- 1Department of Veterinary Clinical Sciences, Small Animal Clinic, Justus-Liebig-University Giessen, Giessen, Germany
- 2Department of Veterinary Clinical Sciences, Clinical Pathology and Clinical Pathophysiology, Justus-Liebig-University Giessen, Giessen, Germany
The aim of this prospective study was to compare quality of blood products produced either by a novel gravity-driven hollow-fiber separation system (HF) or by centrifugation (C). Whole blood was obtained from 31 healthy non-greyhound canine blood donors and separated into fresh frozen plasma and packed red blood cells using either HF or C in a university teaching hospital. Red blood cell (RBC) count, albumin and fibrinogen concentration, prothrombin time (PT), activated partial thromboplastin time (aPTT) and coagulation factor activity (FV, FVIII), von Willebrand Factor (vWF), and antithrombin activity were assessed. Plasma obtained with the HF showed a significantly higher median PT (9.4 vs. 7.9 s, P = 0.0006) and aPTT (14.9 vs. 13.1 s, P = 0.0128) than plasma prepared with C. Lower albumin (21.7 vs. 23.5 g/l, P = 0.0162) and fibrinogen (1.0 vs. 1.5 g/l, P = 0.0005) concentrations and activities of FV (105 vs. 114%, P = 0.0021) and antithrombin (104 vs. 117%, P = 0.0024) were seen in blood products obtained with the HF. In contrast, vWF was not affected by the method of plasma separation. Compared to HF, RBC count as well as hematocrit were not significantly higher (8.0 vs. 8.9 1012/l, P = 0.1308; 0.57 vs. 0.62 l/l, P = 0.0736) when blood products were prepared with C. In conclusion, higher quality of blood products especially regarding coagulation parameters and RBCs was achieved by using C compared to HF. Despite the statistical significances, however, the clinical relevance has to be further elucidated. Nevertheless, HF provides an alternative to produce blood products if a centrifuge is not available.
Introduction
Transfusion of blood components gained increasing importance in veterinary medicine (1, 2). Preparation of blood product components is expensive and time consuming as specific devices for separation such as a centrifuge are needed (3). The presence of commercial veterinary blood banks in the USA improved the availability of blood components (4, 5). However, in other countries, commercial blood banks are scarce. Therefore, blood banks owned by veterinary practitioners became essential in the veterinary field. However, if small quantities of blood products are required in smaller veterinary clinics or practices, the initial costs for separation using a centrifugation protocol are high.
For people, alternative systems have been developed using gravity without the need for heavy centrifuges requiring electric power supply or for larger centrifuges high voltage power (three-phase electric power). They allow the preparation of life-saving blood products in the military field or zones with small blood donation facilities (6–10). These systems consist of several hundreds to thousands of hollow-fibers of various types of materials such as polyethylene (11), polyethersulfone (12–14), or polypropylene (15), through which the blood flow is achieved by gravitation. The hollow-fibers are characterized by different wall thicknesses, inner diameters, and pore sizes, respectively. They are built into a plastic chamber forming a U shape exemplarily shown in Figure 1.
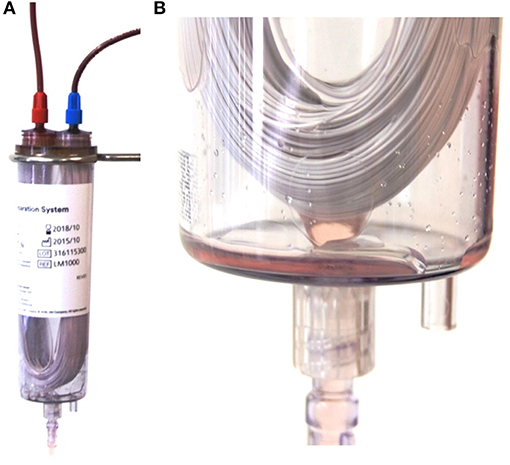
Figure 1. (A) Composition of the hollow-fiber system (HF) showing the U shaped polyethersulfone fibers in a plastic chamber and connected with the whole blood bag (not shown) with the red Luer lock connector (left on the top of the chamber) and the packed red blood cell bag (not shown) with the blue luer lock connector (right on top of the plastic chamber). (B) Detailed image of the U shaped fibers at the bottom of the plastic chamber. Picture taken during the process of plasma separation. Small droplets of plasma can be seen leaving the fibers and entering the plasma bag (not shown) via a luer lock connection (bottom of the picture).
At the distal loop of the fibers, plasma is expressed through the pores and collected inside the chamber, which is further connected with the plasma bag. The remaining plasma poor blood which cannot pass the fiber pores is further driven to an erythrocyte storage bag connected to the system, the packed red blood cell bag (pRBC). In human medicine, the quality of the obtained blood products is regulated by national and international guidelines (16). In the veterinary field, scarce regulations are available. There are national guidelines for the collection, storage, transport and administration of blood and blood products in some countries e.g., in Germany [later referred to as German veterinary guideline for blood products (17)]. Overall, guidelines regulate the quality of the produced pRBC and fresh frozen plasma (FFP) (17).
Based on the German veterinary guideline for blood products (17), quality of the FFP is fulfilled if 50% of the initial coagulation Factor VIII (FVIII) activity is preserved and if it contains <1 × 109/l white blood cells, <30 × 109/l thrombocytes, and <3 × 109/l erythrocytes.
In the pRBC, phosphate (Phos) concentration and lactate dehydrogenase (LDH) activity are of interest as their increase reflects potential hemolysis. Hemolysis should be <0.8% at the end of storage. Quality of the pRBC is further evaluated by measuring the hemoglobin concentration, hematocrit value, and red blood cell count. Potassium (K) concentration is a marker of ATP depletion during storage.
Despite the lack of international veterinary guidelines, some text books (18, 19) offered a non-binding guideline for sampling, preparation, storage, and administration of blood products based on the literature.
To the authors' knowledge, two commercial gravity-driven separation systems are currently available and have been recently evaluated in people (8, 13). It was shown that the use of hollow-fiber (HF) systems causes a significant reduction in Factor V (FV), Factor VIII (FVIII), Factor XI, and fibrinogen concentration. Due to the decrease in FVIII, these plasma products do not fulfill international criteria for plasma quality (16) making HF blood separation systems a less ideal alternative for blood product separation in human medicine (8, 13).
However, to the authors' knowledge there is currently no veterinary study evaluating quality of blood products prepared with gravity-driven separation systems. Thus, the aim of this study was to assess quality of blood products (plasma and pRBC) obtained with a novel HF system in comparison to the traditional method, the centrifuge.
Hypothesis of this study was that the quality of blood products produced by a novel gravity-driven HF separation system is lower than in products generated by centrifugation but still fulfills quality requirements recommended previously for veterinary blood products (17).
Materials and Methods
Study Design
The prospective study was performed between February and June 2017.
Dogs were recruited from the pre-established blood donation program of a veterinary teaching hospital including client owned dogs.
The blood donation program was ethically approved by the regional council (No V 54 - 19 c 20 15 h 02 GI 18/7 No. A 24/2017) and written owner's consent was given.
Criteria for inclusion to the donor program were based on the German veterinary guideline for blood products (17). Briefly, dogs with a body weight >20 kg were included if history and clinical examination were unremarkable and there was no traveling history in the Mediterranean area or other continents. If clinical examination was unremarkable, a 20 G catheter1 was aseptically placed in the cephalic or lateral saphenous vein by a licensed veterinary technician or veterinarian and blood was taken for laboratory examination routinely done prior to each blood donation. Overall, a total of four tubes coated with ethylenediamine tetraacetic acid (EDTA), two lithium heparin tubes, two plain tubes, and four tubes containing 3.2% sodium citrate were drawn, whereby each tube had a volume of 1.3 ml.
Routine laboratory examination included blood typing (if not performed before) using a commercially available quick test2, as well as hematological (CBC) and blood chemical examination. Biochemical evaluation included assessment of electrolytes, urea, creatinine, glucose, total protein, albumin, globulin, and C reactive protein. In case of no clinically significant abnormalities, blood donation was performed and the dog was enrolled in the study. Immediately after sampling, the citrated tubes were centrifuged3 at 3,000 G for 5 min and the supernatant was removed and centrifuged again for 5 min at 3,000 G. Citrated plasma was then stored at −80°C until coagulation analysis.
After blood donation, the dogs were assigned randomly to the groups for hollow-fiber (HF) separation and centrifugation (C) method, respectively such that an approximately equal number of blood donors was achieved in each group whereby a special technique of randomization was not used (20). In both groups, the same procedure of blood donation and the same blood bags4 for transfer and storage of blood products were used.
Donation
A triple bag system4 consisting of a 17G needle for puncturing the jugular vein, a donation bag with 63 ml Citrate-Phosphate-Dextrose (CPD) anticoagulant, a red blood cell bag containing 100 ml Saline Adenine Glucose-Mannitol-Solution (SAG-M), an empty plasma bag as well as a leukocyte depletion filter5, and a Y-connector with screwable luer lock connectors were used for blood collection. The triple blood bag system was provided by the manufacturer and was used for both groups. Donation was performed using gravity until the blood collection mixer6 showed the targeted amount of 10–20 ml/kg of donated blood considered to be well tolerated by the donor (21) so that a final amount of 400–500 ml was approximated (Figure 3).
During donation, the dogs received a continuous infusion of 10 ml/kg/h buffered crystalloid solution7 administered with a volumetric infusion pump8 via the venous catheter to account for any possible hemodynamic effects of blood donation. Every dog received the same rate of crystalloids for the duration of donation process. Donated blood was further gently mixed for a few minutes and allowed to rest at room temperature for a maximum of 1 h. Afterwards, the exact weight of the whole blood bag (WB) was determined and converted into volume whereby the following correction factors were applied: Whole blood: 1,050 g/ml, plasma: 1,030 g/ml, and packed red blood cells: 1,070 g/ml (22, 23), respectively.
Separation Process
Hollow-Fiber Group
The WB was hung up at the stand provided by the manufacturer for separation process (Figure 2).
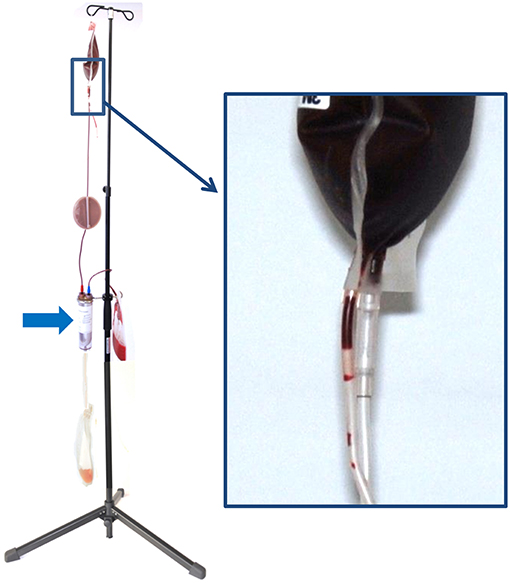
Figure 2. Configuration of the hollow-fiber system (HF) set up on a stand for gravity-driven blood separation. On top, there is the whole blood bag (WB), which is connected via the in-line leukocyte depletion filter (arrow) to the hollow-fiber system (HF). During blood separation, plasma leaving the U-shaped fibers of the HF is collected in the plasma bag (P) and the remainder erythrocytes are stored in the packed red blood cell bag (pRBC). The inset shows the breaking valve of the whole blood bag, which is broken to initiate the process of blood separation.
An in-line leukodepletion filter5 with a screwable luer lock connector was implemented (by the manufacturer)4 into the connection line of the WB. It was attached to the HF filtration polyethersulfone device (MicroPES® TF 10 capillary membrane)9.
The system was further connected to the bag containing SAG-M and to the empty bag for plasma collection after being processed through the filter (Figures 1, 2).
The HF filter consists of polyethersulfone with 100 μm ± 25 wall thickness, an inner diameter of 300 ± 40 μm and a pore size of 0.5 μm (compare Figure 2). The filter is delivered with a 25–35 ml saline priming volume as a dry filter would cause an extremely rapid absorption of plasma resulting in a hemolysis of RBCs (24).
At the distal loop of the fiber filter system, plasma was pressed from the system by gravitation through the membrane. By breaking the valve (Figure 2, inlet), the separation process was started and the time of beginning and finishing noted. After the separation process, the filter system was not rinsed with saline to yield the remaining RBCs as it was not recommended by the manufacturer.
Plasma (P) and erythrocyte bag (pRBC) were disconnected and gently mixed.
Centrifugation Group
Prior to separation via centrifugation, leukodepletion was performed to make the procedure comparable to the HF group. For leukodepletion, the WB with the in-line Leukodepletion filter5 was connected via the luer lock connector to an additional plain, empty plasma bag and the separation process was started by breaking the valve of the WB (as shown in Figure 2 for the HF) and duration of complete separation process was noted. When leukodepletion was finished, the WB was then prepared for centrifugation by placing it upwardly into the centrifugation cup and a further cup of exactly equal weight was prepared and balanced.
Centrifugation was performed for 20 min (plus 10 min deceleration time) at 1,976 G using a commercial centrifuge designed for blood separation10.
After centrifugation, the WB containing the now spun down erythrocytes and plasma was connected to the dedicated plasma bag. Plasma was separated from erythrocytes by applying pressure on the WB with a manual plasma press11. After plasma was harvested, the remainder red blood cells, i.e., the erythrocyte concentrate was transferred to the dedicated SAG-M bag (later referred to as pRBC) by gravitation. Both bags were gently mixed afterwards. The duration of the whole separation process using the centrifuge was recorded and included the time required for leukodepletion, preparing the centrifugation cups, the centrifugation time itself as well as manual expression of plasma into the plasma bag.
Sampling and Sample Processing for Quality Control of Blood Products
The exact procedure of sampling is shown in Figure 3. As seen in the figure and described previously, samples from the donor (D) were obtained for routine hematological and clinical chemical analysis. The results of the CBC, parameters reflecting hemolysis, the albumin concentration as well as the coagulation analysis served for comparison with the results obtained later from the respective blood bags.
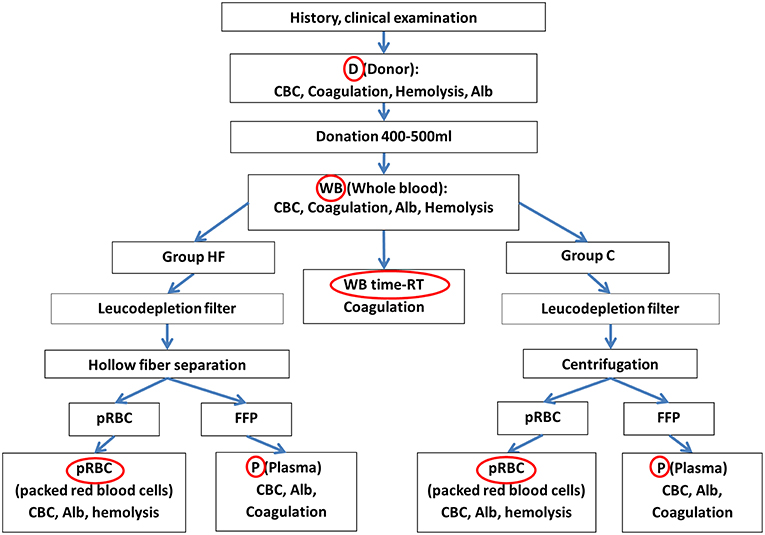
Figure 3. Flow chart representing all steps of the separation process and the origin of sample (red circles) as well as the evaluated parameters. Samples were taken from the donor (D), the whole blood bag (WB) prior to the separation process as well as the packed red blood cell bag (pRBC) and the plasma bag (P). The sole impact of time at room temperature (time-RT) during the separation process was evaluated with a subset of specimens from the WB which was centrifuged and frozen at the end of the procedure (WB time-RT). Alb, albumin; CBC, complete blood cell count including the hematocrit value, red blood cell count, hemoglobin concentration, platelet count, white blood cell count; Coagulation, coagulation parameters including coagulation times PT, aPTT, fibrinogen concentration, activity of antithrombin and factors VIII, V, and von Willebrand factor. FFP, fresh frozen plasma; hemolysis, parameters reflecting hemolysis including concentrations of phosphate, lactate dehydrogenase activity.
All samples taken from the blood bags later on were transferred to 1.3 ml plain tubes. After blood donation, 15 ml of whole blood was aseptically drawn out of the collection bag (WB) and transferred into 10 aliquots for performance of the same analyses as in the blood donor. Six of Ten aliquots (named WB, Figure 3) were directly used for the same analyses as the samples directly taken from the donor (D) to assess the sole impact of anticoagulant in the WB bag. The remainder four of these 10 specimens (named WB time-RT) were left at room temperature during the separation process and served to assess the sole impact of time and room temperature (time-RT) during the process of blood separation on coagulation parameters. Directly at the end of the separation process, plasma was harvested, and kept frozen at −80°C until coagulation analysis.
After the separation process, 8 ml plasma were aseptically drawn from all (HF & C) plasma bags (P), as well as 5 ml erythrocyte concentrate from the respective pRBCs (compare Figure 3). Quality of the plasma was evaluated by assessing the CBC, the albumin concentration and coagulation parameters. Quality of the erythrocyte concentrate was assessed with a CBC, the albumin concentration and parameter reflecting hemolysis.
Hematological analysis was performed immediately after sampling. The remainder specimens were centrifuged as described before and frozen at −80°C until analysis.
After collecting samples, all bags were sealed. The pRBCs were kept refrigerated at 4°C and the plasma bags frozen at −30°C, respectively.
Laboratory Examination
Hematological examination was performed within 4 h after sampling with a commercial automatic laser-based veterinary hematology analyzer12. CBC included hematocrit value (Hct), hemoglobin (hgb), red blood cell (RBC) count, white blood cell (WBC), and platelet count (Plt). Moreover, erythrocyte indices were assessed including the mean corpuscular volume (MCV), the cell hemoglobin concentration mean (CHCM) consistent with the mean hemoglobin concentration measured in every erythrocyte, and the mean corpuscular hemoglobin concentration (MCHC), i.e., the mean hemoglobin concentration calculated by the analyzer from the erythrocyte count and the total hemoglobin concentration.
To quantify the degree of hemolysis due to separation process, hemolysis in percent was calculated by us as reported previously using the Hct value, the total hemoglobin concentration (hgbtotal) and the free hemoglobin concentration (hgbfree) in the blood plasma (25):
Hgbtotal was measured with the hematology analyzer12. Hgbfree was calculated by subtracting the cellular hemoglobin concentration, i.e., the hemoglobin within the erythrocytes (hgbcell) that is not affected by hemolysis from hgbtotal. Hgbcell was calculated by reversing the formula used to calculate the MCHC for the CHCM (hgbcell = CHCM × RBC × MCV/1,000) (26). If hgbtotal was lower than calculated hgbcell due to the imprecision of the methods and multiple calculation steps, a slightly negative result was obtained for hgbfree that was censored as “zero.”
Biochemical analysis included measurement of the albumin concentration and of parameters indicating potential hemolysis, i.e., concentrations Phos and lactate dehydrogenase (LDH) activity. LDH and albumin were assessed with a commercial clinical chemistry benchtop analyzer13.
Coagulation analysis was performed as batch measurement within 4 months after sampling. Samples were allowed to thaw in a 37°C waterbath14 and were centrifuged again for 10 min at 800 G.
Coagulation analysis included measurement of prothrombin time (PT), activated partial thromboplastin time (aPTT), fibrinogen concentration, antithrombin (AT), and factor VIII activity (FVIII) measured with a commercial viscoelastic-based mechanical detection system15 as described previously (27).
Reference intervals have been established and published previously for these methods (27) and were used for comparison with the obtained results. As for measurement of FVIII activity, FV activity was assessed using the automated coagulation analyzer 16 and the canine pool plasma prepared for the previous study (27). A commercial human assay consisting of a FV-deficient plasma substrate was used, whereby the default setting for dilution of the sample was 1:20 and a modified PT was measured. Results were compared with a calibration curve prepared by serial stepwise dilution of canine pool plasma in the dilution steps 1:40: 1:60; 1:80, and 1:100, respectively.
Von Willebrand factor (vWF) activity was measured at an external laboratory16 using immunoturbidometry with a commercial analyzer17. Samples were shipped frozen to the laboratory.
Quality Criteria
Blood products were reviewed afterwards if they fulfilled (1) german national guidelines for the use of blood products in the veterinary field (17) and (2) the European human guidelines (16).
For FFP, the German veterinary guideline for blood products (17) state that at least 50% of the initial FVIII activity should remain after freezing and thawing in the FFP. Leukoreduction is not explicitly requested in the guidelines but there should be <1 × 109 WBC/l, <30 × 109/l thrombocytes, and <3 × 109/l erythrocytes remaining in the plasma bag (compare Table 5). In contrast, the human guidelines in Europe request a WBC of < 0.1 × 106 / Unit for leukodepleted FFP (16) (Table 5). There should be not less than an average of 70 IU FVIII per 100 mL (after freezing and thawing), RBCs < 6.0 × 109/L and Plt < 50 × 109/L per unit.
Based on the German veterinary guideline for blood products (17), a Hct value of 0.5 – 0.75 l/l, a hgb concentration of 17 g/dl, and <0.8% of hemolysis at the end of storage should be achieved for the pRBC (compare Table 5). The human European guideline requests a Hct of 0.5–0.7 l/l and a minimum of 40 g hgb/unit and <1 × 106 WBC / unit in leukodepleted pRBC as well as sterility and absence of discolorisation (compare Table 5).
Statistical Analysis
For all statistical analyses, a commercially available software18 was used.
To verify the assumption of normality, the Shapiro-Wilk test was used. Depending on the results, either unpaired t-test or Mann-Whitney U test was applied to compare differences between the blood products (pRBC and FFP) depending on the method of separation (HF vs. C). The impact of group (HF vs. C) and step of preparing the blood product (origin of sample) was assessed with a two-way repeated measures analysis of variance (ANOVA), which was followed by two post-tests, whereby a correction for multiple comparisons was performed automatically by the software. The Sidak's multiple comparison test was done to evaluate the group effect for each obtained type of sample and blood product (the within column-effect of the statistical table). A possible effect of the procedure of blood separation within each group was investigated with a Tukey's multiple comparison test (the within-row effect of the statistical table).
To evaluate differences in the proportions of blood products fulfilling quality criteria in both groups, a Fisher's exact test was used.
P-values were corrected according to the Bonferroni-Holm method to reduce the probability of a type 1 error (28). Corrections were made seperately for each type of statistical question listed in Tables 1–6 as well as for each origin of sample (D, WB, P, pRBC given in Tables 2, 3) and guideline, respectively (given in Tables 5, 6). For the two-way ANOVA test, p-values obtained for the factors “origin of sample,” “separation method,” and “interaction” between both factors, were corrected separately with the Bonferroni-Holm method.
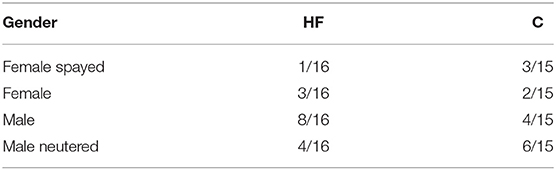
Table 2. Gender and castration/neuter status of the donors in both groups: hollow-fiber system (HF) or centrifuge (C).
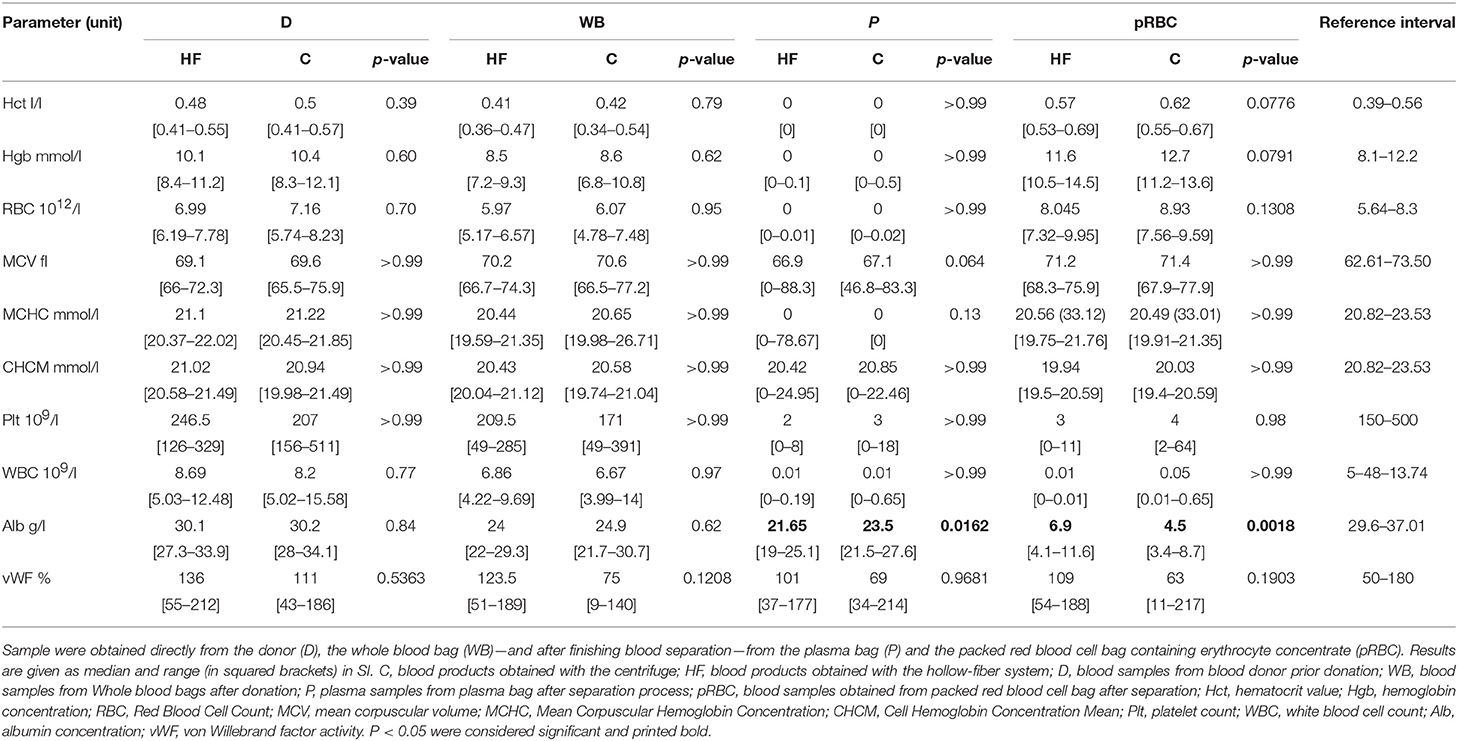
Table 3. Hematological variables, albumin concentration, and von Willebrand Factor activity in samples obtained during different steps of the separation process performed with the centrifuge (C) or the hollow-fiber system (HF).
For all statistical analyses, level of significance was set at p < 0.05.
Results
During the sampling period, 32 non-Greyhound dogs were presented for blood donation. One of 32 dogs assigned to the HF group had to be excluded due to severely lipemic plasma (triglyceride 4.4 mmol/l), which markedly impaired separation process. Failure of separation was characterized by unequal distribution of weight between plasma and erythrocytes (90 vs. 314 g) and remaining 98 g within the filter.
Thus, a total of 31 dogs were enrolled in the study.
Overall, 15/31 whole blood bags were processed with centrifugation protocol, whereas 16/31 bags were processed with the gravity-driven HF system.
Donations
All donations went uneventfully and were finished within a median 6 min (range 5–10 min, Table 1).
As shown in Table 1, age, body weight, and the donated volume did not differ significantly between the two groups. The sex, castration, and neuter status of the donor dogs are shown in Table 2.
Separation Process
The use of the novel gravity-driven HF system was easy, less (working) time consuming than the centrifuge as no preparation of the centrifugation cup, no taring of the centrifugation cups, no unloading and mechanical expression is necessary. It was well-appreciated by technicians and veterinarians. Familiarity was reached after a few separation procedures. There was no difference in separation time between the groups, but the time required for two of 31 separation processes using the HF exceeded the time frame of 90 min recommended by the manufacturer for a separation process (13, 30). Both bags contained a relatively high volume of 496 and 483 ml, respectively, which was at the upper range of the overall donated volume (Table 1).
Quality of the FFP
After the separation process, PT and aPTT assessed in the plasma bag (P) were significantly higher in the HF group compared to C, while fibrinogen concentration as well as FV and AT activity were significantly lower (Figure 4). After separation, the vWF activity was not significantly different between the two groups (Table 3). Comparing WB time-RT and P, there was no impact of time and therefore sample aging during the separation process on the results.
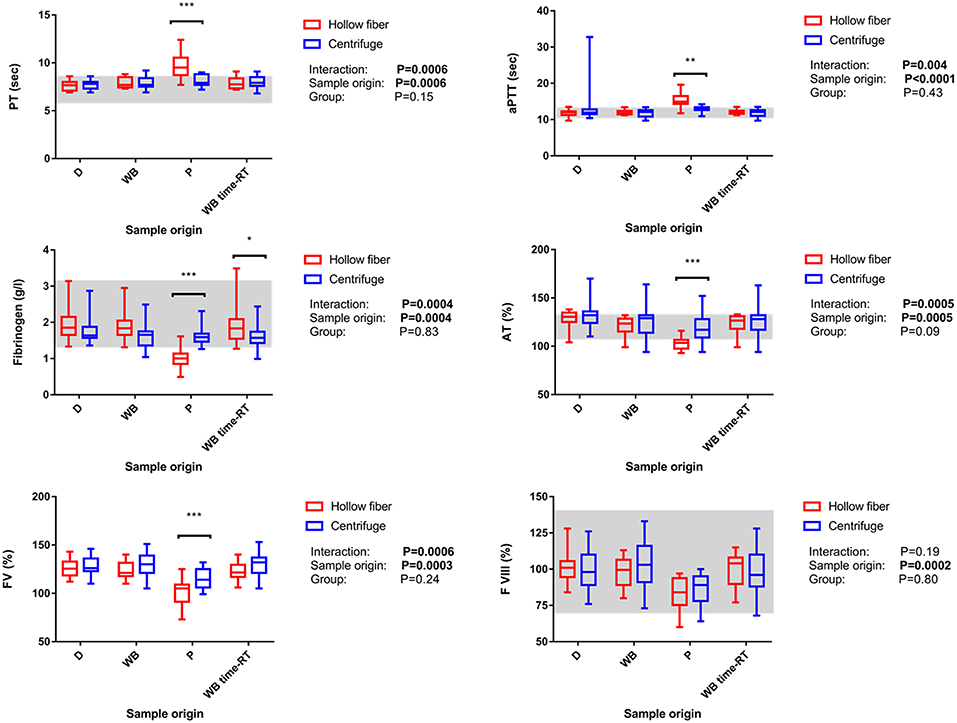
Figure 4. Impact of origin of sample reflecting the different steps of the blood separation process and the system used for blood separation (i.e., centrifuge or the hollow-fiber system) on coagulation parameters as well as interaction between the factors “origin of sample” and “system” Data is shown as box-and-whisker diagrams. The central line represents the median, the box the 25 and 75% percentile, and the whiskers are consistent with the range. The reference interval is indicated as gray area. Significant pairwise comparisons were marked with *-***; whereby *P < 0.05; **P < 0.01, and ***P < 0.001, respectively. D, blood samples from blood donor prior donation; WB, blood samples from Whole blood bags after donation; P, plasma samples from plasma bag after separation process; WB time-RT, sample taken from the whole blood bag prior to the blood separation process to assess the sole impact of time at room temperature; PT, prothrombin time, aPTT, activated partial thromboplastin time; AT, antithrombin activity; FV, factor V activity; FVIII, factor VIII activity.
Following the separation process, fibrinogen concentration assessed in P prepared with the HF system decreased in all but 1/16 HF plasma units below the lower limit of the reference interval. Only 12/16 HF plasma bags contained 50% or more of initial fibrinogen concentration after freezing and thawing (Figure 4).
After leukodepletion and separation, a significant decrease of FVIII activity could be observed in both groups, whereas FVIII remained high in WB time-RT in both groups, i.e. there was no impact of sample aging on the results (Figure 4). In both groups, FVIII remained above 50% of initial activity in all samples (Table 5).
In HF, the separation process resulted in a significantly higher decline of albumin concentration compared to C (Table 3).
Quality of the Erythrocyte Concentrate (pRBC)
The hematocrit value was not—significantly higher in pRBC in group C compared to the HF group. Similar results could be obtained for the hgb concentration (Table 3). In D or WB samples, no significant difference between group HF and C for the Hct, hgb concentration or RBCs was observed.
Erythrocyte indices (MCV, MCHC, and CHCM) in pRBC did not differ between the two study groups (Table 3).
As expected, leukodepletion process resulted in a significant decrease in Plt and WBC counts from baseline (WB) cell counts (Table 3).
There was no significant difference between the two groups at baseline (D and WB) or after the separation process (in P and pRBC) in Plt or WBC count.
Moreover, separation method did not have an impact on Phos concentration, LDH activity as well as calculated %hemolysis in the samples obtained from the D, WB, and pRBC (Table 4).
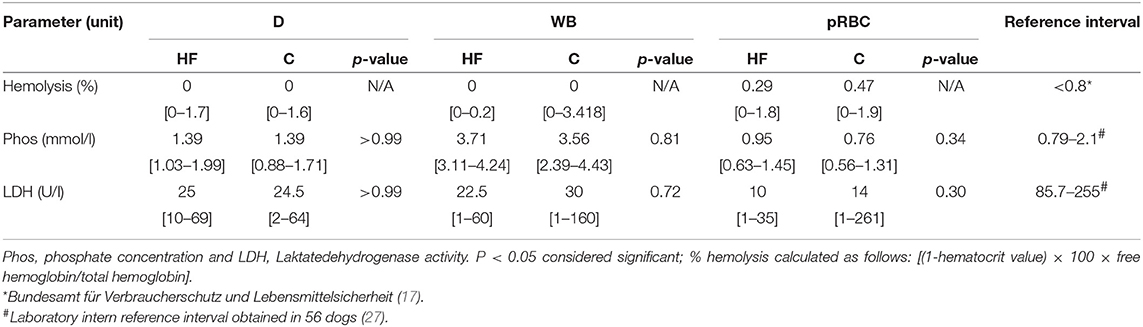
Table 4. Impact of blood separation process on median and range of parameters reflecting hemolysis in samples obtained from the donor (D), the whole blood bag (WB), and the packed red blood cell bag (pRBC).
Comparison With Guidelines Regulating Quality of Blood Products
As shown in Table 5, FVIII activity in the FFP remained high enough to fulfill national veterinary guidelines, but RBC counts were exceeding the allowed cut-off values in more than 40% of plasma products obtained with both separation methods. The maximally allowed WBC counts of <1 × 109/l requested by the German veterinary guideline for blood products could be fulfilled in all plasma bags. On the other hand, the WBC count of <0.1 × 109/l requested by human European guidelines could not be achieved in some FFP in both groups (16).
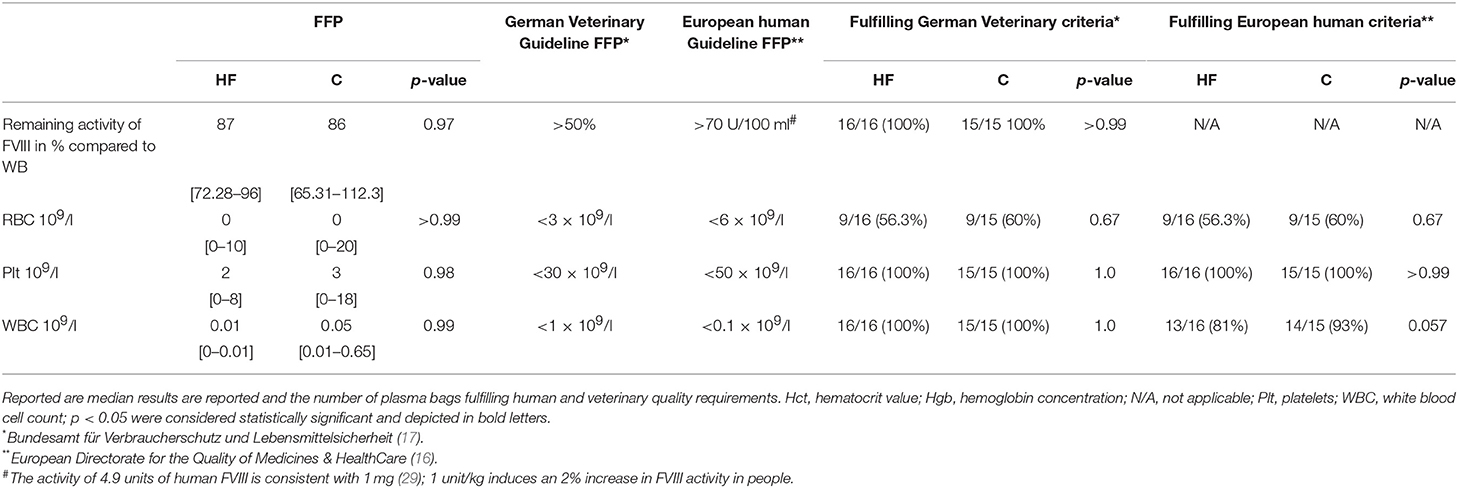
Table 5. Impact of the method of the separation process on quality of the obtained fresh frozen plasma (FFP) as reflected by FVIII activity and the remainder number of WBC, RBC, and Plts.
Regarding quality of the pRBC (Table 6), both separation methods resulted in adequate quality for obtained concentrations of RBCs, hgb, and Htc value. However, irrespective of the separation method, approximately one third of pRBC bags of both groups exceeded the requested cut-off value of 0.8% for hemolysis. Moreover, the degree of achieved leukodepletion was not sufficient for any pRBC prepared here when compared with human European guidelines.
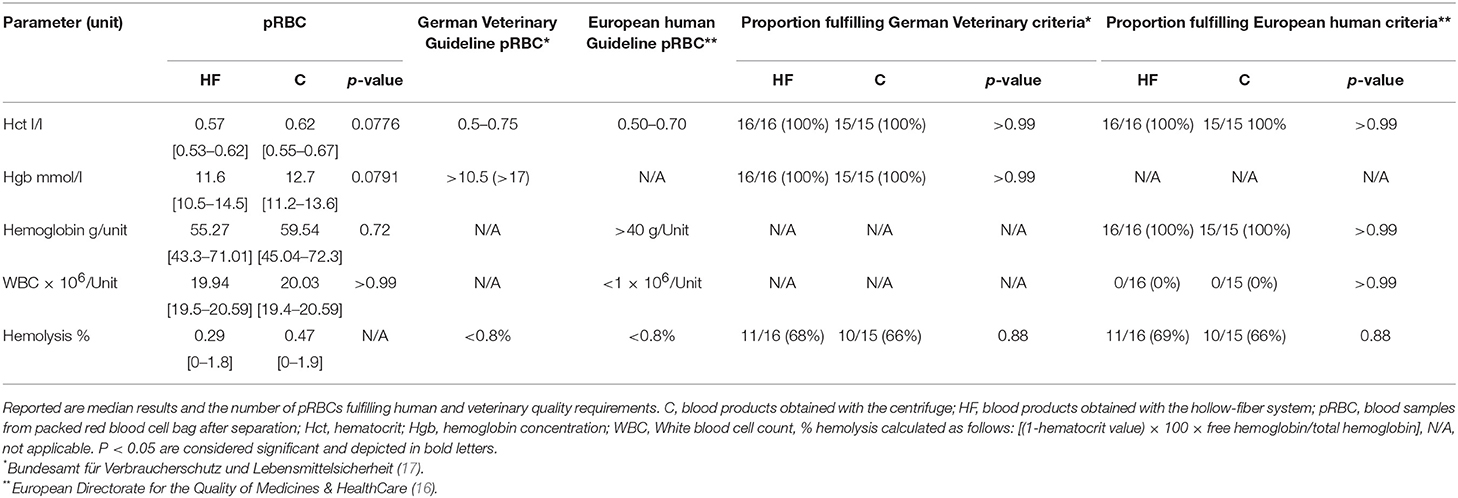
Table 6. Impact of the method of the separation process on quality of the packed red blood cell bag (pRBCs) as reflected by erythrocyte parameters, remainder WBC, and %hemolysis.
Discussion
To the authors' knowledge, this is the first study in veterinary medicine evaluating quality of blood product after the separation process using a HF system.
Due to its ease of use, HF separation would be a fast and feasible tool for veterinary clinics and practices with limited space and resources to use a centrifuge. Theoretically, smaller clinics and veterinary practices might consider using whole blood instead of prepared blood products, however, storage of whole blood at 4°C results in significantly higher decrease of coagulation factor activity than in frozen plasma (31). Moreover, plasma rather than whole blood transfusion is recommended in non-anemic patients only requiring coagulation factors or protein to avoid RBC, volume or iron overload and vice versa dogs with e.g. immune mediated hemolytic anemia with normo- or hypervolemia are at risk to experience Transfusion associated circulatory overload (TACO) (32, 33).
Separation time was similar for both methods evaluated here. However, in accordance with previous investigationevaluating the preparation of human blood products, labor intensity was markedly lowerof the separation process compared to centrifugation (12), as preparation and taring of the centrifugation cup as well as unloading and mechanical expression of the plasma bag is not necessary when using the HF.
However, quality of blood products was impaired when using HF separation process as reflected by a significant decrease in coagulation factor activity and fibrinogen concentration finally leading to increased coagulation times. Fibrinogen concentration was markedly decreased compared to the traditional centrifugation method. In accordance with our results, previous studies in people evaluating HF systems of the same composition, reported lower FVIII activity and fibrinogen concentration (14). On the other hand, acceptable FVIII (69–79% of initial activity present after 1 year of storage) activity has been demonstrated for human FFP as required by the Council of Europe (16) despite a decline in plasma protein content and coagulation factor activity due to postulated absorption by the HF system (12). The utilization of polyethylene instead of polyethersulfone fibers in the filter system showed no obvious impairment of coagulation factor activity in a previous study (34). However, none of the previous studies performed a direct comparison between the two separation methods as we did. A recent study separating human whole blood with a similar device as we did already recognized an increase in PT, aPTT and decrease in fibrinogen concentration, FV, FVII and FVIII activity which is in accordance with our results (8).
In our study, FVIII activity was above 50% of initial activity in both groups. As in people, no further assessment of coagulation factor activity or concentration is demanded in the German veterinary guideline for blood products (17). Nevertheless, we measured activities of FV and vWF as well as the fibrinogen concentration and the coagulation times PT and aPTT to enable a comparison with previous veterinary studies (35, 36). The requirements of European human guidelines could only be partially fulfilled for plasma quality as RBC counts were too high in both groups. Therefore, in regard of fulfilling quality requirements, plasma obtained with the HF group is of lower quality than plasma generated by centrifugation. However, further studies evaluating the clinical utility of plasma products obtained with HF would be needed to demonstrate the clinical significance, i.e., the impact of lower coagulation factor activity or fibrinogen concentrations on patient outcome. Human studies suggest that the degree of fibrinogen substitution might be clinically relevant (37) in the way that that the transfusion of plasma containing high concentrations of fibrinogen is superior to lower concentration products in surgical and massive trauma patients.
The exceedance of separation time of 90 min in two donors can be considered as separation failure as done in a previous study (13). However, the rationale behind such limited time frame given for separation procedure remains unclear as sample aging due to the separation did not affect coagulation results as demonstrated here. The reason for observing prolonged separation time in the 2 blood bags seen here has not been clarified. Theoretically, leukocyte count and donated blood volume causing exhaustion of the HF might affect separation time. Leukocyte counts, however, were not increased in these two P and pRBC bags, so that failure of leukodepletion can be excluded as potentially explanation. A volume of the blood bag not exceeding 450 ± 50 ml has been recommended by the manufacturer (13, 30). It remains unclear if the leukodepletion filter itself, any clots within the leukodepletion filter or HF exhaustion caused the significant increase in separation time. In previous studies, separation times of 40 and 44 min were described using a not further specified Fresenius and a Pall leukodepletion filter (12, 14) similar to the filter used in this study. Studies not using any leukodepletion filter reported a separation time of 11 and 15 min although they used a HF system with similar fiber diameter (328 μm) as in our study but smaller pore size (0.2 μm) andlower wall thickness (30 μm) (11, 34).
Based on the European human guideline for quality of FFP and pRBC recommend a reduction to <1 × 106 WBC per Unit (16), which could not be achieved here and in previous studies (38). Leukodepletion by buffy coat separation prior filtering through a leukodepletion filter might be more efficient, but is not possible in this system or study design (22, 39). It has been stated earlier that leukodepletion might be more efficient after storage for 2–4 h at 4°C (40).
A potential impact of implementing the leukocyte reduction filter on coagulation factor activity was taken into account while planning the study, as leukodepletion was done by the same filter for both groups prior separation process. Decrease in FVIII in both groups can be atrributed to leukodepletion in both groups, as in WB time-RT FVIII activity remained high which excludes time as effector for the decline. It has been shown previously that the use of the same filter as applied in our study (WBF3 Leukodepletion filter)5 causes a significant decline in FVIII activity and fibrinogen concentration (41). Performing the step of leukodepletion after HF separation only for the pRBC bag might positively influence plasma product quality, at least regarding FVIII and fibrinogen.
The crystalloid infusion during the donation process might have some hemodilutional effect on the donated blood and thus have a potential impact on the laboratory parameters assessed here. However, as both groups received the same infusion rate per kg body weight, they experienced the same degree of hemodilution, so that the effect of the method of blood separation, i.e., the group effect can be still compared. The same applies for the dilutional effect ofthe anticoagulant CPD that has a potential impact on the assessed parameters but does not affect the group effect as it is similar in both HF and C.
We appreciate some limitations of our study.
First, we did not use a special technique of randomization so that a selection bias cannot be entirely ruled out. However, the groups did not differ significantly prior to the separation process, a major selection bias appears to be unlikely.
Moreover, our study was focussing on the short-term effect of the method of separation rather than its potential impact on quality of blood products after storage, i.e. the long term effect. Thus, quality of erythrocytes at the end of storage as reflected by the degree of hemolysis in pRBC at the end has not been evaluated as required in the German Veterinary guidelines (17). A previous human study (13) showed, that hemolysis starts to increase after day 22 of storage reaching >0.8% hemolysis on day 29 demanding further research for canine pRBC. Hemolysis has been evaluated for leukodepleted and non-leukodepleted pRBC in veterinary and human literature showing some evidence of increased hemolysis depending on filter type and material (38, 40, 42–44) emphasizing the need for further studies.
We also did not evaluate pRBC quality over time which would have included assessment of 2.3-diphosphoglyceric acid, LDH, K (in humans) and free hemoglobin concentrations as well as bacterial cultures after expiring of the pRBC bags. In people, erythrocytes are of high potassium content and potassium concentration in blood products is therefore a suitable marker of hemolysis and thus the quality of blood products. In contrast, canine erythrocytes are of low potassium content except for some Japanese dog breeds and are therefore not reliably reflecting the degree of hemolysis. Thus, potassium concentration has not been used here to assess the quality of the blood products.
As done previously, plasma quality could be further assessed with thromboelastography (36), to improve predictability of coagulation factor activity in vitro and to estimate patients response to plasma therapy.
While assessment of quality was based on in vitro analyses, its clinical utility, i.e., improvement of coagulation reaction in the patient has not been evaluated.
Conclusion
Using the HF blood separation system provides blood products with impaired quality especially regarding coagulation parameters when compared to the centrifuge, however, the clinical significance of the finding still has to be elucidated.
Nevertheless, the HF is an easy to use alternative, which is interesting for veterinary clinics or practices with limited space and resources to buy a centrifuge.
Ethics Statement
This study was carried out in accordance with the recommendations of Bundesamt für Verbraucherschutz und Lebensmittelsicherheit, 2011. Leitlinien zur Gewinnung, Lagerung, Transport und Verabreichung von Blut und Blutprodukten im Veterinärbereich, Germany. (Federal Office for Consumer Protection and Food Safety, 2011. Guidelines for the collection, storage, transport and administration of blood and blood products in the veterinary field, Germany).
The blood donation program was ethically approved by the regional council (No. V 54 - 19 c 20 15 h 02 GI 18/7 No. A 24/2017) and written owner's consent was given. The blood products were implemented in patient care.
Author Contributions
EMH, NB, AM, EH, and HL designed the study. HL, NB, EMH, and EH drafted the manuscript. HL and EMH collected data and all authors contributed to its review.
Funding
The study was funded by 3M Deutschland GmbH, Germany.
Conflict of Interest Statement
The authors declare that the research was conducted in the absence of any commercial or financial relationships that could be construed as a potential conflict of interest.
Acknowledgments
We thank our technicians and laboratory staff for supporting our study.
Abbreviations
Alb, albumin; aPTT, activated partial thromboplastin time; AT, antithrombin (formerly antithrombin III); C, centrifugation; CBC, Complete Blood Count; CHCM, Cell Hemoglobin Concentration Mean; CPD, Citrat-Phosphate-Dextrose; D, samples prior donation; FFP, fresh frozen plasma; FV, factor V activity; FVIII, factor VIII activity; Hct, hematocrit value; hgb, hemoglobin concentration; HF, hollow-fiber system; K, potassium; LDH, lactate dehydrogenase; MCHC, Mean Corpuscular Hemoglobin Concentration; MCV, mean corpuscular Volume; P, plasma bag; Phos, phosphate; Plt, platelets; pRBC, packed red blood cell bag; PT, prothrombin time; RBC, red blood cells; SAG-M, Saline Adenine Glucose-Mannitol-Solution; vWF, von Willebrand Factor activity; WB, whole blood bag; WBC, white blood cells; WB time-RT, whole blood samples stored at room temperature for the time of the separation process.
Footnotes
1. ^KLINIJECT® venous catheter, KLINIKA Medical GmbH, Usingen, Germany.
2. ^Quick Test DEA 1, Alvedia, Limonest, France.
3. ^Mikro 120, Andreas Hettich GmbH & Co.KG, Tuttlingen, Germany.
4. ^3MTM Blood Separation System – Blood-bag system, 3M Deutschland GmbH, Neuss, Germany (former name PlasmaXpress Membrana, Germany).
5. ^Leukotrap WBF3 (Leukodepletion filter), Haemonetics Corperation, Baintree, MA, USA (former Pall, Pall Biomedical, Portsmouth, UK).
6. ^Blood mix scale BioTrans MW-5001, Möller Feinmechanik GmbH & Co, Fulda, Germany.
7. ^Sterofundin® ISO 1/1 E ISO, B. Braun Melsungen AG, Melsungen, Germany.
8. ^Volumetric infusion pump, Caesarea Industrial Park, Caesarea, Israel.
9. ^3MTM Blood Separation System – Hollow Fiber MicroPES, 3M Deutschland GmbH, Neuss, Germany (former name PlasmaXpress Membrana, Germany).
10. ^ROTANTA 460 R, Andreas Hettich GmbH & Co.KG, Tuttlingen, Germany.
11. ^Manual Plasma Extractor, unknown, unknown.
12. ^ADVIA2120™, Siemens Healthcare GmbH, Erlangen, Germany.
13. ^ABX Pentra 400, HORIBA ABX SAS, Montpellier, France.
14. ^Wasserbad, GFL Gesellschaft für Labortechnik mbH, Burgwedel, Germany.
15. ^STA Compact®, Diagnostica Stago S.A.S., Asnières-sur-Seine, France.
16. ^LABOKLIN GmbH & Co. KG, Labor für klinische Diagnostik, Bad Kissingen, Deutschland.
17. ^Immunoturbidometry, Cobas 8000 c701, Roche Germany Holding GmbH, Germany.
18. ^GraphPad Prism Version 7.0, Graph Pad Software Inc., San Diego, USA.
References
1. Jagodich TA, Holowaychuk MK. Transfusion practice in dogs and cats: An Internet-based survey. J Vet Emerg Crit Care. (2016) 26:360–72. doi: 10.1111/vec.12451
2. Kisielewicz C, Self IA. Canine and feline blood transfusions: controversies and recent advances in administration practices. Vet Anaesth Analg. (2014) 41:233–42. doi: 10.1111/vaa.12135
3. Mansell CL, Boller M. Blood component processing and storage. In: Yagi K, Holowaychuk M, editors. Manual of Veterinary Transfusion Medicine and Blood Banking. Hoboken, NJ: Wiley Blackwell (2016). p. 237–55.
4. Association of Veterinary Hematology and Transfusion Medicine. Veterinary Blood Banks. (2018) Available online at: http://avhtm.org/resourceslinks (accessed February 20, 2018).
5. Lester S. Meeting blood product demands: blood product sources. In: Yagi K, Holowaychuk M, editors. Manual of Veterinary Transfusion Medicine and Blood Banking. Hoboken, NJ: Wiley Blackwell (2016). p. 260.
6. Nollet KE. Blood banking and transfusion medicine in extreme or resource-limited conditions. Transfus Apher Sci. (2013) 49:378–9. doi: 10.1016/j.transci.2013.09.002
7. Natukunda B, Schonewille H, Smit Sibinga CT. Assessment of the clinical transfusion practice at a regional referral hospital in Uganda. Transfus Med. (2010) 20:134–9. doi: 10.1111/j.1365-3148.2010.00992.x
8. Johnson L, Kwok M, Marks DC. Preparation of red blood cell concentrates and plasma units from whole blood held overnight using a hollow-fibre separation system. Transfus Med. (2015) 25:13–9. doi: 10.1111/tme.12192
9. Lund TC, Hume H, Allain JP, McCullough J, Dzik W. The blood supply in Sub-Saharan Africa: needs, challenges, and solutions. Transfus Apher Sci. (2013) 49:416–21. doi: 10.1016/j.transci.2013.06.014
10. Hakre S, Peel SA, O'Connell RJ, Sanders-Buell EE, Jagodzinski LL, Eggleston JC, et al. Transfusion-transmissible viral infections among US military recipients of whole blood and platelets during Operation Enduring Freedom and Operation Iraqi Freedom. Transfusion. (2011) 51:473–85. doi: 10.1111/j.1537-2995.2010.02906.x
11. Schwarzfischer G, Heim MU, Mempel W. Der einsatz eines einfachen hohlfaserfiltersystems zur auftrennung von präoperativen eigenblutspenden. In: W. Mempel, M. U. Heim, G. Schwarzfischer, editors. Hämatologie, Sympomed. Vol. 1. Munich: Sympomed (1992). p. 108–13.
12. Brune T, Hannemann-Pohl K, Nißle K, Ecker N, Garritsen H. Quality, stability, and safety data of packed red cells and plasma processed by gravity separation using a new fully integrated hollow-fibre filter device. Adv Hematol. (2009) 2009:175234. doi: 10.1155/2009/175234
13. Hackstein H, Möller A, Gerlach M, Sachs U, Bein G. Prospective quality control study of a novel gravity-driven whole blood separation system suitable for humanitarian crises. Vox Sang. (2017) 112:806–9. doi: 10.1111/vox.12595
14. Hornsey VS, McColl K, Drummond O, Prowse CV. Separation of whole blood into plasma and red cells by using a hollow-fibre filtration system. Vox Sang. (2005) 89:81–5. doi: 10.1111/j.1423-0410.2005.00660.x
15. Takahashi TA, Hosoda M, Yamamoto S, Nakai K, Sekiguchi S, Yagishita A, et al. A new donor plasmapheresis system with disk type membrane separator. Plasma separation using gravity without any electrical devices. J Jap Soc Blood Transfus. (1990) 36:418–23. doi: 10.3925/jjtc1958.36.418
16. European Directorate for the Quality of Medicines & HealthCare. Guide to the Preparation, Use and Quality Assurance of Blood Components: CD-P-TS. Strasbourg (2017). p. 545.
17. Bundesamt für Verbraucherschutz und Lebensmittelsicherheit. Leitlinien zur Gewinnung, Lagerung, Transport und Verabreichung von Blut und Blutprodukten im Veterinärbereich. Braunschweig (2011). p. 24.
18. Yagi K, Holowaychuk M. Eds. Manual of Veterinary Transfusion Medicine and Blood Banking. Hoboken, NJ: Wiley Blackwell (2016). p. 1928.
19. Day MJ, Kohn B. Eds. BSAVA Manual of Canine and Feline Haematology and Transfusion Medicine. Gloucester: British Small Animal Veterinary Association (2012). p. 1354.
20. Suresh K. An overview of randomization techniques: an unbiased assessment of outcome in clinical research. J Hum Reprod Sci. (2011) 4:8–11. doi: 10.4103/0974-1208.82352
21. Yagi K. Canine blood collection. In: Yagi K, Holowaychuk M, editors. Manual of Veterinary Transfusion Medicine and Blood Banking. Hoboken, NJ: Wiley Blackwell (2016). p. 199–211.
22. Meer PF, Pietersz RNI, Nelis JT, Hinloopen B, Dekker WJA, Reesink HW. Six filters for the removal of white cells from red cell concentrates, evaluated at 4°C and/or at room temperature. Transfusion. (1999) 39:265–70. doi: 10.1046/j.1537-2995.1999.39399219282.x
23. Riggert J, Schwartz DW, Wieding JU, Mayr WR, Kohler M. Prestorage inline filtration of whole blood for obtaining white cell- reduced blood components. Transfusion. (1997) 37:1039–44. doi: 10.1046/j.1537-2995.1997.371098016442.x
24. Florian Bonn. Priming volume of the HF System. Florian Bonn was the Sales and Marketing Manager of 3M at the Time of the Study. Neuss: Personal Communication (2019).
25. Sowemimo-Coker SO. Red blood cell hemolysis during processing. Transfus Med Rev. (2002) 16:46–60. doi: 10.1053/tmrv.2002.29404
26. March H, Barger A, McCullough S, Schaeffer D, MacWilliams P. Use of the ADVIA 120 for differentiating extracellular and intracellular hemoglobin. Vet Clin Pathol. (2005) 34:106–9. doi: 10.1111/j.1939-165X.2005.tb00021.x
27. Bauer N, Eralp O, Moritz A. Reference intervals and method optimization for variables reflecting hypocoagulatory and hypercoagulatory states in dogs using the STA compact automated analyzer. J Vet Diagn Invest. (2009) 21:803–14. doi: 10.1177/104063870902100606
28. Aickin M, Gensler H. Adjusting for multiple testing when reporting research results: the Bonferroni vs Holm methods. Am J Pub Health. (1996) 86:726–8.
29. Fay PJ, Chavin SI, Schroeder D, Young FE, Marder VJ. Purification and characterization of a highly purified human factor VIII consisting of a single type of polypeptide chain. Proc Natl Acad Sci USA. (1982) 79:7200–4.
31. Furlanello T, Caldin M, Stocco A, Tudone E, Tranquillo V, Lubas G, et al. Stability of stored canine plasma for hemostasis testing. Vet Clin Pathol. (2006) 35:204–7. doi: 10.1111/j.1939-165X.2006.tb00115.x
32. Brooks MB. Transfusion of plasma products. In: Weiss DJ, Wardrop KJ, editors. Schalm's Veterinary Hematology. Ames, IA: Wiley Blackwell (2010). p. 744–50.
33. Walker JM. Component Therapy. In: Yagi K, Holowaychuk M, editors. Manual of Veterinary Transfusion Medicine and Blood Banking. Hoboken, NJ: Wiley Blackwell (2016). p. 13–25.
34. Sekiguchi S, Takahashi TA, Yamamoto' S, Hasegawa H, Takenaka Y, Suemitsu J, et al. A new type of blood component collector: plasma separation using gravity without any electrical devices. Vox Sang. (1990) 58:182–7. doi: 10.1159/000461112
35. Iazbik C, Couto CG, Gray TL, Kociba G. Effect of storage conditions on hemostatic parameters of canine plasma obtained for transfusion. Am J Vet Res. (2001) 62:734–5. doi: 10.2460/ajvr.2001.62.734
36. Urban R, Guillermo Couto C, Iazbik MC. Evaluation of hemostatic activity of canine frozen plasma for transfusion by thromboelastography. J Vet Intern Med. (2013) 27:964–9. doi: 10.1111/jvim.12097
37. Kozek-Langenecker S, Sørensen B, Hess JR, Spahn DR. Clinical effectiveness of fresh frozen plasma compared with fibrinogen concentrate: a systematic review. Crit Care. (2011) 15:R239. doi: 10.1186/cc10488
38. Notomi MK, Gopegui RR de, Escodro PB. Haematologic effects of leukoreduction on canine whole blood post-filtration and post-storage. Comp Clin Pathol. (2016) 25:145–9. doi: 10.1007/s00580-015-2155-3
39. Hansen AL, Kurach JD, Turner TR, Jenkins C, Busch MP, Norris PJ, et al. The effect of processing method on the in vitro characteristics of red blood cell products. Vox Sang. (2015) 108:350–8. doi: 10.1111/vox.12233
40. Brownlee L, Wardrop KJ, Sellon RK, Meyers KM. Use of a prestorage leukoreduction filter effectively removes leukocytes from canine whole blood while preserving red blood cell viability. J Vet Int Med. (2000) 14:412. doi: 10.1892/0891-6640(2000)014<0412:UOAPLF>2.3.CO;2
41. Chan KS-K, Sparrow RL. Microparticle profile and procoagulant activity of fresh-frozen plasma is affected by whole blood leukoreduction rather than 24-hour room temperature hold. Transfusion. (2014) 54:1935–44. doi: 10.1111/trf.12602
42. Patterson J, Rousseau A, Kessler RJ, Giger U. In vitro lysis and acute transfusion reactions with hemolysis caused by inappropriate storage of canine red blood cell products. J Vet Intern Med. (2011) 25:927–33. doi: 10.1111/j.1939-1676.2011.0737.x
43. Callan MB, Patel RT, Rux AH, Bandyopadhyay S, Sireci AN, O'Donnell PA, et al. Transfusion of 28-day-old leucoreduced or non-leucoreduced stored red blood cells induces an inflammatory response in healthy dogs. Vox Sang. (2013) 105:319–27. doi: 10.1111/vox.12058
Keywords: polyethersulfone, gravity-driven hollow-fiber, leukodepletion, blood product quality, coagulation
Citation: Lehmann H, Hindricks E, Hassdenteufel EM, Moritz A and Bauer N (2019) Prospective Comparative Quality Control Study of a Novel Gravity-Driven Hollow-Fiber Whole Blood Separation System for the Production of Canine Blood Products. Front. Vet. Sci. 6:149. doi: 10.3389/fvets.2019.00149
Received: 25 December 2018; Accepted: 29 April 2019;
Published: 17 May 2019.
Edited by:
Isabelle Goy-Thollot, VetAgro Sup, FranceReviewed by:
Rene Doerfelt, Ludwig-Maximilians Universität München, GermanySteven Epstein, University of California, Davis, United States
Guillaume Laurent Hoareau, University of California, Davis, United States
Copyright © 2019 Lehmann, Hindricks, Hassdenteufel, Moritz and Bauer. This is an open-access article distributed under the terms of the Creative Commons Attribution License (CC BY). The use, distribution or reproduction in other forums is permitted, provided the original author(s) and the copyright owner(s) are credited and that the original publication in this journal is cited, in accordance with accepted academic practice. No use, distribution or reproduction is permitted which does not comply with these terms.
*Correspondence: Hendrik Lehmann, aGVuZHJpay1sZWhtYW5uQHdlYi5kZQ==