (2012). Facial macrosomatognosia and pain in a case of Wallenberg's syndrome: selective effects of vestibular and transcutaneous stimulations. Neuropsychologia 50, 245–253. doi: 10.1016/j.neuropsychologia.2011.11.018
Rohde, M., Di Luca, M., and Ernst, M. O. (2011). The rubber hand illusion: feeling of ownership and proprioceptive drift do not go hand in hand. PLoS ONE 6:e21659. doi: 10.1371/journal.pone.0021659
Sanchez-Vives, M. V., Spanlang, B., Frisoli, A., Bergamasco, M., and Slater, M. (2010). Virtual hand illusion induced by visuomotor correlations. PLoS ONE 5:e10381. doi: 10.1371/journal.pone.0010381
Schettler, A., Raja, V., and Anderson, M. L. (2019). The embodiment of objects: review, analysis, and future directions. Front. Neurosci. 13:1332. doi: 10.3389/fnins.2019.01332
Serino, S., Pedroli, E., Keizer, A., Triberti, S., Dakanalis, A., Pallavicini, F., et al. (2016). Virtual reality body swapping: a tool for modifying the allocentric memory of the body. Cyberpsychol. Behav. Soc. Network. 19, 127–133. doi: 10.1089/cyber.2015.0229
Slater, M., Perez-Marcos, D., Ehrsson, H. H., and Sanchez-Vives, M. V. (2008). Towards a digital body: the virtual arm illusion. Front. Hum. Neurosci. 2:6. doi: 10.3389/neuro.09.006.2008
Slater, M., Perez-Marcos, D., Ehrsson, H. H., and Sanchez-Vives, M. V. (2009). Inducing illusory ownership of a virtual body. Front. Neurosci. 3, 214–220. doi: 10.3389/neuro.01.029.2009
Spychala, N., Debener, S., Bongartz, E., Müller, H. H. O., Thorne, J. D., Philipsen, A., et al. (2020). Exploring self-paced embodiable neurofeedback for post-stroke motor rehabilitation. Front. Hum. Neurosc. 13:461. doi: 10.3389/fnhum.2019.00461
Tsakiris, M. (2010). My body in the brain: a neurocognitive model of body-ownership. Neuropsychologia 48, 703–712. doi: 10.1016/j.neuropsychologia.2009.09.034
Tsakiris, M., Carpenter, L., James, D., and Fotopoulou, A. (2010). Hands only illusion: multisensory integration elicits sense of ownership for body parts but not for non-corporeal objects. Exp. Brain Res. 204, 343–352. doi: 10.1007/s00221-009-2039-3
Tunç, S., and Başbug, H. S. (2017). Alice in wonderland syndrome: a strange visual perceptual disturbance. Psychiatry Clin. Psychopharmacol. 27, 412–415. doi: 10.1080/24750573.2017.1354655
van der Hoort, B., Guterstam, A., and Ehrsson, H. H. (2011). Being barbie: the size of one's own body determines the perceived size of the world. PLoS ONE 6:e20195. doi: 10.1371/journal.pone.0020195
Venables, P. H., and Mitchell, D. A. (1996). The effects of age, sex and time of testing on skin conductance activity. Biol. Psychol. 12, 87–101. doi: 10.1016/0301-0511(96)05183-6
Weijers, N. R., Rietveld, A., Meijer, F. J. A., and De Leeuw, F. E. (2013). Macrosomatognosia in frontal lobe infarct - a case report. J. Neurol. 260, 925–926. doi: 10.1007/s00415-012-6827-5
Weissenstein, A., Luchter, E., and Stefan Bittmann, M. A. (2014). Alice in Wonderland syndrome: a rare neurological manifestation with microscopy in a 6-year-old child. J. Pediatr. Neurosci. 9, 303–304. doi: 10.4103/1817-1745.147612
Wittkopf, P. G., Lloyd, D. M., and Johnson, M. I. (2017). Changing the size of a mirror-reflected hand moderates the experience of embodiment but not proprioceptive drift: a repeated measures study on healthy human participants. Exp. Brain Res. 235, 1933–1944. doi: 10.1007/s00221-017-4930-7
Keywords: microsomatognosia, macrosomatognosia, virtual hand illusion, body size perception, EDA, body transfer illusion
Citation: Anders D, Berisha A, Selaskowski B, Asché L, Thorne JD, Philipsen A and Braun N (2021) Experimental Induction of Micro- and Macrosomatognosia: A Virtual Hand Illusion Study. Front. Virtual Real. 2:656788. doi: 10.3389/frvir.2021.656788
Received: 21 January 2021; Accepted: 31 March 2021;
Published: 29 April 2021.
Edited by:
Sook-Lei Liew, University of Southern California, United StatesReviewed by:
Maria Pyasik, University of Verona, ItalyThomas W. Schubert, University of Oslo, Norway
Copyright © 2021 Anders, Berisha, Selaskowski, Asché, Thorne, Philipsen and Braun. This is an open-access article distributed under the terms of the Creative Commons Attribution License (CC BY). The use, distribution or reproduction in other forums is permitted, provided the original author(s) and the copyright owner(s) are credited and that the original publication in this journal is cited, in accordance with accepted academic practice. No use, distribution or reproduction is permitted which does not comply with these terms.
*Correspondence: Niclas Braun, bmljbGFzLmJyYXVuQHVrYm9ubi5kZQ==