- 1Institute for Parasitology, University of Veterinary Medicine Hannover, Hannover, Germany
- 2Zoo Osnabrueck, Osnabrueck, Germany
Captive as well as free-ranging wolves, which are currently recolonizing Germany, may harbor a variety of gastrointestinal parasites. This study investigated endoparasites in captive European gray wolves (Canis lupus lupus) using coproscopical methods. Fecal samples were collected monthly between October 2012 and November 2013 from 18 wolf enclosures in 14 German zoological gardens, representing 72 individual wolves. In total, 1,041 fecal samples including 26 bulk samples were analyzed by the sedimentation and flotation method. The most frequently detected egg morphotypes included five nematodes [Ancylostomatidae (Ancylostoma or Uncinaria spp.), Toxocara canis, Toxascaris leonina, Trichuris vulpis, and Capillaria/Eucoleus spp.], one cestode (Taeniidae) and one trematode (Alaria alata). 44.76% of all samples were positive for at least one of these egg morphotypes. Overall, Ancylostomatidae showed the highest frequency (30.84% of all samples), followed by Capillaria/Eucoleus spp. (19.88%), Toxocara canis (5.19%), taeniids (3.75%), Trichuris vulpis and Alaria alata (3.65% each), and Toxascaris leonina (1.25%). As fecal samples were collected from the environment and could not be assigned to individual wolves, sample results were combined per zoo and month. General linear mixed models were employed to analyze the effect of season and management factors on the occurrence of Ancylostomatidae, Capillaria/Eucoleus spp., Toxocara canis and taeniids. No statistically significant effect of season was found, whereas anthelmintic treatment negatively affected Ancylostomatidae egg excretion. Detected parasites and their prevalences are comparable to previous studies on wolf parasitism conducted elsewhere in Europe. As many of the most prevalent helminths are of zoonotic importance, routine anthelmintic treatment of captive wolves should be recommended.
Introduction
Since 2000, the European gray wolf (Canis lupus lupus) has begun to recolonize Germany. The first packs returned from Poland and have established sustainable populations in the federal states of Brandenburg, Mecklenburg–Western Pomerania, Lower Saxony, Saxony, and Saxony-Anhalt (1). In addition, wolves are kept in many zoological gardens, with European gray wolves (Canis lupus lupus), eastern timber wolves (Canis lupus lyacon), and tundra wolves (Canis lupus albus) as the most commonly kept subspecies (2).
Wolves may harbor a variety of parasites with relevance for domestic animals and even human health. For example, both wild and domestic carnivores may act as definitive hosts for cestodes of the family Taeniidae, which may be responsible for morbidity and mortality in their intermediate hosts. Taenia ovis, Taenia hydatigena, and Taenia multiceps, for example, are causative agents of cysticercosis in domestic ruminants, which may compromise herd health and lead to production losses as affected organs or carcasses need to be condemned (3). Echinoccocus granulosus and Echinococcus multilocularis, the causative agents of cystic and alveolar echinococcosis, respectively, are of considerable zoonotic importance, causing potentially fatal disease in humans (4). Furthermore, juvenile stages of the trematode Alaria alata reside in muscle and fatty tissue of paratenic hosts, including humans (5). Zoonotic nematodes of canines include, amongst others, the hookworm Ancylostoma caninum, which may cause the so-called larva migrans cutanea in humans, and the roundworm Toxocara canis, whose infective larvae are responsible for several forms of human toxocarosis, namely larva migrans visceralis, ocular toxocarosis, neurotoxocarosis, and covert toxocarosis (6). Furthermore, wolves may be hosts to zoonotic protozoa, e.g., Giardia duodenalis (7, 8), which may cause enteritis in humans.
Since humans are in quite close contact with animals and their feces in zoos or wildlife parks, captive wolves may pose a potential health risk to animal caretakers. However, also the captive wolves themselves may suffer from parasite infections. Thus, the aim of this study was to describe the helminth fauna of captive European gray wolves (Canis lupus lupus) in Germany. So far, only a few studies exist on the endoparasite fauna of captive wolves in Central Europe. In one study, endoparasites of North American large carnivores, including wolves, in German zoological gardens were described based on zoo health records (9). Another study compared the endoparasite fauna of wild and captive wolves in Poland (10). However, to the best of our knowledge, no study has investigated year-round endoparasite burdens of captive European gray wolves in Germany to assess seasonal patterns. Seasonal differences in helminth egg excretion have been described in several canids, including wild foxes (11) and domestic dogs (12). In wild wolves, seasonal parasite patterns have been mainly attributed to variations in resource use or the presence of susceptible pups (7). In addition, changes in host immunity or the endocrinological status, e.g., related to reproduction, might affect parasite excretion patterns (12); however, evidence regarding this phenomenon in wolves is lacking. To investigate whether or not seasonal patterns in helminth egg excretion occur in captive wolves, a generalized linear-mixed model (GLMM) analysis was included in this study. Furthermore, questionnaire-based data were analyzed to assess possible management factors influencing egg excretion.
Materials and Methods
Study Design and Fecal Sample Collection
Fourteen public and private zoos and wildlife parks (hereafter referred to as zoos) keeping European gray wolves (Canis lupus lupus) distributed across Germany participated in this study. Fecal samples were collected from the ground of the enclosures in monthly intervals from October 2012 to November 2013. Only fresh fecal samples were collected as determined by the presence of a mucus layer and a characteristic smell. As wolves lived in groups and could not be separated, fecal samples could not be assigned to single individuals. Collected samples were transported to the Institute of Parasitology of the University of Veterinary Medicine Hannover for coproscopical examinations.
To gain information on the enclosure size, number, age, and sex of the wolves living in the fenced area, type of husbandry (single or mixed-species exhibit), potential offspring in 2012 or 2013, origin and composition of the wolves’ food, antiparasitic treatment regime and whether dogs were allowed in the park or not, a questionnaire was provided to the zoos.
Coproscopical Examinations
Fecal samples sent by the zoos were weighed and preserved at −20°C until coproscopical examination. First, 5 g of each sample was screened for the presence of parasite eggs by use of the combined sedimentation-flotation method using zinc sulfate solution [ZnSO4, specific gravity (SG) 1.30] as flotation solution and centrifugation at 450 × g to enhance flotation as described by Becker et al. (13). If parasite eggs were detected, the McMaster method was carried out to determine the number of eggs per gram feces (EPG). An amount of 4 g feces was processed with 60 ml flotation solution [saturated NaCl solution (SG 1.2) for hookworm and roundworm eggs; zinc sulfate solution (SG 1.3) for trichurid, capillariid, taeniid, and A. alata eggs] as described by Becker et al. (13) except that four McMaster counting grids were examined. Thus, a sensitivity of 25 EPG was achieved. Presence of coccidian oocysts was noted but not included in subsequent analyses since unsporulated oocysts may not necessarily originate from the wolves, but may represent intestinal passengers originating from food animals, and, furthermore, differentiation of sporulated oocysts was not sufficiently possible due to degradations following the freeze-thaw process.
Statistical Analyses
Generalized linear-mixed models with binomial error structure and logit link function were used to analyze which factors influenced the probability of presence/absence of the most prevalent parasite species (Ancylostomatidae, Capillaria/Eucoleus spp., Taeniidae, and Toxocara canis) in a zoological garden per month (N = 156). The models were implemented in the R environment (14) using the “glmer” function, package lmerTest (15). Season in the respective study year (autumn 2012: October–November 2012; winter 2013: December 2012–February 2013; spring 2013: March–May 2013; summer 2013: June–August 2013; autumn 2013: September–November 2013), the number of samples the zoo contributed per month and anthelmintic treatment (yes/no) were included as fixed factors and zoo identity as a random effect. Because Taeniidae are transmitted to carnivores by ingestion of infected intermediate hosts, the fact whether the zoo fed game or meat from other wildlife (e.g., zoo-bred or roadkilled) was included as an explanatory variable for this parasite. Each full model was compared to a null model comprising only the random effect in a likelihood ratio test using the R-function ANOVA with the argument “test” set to “Chisq.” Statistical significance was inferred if the specific P-value of the factor as well as the P-value of the likelihood ratio test were ≤0.05. Multiple comparisons (Tukey contrasts) between all 5 levels of season (autumn 2012, winter 2012/2013, spring 2013, summer 2013, and autumn 2013) were performed using the function “glht,” package multcomp (16) with single-step P-value adjustment.
Results
Captive Wolf Management
Among the 14 participating zoological gardens, the study population comprised 72 European gray wolves, inhabiting 18 enclosures. Enclosure size ranged from 500 to 11,000 m2. Wolves lived in packs of 2–10 individuals, and the space available per wolf showed a large variation from 265 to 2,857 m2. Only two zoos kept wolves in mixed-species exhibits, together with two brown bears each. In all zoos, wolves were fed one to six times a week on meat originating from slaughterhouses (horse and/or beef). Furthermore, 11 zoos additionally fed wild boar and different deer species from roadkills, as well as excess animals from the zoo itself. In one zoo, wolves also received a small amount of fish. Dogs were allowed to enter all zoos; thus, the impact of this management factor on endoparasite infection of the captive wolves could not be evaluated statistically.
Results of Coproscopical Examinations
In total, 1,041 wolf fecal samples were analyzed. The number of samples per zoo and month ranged from 0 to 25. In 26 cases, bulk samples (i.e., a single mixed sample per zoo and month) were collected. Only in March 2013, all 14 participating zoos contributed samples. In October 2013 and September 2013, 10 zoos contributed samples, and in the remaining study months, the number of contribution zoos was once again lower. Overall, 156 monthly data points (parasite presence/absence on zoo level) resulted from the study period of 14 months.
In total, 23 different parasite egg morphotypes were detected, some of which are considered as gastrointestinal passengers. Seven helminth egg morphotypes were detected with high frequency, including five nematodes (Toxocara canis, Toxascaris leonina, Capillaria/Eucoleus spp., Trichuris vulpis, and Ancylostomatidae [Ancylostoma or Uncinaria spp.]), one cestode (Taeniidae), and one trematode (Alaria alata). A percentage rate of 44.76 of all fecal samples was positive for at least one of these egg morphotypes. Overall, Ancylostomatidae showed the highest frequency (30.84% of all samples), followed by Capillaria/Eucoleus spp. (19.88%), T. canis (5.19%), taeniids (3.75%), T. vulpis and A. alata (3.65% each), and T. leonina (1.25%). Figure 1 shows monthly egg detection frequencies of these parasite eggs among all participating zoos, while Tables 1–7 list monthly egg detection frequencies and EPG ranges among the individual samples from each zoo. The monthly number of positive zoos is pictured in Figure S1 in Supplementary Material. The total egg detection rate for respective parasite eggs per zoo is shown in Figure 2. In addition to above mentioned egg morphotypes, eggs of Spirocerca lupi (0.58%), Opisthorchis spp. (0.19%), and Diphyllobothrium latum (0.10%) were detected. Furthermore, first-stage larvae of Angiostrongulus vasorum were identified; however, the detected frequency of 0.67% is certainly underestimated as the applied sedimentation-flotation method is not reliable for detection of lungworm larvae. Besides eggs of canine-associated parasites, a variety of eggs originating from food animals were found as gastrointestinal passengers: Porrocaecum spp. (2.59%), Parascaris equorum (0.86%), Echinuria spp. (0.38%), Nematodirus spp. (0.48%), Heterakis spp. (0.38%), Moniezia spp. (0.29%), Ascaris suum (0.19%), Acanthocephalus spp. (0.10%), and Paramphistomum spp. (0.10%). Furthermore, in 5.86% of the fecal samples, eggs of Fasciola hepatica were identified. However, it remains unknown whether the wolves, the food animals, or both served as the parasite host. Similarly, coccidian oocysts, which were present in 4.90% of the samples, could not be attributed to the wolves or food animals as they were unsporulated or could not be sufficiently differentiated at their sporulated stage due to freeze-thaw degradation.
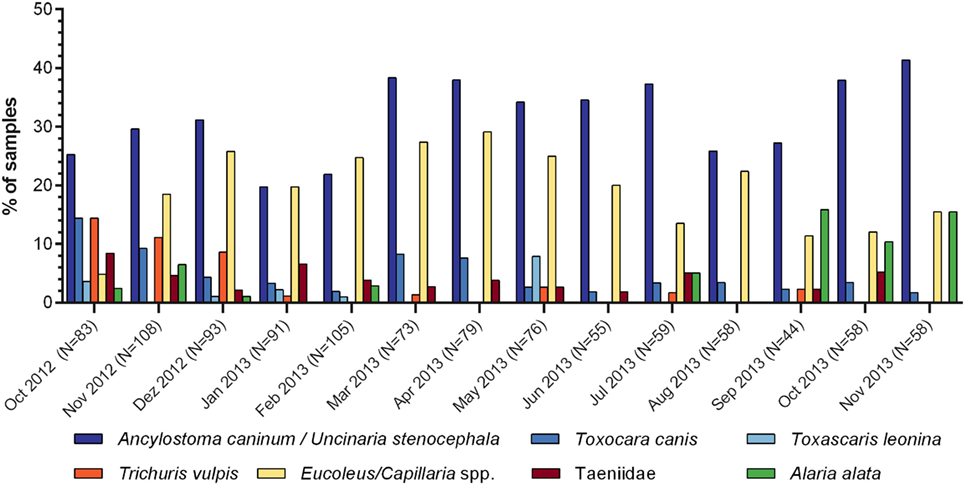
Figure 1. Monthly detection frequencies of Ancylostomatidae, T. canis, T. leonina, T. vulpis, Capillaria/Eucoleus spp., Taeniidae, and A. alata eggs in fecal samples from captive European gray wolves in Germany. Monthly sample size is indicated in brackets.
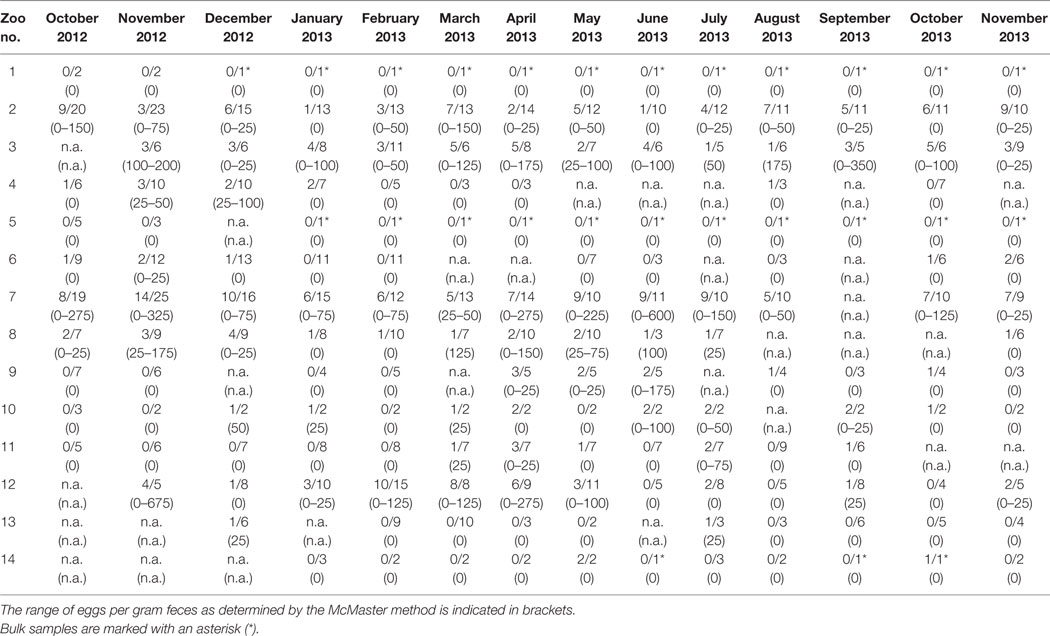
Table 1. Monthly hookworm (Ancylostomatidae) egg detection frequency (number of positive samples/number of contributed samples) per zoo.
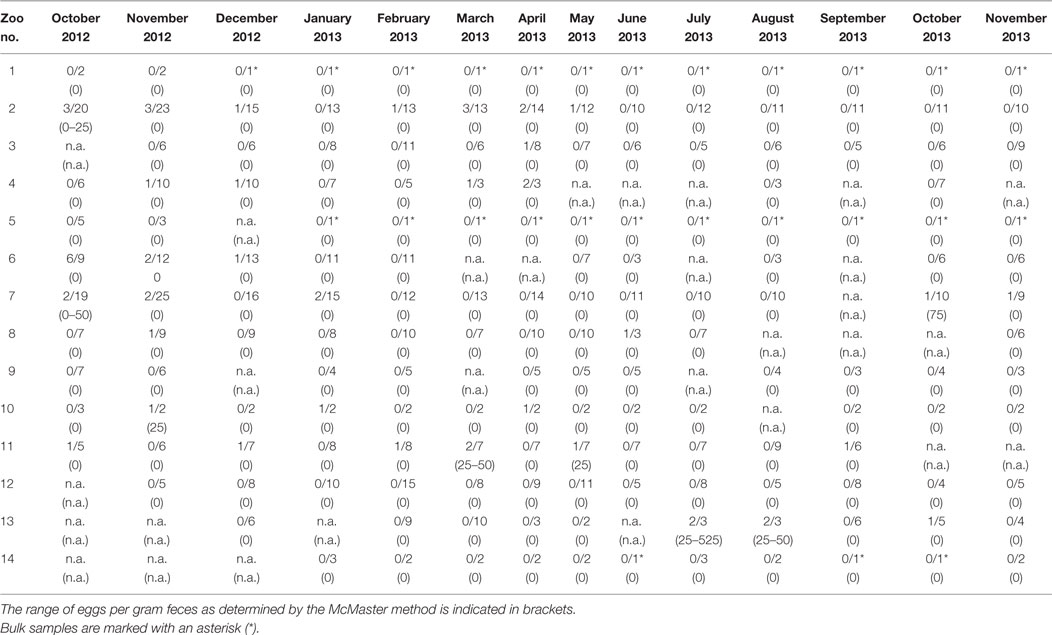
Table 2. Monthly Toxocara canis egg detection frequency (number of positive samples/number of contributed samples) per zoo.
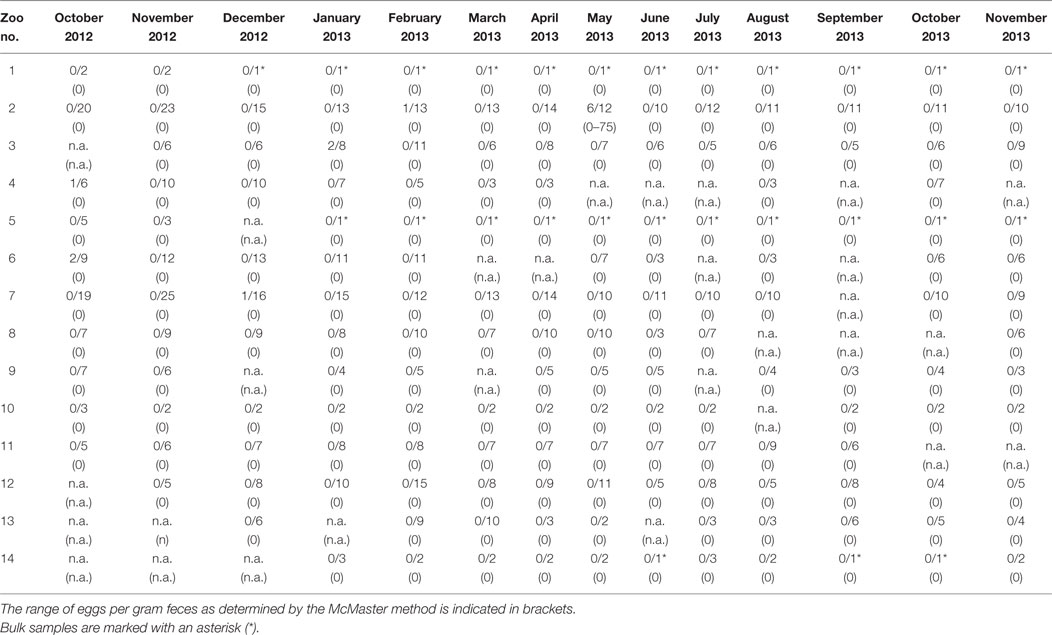
Table 3. Monthly Toxascaris leonina egg detection frequency (number of positive samples/number of contributed samples) per zoo.
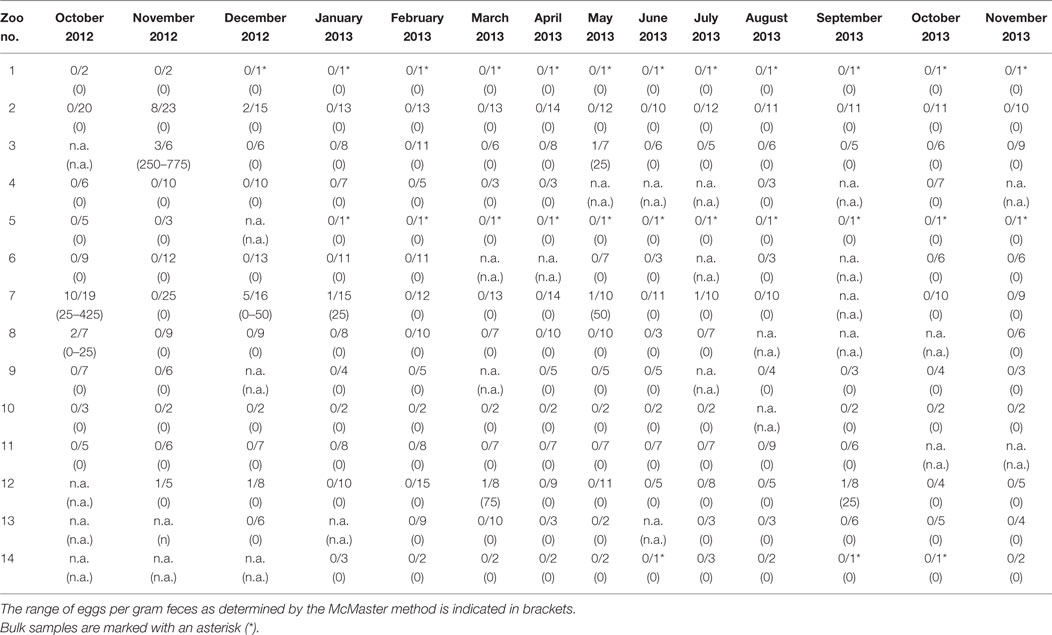
Table 4. Monthly Trichuris vulpis egg detection frequency (number of positive samples/number of contributed samples) per zoo.
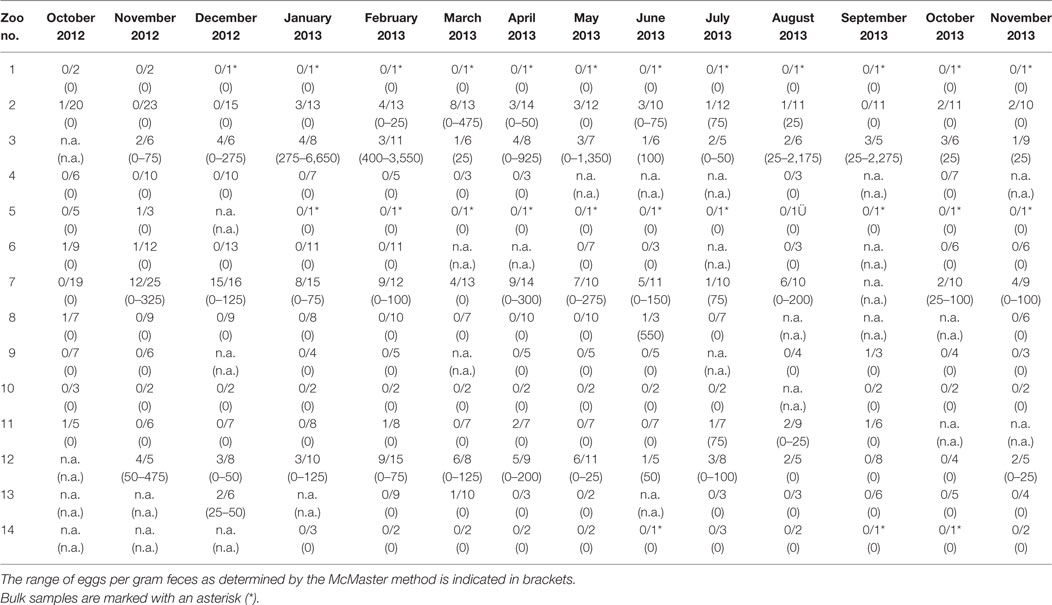
Table 5. Monthly Capillaria/Eucoleus spp. egg detection frequency (number of positive samples/number of contributed samples) per zoo.
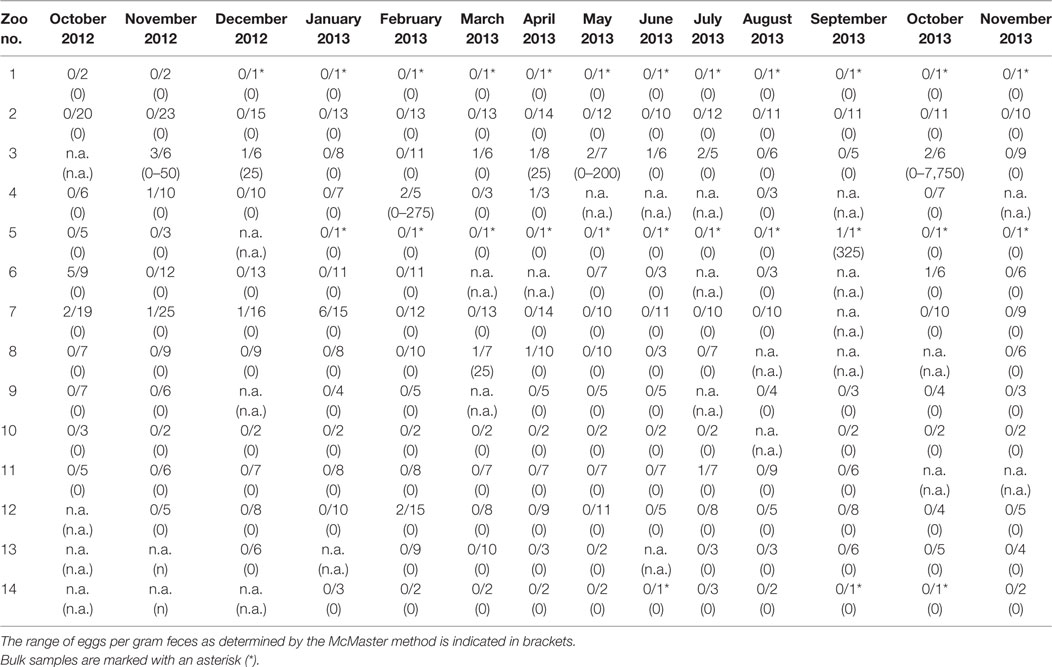
Table 6. Monthly taeniid egg detection frequency (number of positive samples/number of contributed samples) per zoo.
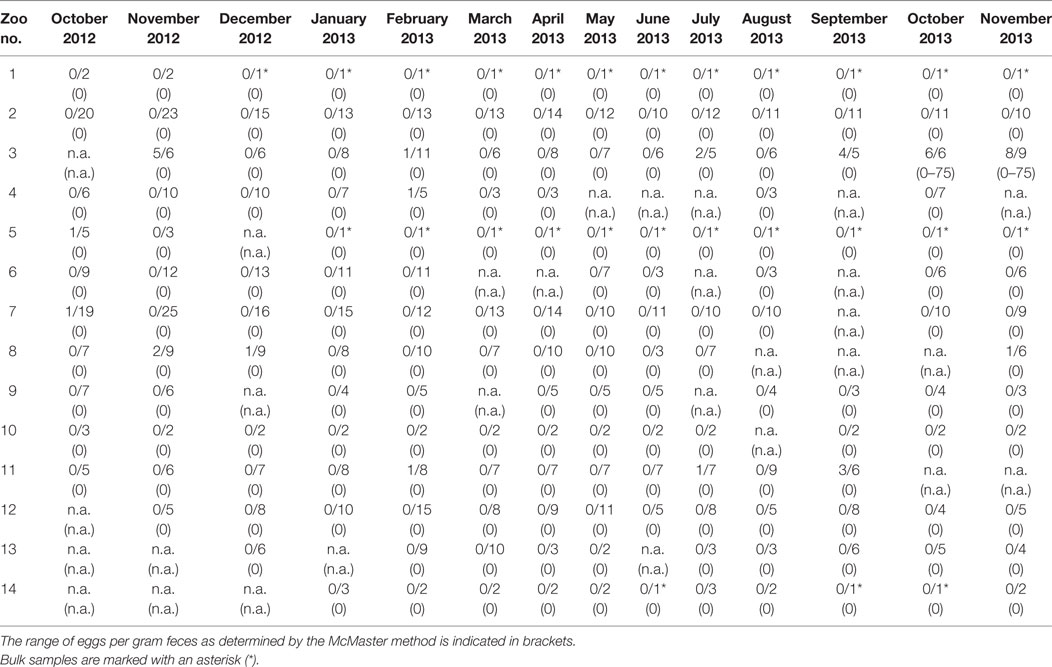
Table 7. Monthly Alaria alata egg detection frequency (number of positive samples/number of contributed samples) per zoo.
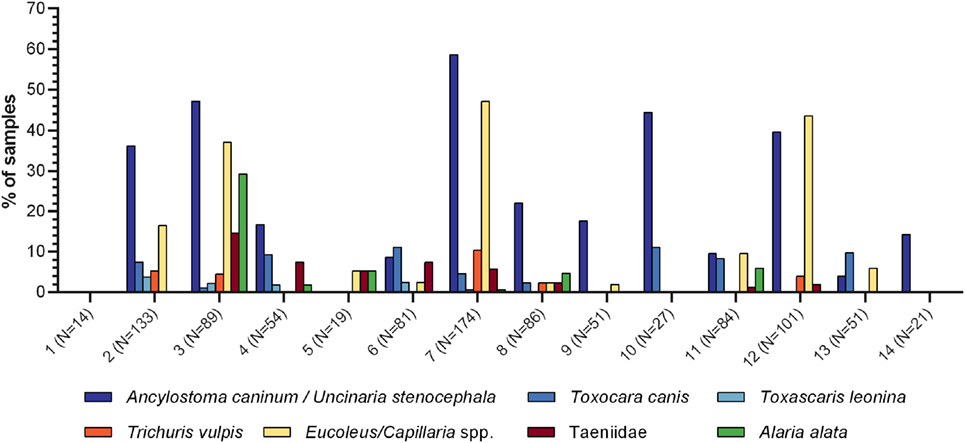
Figure 2. Detection frequencies of Ancylostomatidae, T. canis, T. leonina, T. vulpis, Capillaria/Eucoleus spp., Taeniidae, and A. alata eggs per individual zoo. The sample size for each of the fourteen zoos is indicated in brackets.
Results of Statistical Analyses
The effect of season and management factors was only assessed statistically for the four most prevalent parasites, namely Ancylostomatidae, Capillaria/Eucoleus spp., Taeniidae, and T. canis. Anthelmintic treatment negatively affected the probability of Ancylostomatidae egg detection (Table 8), whereas no statistically significant effects of management factors on the other parasites were found (Tables 9–11). Similarly, statistical analyses did not result in significant differences between seasons for any of these parasites (Tables 10–13). However, an increasing probability of Toxocara canis and Ancylostomatidae egg sdetection with increasing monthly sample size per zoo was observed (Tables 8 and 9).
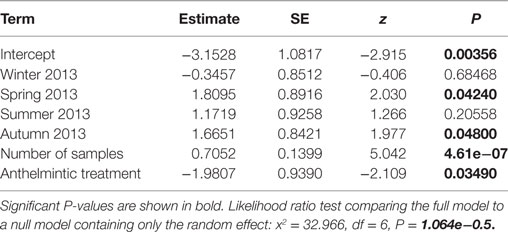
Table 8. Results of generalized linear-mixed model testing the influence of different predictor variables on the probability of being infected with Ancylostomatidae.
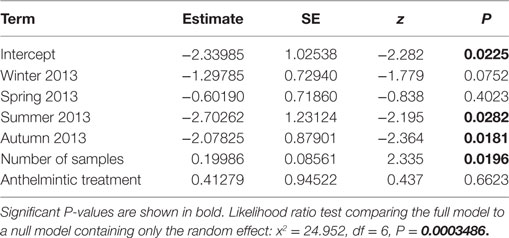
Table 9. Results of generalized linear-mixed model testing the influence of different predictor variables on Toxocara canis infections.
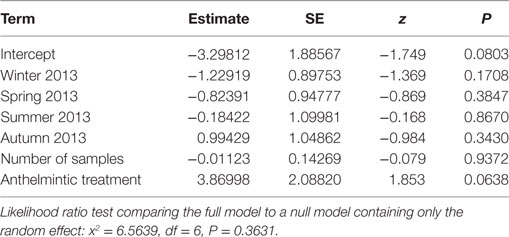
Table 10. Results of generalized linear-mixed model testing of different predictor variables on the probability of Capillaria/Eucoleus spp. infections.
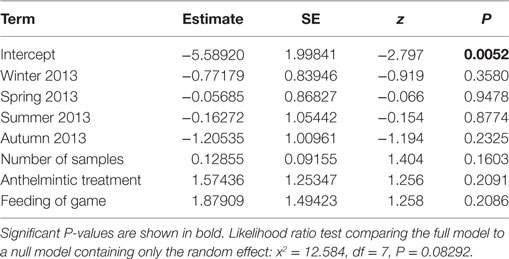
Table 11. Results of generalized linear-mixed model testing the effect of different predictor variables on taeniid infections.
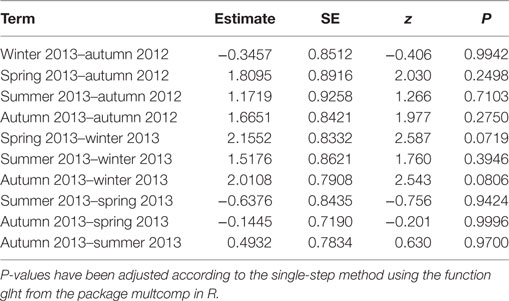
Table 12. Results of multiple comparisons between seasons regarding hookworm (Ancylostomatidae) infections.
Discussion
In this study, gastrointestinal helminths infecting captive European grey wolves (Canis lupus lupus) in 14 zoos distributed across Germany were identified. The most frequent parasites were hookworms, roundworms, whipworms, hairworms, taeniids, and A. alata, all of which have been detected in captive and/or wild wolves in Europe before (7–10, 17–21). For the interpretation of the presented data, it should be kept in mind that we were not able to match samples to single individuals. Thus, certain individuals may have contributed more than one sample per month. Accordingly, this could have lead to an over- or underestimation of true infection rates. Furthermore, it is possible that not all helminth infections were detected due to the limitations of the applied coproscopical techniques. For example, the sensitivity of the sedimentation-flotation technique for the detection of Giardia sp. is limited, as only Giardia cysts can be detected.
Hookworms showed the highest egg detection frequency (30.84% of samples) and occurred in 12 of 14 zoos. However, it has to be taken into account that strongyle eggs from feeder animals may have been subsumed under hookworm eggs, resulting in an overestimation of the actual frequency. Nevertheless, the detection rate of 30.84% in this study is similar to the percentage rate of 35.9% observed in captive wolves in Poland (10). A meta-analysis revealed an overall prevalence of 44.9% in wild wolves in Palaearctic regions (17). By contrast, Markowski (9) reported Ancylostomatidae infections in less than 2% of captive wolves in German zoos based on zoo health records. The reason for this discrepancy is unclear; however, this may be due to a lack of documentation, since hookworm infections are often subclinical and asymptomatic. In addition, anthelmintic treatment of the wolves may have played a role. Indeed, zoos in the present study which practiced anthelmintic treatment were significantly less likely to be positive for Ancylostomatidae in any given month. Nevertheless, 12 of 14 zoos were positive at least once, which requires attention since A. caninum possesses zoonotic potential, causing cutaneous larva migrans in humans (22). Thus, zoo staff should exert caution when handling substrates potentially contaminated with wolf feces, e.g., during cleaning of enclosures.
Capillaria/Eucoleus spp. showed the second highest egg detection rate (19.88%), and occurred in 10 of 14 zoos. Similarly, Markowski (9) found documentation of Capillaria/Eucoleus infection in 10.2% of wolves in German zoos. For free-ranging European gray wolves, no records of Capillaria/Eucoleus infections could be found in the literature. Capillaria/Eucoleus eggs were detected frequently in wild Ethiopian wolves (Canis simensis); however, it was speculated that these eggs actually represented gastrointestinal passengers, passing the intestine after consumption of infected prey (23). It cannot be excluded that this was also the case in the present study. However, it seems unlikely that the high frequency of Capillaria/Eucoleus eggs in the fecal wolf samples relies exclusively on intestinal transit, but the wolves were most likely infected with canine-specific species due to ingestion of embryonated eggs or earthworms containing infective larvae in their tissues. In domestic dogs, Eucoleus aerophilus (syn. Capillaria aerophila), Capillaria putorii (syn. Aonchotheca putorii), Capillaria plica (syn. Pearsonema plica), and Capillaria hepatica are the most common species (24). In wild foxes in Denmark, C. plica and E. aerophilus have been detected at high prevalence (25). However, since C. plica eggs are shed with urine, it is unlikely that these have contributed to the egg detection rate in the present study.
Roundworm prevalence in European wild wolves was reported at 17% for T. canis in Italy (18) and at 5.6–21.2% for T. canis and 1.1–13.5% for T. leonina in Poland (19). Here, T. canis was detected in 5.18% of captive wolf samples, occurring in 11 zoos, while T. leonina occurred in 1.25% of samples from 5 zoos. In contrast, Markowski (9) reported ascarids as the most frequently documented helminths in zoo records of captive wolves in Germany, with a prevalence of 18.3%. As transplacental and lactogenic transmission to the offspring is of particular importance in the epidemiology of T. canis, the lower prevalence detected in the present study may be due to the fact that the sampled population comprised only a single pup. In wild wolves, a seasonal rise in Toxocara egg detection frequency has been related to the presence of susceptible pups (7). In addition, changes in host immunity or the endocrinological status, e.g., related to reproduction, might lead to reactivation of dormant Toxocara larvae, as observed in dogs (12). Wolves show a seasonal reproductive pattern, with a mating period in winter (26). Therefore, a seasonal rise in T. canis egg excretion during winter was expected. However, we did not find any statistical support for this. As a zoonotic parasite, T. canis can cause different clinical manifestations in humans, including the larva migrans visceralis syndrome as well as ocular, neurological and covert toxocarosis (6). Toxocara eggs are not immediately infective when excreted, but may remain infective for several months after the third-stage larvae has developed, and adhere well to surfaces due to their sticky outer shell (27). Thus, contamination of enclosures may represent a source of infection for zoo staff.
Among parasites of carnivores, taeniids are also of particular zoonotic concern. Here, taeniid eggs, representing either Taenia spp. or Echinococcus spp., occurred in 8 of 14 zoos, and showed a prevalence of 3.75% among samples. Markowski (9) reported a prevalence of <2% based on zoo health records. In contrast, taeniids are the most prevalent helminths in wild wolves, with prevalences up to 64% in Spain (20), and 41.7% in Palaearctic regions (17). In contrast, Hermosilla (8) detected Taenia spp. with a prevalence of only 1.5% in Croatian wild wolves. The comparably low prevalence rates in zoos could be due to low prevalences in meat fed to captive wolves, low exposure of wolves to infected small mammals, or anthelmintic treatment. However, when testing the effect of diet on taeniid infections no significant influence was found, probably because all but three zoos fed game. Similarly, no significant influence of anthelmintic treatment could be detected.
Tapeworm infections are often asymptomatic in the definitive host, but may cause serious disease in the intermediate host (3). In humans, Echinococcus granulosus and E. multilocularis may cause cystic and alveolar echinococcosis, respectively, which are potentially fatal diseases (4). Less commonly, Taenia spp. of carnivores may also affect humans (28). In contrast to Toxocara eggs, taeniid eggs are immediately infective when excreted (29). Further research is needed to clarify whether captive wolves are infected with Echinococcus spp., to shed light on the true zoonotic relevance of the detected taeniid infections. In wild wolves in Portugal, mainly Taenia spp. were detected, but 1.5% of wolves also harbored Echinococcus intermedius (30).
T. vulpis and A. alata were both found in five zoos, and each showed a detection frequency of 3.65% among samples, which is considerably lower than detection rates reported for wild wolves. A tenfold higher T. vulpis detection rate (38.5%) was found in wild wolves in Poland (21), and A. alata rates ranged from 17.3% in Byelorussia, known today as Belarus (31), up to 80.1% in Poland (10). In recent years, A. alata mesocercariae have increasingly been detected in meat of wild boar in Germany (32), indicating that feeding of wild boar to wolves serves as a source of infection. As mentioned above, almost all zoos fed game to wolves. While humans may aquire A. alata infections after consumption of raw or undercooked meat of paratenic hosts, thus becoming a new paratenic hosts, eggs shed by definitive hosts do not constitute a source of human infection.
Apart from the abovementioned parasites, eggs of Spirocerca lupi, A. vasorum, Opisthorchis spp., and Diphyllobothrium latum were detected in the fecal samples; however, these eggs occurred at low frequencies of less than one percent. Furthermore, Fasciola hepatica eggs and coccidian oocysts were detected in 5.86 and 4.90% of the wolf samples, respectively. However, no statement can be made whether these originated from the wolves, from animals fed to wolves, or both as parasite host.
Apart from coccidian oocysts, no protozoan parasites were detected in this study. In contrast, Giardia sp. and Cryptosporidium sp., which have zoonotic potential, were detected in wild Croatian wolves at a prevalence of 2.1 and 1.8%, respectively (8), and in wild wolves from Canada at a prevalence of 6.8 and 1.7%, respectively (7). As mentioned above, the sensitivity of the sedimentation-flotation technique for detection of these protozoa is limited, thus, these infections may have been missed.
Conclusion
In this study, several helminths were identified in captive wolves, which potentially compromise the wolves’ health or represent zoonotic parasites, posing a potential infection risk for zoo staff. Thus, regular anthelmintic treatment of captive wolves is recommended. Zoo staff needs to be educated in zoonotic health risks, and preventive measures to minimize these risks, such as wearing of gloves, cleaning of shoes when leaving enclosures, frequent washing of hands, and adequate disposal of feces, should be implemented or reinforced. Furthermore, carcasses and meat should be frozen before feeding to prevent wolf infections with, e.g., A. alata and taeniids in order to protect the health of both wolves and humans.
Author Contributions
JB collected parts of the fecal samples and carried out laboratory work. AS and JB performed statistical analyses. CS and MB designed and coordinated the study. JB, AS, and CS drafted the manuscript. All authors participated in data interpretation and read and approved the final manuscript.
Conflict of Interest Statement
The authors declare that the research was conducted in the absence of any commercial or financial relationships that could be construed as a potential conflict of interest.
The reviewer BP and handling editor declared their shared affiliation.
Acknowledgments
The authors thank the participating zoo directors and staff members for their support and the monthly sample collection.
Supplementary Material
The Supplementary Material for this article can be found online at http://www.frontiersin.org/articles/10.3389/fvets.2017.00228/full#supplementary-material.
References
1. Kontaktbüro Wölfe in Sachsen. Verbreitung in Deutschland. Rietschen, Germany: Kontaktbüro Wölfe in Sachsen (2017). Available from: http://www.wolf-sachsen.de/de/verbreitung-in-deutschland
2. Puschmann W, Zscheile D, Zscheile K. Säugetiere, Zootierhaltung, Tiere in menschlicher Obhut. 5th ed. Frankfurt, Germany: Verlag Harri Deutsch (2009). 977 p.
3. Hoberg EP. Taenia tapeworms: their biology, evolution and socioeconomic significance. Microbes Infect (2002) 8:859–66. doi:10.1016/S1286-4579(02)01606-4
4. Moro P, Schantz PM. Echinococcosis: a review. Int J Infect Dis (2009) 13:125–33. doi:10.1016/j.ijid.2008.03.037
5. Möhl K, Große K, Hamedy A, Wüste T, Kabelitz P, Lücker E. Biology of Alaria spp. and human exposition risk to Alaria mesocercariae – a review. Parasitol Res (2009) 105(1):1–15. doi:10.1007/s00436-009-1444-7
6. Strube C, Heuer L, Janecek E. Toxocara spp. infections in paratenic hosts. Vet Parasitol (2013) 193:375–89. doi:10.1016/j.vetpar.2012.12.033
7. Bryan HM, Darimont CT, Hill JE, Paquet PC, Thomson RCA, Wagner B, et al. Seasonal and biogeographical patterns of gastrointestinal parasites in large carnivores: wolves in a coastal archipelago. Parasitology (2012) 139:781–90. doi:10.1017/S0031182011002319
8. Hermosilla C, Kleinertz S, Silva LMR, Hirzmann J, Huber D, Kusak J, et al. Protozoan and helminth parasite fauna of free-living Croatian wild wolves (Canis lupus) analyzed by scat collection. Vet Parasitol (2017) 233:14–9. doi:10.1016/j.vetpar.2016.11.011
9. Markowski S. Tierart – und erregerspezifische Maßnahmen zur Optimierung des Gesundheitsmanagements für nordamerikanische Säugetiere in Zoologischen Gärten [Dissertation]. Hannover (Germany): University of Veterinary Medicine Hannover (2013).
10. Szafranska E, Wasielewski O, Bereszynski A. A faecal analysis of helminth infections in wild and captive wolves, Canis lupus L., in Poland. J Helminthol (2010) 84(4):415–9. doi:10.1017/S0022149X10000106
11. Reperant LA, Hegglin D, Fischer C, Kohler L, Weber JM, Deplazes P. Influence of urbanization on the epidemiology of intestinal helminths of the red fox (Vulpes vulpes) in Geneva, Switzerland. Parasitol Res (2007) 101:605–11. doi:10.1007/s00436-007-0520-0
12. Nijsse R, Mughini-Gras L, Wagenaar JA, Ploeger HW. Recurrent patent infections with Toxocara canis in household dogs older than six months: a prospective study. Parasit Vectors (2016) 9:531. doi:10.1186/s13071-016-1816-7
13. Becker AC, Kraemer A, Epe C, Strube C. Sensitivity and efficiency of selected coproscopical methods – sedimentation, combined zinc sulfate sedimentation-flotation, and McMaster method. Parasitol Res (2016) 115:2581. doi:10.1007/s00436-016-5003-8
14. R Development Core Team. R: A Language and Environment for Statistical Computing. Vienna, Austria: R Foundation for Statistical Computing (2011). Available from: http://www.R-project.org/
15. Kuznetsova A, Brockhoff PB, Christensen RHB. lmerTest: Tests in Linear Mixed Effects Models. R Package Version 3.0.0. (2016). Available from: https://cran.r-project.org/package=lmerTest
16. Hothorn T, Bretz F, Westfall P. Simultaneous inference in general parametric models. Biom J (2008) 50:346–63. doi:10.1002/bimj.200810425
17. Craig HL, Craig PS. Helminth parasites of wolves (Canis lupus): a species list and an analysis of published prevalence studies in Nearctic and Palaearctic populations. J Helminthol (2005) 79:95–103. doi:10.1079/JOH2005828
18. Guberti V, Stancampiano L, Francisci F. Intestinal helminth parasite community in wolves (Canis lupus) in Italy. Parassitologia (1993) 35:59–65.
19. Okulewicz A, Perec-Matysiak A, Bunkowska K, Hildebrand J. Toxocara canis, Toxocara cati and Toxascaris leonina in wild and domestic carnivores. Helminthologia (2012) 49:3–10. doi:10.2478/s11687-012-0001-6
20. Segovia JMM, Guerrero R, Torres J, Miquel J, Feliu C. Ecological analyses of the intestinal helminth communities of the wolf, Canis lupus, in Spain. Folia Parasitol (2003) 50:231–6. doi:10.14411/fp.2003.041
21. Kloch A, Bednarska M, Bajer A. Intestinal macro- and microparasites of wolves (Canis lupus L.) from north-eastern Poland recovered by coprological study. Ann Agric Environ Med (2005) 12:237–45.
22. Guillot J, Bouree P. Zoonotic worms from carnivorous pets: risk assessment and prevention. Bull Acad Natl Med (2007) 191:67–81.
23. van Kesteren F, Piggott KJ, Bengui T, Kubri SB, Mastin A, Paris M, et al. Helminth parasites in the endangered Ethiopian wolf, Canis simensis. J Helminthol (2014) 89:487–95. doi:10.1017/S0022149X14000534
24. Epe C, Coati N, Schnieder T. Results of parasitological examinations of faecal samples from horses, ruminants, pigs, dogs, cats, hedgehogs and rabbits between 1998 and 2002. Dtsch Tierarztl Wochenschr (2004) 111:243–7.
25. Saeed I, Maddox-Hyttel C, Monrad J, Kapel CMO. Helminths of red foxes (Vulpes vulpes) in Denmark. Vet Parasitol (2006) 139:168–79. doi:10.1016/j.vetpar.2006.02.015
26. Mech LD, Boitani L, editors. Wolves: Behavior, Ecology, and Conservation. Chicago, London: The University of Chicago Press (2006). 472 p.
27. Overgaauw PAM, Van Knapen F. Toxocarosis, an important zoonosis. Eur J Companion Anim Pract (2008) 18:259–66.
28. Cleaveland S, Laurenson MK, Taylor LH. Diseases of humans and their domestic mammals: pathogen characteristics, host range and the risk of emergence. Philos Trans R Soc Lond B Biol Sci (2001) 356:991–9. doi:10.1098/rstb.2001.0889
29. Lawson JR, Gemmell MA. Hydatidosis and cysticercosis: the dynamics of transmission. Adv Parasitol (1983) 22:261–308. doi:10.1016/S0065-308X(08)60464-9
30. Guerra D, Armua-Fernandez MT, Silva M, Bravo I, Santos N, Deplazes P, et al. Taeniid species of the Iberian wolf (Canis lupus signatus) in Portugal with special focus on Echinococcus spp. Int J Parasitol Parasites Wildl (2013) 2:50–3. doi:10.1016/j.ijppaw.2012.11.007
31. Shimalov VV, Shimalov VT. Helminth fauna of the wolf (Canis lupus Linnaeus, 1758) in Belorussian Polesie. Parasitol Res (2000) 86:163–4. doi:10.1007/s004360050026
Keywords: helminths, coproscopy, zoo, zoological garden, zoonosis, Taeniidae, Toxocara spp., hookworms
Citation: Bindke JD, Springer A, Böer M and Strube C (2017) Helminth Fauna in Captive European Gray Wolves (Canis lupus lupus) in Germany. Front. Vet. Sci. 4:228. doi: 10.3389/fvets.2017.00228
Received: 01 November 2017; Accepted: 12 December 2017;
Published: 22 December 2017
Edited by:
Donato Traversa, Università di Teramo, ItalyReviewed by:
Claudio De Liberato, Istituto Zooprofilattico Sperimentale delle Regioni Lazio e Toscana, ItalyBarbara Paoletti, Università di Teramo, Italy
Copyright: © 2017 Bindke, Springer, Böer and Strube. This is an open-access article distributed under the terms of the Creative Commons Attribution License (CC BY). The use, distribution or reproduction in other forums is permitted, provided the original author(s) or licensor are credited and that the original publication in this journal is cited, in accordance with accepted academic practice. No use, distribution or reproduction is permitted which does not comply with these terms.
*Correspondence: Christina Strube, Y2hyaXN0aW5hLnN0cnViZUB0aWhvLWhhbm5vdmVyLmRl