- 1Division of Agronomy, ICAR- Indian Agricultural Research Institute, New Delhi, India
- 2ICAR Research Complex for NEH Region, Sikkim Centre, Gangtok, India
- 3ICAR- Indian Institute of Pulses Research, Kanpur, Uttar Pradesh, India
- 4National Rainfed Area Authority, New Delhi, India
- 5Sardar Vallabhbhai Patel University of Agriculture and Technology, Meerut, Uttar Pradesh, India
- 6ICAR Research Complex for NEH Region Tripura Centre, Agartala, Tripura, India
- 7ICAR Research Complex for NEH Region Umiam, Meghalaya, India
- 8Sher-e-Kashmir University of Agriculture and Technology, Jammu, Jammu and Kashmir, India
- 9Sher-e-Kashmir University of Agriculture and Technology, Srinagar, Jammu and Kashmir, India
- 10ICAR- Central Research Institute on Dryland Agriculture, Hyderabad, Telangana, India
Environmental pollution, resource dwindling, and soil degradation questioned the sustainability of contemporary agricultural production systems. Organic farming is advocated as a sustainable solution for ensuring food security without compromising environmental sustainability. However, poor farm productivity quizzed the sustainability of organic production systems. Hence, a field study was carried out in the Sikkim region of the Indian Himalayas to assess the efficacy of conservation-effective tilling and diversified cropping on system productivity, profitability, environmental quality, and soil nutrient balance in organic farming. Three tillage systems, namely, (i) conventional tillage (CT), (ii) reduced tillage (RT), and (iii) zero tillage (ZT), and four maize based diversified cropping systems (maize–black gram–toria, maize–black gram–buckwheat, maize–rajmash–toria, and maize–rajmash–buckwheat) were tested using a three times replicated split-plot design. The ZT system recorded 13.5 and 3.5% higher system productivity over CT and RT, respectively. Of the four diversified cropping systems, the maize–rajmash–buckwheat system recorded the maximum system productivity (13.99 Mg ha−1) and net returns (3,141 US$ ha−1) followed by the maize–black gram–buckwheat system. Among the tillage practices, ZT recorded the significantly high eco-efficiency index (EEI; 1.55 US$ per kg CO2-eq emission) and the lowest greenhouse gas intensity (GHGI; 0.15 kg CO2-eq per kg production). Of the diversified cropping systems, the maize-rajmash-buckwheat registered the lowest GHGI (0.14 CO2-eq per kg production) and the highest EEI (1.47 US$ per kg CO2-eq emission). Concerning soil nutrient balance, after three cropping cycles, the soil under ZT recorded significantly higher available N (340.0 kg ha−1), P (16.6 kg ha−1), and K (337.3 kg ha−1) over the CT system at 0–10 cm soil depth. Similarly, the soil under the maize–black gram–buckwheat system had the maximum bio-available NPK. Thus, the study suggests that the cultivation of the maize–black gram/rajmash–buckwheat systems under ZT and/or RT would increase farm productivity, profitability, and soil fertility with minimum GHGI in organic farming under the Eastern Himalayan region of India.
1. Introduction
Environmental crises, poor economic returns, declining factor productivity, and resource degradation quizzed the sustainability of contemporary agricultural practices (Yadav et al., 2021b; Ansari et al., 2022). Although the modern/contemporary agricultural production system enhances food production by many folds, but simultaneously creates tremendous pressure on natural resources (Babu et al., 2022). Hence, sustainable food and nutritional security without compromising environmental quality is an indispensable for the planet and population health. After air and water, food security is a basic human need. Achieving sustainable national and household-level food and nutritional security is a complicated and complex target affected by multiple factors like human competency, policies, infrastructure, technological invention, and dissemination (Yadav et al., 2021a; Babu et al., 2022). Under the current scenario of natural resource depletion, climate change further amplified the food and nutritional insecurity challenges (Panwar et al., 2022). The Conference of the Parties of the United Nations Framework Convention on Climate Change (UNFCCC) (COP 27) meeting held at Sharm El-Sheikh, Egypt in November 2022 also emphasized safeguarding the food production system from climate change vulnerability.
Monocropping, poor residue returns, and intensive tillage are the leading causes of soil quality degradation and poor economic returns (Yadav et al., 2021b). Repeated tillage adversely impacts soil porosity, water movement, and soil compactness, which result in poor crop growth and productivity (Yadav et al., 2020). The global cultivated land reached the terrestrial frontier (Henry et al., 2018), and adverse environmental outcomes are expected if traversed by this planetary line (Molotoks et al., 2018). Hence, the main question is how the existing land should be utilized intelligently to enhance farm productivity without jeopardizing environmental quality (Avasthe et al., 2020). This calls for designing and developing improved cropping systems with sustainable management practices. Sustainable intensification can be used to potentially increase food production without bringing additional land under cultivation. However, the selection of an efficient, economically feasible system for a particular site requires robust planning (Avasthe et al., 2020). Pulses, a rich source of dietary protein, capture and fix atmospheric nitrogen into the soil system. Hence, embedding pulses in diversified cropping can improve food, nutrition, and environmental security (Babu et al., 2020b).
Organic farming is widely advocated as a possible solution for achieving sustainable food and environmental security (Singh et al., 2021). However, poor crop productivity under organic agricultural systems as compared to conventional chemical farming has been reported by several researchers (de Ponti et al., 2012; Yadav et al., 2014; Knapp and van der Heijden, 2018). Organically managed farms had ~19% less crop productivity than conventionally managed fields (de Ponti et al., 2012). However, the magnitude of yield reduction varies with soil and crop types and climatic conditions. Organic production systems may be crucial for mitigating negative environmental outcomes but poor crop productivity forces researchers to develop the appropriate location-specific agronomic management practices to boost overall farm productivity and profitability of organic production systems. Adopting farming practices that conserve soil and water through minimal soil disturbances and residue cover is one of the best management practices and is being advocated globally for improving the nutrient status in degraded soils under conventional chemical-based farming (Yadav et al., 2020).
The Indian Himalayan Region (IHR) occupies a 53.7 Mha area and a habitat of ~50 million people. The IHR covers 16.4% of the geographical area of the country and is spread over 13 Indian states. Agriculture in the IHR is organic by default, and the productivity of most of the field crops remains low as compared to the irrigated plain lands of India (Babu et al., 2016; Das et al., 2019). In hilly ecosystems, especially under organic management conditions, the impact of tillage practices and cropping diversity on farm production efficiency and soil fertility is not widely evaluated. Therefore, it is imperative to evaluate the impact of diverse conservation tillage practices and diversified cropping on farm productivity, profitability, environmental outcomes, and soil fertility to formulate the appropriate management policies for the long-term sustainability of Indian hill agriculture. The IHR has vast scopes to increase food production and restore the risk-prone soils of the region through the adoption of conservation agricultural (CAs) practices. Farmers of the IHR, especially the Eastern Himalayan region, generally grow rainy-season crops with minimal organic inputs and keep their land fallow during the winter season due to moisture scarcity. The existing cropping scenarios are the main cause of poor farm productivity in the mountain ecosystem of India (Avasthe et al., 2020). Hence, the diversification of the prevailing cropping systems is urgently required with crops that can potentially improve farm productivity and profitability. Furthermore, the cultivation of more crops in a year as against monocrops would extend the soil covering period, which will help to protect the soil from erosion during heavy rainfall in sloping lands (Babu et al., 2020b). Hence, there is an emerging curiosity among researchers to apply the principle of conservation tillage under organic management to conserve natural resources and sustain farm productivity. The comparative effect of diversified cropping and conservation tillage on system productivity, economic returns, eco-efficiency, and soil nutrient balance is not adequately addressed under organic management, especially for Himalayan ecosystems. Hence, it was hypothesized that the cultivation of diversified cropping along with conservation tillage practices can potentially improve farm productivity, profitability, and soil fertility with minimum negative environmental outcomes under organic farming. To achieve the above hypothesis, a field study was conducted during 2015–2018 with the following objectives (i) to assess the effect of tillage and diversified cropping on system productivity and economic returns and (ii) to assess the effect of diverse tillage and cropping systems on eco-efficiency, greenhouse gas intensity, and soil nutrient availability under organic farming. The findings of the current study will help to achieve India's commitments to food security and climate change mitigation and the related sustainable development goals (SDGs).
2. Materials and methods
2.1. The study site
A 3-year (2015–2018) study was carried out at the research farm, ICAR Research Complex in the North Eastern Hill region, Sikkim Center. The research farm was located in the Tadong area of Gangtok, the capital city of the first certified organic state of India, and lies between 27°32′ N latitude and 88°60′ E longitude with an altitude of 1,350 m above the mean sea level (Figure 1). The Eastern Himalayan region (EHR) is an inimitable ecosystem in the world and is counted as a crisis eco-region. The EH region is extended from Central Nepal to Yunnan in China, including Bhutan, Arunachal Pradesh, Nagaland, Mizoram, Manipur, Sikkim, Meghalaya, Tripura, Manipur, and the hills of West Bengal and Assam, Myanmar, and Southeast Tibet. The soils are predominantly acidic and prone to degradation. The diverse ecology and altitudinal gradient range from 300 to 8,000 m and represent great diversity in flora and fauna (Singh et al., 2021). The Haplumbrept soil of the study area is sandy loam. The mean annual temperature of the region lies between 4 and 22°C. The average rainfall is about 3,000 mm annually, of which 75%−80% is received mainly from June to September.
2.2. Treatment details and crop management
Soil sampling and analysis were carried out before setting the experiment and after three cropping cycles. The experimental soil was high in carbon (17.8 g kg−1), medium in available nitrogen (312 kg ha−1), and phosphorus (15.6 kg ha−1), and high in plant-available potassium (320.2 kg ha−1). Three tillage practices, i.e., conventional tillage (CT), reduced tillage (RT), and zero tillage (ZT) were practiced in the main plots and four diversified cropping systems, i.e., maize (Zea mays L.)–black gram (Vigna mungo var. viridis)–toria (Brassica campestris), maize–black gram–buckwheat (Fagopyrum sp), maize–rajmash (Phaseolus vulgaris)–toria, and maize–rajmash–buckwheat were practiced in the subplots. All the treatments were replicated three times in a fixed pattern under a split plot design. The maize composite cv. DA-61 A (20 kg seed ha−1) was sown at a 50 × 20 cm spacing during March (second fortnight) every year. Black gram and rajmash were grown as late post-rainy season crops sown in the second fortnight of August in each year of experimentation. Black gram (PD-3) and rajmash (SKR-57A) were dibbled with a seed rate of 25 and 75 kg ha−1 at a distance of 30 × 10 and 40 × 10 cm geometry, respectively. The winter crops, namely, toria (M-27) and buckwheat (local Meethey) were sown in November every year with a seed rate of 4 and 40 kg ha−1, respectively. Both the winter season crops were seeded at 30 × 10 cm geometry. The recommended doses of nitrogen for maize, black gram, rajmash, toria, and buckwheat are 60, 20, 60, 40, and 40 kg ha−1, which were supplied through well-decomposed farmyard manure (FYM); ~28% moisture) containing 0.59% N, 0.30% P, and 0.52% K. Full quantity of FYM was applied before 1 week of sowing. Four tilling under CT and two tilling under RT (~8–12 cm depth) were done with the help of a power tiller. Whereas in ZT, the soil was not much disturbed and the tillage operation was restricted to the opening of the furrow by using a ZT row marker. Under RT and ZT, ~30% of the maize residues and the entire residues of the succeeding crops were retained on the soil surface. Irrespective of the tillage practices, two-hand weeding was done for 20 days after sowing (DAS) and after 40 DAS in each crop to manage the weeds. To maintain an optimum plant population, thinning and gap-filling operations were done along with the first weeding. Maize, black gram, and rajmash were grown as rainfed crops. Hence, no artificial irrigation was imposed. However, lifesaving irrigations were given as and when required to winter crops. Seed treatment was done with Trichoderma spp. (4 g kg−1) to reduce pathogen infestation. Neem oil (5 ml per L of water) was sprayed after 20 days of sowing (2–3 times at 10-day intervals) in each crop to avoid/minimize insect and pest infestation.
2.3. Harvesting and yield measurement
At physiological maturity, maize cobs were harvested using iron sickle in the first week of August. After harvesting, the maize cobs were sun-dried for a week on the threshing floor, and thereafter, the grain was removed from the cobs by a manual maize sheller. After cob harvesting, the maize plants were cut ~30 cm above the ground in RT and ZT but at ~5–10 cm in CT plots. The short-duration black gram and rajmash were harvested during the second fortnight of October every year. Similarly, the winter crops (toria and buckwheat) were harvested during March (first fortnight) every year. After harvesting late-rainy and winter crops, the grains of all the crops were threshed manually by beating small bundles (biomass bundles were made plot-wise after harvesting) on an iron drum on a threshing floor. The harvested produce of all the crops was sun-dried. The economic yield of the crops (maize and buckwheat) was observed at 12% moisture content, while the black gram, rajmash, and toria economic yields were recorded at 8% moisture in seed and articulated in Mg ha−1.
2.4. Analysis of system productivity and economic returns
The economic yield of all the crops was converted into the maize equivalent yield (MEY) and expressed as system productivity (SP). The SP measured the productive capacity of the different tillage and diversified systems. The dominant local market rate was deployed for calculating the SP. MEY was worked out as shown in the following equation:
where MEY was Mg ha−1, MY and Yi were the economic yields of maize and ith crops in Mg ha−1, respectively; Pi and Pm were the selling/market rate (US$) of the ith crop and maize, respectively.
The effect of tillage and diversified systems were also assessed on per day farm production capacity by calculating the system production efficiency (SPE) (Equation 2).
For economic accounting, the cultivation cost of all the tillage and diversified systems was estimated based on the diverse inputs incurred and the activities done. The gross economic return was the rate of the economic products in the market. The cost–benefit ratio is the proportional valuation of the treatment in terms of per unit investment. The monetary spending and earnings attained from all the treatments were converted into US$. The system net returns (SNR), and the benefit-to-cost (B:C) ratio were derived with the following equations.
2.5. Estimation of greenhouse gas intensity and eco-efficiency index
The total extent of GHG emissions (CO2 and N2O) released during the entire cropping period was assessed as a CO2 equivalent (CO2-eq) (Yadav et al., 2017). CO2-eq is also called a carbon footprint (CF). In the present study, all the crops were grown under well-drained upland conditions. Therefore, carbon dioxide (CO2) and nitrous oxide (N2O) gases were taken into account for CF estimation. Total CO2 and N2O released from a particular treatment were expressed through CO2-eq. by multiplying the GWP equivalent of 1 and 265 for CO2 and N2O, respectively, for 100 years timeframe (Yadav et al., 2017). Standard emission coefficients were used to estimate the GHG emission from different treatments. The emission factor of 0.01 was multiplied by the total N supplied through organic sources and articulated in N2O kg N input−1 to quantify the total N2O emission (Tubiello et al., 2015).
Global warming potential (GWP) from all the treatments was calculated by summing the total CO2-eq released as follows:
Greenhouse gas intensity (GHGI) was calculated using the following expression:
Eco-efficiency estimation is imperative to judge the environmental robustness of the designed technology. Eco-efficiency indicates the economic returns capacity of a designed technology concerning environmental destruction. In the current experimentation, the ecological impact of different tillage and diversified systems was measured in terms of total GHG emission (kg CO2-eq per year). Eco-efficiency was calculated using the following equation:
2.6. Analysis of available soil nutrients
For the analysis of soil-available NPK, the soil samples were collected after the completion of three cropping cycles. The available P and K were estimated using Bray's P1 (0.03 N NH4F in 0.025 N HCl) pH 4.65 and 1N NH4OAc extractable K at pH 7.0, while available N was evaluated using the alkaline KMnO4 method (Prasad et al., 2006).
2.7. Statistical analysis
The experimental data from different tillage and diversified cropping were statistically evaluated according to the procedure described by Gomez and Gomez (1984). Analysis of variance (ANOVA) and test of significance at p = 0.05 were computed using the SPSS software version 27.0.
3. Results
3.1. Crop productivity
3.1.1. Productivity of maize
Tillage practices exerted a significant effect on maize productivity (Table 1). Of the diverse tillage practices, a considerably higher maize yield (4.02 Mg ha−1) was recorded under RT followed by ZT (3.92 Mg ha−1), respectively. However, the lowest maize grain yield (3.88 Mg ha−1) was recorded under CT. Overall, RT ascribed 3.7 and 2.8% higher economic productivity of maize over CT and ZT, respectively. Similarly, the economic productivity of maize was significantly higher (4.06 Mg ha−1) under the maize–black gram–buckwheat system over the maize–rajmash–toria (3.81 Mg ha−1) and maize–rajmash–buckwheat (3.89 Mg ha−1) systems but remained statistically on par with the maize–black gram–toria (3.99 Mg ha−1) system. Similarly, maize grain yield was also higher under the maize–black gram–toria system over the maize–rajmash–toria and maize–rajmash–buckwheat systems. This indicated that the inclusion of short-duration pulses (black gram) had a beneficial effect on maize yield in the system mode. Overall, the inclusion of black gram increases maize yield by 6.6 and 4.6% over the maize–rajmash–toria and the maize–rajmash–buckwheat systems, respectively.
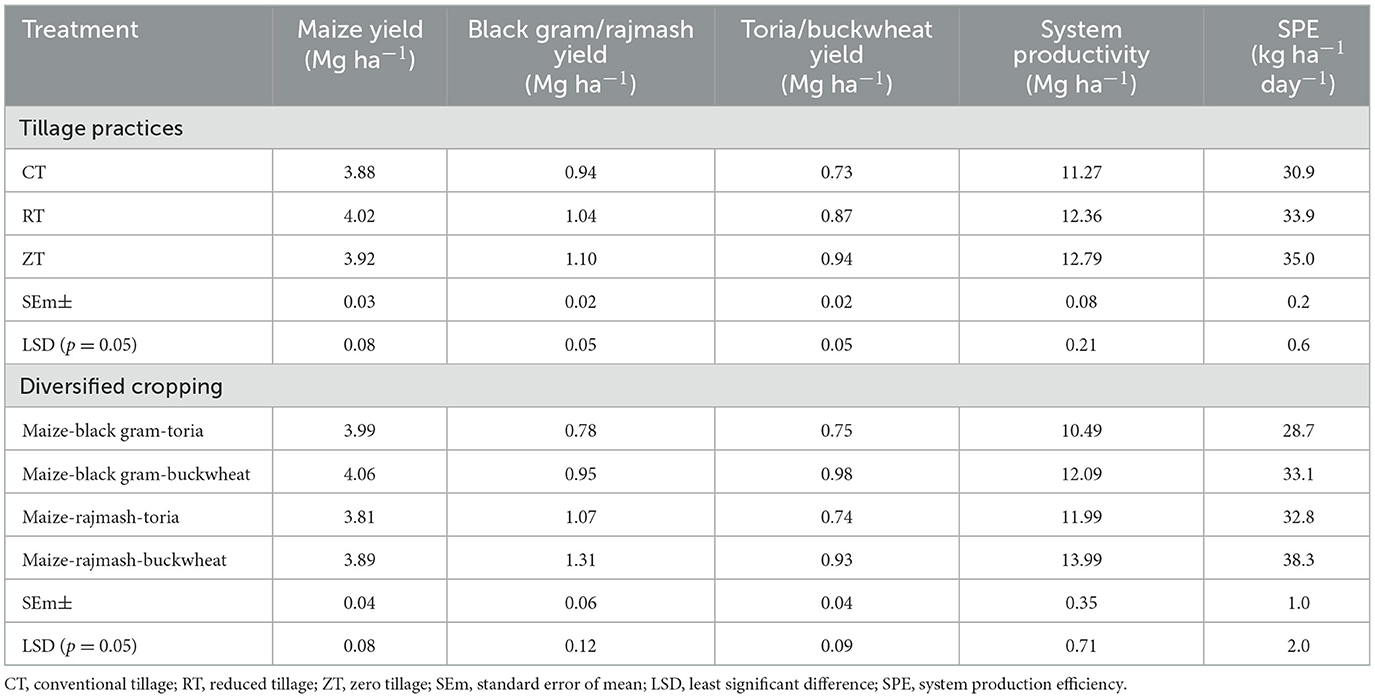
Table 1. Impact of different tillage practices and diversified cropping on crop productivity (mean of 3 years).
3.1.2. Productivity of late-rainy-season crops (black gram/rajmash)
The tillage practices significantly influenced the productivity of late-rainy-season crops (black gram/rajmash). An expressively advanced seed yield of rajmash was recorded under ZT (1.10 Mg ha−1) compared to CT (0.94 Mg ha−1) and RT (1.04 Mg ha−1), respectively. RT also recorded a significantly higher yield than CT. Among the diversified cropping systems, the maize–rajmash–buckwheat system had a significantly higher rajmash yield compared to the other cropping systems. Similarly, under the maize–rajmash–toria system, the rajmash yield was higher as compared to the black gram yield under another cropping system. Overall, the rajmash yield was higher compared to the black gram tested in the cropping system. Concerning to interaction effect of tillage practices and diversified cropping systems (Figure 2), cultivation of maize–rajmash–buckwheat under ZT recorded significantly higher economic yields (1.60 Mg ha−1) over other combinations.
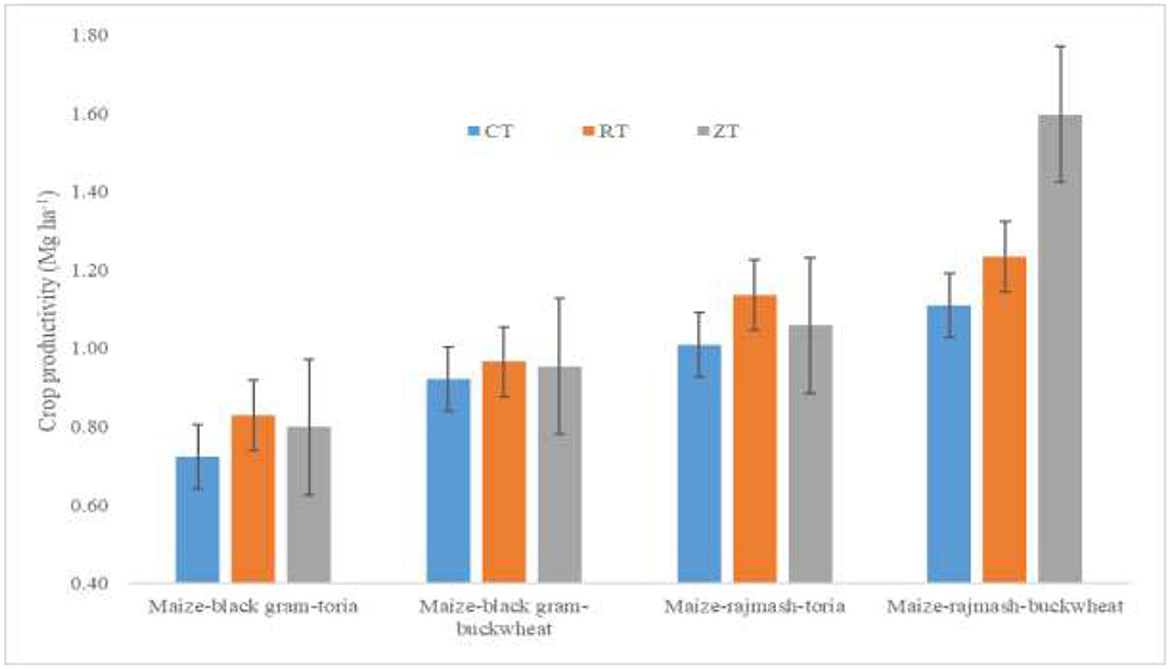
Figure 2. Interactive effect of tillage and diversified cropping on the productivity of late rainy season crops. CT, conventional tillage; RT, reduced tillage; ZT, zero tillage. Error bar indicates the least significant difference (LSD) values at p = 0.05.
3.1.3. Productivity of winter season crops (toria/buckwheat)
The productivity of winter crops had also been significantly influenced by different tillage and diversified cropping. Of various tillage practices, ZT recorded a significantly higher (0.94 Mg ha−1) yield than CT (0.73 Mg ha−1) and RT (0.87 Mg ha−1). The increase in the output of winter crops was 29.0% and 8.2% higher under ZT compared to CT and RT, respectively. Among the diversified cropping systems, buckwheat yield was significantly higher under the maize–black gram–buckwheat system over others but endured statistically on par with the maize–rajmash–buckwheat system (Table 1). Buckwheat yield was comparatively higher than toria yield under different cropping systems. The interactive impact of tillage and diversified cropping was also found to be significant in respect of the productivity of winter crops (Figure 3). The cultivation of maize–black gram–buckwheat under ZT recorded significantly higher (1.01 Mg ha−1) seed yields compared to the other combinations, while the cultivation of maize–black gram–toria under CT recorded the lowest yield (0.53 Mg ha−1).
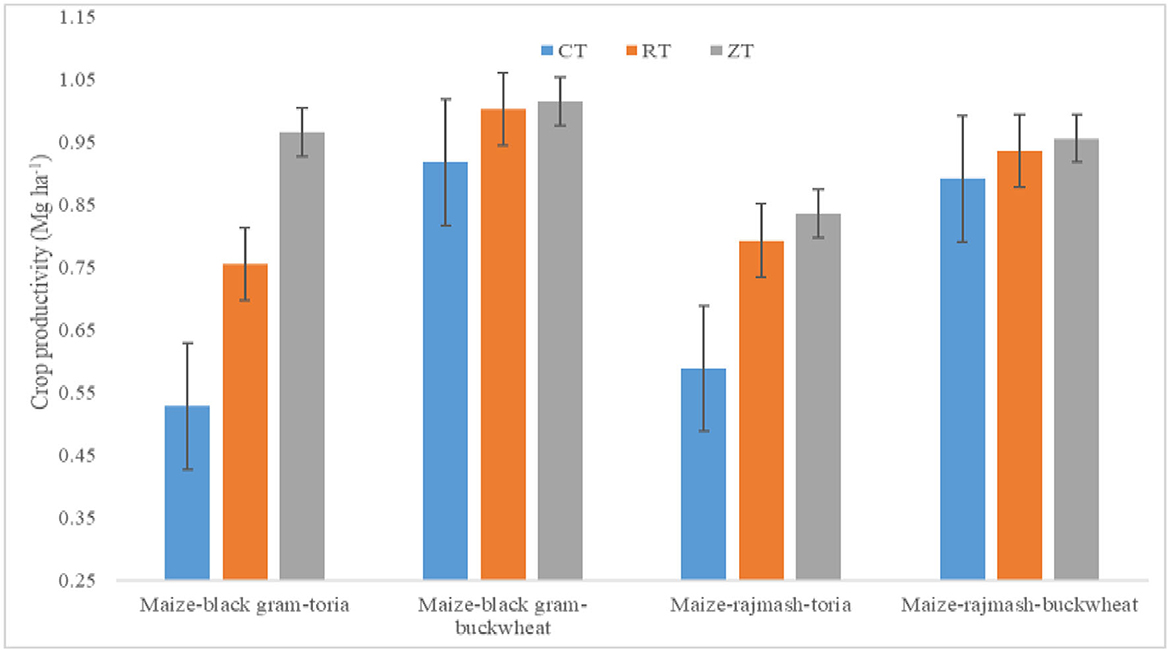
Figure 3. Interactive effect of tillage and diversified cropping on the productivity of winter season crops. CT, conventional tillage; RT, reduced tillage; ZT, zero tillage. Error bar indicates the least significant difference (LSD) values at p = 0.05.
3.2. System productivity and economic returns
The productive capacity of diverse tillage and diversified cropping was assessed in terms of system productivity (SP) and system production efficiency (SPE). Both SP and SPE were significantly affected by the diverse tillage and diversified cropping systems (Table 1). The SP was considerably higher under ZT (12.79 Mg ha−1) than under RT (12.36 Mg ha−1) and CT (11.27 Mg ha−1). The lowest SP was observed under CT. Similarly, the highest SPE was recorded under ZT and the lowest was under CT. The SPE was ~13.5 and 3.5% higher under ZT than those under CT and RT, respectively (Table 1). The production cost analysis revealed that ZT had a 4.9% lower production cost than CT (Table 2). System gross returns, net returns, and the B:C ratio was significantly higher under ZT than CT and RT. The ZT system had 13.5, 24.7, and 30.3% higher system gross returns (SGR), system net returns (SNR), and B:C ratio over CT, respectively (Table 2). Similarly, RT also had a 9.6, 16.7, and 18.98% higher SGR, SNR, and B: C ratio over CT, respectively. Among the diversified cropping, the lowest production cost (1,299 US$ ha−1) was incurred upon cultivation using the maize–black gram–toria system. At the same time, the maximum cost was incurred (1,500 US$ ha−1) for production upon using the maize–rajmash–buckwheat system. Similarly, the maximum SGR was observed for the maize–rajmash–buckwheat system (4,643 US$ ha−1) followed by the maize–black gram–buckwheat system (4,014 US$ ha−1). Similarly, this particular system registered the highest SNR and B: C ratio, while the lowest was under the maize–black gram–toria system (SNR −2,181 US$ ha−1 and B:C ratio −1.68).
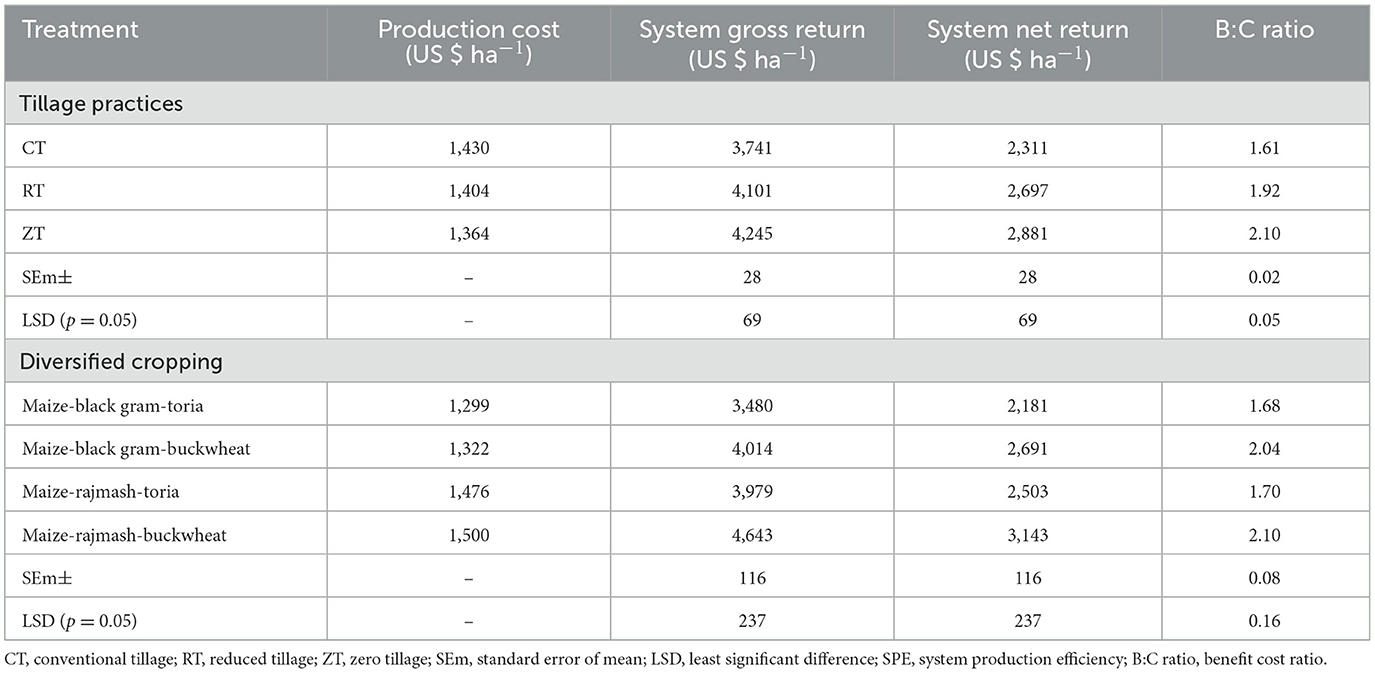
Table 2. Impact of different tillage practices and diversified cropping on economic returns (mean of 3 years).
3.3. Greenhouse gas intensity and eco-efficiency index
The GHGI is the quantity of GHG in terms of CO2-eq released for a unit of economic production. Tillage practices have a significant impact on GHGI under an organic production system. The CT system had the highest GHGI (0.21 kg CO2-eq per kg production), while the ZT recorded the lowest GHGI (0.15 kg CO2-eq per kg production). ZT had 28% and 11.76% less GHGI over CT and RT, respectively (Figure 4). Concerning diversified cropping, the substitution of toria with buckwheat during the winter season and black gram with rajmash during the late rainy season had a significant impact on GHGI. Among the tested diversified cropping systems, the maize–rajmash–buckwheat system had the lowest GHGI (0.14 kg CO2-eq per kg production), while the maize–black gram–toria system had the highest GHGI (0.20 kg CO2-eq per kg production; Figure 5). The eco-efficiency indicates the net economic gain per unit of ecological destruction concerning GHG emission. In the current study, the eco-efficiency index (EEI) was articulated in terms of monetary gain per unit of CO2-eq emission (US$ per kg CO2-eq). Among the tilling practices, CT had the lowest eco-efficiency (1.0 US$ kg−1 CO2-eq emission; Figure 4), whereas ZT had the highest EEI (1.55 US$ per kg CO2-eq emission). It implied that the CT in Eastern Himalayas may be replaced/substituted with conservation effective tillage for improving economic gain and environmental quality. Diversified cropping also exerted a significant impact on EEI. The maize–rajmash–buckwheat system recorded the highest EEI (1.57 US$ per kg CO2-eq emission) followed by the maize–black gram–buckwheat system. The maize–black gram–toria system had the least EEI (1.06 US$ per kg CO2-eq emission; Figure 5).
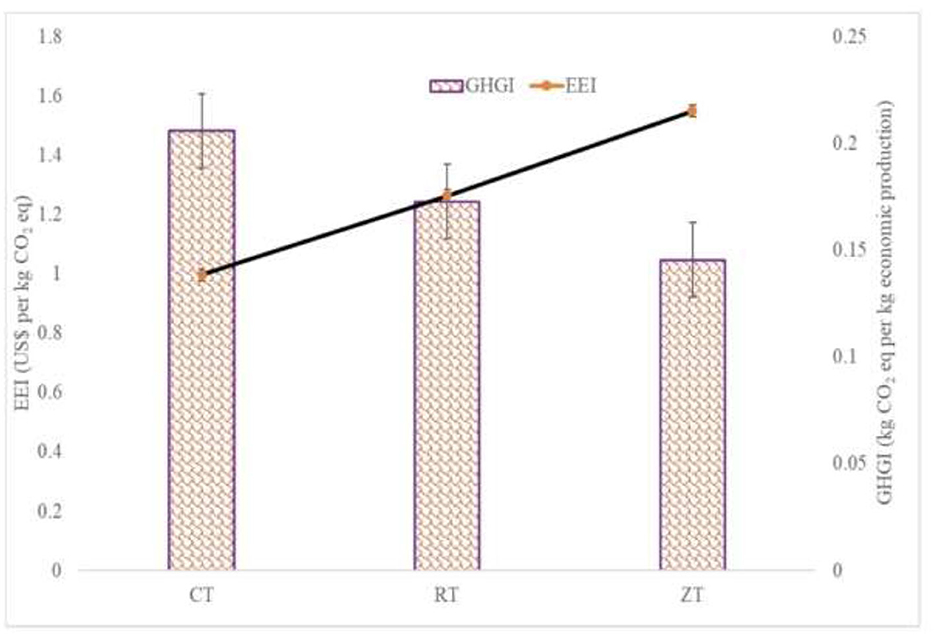
Figure 4. Effect of tillage practices on eco-efficiency index (EEI) and greenhouse gas intensity (GHGI). CT, conventional tillage; RT, reduced tillage; ZT, zero tillage. Error bar indicates the least significant difference (LSD) values at p = 0.05.
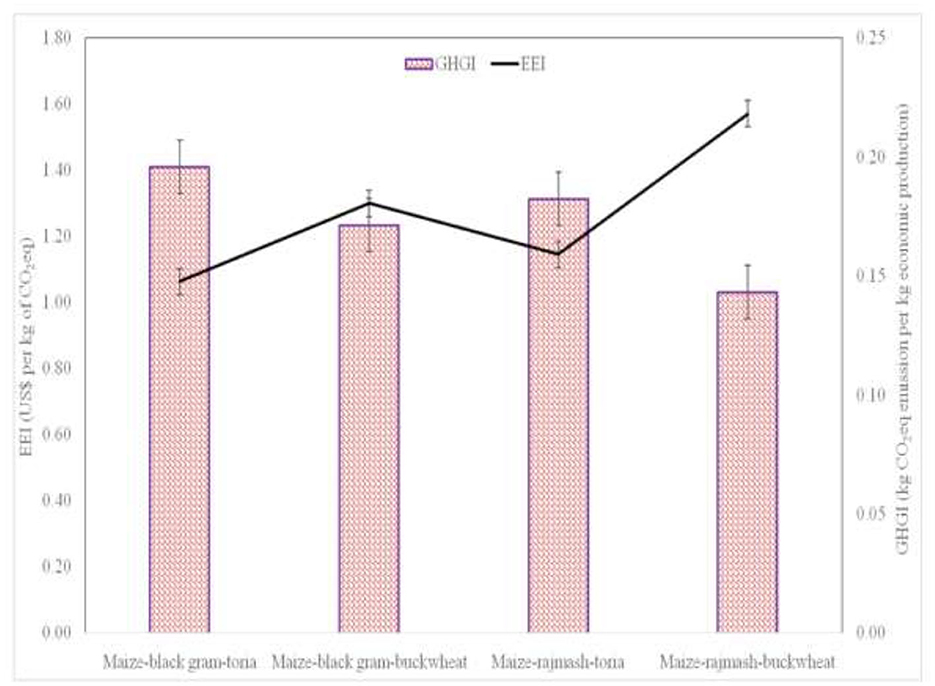
Figure 5. Effect of diversified cropping on eco-efficiency index (EEI) and greenhouse gas intensity (GHGI). Error bar indicates the least significant difference (LSD) values at p = 0.05.
3.4. Soil NPK status
Tillage practices showed significant variations in the soil nutrient status (N, P, and K; Table 3). Soil-available N was the highest in ZT at all the soil depths (340.0, 316.5, and 295.8 kg ha−1 for soil depths of 0–10, 10–20, and 20–30 cm, respectively) than RT and CT. The ZT practice also recorded significantly higher soil-available P (16.6 kg ha−1) than RT and CT at 0–10 cm soil depth. Whereas, at lower soil depths, 10–20 and 20–30 cm, soil-available P status was not affected due to tillage practices. Significantly higher soil-available K at the 0–10 cm soil depth was found under ZT practice (337.3 kg ha−1) followed by RT. However, CT had the lowest available K (327.5 kg ha−1). However, at lower soil depths (10–20 and 20–30 cm), the effect of tillage on soil-available K content was found to be non-significant. Diversified cropping also brings a significant change in plant-available N at soil depths 0–10 and 10–20, and P and K at only surface soil (0–10 cm depth). Among the diversified systems, the soil under the maize–black gram–buckwheat system had significantly higher plant-available N (336.8 kg ha−1 at 0–10 cm and 312.5 kg ha−1 at 10–20 cm). Concerning P and K, the soil under the maize–black gram–buckwheat system had higher plant-available P and K.
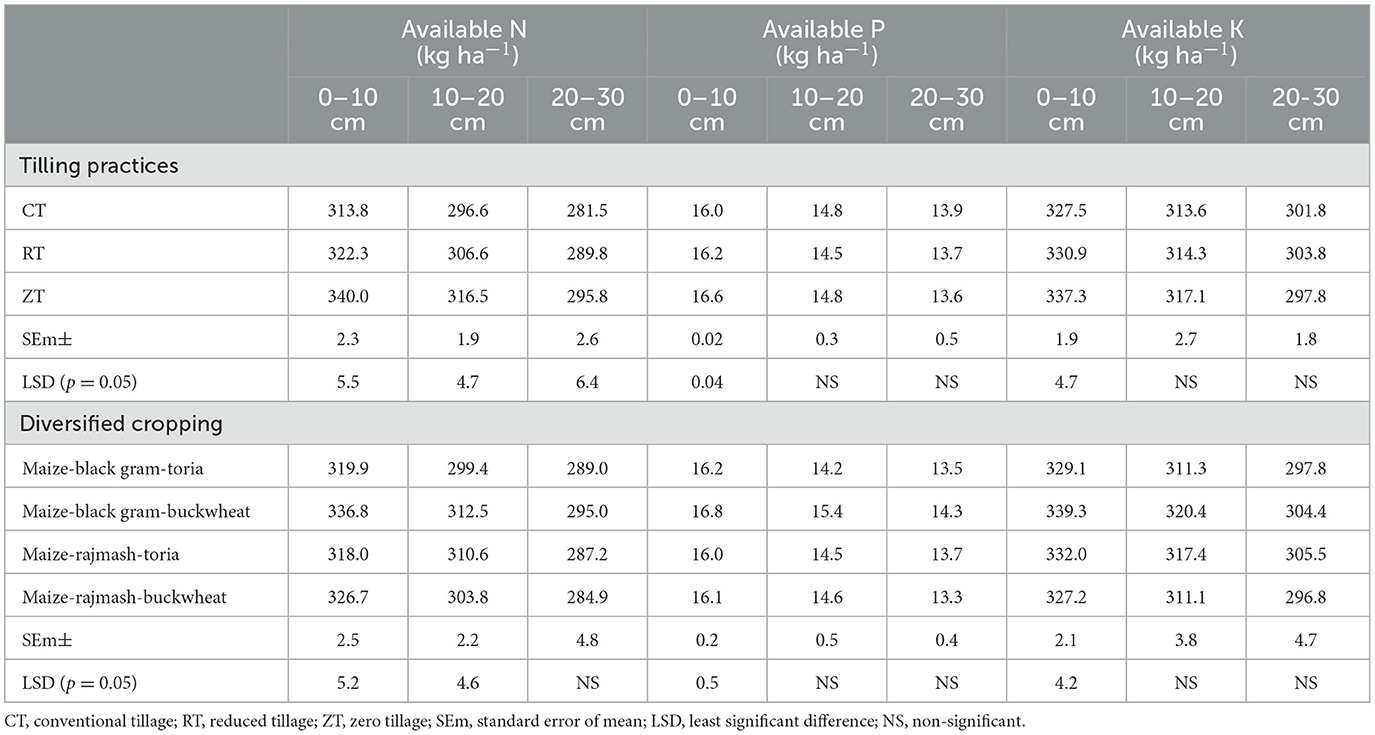
Table 3. Impact of different tillage practices and diversified cropping on available N, P, and K after three cropping cycles.
4. Discussion
Besides the microclimates, comprehensive crop and soil management under conservative agricultural systems regulate the productive capacity of the crops and cropping systems (Das et al., 2020; Raj et al., 2022). The adoption of ZT/RT along with legumes as a component in diversified cropping is an economically feasible and environmentally sustainable production option in many agro-ecoregions (Das et al., 2020; Sayed et al., 2020; Kumar et al., 2021). A positive effect of ZT and RT on crop productivity was reported in the current investigation. The tillage effect was more pronounced in late-rainy season and winter-season crops. ZT increased the productivity of the late-rainy season and winter season crops by 9.6 and 22.3% over the CT system, respectively. The superiority of RT over the ZT and CT systems in terms of maize yield gain might be due to the higher accumulation of SOC and plant-available nutrients in the soil which favored maize growth (Zhang et al., 2015). Under CT, repeated tillage exposed the soil, which may accelerate soil and nutrient erosion during the splendid crop growth stage and result in poor crop productivity (Lal, 2015; Raj et al., 2022). The poor yield of maize under ZT may also be attributed to poor crop establishment during the early-growth stage as compared to RT (Yadav et al., 2018). Furthermore, RT provides a congenial microclimate for the stand establishment of maize crops, which may result in a higher maize yield. Approximately 7%−12% higher maize productivity in coarse loamy soil under RT over ZT and CT was also reported by Das et al. (2020) and Fiorini et al. (2020). Conservation tillage practices like RT and ZT enhance soil microflora and faunal diversity, SOC, and associated soil properties, besides minimizing soil and nutrient erosion (Das et al., 2019; Raj et al., 2022). Higher crop productivity under the ZT/RT system over CT has been reported by several investigators (Islam et al., 2015; Yadav et al., 2020, 2021b). Cropping system diversification also extruded a significant effect on the grain yield of maize and other component crops in the system mode. In the current investigation, maize yield was higher when rajmash was replaced with black gram during the late-rainy season and toria was replaced with buckwheat during the winter season. Rajmash yield was 18.3% higher under the maize–rajmash–buckwheat system as compared to the maize–rajmash–toria system. Similarly, black gram yield was 17.9% higher under the maize–black gram–buckwheat system than the maize–black gram–toria system. The winter crop yield was higher when rajmash was substituted with black gram. These findings suggested that the selection of crops plays a crucial role in determining the system productivity and yield of component crops in the system mode. Crop selection determines the system's economic productivity and yield of different crops accommodated in a year on the same piece of land (Babu et al., 2020b).
Higher crop yield under conservation tillage over the CT system considerably improves the system's production efficiency and economic returns. In the present investigation, ZT and RT saved 26 and 66 US$ ha−1 over the CT system, respectively. Furthermore, the RT and ZT systems registered additional gains of 386 and 570 US$ ha−1 over the CT system, respectively. Similarly, ZT registered a 1.3 times higher B:C ratio over the CT system. This type of trend was due to the higher economic yield of almost all the crops and lesser investment under conservation-effective tillage practices over the CT system. Higher economic returns from different crops under conservation tillage over conventional tillage were also testified by Yadav et al. (2020) and Yadav et al. (2021a). Hence, it can be inferred that the tillage elimination under organic management can be an economically feasible agronomic option. Crop diversification also had a significant impact on overall farm productivity and economic returns. Despite higher production costs, the replacement of toria with buckwheat under the maize–black gram and maize–rajmash systems during the winter season recorded considerably more system yield and economic returns. The replacement of toria with buckwheat under the maize–rajmash system registered 20.36% and 58.8% higher net returns and B:C ratio. Similarly, the replacement of toria with buckwheat had a considerable effect on the crop yield and economic returns of the maize–black gram system. A 10% yield gain under the diversified system over the existing cropping system was also reported by Bennett et al. (2012). Hence, our finding suggested that the inclusion of buckwheat is a more promising option under an intensified maize-based system in the place of toria during the winter season under organic farming in the Eastern Himalayan region of India.
In the current study, the environmental performance of diverse tillage and cropping systems were estimated in terms of GHGI and EEI. The current study inferred that the CT system had 40 and 23.5% higher GHGI over the ZT and RT systems, respectively. The higher GHGI under the CT system may be attributed to the higher energy involvement and fossil fuel combustion during the repeated plowing operations as compared to RT and ZT. The higher GHGI of the CT system over the conservation effective method under similar ecology was also reported by Yadav et al. (2021b). Cropping diversity also had a significant effect on GHGI in the current study. The replacement of toria with buckwheat during the winter season significantly reduced the GHGI. Similarly, the replacement of black gram with rajmash during the late-rainy season under the maize–buckwheat system reduces the GHGI considerably. This inferred that cropping diversity plays a significant role in climate change mitigation. The lower GHGI under the maize–rajmash–buckwheat and maize–black gram–buckwheat systems over the maize–black gram–toria system is primarily due to higher system productivity. Hence, crops must be selected very wisely when designing cropping systems under organic farming. Therefore, low-input demanding and high-productivity crops which contribute less to GHG emissions and have high resource conversion efficiency should be selected. System productivity and GHGI have an inverse relationship (Rathore et al., 2022). The results of this study have the common view that diversified production systems have lower GHGI over the monocropping system. EEI estimation is imperative when evaluating the environmental performance of a designed system. The EEI considers both the financial and ecological dimensions of the production technology (Babu et al., 2020a). CT had the lowest EEI, which indicates that the existing tillage system under the organic farming system of the Indian Himalayan region needs to be shifted toward conservation-effective tilling practices for improving the environmental robustness of the organic farming system. Cropping diversity had a significant impact on EEI. In the present study, the maize–rajmash–buckwheat system had 48.11% higher eco-efficiency than the maize–black gram–toria system. Crops that increase eco-efficiency and reduce GHG emissions can effectively achieve environmentally robust and economically feasible production systems. Thus, it can be emphasized that the adoption of conservation effective tillage with diverse cropping can increase the environmental and economic robustness of organic production systems.
Conservation-effective tillage practices favored the accumulation of SOC which may hasten nutrient accumulation and mobilization. In the present study, ZT registered 4.8%−7.7% higher plant-available N over CT in the entire soil profile up to the 30-cm depth. ZT favors the slow decomposition of organic matter and facilitates the regular supply of plant-available N (Paul et al., 2013; Das et al., 2018). Hence, ZT enhances the N availability in soil over CT and RT. Conservation-effective tillage improves the soil properties (Lal et al., 2018; Sadiq et al., 2021) and enhances soil microbial diversity and activity, thus fueling mineralization and conversion of organic N into plant-available N (López-Garrido et al., 2011; Lal et al., 2018). Furthermore, even a short period of adoption of ZT/RT improved soil health by improving soil biochemical reactions and soil structure. The combined effect of tillage and diversified cropping was also found to be significant in the present investigation at the 0–10 cm soil depth. The significant effect may be attributed due to the higher soil organic matter which increases the soil N status (Feng et al., 2013; Lal et al., 2018). On the contrary, repeated/intensive plowing under CT increases soil compaction and bulk density and reduces SOC, thereby resulting in poor soil conditions (Orzech et al., 2021; Sadiq et al., 2021).
The ZT also improved P availability in soil marginally at a soil depth of 0–10 cm over CT. However, the effect of tillage practice was not significant below the soil depth of 10 cm. An increment in soil-available P after three cropping cycles in sandy clay loam soil was also reported by Lal et al. (2018). ZT promoted SOC accumulation in the surface soil which might alter the immobilized P into the available form (López-Garrido et al., 2011; Yadav et al., 2021a). Of the different systems, higher plant-available P was noticed under the maize–black gram–buckwheat followed by the maize–rajmash–buckwheat system in surface soil (0–10 cm depth). Buckwheat can extract soil P from subsurface soil and accumulate it on the upper surface and thereby increase plant-available P in the soil surface. Higher soil-available P after buckwheat harvest was also recorded by Babu et al. (2018). Furthermore, the constant supply of organic amendments accelerates microbial functions, which reduces occluded P and increases mineralized P (Wang et al., 2012; Das et al., 2018).
In the present study, ZT increases ~2.9% plant-available K in soil over CT after 3 years. Comparatively, ZT increases C input and reduces soil compactness besides improving other soil properties, which in turn increases soil K status (Prasad, 2010; Das et al., 2019). Similarly, among the different cropping systems, cultivation of maize–black gram–buckwheat added more K in the soil through the addition of a different type of residue. An increment in soil K status in response to the addition of a different type of biomass was previously reported by Das et al. (2018). Legumes pumped atmospheric N which may fuel the soil microbial diversity and activities and alleviate the soil nutrients status (Latati et al., 2017). It indicated that legumes, especially black gram and pseudo-cereal-buckwheat, are more justifiable substitutes for rajmash and toria for inclusion in intensified cropping during late-rainy and winter seasons in the Sikkim region of Indian Himalayas, respectively.
5. Policy implications of the study
Agricultural land can store ~1.2 billion tons of C which can reinforce the policy and plans to achieve the goal and targets of the Paris Agreement (Henderson et al., 2022). India announced a five-fold approach to fight climate change during CoP-26 and committed to reducing 1 billion tons of carbon by 2030 and achieving zero-emission targets by 2070. Organic production systems rely upon ecological principles of farming, and the use of synthetic fertilizers and pesticides is prohibited. Hence, it has significantly lower global warming potential than conventional farming. The production of synthetic fertilizers and pesticides are energy-intensive processes. The organic production system requires ~45% less energy and emits ~40% less carbon as compared to conventional farming (Zimmerman, 2020). However, some researchers have reported poor crop yield under organic farming compared to conventional production systems. Conservative agricultural practices can offset GHG emissions besides improving crop productivity and soil quality to meet the growing food demand with minimum environmental footprints (Yadav et al., 2021b; Zhang et al., 2022). Conservation tillage had 26%−31% less global warming potential (Mangalassery et al., 2014) and stored more carbon in the soil than conventional tillage (Yadav et al., 2020). Furthermore, conservational tillage practices have considerably less water footprint than conventional tillage systems (Rahman et al., 2021). The crop diversification portfolio has numerous positive outlooks over monocropping. Crop diversification has a positive impact on farm productivity, profitability, household-level livelihood and nutritional security, and ecosystem services (Mortensen and Smith, 2020). Overall crop diversification can be proposed as a potential risk-coping strategy under current and futuristic climatic scenarios. The findings of the current study suggested that ZT has the potential to reduce the cost of cultivation by 4.9% and increase net income by 24.7% over the CT system under organic management conditions. Furthermore, ZT had 28% less GHGI than CT. Crop selection also played a crucial role in mitigating GHG emissions and increasing farm productivity and profitability under the current study. Hence, we propose that conservation tillage and diversified cropping in organic farming systems can potentially increase soil carbon content, crop productivity, and profitability and curtail GHG emission, which can potentially enhance the economic and environmental benefits of organic production systems. However, the development of cost-effective and practically feasible conservation tillage methods is a great challenge in adopting the ZT and/or RT practices, especially for the organic production scenarios in hill and mountain ecosystems. Diversified cropping is a multiproduct-oriented production system that needs multi specialties and marketing. Capacity building and infrastructure development are also great challenges in practicing crop diversification. Moreover, the development of realistic organic production models is highly individualistic, and location-specific need a proper understanding of available resources. Hence, joint efforts of farmers, researchers, policy planners, and other stakeholders are needed for the wider adoption of conservation agricultural practices under organic farming.
6. Conclusion
Conventional chemical-based farming practices increase food production by many folds to feed the global population but at the same time have a negative impact on natural resources, which amplifies the environmental problems. Organic farming is globally advocated as an environmentally robust agricultural production practice to produce quality food. However, the poor crop yields of organically managed fields over the inorganically managed fields force researchers to devise technologies to improve the productivity of organic agricultural systems without compromising environmental robustness. The findings of the current study proved the hypothesis that conservation-effective tillage and pulses-based diversified cropping will increase productivity, profitability, and eco-efficiency besides improving soil fertility. The study concluded that ZT had significantly higher system net returns (2,881 US$ ha−1) over the CT system besides curtailing the 28% GHGI over the CT system. Furthermore, ZT had 55% higher EEI than the CT system. Concerning crop diversity, the replacement of toria with buckwheat and rajmash with black gram in a maize-based cropping system during winter and late-rainy season in organic farming was found as an economically feasible option to improve the profitability and environmental robustness of organic farming in the Eastern Himalayan region. Furthermore, the maize–rajmash–buckwheat and the maize–black gram–buckwheat systems have higher EEI and lesser GHGI over other diversified systems. Hence, the findings recommended that the implementation of ZT/RT under diversified maize-based systems is a productive, environmentally robust, and soil-supportive practice for obtaining higher economic returns under the organic farming condition of the Indian Himalayas and other similar ecoregions.
Data availability statement
The original contributions presented in the study are included in the article/supplementary material, further inquiries can be directed to the corresponding authors.
Author contributions
SB and RA: visualization, experimentation data curation, supervision, and project administration. RS: conceptualization, experimentation, data analysis, writing of original and first draft, and review and editing of the final draft. SR and AD: data curation, review, and editing. VSh, JL, SK, and VSi: review and editing. OW: review and editing and referencing. All authors contributed to the article and approved the submitted version.
Acknowledgments
The authors are thankful to the Director of the ICAR Research Complex, Umiam, India for providing the research amenities to conduct this research. The authors also express their gratitude to the technical staff and senior research fellows who helped in field data recording and laboratory analyses.
Conflict of interest
The authors declare that the research was conducted in the absence of any commercial or financial relationships that could be construed as a potential conflict of interest.
The reviewer MC declared a shared parent affiliation with the authors SB, RS, RA, SR, AD, JL, and VSh to the handling editor at the time of review.
Publisher's note
All claims expressed in this article are solely those of the authors and do not necessarily represent those of their affiliated organizations, or those of the publisher, the editors and the reviewers. Any product that may be evaluated in this article, or claim that may be made by its manufacturer, is not guaranteed or endorsed by the publisher.
References
Ansari, M. A., Babu, S., Choudhary, J., Ravisankar, N., and Panwar, A. S. (2022). Soil quality restoration and yield stabilization in acidic soils of north eastern Himalayas: five years impact of green manuring and crop residue management. Front. Environ. Sci. 10, 940349. doi: 10.3389/fenvs.2022.940349
Avasthe, R. K., Babu, S., Singh, R., Yadav, G. S., and Kumar, A. (2020). Productivity and profitability assessment of organically grown vegetables embedded in rice based cropping sequences in Sikkim Himalayas, North East India. J. Environ. Biol. 41, 111–117. doi: 10.22438/jeb/41/1/MRN-1146
Babu, S., Mohapatra, K. P., Das, A., Yadav, G. S., Tahasildar, M., Singh, R., et al. (2020a). Designing energy-efficient, economically sustainable and environmentally safe cropping system for the rainfed maize–fallow land of the Eastern Himalayas. Sci. Total Environ. 722, 137874. doi: 10.1016/j.scitotenv.2020.137874
Babu, S., Rathore, S. S., Singh, R., Kumar, S., Singh, V. K., Yadav, S. K., et al. (2022). Exploring agricultural waste biomass for energy, food and feed production and pollution mitigation: a review. Bioresour. Technol. 360, 127566. doi: 10.1016/j.biortech.2022.127566
Babu, S., Singh, R., Avasthe, R. K., Yadav, G. S., Chettri, T. K., and Rajkhowa, D. J. (2016). Productivity, profitability and energetics of buckwheat (Fagopyrum sp.) cultivars as influenced by varying levels of vermicompost in acidic soils of Sikkim Himalayas, India. Indian J. Agric. Sci. 86, 844–848.
Babu, S., Singh, R., Avasthe, R. K., Yadav, G. S., Mohapatra, K. P., Selvan, T., et al. (2020b). Soil carbon dynamics in Indian Himalayan intensified organic rice-based cropping sequences. Ecol. Indic. 114. doi: 10.1016/j.ecolind.2020.106292
Babu, S., Yadav, G. S., Singh, R., Avasthe, R. K., Das, A., Mohapatra, K. P., et al. (2018). Production technology and multifarious uses of buckwheat (Fagopyrum spp.): a review. Indian J. Agron.63, 415–427.
Bennett, A. J., Bending, G. D., Chandler, D. S., and Mills, P. (2012). Meeting the demand for crop production: the challenge of yield decline in crops grown in short rotations. Biol. Rev. Camb. Philos. Soc.87, 52–51. doi: 10.1111/j.1469-185X.2011.00184.x
Das, A., Basavaraj, S., Layek, J., Gandhiji Idapuganti, R., Lal, R., Rangappa, K., et al. (2019). Can conservation tillage and residue management enhance energy use efficiency and sustainability of rice-vegetable pea systems in the Eastern Himalayas? Arch. Agron. Soil Sci. 66, 830–846. doi: 10.1080/03650340.2019.1639157
Das, A., Layek, J., Gi, R., Basavaraj, S., Lal, R., Krishnappa, R., et al. (2020). Conservation tillage and residue management improves soil properties under upland rice–rapeseed system in subtropical eastern Himalayas. Land Degrad Dev. 31, 1775–1791. doi: 10.1002/ldr.3568
Das, A., Lyngdoh, D., Ghosh, P. K., Lal, R., Layek, J., and Ramkrushna, G. I. (2018). Tillage and cropping sequence effect on physico-chemical and biological properties of soil in Eastern Himalayas, India. Soil Till. Res. 180, 182–193. doi: 10.1016/j.still.2018.03.005
de Ponti, T., Rijk, B., and van Ittersum, M. K. (2012). The crop yield gap between organic and conventional agriculture. Agric. Syst. 108, 1–9. doi: 10.1016/j.agsy.2011.12.004
Feng, W., Plante, A. F., and Six, J. (2013). Improving estimates of maximal organic carbon stabilization by fine soil particles. Biogeochemistry 112, 81–93. doi: 10.1007/s10533-011-9679-7
Fiorini, A., Maris, S. C., Abalos, D., Amaducci, S., and Tabaglio, V. (2020). Combining no-till with rye (Secale cereale L.) cover crop mitigates nitrous oxide emissions without decreasing yield. Soil Till. Res. 196, 104442. doi: 10.1016/j.still.2019.104442
Gomez, K. A., and Gomez, A. A. (1984). A Statistical Procedure for Agricultural Research, 2nd ed. New York, NY: International Rice Research Institute, John Wiley, and Sons.
Henderson, B., Lankoskii, J., Flynni, E., Sykesii, A., Payenii, F., and MacLeodii, M. (2022). Soil Carbon Sequestration by Agriculture: Policy Options. OECD Food, Agriculture and Fisheries Papers, No. 174. Paris: OECD Publishing.
Henry, B., Murphy, B., and Cowie, A. (2018). Sustainable Land Management for Environmental Benefits and Food Security. A Synthesis Report for the GEF, 85.
Islam, M. S., Ahmed, M. K., Raknuzzaman, M., Habibullah-Al-Mamun, M., and Masunaga, S. (2015). Metal speciation in sediment and their bioaccumulation in fish species of three urban rivers in Bangladesh. Arch. Environ. Contam. Toxicol. 68, 92–106. doi: 10.1007/s00244-014-0079-6
Knapp, S., and van der Heijden, M. G. A. (2018). A global meta-analysis of yield stability in organic and conservation agriculture. Nat. Commun.9, 3632. doi: 10.1038/s41467-018-05956-1
Kumar, M., Mitra, S., Mazumdar, S. P., Majumdar, B., Saha, A. R., Singh, S. R., et al. (2021). Improvement of soil health and system productivity through crop diversification and residue incorporation under jute-based different cropping systems. Agronomy 11, 1622. doi: 10.3390/agronomy11081622
Lal, R. (2015). Sequestering carbon and increasing productivity by conservation agriculture. J. Soil Water Conserv. 70, 55A−2A. doi: 10.2489/jswc.70.3.55A
Lal, R., Smith, P., Hermann, F. J., Lehmann, W. M. J., Nair, P. K. R., McBratney, A. B., et al. (2018). The carbon sequestration potential of terrestrial ecosystems. J. Soil Water Conserv. 73, 145–152. doi: 10.2489/jswc.73.6.145A
Latati, M., Aouiche, A., Tellah, S., Laribi, A., Benlahrech, S., Kaci, G., et al. (2017). Intercropping maize and common bean enhances microbial carbon and nitrogen availability in low phosphorus soil under Mediterranean conditions. Eur. J. Soil Biol. 80, 9–18. doi: 10.1016/j.ejsobi.2017.03.003
López-Garrido, R., Madejón, E., Murillo, J. M., and Moreno, F. (2011). Short and long-term distribution with depth of soil organic carbon and nutrients under traditional and conservation tillage in a Mediterranean environment (southwest Spain). Soil Use Manag. 27, 177–185. doi: 10.1111/j.1475-2743.2011.00329.x
Mangalassery, S., Sjögersten, S., Sparkes, D. L., Sturrock, C. J., Craigon, J., Mooney, S. J., et al. (2014). To what extent can zero tillage lead to a reduction in greenhouse gas emissions from temperate soils? Sci. Rep. 4, 4586. doi: 10.1038/srep04586
Molotoks, A., Stehfest, E., Doelman, J., Albanito, F., Fitton, N., Dawson, T. P., et al. (2018). Global projections of future cropland expansion to 2050 and direct impacts on biodiversity and carbon storage. Glob. Chang. Biol. 24, 5895–5908. doi: 10.1111/gcb.14459
Mortensen, D. A., and Smith, R. G. (2020). Confronting barriers to cropping system diversification. Front. Sustain. Food Syst. 4, 564197. doi: 10.3389/fsufs.2020.564197
Orzech, K., Wanic, M., and Załuski, D. (2021). The effects of soil compaction and different tillage systems on the bulk density and moisture content of soil and the yields of winter oilseed rape and cereals. Agriculture 11, 666. doi: 10.3390/agriculture11070666
Panwar A. S. Ansari M. A. Ravisankar N. Babu S. Prusty A. K. Ghasal P. C. (2022), Effect of organic farming on the restoration of soil quality, ecosystem services, productivity in rice–wheat agro-ecosystems. Front. Environ. Sci. 10, 972394. doi: 10.3389/fenvs.2022.972394.
Paul, F., Barrand, N. E., Baumann, S., Berthier, E., Bolch, T., Casey, K., et al. (2013). On the accuracy of glacier outlines derived from remote-sensing data. Ann. Glaciol. 54, 171–182. doi: 10.3189/2013AoG63A296
Prasad, J. (2010). Forms of potassium in shallow soils of different origin and land uses in Nagpur district of Maharashtra. J. Indian Soc. Soil Sci. 58, 327–330.
Prasad, R., Shivay, Y. S., Kumar, D., and Sharma, S. N. (2006). Learning by Doing Exercise in Soil Fertility- A Practical Manual for Soil Fertility. New Delhi, India: Division of Agronomy, IARI, 68.
Rahman, M. D. M., Aravindakshan, S., Hoque, M. A., Rahman, M. A., Gulandaz, M. A, Rahman, J., et al. (2021). Conservation tillage (CT) for climate-smart sustainable intensification: assessing the impact of CT on soil organic carbon accumulation, greenhouse gas emission and water footprint of wheat cultivation in Bangladesh. Environ. Sustain. Indic. 10. doi: 10.1016/j.indic.2021.100106
Raj, R., Das, T. K., Pankaj, S., Banerjee, T., Ghosh, A., Bhattacharyya, R., et al. (2022). Co-implementation of conservation tillage and herbicides reduces weed and nematode infestation and enhances the productivity of direct-seeded rice in North-western Indo-Gangetic Plains. Front. Sustain. Food Syst. 6, 1017013. doi: 10.3389/fsufs.2022.1017013
Rathore, S. S., Babu, S., Shekhawat, K., Singh, R., Yadav, S. K., Singh, V. K., et al. (2022). Designing energy cum carbon-efficient environmentally clean production system for achieving green economy in agriculture. Sustain. Energy Technol. Assess. doi: 10.1016/j.seta.2022.102190
Sadiq, M., Li, G., Rahim, N., and Tahir, M. M. (2021). Sustainable conservation tillage technique for improving soil health by enhancing soil physicochemical quality indicators under wheat mono-cropping system conditions. Sustainability 13, 8177. doi: 10.3390/su13158177
Sayed, A., Sarker, A., Jang-Eok, K., Mashiur, R., Mahmud, M. D., and Golam, A. (2020). Environmental sustainability and water productivity on conservation tillage of irrigated maize in red brown terrace soil of Bangladesh. J. Saudi Soc. Agric. Sci 19, 276–284. doi: 10.1016/j.jssas.2019.03.002
Singh, R., Babu, S., Avasthe, R. K., Yadav, G. S., Das, A., Mohapatra, K. P., et al. (2021). Crop productivity, soil health, and energy dynamics of Indian Himalayan intensified organic maize-based systems. Int. Soil Water Conserv. Res. 9, 260–270. doi: 10.1016/j.iswcr.2020.11.003
Tubiello, F. N., Condor-Golec, R. D., Salvatore, M., Piersante, A., Federici, S., Ferrara, A., et al (2015). Estimating Greenhouse Gas Emissions in Agriculture: A Manual to addrESS data Requirements for Developing Countries. Rome: Food and Agriculture Organization of the United Nations. Available online at: www.fao.org/publications (accessed January 10, 2023).
Wang, S. X., Liu, G. R., Luo, Q. Q., Liu, X. M., Wang, P., Xia, W. J., et al. (2012). Research advance in phosphorous accumulation and its loss potential in paddy soils. Acta Agric. Jiangxi 24, 98–103.
Yadav, G. S., Babu, S., Das, A., Datta, M., Mohapatra, K. P., Singh, R., et al. (2021a). Productivity, soil health, and carbon management index of Indian Himalayan intensified maize-based cropping systems under live mulch-based conservation tillage practices. Field Crops Res. 264, 108080. doi: 10.1016/j.fcr.2021.108080
Yadav, G. S., Babu, S., Das, A., Mohapatra, K. P., Singh, R., Avasthe, R. K., et al. (2020). No till and mulching enhance energy use efficiency and reduce carbon footprint of a direct-seeded upland rice production system. J. Clean. Prod. 271, 122700. doi: 10.1016/j.jclepro.2020.122700
Yadav, G. S., Das, A., Kandpal, B. K., Babu, S., Lal, R., Datta, M., et al. (2021b). The food-energy-water-carbon nexus in a maize-maize-toria cropping sequence of the Indian Himalayas: an impact of tillage-cum-live mulching. Renew. Sustain. Energy Rev. doi: 10.1016/j.rser.2021.111602
Yadav, G. S., Lal, R., Meena, R. S., Datta, M., Babu, S., Das, A., et al. (2017). Energy budgeting for designing sustainable and environmentally clean/safer cropping systems for rainfed rice fallow lands in India. J. Clean. Prod. 158, 29–37. doi: 10.1016/j.jclepro.2017.04.170
Yadav, G. S., Saha, P., Babu, S., Das, A., Layek, J., and Debnath, C. (2018). Effect of no-till and raised-bed planting on soil moisture conservation and productivity of summer maize (Zea mays) in Eastern Himalayas. Agric. Res. 7, 300–310. doi: 10.1007/s40003-018-0308-8
Yadav, S. K., Babu, S., Yadav, M. K., Singh, Y., and Singh, K. (2014). Profitability of high-value crops with organic nitrogen sources under rice (Oryza sativa) based cropping sequence. Indian J. Agric. Sci. 84, 343–348.
Zhang, H., Hobbie, E. A., Feng, P., Niu, L., and Hu, K. (2022). Can conservation agriculture mitigate climate change and reduce environmental impacts for intensive cropping systems in North China Plain? Sci. Total Environ. 806(Pt 3), 151194. doi: 10.1016/j.scitotenv.2021.151194
Zhang, J., Wang, Y., and Li, F. C. (2015). Soil organic carbon and nitrogen losses due to soil erosion and cropping in a sloping terrace landscape. Soil Res. 53, 87–96. doi: 10.1071/SR14151
Zimmerman, A. (2020). Columbia Climate School. So, Is Organic Food Actually More Sustainable? Available online at: https://news.climate.columbia.edu/2020/02/05/organic-sustainable-food/#:~:text=Research%20has%20shown%20that%20organic,always%20the%20most%20sustainable%20option (accessed January 10, 2023).
Keywords: buckwheat, crop productivity, economic returns, Himalayas, soil nutrients, sustainability
Citation: Babu S, Singh R, Avasthe R, Rathore SS, Kumar S, Das A, Layek J, Sharma V, Wani OA and Singh VK (2023) Conservation tillage and diversified cropping enhance system productivity and eco-efficiency and reduce greenhouse gas intensity in organic farming. Front. Sustain. Food Syst. 7:1114617. doi: 10.3389/fsufs.2023.1114617
Received: 02 December 2022; Accepted: 31 January 2023;
Published: 02 March 2023.
Edited by:
Sudhakar Srivastava, Banaras Hindu University, IndiaReviewed by:
Muhammad Imran Ghani, Guizhou University, ChinaManoj Chaudhary, Indian Council of Agricultural Research (ICAR), India
Asha Ram, Central Agroforestry Research Institute (CAFRI), India
Copyright © 2023 Babu, Singh, Avasthe, Rathore, Kumar, Das, Layek, Sharma, Wani and Singh. This is an open-access article distributed under the terms of the Creative Commons Attribution License (CC BY). The use, distribution or reproduction in other forums is permitted, provided the original author(s) and the copyright owner(s) are credited and that the original publication in this journal is cited, in accordance with accepted academic practice. No use, distribution or reproduction is permitted which does not comply with these terms.
*Correspondence: Sanjay Singh Rathore, sanjayrathorears@gmail.com; Subhash Babu, subhiari@gmail.com; Raghavendra Singh, raghavenupc@gmail.com