- 1Molecular Glycobiotechnology Group, Biochemistry, School of Natural Sciences, Ryan Institute and MaREI, National University of Ireland, Galway, Ireland
- 2Anglo Beef Processors Ireland, Louth, Ireland
Paunch contents are the recalcitrant, lignocellulose-rich, partially-digested feed present in the rumen of ruminant animals. Cattle forage in Europe is primarily from perennial and Italian ryegrasses and/or white clover, so paunch contents from forage-fed cattle in Europe is enriched in these feedstuffs. Globally, due to its underutilisation, the potential energy in cattle paunch contents annually represents an energy loss of 23,216,548,750–27,804,250,000 Megajoules (MJ) and financial loss of up to ~€800,000,000. Therefore, this review aims to describe progress made to-date in optimising sustainable energy recovery from paunch contents. Furthermore, analyses to determine the economic feasibility/potential of recovering sustainable energy from paunch contents was carried out. The primary method used to recover sustainable energy from paunch contents to-date has involved biomethane production through anaerobic digestion (AD). The major bottleneck in its utilisation through AD is its recalcitrance, resulting in build-up of fibrous material. Pre-treatments partially degrade the lignocellulose in lignocellulose-rich wastes, reducing their recalcitrance. Enzyme systems could be inexpensive and more environmentally compatible than conventional solvent pre-treatments. A potential source of enzyme systems is the rumen microbiome, whose efficiency in lignocellulose degradation is attracting significant research interest. Therefore, the application of rumen fluid (liquid derived from dewatering of paunch contents) to improve biomethane production from AD of lignocellulosic wastes is included in this review. Analysis of a study where rumen fluid was used to pre-treat paper sludge from a paper mill prior to AD for biomethane production suggested economic feasibility for CHP combustion, with potential savings of ~€11,000 annually. Meta-genomic studies of bacterial/archaeal populations have been carried out to understand their ruminal functions. However, despite their importance in degrading lignocellulose in nature, rumen fungi remain comparatively under-investigated. Further investigation of rumen microbes, their cultivation and their enzyme systems, and the role of rumen fluid in degrading lignocellulosic wastes, could provide efficient pre-treatments and co-digestion strategies to maximise biomethane yield from a range of lignocellulosic wastes. This review describes current progress in optimising sustainable energy recovery from paunch contents, and the potential of rumen fluid as a pre-treatment and co-substrate to recover sustainable energy from lignocellulosic wastes using AD.
Introduction
Climate change is having a devastating impact on the planet through natural disasters, like floods, droughts, severe storms, fires, increased global temperatures, rising sea levels and increased precipitation (Fawzy et al., 2020). One way to tackle this is through increased use of low-carbon energy, e.g., solar energy, wind energy, hydro energy and bioenergy to displace fossil fuel-derived energy. Over the past decade, renewable energy production has increased steadily worldwide, and is growing at a faster rate than fossil fuel-based energy production. If the increase in the annual production of renewable energy is greater than the increase in energy demand, there will be an overall decrease in fossil fuel energy production, and therefore a reduction in global CO2 emissions (Bertram et al., 2021).
The Relevance of Paunch Contents as a Sustainable Energy Source
Lignocellulosic biomass is an abundant sustainable energy source. Sources include by-products from forestry, paper and pulp industries, and the agricultural industry (Abdeshahian et al., 2020). Paunch contents are an agri-food industry by-product. Currently this biomass-rich waste provides no value to meat processors but has the potential to be utilised for renewable energy due to its high lignocellulose content of c.63.65%; perennial and Italian ryegrasses (Lolium perenne and Lolium multiflorum) dominate European pastureland with 70% coverage (Fowler et al., 2003), resulting in paunch contents being enriched in these feedstuffs (see Table 1). Approximately 8,593 dry-matter tonnes (DMT) of paunch contents are produced from cattle in Ireland annually, according to calculations based on Annual Environmental Reports (AERs) (AERs-Environmental Protection Agency (EPA) Ireland, 2021). The potential energy of this dry matter (DM) is 16.7–20 MJ/kg (Ricci, 1977; Spence, 2017). Currently, 143,503,100–171,860,000 MJ of potential energy per year in Ireland, and 23,216,548,750–27,804,250,000 MJ globally, could be recovered from paunch contents (see Section Volume of Paunch Contents and Rumen Fluid Generated Globally).
In the EU, waste by-product recovery has become important to slaughterhouses that have a 50 tonnes/day slaughter capacity (all beef slaughterhouses in Ireland), as it is now their legal obligation to use the current Best Available Techniques (BATs) for industrial emissions (BAT Guidance Note on Best Available Techniques for the Slaughtering Sector, 2008; Directive 2010/75/EU, 2010). In Ireland, this obligation has particular relevance as its agricultural sector accounts for 33% of all greenhouse gas emissions and 45% of all Irish non-Emissions Trading System (ETS) emissions. The EU has set obligatory targets for non-ETS emissions reduction by 2020 and 2030. For 2020, Ireland's target was 20% reduction in non-ETS emissions compared to 2005, but in 2018 the country was on track to achieve just a 1% reduction (CO2 Emissions | Energy Statistics in Ireland | Sustainable Energy Authority of Ireland, 2020-2022). By 2030, a 30% reduction on 2005 figures must be achieved. To meet this target, Ireland's agri-food sector needs to harness all routes to reduce greenhouse gas emissions. Therefore, immediate action is required to enable countries with important agri-economies, like Ireland, to make the necessary reductions in non-ETS emissions (SEAI, 2018).
Methods Utilised to-Date to Recover Energy From Paunch Contents
The energy potential of paunch contents has been highlighted using biomethane potential (BMP) tests, where paunch contents were co-digested with other slaughterhouse wastes (Ware and Power, 2016). This study showed that one Irish beef slaughterhouse, which accounts for ~3% of cattle slaughtered every year nationally, could meet its energy needs in full (with a surplus), from the biogas produced. Conventional pre-treatments have been shown to increase hydrolysis of lignocellulosic materials in AD (Nkemka et al., 2015). However, some pre-treatments can result in the overall process costing more than the value of biogas produced. Pre-treatment examples include thermal, physical, biological and chemical pre-treatments. Biological pre-treatments, e.g., enzymatic approaches, hold advantages over conventional pre-treatments, as they require less energy, are more controllable, need uncomplicated equipment and operating conditions, have a lower chemical recycling requirement after the treatment, can be combined with low-cost downstream processing, and result in no/very little inhibitor formation (Xing et al., 2020).
Other sustainable energy-recovery options have also been investigated for paunch contents, including its co-combustion (Bridle, 2011, 2012; Spence, 2012, 2017). Experiments showed that to be used as a boiler fuel, paunch contents should have a moisture content ≤ 35%, with Spence (2017) finding that this was the best moisture content for energy recovery from paunch contents. These experiments also showed that slaughterhouses could make financial savings of $26,000 AUD–$90,000 AUD annually by using this waste as a boiler fuel. However, AD appears to be the predominant method employed to optimise energy recovery from paunch contents to-date.
Role of Rumen Fluid and the Rumen Microbiome in Lignocellulosic Degradation
The rumen microbiome is very efficient at degrading lignocellulosic material. Consequently, much interest exists in obtaining a better understanding of the biological mechanisms involved in this degradation (Henderson et al., 2015). Recent research has shown that rumen fluid (the liquid fraction contained in the paunch) can be used as a pre-treatment and co-substrate prior to/during AD to improve the methane yield from lignocellulosic wastes like paper waste (PW) (Baba et al., 2013; Takizawa et al., 2019), paper sludge (Takizawa et al., 2018), and rice straw (Zhang et al., 2016). There is still much to discover about the functions of rumen microorganisms. Once a better understanding of these functions is gained, opportunities will result to improve the degradation of paunch contents and other lignocellulose-rich wastes, e.g., by replicating their mechanisms outside of the rumen for the biological pre-treatment of lignocellulosic feedstock.
Aims of Review
Sustainable energy sources that are currently underutilised, like paunch contents derived from meat production and lignocellulosic wastes from other industries, e.g., paper waste, must be explored to minimise human impact on the environment. This review aims to describe the progress being made in utilising paunch contents for energy recovery and some of the current challenges that exist. The review also explores the use of rumen fluid in degrading lignocellulosic wastes to improve biomethane production from AD, as well as recent developments in rumen microbiome discovery.
Current and Historical Methods for Treatment and Disposal of Paunch Contents
Historically, paunch contents have been challenging to treat. Underlying reasons include the associated high biochemical oxygen demand, its tendency to form masses that block bar screens, pits, hopper bottoms and suction pumps, as well as increased settlement over time at the bottom of tanks and in pipes where paunch contents can become as hard as low-density rock. Removing these settlements requires the use of an auger. Furthermore, compaction reduces accessibility and digestibility of the cellulose fraction in anaerobic digesters, thus causing further blockages and capacity issues over time. Paunch contents also have low protein and high moisture contents (see Table 1). For these reasons amongst others, including odour and designation as a high-risk biological waste, paunch contents are not treated conventionally, e.g., in sewage plants (Witherow and Scaief, 1976; Ricci, 1977).
Historically, disposal methods have ranged from incineration to spreading on non-agriculture land to landfilling, all of which are no longer considered best practise. Today, over 40 years after these issues were described, paunch contents remain difficult to treat. For instance, hydrolysis of the fibrous material contained in paunch contents by microbes continues to be the rate-limiting step in recovering potential energy from AD (Nkemka et al., 2015). Until recently, paunch contents were sent by beef processors to landfill (Murphy et al., 2011; AERs-Environmental Protection Agency (EPA) Ireland, 2021). However, to minimise the environmental impact of waste by-products like paunch contents from the slaughterhouses, current BATs now must be applied (BAT Guidance Note on Best Available Techniques for the Slaughtering Sector, 2008; Directive 2010/75/EU, 2010, 2016).
After the paunch has been removed from the cow, dry dumping (water is not added to aid in emptying paunch) is the current BAT, as opposed to wet dumping (where contents are manually flushed out of the paunch). With wet dumping, only 10–30% of nitrogen and phosphorous and 40% of total solids can be recovered from the waste stream by sedimentation or screening, resulting in the waste stream being high in pollutants. Dry dumping reduces the water quantity used during paunch handling by ≤ 90% (Hui et al., 2001; Integrated Pollution Prevention Control Reference Document on Best Available Techniques in the Slaughterhouses Animal by-products Industries, 2005; BAT Guidance Note on Best Available Techniques for the Slaughtering Sector, 2008).
After paunch-emptying, beef processors usually dewater paunch contents with a paunch press (average DM content is around 23.4% after this according to AERs) and send it off-site for land spreading in accordance with BAT (Integrated Pollution Prevention Control Reference Document on Best Available Techniques in the Slaughterhouses Animal by-products Industries, 2005; Murphy et al., 2011; BAT Documents (BREFs) / Commission Implementing Decisions - Environmental Protection Agency, 2021). The screw press produces paunch contents with the highest DM quantity, followed by the screen press, wedge wire screen and the vibrating screen, respectively, in terms of dewatering capability (EPA Tasmania, 2017; Paunch Contents Land Spreading Management Guidelines, 2017). The rumen fluid from the dewatered paunch contents is treated in a wastewater treatment plant on-site, after which it, and other treated sludges, are recycled/reclaimed through composting and other biological transformation processes (AERs-Environmental Protection Agency (EPA) Ireland, 2021).
Following dewatering, current BATs for the treatment of paunch contents involve composting, AD and land spreading/injection (BAT Guidance Note on Best Available Techniques for the Slaughtering Sector, 2008). Sending paunch contents off-site for recovery as fertiliser helps slaughterhouses to reduce their waste-to-landfill output (A Resource Opportunity- Waste Management Policy in Ireland, 2012), but the composting of paunch contents is being restricted and regulated increasingly and the process is a major source of odour (Jensen et al., 2016).
Volume of Paunch Contents and Rumen Fluid Generated Globally
The paunch contents quantity produced per head of cattle varies significantly. It can depend on several factors including the animal size, the fasting period length before slaughter, the type of feed given and the animal's age (Tritt and Kang, 1991). Depending on the time of year and their life-stage, an animal's diet will vary. It will also vary depending on whether the cattle are being prepared for the premium or non-premium beef market. Furthermore, individual farmers may have different feeding preferences. In addition, cattle eat silage, fresh grass or processed feed in the UK (as in Ireland), depending on the time of year and weather (Garnett, 2007). Consequently, there are many variables that underpin paunch contents heterogeneity, volume and composition.
The values for paunch contents produced per head of cattle vary in the literature, due to the aforementioned factors. Furthermore, reported paunch contents values may be based on wet, dewatered or DM. Approximately 3.8 kg of dry paunch contents is produced per head of cattle (Baumann, 1971; Witherow and Scaief, 1976; Ricci, 1977). In Ireland, dewatered paunch contents are ~20 ± 8 kg per head of cattle (Sustainable Practices in Irish Beef Processing, 2009). This is comparable to some available international values, e.g., 20 kg per head of cattle in Tasmania, Australia (EPA Tasmania, 2017). However, the range of values reported is much wider for wet paunch contents per head of cattle, which can be expected due to variable moisture contents. For example, earlier studies reported 23–25 kg of wet paunch contents produced per head of cattle (Baumann, 1971; Witherow and Scaief, 1976; Ricci, 1977). However, according to the Wilson (1992), and United Nations Environment Programme and EPA Denmark (2000), wet paunch contents are 36–45 kg per head of cattle. In a report by Verheijen et al. (1996), 27–40 kg of wet paunch contents were produced per head of cattle, which is similar to values (25–40 kg) reported by Antille et al. (2019), while Tritt and Kang (1991) reported 40–60 kg of wet paunch contents per head of cattle.
Taking 20 kg as the average quantity of dewatered paunch contents per head of cattle (Sustainable Practices in Irish Beef Processing, 2009; EPA Tasmania, 2017), and a DM content of 23.4% from analysis of the AERs from Irish beef processors (AERs-Environmental Protection Agency (EPA) Ireland, 2021), the average dry paunch contents quantity produced per head of cattle is 4.68 kg, similar to previous reports (Baumann, 1971; Witherow and Scaief, 1976; Ricci, 1977). In the literature, the average moisture content of dewatered paunch contents was also reported as being ~80–86% (Witherow and Scaief, 1976; Ockerman and Hansen, 1988; Abouheif et al., 1999), which is in line with an approximate DM content reported in the literature of ~15.8% (Table 1).
From 2016 to 2019, an average of 1,836,083 bovines were slaughtered annually in Ireland (Eurostat, 2020), accounting for ~8,593 DMT paunch contents. In the EU, the average annual slaughter numbers from 2016 to 2019 were 26,514,158 bovines (including the UK) (FAOSTAT, 2020). This equates to an annual paunch contents production of c.124,086.26 DMT (including the UK) within the EU. The average number of cattle slaughtered annually worldwide from 2015 to 2018 was 297,053,954 (FAOSTAT, 2020), corresponding to c.1,390,212.5 DMT global paunch contents. These values highlight the abundance of this underutilised waste product globally. Table 2 describes this potential value in economic terms and reveals that €803 million worth of energy is available in paunch contents globally annually.
Reports on rumen fluid volumes per head of cattle have been more uniform, with volumes of 44–54.7 L being reported (Bauman et al., 1971; Wohlt et al., 1976; Rogers et al., 1982; Estell and Galyean, 1985; Stokes et al., 1985; Moloney et al., 1993). This translates into per annum volumes of c.80,787,652–100,433,740 L of rumen fluid in Ireland (2016-2019), c.1,166,622,952–1,450,324,443 L in the EU (including the UK between 2016 and 2019), and c.13,070,373,980–16,248,851,280 L globally between 2015 and 2018. These values highlight the volumes of rumen fluid that are underutilised globally despite the significant potential to utilise rumen fluid, rich in lignocellulolytic enzymes, as a pre-treatment of lignocellulosic wastes prior to AD or as an inoculum during AD to improve methane yields (see Sections Rumen Fluid From Cattle as a Source of Lignocellulolytic Enzymes for AD and The Rumen Microbiome).
Sustainable Energy Production From Paunch Contents
Paunch contents could be of high value to the meat-processing industry as a sustainable source of energy (Table 2). However, it is currently being lost due to offsite spreading practises. The major bottleneck in using this waste for sustainable energy recovery is its recalcitrance (Nkemka et al., 2015), meaning that post-AD, residual paunch waste remains at the bottom of the digester, rich in unutilised carbon, that needs to be spread offsite. However, if this bottleneck was overcome, the recovered energy would reduce the meat processors' impact on the environment by decreasing fossil fuel dependency of slaughterhouses. This would in turn create a more sustainable meat-processing industry. Different methods have been suggested for energy recovery and bioconversion of paunch contents, including pyrolysis, torrefaction, liquefaction and gasification and hydrothermal carbonisation (Marzbali et al., 2021). Combustion as a boiler fuel and biogas production through AD have been the methods used most to date for sustainable energy recovery from paunch contents (Ware and Power, 2016; Spence, 2017). Paunch contents as a boiler fuel will first be described. However, AD will be the focus, as it has been the main approach described in the literature for biomethane generation from paunch contents.
Using Paunch Contents as a Boiler Fuel
While most efforts to produce sustainable energy from paunch contents have focused on AD, their use as a boiler fuel has been investigated for energy recovery. Bridle (2011) investigated co-combustion of paunch contents dewatered to <30% solids content with sawdust and later investigated co-combustion of dewatered paunch contents (<30% solids) with DAF sludge and wood waste as a boiler fuel (Bridle, 2012). In these studies, anything higher than 30% moisture content in the paunch contents was deemed inefficient for co-combustion (Bridle, 2011, 2012). A moisture content >85% has been shown to have a negative energy value, with the net calorific value being −2.5 MJ/kg when the moisture content is 100% (full saturation) (Spence, 2017). Also, a loss of 0.1% in energy efficiency for every 1% increase in the moisture content of paunch contents has been reported (Hatt, 1997). Therefore, the lower the moisture content, the better the energy output from co-combustion, pyrolysis and gasification, all of which are alternative methods to produce energy from paunch contents. However, this relationship appears to be true up only to a certain point, as Spence (2017) demonstrated the best moisture content for energy return from both grass and grain paunch contents was 35%; in this context, the moisture may have acted as a lubricant and increased the force of compaction on the paunch contents (Spence, 2017).
The Bridle (2011, 2012) studies showed that co-combustion of paunch contents with DAF sludge or sawdust had the potential to reduce greenhouse gas emissions and provide energy for the slaughterhouses, while reducing amounts of paunch contents sent off-site for composting or land spreading. A cost-benefit analysis of co-combustion with sawdust revealed potential yearly savings of ~$72,000 AUD on fuel costs and ~$90,000 AUD on disposal/recovery costs for the slaughterhouse in question, while a cost-benefit analysis of co-combustion with wood waste showed yearly savings of ~$39,000 AUD and ~$26,000 AUD on fuel and disposal/recovery costs (Bridle, 2011, 2012; Spence, 2017). On the basis that paunch contents need a DM content of ≥70% to be useful as a biofuel in processes like pyrolysis, gasification and to replace coal as boiler fuel, Spence (2017) determined predictive equations and paunch contents characteristics and developed new coefficients for the Hukill equation. This will enable further work toward developing an ideal paunch contents dryer (Spence, 2017).
Another study investigated the theoretical feasibility of immersion-frying to dewater paunch contents and DAF sludge to a moisture content of <5%, to make high-energy pellets suitable for combustion as a boiler fuel in slaughterhouses (Hamawand et al., 2017). It was hypothesised that by reducing the moisture content to this level, combustion would be more stable, and less CO2 would be produced, compared to paunch contents dewatered to a 20–29% solids content. The pay-back period would be 1.8–3.2 years. The study concluded that this approach was economically feasible and that pellets produced would have the energy equivalent of coal/kg but would cost half as much as coal. Further experiments must be carried out to demonstrate whether this process is feasible at-scale and to identify technical constraints which may arise from its integration into existing meat-processing facilities (Hamawand et al., 2017). However, it must also be considered that Spence (2017) established that the optimum energy output from paunch contents was achieved at a moisture content of 35%, so dewatering paunch contents to <5% moisture content may reduce the net energy gain. Although drier paunch contents have a higher calorific value, the bulk density is lower due to empty pockets of air present, which reduces energy output/m3 (Spence, 2017).
Anaerobic Digestion of Paunch Contents
The AD of paunch contents showed that they have higher first-order decay rates compared to virgin plant biomass, e.g., Napier grass, because paunch contents are already partially digested in the animal rumen, which enables more rapid AD outside of the rumen (Tritt and Kang, 1991). This natural pre-treatment speeds up the digestion process so that up to 80% of the biomethane yield can be obtained within 16–17 days of digestion, thereby making it feasible to use as an industrial feedstock (Ware and Power, 2016). So far, research into the potential of using paunch contents alone as an energy feedstock for AD has been limited. Most studies have focused on paunch contents co-digestion with other wastes. The presence of rumen microorganisms in paunch contents is thought to aid the hydrolysis of complex carbohydrates like cellulose. Although a synergistic effect has been shown when different wastes are co-digested, uncaptured energy remains in recalcitrant lignocellulosic material left over at the bottom of the digester (Banks and Wang, 1999; Edström et al., 2003; Ló Pez et al., 2006; Buendía et al., 2009; Palatsi et al., 2011; Browne et al., 2013; Jensen, 2013; Astals et al., 2014).
Is AD of Paunch Contents Economically Feasible for Sustainable Energy Recovery?
According to Jensen et al. (2016), whether the process of anaerobically digesting paunch contents is economically feasible or not is dependent on (a) the methane potential (can the methane produced reduce slaughterhouse energy costs?), (b) the degradable fraction (will it reduce recovery costs?), and (c) the time the paunch contents take to degrade (this will affect the digester size and overall cost of running the process).
Ware and Power (2016) showed that paunch contents co-digested with dissolved air floatation sludge and soft offal for biomethane production is economically feasible for slaughterhouses. Using BMP tests, the biomethane yield from paunch contents waste alone was 229 L CH4/kg VS under mesophilic conditions, while the mixed waste stream had a biomethane yield of 641.55 L CH4/kg VS. The inoculum:substrate used for each BMP test was 2:1. The maximum methane potential and specific methane yield (SMY) values were very similar (226.7 L CH4/kg VS & 228.8 L CH4/kg VS), indicating that the maximum methane amount was produced over the 30-day reaction (Ware and Power, 2016).
A detailed net energy analysis was conducted by Ware and Power (2016) to assess the economic feasibility of using these wastes as a feedstock to produce biomethane to power the slaughterhouse activities on-site. Bishop et al. (2009) concluded that the predicted methane production from BMP tests correlates well with actual methane production from a full-scale reactor (R2 = 0.83 and the over-prediction value = 1.54%). To make this net energy analysis more accurate, only 80% of the SMY produced by the mixed waste was used in the calculations. If 100% of the SMY was considered, the hydraulic retention time (HRT) would be assumed to be 50 days, which would be unrealistic in industry. Usually, the HRT would be ~20 days in a continuously-fed bioreactor. A further consideration made to correlate a continuously-fed bioreactor at full-scale with a batch-fed BMP test, omitted the lag phase (12.5 days) from calculations as it would not exist in a continuously-fed bioreactor (the microorganisms would already be in the log phase of growth). Therefore, it was determined that 80% of the SMY would be produced within 16.5 days, which is more representative of a full-scale operation (Ware and Power, 2016).
An annual gross methane yield of 1,628,258 m3 was reported by Ware and Power (2016). Combusting this directly in a CHP unit resulted in 15,061 MWh of energy being recovered. The annual energy demand of the test slaughterhouse was 5,874 MWh of electricity and 7,786 MWh of thermal energy. In Ireland, the average cost of 1 kWh of electricity for businesses in 2020 was 15.89c, and 3.44c for gas (including Value Added Tax) (Prices | Energy Statistics in Ireland | Sustainable Energy Authority of Ireland, 2021). This translates into an annual expenditure of >€1.2 million to power this slaughterhouse, when gas is used to provide thermal energy, or €2.17 million if electricity provides the thermal energy. The biomethane produced from the co-digestion process has an energy value greater than that of the annual energy use of the slaughterhouse (by 1,401 MWh). Therefore, this slaughterhouse, which processes ~52,000 head of cattle annually, could become completely self-sufficient with excess energy left over, and potential annual savings of €1.2–2.17 million. Biomethane production used 690 MWh of electricity, leaving 711 MWh of surplus energy. In 2018, 30,238 GWh of energy was used by all industry in Ireland (Energy Use Overview | Energy Statistics in Ireland | Sustainable Energy Authority of Ireland, 2021). Ware and Power (2016) calculated that 458.6 GWh of gross energy could be produced if 90% of the national mixed slaughterhouse waste stream was used to recover biomethane. This would allow combustion of the resulting biomethane in CHP units to subsidise 1.52% of the total national industrial energy requirement. This development would increase Irish Renewable Energy Shares, contribute to Ireland's renewable energy targets, and help slaughterhouses to become circular economies. Labour costs, e.g., loading, unloading and maintenance of the digester, were not included in this analysis.
The organic fraction of paunch contents has been shown to be ~59–70% biodegradable in AD. Tritt and Kang (1991) carried out batch reactor tests at mesophilic temperatures to determine the ultimate biodegradability of cattle paunch contents, using substrate:inoculum between 0.2:1 and 10:1 (total VS mass of paunch contents:total VS mass of the inoculum). The paunch contents feed total solids content was 11.3% (dry weight). A maximum BMP of 64–70% was reported and the highest biomethane yield reported was 323 L CH4/kg VS at a substrate:inoculum of 1:1. The highest value for ultimate biodegradability, also at a substrate:inoculum of 1:1, was 83% of total VS (dry weight), after a HRT of 49 days. Digesters containing substrate:inoculum <5:1 showed efficient degradation of paunch contents, with steady-state decay rates of 0.044–0.064 per day for the first 5–40 days of the reaction. Digesters containing higher substrate:inoculum of 7.5:1 and 10:1 had lower decay rates than those with ratios <5:1 (by one-half and one-third, respectively) and were inefficient at removing substrate VS because of reduced bioavailability of paunch contents to the degrading microorganisms, at the higher reactor loading rates. Overall, it was concluded that a substrate:inoculum ≤ 2.5:1 allowed rapid start-up of a full-scale anaerobic digester with cow paunch contents as substrate. This ratio allowed for 69% VS removal over 49 days. However, to achieve this degradation level a long HRT was necessary, which is less desirable in industry.
This long HRT issue was described also by Nkemka et al. (2015), who carried out AD of cattle paunch contents in a continuous stirred tank reactor (CSTR) at mesophilic temperatures. Two reactors were run with HRTs of 30 days (organic loading rate, OLR, of 2.8 g VS/L/day) and 60 days (OLR of 1.4 g VS/L/day), with paunch contents fed at 10% total solids (w/v). The biomethane yield was analysed every 1–2 days in the bioreactors. The higher OLR in the 30-day bioreactor yielded an increase in the biomethane production rate, but a lower overall biomethane yield, i.e., 213 L CH4/kg VS in the 30-day bioreactor vs. 284 L CH4/kg VS in the 60-day bioreactor. This finding suggested that the microorganisms in the digester did not have enough time to digest the available substrate and convert the organic content to biomethane. The low VFA concentrations (0.22 ± 0.04 g/L and 0.22 ± 0.02 g/L) in the effluent on days 30 and 60 of HRT indicated that methanogenesis was not the rate limiting step, but that hydrolysis of particulate organic matter was the barrier. As a result, increasing the HRT was recommended to improve degradation of the lignocellulose (Nkemka et al., 2015). However, increasing the HRT is not feasible in an industrial setting where the average AD time is ~20 days (Ware and Power, 2016).
While AD experiments have been carried out, mainly under mesophilic temperatures, temperature-phased AD (TPAD) has also been utilised. However, TPAD of paunch contents does not seem to hold benefits over the mesophilic AD process in terms of increasing biodegradability of paunch contents or increasing biomethane yield. Results from TPAD in one study showed production of 220 L CH4/kg VS, with a biodegradation value of 60% (Mehta et al., 2015). Yi et al. (2014) and Jensen et al. (2016) have also carried out TPAD and both studies showed that TPAD does not substantially improve paunch contents degradation compared to batch mesophilic AD. A maximum BMP of 230 L CH4/kg VS was recorded in batch mesophilic AD, with a biomethane yield of 63%. However, TPAD did reduce the residual paunch contents quantity through greater destruction of VS (Jensen et al., 2016). Overall, it seems to be more beneficial to operate digesters at mesophilic temperatures to save on heating costs.
Studies to-date suggest that paunch contents co-digested with other slaughterhouse wastes to recover biomethane is economically feasible, more so than digesting paunch contents on their own (Browne et al., 2013; Astals et al., 2014). It has been shown that the VS removal from paunch contents can be 75–81% when co-digested with activated sludge waste at a 25:75 ratio. This approach yielded an increase in the readily degradable fraction of paunch contents by ≤ 49%, and a four-fold increase in BMP (Buendía et al., 2009). As paunch contents may contain blood, which results in increased ammonia in the digester, it is hypothesised that by adding activated sludge waste, the C:N ratio is balanced and biomethane production is promoted (Browne et al., 2013). The higher methane yield from co-digesting slaughterhouse wastes in AD compared to that obtained from digesting paunch contents alone was described by Browne et al. (2013). An average biomethane yield of 238 L/kg VS was reported from paunch contents produced in an Irish slaughterhouse using BMP tests. The inoculum was obtained from a mesophilic, farm-scale digester, and the inoculum:substrate was 2:1. Co-digesting paunch contents, green sludge and dewatered activated sludge gave a higher biomethane yield (positive synergy) than respective yields for each individual substrate. The combined sample gave a BMP of 336 ± 15.0 L CH4/kg VS, while the weighted average of the three individually digested substrates was 288 ± 11 L CH4/kg VS. Jensen (2013) also showed that co-digesting DAF sludge with paunch contents had a synergistic effect on the BMP under mesophilic conditions, because of the improved C:N ratio (Browne et al., 2013) and because rumen microorganisms in the paunch contents were likely to be capable of lipolytic activity (Jensen, 2013).
However, the long HRT required to biodegrade recalcitrant lignocellulose in paunch contents remains to be resolved and is an important technological issue to solve to maximise energy recovery. To overcome the obstacle of hydrolysis as the rate-limiting step, additional pre-treatments could provide a viable improvement by making the lignocellulose matrix more accessible to microbes. By pre-treating high-fat wastes and co-digesting with other sludge wastes prior to AD, it can increase the biomethane yield relative to other biogases present, with increased waste biodegradability overall (Buendía et al., 2009; dos Santos Ferreira et al., 2020). Further methods to optimise co-digesting paunch contents with DAF sludge, along with the impacts of pre-treatments for these wastes on the BMP, must be investigated.
Pre-treatment of Paunch Contents Prior to AD
As highlighted in section Is AD of Paunch Contents Economically Feasible for Sustainable Energy Recovery?, co-digesting paunch contents with other slaughterhouse wastes generally increases biomethane production, improves the overall waste biodegradability, and is economically feasible. However, a suitable pre-treatment to increase microbial accessibility to the lignocellulose fraction of paunch contents may further increase the biomethane yield from paunch contents, both when digested alone and with other substrates. Astals et al. (2014) reported the BMP for solid paunch contents waste as 237 L CH4/kg VS for batch mesophilic AD, which corresponds to a degradable fraction of ~60%, and a hydrolysis rate of ~11% per day. As the hydrolysis rate and methane yields were low compared to other slaughterhouse wastes, pre-treatment technologies aimed at improving digestion of paunch contents could be used to improve the overall digestion process.
Few studies have been carried out specifically on the pre-treatment of paunch contents. In a study by Nkemka et al. (2015), the BMP of paunch contents, pre-treated with NaOH at 22°C for 3 days, and at 70 and 100°C for 24 h, was defined using a BMP batch test and compared with the BMP of untreated paunch contents, over 27 days at 40°C. The BMP increased with the NaOH pre-treatment. Six percent NaOH pre-treatment at 70 and 100°C increased the paunch contents solubility the most, but the difference between BMP outputs for untreated (299 L CH4/kg VS) and pre-treated paunch contents (318 L CH4/kg VS) was not considered significant. After 12.4 days in the bioreactor, the pre-treated paunch contents increased the production rate of the digester, yielding a 32% increase in biomethane levels, compared to the digester with the untreated paunch contents. Twenty-seven days was long enough for most of the available energy in the form of biomethane to be produced (Nkemka et al., 2015). Methods applying NaOH and heat as pre-treatments have been used frequently when anaerobically digesting grasses, to delignify lignocellulosic material, thus making it less recalcitrant (Wang et al., 2010; Kataria and Ghosh, 2014; Karp et al., 2015; Rodriguez et al., 2017), and the paper by Nkemka et al. (2015) shows that it can be effective in pre-treating paunch contents also.
Pre-treatments that have been used on grass include physical, thermal, chemical, ultrasound and microwave (Rodriguez et al., 2017). These pre-treatments could also work to pre-treat paunch contents given their similar compositions (Table 1). However, they are not generally used in combination with AD as the energy quantity recovered can make the investment not worthwhile. Biological pre-treatments, e.g., fungal, ensiling, microbial consortium or enzymatic, however, are advantageous over the conventional physicochemical pre-treatments, as they require less energy, uncomplicated equipment and operating conditions, can be more targeted and controllable, recycling of chemicals after the treatment is unnecessary, downstream processing is low-cost, and there is very little, or no, inhibitor formation (Xing et al., 2020).
Despite the benefits of using biological pre-treatments on lignocellulosic wastes, at the moment, the overall economic feasibility of using a biological pre-treatment on paunch contents in industry is unclear, as studies to date have been focused on the scientific aspects of the pre-treatments. A financial barrier could arise from the high cost of commercial enzymes (Hosseini Koupaie et al., 2019), but this could be bypassed by the development of low-cost bulk enzyme cocktails or recycling enzyme cocktails, for example. To the authors' best knowledge, paunch contents have not been pre-treated biologically to-date outside of the rumen.
Rumen Fluid From Cattle as a Source of Lignocellulolytic Enzymes for AD
In addition to being a source of biomass in the form of paunch contents, the rumen is potentially an excellent source of microorganisms with lignocellulolytic capability. In the environment of the rumen, microbes degrade recalcitrant lignocellulose. Rumen fluid has been used to improve the degradation of alternative lignocellulose-rich wastes like PW (Baba et al., 2013; Takizawa et al., 2019), paper sludge (Takizawa et al., 2018), rice straw (Zhang et al., 2016; Deng et al., 2017), corn straw (Jin et al., 2018), corn stover (Li et al., 2017a,b), grass clippings (Wang et al., 2018), and rapeseed (Baba et al., 2017, 2019) through AD. Key enzymes are likely to include cellulases and hemicellulases, which are important in lignocellulolytic degradation (Romano et al., 2009). A study by Takizawa et al. (2020b), for example, showed that endoglucanases produced by microorganisms in rumen fluid exhibit high activity over a 24 h period when rumen fluid was used to treat carboxymethylcellulose. The endoglucanases were likely to be from uncharacterised and non-dominant microbes, which reinforces the fact that much of the rumen microbiome and its functions are yet to be discovered (see section The Rumen Microbiome). Also, some endoglucanases were shown to be active between 0 and 12 h, while the others were active between 12 and 24 h. This reflects microbial changes in the rumen community over time and the production of varying endoglucanases by different microbes (Takizawa et al., 2020b).
Using Cattle Rumen Fluid as a Pre-treatment to Improve Biomethane Yields From Lignocellulosic Wastes During AD
From previous studies, rumen fluid from grass-fed cattle, used to pre-treat PW prior to semi-continuous mesophilic AD, increased the daily methane yield 2.6-fold, with a 73.4% BMP, after a pre-treatment time of only 6 h (Baba et al., 2013). The rumen fluid (300 mL) was used to pre-treat 0.3 g of PW for 6 and 24 h at 37°C. Then 30 mL of pre-treated PW was added to 600 mL of seed sludge in duplicate 1 L bioreactors maintained at 35°C for 20 days (untreated PW as control). There were improvements in the degradation rates of cellulose (from 76.5% in control reactor to 87.9 and 85.8% in the test reactors) and hemicellulose (from 40.6% in control reactor to 55.2 and 57.5% in the test reactors) with pre-treated PW as substrate. Lignin degradation improved significantly from 10.7% in the control reactor to 39.4% when 6-h pre-treated PW, and to 51.8% when 24 h-pre-treated PW was used in the reactor. The theoretical methane yields for the control and post-AD 6 h-pre-treated PW and 24 h-pre-treated PW were 60.8, 73.4, and 63.2%, respectively. The lower methane yield at the longer (24 h) pre-treatment reflected greater loss of carbon through microbial gas (CO2) production. Overall, this study highlighted the value of rumen liquid in the pre-treatment of cellulose, hemicellulose and lignin-containing wastes. The short pre-treatment time of 6 h and 20-day reaction length in semi-continuous bioreactors make this option industrially attractive (Baba et al., 2013).
Takizawa et al. (2019) also carried out PW pre-treatment with rumen fluid at 37°C for 48 h. The rumen fluid, also from grass-fed cattle, was preserved at 4, 20, and 35°C for 7 days. Preservation at 4°C retained the hydrolytic capabilities of microorganisms in the fluid, yielded an increase in dissolved COD and a reduction in organic carbon loss. Rumen fluid preserved at 20 and 35°C, however, effected a 1.8- and 3.5-fold increase in CO2 production during PW. This study provides useful information in terms of transportation and storage longevity of rumen fluid, but further investigation of a longer preservation period would inform long-term storage possibilities.
In an earlier study by Takizawa et al. (2018), dewatered paper sludge (PS) was pre-treated with rumen fluid from grass-fed cattle for 6 h at 37°C, prior to batch mesophilic AD for 20 days. Rumen fluid pre-treatment increased the biomethane yield 3.4-fold compared to the untreated paper sludge. PS is the leftover material after production of recycled paper from wastepaper and is composed of organic fibres (lignocellulose) and ash. Levels of lignin and ash are higher than in PW, making it more recalcitrant. Neutral Detergent Fibre, Acid Detergent Fibre and Acid Detergent Lignin degradability increased from 30.3 to 37.2%, 36.5 to 38.2%, and 17 to 20.9%, respectively, post-AD when pre-treated paper sludge was used as substrate. The biomethane yield from untreated and pre-treated paper sludges post-AD were 67.9 L CH4/kg VS and 231.3 L CH4/kg VS, demonstrating that pre-treatment improved methane yield of paper sludge 3.4 times. Here again, the short pre-treatment time of 6 h and AD running time of 20 days would be attractive to industry.
Baba et al. (2019) used rumen fluid to treat rapeseed prior to batch AD fermentation and reported the effects that the rumen microorganisms had on the indigenous microorganisms in the seed sludge. The rapeseed was either untreated (control) or pre-treated for 6 or 24 h before being added in 200 mL volumes to duplicate 1 L batch digesters containing 400 mL of seed sludge. The digesters operated at 35°C for 32 days. Pre-treated rapeseed significantly improved the biomethane yield (2,077.3 mL/reactor for 6 h and 1790.4 mL/reactor for 24 h pre-treatment) in contrast to untreated rapeseed (1,325.8 mL/reactor). The effects of rumen microorganisms on flora in native seed sludge were also significant. Compared to the control digester, many hemicellulolytic and cellulolytic bacteria native to the seed sludge either decreased or did not increase in abundance. Conversely, rumen fluid bacteria closely associated with Ruminococcus albus, an established cellulolytic and hemicellulolytic bacterial species (see The Rumen Microbiome) remained established in the fermenter until the end of the process. Furthermore, a rumen archaeon associated with the established H2-utilising archaea Methanosphaera and Methanobrevibacter, was detectable until the end of the process. This study revealed the importance of microbial community structure during AD in optimising methane production and highlights again that much remains to discover about the rumen microbiome.
Rice straw, one of the main agricultural wastes in China, was pre-treated using rumen fluid by Zhang et al. (2016). Rice straw was pre-treated at 39°C for 120 h prior to AD at 35°C for 30 days in 1 L batch digesters with a working volume of 500 mL; untreated rice straw was used as the control. The biomethane yield increased by 40.5–82.6% when pre-treated rice straw was digested vs. the untreated rice straw (pre-treated rice straw methane yields ranged from 218.5 to 285.1 L CH4/kg VS, while the control yielded 156.1 L CH4/kg VS). The optimal pre-treatment time for biomethane yield, in this study was 24 h. Between 12 and 24 h, the biomethane yield increased and when the pre-treatment was longer than 24 h the yield decreased. After 24 h of pre-treatment a significant degradation of lignin (~15%), hemicellulose (~38%), and cellulose (~28%) was observed. However, this study did not analyse the effects of pre-treatments <12 h on methane yield post-AD, which based on studies by Baba et al. (2013, 2019) would be interesting.
Co-digestion of Lignocellulosic Wastes With Cattle Rumen Fluid to Improve Biomethane Yields During AD
Another approach to promote increased biomethane production from lignocellulosic wastes is through co-digestion with rumen fluid. Ince et al. (2019) added rumen fluid directly to the seed sludge in a batch fermenter, with untreated barley as the lignocellulosic substrate. Compared to AD of seed sludge and barley only, the biomethane yield was increased by 18% when rumen fluid was added (from 235 L CH4/kg VS to 278 L CH4/kg VS) and digesters were operated at 37°C, and an inoculum to substrate ratio of 1:2. Rikenella, a known cellulolytic genus, had a significantly higher abundance in digesters containing rumen fluid, while Methanobrevibacter species were the dominant methanogens. In contrast, Methanobacterium was the dominant genus in the digesters without rumen fluid. The work by Ince et al. (2019), like that of Baba et al. (2019) suggests the importance of microbial community structure during AD in the optimisation of biomethane production.
A study by Wall et al. (2015) examined co-digestion of grass silage with rumen fluid. Grass silage used in this study was at an advanced stage of growth, of low quality and high in fibre, making it very recalcitrant. The study examined two different sizes of silage particles (1 cm and 3 cm in size). Batch fermentation tests were carried out in triplicate for 30 days at 37°C in 750 mL fermenters (400 mL working volumes) at a 1:2 inoculum to substrate ratio. Interestingly, when BMP tests were carried out on the digesters, no statistically significant difference existed between any of the SMYs, with or without rumen fluid. All were 340–350 L CH4/kg VS, suggesting that the rumen fluid or silage particle size had no impact on the SMYs. The 1 cm and 3 cm silage particles with or without rumen fluid were also digested in 5 L CSTRs (4 L working volume) over a HRT of 31 days at 37°C. The OLR was maintained at 2.5 kg VS/m3/day. No impact of the addition of rumen fluid on the methane yield was observed when co-digested with 3 cm silage particles (343 and 342 L CH4/kg VS SMYs for the digesters ± rumen fluid). In contrast, the 1 cm silage particles co-digested with rumen fluid, in the semi-continuous reactor, produced a higher SMY than the reactor without rumen fluid with the same sized silage particles (371 L CH4/kg VS). This digester also had a significantly higher SMY than the corresponding predicted BMP of 350 L CH4/kg VS, and digester stability was much greater in the rumen fluid-containing digester overall. The similar SMYs between the semi-continuous reactors with 3 cm particles of silage, with and without rumen fluid, could be explained by mechanical issues, blockage of the outlet port and silage floating to the reactor surface. Overall, this study showed that rumen fluid can improve biomethane yield from a semi-continuous reactor when added as a co-substrate and can increase stability of the microbial community in the digester. It also demonstrates the difficulties in using BMP tests to predict SMYs of larger, continuous digesters, as variables like mechanical stress are not included.
Nagler et al. (2019) studied the co-digestion of rumen fluid, corn straw and cow manure (working volume 300 mL) in 500 mL batch fermenters at 37°C over 31 days. The substrate ratios in each fermenter were either 20% rumen fluid to 80% manure, 40% rumen fluid to 60% manure, 100% manure (control 1), or 100% rumen fluid (control 2), followed by the addition of 16 g/L corn straw to the test (not control) fermenters. The methane production for the fermenters after 31 days with 20 and 40% rumen fluid was 292 and 279 L CH4/kg VS, respectively. In the fermenters containing 100% manure and 100% rumen fluid, 260 and 6.1 L CH4/kg VS were produced. Therefore, the effect of rumen fluid on methane amount compared to the fermenter with cattle manure only was not significant, though as rumen fluid volume in the fermenter increased, methane formation accelerated. By day 22, 90% of the total biomethane yield was reached in the 100% manure fermenter, whereas it was achieved by day 16 and day 17 in the 40 and 20% rumen fluid-containing fermenters, respectively. After 31 days, 49, 78, 78, and 81% of hemicellulose and 50, 77, 83, and 84% of cellulose were degraded in the 100% rumen fluid, 100% manure, 20% rumen fluid, and 40% rumen fluid-containing fermenters. However, lignin was not significantly degraded in any of the fermenters, even with the long HRT of 31 days. The optimal ratio for rumen fluid to substrate in batch AD needs to be further investigated, to maximise methane yield and reduce the HRT and increase industrial feasibility.
Corn straw was also semi-continuously co-digested with rumen fluid and pig manure by Jin et al. (2018), using a CSTR at 39°C with an HRT of 36 days. First, using batch fermentation, pig manure was fermented with corn straw for 3 days at 39°C at a ratio of 1:1. This facilitated optimal VS degradation (79%) of these substrates. Then, the pre-treated substrates were added to a 4 L CSTR containing 1 L of rumen fluid and 3 L of tap water saturated with CO2 at an OLR of 1 g VS/L/day (first 27 days of HRT) or 2 g VS/L/day (last 9 days of HRT). A biogas yield of 430 L/kg VS was obtained, with 60% biomethane content. Although the pre-treatment was carried out prior to CSTR, this study concluded that the co-digestion of substrates with rumen fluid played the largest role in biomethane production, which concurs with findings by Ince et al. (2019) and Nagler et al. (2019). Furthermore, biogas production almost doubled from 1.72 to 3.4 L/kg/day when the OLR was increased to 2 g VS/L/day. Further research is needed to determine the optimal OLR of corn straw to maximise methane production and to reduce the process HRT.
Deng et al. (2017) co-digested rumen fluid with methanogenic seed sludge and rice straw in a 3 L semi-continuous digester (working volume of 2 L) over 48 days at 39°C. The control reactor did not contain the methanogenic seed sludge. The OLR was varied every 16 days during the process—for the first 16 days it was 3 g/day, 7 g/day for the next 16 days and 14 g/day for the final 16 days. The co-inoculum consisted of 20% (v/v) rumen fluid with the seed sludge, and the rice straw was added every 4 days. On day 3, the methane yield in the test reactor was 132.32 mL/day, on day 7 it was 240.53 mL/day, and on day 17 it was 357.68 mL/day. These methane yields were 1.79, 2.07, and 2.26-fold higher than the yields in the control reactor at the same stages. An increase in OLR directly correlates with biogas production in the digester, while the methane content of the biogas produced was 32–44% in the test reactor compared to 24–28% in the control reactor. According to Deng et al. (2017), increased methane content in the test reactor was due to the consumption of VFAs, produced during the hydrolytic action of rumen microbes, by methanogens in the seed sludge. The dominant methanogenic archaeal genera were Methanobrevibacter and Methanosarcina in the test reactor. A decrease in the abundance of Methanobacterium and Methanosphaera genera was noted, in agreement with the Ince et al. (2019) who identified Methanobacterium as the dominant archaeon in the digester with no rumen fluid. Co-inoculation also changed the cellulolytic bacterial composition in the test reactor, with Clostridium and Ruminococcus increasing, and some rumen bacterial genera disappearing, e.g., Fibrobacter, Bacteroides, and Acetivibrio. Therefore, the combination of rumen fluid with a methanogenic seed sludge during AD of rice straw appeared to promote synergy between cellulolytic and methanogenic microorganisms in the digester thereby increasing biomethane yield. The authors proposed that synergy occurs specifically between the Ruminococcus and Methanobrevibacter species. Similar changes in digester microbiota profiles were reported by Baba et al. (2019) (see section Using Cattle Rumen Fluid as a Pre-treatment to Improve Biomethane Yields From Lignocellulosic Wastes During AD), with both these genera establishing themselves during AD until the end of the AD process. Ince et al. (2019) also reported Methanobrevibacter as the dominant methanogen in co-digestion of rumen fluid, seed sludge and barley, while Ozbayram et al. (2017) reported the crucial role of Ruminococcaceae, amongst other bacterial families, in methane production during the co-digestion of rumen fluid with cow manure. Investigation of potential synergistic interactions between Ruminococcus and Methanobrevibacter during co-digestion of rumen fluid, methanogenic seed sludge and lignocellulosic wastes, is needed to improve the biomethane production in the digester.
Is Using Rumen Fluid as a Pre-treatment or as a Co-substrate for Lignocellulosic Wastes Prior to/During AD Economically Feasible?
The economic feasibility of using rumen fluid as a pre-treatment or co-substrate for lignocellulosic wastes in AD is uncertain, as studies to date have focused on the scientific aspects of the process rather than the economic aspects. For slaughterhouses, the rumen fluid from the dewatering of paunch contents could be filtered and used to pre-treat or co-digest paunch contents prior to/during AD rather than sending it to a wastewater treatment plant. This could prove to be economically favourable, as pre-treatments and co-substrates would not have to be sourced externally. Supplying other AD facilities with rumen fluid would also reduce the waste sent to wastewater treatment plants. However, economic feasibility for other industries would depend on variables like the storage conditions necessary to preserve rumen fluid, transportation distance and costs, the cost and installation of an anaerobic digester, and labour costs.
As mentioned previously, Takizawa et al. (2019) showed that for rumen fluid to be effectively preserved to retain most of the microbial hydrolytic activities, it should be stored at 4°C. When stored at 20°C, the hydrolytic activity of the rumen fluid on PW dramatically decreased over 7 days. Therefore, refrigeration units would be needed to maintain the hydrolytic capabilities of rumen fluid. As it is unclear, from this study, if rumen fluid hydrolytic abilities are maintained after 7 days at 4°C, further research on its hydrolytic longevity should be carried out at this temperature to establish duration of storage. Using rumen fluid as a pre-treatment or co-substrate prior to/during AD could therefore be feasible for AD facilities located close to a slaughterhouse, where frequent collection of fresh rumen fluid is feasible to avoid storage costs.
A way to reduce the costs of transporting and storing rumen fluids could be to concentrate the rumen fluid. Takizawa et al. (2020a) carried out rumen fluid flocculation with 0.7% poly-ferric sulphate to reduce the liquid volume by 77.3%, while maintaining its fibrolytic capabilities against tomato leaves. However, this method of concentration warrants further investigation to fully uncover its effects on the rumen fluid microbiota. Functional assessment of the concentrated rumen fluid in efficient degradation of alternative lignocellulosics needs to be explored.
The Economic Feasibility of Using Rumen Fluid to Pre-treat Paper Sludge Waste at an Industrial Paper Mill for Biomethane Production Through AD
A paper mill in Southern Italy, the largest in the Campania region, with a recycled paper production capacity of 20 tonnes/day, was analysed for its potential to produce biomethane for use in an existing CHP system, from rumen fluid pre-treated paper sludge. An environmental assessment carried out by Ferrara and De Feo (2021) detailed the energy inputs into the facility and amounts of electrical and thermal energy that are produced from the CHP unit on-site, as well as waste products and quantities produced by the paper production process. These energy values and waste quantities were used as the basis of the analysis in this review.
The Italian paper mill produces packaging paper. The materials used are all recycled—the cardboard and paper waste used are sourced from separated municipal solid waste. The production of recycled paper is very energy intensive, e.g., 453.76 kWh of electrical energy and 1,122.84 kWh of thermal energy per tonne, provided by a natural gas-fired CHP system. The CHP system provides surplus electrical energy (246 kWh/tonne paper produced), which is sold to the national electrical grid. When the CHP unit has maintenance downtime, electricity (26.76 kWh/tonne paper produced) is sourced from the electricity grid to power the mill (Ferrara and De Feo, 2021).
The wastes produced are mostly pulper waste and metals; pulper waste consists mainly of plastics and fibre waste. Fibre waste is also known as paper sludge, which is the lignocellulosic material and ash left over after the production of recycled paper (Takizawa et al., 2018). Per tonne of paper, 52.61 kg of paper sludge is produced, all of which is sent for landfilling or incineration (Ferrara and De Feo, 2021).
Based on the study by Takizawa et al. (2018) where paper sludge pre-treated with rumen fluid prior to batch AD yielded a 3.4 fold increase in biomethane production (section Using Cattle Rumen Fluid as a Pre-treatment to Improve Biomethane Yields From Lignocellulosic Wastes During AD), digesting pre-treated paper sludge could be a way to obtain sustainable energy for this paper mill. Assuming a total solids content of 49.3% (Takizawa et al., 2018), 52.61 kg of paper sludge waste (Ferrara and De Feo, 2021) has 25.94 kg total solids. An assumed VS content of 31.2% of the total solids (Takizawa et al., 2018), would give 8.09 VS/kg waste. Therefore, per tonne of paper produced, the potential biomethane yield could be 1.86 m3, if rumen fluid pre-treated paper sludge was anaerobically digested. Based on annual production of 7,300 tonnes of paper (20 tonnes/day), 13,578 m3 of biomethane could potentially be produced from the paper sludge waste (see Table 3). Per m3 methane, there are 37 MJ of energy potential (Ware and Power, 2016). Annual energy consumption of the paper mill is therefore 135,644.26 kWh [biogas losses included at 2.8% (Ware and Power, 2016)]. If combusted in a CHP unit with a thermal efficiency of 55% (Ferrara and De Feo, 2021), 74,604.34 kWh of thermal energy would be produced. With an electrical efficiency of 45%, 61,039.92 kWh of electrical energy could be produced from the CHP unit.
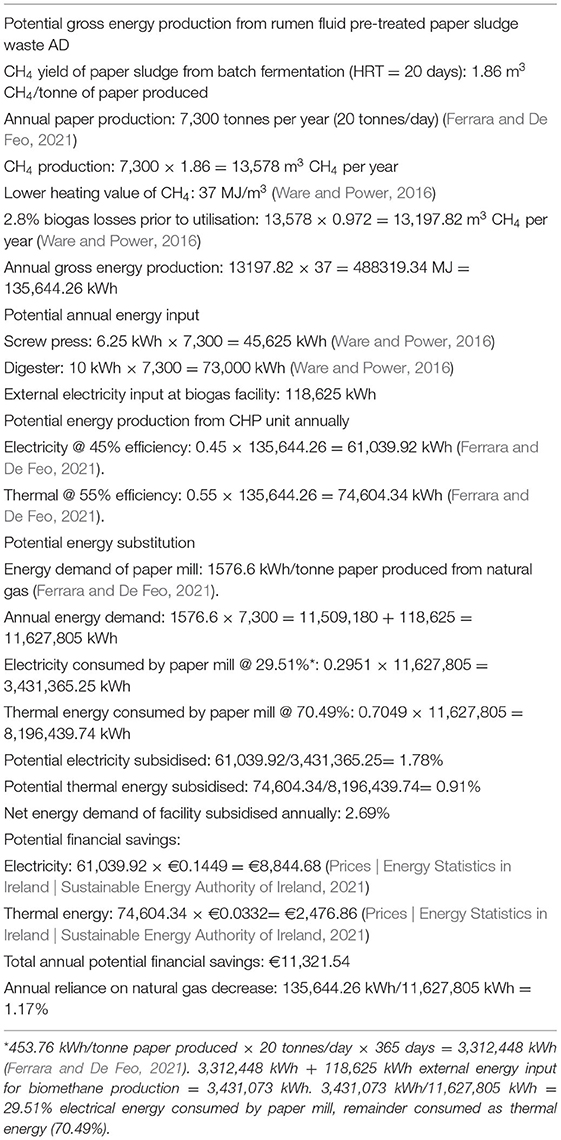
Table 3. Energy analysis of biomethane produced annually from rumen fluid pre-treated paper sludge waste available from an Italian paper mill combusted using a CHP system.
Biomethane production costs would need to be considered. For example, the energy consumption of a bioreactor is taken as 10 kWh/tonne feedstock added (Ware and Power, 2016), i.e., an annual electrical energy consumption of 73,000 kWh. A screw press to dewater the paper sludge could require 6.25 kWh electrical energy/tonne paper produced (Ware and Power, 2016), or an electrical energy consumption of 45,625 kWh annually. This leaves 118,625 kWh net electrical energy input into the biomethane production process.
Overall, 1.78 and 0.91% of electrical and thermal energy required annually by the paper mill could be supplied by AD of rumen fluid pre-treated paper sludge. In the Euro zone, 1 kWh of electricity to businesses costs on average 14.49c, while 1 kWh of gas was 3.32c (business), excluding Value Added Tax (Prices | Energy Statistics in Ireland | Sustainable Energy Authority of Ireland, 2021). Therefore, the paper mill could save ~€8,844.68 and ~€2,476.86 of electrical and thermal energy annually (see Table 3). The energy recovery from rumen fluid pre-treated paper sludge would benefit the paper mill environmentally, as it would reduce the waste output to landfill or for incineration, while reducing the annual energy required from natural gas by 1.17%. However, labour and digester maintenance costs are not considered in this analysis. Furthermore, this paper mill does not have an anaerobic digester on-site. Therefore, an initial investment of $500,000–$6,000,000 USD in a bioreactor would be required (Funding On-Farm Anaerobic Digestion EPA AgStar, 2012). Moreover, if the paper mill did not have a slaughterhouse nearby, with the current knowledge available on preservation and concentration of rumen fluid (Takizawa et al., 2019, 2020a), it may be less feasible to use rumen fluid daily both in terms of longevity of the rumen fluid and the transportation costs. If adopted, it could take time to financially profit from this system due to the large initial investment in an anaerobic digester, and relatively small annual energy savings, but co-digestion with complementary substrates, e.g., food waste could be considered to increase the biomethane yield (Lin et al., 2013).
The Rumen Microbiome
At the moment, although our knowledge of the rumen microbiome is growing, very few microorganisms from this environment have been cultured or are well-understood. To use rumen microorganisms and their enzymes to develop efficient pre-treatments, and to optimise co-digestion protocols, requires better understanding of their functions. Over the last decade, because of its exceptional ability to degrade recalcitrant material, significant research has been undertaken on the rumen microbiome, in particular, its bacterial and archaeal microbiomes. Various metagenomic studies using high-throughput sequencing of 16s and/or 18s rRNA PCR products showed that bacteria make up the vast proportion, population-wise, of the rumen microbiome (Kim et al., 2011; Henderson et al., 2015; Xing et al., 2020). In examples where the relative abundance of the phyla differ between studies, the age of the animal, diet and the DNA extraction method used are thought to underpin observed differences (Henderson et al., 2013, 2015; Jami et al., 2013). CAZymes from rumen microorganisms are the focus of this section.
Bacterial and Archaeal Carbohydrate-Active Enzymes (CAZymes) in the Rumen
The hydrolysis of complex lignocellulosic substrates is mediated by a plethora of CAZymes (and accessory proteins), with complementary and synergistic activities against polysaccharides present in a given substrate. A metagenomic sequencing study carried out by Stewart et al. (2018) resulted in the assembly of 913 draft genomes of bacteria and archaea from the 43 Scottish cattle rumens and established the predicted CAZymes present in their genomes. This study revealed very similar bacterial and archaeal relative abundances to those reported previously (Jami et al., 2013; Henderson et al., 2015; Seshadri et al., 2018). The 913 draft genomes contained almost 2,000,000 predicted proteins, of which almost 70,000 were predicted as carbohydrate-active when searched against the CAZy database. To cheque for novelty, these carbohydrate-active proteins were compared to gene predictions from Hess et al. (2011), in UniProt, TrEMBL, nr, ENV-nr, and M5nr. Only 8.7% had >95% identity to any of the sequences in these databases, meaning that almost 64,000 of the proteins were novel. Altogether, the draft genomes contained ~40,000 glycoside hydrolases, ~20,000 glycosyl transferases, ~9,000 carbohydrate esterases, ~1,000 polysaccharide lyases, ~2,500 carbohydrate-binding proteins and 154 proteins with auxiliary activities. The glycoside hydrolases and glycosyl transferases were numerous in the family Prevotellaceae and in other members of the order Bacteroidales, along with some members of the order Clostridiales. However, they were largely absent in the Proteobacteria and Archaea. All predicted carbohydrate-active proteins (except for auxiliary activities) were found to be 65–72% identical to the protein sequences publicly available at the time. The median identity of the protein sequences with auxiliary activities was about 83%. Furthermore, 15 of the 913 draft genomes encoded predicted proteins with cohesin domains, indicating that the source microbes may produce cellulosomes. Nine of the draft genomes appeared to cluster closely with R. flavefaciens, a species known to produce cellulosomes and a dominant bacterium with cellulolytic capabilities in the rumen (Bayer et al., 2004; Israeli-Ruimy et al., 2017). However, the predicted proteins were only 70–80% identical to those of R. flavefaciens, suggesting that, at the protein level, the 9 draft genomes are notably different to the Ruminococcaceae identified prior to the study by Stewart et al. (2018). Five of the 15 mapped to the order Clostridiales, while the remaining draft genome was assigned to the family Prevotellaceae. Polysaccharide-utilisation loci (1,743) were identified in 203 of the draft genomes, with 187 genomes having more than one locus. The two draft genomes with the largest number of loci (37+35), and others that had large numbers of loci, clustered with Prevotella multisaccharivorax, whose ability to use many polysaccharide substrates is known (Sakamoto et al., 2005). Beta- and alpha- glucosidases were the best represented enzymes in loci within the draft genomes, followed by beta-galactosidase. Other enzymes commonly found in these loci were arabinanases, xylanases, levanases, and enzymes involved in the degradation of mannans and pectins (Stewart et al., 2018).
A follow-on study by Stewart et al. (2019) increased the number of assembled rumen genomes to 5,845. The relative abundances of bacteria and archaea in this 2019 study remained very similar to the studies mentioned previously. However, in the Global Rumen Census by Henderson et al. (2015), a core rumen microbiome was said to be comprised of Prevotella, Butyvibrio, and Ruminococcus, along with unclassified Lachnospiraceae, Ruminococcaceae, Bacteroidales, and Clostridiales. While these species were dominant in the 2019 study, multiple abundant Proteobacteria were also identified in this dataset (and in the Rumen Census data). Therefore, the authors of the 2019 study proposed adding these Proteobacteria to the core rumen microbiome (Stewart et al., 2019).
There were 10.69 million predicted proteins in the draft genomes of the study by Stewart et al. (2019). However, none of the carbohydrate-active proteins had a mean protein identity >60%, suggesting that the CAZy database may not currently reflect the carbohydrate-active enzyme diversity present in ruminant microbe genomes. Proteins with auxiliary activities had <30% mean protein identity with CAZy database proteins. As this class includes lytic polysaccharide monooxygenases and lignin-degrading enzymes, the findings highlight the potential of the rumen to harbour novel ligninolytic enzymes and suggest the need to mine further datasets and samples to increase scientific knowledge of this multi-component, efficient microbial system for biomass pre-treatment. Such studies would identify and enhance the likelihood of future biotechnological applications.
Fungi in the Rumen: Less Well-Understood Agents of Biodegradation
While our understanding of bacteria and archaea in the rumen microbiome has expanded significantly, our understanding of fungi in the rumen, their true diversity and function as biomass degraders is comparatively less well-understood. Studies have revealed that fungi in the rumen are very important for breakdown of complex plant organic matter (i.e., cell wall) (Youssef et al., 2013; Solomon et al., 2016; Haitjema et al., 2017; Hagen et al., 2020; Stabel et al., 2020). At present, 20 genera of rumen anaerobic fungi have been isolated and described from various herbivores; all fungi belong to the Neocallimastigomycota (see Table 4; Youssef et al., 2013; Haitjema et al., 2017; Hanafy et al., 2017, 2020, 2021; Paul et al., 2018; Stabel et al., 2020). In previous studies, the potential of specific anaerobic fungi to produce lignocellulose degrading enzymes had been identified (Ljungdahl, 2008). However, using transcriptomics, it has been shown that rumen fungi produce a huge range of CAZymes, reinforcing their importance in the degradation of lignocellulose (Henske et al., 2018; Gruninger et al., 2019).
A recent study by Hagen et al. (2020) attempted to define the functional roles of rumen fungi through meta-proteomics. Air-dried switchgrass was incubated in two cannulated Holstein-Friesian cows for 48h (in nylon bags to select for lignocellulolytic enzymes). Protein and RNA extraction was carried out on the switchgrass after this period for proteomics and transcriptomics analyses. The protein profiles from the switchgrass fibre and the rumen fluid were mapped against RUS-refDB, a database containing 122 microbial genomes associated with the rumen, including bacterial metagenome-assembled genomes and isolates from bacteria, fungi and archaea, along with metagenome assembled viral scaffolds recovered from metagenome analysis (Hess et al., 2011; Parks et al., 2017). This mapping allowed the identification of 4,673 protein groups and showed a strong positive correlation between the proteins present in the samples from both Friesian cows. A large proportion of proteins detected in the meta-proteome that were of fungal origin (316–787 proteins) aligned well with predicted proteins present in the genomes of Neocallimastix californiae and Piromyces finnis. Interestingly, not many proteins mapped to both prokaryotes and fungi, despite evidence from previous studies of extensive levels of horizontal gene transfer between the microorganisms in the rumen (Haitjema et al., 2017; Murphy et al., 2019; Wang et al., 2019; Hagen et al., 2020). This suggests divergent evolution of specific proteins of potential functional significance in fungi and prokaryotes.
Fungal CAZymes appeared to be more associated with fibre-adherent samples than in the rumen fluid samples, whereas bacterial CAZyme levels did not appear to differ as significantly between the rumen fluid and fibre-adherent samples. Glycosyl hydrolases in GH48 and GH6 families accounted for the largest group of fibre-adherent proteins associated with rumen fungi in this study. GH48 family proteins include reducing-end cellobiohydrolases, endo-1,4-β-glucanases and chitinases, known to synergise with other cellulolytic enzymes in the degradation of recalcitrant cellulosic biomass (Parsiegla et al., 2008). The GH48 enzymes in this dataset were found to contain Type-II dockerin domains in tandem repeats, which suggests their presence in cellulosome complexes, and therefore their potential inclusion in enzyme complexes with multiple modules that efficiently degrade cellulose in its crystalline form. The GH48 family enzymes aligned well with predicted proteins from Orpinomyces sp., as did proteins from families GH1, GH3, GH5_1 and GH6. Protein sequences of xylanases belonging to the GH43 family were also well-aligned to the reference fungal genomes. Interestingly, no polysaccharide lyase proteins were detected in the meta-proteome, although the reference database contained 314 genes encoding GH43 members. No enzymes that play a key role in lignin degradation were found in the metaproteome. Degradation of their substrates (lignin and pectin) occurs later on in the digestion process, i.e., beyond the 48 h sampling point (Hagen et al., 2020).
Rumen fungi also degrade the sclerenchyma, which are the hardest cell wall tissues, to a greater degree than rumen bacteria. Fungal rhizoids can penetrate cell walls, which also facilitates access by other lignocellulolytic microbes like bacteria into the cell wall thereby promoting further cell wall degradation. So far, as mentioned earlier, there are only 20 cultured genera of fungi from the rumen, many of which have only been cultured in recent years (Table 4). Clearly, there is much work to be done, on the identification and culturing of rumen fungi, as information from earlier studies may have been limited by the paucity of sequence information and tools to identify new genera and species. Furthermore, it is important to elucidate the arsenal of enzymes and accessory proteins rumen fungi produce for lignocellulose degradation, both in isolated cultures and in co-cultures with other rumen microorganisms, using functional proteomics approaches. In this way, we can begin to fully understand the true importance and contribution of these important eukaryotic microorganisms to the degradation of the most recalcitrant fractions of grass (Akin et al., 1983; Grenet et al., 1989; Paul et al., 2018).
Discussion and Future Perspectives
It is clear that paunch contents are a viable option as a source of sustainable energy. Not only would the widespread use of paunch contents in AD reduce slaughterhouses energy costs, but it would help to create a circular economy within slaughterhouses. In an Irish context, this would have the potential to increase Ireland's Renewable Energy Shares (Ware and Power, 2016). If AD of paunch contents was to become more efficient in terms of biomethane production and better utilisation of the substrate, the slaughterhouses could potentially profit by contributing excess biomethane into the national gas grid. Ways to increase the biomethane yield of paunch contents discussed in this review include the development of a suitable pre-treatment prior to AD, or the development of a co-digestion protocol with rumen fluid which could reduce the recalcitrance of the lignocellulose-rich paunch contents (Nkemka et al., 2015). This review also discusses the uses of rumen fluid as a pre-treatment or co-substrate prior to/during AD for biomethane production from alternative lignocellulosic wastes. The approach of using the entire rumen fluid microbiome to improve biomethane yield from AD is promising but needs optimisation. The study of a paper mill in this review (section Rumen Fluid From Cattle as a Source of Lignocellulolytic Enzymes for AD) showed that utilising rumen fluid pre-treated paper sludge in AD has the potential to reduce the paper mill's carbon footprint by reducing annual natural gas usage. Approaches to increase the biomethane yield from this process should be investigated. It may be possible to develop enzymatic pre-treatments derived from rumen microorganisms, as the rumen microbiome is an extremely efficient degrader of lignocellulose (sections Pre-treatment of Paunch Contents Prior to AD, Rumen Fluid From Cattle as a Source of Lignocellulolytic Enzymes for AD, Using Cattle Rumen Fluid as a Pre-treatment to Improve Biomethane Yields From Lignocellulosic Wastes During AD, Co-digestion of Lignocellulosic Wastes With Cattle Rumen Fluid to Improve Biomethane Yields During AD, Is Using Rumen Fluid as a Pre-treatment or as a Co-substrate for Lignocellulosic Wastes Prior to/During AD Economically Feasible?, The Economic Feasibility of Using Rumen Fluid to Pre-treat Paper Sludge Waste at an Industrial Paper Mill for Biomethane Production Through AD, The Rumen Microbiome), to also degrade other lignocellulose-rich wastes. However, before this is possible, many more rumen microorganisms must be cultured, their CAZymes studied in detail using proteomics, their potential synergies investigated, and the applicability of these cell factories as an enabling technology investigated further. As highlighted, much remains to be done to achieve this goal, primarily due to the difficulties in culturing anaerobic microorganisms. It is clear also that more bio-discovery studies are required, as to-date an overwhelming focus has been placed on studying the bacterial and archaeal populations in the rumen, even though it has been shown that fungi are responsible for degrading the most recalcitrant fraction of the grass (section The Rumen Microbiome). As many rumen microorganisms are yet to be cultured and studied in detail, novel microorganisms from environments other than the rumen could also be used to prepare inexpensive and effective pre-treatments for paunch contents. For example, alkali- and thermotolerant xylanases were discovered in marine fungi (Raghukumar et al., 2004). Therefore, their enzymes could be used with NaOH or thermal pre-treatments, in a combined biological and chemical pre-treatment strategy. Overall, further research into potential pre-treatments or combinations of pre-treatments, and approaches to utilise rumen microorganisms (especially fungi) is needed to make the AD of valuable resources like paunch contents and other lignocellulosic wastes cost and energy efficient.
Author Contributions
BD drafted the manuscript with contributions from MT and DM. BD and MT revised the manuscript and prepared tables. DM provided important national and global industry perspectives on the current state of the art in relation to the utilisation of the waste. All authors contributed to the article and approved the submitted version.
Funding
This research was funded by Science Foundation Ireland (SFI) through MaREI, the SFI Research Centre for Energy, Climate, and Marine (Grant No: 12/RC/2302_16/SP/3829), with supporting funding obtained from the ABP Food Group. ABP Food Group was not involved in the study design, collection, analysis, interpretation of data, the writing of this article or the decision to submit it for publication.
Conflict of Interest
DM was employed by Anglo Beef Processors Ireland.
The remaining authors declare that the research was conducted in the absence of any commercial or financial relationships that could be construed as a potential conflict of interest.
Publisher's Note
All claims expressed in this article are solely those of the authors and do not necessarily represent those of their affiliated organizations, or those of the publisher, the editors and the reviewers. Any product that may be evaluated in this article, or claim that may be made by its manufacturer, is not guaranteed or endorsed by the publisher.
Acknowledgments
The authors are thankful to the funders of this project, the Science Foundation of Ireland and ABP Food Group, and to colleagues in the Sustainable Energy and Fuel Efficiency (SEFE) Spoke, MaREI, and Energy research clusters in the Ryan Institute, NUI Galway.
Supplementary Material
The Supplementary Material for this article can be found online at: https://www.frontiersin.org/articles/10.3389/fsufs.2022.722424/full#supplementary-material
References
A Resource Opportunity- Waste Management Policy in Ireland (2012). Available online at: https://www.gov.ie/en/publication/a9d98-a-resource-opportunity-waste-management-policy-in-ireland/ (accessed May 4, 2020).
Abdeshahian, P., Kadier, A., Rai, P. K., and da Silva, S. S. (2020). “Lignocellulose as a renewable carbon source for microbial synthesis of different enzymes,” in Lignocellulosic Biorefining Technologies, eds A. P. Ingle, A. K. Chandel, and S. S. Silva (West Sussex, UK: Wiley-Blackwell), 185–202.
Abouheif, M. A., Kraidees, M. S., and Al-Selbood, B. A. (1999). The utilization of rumen content-barley meal in diets of growing lambs. Asian Australas. J. Anim. Sci. 12, 1234–1240. doi: 10.5713/ajas.1999.1234
Aganga, A. A., Omphile, U. J., Thema, T., and Wilson, L. Z. (2004). Chemical composition of ryegrass (Lolium multiflorum) at different stages of growth and ryegrass silages with additives. J. Biol. Sci. 4, 645–649. doi: 10.3923/jbs.2004.645.649
Akin, D. E., Gordon, G. L. R., and Hogan, J. P. (1983). Rumen bacterial and fungal degradation of digitaria pentzii grown with or without sulfur. Appl. Environ. Microbiol. 46, 738–748.
Alende, M., Fluck, A. C., Volpi-Lagreca, G., and Andrae, J. G. (2020). Chemical composition and in vitro digestibility of annual ryegrass varieties grown in greenhouse conditions. Rev. de Investig. Agropecu. 46, 50–55. Available online at: https://www.redalyc.org/journal/864/86463754005/html/
Antille, D. L., O'Sullivan, K. C., McCabe, B. K., Marchuk, S., Eberhard, J., Lee, S., et al. (2019). Long-term land application of slaughterhouse cattle paunch effects on soil properties. ASABE 2019 Annu. Int. Meet. 190004. doi: 10.13031/aim.201900047
Astals, S., Batstone, D. J., Mata-Alvarez, J., and Jensen, P. D. (2014). Identification of synergistic impacts during anaerobic co-digestion of organic wastes. Bioresour. Technol. 169, 421–427. doi: 10.1016/j.biortech.2014.07.024
Baba, Y., Matsuki, Y., Mori, Y., Suyama, Y., Tada, C., Fukuda, Y., et al. (2017). Pretreatment of lignocellulosic biomass by cattle rumen fluid for methane production: bacterial flora and enzyme activity analysis. J. Biosci. Bioeng. 123, 489–496. doi: 10.1016/j.jbiosc.2016.11.008
Baba, Y., Matsuki, Y., Takizawa, S., Suyama, Y., Tada, C., Fukuda, Y., et al. (2019). Pretreatment of lignocellulosic biomass with cattle rumen fluid for methane production: fate of added rumen microbes and indigenous microbes of methane seed sludge. Microbes Environ. 34, 421–428. doi: 10.1264/jsme2.ME19113
Baba, Y., Tada, C., Fukuda, Y., and Nakai, Y. (2013). Improvement of methane production from waste paper by pretreatment with rumen fluid. Biores. Tech. 128, 94–99. doi: 10.1016/j.biortech.2012.09.077
Banks, C. J., and Wang, Z. (1999). Development of a two phase anaerobic digester for the treatment of mixed abattoir wastes. Water Sci. Technol. 40, 69–76. doi: 10.2166/wst.1999.0016
Barr, D. J. S., Kudo, H., Jakober, K. D., and Cheng, K.-J. (1989). Morphology and development of rumen fungi: Neocallimastix sp., Piromyces communis, and Orpinomyces bovis gen.nov., sp.nov. Can. J. Bot. 67, 2815–2824. doi: 10.1139/b89-361
BAT Documents (BREFs) / Commission Implementing Decisions - Environmental Protection Agency Ireland. (2021). Available online at: https://www.epa.ie/licensing/industrialemissionslicensing/brefcid/ (accessed April 27, 2021).
BAT Guidance Note on Best Available Techniques for the Slaughtering Sector (2008). Environmental Protection Agency, Ireland.
Bauman, D. E., Davis, C. L., Frobish, R. A., and Sachan, D. S. (1971). Evaluation of polyethylene glycol method in determining rumen fluid volume in dairy cows fed different diets. J. Dairy Sci. 54, 928–930. doi: 10.3168/jds.S0022-0302(71)85947-7
Baumann, D. J. (1971). Elimination of Water Pollution by Packinghouse Animal Paunch and Blood. Available online at: https://nepis.epa.gov/
Bayer, E. A., Belaich, J. P., Shoham, Y., and Lamed, R. (2004). The cellulosomes: multienzyme machines for degradation of plant cell wall polysaccharides. Annu. Rev. Microbiol. 58, 521–554. doi: 10.1146/annurev.micro.57.030502.091022
Bertram, C., Luderer, G., Creutzig, F., Bauer, N., Ueckerdt, F., Malik, A., et al. (2021). COVID-19-induced low power demand and market forces starkly reduce CO2 emissions. Nat. Clim. Chang. 11, 193–196. doi: 10.1038/s41558-021-00987-x
Bishop, G. C., Burns, R. T., Shepherd, T. A., Moody, L. B., and Gooch, C. A. (2009). “Evaluation of laboratory biochemical methane potentials as a predictor of anaerobic dairy manure digester biogas and methane production,” in Proceedings of the 2009 ASABE International Meeting (Reno, NV).
Breton, A. (1989). Morphological and metabolic characterization of a new species of strictly anaerobic rumen fungus: Neocallimastix joyonii. FEMS Microbiol. Lett. 58, 309–314. doi: 10.1111/j.1574-6968.1989.tb03065.x
Breton, A., Bernalier, A., Dusser, M., Fonty, G., Gaillard-Martinie, B., and Guitlot, J. (1990). Anaeromyces mucronatus nov. gen., nov. sp. A new strictly anaerobic rumen fungus with polycentric thallus. FEMS Microbiol. Lett. 70, 177–182. doi: 10.1111/j.1574-6968.1990.tb13974.x
Breton, A., Dusser, M., Rd-Martinie, B. G., Guillot, J., Millet, L., and Prensier, G. (1991). Piromyces rhizinflata nov. sp., a strictly anaerobic fungus from faeces of the Saharian ass: a morphological, metabolic and ultrastructural study. FEMS Microbiol. Lett. 82, 1–8. doi: 10.1111/j.1574-6968.1991.tb04830.x
Bridle, T. (2011). Use of Paunch Waste as a Boiler Fuel. MLA. Available online at: https://www.mla.com.au/research-and-development/reports/2011/paunch-as-boiler-fuel/
Bridle, T. (2012). Use of Dewatered Paunch Waste and DAF Sludge as a Boiler Fuel. MLA. Available online at: https://www.mla.com.au/contentassets/6033621c2d824173abdb2fc720f88a9e/a.env.0106_paunch_waste_final_report.pdf
Browne, J. D., Allen, E., and Murphy, J. D. (2013). Evaluation of the biomethane potential from multiple waste streams for a proposed community scale anaerobic digester. Environ. Technol. 34, 2027–2038. doi: 10.1080/09593330.2013.812669
Buendía, I. M., Fernández, F. J., Villaseñor, J., and Rodríguez, L. (2009). Feasibility of anaerobic co-digestion as a treatment option of meat industry wastes. Bioresour. Technol. 100, 1903–1909. doi: 10.1016/j.biortech.2008.10.013
Burns, G. A., O'Kiely, P., Grogan, D., Watson, S., and Gilliland, T. J. (2015). Comparison of herbage yield, nutritive value and ensilability traits of three ryegrass species evaluated for the Irish Recommended List. Irish J. Agric. Food Res. 54, 31–40. doi: 10.1515/ijafr-2015-0003
Callaghan, T. M., Podmirseg, S. M., Hohlweck, D., Edwards, J. E., Puniya, A. K., Dagar, S. S., et al. (2015). Buwchfawromyces eastonii gen. nov., sp. nov.: a new anaerobic fungus (Neocallimastigomycota) isolated from buffalo faeces. MycoKeys 9, 11–28. doi: 10.3897/mycokeys.9.9032
Cammell, S. B., Thomson, D. J., Beever, D. E., Haines, M. J., Dhanoa, M. S., and Spooner, M. C. (1986). The efficiency of energy utilization in growing cattle consuming fresh perennial ryegrass (Lolium perenne cv. Melle) or white clover (Trifolium repens cv. Blanca). Br. J. Nutr. 55, 669–680. doi: 10.1079/BJN19860073
Chaves, A. V. (2003). Digestion Characteristics of Forages, Including Perennial Ryegrass at Different Stages of Maturity, and Supplementary Feeding for Dairy Cows Grazing Pasture. Available online at: http://hdl.handle.net/10179/1160 (accessed June 4, 2020).
Chen, Y.-C., Hseu, R.-S., and Chien, C.-Y. (2002). Piromyces polycephalus (Neocallimastigaceae), a new rumen fungus. Nova Hedwigia 75, 409–414. doi: 10.1127/0029-5035/2002/0075-0409
Chen, Y.-C., Tsai, S.-D., Cheng, H.-L., Chien, C.-Y., Hu, C.-Y., and Cheng, T.-Y. (2007). Caecomyces sympodialis sp nov., a new rumen fungus isolated from Bos indicus. Mycologia 99, 125–130. doi: 10.1080/15572536.2007.11832607
CO2 Emissions | Energy Statistics in Ireland | Sustainable Energy Authority of Ireland (2020-2022). Available online at: https://www.seai.ie/data-and-insights/seai-statistics/key-statistics/co2/
Crush, J. R. M., Evans, J. P., and Cosgrove, G. P. (1989). Chemical composition of ryegrass (Lolium perenne L.) and prairie grass (Bromus willdenowii Kunth) pastures. N. Zeal. J. Agric. Res. 32, 461–468. doi: 10.1080/00288233.1989.10417918
Dagar, S. S., Kumar, S., Griffith, G. W., Edwards, J. E., Callaghan, T. M., Singh, R., et al. (2015). A new anaerobic fungus (Oontomyces anksri gen. nov., sp. nov.) from the digestive tract of the Indian camel (Camelus dromedarius). Fungal Biol. 119, 731–737. doi: 10.1016/j.funbio.2015.04.005
Dai, X., Li, X., Zhang, D., Chen, Y., and Dai, L. (2016). Simultaneous enhancement of methane production and methane content in biogas from waste activated sludge and perennial ryegrass anaerobic co-digestion: the effects of pH and C/N ratio. Bioresour. Technol. 216, 323–330. doi: 10.1016/j.biortech.2016.05.100
Deng, Y., Huang, Z., Ruan, W., Zhao, M., Miao, H., and Ren, H. (2017). Co-inoculation of cellulolytic rumen bacteria with methanogenic sludge to enhance methanogenesis of rice straw. Int. Biodeterior. Biodegrad. 117, 224–235. doi: 10.1016/j.ibiod.2017.01.017
Directive 2010/75/EU (2010). Directive 2010/75/EU of the European Parliament and of the Council of 24 November 2010 on Industrial Emissions. Integrated Pollution Prevention and Control, Recast, Brussels, Belgium.
dos Santos Ferreira, J., de Oliveira, D., Maldonado, R. R., Kamimura, E. S., and Furigo, A. (2020). Enzymatic pretreatment and anaerobic co-digestion as a new technology to high-methane production. Appl. Microbiol. Biotechnol. 104, 4235–4246. doi: 10.1007/s00253-020-10526-x
dos Santos, S. K., Falbo, M. K., Sandini, I. E., Ishiy, L. E., Deliberalli, A., and Dal Posso, L. D. (2017). Ryegrass pasture supplementation strategies for lactating ewe performance. Turkish J. Vet. Anim. Sci. 41, 787–792. doi: 10.3906/vet-1703-86
Edström, M., Nordberg, Å., and Thyselius, L. (2003). Anaerobic treatment of animal by-products from Slaughterhouses at laboratory and pilot scale. Appl. Biochem. Biotechnol. 109, 127–138. doi: 10.1385/ABAB:109:1-3:127
Electricity Prices Around the World (2021). GlobalPetrolPrices.com. Available online at: https://www.globalpetrolprices.com/electricity_prices/ (accessed August 18, 2021).
Energy Use Overview | Energy Statistics in Ireland | Sustainable Energy Authority of Ireland (2021). Available online at: https://www.seai.ie/data-and-insights/seai-statistics/key-statistics/energy-use-overview/ (accessed August 16, 2021).
Environmental Protection Agency (EPA) Ireland. (2021). Annual Environmental Reports (2021). Environmental Protection Agency, Ireland. Available online at: https://www.epa.ie/our-services/compliance-enforcement/whats-happening/annual-environmental-reports
EPA Tasmania. (2017). Paunch Contents Land Spreading Management Guidelines (2017). EPA Tasmania. Available online at: https://epa.tas.gov.au/Documents/Paunch%20Contents%20Management%20Guidelines%20March%202017.pdf
Estell, R. E., and Galyean, M. L. (1985). Relationship of rumen fluid dilution rate to rumen fermentation and dietary characteristics of beef steers. J. Anim. Sci. 60, 1061–1071. doi: 10.2527/jas1985.6041061x
Eurostat (2020). Available online at: https://appsso.eurostat.ec.europa.eu/nui/submitViewTableAction.do (accessed April 14, 2020).
FAOSTAT (2020). Available online at: http://www.fao.org/faostat/en/?#data/QL (accessed April 28, 2021).
Fawzy, S., Osman, A. I., Doran, J., and Rooney, D. W. (2020). Strategies for mitigation of climate change: a review. Environ. Chem. Lett. 18, 2069–2094. doi: 10.1007/s10311-020-01059-w
Ferrara, C., and De Feo, G. (2021). Environmental assessment of the recycled paper production: the effects of energy supply source. Sustainability 13, 4841. doi: 10.3390/su13094841
Fowler, P., Mclauchlin, A., and Hall, L. M. (2003). The Potential Industrial Uses of Forage Grasses Including Miscanthus. Available online at: https://www.researchgate.net/publication/251515059 (accessed June 9, 2020).
Funding On-Farm Anaerobic Digestion EPA AgStar (2012). Available online at: https://www.epa.gov/agstar/funding-farm-anaerobic-digestion (accessed January 29, 2022).
Gaillard-Martinie, B., Breton, A., Dusser, M., and Julliand', V. (1995). Piromyces citronii sp. nov., a strictly anaerobic fungus from the equine caecum: a morphological, metabolic, and ultrastructural study. FEMS Microbiol. Lett. 130, 321–326. doi: 10.1111/j.1574-6968.1995.tb07738.x
Garcia, T. J., Brady, J. A., Guay, K. A., Muir, J. P., and Smith, W. B. (2019). Reduce Reuse ReRumen: variability in nutritive value of paunch manure. J. Anim. Sci. 97, 66–67. doi: 10.1093/jas/skz053.150
Garnett, T. (2007). Meat and Dairy Production and Consumption- Exploring the livestock sector's contribution to the UK's greenhouse gas emissions and assessing what less greenhouse gas intensive systems of production and consumption might look like. Food Climate Research Network.
Gold, J. J., Brent Heath, I., and Bauchop, T. (1988). Ultrastructural description of a new chytrid genus of caecum anaerobe, Caecomyces equi gen. nov., sp. nov., assigned to the Neocallimasticaceae. BioSystems 21, 403–415. doi: 10.1016/0303-2647(88)90039-1
Grenet, E., Breton, A., Barry, P., and Fonty, G. (1989). Rumen anaerobic fungi and plant substrate colonization as affected by diet composition. Anim. Feed Sci. Technol. 26, 55–70. doi: 10.1016/0377-8401(89)90006-0
Gruninger, R. J., Ribeiro, G. O., Cameron, A., and Mcallister, T. A. (2019). Invited review: application of meta-omics to understand the dynamic nature of the rumen microbiome and how it responds to diet in ruminants. Animal 13, 1843–1854. doi: 10.1017/S1751731119000752
Hagen, L. H., Brooke, C. G., Shaw, C. A., Norbeck, A. D., Piao, H., Arntzen, M. Ø., et al. (2020). Proteome specialization of anaerobic fungi during ruminal degradation of recalcitrant plant fiber. ISME J. 15, 421–434. doi: 10.1038/s41396-020-00769-x
Haitjema, C. H., Gilmore, S. P., Henske, J. K., Solomon, K. v., de Groot, R., et al. (2017). A parts list for fungal cellulosomes revealed by comparative genomics. Nat. Microbiol. Lett. 2, 1–8. doi: 10.1038/nmicrobiol.2017.87
Hamawand, I., Pittaway, P., Lewis, L., Chakrabarty, S., Caldwell, J., Eberhard, J., et al. (2017). Waste management in the meat processing industry: conversion of paunch and DAF sludge into solid fuel. J. Waste Manag. 60, 340–350. doi: 10.1016/j.wasman.2016.11.034
Hanafy, R. A., Elshahed, M. S., Liggenstoffer, A. S., Griffith, G. W., and Youssef, N. H. (2017). Pecoramyces ruminantium, gen. nov., sp. nov., an anaerobic gut fungus from the feces of cattle and sheep. Mycologia 109, 231–243. doi: 10.1080/00275514.2017.1317190
Hanafy, R. A., Elshahed, M. S., and Youssef, N. H. (2018). Feramyces austinii, gen. Nov., sp. nov., an anaerobic gut fungus from rumen and fecal samples of wild barbary sheep and fallow deer. Mycologia 110, 513–525. doi: 10.1080/00275514.2018.1466610
Hanafy, R. A., Lanjekar, V. B., Dhakephalkar, P. K., Callaghan, T. M., Dagar, S. S., Griffith, G. W., et al. (2020). Seven new Neocallimastigomycota genera from wild, zoo-housed, and domesticated herbivores greatly expand the taxonomic diversity of the phylum. Mycologia 112, 1212–1239. doi: 10.1080/00275514.2019.1696619
Hanafy, R. A., Youssef, N. H., and Elshahed, M. S. (2021). Paucimyces polynucleatus gen. Nov, sp. nov., a novel polycentric genus of anaerobic gut fungi from the faeces of a wild blackbuck antelope. Int. J. Syst. Evol. Microbiol. 71:004832. doi: 10.1099/ijsem.0.004832
Hannaway, D., Fransen, S., Cropper, J., Teel, M., Chaney, M., Griggs, T., et al. (1999). Perennial ryegrass (Lolium perenne L.). PNW. 503, 1–20. Available online at: https://www.researchgate.net/publication/45793996
Hatt, R. (1997). Moisture Impacts on Coal Handling and Heat Rate or, as published by World Coal, “Sticky When Wet.” World Coal. Available online at: https://www.worldcoal.org/
Henderson, G., Cox, F., Ganesh, S., Jonker, A., Young, W., Janssen, P. H., et al. (2015). Rumen microbial community composition varies with diet and host, but a core microbiome is found across a wide geographical range. Sci. Rep. 5, 1–13. doi: 10.1038/srep14567
Henderson, G., Cox, F., Kittelmann, S., Miri, V. H., and Zethof, M. (2013). Effect of DNA extraction methods and sampling techniques on the apparent structure of cow and sheep rumen microbial communities effect of DNA extraction methods and sampling techniques on the apparent structure of cow and sheep rumen microbial communities. PLoS ONE 8, 74787. doi: 10.1371/journal.pone.0074787
Henske, J. K., Wilken, S. E., Solomon, K. v., Smallwood, C. R., Shutthanandan, V., Evans, J. E., et al. (2018). Metabolic characterization of anaerobic fungi provides a path forward for bioprocessing of crude lignocellulose. Biotechnol. Bioeng. 115, 874–884. doi: 10.1002/bit.26515
Hess, M., Sczyrba, A., Egan, R., Kim, T.-W., Chokhawala, H., Schroth, G., et al. (2011). Metagenomic discovery of biomass-degrading genes and genomes from cow rumen. Science 331, 463–467. doi: 10.1126/science.1200387
Ho, Y. W., and Barr, D. J. S. (1995). Classification of anaerobic gut fungi from herbivores with emphasis on rumen fungi from Malaysia. Mycologia 87, 655–677. doi: 10.2307/3760810
Ho, Y. W., Barr, D. J. S., Abdullah, N., Jalaludin, S., and Kudo, H. (1993). Neocallimastix variabilis, a new species of anaerobic fungus from the rumen of cattle. Mycotaxon 46, 241–258.
Ho, Y. W., Bauchop, T., Abdullah, N., and Jalaludin, S. (1990). Ruminomyces elegans gen. et sp. nov., a polycentric anaerobic rumen fungus from cattle. Mycotaxon 38, 397–405.
Hopkins, C. (2003). The nutritive value of Italian ryegrass (Lolium multiflorum) selected for high dry matter and nonstructural carbohydrate contents (Doctor of Philosophy thesis). University of Natal, Natal, South Africa.
Hosseini Koupaie, E., Dahadha, S., Bazyar Lakeh, A. A., Azizi, A., and Elbeshbishy, E. (2019). Enzymatic pretreatment of lignocellulosic biomass for enhanced biomethane production- a review. J. Environ. Manage. 233, 774–784. doi: 10.1016/j.jenvman.2018.09.106
Hui, Y. H., Nip, W.-K., Rogers, R. W., and Young, O. A. (2001). “Waste management,” in Meat Science and Applications, eds Y.-H. Hui, W.-K. Nip, R. W. Rogers, and O. A. Young (New York, NY; Basel: Marcel Dekker Inc.), 645–645.
Ince, O., Akyol, Ç., Ozbayram, E. G., Tutal, B., and Ince, B. (2019). Enhancing methane production from anaerobic co-digestion of cow manure and barley: link between process parameters and microbial community dynamics. Environ. Prog. Sustain. Energy 39, e13292. doi: 10.1002/ep.13292
Integrated Pollution Prevention Control Reference Document on Best Available Techniques in the Slaughterhouses Animal by-products Industries (2005). European Commission. Available online at: https://eippcb.jrc.ec.europa.eu/
Israeli-Ruimy, V., Bule, P., Jindou, S., Dassa, B., Moraïs, S., Borovok, I., et al. (2017). Complexity of the Ruminococcus flavefaciens FD-1 cellulosome reflects an expansion of family-related protein-protein interactions. Nat. Sci. Rep. 7:42355. doi: 10.1038/srep42355
Jami, E., Israel, A., Kotser, A., and Mizrahi, I. (2013). Exploring the bovine rumen bacterial community from birth to adulthood. ISME J. 7, 1069–1079. doi: 10.1038/ismej.2013.2
Jensen, P. (2013). Anaerobic Co-Digestion of Paunch and DAF Sludge. Available online at: https://www.mla.com.au/contentassets/f90fc3cc14634fcf92184042d54a4ea1/a.env.0155_final_report.pdf.
Jensen, P. D., Mehta, C. M., Carney, C., and Batstone, D. J. (2016). Recovery of energy and nutrient resources from cattle paunch waste using temperature phased anaerobic digestion. J. Waste Manag. 51, 72–80. doi: 10.1016/j.wasman.2016.02.039
Jin, W., Xu, X., Yang, F., Li, C., and Zhou, M. (2018). Performance enhancement by rumen cultures in anaerobic co-digestion of corn straw with pig manure. Biomass Bioenergy 115, 120–129. doi: 10.1016/j.biombioe.2018.05.001
Joshi, A., Lanjekar, V. B., Dhakephalkar, P. K., Callaghan, T. M., Griffith, G. W., and Singh Dagar, S. (2018). Liebetanzomyces polymorphus gen. sp. nov., a new anaerobic fungus (Neocallimastigomycota) isolated from the rumen of a goat. MycoKeys 40, 89–110. doi: 10.3897/mycokeys.40.28337
Karp, E. M., Resch, M. G., Donohoe, B. S., Ciesielski, P. N., O'brien, M. H., Nill, J. E., et al. (2015). Alkaline Pretreatment of Switchgrass. ACS Sustain. Chem. Eng. 3, 1479–1491. doi: 10.1021/acssuschemeng.5b00201
Kataria, R., and Ghosh, S. (2014). NaOH pretreatment and enzymatic hydrolysis of Saccharum spontaneum for reducing sugars production. Energ. Source Part A 36, 1028–1035. doi: 10.1080/15567036.2010.551268
Keating, T., and O'Kiely, P. (2000). Comparison of old permanent grassland, Lolium perenne and Lolium multiflorum swards grown for silage: 1. Effects on beef production per hectare. Irish J. Agric. Food Res. 39, 1–24. Available online at: http://www.jstor.org/stable/25562369
Kim, M., Morrison, M., and Yu, Z. (2011). Status of the phylogenetic diversity census of ruminal microbiomes. FEMS Microbiol. Ecol. 76, 49–63. doi: 10.1111/j.1574-6941.2010.01029.x
Li, F., Zhang, P., Zhang, G., Tang, X., Wang, S., and Jin, S. (2017a). Enhancement of corn stover hydrolysis with rumen fluid pretreatment at different solid contents: effect, structural changes and enzymes participation. Int. Biodeterior. Biodegrad. 119, 405–412. doi: 10.1016/j.ibiod.2016.10.038
Li, J., Heath, I. B., and Bauchop, T. (1990). Piromyces mae and Piromyces dumbonica, two new species of uniflagellate anaerobic chytridiomycete fungi from the hindgut of the horse and elephant. Can. J. Bot. 68, 1021–1033. doi: 10.1139/b90-129
Li, K., Zhu, H., Zhang, Y., and Zhang, H. (2017b). Characterization of the microbial communities in rumen fluid inoculated reactors for the biogas digestion of wheat straw. Sustainability 9, 243. doi: 10.3390/su9020243
Lin, Y., Wu, S., and Wang, D. (2013). Hydrogen-methane production from pulp & paper sludge and food waste by mesophilic-thermophilic anaerobic co-digestion. Int. J. Hydrogen Energy 38, 15055–15062. doi: 10.1016/j.ijhydene.2012.01.051
Ljungdahl, L. G. (2008) The cellulase/hemicellulase system of the anaerobic fungus Orpinomyces PC-2 and aspects of its applied use. Ann. N. Y. Acad. Sci. 1125, 308–321. doi: 10.1196/annals.1419.030.
Ló Pez, I., Passeggi, M., and Borzacconi, L. (2006). Co-digestion of ruminal content and blood from slaughterhouse industries: influence of solid concentration and ammonium generation. Water Sci. Technol. 54, 231–236. doi: 10.2166/wst.2006.510
Mackenzie, D. J., and Wylam, C. B. (1957). Analytical studies on the carbohydrates of grasses and clovers. VIII. Changes in carbohydrate composition during the growth of perennial rye-grass. J. Sci. Food Agric. 45, 343. doi: 10.1002/jsfa.2740080107
Marzbali, M. H., Kundu, S., Patel, S., Halder, P., Paz-Ferreiro, J., Madapusi, S., et al. (2021). Hydrothermal carbonisation of raw and dewatered paunch waste: experimental observations, process modelling and techno-economic analysis. Energy Convers. Manag. 24, 114631. doi: 10.1016/j.enconman.2021.114631
McEniry, J., and O'Kiely, P. (2013). Anaerobic methane production from five common grassland species at sequential stages of maturity. Bioresour. Technol. 127, 143–150. doi: 10.1016/j.biortech.2012.09.084
Meeske, R., Botha, P. R., van der Merwe, G. D., Greyling, J. F., Hopkins, C., and Marais, J. P. (2009). Milk production potential of two ryegrass cultivars with different total non-structural carbohydrate contents. S. Afr. J. Anim. Sci. 39, 15–21. doi: 10.4314/sajas.v39i1.43541
Mehta, C. M., Jensen, P. D., and Batstone, D. J. (2015). “Pilot scale resource recovery from cattle Paunch Waste,” in IWA International Symposium on Waste Management Problems in Agro-Industries. Available online at: https://espace.library.uq.edu.au/
Moloney, A. P., Mchugh, T. V., and Moloney, B. C. (1993). Volume of liquid in the rumen of friesian steers offered diets based on grass silage. Irish J. Agric. Food Res. 33, 133–145.
Murphy, C. L., Youssef, N. H., Hanafy, R. A., Couger, M. B., Stajich, J. E., Wang, Y., et al. (2019). Horizontal gene transfer as an indispensable driver for evolution of neocallimastigomycota into a distinct gut-dwelling fungal lineage. Appl. Environ. Microbiol. 85, 1–22. doi: 10.1101/487215
Murphy, J. D., Korres, N. E., Singh, A., Smyth, B., Nizami, A.-S., and Thamsiriroj, T. (2011). The Potential for Grass Biomethane as a Biofuel. EPA. Available online at: https://www.epa.ie/publications/research/climate-change/ccrp-11—the-potential-for-grass-biomethane-as-a-biofuel.php
Nagler, M., Kozjek, K., Etemadi, M., Insam, H., and Podmirseg, S. M. (2019). Simple yet effective: microbial and biotechnological benefits of rumen liquid addition to lignocellulose-degrading biogas plants. J. Biotechnol. 300, 1–10. doi: 10.1016/j.jbiotec.2019.05.004
Nkemka, V. N., and Hao, X. (2018). Start-up of a sequential dry anaerobic digestion of paunch under psychrophilic and mesophilic temperatures. J. Waste Manag. 74, 144–149. doi: 10.1016/j.wasman.2016.06.022
Nkemka, V. N., Marchbank, D. H., and Hao, X. (2015). Anaerobic digestion of paunch in a CSTR for renewable energy production and nutrient mineralization. J. Waste Manag. 43, 123–129. doi: 10.1016/j.wasman.2015.05.016
Oamen, H. P., Ojo, E. O., Hobbs, P. J., and Retter, A. (2019). Hydrolysis of plant biomass at different growth stages using enzyme cocktails for increased fermentable hydrolysates. Sci. Afr. 3, e00077. doi: 10.1016/j.sciaf.2019.e00077
Ockerman, H. W., and Hansen, C. L. (1988). “Animal processing waste disposal, reduction and utilization,” in Animal By-Product-Processing, eds I. D. Morton, R. Scott, D. H. Watson, and M. Lewis (VCH, Cambridge: Ellis Horwood Ltd.), 250–251.
Orpin, C. G. (1975). Studies on the Rumen Flagellate Neocallimastix frontalis. J. Gen. Microbiol. 91, 249–262. doi: 10.1099/00221287-91-2-249
Orpin, C. G. (1976). Studies on the rumen flagellate Sphaeromonas communis. J. Gen. Microbiol. 94, 270–280. doi: 10.1099/00221287-94-2-270
Orpin, C. G., and Munn, E. A. (1986). Neocallimastix patriciarum sp.nov., a new member of the Neocallimasticaceae inhabiting the rumen of sheep. Trans. Brit. Mycol. Soc. 86, 178–181. doi: 10.1016/S0007-1536(86)80138-3
Ozbayram, E. G., Akyol, C., Ince, B., Karakoc, C., and Ince, O. (2017). Rumen bacteria at work: bioaugmentation strategies to enhance biogas production from cow manure. J. Appl. Microbiol. 124, 491–502. doi: 10.1111/jam.13668
Özelçam, H., Kirkpinar, F., and Tan, K. (2015). Chemical composition, in vivo digestibility and metabolizable energy values of caramba (Lolium multiflorum cv. caramba) fresh, silage and hay. Asian-australas. J. Anim. Sci 28, 1427–1432. doi: 10.5713/ajas.15.0074
Ozkose, E., Thomas, B. J., Davies, D. R., Griffith, G. W., and Theodorou, M. K. (2001). Cyllamyces aberensis gen.nov. sp.nov., a new anaerobic gut fungus with branched sporangiophores isolated from cattle. Can. J. Bot. 79, 666–673. doi: 10.1139/b01-047
Palatsi, J., Viñas, M., Guivernau, M., Fernandez, B., and Flotats, X. (2011). Anaerobic digestion of slaughterhouse waste: main process limitations and microbial community interactions. Bioresour. Technol. 102, 2219–2227. doi: 10.1016/j.biortech.2010.09.121
Parks, D. H., Rinke, C., Chuvochina, M., Chaumeil, P. A., Woodcroft, B. J., Evans, P. N., et al. (2017). Recovery of nearly 8,000 metagenome-assembled genomes substantially expands the tree of life. Nat. Microbiol. 2, 1533–1542. doi: 10.1038/s41564-017-0012-7
Parsiegla, G., Reverbel, C., Tardif, C., Driguez, H., and Haser, R. (2008). Structures of mutants of cellulase Cel48F of Clostridium cellulolyticum in complex with long hemithiocellooligosaccharides give rise to a new view of the substrate pathway during processive action. J. Mol. Biol. 375, 499–510. doi: 10.1016/j.jmb.2007.10.039
Paul, S. S., Bu, D., Xu, J., Hyde, K. D., and Yu, Z. (2018). A phylogenetic census of global diversity of gut anaerobic fungi and a new taxonomic framework. Fungal Divers. 89, 253–266. doi: 10.1007/s13225-018-0396-6
Paunch Contents Land Spreading Management Guidelines (2017). Available online at: www.epa.tas.gov.au (accessed April 15, 2020).
Prices | Energy Statistics in Ireland | Sustainable Energy Authority of Ireland (2021). Available online at: http://seai.ie/data-and-insights/seai-statistics/key-statistics/prices/ (accessed August 13, 2021).
Raghukumar, C., Muraleedharan, U., Gaud, V. R., and Mishra, R. (2004). Xylanases of marine fungi of potential use for biobleaching of paper pulp. J. Ind. Microbiol. Biotechnol. 31, 433–441. doi: 10.1007/s10295-004-0165-2
Ricci, R. (1977). A Method of Manure Disposal for a Beef Packing Operation: First Interim Technical Report. Available online at: https://nepis.epa.gov/ (accessed March 16, 2020).
Rodriguez, C., Alaswad, A., Benyounis, K. Y., and Olabi, A. G. (2017). Pre-treatment techniques used in biogas production from grass. Renew. Sust. Energ. Rev. 68, 1193–1204. doi: 10.1016/j.rser.2016.02.022
Rogers, J. A., Davis, C. L., and Clark, J. H. (1982). Alteration of rumen fermentation, milk fat synthesis, and nutrient utilization with mineral salts in dairy cows. J. Dairy Sci. 65, 577–586. doi: 10.3168/jds.S0022-0302(82)82235-2
Romano, R. T., Zhang, R., Teter, S., and McGarvey, J. A. (2009). The effect of enzyme addition on anaerobic digestion of Jose Tall Wheat Grass. Bioresour. Technol. 100, 4564–4457. doi: 10.1016/j.biortech.2008.12.065
Sakamoto, M., Umeda, M., Ishikawa, I., and Benno, Y. (2005). Prevotella multisaccharivorax sp. nov., isolated from human subgingival plaque. Int. J. Syst. Evol. 55, 1839–1843. doi: 10.1099/ijs.0.63739-0
SEAI (2018). National Energy Projections to 2030- Understanding Ireland's Energy Transition. Available online at: https://www.seai.ie/publications/National-Energy-Projections-to-2030.pdf
Seshadri, R., Leahy, S. C., Attwood, G. T., and Teh, K. H. (2018). Cultivation and sequencing of rumen microbiome members from the Hungate1000 collection. Nat. Biotechnol. 36, 359–366. doi: 10.1038/nbt.4110
So, M. J., Kim, H. S., Kim, J. H., Lee, S.-H., Lee, K.-W., Jung, J. S., et al. (2015). Comparison of forage production and nutritional value of italian ryegrass, rye and whole crop barley as winter forage crops in southern region of Korea. J. Kor. Grassl. Forage. Sci. 35, 245–250. doi: 10.5333/KGFS.2015.35.3.245
Sokolović, D., Babić, S., Marković, J., Radović, J., Lugić, Z., Živković, B., et al. (2010). “Dry matter production and nutritive value of perennial ryegrass cultivars collection,” in Sustainable Use of Genetic Diversity in Forage and Turf Breeding, ed C. Huyghe (Springer Netherlands), 341–346.
Solomon, K. V., Haitjema, C. H., Henske, J. K., Gilmore, S. P., Borges-Rivera, D., Lipzen, A., et al. (2016). Early-branching gut fungi possess a large, comprehensive array of biomass-degrading enzymes. Science 351, 1192–1195. doi: 10.1126/science.aad1431
Spence, J. E. (2012). Renewable energy in the australian red meat processing industry; the viability of paunch as a biofuel (MSc Thesis). Available online at: http://eprints.usq.edu.au/id/eprint/23223
Spence, J. E. (2017). The drying of abattoir paunch for waste-to-energy conversion (PhD Thesis). Available online at: https://eprints.usq.edu.au/34276
Stabel, M., Hanafy, R. A., Schweitzer, T., Greif, M., Aliyu, H., Flad, V., et al. (2020). Aestipascuomyces dupliciliberans gen. nov, sp. Nov., the first cultured representative of the uncultured SK4 clade from aoudad sheep and alpaca. MDPI Microorgan. 8:1734. doi: 10.20944/preprints202010.0333.v1
Stewart, R. D., Auffret, M. D., Warr, A., Walker, A. W., Roehe, R., and Watson, M. (2019). Compendium of 4,941 rumen metagenome-assembled genomes for rumen microbiome biology and enzyme discovery. Nat. Biotechnol. 37, 953–961. doi: 10.1038/s41587-019-0202-3
Stewart, R. D., Auffret, M. D., Warr, A., Wiser, A. H., Press, M. O., and Langford, K. W. (2018). Assembly of 913 microbial genomes from metagenomic sequencing of the cow rumen. Nat. Commun. 9, 1–11. doi: 10.1038/s41467-018-03317-6
Stokes, M. R., Bull, L. S., and Halteman, W. A. (1985). Rumen liquid dilution rate in dairy cows fed once daily: effects of diet and sodium bicarbonate supplementation. J. Dairy Sci. 68, 1171–1180. doi: 10.3168/jds.S0022-0302(85)80944-9
Sun, X. Z., Waghorn, G. C., and Clark, H. (2010). Composition and in sacco degradation characteristics of winter growth of Lolium perenne, Lolium multiflorum and Lolium perenne × multiflorum ryegrasses. Proc. N. Z. Soc. Anim. Prod. 70, 206–211. Available online at: https://www.nzsap.org/proceedings/2010/composition-and-sacco-degradation-characteristics-winter-growth-lolium-perenne
Sustainable Practices in Irish Beef Processing (2009). Available online at: https://www.leanbusinessireland.ie/includes/documents/Sustainable Practices Beef Report Full Report.pdf (accessed April 15, 2020).
Takizawa, S., Abe, K., Fukuda, Y., Feng, M., Baba, Y., Tada, C., et al. (2020a). Recovery of the fibrolytic microorganisms from rumen fluid by flocculation for simultaneous treatment of lignocellulosic biomass and volatile fatty acid production. J. Clean. Prod. 257, 120626. doi: 10.1016/j.jclepro.2020.120626
Takizawa, S., Asano, R., Fukuda, Y., Feng, M., Baba, Y., Abe, K., et al. (2020b). Change of endoglucanase activity and rumen microbial community during biodegradation of cellulose using rumen microbiota. Front. Microbiol. 11, 1–12. doi: 10.3389/fmicb.2020.603818
Takizawa, S., Baba, Y., Tada, C., Fukuda, Y., and Nakai, Y. (2018). Pretreatment with rumen fluid improves methane production in the anaerobic digestion of paper sludge. J. Waste Manag. 78, 379–384. doi: 10.1016/j.wasman.2018.05.046
Takizawa, S., Baba, Y., Tada, C., Fukuda, Y., and Nakai, Y. (2019). Preservation of rumen fluid for the pretreatment of waste paper to improve methane production. Waste Manag. 87, 672–678. doi: 10.1016/j.wasman.2019.02.043
Tritt, W. P., and Kang, H. (1991). Ultimate biodegradability and decay rates of cow paunch manure under anaerobic conditions. Bioresour. Technol. 36, 161–165. doi: 10.1016/0960-8524(91)90174-I
Ulyatt, M. J. (1971). Studies on the causes of the differences in pasture quality between perennial ryegrass, short-rotation ryegrass, and white clover. N. Zeal. J. Agric. Res. 14, 352–367. doi: 10.1080/00288233.1971.10427100
United Nations Environment Programme EPA Denmark (eds.),. (2000). Cleaner Production Assessment in Meat Processing. Available online at: https://digitallibrary.un.org/record/4417711?ln=en.
Verheijen, L. A. H. M., Wiersema, D., and Hulshoff Pol, L. W. (1996). Management of Waste From Animal Product Processing. Available online at: http://www.fao.org/3/x6114e/x6114e00.htm (accessed April 15, 2020).
Waghorn, G. C., Shelton, I. D., and Thomas, V. J. (1989). Particle breakdown and rumen digestion of fresh ryegrass (Lolium perenne L.) and lucerne (Medicago sativa L.) fed to cows during a restricted feeding period. Br. J. Nutr. 61, 409–423. doi: 10.1079/BJN19890127
Wall, D. M., Straccialini, B., Allen, E., Nolan, P., Herrmann, C., O'Kiely, P., et al. (2015). Investigation of effect of particle size and rumen fluid addition on specific methane yields of high lignocellulose grass silage. Bioresour. Technol. 192, 266–271. doi: 10.1016/j.biortech.2015.05.078
Wang, S., Zhang, G., Zhang, P., Ma, X., Li, F., Zhang, H., et al. (2018). Rumen fluid fermentation for enhancement of hydrolysis and acidification of grass clipping. J. Environ. Manage. 220, 142–148. doi: 10.1016/j.jenvman.2018.05.027
Wang, Y., Youssef, N. H., Couger, M. B., Hanafy, R. A., Elshahed, M. S., and Stajich, J. E. (2019). Molecular dating of the emergence of anaerobic rumen fungi and the impact of laterally acquired genes. mSystems 4:e00247-19. doi: 10.1128/mSystems.00247-19
Wang, Z., Keshwani, D. R., Redding, A. P., and Cheng, J. J. (2010). Sodium hydroxide pretreatment and enzymatic hydrolysis of coastal Bermuda grass. Bioresour. Technol. 101, 3583–3585. doi: 10.1016/j.biortech.2009.12.097
Ware, A., and Power, N. (2016). Biogas from cattle slaughterhouse waste: energy recovery towards an energy self-sufficient industry in Ireland. Renew. Energy 97, 541–549. doi: 10.1016/j.renene.2016.05.068
Webb, J., and Theodorou, M. K. (1991). Neocallimastix hurleyensis sp.nov., an anaerobic fungus from the ovine rumen. Can. J. Bot. 69, 1220–1224. doi: 10.1139/b91-156
Wilkinson, J. M., le Du, Y. L. P., Cook, J. E., and Baker, R. D. (1982). The intake and feeding value for young beef cattle of two cultivars of tetraploid Italian ryegrass when grazed or conserved by artificial dehydration or ensilage. Grass Forage Sci. 37, 29–38. doi: 10.1111/j.1365-2494.1982.tb01573.x
Wilson, D. (1992). “Methods of disposal of paunch contents with emphasis on composting,” in Inedible Meat by-Products, eds A. M. Pearson and T. R. Dutson (Dordrecht: Springer Netherlands), 265–281.
Wims, C. M., McEvoy, M., Delaby, L., Boland, T. M., and O'Donovan, M. (2013). Effect of perennial ryegrass (Lolium perenne L.) cultivars on the milk yield of grazing dairy cows. Animal 7, 410–421. doi: 10.1017/S1751731112001814
Witherow, J., and Scaief, J. (1976). Workshop on in-Plant Waste Reduction in the Meat Industry. Madison, WI: University of Madison.
Wohlt, J. E., Clark, J. H., and Blaisdell, F. S. (1976). Effect of sampling location, time, and method of concentration of ammonia nitrogen in rumen fluid. J. Dairy Sci. 59, 459–464. doi: 10.3168/jds.S0022-0302(76)84227-0
Xing, B. S., Han, Y., Wang, X. C., Wen, J., Cao, S., Zhang, K., et al. (2020). Persistent action of cow rumen microorganisms in enhancing biodegradation of wheat straw by rumen fermentation. Sci. Total Environ. 715, 1–13. doi: 10.1016/j.scitotenv.2020.136529
Yi, J., Dong, B., Jingwei, J., and Dai, X. (2014). Effect of increasing total solids contents on anaerobic digestion of food waste under mesophilic conditions: performance and microbial characteristics analysis. PLoS ONE 9, e102548. doi: 10.1371/journal.pone.0102548
Youssef, N. H., Couger, M. B., Struchtemeyer, C. G., Liggenstoffer, A. S., Prade, R. A., Najar, F. Z., et al. (2013). The genome of the anaerobic Fungus orpinomyces sp. strain C1A reveals the unique evolutionary history of a remarkable plant biomass degrader. Appl. Environ. Microbiol. 79, 4620–4634. doi: 10.1128/AEM.00821-13
Keywords: paunch contents, bellygrass, circular economy, sustainable energy, anaerobic digestion (AD), slaughterhouse waste, rumen microbiome, rumen fluid
Citation: Dowd B, McDonnell D and Tuohy MG (2022) Current Progress in Optimising Sustainable Energy Recovery From Cattle Paunch Contents, a Slaughterhouse Waste Product. Front. Sustain. Food Syst. 6:722424. doi: 10.3389/fsufs.2022.722424
Received: 13 June 2021; Accepted: 17 March 2022;
Published: 04 May 2022.
Edited by:
José Luis Garcia-Morales, University of Cádiz, SpainReviewed by:
José Luis Campos Gómez, Adolfo Ibáñez University, ChileChika Tada, Tohoku University, Japan
Copyright © 2022 Dowd, McDonnell and Tuohy. This is an open-access article distributed under the terms of the Creative Commons Attribution License (CC BY). The use, distribution or reproduction in other forums is permitted, provided the original author(s) and the copyright owner(s) are credited and that the original publication in this journal is cited, in accordance with accepted academic practice. No use, distribution or reproduction is permitted which does not comply with these terms.
*Correspondence: Maria G. Tuohy, maria.tuohy@nuigalway.ie; Bronwyn Dowd, b.dowd1@nuigalway.ie