- 1Universidad de Buenos Aires, Facultad de Ciencias Veterinarias, Cátedra de Enfermedades Infecciosas, Buenos Aires, Argentina
- 2Consejo Nacional de Investigaciones Científicas y Técnicas, Buenos Aires, Argentina
- 3Veterinary Population Medicine Department, Center for Animal Health and Food Safety, College of Veterinary Medicine, University of Minnesota, St. Paul, MN, United States
- 4Instituto de Patobiología, Veterinaria, UEDD INTA-CONICET, Buenos Aires, Argentina
- 5Instituto de Agrobiotecnología y Biología Molecular, UEDD INTA-CONICET, Buenos Aires, Argentina
Infection with the Mycobacterium bovis (M. bovis) causes a disease referred to as bovine tuberculosis (bTB), which affects a wide range of mammal hosts. Many countries have implemented control and eradication plans that have resulted in variable levels of efficacy and success. Although bTB is a notifiable disease in Argentina, and a control plan that targets cattle herds has been in place for decades, M. bovis is still prevalent in cattle, swine, and certain wild species. The aim of the paper here was to assess the sensitivity (Se), specificity (Sp), and positive and negative predictive values (PPV and NPV) of PCR from tissue, which is a test for rapid M. bovis detection in swine. Bacteriological culture was also performed for comparison purposes. A Bayesian approach was applied to estimate the accuracy of the diagnostic tests, PCR and bacteriological culture, in 266 swine samples with bTB-like lesions recovered during routine official inspections at slaughterhouses. A one-population model, assuming conditional dependence between test results, and incorporating prior information on the performance of the tests obtained from the literature, was used to estimate the tests Se and Sp. The accuracy of the combined (in parallel) application of both tests was also estimated. The Se of the PCR (82.9%) was higher than the Se of the bacteriological culture (79.9%), whereas the Sp of both tests was similar (88.5 and 89.0%, respectively). Furthermore, when both techniques were assessed in parallel, the Se of the diagnostic system increased substantially (Se = 96.6%) with a moderate Sp loss (Sp = 78.8%; PPV = 92.8%; NPV = 89%). Results suggest that the PCR, or the combined application of bacteriological culture and PCR, may serve as an accurate diagnostic tool to confirm bTB in swine samples. Results here will help the design and implementation of effective surveillance strategies for the disease in swine of Argentina and other settings in which the disease is prevalent.
Introduction
Mycobacterium bovis (M. bovis) is a member of the Mycobacterium tuberculosis complex (MTC) (1). MTC members cause tuberculosis (TB) in a wide range of host species worldwide, and M. bovis is a major causative agent of bovine tuberculosis (bTB), holding a significant zoonotic potential (2). Globally, bTB prevalence is quite heterogeneous and somehow related to the social features of the setting, with the disease being endemic in most developing countries and eradicated from many developed regions (3). Factors suggested to have impaired the efficacy of bTB control programs include limited political willingness, resources scarcity, existence of wildlife reservoirs, and limitations of the available diagnostic tests (4–8).
There are many tests available and widely used to diagnose the disease (9, 10). Many bTB diagnostic tests have been applied for decades, and rely on a variety of biological principles, including the measurement of the cellular response in the host following the application of an intradermal test, the histopathology of postmortem specimens, or the bacteriological culture (BC) of the agent (11). Currently, there are many other testing strategies available, such as the interferon gamma essay, antibody-based assays, or the detection of bTB DNA by PCR, which presents new opportunities to improve or develop control plans, but for which there is still a need to gain understanding of their performance in field conditions (9, 12, 13). However, because those diagnostic techniques have values of sensitivity (Se) and specificity (Sp) that vary on each animal species and the specific epidemiological situation, estimation of the test accuracy is challenging (14–16).
In Argentina, bTB is endemic in both livestock and wildlife populations (17). The protein purified derivative (PPD) skin test and the meat inspection of carcasses at slaughterhouses are the actions approved and used in the bTB's Control and Eradication National plan (SENASA, Res.128/2012). However, PPD testing is compulsory only for dairy cattle, dairy goats, dairy sheep, and genetic nuclei and multipliers. Control activities are voluntary for other species, including swine.
Official records estimated that 0.3% of inspected pigs in Argentina showed TB-like lesions, as observed by the Argentine Animal Health Service (SENASA) inspectors at slaughterhouses. However, evidence suggests that the figure may have been underestimated (18–20).
The BC is considered the reference technique for bTB diagnosis, even though the Se of the test is only ~80% (10, 12), impairing its systematic application on disease control programs. Moreover, it is a laborious technique, which requires high biosecurity facilities and relatively specialized workforce for implementation. Also, because the technique depends on the agent's viability, preservation, and quality of the collected sample drastically affects the results (10, 21). Another key limitation is the relatively long turnaround time of the techniques (on average, between 2 and 3 months), which jeopardizes the ability to inform decision-makers on a timely manner (22, 23). For that reason, the BC has important limitations as a confirmatory test for the macroscopic inspection at slaughterhouses (Table 1).
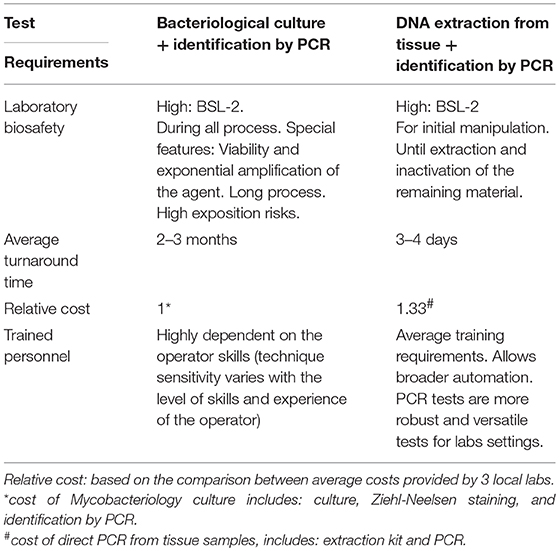
Table 1. Key features of both diagnostic tests that influence the feasibility of implementation in the context of a control plan.
The direct PCR analysis from tissue samples has been developed as an alternative technique to obtain a relatively fast confirmation of the infection. Direct PCR is believed to allow for the rapid, specific detection of Mycobacteria, and it is independent of the agent's viability on the sample. Some studies have reported the performance of the direct PCR in samples from cattle, buffaloes, humans, and some wildlife species (10, 14, 16). However, to the best of the authors' knowledge, the accuracy of the test is yet-to-be-assessed in swine (12).
The evaluation of diagnostic test performance, traditionally, has been based on the comparison of test results against a gold standard, allowing the assessment and validation of new techniques in comparison to a reference test. The limitation of such analytical approach is that the assumption of perfect Se and Sp of the reference test is, typically, questionable for many diseases, including bTB (21). Alternatively, Bayesian methods have been proposed as an analytical option to assess the accuracy of diagnostic tests without the requirement of a reference test (24). Bayesian methods have previously been used to estimate the accuracy of TB diagnostic techniques in bovine populations (8, 10, 25).
The aim of the study here was to estimate the Se and Sp of the BC, and of a rapid diagnostic test (PCR from tissue) on swine TB-like lesions obtained at slaughterhouses, and thereafter to evaluate the combined performance of those tests. Results will inform current discussions regarding the evaluation and potential modifications to the bTB control strategies in the target population and in the context of the Argentine disease control plan. Results may also be useful for countries in which bTB is prevalent in swine populations.
Materials and Methods
Sample Collection
Swine samples (n = 266) showing bTB-like lesions (TBL) were collected in multiple visits to three slaughterhouses located in the Province of Buenos Aires between 2015 and 2017. Those three slaughterhouses processed pigs from the main productive region of Argentina, which includes the provinces of Buenos Aires, Córdoba, Santa Fe, La Pampa, and Entre Ríos. Approximate 4 × 4 cm cuts of lymph nodes showing bTB-like lesions were collected. Additionally, tissue samples from swine shipped from bTB-free premises were also collected in order to validate the DNA extraction and PCR assay.
All samples were stored at −20°C. BC and PCR were carried out at the Infectious Disease Department's Mycobacterial diagnosis laboratory of the Veterinary School of the University of Buenos Aires. Because samples were collected from animals inspected post-mortem by the national authority and according to national regulations, no ethical or farmer's consent approval was required.
Diagnostic Procedures
Preparation of the Samples for PCR and Culture
Samples (4–7 g) of each individual lymph node were placed into a mortar and crushed with sterile sand and 10 mL of sterile bi-distilled water for homogenization. Two milliliters of this homogenate were transferred into a 15 mL tube and 4 mL of 4% NaOH were added to decontaminate the sample using the Petroff's modified method described elsewhere (26). A portion (~400 μL) of the homogenate was separated and frozen at −20°C for further DNA extraction.
DNA Extraction
Invitrogen™ PureLink™ Genomic DNA Mini Kit (Invitrogen, California, USA) was used for DNA extraction directly from tissue, according to the manufacturer's protocol. The obtained DNA was stored at −20°C until use for the PCR assay.
Bacteriological Culture
Bacteriological culture was performed following a protocol established elsewhere (26). Stonebrink and Löwenstein Jensen media were inoculated and incubated up to 60 days at 37°C and examined every 2 weeks. When bacterial growth was observed, Ziehl Neelsen staining was performed to observe acid fast bacilli, and, if positive staining, a loop full of bacteria was suspended in 200 μL of bi-distilled water and thermal lysis was performed at 95°C for 45 min. Lysates obtained were stored at −20°C until PCR assay.
Polymerase Chain Reaction (PCR) Assay for M. Bovis Detection
PCR was conducted in both DNA extracted from tissue and colonies lysates, for the detection of the insertion sequence 6110 (IS6110) characteristic of the MTC (27). Positive (bTB-confirmed sample) and negative controls (bi-distilled water) were also evaluated by the PCR. The primers used for the amplification, as well as PCR cycling conditions, have been described elsewhere (28). Colonies grown in Stonebrink media and IS6110-PCR positive were subject to spoligotyping to identify and to type the M. bovis isolates, following the protocol described by Kamerbeek et al. (29). Spoligotyping was carried out using the spoligotyping kit (Mapmygenome India). M. tuberculosis H37Rv (ATCC 27294) and M. bovis Bacillus Calmette-Guerin (BCG) (ATCC 27289) were included as reference strains for each assay.
Detection of Mycobacteria other than Tuberculosis
Ziehl Neelsen staining-positive isolates that were IS6110-PCR negative, were tested for its identification. The IS1245-PCR was used to detect the Mycobacterium avium (M. avium) complex (30). PCR controls were also conducted using a strain of M. avium obtained from a pure culture by thermal lysis as a positive control and bi-distilled water as a negative control.
Statistical Model
Latent Class Analysis
A Bayesian approach was used to estimate the Se and Sp of the BC and the PCR test (24) in samples showing bTB-like lesions (n = 266) and in the absence of a gold standard. Samples were considered to have originated from one single population, given that only samples showing bTB-like lesions were evaluated. For the analysis, results from both tests were assumed to be conditionally dependent because, although biological principles of both tests are different (the culture required that the pathogenic agent was viable, whereas the PCR only requires the presence of the genetic material in sufficient quantity), both tests are based on the detection of the mycobacteria. For that reason, we preferred to follow the conservative assumption that results were not independent.
Prior distributions for model parameters (including test Se and Sp and the disease prevalence, p) were initially approximated using information on the expected values and uncertainty around that expectation, from data reported in the peer-reviewed literature (Table 2). Beta distributions of the parameters were fitted using BetaBuster (https://betabuster.software.informer.com/), using a most likely value based on the median of estimates published in the literature, and a lower bound of the credibility interval that was approximately three standard deviations below the median, according to the published data (lower 99%CI Se = 0.58; Sp = 0.81). We preferred to use wide prior standard deviations to reflect the uncertainty around the true value of the parameters, considering that uncertainty related to the true value of the parameters is likely larger than simply the 95% CI of the results reported in the literature. Uniform distributions were used for the two co-variances (33). Field data were then used to modify the prior distributions and estimate posterior distributions in a Bayesian framework, using a one-population model and assuming conditional dependence between test results. Posterior distributions were reported as the posterior estimates of the median and posterior probability intervals (95% PPI). The code is provided as Supplementary Table 2. All analyses were implemented in the WinBUGS software, version 1.4, and results were computed for 10,000 iterations, after the first 1,000 were burnt-in. Autocorrelation was eliminated through thinning the chains by collecting one in 10 consecutive samples (https://www.mrc-bsu.cam.ac.uk/software/bugs/the-bugs-project-winbugs/). The influence of the selected priors on the posterior distributions was evaluated by comparing the initial models with a model fitted using non-informative uniform (0.1) distributions alternatively for the parameters of each test and the prevalence (Supplementary Table 1). The outputs including the MCMC trace-plots, posterior density distribution plots and convergence were visually assessed (Supplementary Figure 1).
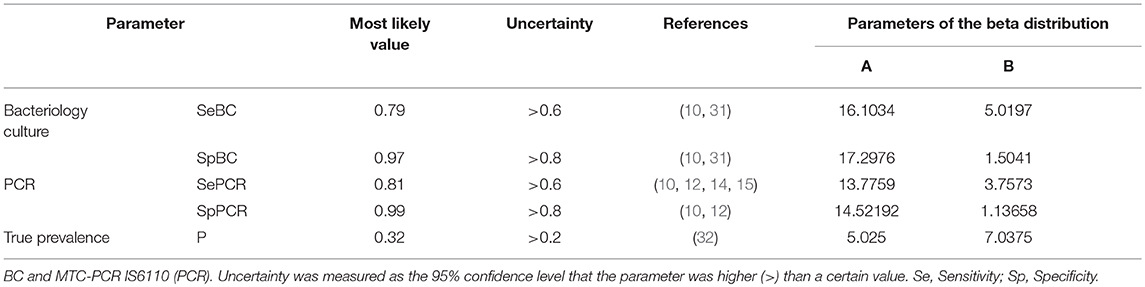
Table 2. Parameters of the beta distribution and source of data used to estimate the accuracy of both bTB tests in swine samples from Argentina.
Agreement between the results obtained from both test was measured using the kappa statistic. The Kappa coefficient, combined Se and Sp of the tests used in series and in parallel, and the positive and negative predictive values of the tests were calculated using the posterior estimates of the model and using the WinEpi software (34) as:
Se series = SePCR × SeBC
Se parallel = 1–(1–SePCR) × (1–SeBC)
Sp(series) = 1– (1–SpPCR) × (1–SpBC)
Sp(parallel) = SpPCR × SpBC
For presenting the results here, we followed the guidelines for reporting of diagnostic accuracy in studies that use Bayesian Latent class models (STARD-BLCM) described elsewhere (35).
Results
Descriptive Results
Most (171/266, 64.8%) samples were culture-positive, and most of those samples (137/171, 80.1%) were also MTC-IS6110+ PCR-positive. Out of the PCR-positive samples (176/266, 66.2%), only some (39/176, 15%) were BC-negative. A few (13/56, 23.2%) of the remaining 21% culture and PCR-negative samples (i.e., 4.9% of all the samples) were M. avium complex (IS1245+)-positive (Table 3). All IS6110-positive samples showed spoligotypes that were characteristic of M. bovis species, due to the absence of the 3, 9, 16, and 39–43 spacers.
Estimation of Tests Se and Sp
The estimated (posterior) Se of the bacteriological culture and of the PCR were 79.9% (95% posterior probability intervals, PPI: 71.69–88.7%) and 82.9% (95% PPI: 74.35–92.3%), respectively. The estimated (posterior) Sp was similar for both tests, with a value of 88.5% (95% PPI: 67.2–99.5%) for culture, and of 89.05% (95% PPI: 69.8–99.1%) for PCR.
Bovine tuberculosis prevalence in TB-like samples was 74.39% (95% PPI: 63.3–83.5%). The agreement of both tests was moderate (Kappa coefficient = 0.395; 95% CI (confidence interval) = 0.304–0.486). The negative and positive posterior correlation estimated between the diagnostic tests was uncertain, −0.02 (95% PPI: −0.2–0.33) and 0.16 (95% PPI: −0.15–0.74), respectively. The low correlations between the two test Se and between the two test Sp for samples showing bTB-like lesions suggests that the results of both tests were independent from each other.
Combination of both tests was evaluated considering the estimated prevalence, obtaining a Se and Sp of 66.2 and 98.7% (positive predictive value, PPV = 92.8%; negative predictive value, NPV = 89%) when the test were combined in series, and a Se and Sp of 96.6 and 78.8% (PPV = 92.8%; NPV = 89%) when combined in parallel. Results for a broad range of hypothetical prevalence values are presented in Figure 1 to illustrate the expected variation on the PPV and NPV for alternative scenarios of disease prevalence when those two techniques were combined in parallel.
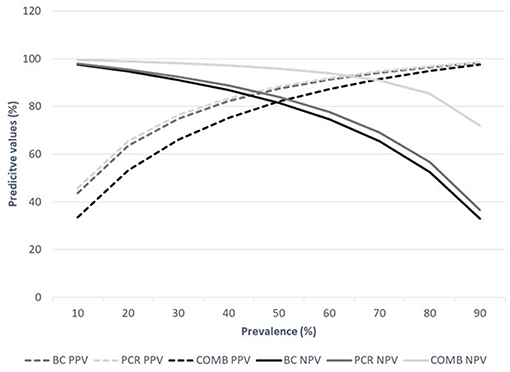
Figure 1. Estimates of predictive values for a range of prevalence values based on the Se and Sp obtained in the analyses presented here. PPV, Positive predictive value; NPV, Negative predictive value; BC, Bacteriological culture; PCR, Polymerase Chain Reaction; COMB, Combined techniques in parallel.
Results were not sensitive to the selection of the prior distributions, as suggested by the relatively consistency (magnitudes of percent differences <9%) in the results when using non-informative priors (Supplementary Table 1), to the posterior distribution of Se and Sp for both tests except for the Sp of the tests in which a reduction of 26.6% for the culture and 29.1 for the PCR was estimated.
Discussion
Although significant improvements have been made in the last 20 years, control and eradication of bTB continues to be a major challenge for Latin American countries (36, 37). Furthermore, the disease has re-emerged in humans in the region, reinforcing the need to understand the role of domestic and wild animals on the disease epidemiology, and, most importantly, the need for developing new strategies to effectively and efficiently prevent and control disease spread (37–40). Some of the challenges associated with bTB diagnosis in swine include presence of fewer bacilli in bTB lesions compared to other species (41, 42), relatively high susceptibility to avian TB infection, being those lesions undistinguishable from those produced by M. bovis (20, 32), and the lack, despite some promising advances (43), of simple, affordable, and sensitive diagnostic tools to identify infected animals at the farm level, like the PPD test use routinely in cattle (43–45). Here, we provided evidence suggesting that the PCR may be used as an effective tool for the rapid and effective detection of the infection in swine routinely inspected at slaughterhouses in Argentina. Ultimately, results presented here will help to inform decisions intended to update control strategies in endemic settings.
The high frequency of M. bovis infection in bTB-like lesions estimated in this assay (74.39%), suggests that the disease continues to be prevalent in swine populations of Argentina. Furthermore, the figure is substantially higher compared to those reported elsewhere (12, 32), who detected MTC in 32.7% of TBL samples from South West Iberian Peninsula and absence of MTC positive results from samples obtained in Sao Paulo region, respectively. Still, the high frequency (31%) of bTB reported in wild boar samples from the south of Brazil (46) would suggest the need for further studies, increasing the sample size, and targeting specific risk populations. The relative high bTB prevalence in Argentine swine is likely associated with different rearing systems. Such conclusion is also supported by the observation that the risk for bTB is relatively high in pigs reared in extensive, small (<50 sow) farms that are co-located with cattle (unpublished data). In this regard, unfortunately, not much evidence has been reported regarding the interspecies dynamic of transmission of bovine tuberculosis in farms where the cohabitation with other species, like cattle, exits. A report from the Association of Veterinarians of Buenos Aires province (47) provided preliminary evidence that supports this hypothesis, describing a different frequency of slaughterhouse findings of TBL based on rearing system being more frequent in extensive farms. Biosecurity in extensive farm is usually less strict than in intensive systems, increasing the risk for bTB (48).
The Se of the PCR test estimated here (83%) is similar to that reported for cattle (10), and higher than values previously reported for pig (12). The difference may be explained, at least in part, by differences in the study design, given that previous studies have used BC as the gold standard, whereas we have used a Bayesian approach that does not make use of such a questionable assumption. Another explanation may be that we used a larger sample size (4–7 g range of homogenized tissue for initial extraction) compared to previous studies (10, 12, 49). Increasing the volume of the samples may have increased the analytical Se (and thus the diagnostic test Se) of the test, without affecting the test Sp (13). One study (12) reported a detection rate of 77.3% of culture positive samples by RT-PCR and others (31) showed a Se of 66.1%, compared to 80.1% obtained in this work using direct PCR. Only one other study (49), reported a frequency of PCR-positive results similar to ours, working with bovine samples.
The bTB control program in Argentine swine is voluntary and its implementation is based on the use of PPD test at farm level and identification of bTB-like lesions in swine without the use of a confirmatory test. Here, we estimated that ~25% of the bTB-like lesions observed at the time of slaughtering in swine are non-infected, suggesting a Sp of the inspection of ~75% (95% CI: 63–85%). Furthermore, results suggest that the PCR may be used as a rapid, effective, confirmatory test for bTB-like lesions detected in pigs at the time of slaughtering, given that the test accuracy was similar (and on average, slightly higher) to that reported for the BC. Furthermore, the significant reduction of the turnaround time between the sample submission and the result would facilitate the follow up actions on positive cases by the sanitary authority. As expected, in-series combination of the tests impaired the combined Se value (66.6%), making it not suitable as a screening protocol. Conversely, in scenarios of relatively high bTB prevalence, such as those observed in Argentina, the in-parallel use of the BC and PCR (Figure 1) showed a good performance suggesting that the combined used of those techniques would be appropriate for the confirmation of the disease in bTB-like lesions. Consequently, it is recommended that bTB-like lesions were run by PCR and the positive samples be considered bTB infected, whereas negative samples would be run by BC, and considered as infected if positive, and non-infected otherwise. Moreover, the NPV (probability that a sample negative to a screening test was non-infected) and PPV (probability that a sample positive to a screening test was infected) of the in parallel use of both tests (Figure 1) showed that this combination would be suitable for a wide range of prevalence. The combination of both tests showed much better NPV than the individual tests (% of increase, 8.5–35.3%) with prevalence values higher than 0.4; however, the combined used of the tests did not substantially impact the PPV, showing <1–8.5% reductions. Similar findings were also reported elsewhere (50–52). Results suggest that the combined use of those techniques would be appropriate for disease confirmation in bTB-like lesions and in the context of a TB control plan. The use of PCR as a routine confirmatory technique is commonly questioned taking into account only the associated direct costs (53). However, direct application of the PCR technique in tissues brings certain benefits such as the reduction of both the laboratory turnaround time and indirect costs associated with maintenance of personnel and facilities. Furthermore, bacteriological culture required significant investments in personnel training and the biosafety protocols and facilities, as it represents a much greater risk of exposure to the agent (Table 1).
In conclusion, these results suggest that bTB is still highly prevalent in swine populations of Argentina, and that the PCR may serve as an effective and rapid test for the confirmation of the agent in bTB-like lesions macroscopically detected at the time of slaughtering in the country. The results here may ultimately help to update current strategies used to prevent and control of the disease in settings in which the disease is yet-to-be eradicated.
Author Contributions
SB, MP, and AP conceived and performed the statistical analysis. SB and MP drafted the manuscript. MJM, MM, NA, MZ, and SB participated in the generation, collection and curation of the data, and collaborated in interpretation of the results. SB and AP designed the study and coordinated the work. All authors read and approved the final manuscript.
Funding
This study was supported by the UBACyT Project: 20020130100082 (2014–2017) from the University of Buenos Aires.
Conflict of Interest Statement
The authors declare that the research was conducted in the absence of any commercial or financial relationships that could be construed as a potential conflict of interest.
Acknowledgments
We thank Juan Botto, Natalia Botto, and Andrea Sala (slaughterhouse workers) and Jorge Neira (veterinarian from the Official Veterinary Service (SENASA) for their technical support.
Supplementary Material
The Supplementary Material for this article can be found online at: https://www.frontiersin.org/articles/10.3389/fvets.2019.00204/full#supplementary-material
References
1. Brosch R, Gordon SV, Marmiesse M, Brodin P, Buchrieser C, Eiglmeier K, et al. A new evolutionary scenario for the Mycobacterium tuberculosis complex. Proc Natl Acad Sci USA. (2002) 99:3684–9. doi: 10.1073/pnas.052548299
2. Olea-Popelka F, Muwonge A, Perera A, Dean AS, Mumford E, Erlacher-Vindel E, et al. Zoonotic tuberculosis in human beings caused by Mycobacterium bovis—a call for action. Lancet Infect Dis. (2016) 17:e21–5. doi: 10.1016/S1473-3099(16)30139-6
3. Katale BZ, Mbugi EV, Kendal S, Fyumagwa RD, Kibiki GS, Godfrey-Faussett P, et al. Bovine tuberculosis at the human-livestock-wildlife interface: is it a public health problem in Tanzania? A review the onderstepoort. J Vet Res. (2012) 79:463. doi: 10.4102/ojvr.v79i2.463
4. Robinson PA. A history of bovine tuberculosis eradication policy in Northern Ireland. Epidemiol Infect. (2015) 143:3182–95. doi: 10.1017/S0950268815000291
5. McCallan L, Brooks C, Couzens C, Young F, McNair J, Byrne AW. Assessment of serological tests for diagnosis of bovine tuberculosis. Vet Rec. (2017) 181:90. doi: 10.1136/vr.104272
6. Gormley E, Corner LAL. Pathogenesis of Mycobacterium bovis infection: the badger model as a paradigm for understanding tuberculosis in animals. Front Vet Sci. (2017) 4:247. doi: 10.3389/fvets.2017.00247
7. Gormley AM, Anderson DP, Nugent G. Cost-based optimization of the stopping threshold for local disease surveillance during progressive eradication of tuberculosis from New Zealand wildlife. Transbound Emerg Dis. (2018) 65:186–96. doi: 10.1111/tbed.12647
8. Lahuerta-Marin A, Milne MG, McNair J, Skuce RA, McBride SH, Menzies FD, et al. Bayesian latent class estimation of sensitivity and specificity parameters of diagnostic tests for bovine tuberculosis in chronically infected herds in Northern Ireland. Vet J. (2018) 238:15–21. doi: 10.1016/j.tvjl.2018.04.019
9. Schiller I, Oesch B, Vordermeier HM, Palmer MV, Harris BN, Orloski KA, et al. Bovine tuberculosis: a review of current and emerging diagnostic techniques in view of their relevance for disease control and eradication. Transbound Emerg Dis. (2010) 57:205–20. doi: 10.1111/j.1865-1682.2010.01148.x
10. Courcoul A, Moyen JL, Brugère L, Faye S, Hénault S, Gares H, et al. Estimation of sensitivity and specificity of bacteriology, histopathology and PCR for the confirmatory diagnosis of bovine tuberculosis using latent class analysis. PLoS ONE. (2014) 9:e90334. doi: 10.1371/journal.pone.0090334
11. de la Rua-Domenech R. Human Mycobacterium bovis infection in the United Kingdom: incidence, risks, control measures and review of the zoonotic aspects of bovine tuberculosis. Tuberculosis. (2006) 86:77–109. doi: 10.1016/j.tube.2005.05.002
12. Cardoso-Toset F, Luque I, Amarilla SP, Gómez-Gascón L, Fernández L, Huerta B, et al. Evaluation of rapid methods for diagnosis of tuberculosis in slaughtered free-range pigs. Vet J. (2015) 204:232–4. doi: 10.1016/j.tvjl.2015.01.022
13. Fell S, Bröckl S, Büttner M, Rettinger A, Zimmermann P, Straubinger RK. Two alternative DNA extraction methods to improve the detection of Mycobacterium-tuberculosis-complex members in cattle and red deer tissue samples. BMC Microbiol. (2016) 16:213. doi: 10.1186/s12866-016-0816-2
14. Taylor GM, Worth DR, Palmer S, Jahans K, Hewinson RG. Rapid detection of Mycobacterium bovis DNA in cattle lymph nodes with visible lesions using PCR. BMC Vet Res. (2007) 3:12. doi: 10.1186/1746-6148-3-12
15. Parra A, García N, García A, Lacombe A, Moreno F, Freire F, et al. Development of a molecular diagnostic test applied to experimental abattoir surveillance on bovine tuberculosis. Vet Microbiol. (2008) 127:315–24. doi: 10.1016/j.vetmic.2007.09.001
16. Araújo CP, Osório ALAR, Jorge KSG, Ramos CAN, Souza Filho AF, Vidal CES, et al. Direct detection of Mycobacterium tuberculosis complex in bovine and bubaline tissues through nested-PCR. Braz J Microbiol. (2014) 45:633–40. doi: 10.1590/S1517-83822014000200035
17. Torres P. Bovine Tuberculosis in Argentina, Current Situation. Buenos Aires (2016). Retrieved from: http://www.senasa.gob.ar/sites/default/files/ARBOL_SENASA/ANIMAL/BOVINOS_BUBALINOS/PROD_PRIMARIA/SANIDAD/ENF_Y_ESTRAT/TUBERCULOSIS/situacion_tuberculosis_bovina_rep._argentina_2015.pdf
18. Barandiaran S, Martínez Vivot M, Moras EV, Cataldi AA, Zumárraga MJ. Mycobacterium bovis in Swine: spoligotyping of isolates from Argentina. Vet Med Int. (2011) 2011:979647. doi: 10.4061/2011/979647
19. Barandiaran S, Martínez Vivot M, Pérez AM, Cataldi AA, Zumárraga MJ. Bovine tuberculosis in domestic pigs: genotyping and distribution of isolates in Argentina. Res Vet Sci. (2015) 103:44–50. doi: 10.1016/j.rvsc.2015.09.013
20. Barandiaran S, Perez AM, Giofre AK, Martinez Vivot M, Cataldi AA, Zumarraga MJ. Tuberculosis in swine co-infected with Mycobacterium avium subsp. hominissuis and Mycobacterium bovis in a cluster from Argentina. Epidemiol Infect. (2015)143:966–74. doi: 10.1017/S095026881400332X
21. de la Rua-Domenech R, Goodchild AT, Vordermeier HM, Hewinson RG, Christiansen KH, Clifton-Hadley RS. Ante mortem diagnosis of tuberculosis in cattle: a review of the tuberculin tests, γ-interferon assay and other ancillary diagnostic techniques. Res Vet Sci. (2006) 81:190–210. doi: 10.1016/j.rvsc.2005.11.005
22. de Lisle GW, Bengis RG, Schmitt SM, O'Brien DJ. Tuberculosis in free-ranging wildlife: detection, diagnosis and management. Revue Sci Tech. (2002) 21:317–34. doi: 10.20506/rst.21.2.1339
23. Buddle BM, de Lisle GW, Griffin JFT, Hutchings SA. Epidemiology, diagnostics, and management of tuberculosis in domestic cattle and deer in New Zealand in the face of a wildlife reservoir. N Z Vet J. (2015) 63 (Suppl 1):19–27. doi: 10.1080/00480169.2014.929518
24. Branscum AJ, Gardner IA, Johnson WO. Estimation of diagnostic-test sensitivity and specificity through Bayesian modeling. Prevent Vet Med. (2005) 68:145–63. doi: 10.1016/j.prevetmed.2004.12.005
25. Al-Mouqatea S, Alkhamis M, Akbar B, Ali A, Al-Aqeel H, Bin-Heji A, et al. Bayesian estimation of ELISA and gamma interferon test accuracy for the detection of bovine tuberculosis in caudal fold test-negative dairy cattle in Kuwait. J Vet Diagnost Invest. (2018) 30:468–70. doi: 10.1177/1040638718759574
26. Latini O, Amadio G, Di Lonardo M, Barrera L, Kantor I. Metodos Bacteriologicos Empleados En El Diagnostico de la Tuberculosis en la Argentina: Evaluacion de la Calidad. Boletín de la Oficina Sanitaria Panamericana (OSP);104. (1988). Retrivered from: http://iris.paho.org/xmlui/handle/123456789/15779
27. Hermans PW, Schuitema AR, Van Soolingen D, Verstynen CP, Bik EM, Thole JE, et al. Specific detection of Mycobacterium tuberculosis complex strains by polymerase chain reaction. J Clin Microbiol. (1990) 28:1204–13.
28. Zumárraga MJ, Meikle V, Bernardelli A, Abdala A, Tarabla H, Romano MI, et al. Use of touch-down polymerase chain reaction to enhance the sensitivity of Mycobacterium bovis detection. J Vet Diagnost Invest. (2005) 17:232–8. doi: 10.1177/104063870501700303
29. Kamerbeek J, Schouls L, Kolk A, van Agterveld M, van Soolingen D, Kuijper S, et al. Simultaneous detection and strain differentiation of Mycobacterium tuberculosis for diagnosis and epidemiology. J Clin Microbiol. (1997) 35:907–14.
30. Guerrero C, Bernasconi C, Burki D, Bodmer T, Telenti A. A novel insertion element from Mycobacterium avium, IS1245, is a specific target for analysis of strain relatedness. J Clin Microbiol. (1995) 33:304–7.
31. Santos N, Geraldes M, Afonso A, Almeida V, Correia-Neves M. Diagnosis of tuberculosis in the wild boar (sus scrofa): a comparison of methods applicable to hunter-harvested animals. PLoS ONE. (2010) 5:12663. doi: 10.1371/journal.pone.0012663
32. Lara GHB, Ribeiro MG, Leite CQF, Paes AC, Guazzelli A, Silva AV, et al. Occurrence of Mycobacterium spp. and other pathogens in lymph nodes of slaughtered swine and wild boars (Sus scrofa). Res Vet Sci. (2011) 90:185–8. doi: 10.1016/j.rvsc.2010.06.009
33. Dendukuri N, Joseph L. Bayesian approaches to modeling the conditional dependence between multiple diagnostic tests. Biometrics. (2001) 57:158–67. doi: 10.1111/j.0006-341X.2001.00158.x
34. de Blas I, Ruiz-Zarzuela I, Vallejo A. WinEpi: Working In Epidemiology. An Online Epidemiological Tool. In: International Symposium on Veterinary Epidemiology and Economics. Cairns, QLD: 11th ISVEE (2006).
35. Kostoulas P, Nielsen SS, Branscum AJ, Johnson WO, Dendukuri N, Dhand NK, et al. STARD-BLCM: standards for the reporting of diagnostic accuracy studies that use bayesian latent class models. Prev Vet Med. (2017) 138:37–47. doi: 10.1016/j.prevetmed.2017.01.006
36. Picasso C, Alvarez J, VanderWaal KL, Fernandez F, Gil A, Wells SJ, et al. Epidemiological investigation of bovine tuberculosis outbreaks in Uruguay (2011–2013). Prev Vet Med. (2017) 138:156–61. doi: 10.1016/j.prevetmed.2017.01.010
37. WHO OIE FAO. Roadmap for Zoonotic Tuberculosis. (p. 21). WHO Press (2017). Retrieved from: http://www.who.int/
38. Abalos P, Retamal P. Tuberculosis: a re-emerging zoonosis? Rev Sci Techn de l'OIE. (2004) 23:583–94. doi: 10.20506/rst.23.2.1502
39. Zumárraga MJ, Arriaga C, Barandiaran S, Cobos-Marín L, de Waard J, Estrada-Garcia I, et al. Understanding the relationship between Mycobacterium bovis spoligotypes from cattle in Latin American Countries. Res Vet Sci. (2013) 94:9–21. doi: 10.1016/j.rvsc.2012.07.012
40. Silva MR, da Rocha AS, Araújo FR, Fonseca-Júnior AA, Alencar AP, de Suffys PN, et al. Risk factors for human Mycobacterium bovis infections in an urban area of Brazil. Mem Do Instit Oswaldo Cruz. (2018) 113:e170445. doi: 10.1590/0074-02760170445
41. Nugent G. Maintenance, spillover and spillback transmission of bovine tuberculosis in multi-host wildlife complexes: a New Zealand case study. Vet Microbiol. (2011) 151:34–42. doi: 10.1016/j.vetmic.2011.02.023
42. García-Jiménez WL, Salguero FJ, Fernández-Llario P, Martínez R, Risco D, Gough J, et al. Immunopathology of granulomas produced by Mycobacterium bovis in naturally infected wild boar. Vet Immunol Immunopathol. (2013) 156:54–63. doi: 10.1016/j.vetimm.2013.09.008
43. Thomas J, Infantes-Lorenzo JA, Moreno I, Cano-Terriza D, de Juan L, García-Bocanegra I, et al. Validation of a new serological assay for the identification of Mycobacterium tuberculosis complex-specific antibodies in pigs and wild boar. Prevent Vet Med. (2019) 162:11–7. doi: 10.1016/j.prevetmed.2018.11.004
44. Magnano GG, Schneider MO, Urbani CE, Ambrogi A, Zapata L, Jorge MC. Comparación de Técnicas Diagnósticas de Tuberculosis Porcina en dos Establecimientos De Cría Confinada en Argentina. InVet, 25–31 (2010). Retrieved from: http://www.scielo.org.ar/scielo.php?script=sci_arttextandpid=S1668-34982010000100004andlang=pt
45. de la Cruz ML, Branscum AJ, Nacar J, Pages E, Pozo P, Perez A, et al. Evaluation of the performance of the IDvet IFN-Gamma test for diagnosis of bovine tuberculosis in Spain. Front Vet Sci. (2018) 5:229. doi: 10.3389/fvets.2018.00229
46. Maciel ALG, Loiko MR, Bueno TS, Moreira JG, Coppola M, Dalla Costa ER, et al. Tuberculosis in Southern Brazilian wild boars (Sus scrofa): first epidemiological findings. Transbound Emerg Dis. (2018) 65:518–26. doi: 10.1111/tbed.12734
47. Dumrauf D, Passucci G, Rodriguez G, Diaz M. Hallazgos patologicos en la faena de cerdos adultos. Revista del Colegio de Veterinarios de la Provincia de Buenos Aires. (2015).
48. Dibarbora M, Cappuccio JA, Aznar MN, Bessone FA, Piscitelli H, Pereda AJ, et al. Detección serológica de Brucella suis, virus de influenza y virus de la enfermedad de Aujeszky en criaderos porcinos familiares de menos de 100 madres en Argentina. Rev Argent Microbiol. (2017) 49:158–65. doi: 10.1016/j.ram.2016.09.010
49. Stewart LD, McNair J, McCallan L, Gordon A, Grant IR. Improved detection of Mycobacterium bovis Infection in Bovine lymph node tissue using immunomagnetic separation (IMS)-based methods. PLoS ONE. (2013) 8:e58374. doi: 10.1371/journal.pone.0058374
50. Toft N, Åkerstedt J, Tharaldsen J, Hopp P. Evaluation of three serological tests for diagnosis of Maedi-Visna virus infection using latent class analysis. Vet Microbiol. (2007) 120:77–86. doi: 10.1016/j.vetmic.2006.10.025
51. de Arruda MM, Figueiredo FB, Marcelino AP, Barbosa JR, Werneck GL, Noronha EF, et al. Sensitivity and specificity of parallel or serial serological testing for detection of canine Leishmania infection. Mem Do Inst Oswaldo Cruz. (2016) 111:168–73. doi: 10.1590/0074-02760150364
52. Franco F, Di Napoli A. Evaluation of diagnostic tests in parallel and in series. G Di Tecniche Nefrologiche e Dialitiche. (2016) 28:212–5. doi: 10.5301/GTND.2016.15992
Keywords: tuberculosis, swine, diagnosis, bacteriology culture, PCR, bayesian modeling
Citation: Barandiaran S, Pérez Aguirreburualde MS, Marfil MJ, Martínez Vivot M, Aznar N, Zumárraga M and Perez AM (2019) Bayesian Assessment of the Accuracy of a PCR-Based Rapid Diagnostic Test for Bovine Tuberculosis in Swine. Front. Vet. Sci. 6:204. doi: 10.3389/fvets.2019.00204
Received: 29 January 2019; Accepted: 07 June 2019;
Published: 26 June 2019.
Edited by:
Marta Hernandez-Jover, Charles Sturt University, AustraliaReviewed by:
Krishna Thakur, University of Prince Edward Island, CanadaPeter Campbell Thomson, University of Sydney, Australia
Shumaila Arif, Charles Sturt University, Australia
Copyright © 2019 Barandiaran, Pérez Aguirreburualde, Marfil, Martínez Vivot, Aznar, Zumárraga and Perez. This is an open-access article distributed under the terms of the Creative Commons Attribution License (CC BY). The use, distribution or reproduction in other forums is permitted, provided the original author(s) and the copyright owner(s) are credited and that the original publication in this journal is cited, in accordance with accepted academic practice. No use, distribution or reproduction is permitted which does not comply with these terms.
*Correspondence: Soledad Barandiaran, c2JhcmFuQGZ2ZXQudWJhLmFy