- 1Department of Otorhinolaryngology, Head and Neck Surgery, Kuopio University Hospital, Kuopio, Finland
- 2University of Eastern Finland, School of Medicine, Institute of Clinical Medicine, Kuopio, Finland
- 3Microsurgery Training Center, Kuopio University Hospital, Kuopio, Finland
- 4School of Computing, University of Eastern Finland, Kuopio, Finland
- 5Department of Otorhinolaryngology—Head and Neck Surgery, Helsinki University Hospital and University of Helsinki, Helsinki, Finland
- 6Tauno Palva Laboratory, Helsinki University Hospital, Helsinki, Finland
- 7Department of Otorhinolaryngology, Head and Neck Surgery, Oulu University Hospital, Oulu University, PEDEGO, Oulu, Finland
- 8Department of Neurosurgery, Kuopio University Hospital, Kuopio, Finland
Introduction: In recent decades, the lack of educational resources for cadaveric dissections has complicated the hands-on otological surgical training of otorhinolaryngology residents due to the poor availability of cadaver temporal bones, facilities, and limited hours for practice. Since students must gain adequate and patient-safe surgical skills, novel training methods need to be considered. In this proof-of-concept study, a new virtual reality (VR) software is described; this was used during a national temporal bone dissection course where we investigated its feasibility for otological surgical training.
Methods: A total of 11 otorhinolaryngology residents attended the annual 2-day hands-on temporal bone dissection course; they were divided into two groups with similar experience levels. Both groups received a lecture on temporal bone anatomy. A total of 22 cadaver temporal bones were harvested for the course; 11 of these bones were imaged by computed tomography. VR software designed for preoperative planning was then used to create 3D models of the imaged temporal bones. Prior to dissection training, the first group underwent a 30-min VR session, where they identified 24 surgically relevant anatomical landmarks on their individual temporal bone. The second group proceeded directly to dissection training. On the second day, the groups were switched. The feasibility of VR training was assessed with three different metrics: surgical performance evaluation using a modified Hopkins objective structured assessment of technical skill (OSATS), time for the surgical exposure of anatomical landmarks, and the user experience collected with a Likert scale questionnaire.
Results: No differences were noted in the overall performance between the groups. However, participants with prior VR training had a lower mean time for surgical exposure of anatomical landmarks (antrum 22.09 vs. 27.64 min, p = 0.33; incus 60.00 vs. 76.00, p = 0.03; PSCC 71.83 vs. 88.50, p = 0.17) during dissection training. The participants considered VR beneficial for anatomy teaching, surgery planning, and training.
Conclusion: This study demonstrated the feasibility of implementing VR training in a temporal bone dissection course. The VR training demonstrated that even short expert-guided VR sessions are beneficial, and VR training prior to the dissections has a positive effect on the time needed to perform surgical tasks while maintaining comparable performance scores.
Introduction
The human temporal bone is one of the most complex anatomical regions due to its complicated spatial relationship with neurovascular structures and the extensive individual anatomical variety of mastoid air cell pneumatization. Knowledge of key surface landmarks; internal structures such as ossicles, cochlea, internal carotid artery, sigmoid sinus, middle ear, and posterior cranial fossa; and their relationships is crucial for the safe and effective execution of temporal bone surgery. Indeed, the foundation of temporal bone surgery relies on the identification of surface and intrinsic anatomical landmarks. Since these extremely small (e.g., auditory ossicles, 3–8 mm) internal structures are housed within the temporal bone and are not obviously visible, dissection by drilling the bone and its air-filled cells is necessary if one wishes to access the landmarks or reveal the potential pathology. In order for the surgeon to navigate safely during surgery, it is necessary that he/she have precise knowledge of these landmarks and their topographical relationships (Sanna et al., 2018). In temporal bone cadaveric dissection training, the bone is positioned on a fixator, thus simulating the real-life surgical position of the patient, and furthermore, to mimic operating room conditions, the bone is magnified using an operating microscope and the dissection is performed with a motorized high-speed drill with irrigation to locate and expose the anatomical landmarks essential for successful surgery (Dedmon et al., 2017).
Understandably, high-quality surgical training and education are crucial to allow otorhinolaryngology residents to gain the surgical competency that leads to improved patient-safe surgical outcomes. Traditionally, otological surgical training has relied on cadaveric dissections, which have been considered the gold-standard training method (George and De, 2010). In addition, training has been supplemented with lectures, surgical videos, and guided practice during live surgery in the operating theater under the supervision of an experienced otosurgeon. Nonetheless, temporal bone dissection courses are still a mainstay for gaining surgical competency and anatomical knowledge. Generally, these courses combine lectures and hands-on cadaveric dissection training in focused full-day training, spanning several days. In recent decades, however, the opportunities for cadaveric dissections have significantly declined due to the limited access to temporal bones, facilities, and training resources (Mills and Lee, 2003; Frithioff et al., 2018). Senior surgeons are less available for teaching due to long working hours and the increased demands on productivity (Reznick and MacRae, 2006; Ghosh, 2017). Consequently, residents and novice surgeons often struggle with acquiring an in-depth understanding of the complex three-dimensional (3D) anatomy of the temporal bone from two-dimensional (2D) images (e.g., cross-sections of computed tomography) and illustrations. In order to gain a profound mental concept of the anatomy and possess the necessary preoperative planning skills, the surgeon needs to extract the information from 2D images and illustrations of temporal bone anatomy, which requires an extensive amount of rehearsal and experience in preoperative planning, radiological image viewing, and live surgery.
Virtual reality (VR) and its applications hold great promise as ways to overcome the current shortage of resources in surgical education (Herur-Raman et al., 2021; Xu et al., 2021). The reduced costs and advances in virtual reality (VR) technology have opened new possibilities to overcome the restrictions in contemporary otological surgery training and anatomic education. There are now a growing number of studies highlighting that the VR simulators available on various platforms are efficient educational tools in the surgical training of novices (Arora et al., 2012; Fang et al., 2014; Lui and Hoy, 2017; Compton et al., 2020; Li et al., 2021; Sánchez-Margallo et al., 2021).
Current VR technology is able to provide a 3D computer-generated environment that allows interaction with objects in a stereoscopic 3D view from any angle. By wearing a head-mounted display and using hand-held controllers, the multidimensional anatomy of the temporal bone can be examined in a way that otherwise would be very challenging. Prior research on self-guided VR simulators has shown that in novices’ hands, VR training can achieve significantly better performance scores in comparison to traditional methods (e.g., textbooks, temporal bone dissection manuals, and cadaveric dissection) in mastoidectomy training (Zhao et al., 2011a; Andersen et al., 2016). The use of VR in case-specific training and preoperative preparation has evoked the interest of researchers; however, the current literature has remained very limited (Sethia and Wiet, 2015). Recent research has demonstrated that a patient-specific temporal bone rehearsal with VR is feasible and potentially provides valuable insights prior to surgery (Andersen et al., 2021). However, it remains to be shown how beneficial and feasible VR training can be in obtaining microsurgical competency as a part of a temporal bone dissection course.
Here, we describe a proof-of-concept trial that examines the educational value of VR software, designed for preoperative planning on otorhinolaryngology residents’ dissection performance incorporated into a national temporal bone dissection course. The performance scores, surgical time, and user experience in dissection tasks were measured to evaluate the feasibility of exploiting a VR environment as a part of a surgical training course.
Materials and methods
Participants and study design
A total of 22 donated cadaveric temporal bones with normal anatomy were harvested by the Department of Forensic Medicine for the course. Eleven of these temporal bones used in VR training were imaged by high-resolution computed tomography. VR software designed for preoperative planning was then used to create 3D models of the imaged temporal bones.
A total of 11 otorhinolaryngology residents attended the annual 2-day hands-on temporal bone dissection course; they were divided into two groups. On the first day, all participants attended the introductory lecture about otosurgical instruments, fundamental techniques of otosurgery, and temporal bone anatomy. After the lecture, the first group underwent a 30-min individual VR session, where each participant investigated their designated temporal bone. Then, they continued to the dissection session, where each participant dissected the same temporal bone they had examined previously in the VR session. The second group started the dissection session immediately after the introductory lecture. On the second day of the course, with new individual temporal bones, the groups were switched so that the first group started directly with the dissection session and the second group with the VR session. A flowchart presenting the setup of the study is shown in Figure 1.
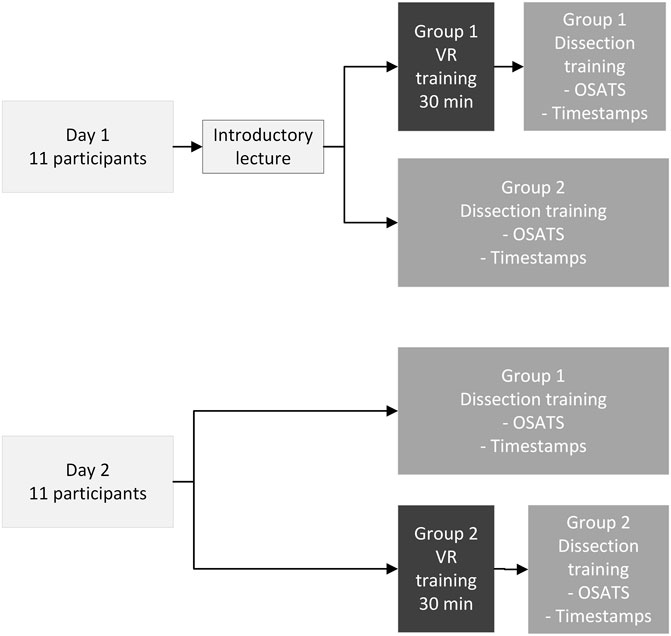
FIGURE 1. Flowchart. Group 1 consisted of five and group 2 six participants, respectively. Every 715 participant had individual temporal bones for each day. The VR training was done first and after 716 that the same temporal bone was used during dissection training. The dissection performances were 717 assessed during and at the end of the dissection with modified OSATS. Surgical time (timestamps) 718 for the exposure of anatomical landmarks was monitored. VR = virtual reality.
We assessed the feasibility of VR training in each resident as a combination of two objective factors and one subjective measurement: 1) surgical performance, 2) surgical time, and 3) subjective user experience (Figure 2). Surgical performance was evaluated by experienced otosurgeons using Hopkins objective structured assessment of technical skill (OSATS) (Laeeq et al., 2009) which was modified to serve the schedule and the extent of dissection trained in the course. The excluded items from the original form were initial bone cuts, open facial recess, posterior atticotomy, and the global section consisting of 10 items that assess processes and preparation. The OSATS scale ranged between 1 (unable to perform) and 5 (performs easily with good flow). The score of 3 represented performs with minimal prompting (Supplementary Material S2).
Surgical time was defined as the time required by every participant for the dissection of the key anatomical landmarks on both days of the course. The time was measured from the beginning of drilling to the exposure of three different landmarks (antrum, incus, and posterior semicircular canal) with a digital clock. Finally, the user experience of the surgical training in VR was assessed using a 5-point Likert scale questionnaire. The section Questionnaires describes scales and metrics in greater detail.
Ethics and permissions
The study fulfilled the Helsinki Declaration for Ethical Use of Human Material and had institutional approval (No. 5551865/125/2019). The anonymous cadaveric temporal bones used in this study were dissected with the permission of the National Supervisory Authority for Welfare and Health (Valvira, no. 6834/06.01.03.01/2013) in the Finnish Institute for Health and Welfare’s Department of Forensic Medicine. The anonymity of participants was guaranteed.
Data collection
Prior to the data collection, experienced otorhinolaryngologists and otosurgeons scanned authentic cadaveric temporal bones and created 3D models specifically for the VR training in the annual course. A 16-slice CT scanner (BrightSpeed, GE Healthcare, Chicago, Illinois, United States) was used for high-resolution computed tomography (HRCT) images (120 kV/100 kV auto mA, speed 5.06, interval 0.310, NI 7, DFOV 85 mm, pitch 0.563:1, and 0.625 mm slice thickness) of the 11 cadaveric temporal bones used in VR training during the course. The imaging process was completed under the supervision of an otosurgeon (VL). The temporal bones used only in dissection training without prior VR training were not imaged with HRCT. The adequacy of the HRCT quality for conventional cross-sectional viewing and VR environment was evaluated with a visual inspection, which included the identification of crucial anatomical landmarks (e.g., facial nerve, ossicles, and cochlea) by four experienced otosurgeons (for HRCT S.S., V.L. and for VR M.I-M., A.D.).
The 3D model from the HRCT data in the VR environment was created with Adesante SurgeryVision™ (Adesante Oy, Turku, Finland) medical software that is based on the Unity3D (Unity Technologies, San Francisco, United States) platform. The acquired image data were imported into the software by two experienced otorhinolaryngologists (TT and MI-M). The 3D models in the VR environment generated by the software were visualized and manipulated by the participating residents with head-mounted displays (HTC Vive Pro and Cosmos, HTC, New Taipei, Taiwan) and pairs of controllers (Figure 3). The controllers allowed participants to freely control magnification, cut through, grab, and rotate the model in the image view at any desired angle. All the VR tasks were performed on computers running Microsoft Windows 10 (Microsoft; Redmond, Washington, United States) with an Intel Core i7-7700HQ CPU @ 2.80 GHz processor (Intel Corporation; Santa Clara, California, United States) and NVIDIA GeForce GTX 1060 graphics card (Nvidia Corporation, Santa Clara, California, United States).
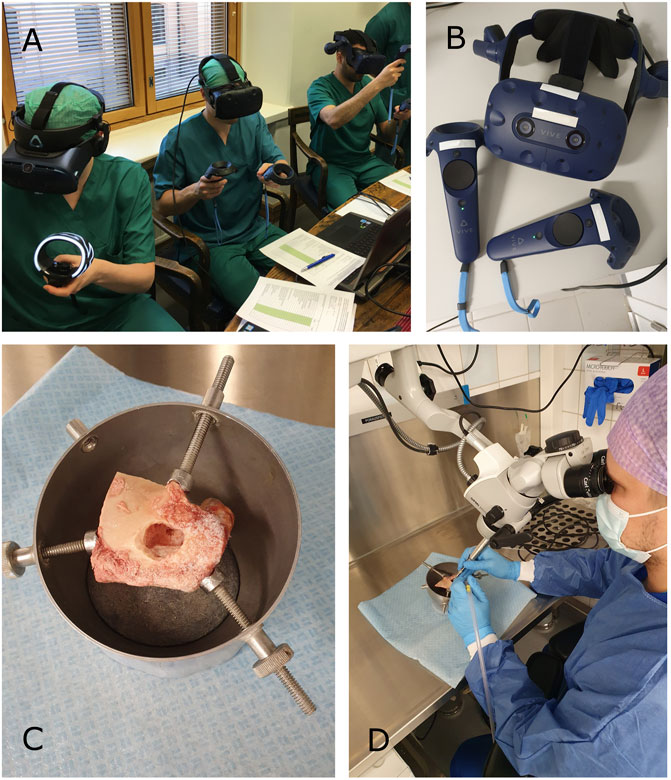
FIGURE 3. (A) Setup of the VR interface during training, (B) Head-mounted display and a pair of 711 controllers, (C) a partially dissected cadaveric temporal bone, (D) setup of the dissection training. 712 (Figure is published with the consent of participants).
Temporal bone dissection course
The data collection was conducted during the annual 2-day temporal bone dissection course organized by the Department of Otorhinolaryngology of Helsinki University Hospital and The Finnish Society of Ear Surgery in Tauno Palva Temporal Bone Laboratory, Helsinki, Finland, in 2020. The course is designed for otorhinolaryngology residents as an introduction to temporal bone surgery and includes lectures, dissection demonstrations, and hands-on dissection training using cadaveric temporal bones. The hands-on dissection session was supervised by experienced otosurgeons. The VR training described in this study was used for the first time during the course’s history.
Virtual reality session
The VR session started with a 10-min demonstration of the VR interface and an introduction to the key anatomical landmarks by a senior otorhinolaryngologist (TT). In order to optimize the training and increase usability and visualization, three presets 1) bone, 2) soft tissue, and 3) a translucent bone were optimized by a senior otosurgeon (MI-M) for the session (Figure 4). The participants were asked to identify 24 surgically relevant anatomical landmarks which had been demonstrated in the introduction to VR from their individual temporal bones to ensure a systematic and structured evaluation of temporal bones in the VR images (Figures 3, 4, and Supplementary Material S1). No colors were used to highlight the landmarks, since application for segmentation was not available. The task performance was supervised, and the number of misidentified landmarks was collected by two senior otolaryngologists (TT and MI-M). The time limit for identification was set to 20 min.
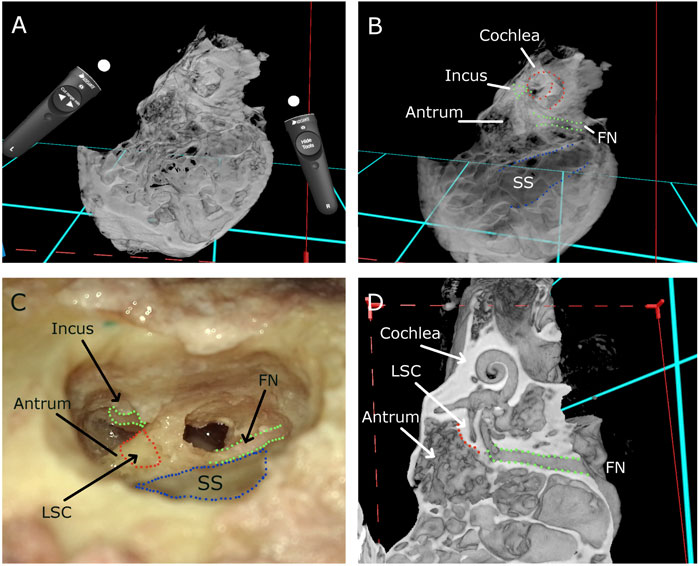
FIGURE 4. (A) Temporal bone and a pair of controllers in VR environment, (B) temporal bone with translucent image windowing, (C) dissected cadaveric temporal bone, and (D) temporal bone and surgical landmarks in VR environment. FN = facial nerve, LSC = lateral semicircular canal, and SS = sigmoid sinus.
Dissection session
During the course, each participant practiced their otosurgical skills (e.g., mastoidectomy) and dissected two cadaveric temporal bones using surgical microscopes and high-speed drills (Figures 3, 4). Senior otosurgeons guided the participants during the dissection but did not perform any dissections on behalf of the participants. On each day, 5 h were reserved for guided cadaveric dissection training.
During and after the dissections, the participant’s surgical performance of mastoidectomy was evaluated by two senior otosurgeons (M.I-M and A.D) with the modified OSATS (Laeeq et al., 2009).
The dissection task was measured from the beginning of drilling to the opening of the mastoid antrum for each participant on both days of the course. On the first day, the task duration included the identification of the corpus of the incus. On the second day with new temporal bones, the task duration could not include the same landmark due to prior training of the dissection of ossicles (incus was removed) during the second day of the course. Instead, on the second day, the task duration was measured up to the identification of the posterior semicircular canal (PSCC).
Questionnaires
The participants’ background and surgical experience were collected prior to the course via a set of questionnaires (Supplementary Material S3). The participants were asked to self-evaluate their understanding of temporal bone anatomy in general and radiologically on a modified Likert scale 1–5 both pre- and post-course, where score 1 means very poor and score 5 means excellent. A score of 3 was considered fair. User experience and feedback with regard to the VR planning and training were collected post-course with a modified 5-point Likert scale questionnaire (Supplementary Material S4) (score 1 means not true/realistic/useful and 5 means very true/realistic/useful; a score of 3 was considered neutral).
Statistics
A linear mixed-effect model was fitted to examine the association between the surgical time of temporal bone dissection with respect to anatomical landmarks and training method (dissection training with or without prior VR training), accounting for repeated measures. The user experience questionnaire and surgical performance results were compared using the Wilcoxon signed-rank test. To reduce bias, Hedges’ g was counted to evaluate the difference in the means of the pooled standard deviation (effect size). Pearson’s r was used in the analysis of the correlations with group demographics. The data were analyzed using IBM SPSS statistics version 27 (IBM SPSS, SPSS Inc., Chicago, IL, United States) after consultation with a statistician. The level of significance was set to p-value < 0.05.
Results
Participant demographics
The demographics and surgical experience of groups are presented in Table 1. Six males and five females, mean age 32.2 (SD 2.6), attended the course and were divided into two groups with similar experience levels [years in residency 2.8 (SD 0.9) vs. 3.1 (SD 0.6)]. The first group consisted of five participants, and the second group comprised six participants. The number of otological operations performed by the main surgeon revealed a statistically significant difference between the groups (p = 0.001) due to one participant’s surgical experience. The analysis of free-text responses revealed that 5 out of 11 participants had a varying level of computer/console gaming experience in recent years and 3 out of 11 reported having hobbies that could support their acquisition of advanced 3D perception (e.g., woodwork and 3D gaming).
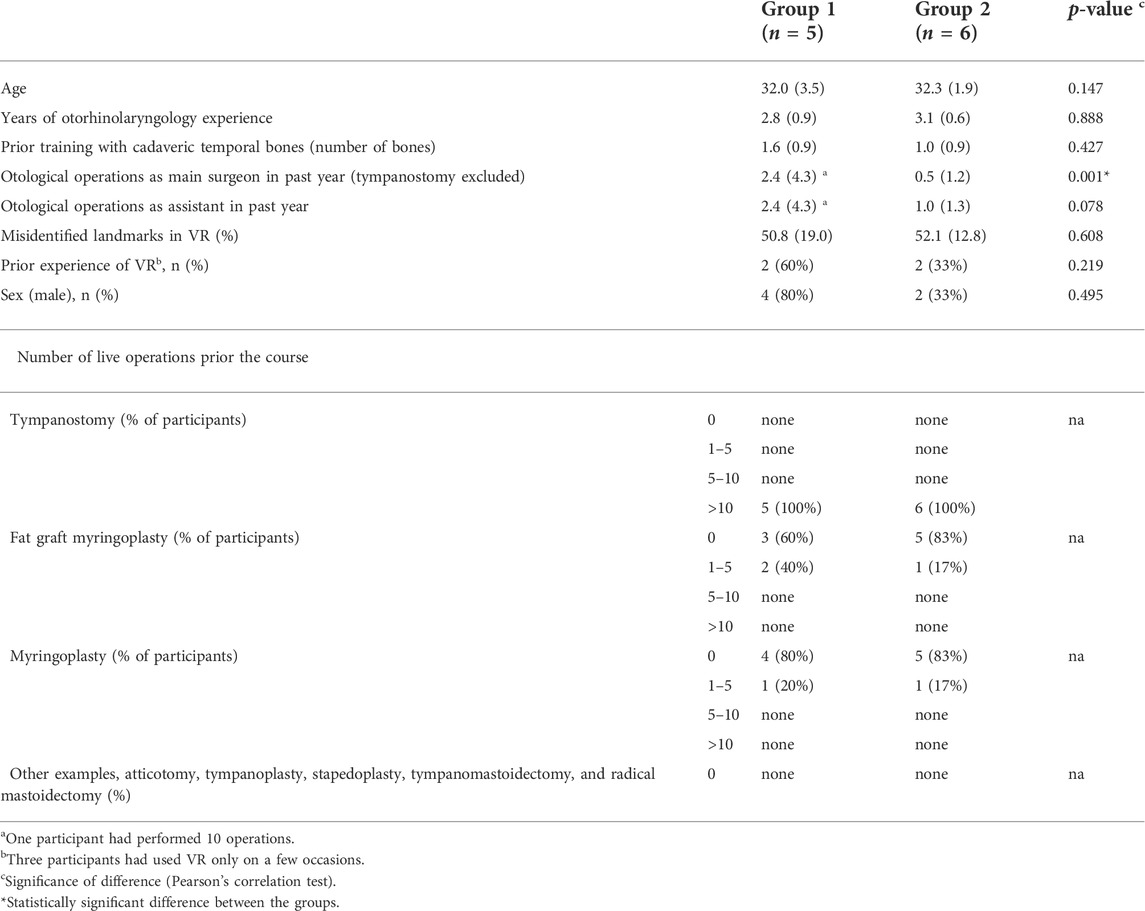
TABLE 1. Participant demographics and surgical experience. The values are mean (SD) unless otherwise stated.
Objective feasibility
The use of VR (group 1 on the first day and group 2 on the second day) did not change the mean OSATS scores (2.95 vs. 2.88, p = 0.80, Hedges’s g = 0.05) with dissection without VR (group 1 for the second day and group 2 for the first day) when both days’ combined results were compared. On the first day, there was no significant difference in dissection performance scores between the groups (group 1 (VR) vs. group 2 (non-VR); 2.56 vs. 2.59, p = 0.60, Hedges’s g = 0.02). The respective scores on the second day were (group 2 (VR) vs. group 1 (non-VR); 3.31 vs. 3.28, p = 0.40, Hedges’s g = 0.03). The OSATS scores significantly increased on the second day in both groups when compared with days 1 and 2 (group 1: 2.56 vs. 3.28, p = 0.002, Hedges’s g = 0.59; group 2: 2.59 vs. 3.31, p = 0.008, Hedges’s g = 0.53). The dissection performance scores (OSATS) are illustrated in Figure 5.
The analysis of task durations demonstrated that the groups with VR training prior to dissection required less time to perform the tasks when compared with those not having access to VR (Table 2). The mean duration of the opening of the antrum in the VR group was 22.09, and in the non-VR group, 27.64 min (p = 0.33, Hedges’s g = 0.42) when both days were summed. On the first day, the incus was opened significantly more rapidly in the VR group as compared to the non-VR group (60.00 vs. 76.00 min, p = 0.03, Hedges’s g = 1.63), and on the second day, the PSCC was identified with a lower mean time in the VR group when compared to the non-VR group (71.83 vs. 88.50 min, p = 0.17, Hedges’s g = 1.02). The feasibility assessment of the VR training prior to dissection had a comparable effect on surgical performance scores with lower mean surgical times.
Subjective feasibility
Participants’ self-evaluated scores of how well they understood the temporal bone’s anatomy in general improved significantly (p = 0.03, Hedges’s g = 1.24) after the course. Table 3 presents the self-evaluation scores for pre- and post-course with respect to understanding the anatomy of the temporal bone.
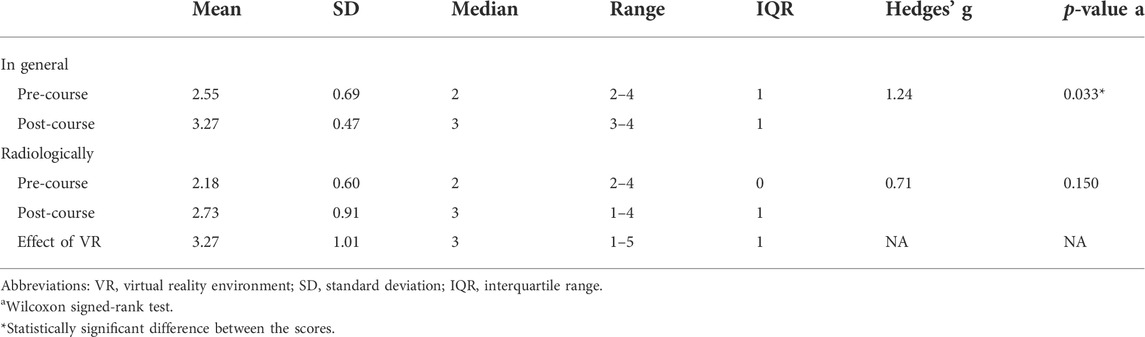
TABLE 3. Scores from the participants self-evaluation of temporal bone anatomy understanding and effect of VR. All values are nominal (SD) on a 5-point Likert scale.
The overall mean score of user experience in VR was 3.70 on the Likert scale of 1 to 5, and all domains were rated above the average. The depth perception (mean = 4.27) and recommendation to colleagues (mean = 4.09) scored the highest. The haptic feedback (mean = 3.09) and learning of surgical planning (mean = 3.09) scored the lowest. Table 4 presents details of the user experience scores for VR.
In the follow-up written debriefing, the participants suggested that more time should be allocated for the VR demonstration and also for the VR training session, as well as more individual guidance for the anatomical landmark identification task. The participants reported that their unfamiliarity with temporal bone anatomy might limit their effective use of VR. Additionally, two participants highlighted the somewhat limited quality of the graphics and images in the VR. Despite these drawbacks, VR training was generally well accepted, and none of the participants reported nausea or vertigo during or after the VR use.
Overall, the participants appreciated the benefits of VR training, such as the possibility to view the 3D topographical anatomy and explore the spatial relationship and distances between the anatomical landmarks. Most importantly, VR training allowed the participants to repeatedly approach and cut through the temporal bone from any angle, a procedure that would not be possible with a physical model. After the course, the participants generally agreed that VR training was a novel and feasible method for teaching anatomy, surgical planning, and surgery training, leading to a better understanding of the 3D arrangement of this complex structure.
Discussion
The aim of this study was to assess the feasibility of VR training with three different metrics during a temporal bone dissection course and examined whether the use of VR training prior to the temporal bone dissection exerted any effect on dissection proficiency. We conducted a full 2-day in situ surgical training using a novel technique for the first time in the course’s history. VR training was adopted by otological surgeons for training residents and applied as a part of a genuine dissection course using actual cadaveric temporal bones. We did not just concentrate on simulating the situation and conditions with a random population. Although the total dissection performance scores between groups were comparable, the provision of VR training prior to the dissection seemed to be associated with a lower mean surgical time on all measured landmarks without compromising the quality of the surgical performance, which is in accordance with previous research (Nash et al., 2012; Lui and Hoy, 2017; Andersen et al., 2018). These findings suggest that preoperative immersive viewing and freedom to cut through and manipulate the temporal bone VR models at many desired angles may have improved the mental representation of temporal bone anatomy in inexperienced trainees, which in turn was reflected in the dissection task performance and especially in the time needed to conduct the surgical tasks. Additionally, surgical time has been utilized previously as an adequate indicator of surgical skills and performance (D’Angelo et al., 2015; Mohamadipanah et al., 2021; Rajpal et al., 2020). Therefore, considering the limited time for the VR session during the course, the positive effect of VR training on the surgical time was rather encouraging.
In clinical work, the radiological imaging of a patient’s temporal bone region is nowadays more and more frequently carried out before any intervention involving the mastoid of the temporal bone (Thukral et al., 2015). Traditionally, radiology images are visualized and examined in 2D cross-sections displayed on 2D monitors. However, trainees and inexperienced surgeons often struggle to gain a profound understanding of the patient’s 3D anatomy from the 2D visualizations (Yammine and Violato, 2015), which is a real obstacle to effective education of temporal bone anatomy. To adequately extract all the information from 2D cross-sectional images of the complex anatomy, the surgeon needs to acquire ample experience in preoperative planning, cross-sectional image viewing, and following live surgery. Ultimately, these consolidate the surgeon’s mental concept of the surgical situation and improve his/her preoperative planning skills. Unfortunately, interpretation of a radiological image or training in preoperative planning is not commonly implemented in trainee-level temporal bone dissection courses. Previous studies have shown that VR planning and simulation may offer a feasible method to enhance the student’s understanding of the anatomy of the temporal bone and provide more effective training for novice surgeons and trainees (Al-Noury, 2012; Locketz et al., 2017; Timonen et al., 2021).
To test the feasibility of VR in combination with hands-on surgical education, we included VR training in the course curriculum for the first time in course history. The initial adoption of VR was not without some limitations. The participants reported that the time scheduled for VR was too short and that they would have needed more guidance to allow them to become better acquainted with the VR system. Several participants experienced issues with the graphics and the resolution quality of the image. The inadequate positioning of the HMD and eyeline (e.g., HMD straps were too loose) or possible eye disorders may have been the basis for these reports although the quality of HRCT image data in our VR setup was evaluated by experienced otosurgeons prior to the course. Despite these shortcomings, the participants positively evaluated VR training and planning during the course, which was in line with previous research (Timonen et al., 2021, 2020).
When examining the specimen-specific anatomy, VR training was very well received by all participants and may have contributed to the residents gaining a better understanding of the displayed anatomy, which eventually propagated into the more proficient dissection. These findings are in line with the previous studies that have reported similar or improved performance when VR training was combined with traditional teaching methods (e.g., lectures) prior to cadaveric dissection (Zhao et al., 2011a; Francis et al., 2012; Andersen et al., 2016). Our results showed that even limited exposure to VR was beneficial in the training of the residents and increased their anatomical knowledge and confidence.
Traditional cadaveric dissections under the supervision of a senior surgeon are indispensable in acquiring profound surgical skills and expertise, and VR simulations and training will be unlikely to replace this traditional form of education. Instead, VR represents a valuable supplemental educational tool to allow trainees to acquire the necessary expertise and speed up the learning curve for understanding the fundamentals of temporal bone surgical anatomy.
Since learning is a process that normally involves repeated experiences, it is debatable whether skills acquired by a single practice session represent genuine learning. Accordingly, distributed training (i.e., training over a longer period of time) as opposed to massed practice has been shown to be more efficient for learning surgical skills (Mackay et al., 2002) and also yielded significantly superior final product results in virtual mastoidectomy (Andersen et al., 2015). Tutoring integrated into the mastoidectomy simulator enables self-directed learning, which further improved the initial learning curve, surgical skill performance, and final product results (Andersen et al., 2018, 2015). However, traditional dissection courses are examples of massed practice, where trainees are typically offered single sessions without the opportunity for repeated practice. Therefore, traditional dissection workshops are suboptimal since they are not very effective for the acquisition and consolidation of the required skills. From this perspective, new approaches such as VR training and VR simulators may represent feasible tools for improving the proficiency and design of dissection courses.
Previous research comparing the efficiency of anatomy education with the application of VR, cadaver skulls, or even anatomical atlases has been conflicting (Chen et al., 2020; Zhao et al., 2020). Some research has demonstrated the same efficiency of anatomy education with different methods, whereas others could demonstrate the benefits of VR. A recent meta-analysis concluded that VR can potentially improve the teaching effectiveness of anatomy compared to traditional or 2D digital methods. Furthermore, VR was regarded as a beneficial complementary training method that could lead to savings in training time and use of resources (Zhao et al., 2020). More research is needed to assess the role of VR training in learning anatomy and surgical planning. In our study setting, VR was a complementary training method prior to the dissections. All participants were also given the normal dissection lectures including temporal bone anatomy. The trainees were given the opportunity to assess the specific temporal bone they were going to dissect, which therefore simulated the clinical procedure more adequately.
In addition, artificial 3D printed temporal bone models in combination with traditional training may be similarly beneficial in training and education (Kashikar et al., 2019; Gadaleta et al., 2020). Created 3D reconstructions can be visualized on a 2D monitor. However, the better immersion and stereoscopicity provided by the VR visualization is superior to 3D reconstructions visualized in 2D monitors. The more natural movements and manipulation of objects in the virtual environment correspond better to surgical reality. In the future, it would be interesting to compare the 3D reconstructions on 2D monitors and VR for surgical training.
Limitations
One limitation of our study was the low number of participants and the short time spent using VR; these were due to time restraints of the course and especially its access to the training facilities. It is acknowledged that the evaluation of the effects of VR on participants’ performance has limitations since the course consisted of other training methods (e.g., lectures, constant guidance in dissections). Second, since all course participants underwent VR training (either on the first or the second day), a control group without the use of VR was missing. This was in line with the main objectives of the course: to introduce residents to otosurgery and to evaluate a new educational method for surgical training. In future work, we will examine the role of VR and contrast it with traditional education in courses teaching dissection techniques. Third, in our study, the time reserved for VR training was brief and did not allow for task repetitions. In contrast, previous studies on VR training have had more time to allow repeated VR sessions and reserved a long time for the VR sessions, ranging from 40 to 120 min (Zhao et al., 2011b; Gawęcki et al., 2020). Despite the lack of time to allow repetitions in VR training, our results highlighted that VR training may have exerted a positive effect on surgical time while maintaining comparable surgical performance and was rated as a positive user experience. Fourth, however, the effect sizes (Hedges’ g) in these data varied to some degree, the majority of surgical time results had medium (>0.5) to large effects (>0.8) (Lakens, 2013), and can be interpreted as a positive result in favor of VR training. In future research, we will focus on evaluating the benefits of VR training with respect to its cost-effectiveness and in particular, examine the relationship between the time spent in VR and proficiency in dissection techniques.
Conclusion
This study represents a proof-of-concept of VR training in a course teaching temporal bone dissection. We demonstrated that even a limited, expert-guided VR training session prior to the temporal bone dissection was feasible and may have contributed a positive effect on the duration of surgical tasks and performance during the dissections. Trainees stated that the examination of their specimens by VR prior to dissection represented a novel and beneficial supplement to the course.
Data availability statement
The datasets used and/or analyzed during the current study are available from the corresponding author on reasonable request.
Ethics statement
Written informed consent was obtained from the individual(s) for the publication of any potentially identifiable images or data included in this article.
Author contributions
TT, MI-M, SS, A-PE, and AD conceptualized and designed the study. TT, MI-M, SS, VL, JL, and AD collected the data. TT analyzed the data. MI-M, PL, HV, SS, and AD contributed to analysis refinement. TT wrote the first draft of the manuscript. All authors substantially contributed to the final data synthesis and in revising the final manuscript. All authors gave final approval to the submitted manuscript and are accountable for all aspects of the work.
Funding
The study was funded by the Academy of Finland (AD Grant No. 333525), State Research Funding of the Kuopio University Hospital (TT Grant No. 5551865, AD Grant No. 5551853), The Finnish ORL-HNS Foundation (TT Grant No. 20210002 and No. 20220027), North Savo Regional Fund (TT Grant No. 65202121, AD Grant No. 65202054), Finnish Cultural Foundation (TT Grant No. 00211098), and The Finnish Society of Ear Surgery.
Acknowledgments
The authors want to thank Tuomas Selander, MSc., for his assistance in the statistical analyses. We are grateful to Ewen MacDonald, Pharm D., for his careful revision of the English language.
Conflict of interest
The authors declare that the research was conducted in the absence of any commercial or financial relationships that could be construed as a potential conflict of interest.
Publisher’s note
All claims expressed in this article are solely those of the authors and do not necessarily represent those of their affiliated organizations, or those of the publisher, the editors, and the reviewers. Any product that may be evaluated in this article, or claim that may be made by its manufacturer, is not guaranteed or endorsed by the publisher.
Supplementary material
The Supplementary Material for this article can be found online at: https://www.frontiersin.org/articles/10.3389/frvir.2022.957230/full#supplementary-material
Abbreviations
3D, three-dimensional; 2D, two-dimensional; HRCT, high-resolution computed tomography; OSATS, objective structured assessment of technical skill; VR, virtual reality; PSCC, posterior semicircular canal.
References
Al-Noury, K. (2012). Virtual reality simulation in ear microsurgery: A pilot study. Indian J. Otolaryngol. Head. Neck Surg. 64, 162–166. doi:10.1007/s12070-011-0290-y
Andersen, S. A. W., Foghsgaard, S., Cayé-Thomasen, P., and Sørensen, M. S. (2018). The effect of a distributed virtual reality simulation training program on dissection mastoidectomy performance. Otol. Neurotol. 39, 1277–1284. doi:10.1097/MAO.0000000000002031
Andersen, S. A. W., Foghsgaard, S., Konge, L., Cayé-Thomasen, P., and Sørensen, M. S. (2016). The effect of self-directed virtual reality simulation on dissection training performance in mastoidectomy. Laryngoscope 126, 1883–1888. doi:10.1002/lary.25710
Andersen, S. A. W., Konge, L., Cayé-Thomasen, P., and Sørensen, M. S. (2015). Learning curves of virtual mastoidectomy in distributed and massed practice. JAMA Otolaryngol. Head. Neck Surg. 141, 1–918. doi:10.1001/jamaoto.2015.1563
Andersen, S. A. W., Varadarajan, V. V., Moberly, A. C., Hittle, B., Powell, K. A., and Wiet, G. J. (2021). Patient‐specific virtual temporal bone simulation based on clinical cone‐beam computed tomography. Laryngoscope 131, 1855–1862. doi:10.1002/lary.29542
Arora, A., Khemani, S., Tolley, N., Singh, A., Budge, J., Varela, D. A. D. V., et al. (2012). Face and content validation of a virtual reality temporal bone simulator. Otolaryngol. Head. Neck Surg. 146, 497–503. doi:10.1177/0194599811427385
Chen, S., Zhu, J., Cheng, C., Pan, Z., Liu, L., Du, J., et al. (2020). Can virtual reality improve traditional anatomy education programmes? A mixed-methods study on the use of a 3D skull model. BMC Med. Educ. 20, 395. doi:10.1186/s12909-020-02255-6
Compton, E. C., Agrawal, S. K., Ladak, H. M., Chan, S., Hoy, M., Nakoneshny, S. C., et al. (2020). Assessment of a virtual reality temporal bone surgical simulator: A national face and content validity study. J Otolaryngol - Head Neck Surg 49, 17. doi:10.1186/s40463-020-00411-y
D'Angelo, A.-L. D., Ray, D. N., Kwan, R. D., Mason, S., Pugh, C., Cohen, E. R., et al. (2015). Idle time: An underdeveloped performance metric for assessing surgical skill. Am. J. Surg. 209, 645–651. doi:10.1016/j.amjsurg.2014.12.013
Dedmon, M. M., O'Connell, B. P., Adams, A. S., Wanna, G. P., and Haynes, D. S. (2017). Improving resident familiarity with the translabyrinthine approach to the internal auditory canal. Am. J. Otolaryngology 38, 526–528. doi:10.1016/j.amjoto.2017.04.005
Fang, T.-Y., Wang, P.-C., Liu, C.-H., Su, M.-C., and Yeh, S.-C. (2014). Evaluation of a haptics-based virtual reality temporal bone simulator for anatomy and surgery training. Comput. Methods Programs Biomed. 113, 674–681. doi:10.1016/j.cmpb.2013.11.005
Francis, H. W., Malik, M. U., Diaz Voss Varela, D. A., Barffour, M. A., Chien, W. W., Carey, J. P., et al. (2012). Technical skills improve after practice on virtual-reality temporal bone simulator. Laryngoscope 122, 1385–1391. doi:10.1002/lary.22378
Frithioff, A., Sørensen, M., and Andersen, S. (2018). European status on temporal bone training: A questionnaire study. Eur. Arch. Otorhinolaryngol. 275, 357–363. doi:10.1007/s00405-017-4824-0
Gadaleta, D. J., Huang, D., Rankin, N., Hsue, V., Sakkal, M., Bovenzi, C., et al. (2020). 3D printed temporal bone as a tool for otologic surgery simulation. Am. J. Otolaryngology 41, 102273. doi:10.1016/j.amjoto.2019.08.004
Gawęcki, W., Węgrzyniak, M., Mickiewicz, P., Gawłowska, M. B., Talar, M., and Wierzbicka, M. (2020). The impact of virtual reality training on the quality of real antromastoidectomy performance. Jcm 9, 3197. doi:10.3390/jcm9103197
George, A. P., and De, R. (2010). Review of temporal bone dissection teaching: How it was, is and will be. J. Laryngol. Otol. 124, 119–125. doi:10.1017/S0022215109991617
Ghosh, S. K. (2017). Cadaveric dissection as an educational tool for anatomical sciences in the 21st century. Am. Assoc. Anatomists 10, 286–299. doi:10.1002/ase.1649
Herur-Raman, A., Almeida, N. D., Greenleaf, W., Williams, D., Karshenas, A., and Sherman, J. H. (2021). Next-generation simulation-integrating extended reality technology into medical education. Front. Virtual Real. 2, 693399. doi:10.3389/frvir.2021.693399
Kashikar, T. S., Kerwin, T. F., Moberly, A. C., and Wiet, G. J. (2019). A review of simulation applications in temporal bone surgery. Laryngoscope Investig. Otolaryngol. 4, 420–424. doi:10.1002/lio2.277
Laeeq, K., Bhatti, N. I., Carey, J. P., Della Santina, C. C., Limb, C. J., Niparko, J. K., et al. (2009). Pilot testing of an assessment tool for competency in mastoidectomy. Laryngoscope 119, 2402–2410. doi:10.1002/lary.20678
Lakens, D. (2013). Calculating and reporting effect sizes to facilitate cumulative science: A practical primer for t-tests and ANOVAs. Front. Psychol. 4, 1–12. doi:10.3389/fpsyg.2013.00863
Li, M., Ganni, S., Albayrak, A., Rutkowski, A. F., van Eijk, D., and Jakimowicz, J. (2021). Proficiency from immersion: A human-centered design in cross-cultural surgical training. Front. Virtual Real. 2, 675334. doi:10.3389/frvir.2021.675334
Locketz, G. D., Lui, J. T., Chan, S., Salisbury, K., Dort, J. C., Youngblood, P., et al. (2017). Anatomy-specific virtual reality simulation in temporal bone dissection: Perceived utility and impact on surgeon confidence. Otolaryngol. Head. Neck Surg. 156, 1142–1149. doi:10.1177/0194599817691474
Lui, J. T., and Hoy, M. Y. (2017). Evaluating the effect of virtual reality temporal bone simulation on mastoidectomy performance: A meta-analysis. Otolaryngol. Head. Neck Surg. 156, 1018–1024. doi:10.1177/0194599817698440
Mackay, S., Morgan, P., Datta, V., Chang, A., and Darzi, A. (2002). Practice distribution in procedural skills training. Surg. Endosc. 16, 957–961. doi:10.1007/s00464-001-9132-4
Mills, R., and Lee, P. (2003). Surgical skills training in middle-ear surgery. J. Laryngol. Otol. 117, 159–163. doi:10.1258/002221503321192412
Mohamadipanah, H., Wise, B., Witt, A., Goll, C., Yang, S., Perumalla, C., et al. (2021). Performance assessment using sensor technology. J. Surg. Oncol. 124, 200–215. doi:10.1002/jso.26519
Nash, R., Sykes, R., Majithia, A., Arora, A., Singh, A., and Khemani, S. (2012). Objective assessment of learning curves for the Voxel-Man TempoSurg temporal bone surgery computer simulator. J. Laryngol. Otol. 126, 663–669. doi:10.1017/S0022215112000734
Rajpal, S., Shah, M., Vivek, N., and Burneikiene, S. (2020). Analyzing the correlation between surgeon experience and patient length of hospital stay. Curēus Palo Alto CA 12, e10099. doi:10.7759/cureus.10099
Reznick, R. K., and MacRae, H. (2006). Teaching surgical skills - changes in the wind. N. Engl. J. Med. 355, 2664–2669. doi:10.1056/NEJMra054785
Sánchez-Margallo, J. A., Plaza de Miguel, C., Fernández Anzules, R. A., and Sánchez-Margallo, F. M. (2021). Application of mixed reality in medical training and surgical planning focused on minimally invasive surgery. Front. Virtual Real. 2, 692641. doi:10.3389/frvir.2021.692641
Sanna, M., Russo, A., and Taibah, A. (2018). The temporal bone: Anatomical dissection and surgical approaches. Incorporated, NEW YORK: Thieme Medical Publishers.
Sethia, R., and Wiet, G. J. (2015). Preoperative preparation for otologic surgery. Curr. Opin. Otolaryngology Head Neck Surg. 23, 355–359. doi:10.1097/MOO.0000000000000181
Thukral, C. L., Singh, A., Singh, S., Sood, A. S., and Singh, K. (2015). Role of high resolution computed tomography in evaluation of pathologies of temporal bone. Jcdr 9, TC07–TC10. doi:10.7860/JCDR/2015/12268.6508
Timonen, T., Dietz, A., Linder, P., Lehtimäki, A., Löppönen, H., Elomaa, A.-P., et al. (2021). The effect of virtual reality on temporal bone anatomy evaluation and performance. Eur. Arch. Otorhinolaryngol. 279, 4303–4312. doi:10.1007/s00405-021-07183-9
Timonen, T., Iso-Mustajärvi, M., Linder, P., Lehtimäki, A., Löppönen, H., Elomaa, A. P., et al. (2020). Virtual reality improves the accuracy of simulated preoperative planning in temporal bones: A feasibility and validation study. Eur. Arch. Otorhinolaryngol. 278, 2795–2806. doi:10.1007/s00405-020-06360-6
Xu, X., Mangina, E., and Campbell, A. G. (2021). HMD-based virtual and augmented reality in medical education: A systematic review. Front. Virtual Real. 2, 692103. doi:10.3389/frvir.2021.692103
Yammine, K., and Violato, C. (2015). A meta-analysis of the educational effectiveness of three-dimensional visualization technologies in teaching anatomy. Am. Assoc. Anatomists 8, 525–538. doi:10.1002/ase.1510
Zhao, J., Xu, X., Jiang, H., and Ding, Y. (2020). The effectiveness of virtual reality-based technology on anatomy teaching: A meta-analysis of randomized controlled studies. BMC Med. Educ. 20, 127. doi:10.1186/s12909-020-1994-z
Zhao, Y. C., Kennedy, G., Yukawa, K., Pyman, B., and O’Leary, S. (2011a). Improving temporal bone dissection using self-directed virtual reality simulation. Otolaryngol. Head. Neck Surg. 144, 357–364. doi:10.1177/0194599810391624
Keywords: virtual reality, surgical training, surgical planning, temporal bone, cadaver, surgical performance
Citation: Timonen T, Iso-Mustajärvi M, Linder P, Vrzakova H, Sinkkonen ST, Luukkainen V, Laitakari J, Elomaa A-P and Dietz A (2022) The feasibility of virtual reality for anatomic training during temporal bone dissection course. Front. Virtual Real. 3:957230. doi: 10.3389/frvir.2022.957230
Received: 30 May 2022; Accepted: 22 August 2022;
Published: 15 September 2022.
Edited by:
Maxime Ros, Université de Montpellier, FranceReviewed by:
Steven Andersen, Head and Neck Surgery & Audiology, DenmarkPierre Boulanger, University of Alberta, Canada
Copyright © 2022 Timonen, Iso-Mustajärvi, Linder, Vrzakova, Sinkkonen, Luukkainen, Laitakari, Elomaa and Dietz. This is an open-access article distributed under the terms of the Creative Commons Attribution License (CC BY). The use, distribution or reproduction in other forums is permitted, provided the original author(s) and the copyright owner(s) are credited and that the original publication in this journal is cited, in accordance with accepted academic practice. No use, distribution or reproduction is permitted which does not comply with these terms.
*Correspondence: Tomi Timonen, tomi.timonen@kuh.fi
†ORCID: Tomi Timonen, orcid.org/0000-0002-4160-5036; Matti Iso-Mustajärvi, orcid.org/0000-0003-3871-8504; Pia Linder, orcid.org/0000-0002-3517-6335; Hana Vrzakova, orcid.org/0000-0002-5624-8588; Saku Sinkkonen, orcid.org/0000-0002-6601-4216; Veera Luukkainen, orcid.org/0000-0001-5295-6117; Jaakko Laitakari, orcid.org/0000-0001-9768-5074; Antti-Pekka Elomaa, orcid.org/0000-0001-9701-6882; Aarno Dietz, orcid.org/0000-0001-6076-1037