- 1InTheRes, Université de Toulouse, INRA, ENVT, Toulouse, France
- 2Da Volterra, Paris, France
- 3Departamento de Sanidad Animal, Facultad de Veterinaria y Centro de Vigilancia Sanitaria Veterinaria (VISAVET), Universidad Complutense de Madrid, Madrid, Spain
- 4UDEAR, Université de Toulouse, INSERM, ENVT, Toulouse, France
- 5Centro Veterinario Loranca, Fuenlabrada, Spain
Quantitative data on fecal shedding of antimicrobial-resistant bacteria are crucial to assess the risk of transmission from dogs to humans. Our first objective was to investigate the prevalence of quinolone/fluoroquinolone-resistant and beta-lactam-resistant Enterobacteriaceae in dogs in France and Spain. Due to the particular concern about possible transmission of extended-spectrum cephalosporin (ESC)-resistant isolates from dogs to their owners, we characterized the ESBL/pAmpC producers collected from dogs. Rectal swabs from 188 dogs, without signs of diarrhea and that had not received antimicrobials for 4 weeks before the study, were quantified for total and resistant Enterobacteriaceae on selective media alone or containing relevant antibiotic concentrations. Information that might explain antibiotic resistance was collected for each dog. Extended-spectrum cephalosporin-resistant isolates were subjected to bacterial species identification (API20E), genetic lineage characterization (MLST), ESBL/pAmpC genes identification (sequencing), and plasmid characterization (pMLST). Regarding beta-lactam resistance, amoxicillin- (AMX) and cefotaxime- (CTX) resistant Enterobacteriaceae were detected in 70 and 18% of the dogs, respectively, whereas for quinolone/fluoroquinolone-resistance, Nalidixic acid- (NAL) and ciprofloxacin- (CIP) resistant Enterobacteriaceae were detected in 36 and 18% of the dogs, respectively. Medical rather than preventive consultation was a risk marker for the presence of NAL and CIP resistance. CTX resistance was mainly due to a combination of specific ESBL/pAmpC genes and particular conjugative plasmids already identified in human patients: blaCTX−M−1/IncI1/ST3 (n = 4), blaCMY−2/IncI1/ST12 (n = 2), and blaCTX−M−15/IncI1/ST31 (n = 1). blaSHV−12 (n = 3) was detected in various plasmid lineages (InI1/ST3, IncI1/ST26, and IncFII). ESBL/pAmpC plasmids were located in different genetic lineages of E. coli, with the exception of two strains in France (ST6998) and two in Spain (ST602). Our study highlights dogs as a potential source of Q/FQ-resistant and ESBL/pAmpC-producing bacteria that might further disseminate to humans, and notably a serious risk of future acquisition of CTX-M-1 and CMY-2 plasmids by the owners of dogs.
Introduction
Extended-spectrum and plasmidic-AmpC beta-lactamases (ESBL/pAmpC), which cause resistance to extended-spectrum cephalosporins (ESCs), are of considerable concern in veterinary and human medicine. This is because resistance to ESCs and co-resistance to other antimicrobial families (e.g., fluoroquinolones) limits the treatment options for infections with ESBL/pAmpC-producing bacteria. ESCs and fluoroquinolones, also used to treat animals, have been identified as critically important antibiotics in human medicine (1).
The emergence of ESBL/pAmpC-producing bacteria in animals and the environment is raising serious concerns (2, 3). In particular, the gastrointestinal tract of animals, including domestic pets, may serve as reservoirs of resistant bacteria that can cause opportunistic infections in vulnerable dogs and could be a source of contamination for humans. For example, dogs could possibly transmit ESC-resistant bacteria due to their close contacts with humans, the high consumption of beta-lactams in small animal veterinary practice (4), and the frequent occurrence of ESBL/pAmpC-producing Escherichia coli (5). Recently, human-related pathogenic strains have been identified in dogs (6), and the sharing of identical ESBL/pAmpC strains between humans and dogs from the same households has also been demonstrated (7).
Although ESBL/pAmpC producers can spread clonally (8), there is evidence that mobile genetic elements carrying antimicrobial resistant genes can be transferred between bacteria, notably from commensal to pathogenic Enterobacteriaceae (9). Many studies have highlighted the existence of different E. coli bacteria harboring blaCTX−M or blaCMY−2-carrying plasmids, some being shared between human and animal strains (5). Such successful plasmids efficiently contribute to the spread of resistance determinants (10). In addition, the fact that many strains harboring blaCTX−M−1 or blaCMY−2 genes are resistant to other antimicrobial classes may also increase the threat. The use of expanded-spectrum cephalosporins and of fluoroquinolones in dogs is known to select ESBL/pAmpC producers in the fecal microbiota (11–13).
Over the past 5 years, the presence of ESBL/pAmpC genes in Enterobacteriaceae strains from the feces of healthy dogs in Europe has been reported in several studies (5, 7, 14–16). However, information on the clonality of the ESBL/pAmpC isolates and knowledge about the plasmids carrying these ESBL/pAmpC genes is much more limited (5, 15, 17, 18).
Thus, examination of the occurrence of ESBL/pAmpC bacteria together with plasmids circulating in healthy pets, and their associated co-resistance, is of major importance with respect to the risk of transfer to humans in daily situations of close contact and will also extend understanding of the dissemination and persistence of ESBL and pAmpC beta-lactamase genes.
The present study was designed to assess the prevalence of beta-lactam and quinolone/fluoroquinolone resistance in fecal Enterobacteriaceae from dogs in Spain (Madrid) and France (Toulouse), to identify the potential risk factors for the presence of antibiotic resistance, and to characterize the ESC-resistant isolates, the genes coding for and plasmids carrying ESBL/pAmpC resistance in those isolates.
Materials and Methods
Isolation of Enterobacteriaceae Strains
Between March 2013 and January 2014, fecal specimens from 269 different dogs presented at two veterinary clinics (158 at the small animal clinics of the veterinary teaching hospital in Toulouse, France and 111 at a veterinary clinic in Madrid, Spain) were sampled. These dogs were being admitted for a preventive health service, vaccination or medical consultation (disease, trauma, etc.). Dogs were included in the study if they had not received any systemic or local antimicrobial during the previous 4 weeks, according to the owner. Animals with diarrhea were not included in the study. Breed, age, weight, diet (commercial, homemade, other), and reason for the visit (preventive, medical) were recorded for each dog. According to EU law (Directive 2010/63/UE), procedures which use animals according to common veterinary practice did not require minister permission and ethics committee opinion. Sampling were performed by veterinarians in veterinary clinics. They consisted in non-invasive samples (rectal swabbing) performed on client-owned animals and were acquired with the written consent from all owners.
Samples were collected by swabbing, immediately suspended in liquid amies preservation medium (Copan eSwabs Amies liquid, Biomerieux), stored at +4°C and analyzed within 24 h after sampling. Ten-fold dilutions were prepared in 0.9% sterile saline. One hundred microliter were inoculated (i) on MacConkey agar not supplemented with antimicrobials to obtain counts of total Enterobactericeae; (ii) on MacConkey agar supplemented with amoxicillin (AMX 100 μg/mL) or cefotaxime (CTX 1.5 μg/mL) to obtain counts of penicillin- or cefotaxime-resistant Enterobacteriaceae, and (iii) on MacConkey agar supplemented with nalidixic acid (NAL 20 μg/mL) or ciprofloxacin (CIP 2 μg/mL) to obtain counts of quinolones- (Q) or fluoroquinolones- (FQ) resistant Enterobacteriaceae. These antibiotic concentrations have been chosen according to the European Committee on Antimicrobial Susceptibility Testing Clinical breakpoint (www.eucast.org) to obtain non-susceptible/resistant Enterobacteriaceae.
All batches of media supplemented with antibiotics were tested with positive (resistant) and negative (susceptible) control strains. Total or resistant Enterobacteriaceae (cfu/mL) were counted after incubating these MacConkey agar plates for 24 h at 37°C. The limit of detection was 10 cfu/mL of undiluted liquid preservative medium. The prevalence of dogs carrying resistant Enterobacteriaceae (%) was estimated. Samples containing at least 104 total Enterobacteriaceae/mL were included in the analysis to limit the risk of underestimating the prevalence. Of the 269 fecal swabs collected, 188 (n = 90 from Toulouse and n = 98 from Madrid) were included in the analysis.
Multivariate logistic regression analyses were performed to identify independent predictors (sampling site, age, weight, diet, and reason for visit) of resistance carriage (prevalence of AMX-R, CTX-R, NAL-R, and CIP-R) among the investigated animals, using Systat v13.1 version. Statistical significance was set at p < 0.05. Comparisons of resistance abundance were done using a chi-square test with the significance level set at 0.05.
Identification and Typing of CTX-Resistant Bacteria
CTX-resistant colonies of Enterobacteriaceae were collected (one colony per animal), and identified by using API 20E galleries (bioMérieux). Non-Enterobacteriaceae isolates (mostly Pseudomonas spp.) were discarded.
Multilocus sequence typing (MLST) was carried out according to the protocol described on the E. coli (http://mlst.warwick.ac.uk/mlst/dbs/Ecoli) and C. freundii (https://pubmlst.org/cfreundii) MLST website.
Antibiotic Susceptibility Testing
The antibiotic susceptibilities of CTX-R E. coli and their transconjugants were determined by disk diffusion method according to CLSI protocols (19). The following antibiotic disks were used: ampicillin (10 μg), amoxicillin (20 μg) plus clavulanic acid (10 μg), cefoxitin (30 μg), ceftazidime (30 μg), cefotaxime (30 μg), cefepime (30 μg), aztreonam (30 μg), imipenem (10 μg), streptomycin (10 μg), gentamicin (10 μg), kanamycin (30 μg), nalidixic acid (30 μg), ciprofloxacin (5 μg), tetracycline (30 μg), chloramphenicol (30 μg), trimethoprim (5 μg), and sulfonamides (300 μg) (Bio-Rad, Marnes-la-Coquette, France if available or Oxoid, Dardilly, France). The susceptibility breakpoints for all antimicrobials were those recommended by CLSI (20).
ESBL production was detected by double-disk synergy test on Mueller-Hinton agar between clavulanic acid and ceftazidime, cefotaxime, or cefepime (20, 21). AmpC beta-lactamases detection was based on the inhibitory effect of cloxacillin on AmpC production observed on plates supplemented with 200 mg/L cloxacillin. The control strains used were E. coli ATCC 25922, K. pneumoniae ATCC 700603, and K. pneumoniae CMY-2 from Pr R. Bonnet, France.
Characterization of blaESBL/AmpC Genes
After bacterial DNA extraction with a DNeasy blood and tissue kit (Qiagen, Hilden, Germany), the blaTEM, blaSHV, blaCTX−M, blaCMY−2, genes were detected by PCR and sequenced using specific previously-described primers (22, 23). Sequences were analyzed by the BLAST Internet services (www.ncbi.nlm.nih.gov/BLAST).
Transferability of the blaESBL/pAmpC Genes and Plasmid Characterization
Plasmids were transferred by conjugation to rifampin-resistant E. coli recipient strains. One-milliliter of a LB culture of the ESBL/AmpC-producing donor strain (108 cfu/mL) and one mL of the J0C0J7-rifR4 rifampicin-resistant recipient (108 cfu/mL) strain were added to 3 mL LB broth and incubated for 2 h at 37°C without agitation. Transconjugants were selected on LB agar containing 1.5 μg/mL of cefotaxime and 100 μg/mL of rifampicin. Plasmid DNA from transconjugants was purified and resolved by electrophoresis in 0.7% agarose, as described previously (24), to confirm that cells carried only one conjugative plasmid.
Plasmid replicon types were determined using the PCR-based replicon typing (PBRT) scheme (25) and plasmid sequence subtypes by applying plasmid multilocus sequence typing (pMLST) for IncI1 (http://pubmlst.org/plasmid/).
Results
Prevalence of Resistant Enterobacteriaceae
The characteristics of the dog population studied are given in Table 1. A total of 188 dogs (90 from Toulouse and 98 from Madrid), with ages ranging from 4 months to 16 years (median age 5 years), and feces containing at least 104 cfu/mL of total Enterobacteriaceae, were included in the study. Body weights ranged from 1 to 53 kg (median weight 10 kg), and most of the dogs (73%) were fed with commercial feed. Eighty-four dogs (44.5%) were admitted for medical consultation, mainly in Toulouse (36.2%), most of them for skin disorders, whereas 94 dogs (50.0%) were admitted for preventive consultation, mainly in Madrid (38.8%), the majority being admitted for vaccination.
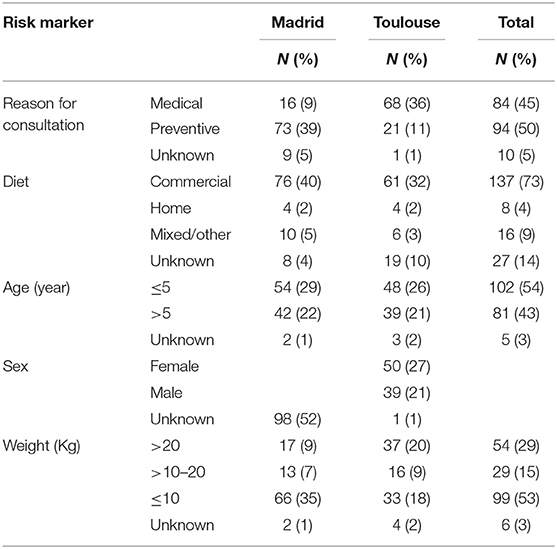
Table 1. Distribution of the predictor variable from dogs in Madrid and Toulouse veterinary clinics.
The prevalences of dogs carrying beta-lactam- or Q/FQ-resistant bacteria and its association with predictor (explanatory) variables are shown in Table 2 and Figure 1. Regarding the beta-lactam-resistant Enterobacteriaceae in feces, approximately 70% of the dogs carried AMX-R and 18% carried CTX-R Enterobacteriaceae (19% in Toulouse and 16% in Madrid). Multivariate regression analysis revealed that dogs in Toulouse were more frequently colonized by AMX-R Enterobacteriaceae (78%) than those from Madrid (63%; p < 0.01). The reason for consultation, diet, age and weight were not predictors of beta-lactam resistance carriage among animals (Table 2 and Figure 1).
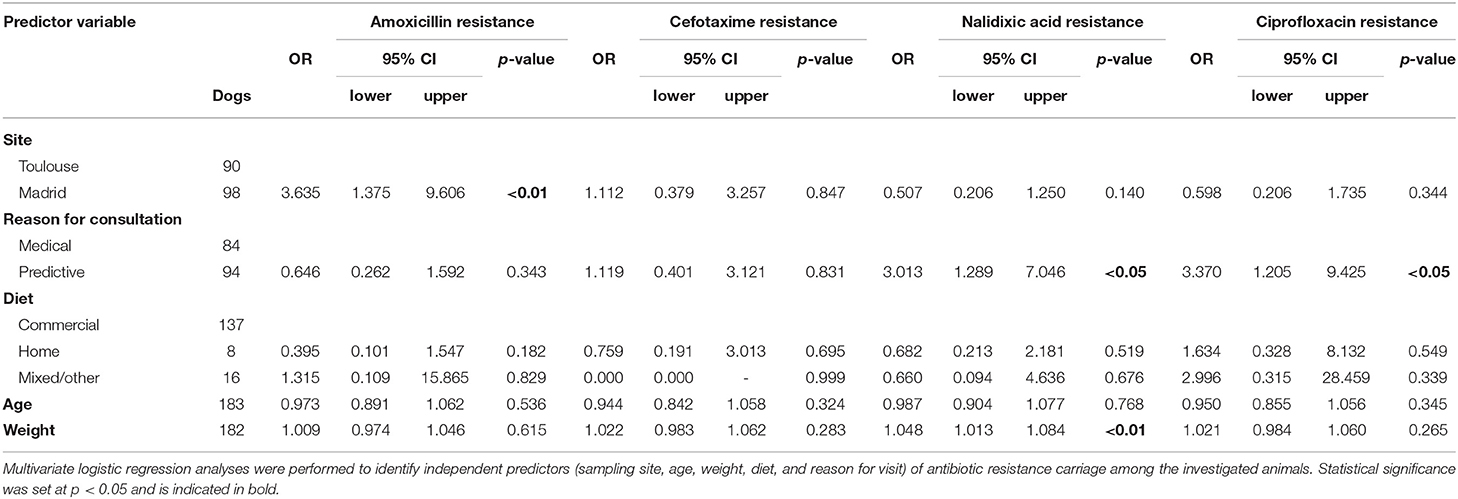
Table 2. Multivariate logistic regression analysis for predictor variable associated with antibiotic resistance carriage.
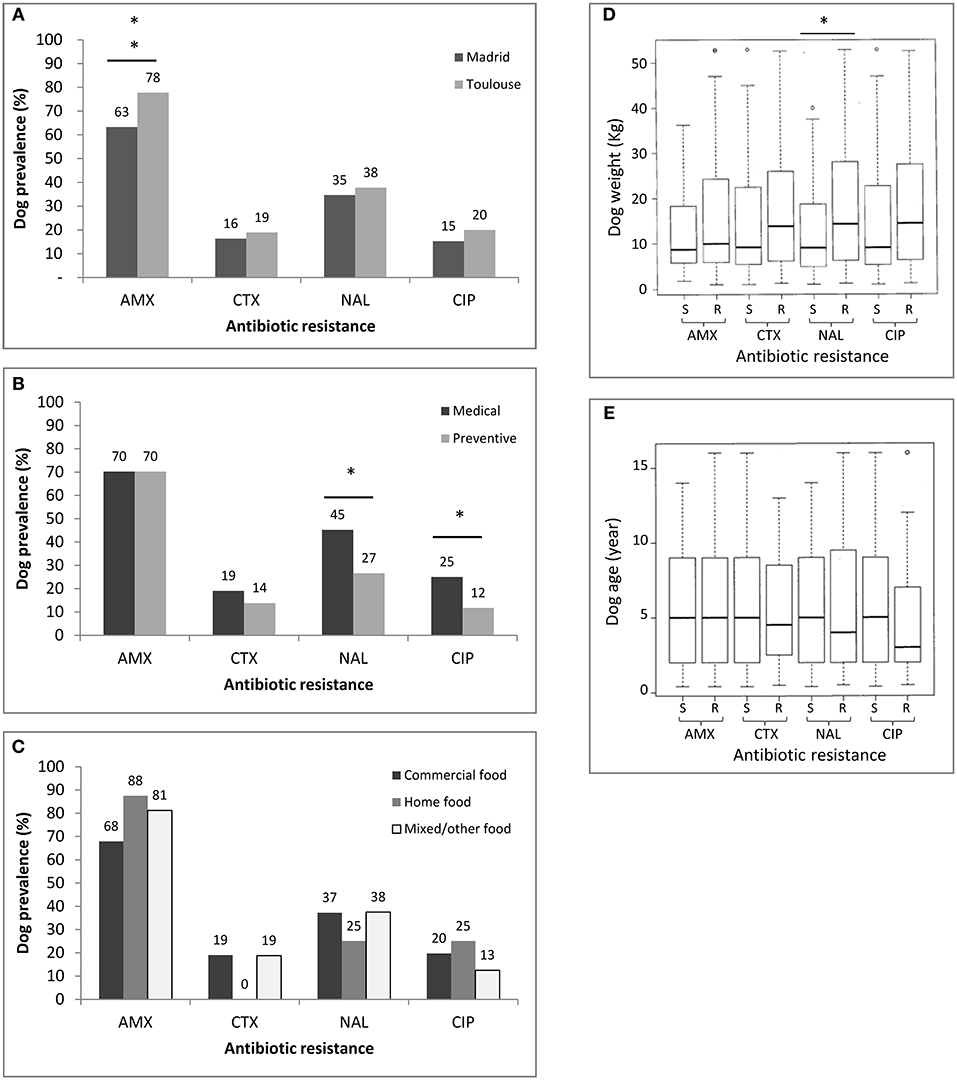
Figure 1. Prevalence of Enterobacteriaceae resistant to amoxicillin (AMX), cefotaxime (CTX), nalidixic acid (NAL), and ciprofloxacin (CIP) in the population of 188 dogs without recent antimicrobial treatment according to the predictor variable. Antibiotic resistance according to site of sampling (Madrid vs. Toulouse) (A), the reason for consultation (Medical vs. preventive) (B), the type of diet (C), dog weight (D), and dog age (E). Possible associations between sampling site, age, weight, diet, reason for visit, and carriage of antibiotic resistance were analyzed by logistic regression models. Asterisks indicate a significant difference, **p < 0.01 and *p < 0.05. S, susceptible; R, resistant.
Regarding quinolone/fluoroquinolone-resistant Enterobacteriaceae, the overall frequency of carriage of NAL-R bacteria was 36% (38% in Toulouse and 35% in Madrid) and that of CIP-R bacteria was 18% (20% in Toulouse and 15% in Madrid). The multivariate logistic regression analysis indicated that the reason for the dog's visit to the veterinary clinic was a predictor of Q/FQ resistance (p < 0.05). Animals brought in for preventive measures were less likely to carry Enterobacteriaceae resistant to Q/FQ than those presented for medical treatment (27 vs. 45% for NAL and 12 vs. 25% for CIP). Dog weight was also found to be a predictor of resistance prevalence, but only for NAL (p < 0.01). The population of dogs harboring fecal bacteria resistant to NAL weighed more (median = 14.30 kg) than the population of dogs without resistant bacteria (median = 9.10 kg). A similar trend, but without statistical significance, was apparent for other antibiotics (CTX and CIP).
Characterization of ESBL/AmpC Producers, Genes, Plasmids
ESBL/AmpC genes and the plasmids carrying them were identified in 14 cefotaxime-R Enterobacteriaceae isolated from the feces (Table 3). They included 10 ESBL-producing E. coli and 4 AmpC-carrying strains (2 E. coli, 1 P. mirabilis having acquired AmpC enzyme and 1 C. freundii that carries naturally a chromosomal AmpC). According to the MLST database, the 12 E. coli were associated with 10 different sequence types (STs) of which nine have been previously reported. ESBL-producing E. coli presented multiple associated resistances. All strains were resistant to sulfonamides and tetracycline, and some to trimethoprim (5/10), chloramphenicol (4/10), and streptomycin (2/10). ESBL-producers in Madrid were always co-resistant to NAL and CIP whereas those isolated in Toulouse were not, despite the presence of a significant level of Q/FQ resistant strains in the feces. The ESBL phenotype was mostly due to the blaCTX−M−1 gene (n = 6), whereas the blaCTX−M−15 gene (n = 1 in Toulouse) was rare. The 6 blaCTX−M−1 genes were systematically carried by the conjugative IncI1/ST3 plasmid subtype that conferred additional resistance to tetracyclines and sulphonamide. The blaCTX−M−1 IncI1/ST3 plasmid was found in isolates belonging to four different STs (ST6998 (n = 2), ST227 (n = 1), and ST38 (n = 1) in Toulouse and ST602 (n = 2) in Madrid). The blaCTX−M−15 gene was on a conjugative IncI1/ST31 plasmid which did not confer other resistances to its host strain which belonged to ST2449. Three blaSHV−12 genes were also identified, only in Madrid, and were carried by a diversity of conjugative plasmids found in MLST clonally unrelated E. coli: blaSHV−12 IncI1/ST3 plasmid associated with ST453(STC86), blaSHV−12 IncI1/ST26 with a new ST and blaSHV−12 IncFII plasmid with ST1594.
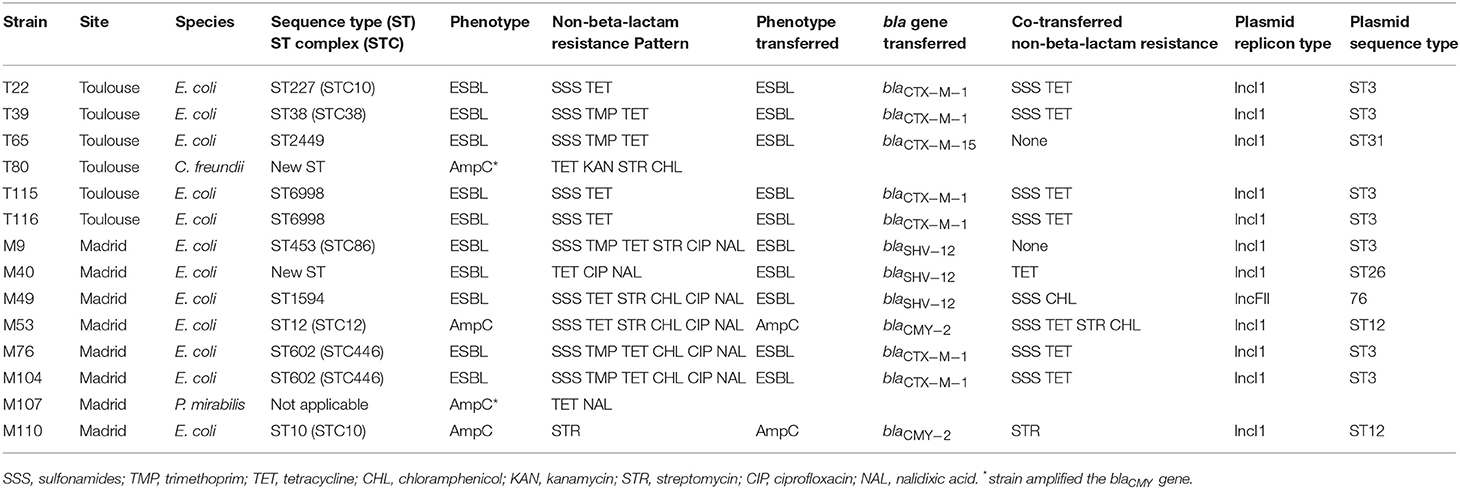
Table 3. Extended-spectrum cephalosporinase of Enterobacteriaceae isolated from feces of healthy dogs in France and Spain and molecular characterization of blaCTX−M and blaCMY genes and the plasmids carrying them.
AmpC beta-lactamases were detected in 4 isolates: 1 C. freundii isolated from Toulouse dogs and 2 E. coli, 1 P. mirabilis from Madrid. As CMY-2 is the most common type of AmpC and pAmpC enzymes among Enterobacteriaceae, we have focused on the detection of blaCMY−2 gene. For the 2 E. coli, belonging to ST10 and ST12, the blaCMY−2 gene was carried by the IncI1/ST12 conjugative plasmid, one carrying only streptomycin resistance while the second was associated with multiple resistances such as sulfonamide, tetracycline, streptomycin and chloramphenicol. The remaining 2, the blaCMY−2 gene in P. mirabilis and the blaCMY−80 gene in C. freundii, were not located on conjugative plasmids.
Discussion
The resistance phenotypes investigated in this study are of high clinical relevance in small animal veterinary practice in view of the widespread use of beta-lactams and fluoroquinolones for treating common infections in dogs (4) and the potential risk of resistance transmission (bacteria or genes) to humans through direct contact (1).
Prevalence of Resistant Enterobacteriaceae
Even if comparison between different studies is difficult due to biases associated with methodological factors, the high prevalences of AMX-R (70%) and CTX-R (18%) Enterobacteriaceae were similar to those already reported, over the last 5 years, in healthy dogs in various European countries (5, 14, 26, 27), but higher than those reported in Denmark, Sweden and the UK (7, 15, 28). In our study, the prevalence of AMX-R isolates included Enterobacteriaceae species naturally resistant to penicillin like K. pneumoniae, C. freundii, Enterobacter and those having acquired such resistance like E. coli, P. mirabilis.
Similarly, a high prevalence of NAL-R (35–38%) and CIP-R (15–20%) carriers was detected, as previously reported in Portugal (27), but higher than that reported in the UK (29). The use of critically important antimicrobials (3rd and 4th generation cephalosporin and quinolone/fluoroquinolone) is more commonly cited as being prescribed for dogs in Spain, France and Germany than in Sweden and the UK (4). We therefore believe that the observed high prevalence of cefotaxime (CTX) and Q/FQ resistance in dogs may be associated with the use of critically important antimicrobials for canine treatments.
The prevalence of AMX-R was higher in Toulouse (78%) than in Madrid (63%) but such result is difficult to interpret in light of antibiotic usage data described in the literature for the two countries (4). Dog weight (Kg) was a risk marker significantly associated with nalidixic acid resistance whereas age was not. This result is difficult to explain but the possible difficulty of precisely administering the recommended dose according to the dog's weight might sometimes lead to over-dosing (over-exposure of fecal flora to antibiotics) or under-dosing (which might necessitate a change of antibiotherapy), both situations promoting the development of antibiotic resistance.
The significantly higher prevalence of NAL-R and CIP-R in dogs presented for medical consultation has already been reported (27) and might be related to the fact that healthy animals have fewer opportunities for contact with antimicrobials. However, the past medical histories of these dogs were not recorded. Exposure to quinolones had previously been indicated as a risk factor for the emergence of resistance to several antibiotics in E. coli isolated from dogs (27) but the involvement of other antimicrobial classes (e.g., tetracyclines, sulfonamides, aminoglycosides), that are frequently used in canine therapy, cannot be excluded. Because no antimicrobial was administered to the dogs during the month prior to sampling, our results are consistent with the hypothesis that the reversibility of resistance in the absence of antimicrobials is a slow process.
It is noteworthy that as almost 20% of the dogs, independently of origin, were CTX-R (ESBL or AmpC) or FQ-R carriers, the owners (including family members) could be at risk of acquiring these resistant bacteria and genes from their dogs (7) especially if they are carried by conjugative plasmids. FQ resistance was mainly mediated by mutation in chromosomic genes whereas plasmid-mediated FQ resistance (PMQR) determinants only confer low-level resistance to fluoroquinolones. On the 16 CIP-R and 27 NAL-R Enterobacteriaceae isolated in our study, we performed the detection for predominant PMQR qnrA, qnrB, qnrS, and aac(6′)- Ib-cr by PCR as described elsewhere (30, 31). No PMQR were detected (data not shown) but we know that among fluoroquinolone non-wild Enterobacteriaceae, only 20% carried at least one PMQR determinant (32).
Characterization of ESBL/AmpC Producers, Genes, and Plasmids
Among the ESBL genes identified in Enterobacteriaceae isolated from different dogs in the two countries, the blaCTX−M−1 gene was exclusively located on the specific conjugative IncI1/ST3 plasmid. Although this study is the first to identify blaCTX−M−1 on IncI1/ST3 plasmid in Spain, such a combination has recently been reported in France in healthy urban dogs (5), in both diseased and healthy humans (33) but also in food-producing animals and the environment (22, 34). The blaCTX−M−1 on IncI1/ST3 plasmid was detected in four different E. coli ST, including ST38 and ST602 which have frequently been identified in different situations (human pathogens or not, livestock, environment) but are only rarely found in dogs. The E. coli ST38 and ST602 lineages carrying blaCTX−M−1 on IncI1/ST3 plasmids have previously been reported in food-producing animals in Portugal (35) and healthy dogs (36) in Tunisia, respectively. The diversity of the analyzed strains (date and place of isolation) and the diversity of the E. coli ST, in contrast to the homogeneity of the blaCTX−M−1 plasmid type, confirm the specific capability of the blaCTX−M−1-carrying IncI1/ST3 plasmid to disseminate through unrelated bacterial strains.
In our study, one blaCTX−M−15/IncI1/ST31 conjugative plasmid, carrying an additional blaTEM−1 gene without additional resistance, was identified in a French isolate. Such a plasmid has already been detected in E.coli and Shigella sonnei clinical isolates from cattle and humans in different European countries (37, 38). This plasmid was found in E. coli ST2449 which has been reported once for a human pathogen in Europe and twice on the American continent for animal pathogens (http://enterobase.warwick.ac.uk/species/ecoli/search_strains).
Three blaSHV−12 genes were identified only in Spain and were carried by three different conjugative plasmids: the blaSHV−12 IncI1/ST3 plasmid previously reported in 2 E. coli of poultry origin in Italy (39), the blaSHV−12 IncI1/ST26 plasmid previously identified in E. coli of human origin in Italy (39), from food animals and products in Portugal (35) and the blaSHV−12 F76:A-:B- FII plasmid not yet described. In addition, SHV-12-carrying plasmids were located in three different genetic lineage strains. Among them, a new ST and 2 other STs (ST453, ST1594) that have occasionally been detected in livestock and poultry on different continents as well as in the environment and humans for ST453 (40, 41). The ability of blaSHV−12 genes to combine with different plasmids allows the enzymes to attain diverse niches worldwide, as previously demonstrated (42). Prevalence of the blaSHV−12 gene seemed higher in Spain but the number of cefotaxime-R isolates in France was too small to draw a conclusion.
Three AmpC were detected among the eight cefotaxime-R Enterobacteriaceae in Spain but only one in France. The blaCMY−2 on IncI1/ST12 plasmid was the only conjugative plasmid-encoded pAmpC gene that we detected in two different E. coli lineages (ST10 and ST12). The E. coli ST10 and ST12 have repeatedly been isolated from various reservoirs i.e., healthy or diseased animals including dogs, humans and the environment (40). Previous studies support the hypothesis that plasmids play the major role in the transmission of blaCMY−2 between reservoirs. The widespread distribution of blaCMY−2-carrying IncI1/ST12 plasmid has been reported among various E. coli STs from human patients, livestock animals, food, environment, and also from healthy dogs in Tunisia (17, 35, 36, 41, 43). However, to our knowledge, this is the first time that the blaCMY−2 IncI1/ST12 plasmid has been identified in healthy dogs in Europe although it was recently identified in a single clinical isolate associated with another ST in Denmark (17). Until now, the blaCMY−2 on IncI1/ST2 plasmid was the only one reported in healthy dogs in Europe (5, 11, 17). E. coli ST10 harboring blaCMY−2 on the IncI1/ST12 plasmid seems to circulate in European broiler production (17). In addition to food animals, which are often proposed as possible reservoirs for blaCMY−2 in humans through the spread of specific plasmids, our results also raise questions about the possible contribution, to this burden in Europe, of blaCMY−2-carrying IncI1/ST12 plasmid from companion animals.
The third AmpC-carrying isolate in Spain was a CMY-2-producing Proteus mirabilis in which mobilization of the blaCMY−2 gene does not involve a plasmid. Hybridization experiments are needed to confirm the chromosomal location of this blaCMY−2 gene. In France, a single CMY-80-producing Citrobacter freundii was found in canine feces and the blaCMY variant gene was not carried by a conjugative plasmid. Such a blaCMY variant was only found in C. freundii isolated from human urine samples in Spain (GenBank Accession Number JQ733577).
Overall, a diversity of blaESBL/pAmpC genes were found but the blaCTX−M−15 which is highly predominant in the ESBL-producers in human and dog pathogens seems minor in the commensal digestive flora (44–47). In the other hand, blaCTX−M−1 and blaSHV−12 have recently emerged in dog pathogens (45). ESBL/AmpC genes were found in different clonal lineages of E. coli but not in the most prevalent ST in human (ST131, ST648) and dog (ST410) pathogens. However, some successful ESBL/pAmpC plasmid lineages have been described in human and dog pathogens.
In our study, most of the ESBL/AmpC strain isolated from Madrid presented the broadest multiresistance pattern, showing co-resistance to ESC and FQ. Similarly, such co-resistance in E. coli from food or food-producing animals in Europe was shown to occur at the highest frequency in Spain (48). We performed the detection for predominant PMQR qnrA, qnrB, qnrS, and aac(6')- Ib-cr by PCR as described elsewhere on ESBL/AmpC strain but no PMQR were detected except a qnrA gene in the AmpC-producer C. freundii (data not shown). In our study, the low prevalence of PMQR contrast with the high prevalence previously described in dog pathogens (32, 44, 49). Anyway, multiresistance to different classes of antibiotic used in veterinary medicine, conferred by conjugative plasmids and/or by the host strains, may increase the potential risk of co-selection, maintenance, transmission, and propagation of the multidrug-resistant E. coli isolates and/or multidrug-resistant plasmids in digestive tract. Moreover, despite the few strains studied here, and in addition to the greater multiresistance reported in Spain, our results suggest that the diversity of ESBL/pAmpC genes and plasmids is greater in Spain than in France. This may be due to a geographical factor, differences in the use of antibiotics in dogs or differences in the distribution of ESBL/Ampc strains and plasmids in the environment of dogs (humans, water, and food). The sharing of ESBL/pAmpC-carrying plasmids between healthy dogs and humans is of particular concern.
In conclusion, our study reveals a high prevalence of resistance to fluoroquinolones (18%) and extended-spectrum-cephalosporins (18%) in feces from dogs in Toulouse and Madrid. The state of health of the animals is a risk factor for quinolone-resistance carriage, probably related to previous treatments. In unrelated dogs in Spain and/or France, the spread of extended-spectrum-cephalosporinases can be due to successful combination of particular ESBL/pAmpC genes and specific plasmid ST, as for blaCTX−M−1/IncI1/ST3 and blaCMY−2/IncI1/ST12. On the contrary, the blaSHV−12 gene combines with different plasmid lineages. Such highly conjugative plasmids have previously been described in healthy or pathogenic E. coli isolates of human, animal and food origin. They are located in different E. coli ST, and some are increasingly being identified in various known reservoirs. Considering the prevalence of resistance to fluoroquinolones and extended-spectrum-cephalosporinases (notably the large reservoir of CTX-M-1 and CMY-2 producers in dogs) there is a serious and plausible risk of future acquisition of CTX-M-1 and CMY-2 plasmids by their owners.
Data Availability
The datasets generated for this study are available on request. The raw data supporting the conclusions of this manuscript will be made available by the authors, without undue reservation, to any qualified researcher.
Author Contributions
VD, MA, SS-J, NS-L, BG-Z, AA, and AB-M designed the study. M-CC and DL-P collected the feces. VD, MA, GM, DB, and NA performed bacterial counts and the storage of resistant isolates. VD carried out the statistical tests and analyzed the DNA sequences. VD and NA analyzed the ESBL/AmpC isolates. VD, JdG, BG-Z, AA, and AB-M drafted the manuscript. All the authors edited the manuscript and approved the paper.
Conflict of Interest Statement
MA, SS-J, and NS-L are employees of Da Volterra. JdG and AA are consultants of Da Volterra. The authors declare that this study received funding from the SME Da Voltera. The funder had the following involvement with the study: study design, data collection, the writing of this article.
The remaining authors declare that the research was conducted in the absence of any commercial or financial relationships that could be construed as a potential conflict of interest.
Acknowledgments
The authors would like to thank Didier Concordet for his help with the statistical analyses. We would also like to thank Caroline Feuillastre for her assistance during the experiment. We are grateful to the Genotoul bioinformatics platform Toulouse Midi-Pyrenees for sequencing. The Spanish Action Plan on Antibiotic Resistance, PRAN, is acknowledged for their support.
References
1. WHO. Critically Important Antimicrobials for Human Medicine. Geneva: World Health Organization (2011).
2. Ewers C, Bethe A, Semmler T, Guenther S, Wieler LH. Extended-spectrum beta-lactamase-producing and AmpC-producing Escherichia coli from livestock and companion animals, and their putative impact on public health: a global perspective. Clin Microbiol Infect. (2012) 18:646–55. doi: 10.1111/j.1469-0691.2012.03850.x
3. Gonzalez-Zorn B, Escudero JA. Ecology of antimicrobial resistance: humans, animals, food and environment. Int Microbiol. (2012) 15:101–9. doi: 10.2436/20.1501.01.163
4. De Briyne N, Atkinson J, Pokludova L, Borriello SP. Antibiotics used most commonly to treat animals in Europe. Vet Rec. (2014) 175:325. doi: 10.1136/vr.102462
5. Haenni M, Saras E, Metayer V, Medaille C, Madec JY. High prevalence of blaCTX−M−1/IncI1/ST3 and blaCMY−2/IncI1/ST2 plasmids in healthy urban dogs in France. Antimicrob Agents Chemother. (2014) 58:5358–62. doi: 10.1128/AAC.02545-14
6. Ovejero CM, Escudero JA, Thomas-Lopez D, Hoefer A, Moyano G, Montero N, et al. Highly tigecycline-resistant klebsiella pneumoniae sequence type 11 (ST11) and ST147 isolates from companion animals. Antimicrob Agents Chemother. (2017) 61:e02640-16. doi: 10.1128/AAC.02640-16
7. Ljungquist O, Ljungquist D, Myrenas M, Ryden C, Finn M, Bengtsson B. Evidence of household transfer of ESBL-/pAmpC-producing Enterobacteriaceae between humans and dogs - a pilot study. Infect Ecol Epidemiol. (2016) 6:31514. doi: 10.3402/iee.v6.31514
8. Ewers C, Grobbel M, Stamm I, Kopp PA, Diehl I, Semmler T, et al. Emergence of human pandemic O25:H4-ST131 CTX-M-15 extended-spectrum-beta-lactamase-producing Escherichia coli among companion animals. J Antimicrob Chemother. (2010) 65:651–60. doi: 10.1093/jac/dkq004
9. Blake DP, Hillman K, Fenlon DR, Low JC. Transfer of antibiotic resistance between commensal and pathogenic members of the Enterobacteriaceae under ileal conditions. J Appl Microbiol. (2003) 95:428–36. doi: 10.1046/j.1365-2672.2003.01988.x
10. Carattoli A. Plasmids and the spread of resistance. Int J Med Microbiol. (2013) 303:298–304. doi: 10.1016/j.ijmm.2013.02.001
11. Damborg P, Gaustad IB, Olsen JE, Guardabassi L. Selection of CMY-2 producing Escherichia coli in the faecal flora of dogs treated with cephalexin. Vet Microbiol. (2011) 151:404–8. doi: 10.1016/j.vetmic.2011.03.015
12. Moreno A, Bello H, Guggiana D, Dominguez M, Gonzalez G. Extended-spectrum beta-lactamases belonging to CTX-M group produced by Escherichia coli strains isolated from companion animals treated with enrofloxacin. Vet Microbiol. (2008) 129:203–8. doi: 10.1016/j.vetmic.2007.11.011
13. Kimura A, Yossapol M, Shibata S, Asai T. Selection of broad-spectrum cephalosporin-resistant Escherichia coli in the feces of healthy dogs after administration of first-generation cephalosporins. Microbiol Immunol. (2017) 61:34–41. doi: 10.1111/1348-0421.12466
14. Hordijk J, Schoormans A, Kwakernaak M, Duim B, Broens E, Dierikx C, et al. High prevalence of fecal carriage of extended spectrum beta-lactamase/AmpC-producing Enterobacteriaceae in cats and dogs. Front Microbiol. (2013) 4:242. doi: 10.3389/fmicb.2013.00242
15. Damborg P, Morsing MK, Petersen T, Bortolaia V, Guardabassi L. CTX-M-1 and CTX-M-15-producing Escherichia coli in dog faeces from public gardens. Acta Vet Scand. (2015) 57:83. doi: 10.1186/s13028-015-0174-3
16. Belas A, Salazar AS, Gama LT, Couto N, Pomba C. Risk factors for faecal colonisation with Escherichia coli producing extended-spectrum and plasmid-mediated AmpC beta-lactamases in dogs. Vet Rec. (2014) 175:202. doi: 10.1136/vr.101978
17. Hansen KH, Bortolaia V, Nielsen CA, Nielsen JB, Schonning K, Agerso Y, et al. Host-specific patterns of genetic diversity among IncI1-Iγ and IncK plasmids encoding CMY-2 beta-lactamase in Escherichia coli isolates from humans, poultry meat, poultry, and dogs in denmark. Appl Environ Microbiol. (2016) 82:4705–14. doi: 10.1128/AEM.00495-16
18. Boehmer T, Vogler AJ, Thomas A, Sauer S, Hergenroether M, Straubinger RK, et al. Phenotypic characterization and whole genome analysis of extended-spectrum beta- lactamase-producing bacteria isolated from dogs in Germany. PLoS ONE. (2018) 13:e0206252. doi: 10.1371/journal.pone.0206252
19. CLSI. Performance Standards for Antimicobial Disk Susceptibility Tests. Approved Standard-Tenth Edition. Document M02-A10. Wayne, PA: CALS Institute (2009).
20. CLSI. Performance Standards for Antimicrobial Testing, 19th Informational Supplement. Document M100-S19. Wayne, PA: CALS Institute (2009).
21. Derbyshire H, Kay G, Evans K, Vaughan C, Kavuri U, Winstanley T. A simple disc diffusion method for detecting AmpC and extended-spectrum beta-lactamases in clinical isolates of Enterobacteriaceae. J Antimicrob Chemother. (2009) 63:497–501. doi: 10.1093/jac/dkn535
22. Dupouy V, Doublet B, Arpaillange N, Praud K, Bibbal D, Brugere H, et al. Dominant plasmids carrying extended-spectrum beta-lactamases blaCTX-M genes in genetically diverse Escherichia coli from slaughterhouse and urban wastewaters. Environ Microbiol Rep. (2016) 8:789–97. doi: 10.1111/1758-2229.12440
23. Dierikx CM, Van Duijkeren E, Schoormans AH, Van Essen-Zandbergen A, Veldman K, Kant A, et al. Occurrence and characteristics of extended-spectrum-beta-lactamase- and AmpC-producing clinical isolates derived from companion animals and horses. J Antimicrob Chemother. (2012) 67:1368–74. doi: 10.1093/jac/dks049
24. Kado CI, Liu ST. Rapid procedure for detection and isolation of large and small plasmids. J Bacteriol. (1981) 145:1365–73.
25. Carattoli A, Bertini A, Villa L, Falbo V, Hopkins KL, Threlfall EJ. Identification of plasmids by PCR-based replicon typing. J Microbiol Methods. (2005) 63:219–28. doi: 10.1016/j.mimet.2005.03.018
26. Schmidt VM, Pinchbeck GL, Nuttall T, Mcewan N, Dawson S, Williams NJ. Antimicrobial resistance risk factors and characterisation of faecal E. coli isolated from healthy Labrador retrievers in the United Kingdom. Prev Vet Med. (2015) 119:31–40. doi: 10.1016/j.prevetmed.2015.01.013
27. Leite-Martins LR, Mahu MI, Costa AL, Mendes A, Lopes E, Mendonca DM, et al. Prevalence of antimicrobial resistance in enteric Escherichia coli from domestic pets and assessment of associated risk markers using a generalized linear mixed model. Prev Vet Med. (2014) 117:28–39. doi: 10.1016/j.prevetmed.2014.09.008
28. Espinosa-Gongora C, Shah SQ, Jessen LR, Bortolaia V, Langebaek R, Bjornvad CR, et al. Quantitative assessment of faecal shedding of beta-lactam-resistant Escherichia coli and enterococci in dogs. Vet Microbiol. (2015) 181:298–302. doi: 10.1016/j.vetmic.2015.10.004
29. Wedley AL, Dawson S, Maddox TW, Coyne KP, Pinchbeck GL, Clegg P, et al. Carriage of antimicrobial resistant Escherichia coli in dogs: prevalence, associated risk factors and molecular characteristics. Vet Microbiol. (2017) 199:23–30. doi: 10.1016/j.vetmic.2016.11.017
30. Lindemann PC, Risberg K, Wiker HG, Mylvaganam H. Aminoglycoside resistance in clinical Escherichia coli and Klebsiella pneumoniae isolates from Western Norway. APMIS. (2012) 120:495–502. doi: 10.1111/j.1600-0463.2011.02856.x
31. Marti E, Balcazar JL. Real-Time PCR assays for quantification of qnr genes in environmental water samples and chicken feces. Appl Environ Microbiol. (2013) 79:1743–5. doi: 10.1128/AEM.03409-12
32. Zong Z, Ginn AN, Dobiasova H, Iredell JR, Partridge SR. Different IncI1 plasmids from Escherichia coli carry ISEcp1-blaCTX-M-15 associated with different Tn2-derived elements. Plasmid. (2015) 80:118–26. doi: 10.1016/j.plasmid.2015.04.007
33. Madec JY, Haenni M, Metayer V, Saras E, Nicolas-Chanoine MH. High prevalence of the animal-associated blaCTX−M−1 IncI1/ST3 plasmid in human Escherichia coli isolates. Antimicrob Agents Chemother. (2015) 59:5860–1. doi: 10.1128/AAC.00819-15
34. Zurfluh K, Jakobi G, Stephan R, Hachler H, Nuesch-Inderbinen M. Replicon typing of plasmids carrying blaCTX−M−1 in Enterobacteriaceae of animal, environmental and human origin. Front Microbiol. (2014) 5:555. doi: 10.3389/fmicb.2014.00555
35. Jones-Dias D, Manageiro V, Martins AP, Ferreira E, Canica M. New class 2 Integron In2-4 among IncI1-positive Escherichia coli isolates carrying ESBL and PMAβ genes from food animals in Portugal. Foodborne Pathog Dis. (2016) 13:36–9. doi: 10.1089/fpd.2015.1972
36. Ben Sallem R, Ben Slama K, Rojo-Bezares B, Porres-Osante N, Jouini A, Klibi N, et al. IncI1 plasmids carrying bla(CTX-M-1) or bla(CMY-2) genes in Escherichia coli from healthy humans and animals in Tunisia. Microb Drug Resist. (2014) 20:495–500. doi: 10.1089/mdr.2013.0224
37. Madec JY, Poirel L, Saras E, Gourguechon A, Girlich D, Nordmann P, et al. Non-ST131 Escherichia coli from cattle harbouring human-like blaCTX−M−15-carrying plasmids. J Antimicrob Chemother. (2012) 67:578–81. doi: 10.1093/jac/dkr542
38. Arvand M, Bettge-Weller G, Fruth A, Uphoff H, Pfeifer Y. Extended-spectrum beta-lactamase-producing Shiga toxin gene (stx1)-positive Escherichia coli O91:H14 carrying blaCTX−M−15 on an IncI1-ST31 plasmid isolated from a human patient in Germany. Int J Med Microbiol. (2015) 305:404–7. doi: 10.1016/j.ijmm.2015.03.003
39. Accogli M, Fortini D, Giufre M, Graziani C, Dolejska M, Carattoli A, et al. IncI1 plasmids associated with the spread of CMY-2, CTX-M-1 and SHV-12 in Escherichia coli of animal and human origin. Clin Microbiol Infect. (2013) 19:E238–40. doi: 10.1111/1469-0691.12128
40. Enterobase. (2018). Available online at: http://enterobase.warwick.ac.uk/species/ecoli/search_strains (accessed March 26, 2019).
41. Pietsch M, Irrgang A, Roschanski N, Brenner Michael G, Hamprecht A, Rieber H, et al. Whole genome analyses of CMY-2-producing Escherichia coli isolates from humans, animals and food in Germany. BMC Genomics. (2018) 19:601. doi: 10.1186/s12864-018-4976-3
42. Liakopoulos A, Mevius D, Ceccarelli D. A review of SHV extended-spectrum beta-lactamases: neglected yet ubiquitous. Front Microbiol. (2016) 7:1374. doi: 10.3389/fmicb.2016.01374
43. Alonso N, Miro E, Pascual V, Rivera A, Simo M, Garcia MC, et al. Molecular characterisation of acquired and overproduced chromosomal blaAmpC in Escherichia coli clinical isolates. Int J Antimicrob Agents. (2016) 47:62–8. doi: 10.1016/j.ijantimicag.2015.10.007
44. Dahmen S, Haenni M, Chatre P, Madec JY. Characterization of blaCTX−M IncFII plasmids and clones of Escherichia coli from pets in France. J Antimicrob Chemother. (2013) 68:2797–801. doi: 10.1093/jac/dkt291
45. Zogg AL, Simmen S, Zurfluh K, Stephan R, Schmitt SN, Nuesch-Inderbinen M. High prevalence of extended-spectrum beta-lactamase producing Enterobacteriaceae among clinical isolates from cats and dogs admitted to a Veterinary Hospital in Switzerland. Front Vet Sci. (2018) 5:62. doi: 10.3389/fvets.2018.00062
46. Blanco M, Alonso MP, Nicolas-Chanoine MH, Dahbi G, Mora A, Blanco JE, et al. Molecular epidemiology of Escherichia coli producing extended-spectrum {beta}-lactamases in Lugo (Spain): dissemination of clone O25b:H4-ST131 producing CTX-M-15. J Antimicrob Chemother. (2009) 63:1135–41. doi: 10.1093/jac/dkp122
47. Courpon-Claudinon A, Lefort A, Panhard X, Clermont O, Dornic Q, Fantin B, et al. Bacteraemia caused by third-generation cephalosporin-resistant Escherichia coli in France: prevalence, molecular epidemiology and clinical features. Clin Microbiol Infect. (2011) 17:557–65. doi: 10.1111/j.1469-0691.2010.03298.x
48. ECDC. The European Union Summary Report on Antimicrobial Resistance in Zoonotic and Indicator Bacteria From Humans, Animals and Food in 2014. European Centre for Disease Prevention and Control (2016).
Keywords: dog, feces, antibiotic resistance, ESBL/pAmpC, fluoroquinolone, plasmid
Citation: Dupouy V, Abdelli M, Moyano G, Arpaillange N, Bibbal D, Cadiergues M-C, Lopez-Pulin D, Sayah-Jeanne S, de Gunzburg J, Saint-Lu N, Gonzalez-Zorn B, Andremont A and Bousquet-Mélou A (2019) Prevalence of Beta-Lactam and Quinolone/Fluoroquinolone Resistance in Enterobacteriaceae From Dogs in France and Spain—Characterization of ESBL/pAmpC Isolates, Genes, and Conjugative Plasmids. Front. Vet. Sci. 6:279. doi: 10.3389/fvets.2019.00279
Received: 31 May 2019; Accepted: 07 August 2019;
Published: 30 August 2019.
Edited by:
Silke Salavati, University of Edinburgh, United KingdomReviewed by:
Roger Stephan, University of Zurich, SwitzerlandAbdelaziz Touati, University of Béjaïa, Algeria
Torahiko Okubo, Hokkaido University, Japan
Copyright © 2019 Dupouy, Abdelli, Moyano, Arpaillange, Bibbal, Cadiergues, Lopez-Pulin, Sayah-Jeanne, de Gunzburg, Saint-Lu, Gonzalez-Zorn, Andremont and Bousquet-Mélou. This is an open-access article distributed under the terms of the Creative Commons Attribution License (CC BY). The use, distribution or reproduction in other forums is permitted, provided the original author(s) and the copyright owner(s) are credited and that the original publication in this journal is cited, in accordance with accepted academic practice. No use, distribution or reproduction is permitted which does not comply with these terms.
*Correspondence: Véronique Dupouy, di5kdXBvdXlAZW52dC5mcg==