- Department of Chemical Engineering and Applied Chemistry, Chungnam National University, Daejeon, South Korea
It is unsatisfactory to define the field of microfluidics exclusively through its ability to scale down the inputs. In addition to the various well-known advantages of microfluidics, it also allows precise control over the experimental environment through the concept of laminar flow, fluid mixing, and more general fluid behavior. Techniques benefiting from the precise, small-volume fluidic control offered by microfluidics include genetic analysis, capillary electrophoresis, DNA amplification, clinical chemistry, cell-based assays, drug discovery, diagnostics, single-cell analysis, cell-cell interaction, small molecules, and synthesis of nanomaterials. Apart from the advantages of fluid dynamics in a miniaturized system, its architectures are ideal for patterning and growing artificial tissues and organs for monitoring responses to various stimuli. This unexpected reality emerged as a result of the flexibility of microfluidics to create patterns at various scales, and it also provides flow regimes that are similar to living tissue but confined to two dimensions to allow easy interrogation and interpretation. Alternatively, it can also be used to concentrate rare biological species above the common background due to the highly controllable laminar nature of the flow, ensuring fluid motion can thus be engineered for the required purpose. Some of the recognized applications are cell trapping, gradient generation, and tumor cell isolation, which seems improbable in the macroscale environment because of hindrance by chaotic fluid motion. Furthermore, microfluidics holds tremendous promise to synthesize nano- and microscale droplets (1 nm~500 μm), which opens novel approaches in drug microencapsulation. A modified version of such devices can help control droplet size and physical characteristics, which further allow us to control drug release time and particle lifetime within blood circulation as well as enhance target specificity. Principally, it has advanced in the production of monodisperse droplets, emulsions, including double and triple emulsions, bubbles, and complex particles and has opened a wide range of applications in medicine and cosmetics. It would thus be erroneous to link microfluidics specifically to a lab-on-a-chip device for biomedical application even though, at the moment, it is fostered by technical demands in the clinical area. It is thus expected to expand in the upcoming years and become fully established as a branch of Physico-chemical fluid dynamics.
Here, we focused on how microfluidics can help in a deep understanding of the mechanism that underlies cell-cell interaction. As we all know, macroscopic communication has an essential requirement of reciprocal understanding, transference of knowledge, and growth in the environment. This trend applies to the single cells that form multi-microsocieties, which are constantly in a dynamic state through a complex network of the signaling pathways. Cell-cell interaction is thus heavily centered on a signaling cascade. This cascade provides an evocative illustration of logic for cellular dynamics. These dynamics further enable the coordinated functioning of multicellular organisms among which cell-cell interaction drives numerous physiological processes. However, it is challenging to distinguish the exclusive effect of cell-cell interaction from cell-substrate interaction in adherent cells. The reverse is rudimentary because some cell models can be studied with sparsely plated cells. Both interactions play a vital role in ensuring tissue composition, architecture, formation, and functions which are largely mediated by indirect communication through cellular components such as amino acids, water, ions, and other solutes. In addition to indirect communication (intracrine, autocrine, and juxtacrine), cells rely on direct interaction through communication junctions (Connexins, plasmodesmata, occluding junction, and anchoring junction) to maintain tissue integrity and provide structural support. Thus, dysfunctioning of the signaling cascade that sustains fundamental cellular communication is associated with pathological conditions, including cancer. Being able to decipher the molecular basis for a particular phenotype will provide insight into the logical cascade and open ways to new therapies to fight improper signal transduction. Assays at the single-cell level will give a more comprehensive and sophisticated understanding. For instance, it is known that bacterial cells in the gastrointestinal (GI) tract play a vital role in maintaining human health by complex signaling networks of the fundamental processes that determine larger scale characteristics. Despite it, we lack the understanding of homeostasis maintained by bacteria and mammalian cells in the GI tract. Monitoring a few dominating species might give insight into the networking dynamics and elucidate the fundamental mechanism of host physiology and synbiotic relation. A great potential of microfluidic devices results from its outperforming capability compared to conventional methods, overcoming limitations like protein denaturation, protein purification, limited capture agents, low detection quality, and special sorting conditions due to its automation capability, low reagent volume requirement, and fast reaction kinetics. Microarray technology can be coupled with microfluidic platforms, enabling thousands of multiple experiments to be performed in a single device (Figure 1). Additionally, by combining with microscopic imaging of live cells, the microfluidic tools allow a precise and fast measurement of several key parameters related to intercellular dynamics.
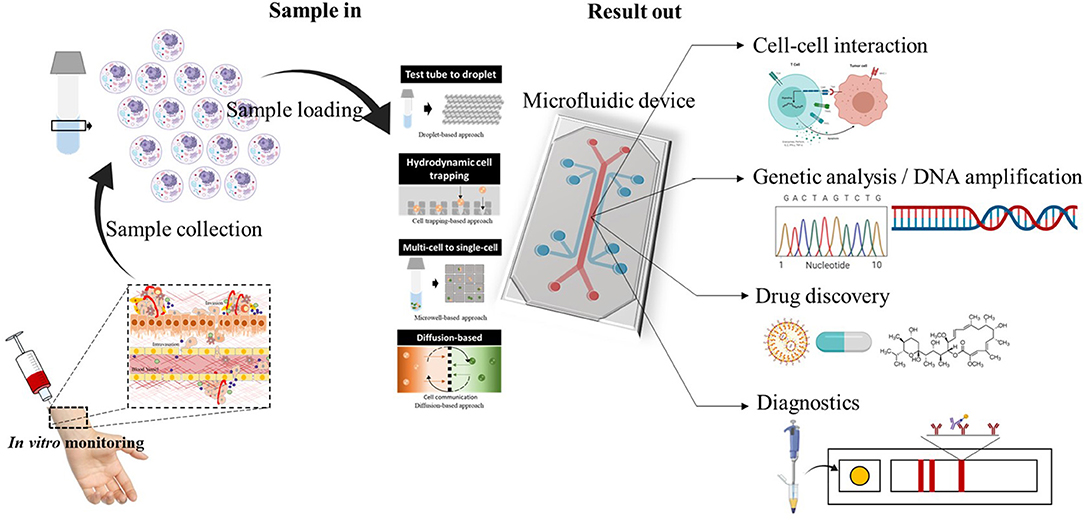
Figure 1. This figure show simple “sample-in/result-out” ability of microfluidics by integrating biological and engineering techniques.
Despite these existing capabilities it is still challenging to fully characterize cell-cell interaction. The current understanding of junctional roles is limited and, therefore, has little predictive power. It is still unclear which protein plays a major role in cell-cell adhesion. Knowing and tracking all the key players at a junction and other places in the microenvironment is of considerable interest. However, lack of resolution up to the subcellular level or requirement of overexpression has impeded broad study on these areas. Furthermore, while microfluidics has been a revolutionary approach to address cell biology at the single-cell level, relatively few chip designs are being used repeatedly for coculture of different cell lines. Much research has aimed to unfold cellular morphology, movement, and cytokine secretion or for the purposes of therapeutic medicine to show clinical utility. Even at its outstanding performance, microfluidics slows down the development due to factors like unstable cell viability in the chip and fluid shear stress, which affects the cellular interaction study. Hence, complex studies including the role of junctional protein in signaling, notably mechanotransductive signaling remain unexplored. Although microfluidics promises to be a conceivable technology, the greatest current limitation is arguably an indirect correlation between protein abundance and protein activity. Proteins such as protease, kinase, and phosphatase are synthesized and secreted in an inactive form, which is converted to an active form by enzymatic cleavage. Thus, a complete realization of cell-cell interaction through microfluidics is yet far from pragmatic. In terms of improving techniques, its acceptance for broad application as an alternative to conventional methods is yet far from reality. Most of the efforts are proof of principle in nature rather than demonstrations of large-scale applications. Many companies are commercializing biochips, in vitro cell models, and biosensors but are also creating relevant microdevices for cell–cell interaction studies; a lack of limitation is also desirable. Beyond the specific challenges in the study of cell-cell interaction, microfluidics has numerous obstacles in biomedical applications, particularly for diagnostic devices where subtle interactions can result in an inaccurate assessment. The interplay between plastics, adhesive, and other materials can dramatically alter assay function. Thus, microfluidic technology has to be rooted in practical applications that can be cost-effectively manufactured at a large scale. Also, it is a highly interdisciplinary domain that needs the combined involvement of fluidic expertise, assay scientists, material experts, and instrument experts to deliver the appropriate and desired functionality to a device. Besides technical improvement, it is advisable also to focus on profiling disease-associated organs and tissues, which will unfold potential biomarkers and drug targets. Finally, effective analyses and data mining through sophisticated microfluidic devices have tremendous potential to fill the information gap that existing technology has so far failed to unravel.
Author Contributions
The author confirms being the sole contributor of this work and has approved it for publication.
Funding
This research was supported by the Global Research Laboratory (GRL) Program through the National Research Foundation of Korea (NRF), which is funded by the Ministry of Science and ICT (NRF-2015K1A1A2033054).
Conflict of Interest
The author declares that the research was conducted in the absence of any commercial or financial relationships that could be construed as a potential conflict of interest.
Keywords: microfluics, physico-chemical fluid dynamics, cell-cell interaction, diagnostic device, biosensors
Citation: Lee C-S (2020) Grand Challenges in Microfluidics: A Call for Biological and Engineering Action. Front. Sens. 1:583035. doi: 10.3389/fsens.2020.583035
Received: 14 July 2020; Accepted: 14 August 2020;
Published: 04 September 2020.
Edited and reviewed by: Dermot Diamond, Dublin City University, Ireland
Copyright © 2020 Lee. This is an open-access article distributed under the terms of the Creative Commons Attribution License (CC BY). The use, distribution or reproduction in other forums is permitted, provided the original author(s) and the copyright owner(s) are credited and that the original publication in this journal is cited, in accordance with accepted academic practice. No use, distribution or reproduction is permitted which does not comply with these terms.
*Correspondence: Chang-Soo Lee, cmhhZHVtQGNudS5hYy5rcg==