- 1Department of Emergency and Critical Care, Auburn University Veterinary Teaching Hospital, Auburn, AL, United States
- 2Department of Pathobiology, Auburn University Veterinary Teaching Hospital, Auburn, AL, United States
- 3Department of Dermatology, Auburn University Veterinary Teaching Hospital, Auburn, AL, United States
Introduction: Hyperbaric oxygen therapy (HBOT) involves breathing 100% oxygen in a specialized compression chamber leading to hyperoxia. This treatment modality is associated with anti-inflammatory, antioxidant, and healing properties in people and laboratory animals. However, there are relatively few reports that evaluate the effects of HBOT in companion animals. The goal of this study was to investigate the physiological effects of HBOT on surgically induced systemic inflammation and oxidation in dogs.
Material and Methods: Twelve healthy female beagle dogs were spayed and randomized into control and HBOT groups (n = 6). Both groups received conventional post-ovariohysterectomy therapy, and the HBOT group received two hyperbaric treatments at 2.0 atmosphere of absolute pressure and 100% oxygen for 35 min, 6 and 18 h after surgery. Blood samples were collected 3 h prior to ovariohysterectomy, 6, 18, and 30 h after surgery, prior to HBOT when applicable. Inflammatory biomarkers, including C-reactive protein, circulating cytokines, and changes in iron homeostasis were evaluated at each time point to determine the effects of surgery and HBOT on inflammation. Similarly, serum total oxidant status and total antioxidant status were measured to assess the oxidative stress. Pain and incision scores were recorded and compared between groups.
Results: Following ovariohysterectomy, all dogs had significantly increased serum concentrations of C-reactive protein, KC-like, IL-6, and increased unsaturated iron-binding capacity compared to their pre-surgical values (p < 0.02), while serum iron, total iron-binding capacity and transferrin saturation were significantly decreased after surgery (p < 0.02). There was no significant difference between the control group and the HBOT group for any of the variables. There were no overt adverse effects in the HBOT group.
Conclusion: This is the first prospective randomized controlled study to investigate the effects of HBOT on surgically induced systemic inflammation in dogs. While elective ovariohysterectomy resulted in mild inflammation, the described HBOT protocol portrayed no outward adverse effect and did not induce any detectable pro-inflammatory, anti-inflammatory, or antioxidant effects. Additional investigation is required to identify objective markers to quantify the response to HBOT and determine its role as an adjunctive therapy in dogs with more severe, complicated or chronic diseases.
Introduction
Hyperbaric oxygen therapy (HBOT) is a medical treatment modality gaining popularity in both human and veterinary medicine for its apparent acceleration of tissue healing (1–8). During treatment, the patient breathes 100% oxygen inside a pressurized chamber above normal, sea level, atmospheric pressure [1 atmosphere of absolute pressure (ATA)]. Under these conditions, based on ideal oxygen pressure laws and an oxygen solubility in blood of 0.0031 mL/dL of blood per mmHg of arterial oxygen tension, the partial pressure of alveolar oxygen greatly increases from 100 mmHg on room air at 1 ATA to 1,400 mmHg on 100% oxygen at 2 ATA leading to transient hyperoxia (9). Primary effects of HBOT are improved tissue oxygenation (9) and reduction of intravascular and tissue gas bubble in relation to Boyle's law (10). Secondary physiologic effects are the results of short-term sub-lethal oxidative stress and include compensatory antioxidant response (3, 11, 12), modulation of inflammation and immune function (4, 5, 13), increased antimicrobial activity (14, 15), angiogenesis (16), enhanced fibroblast activation (17), upregulation of growth factors (18), vasoconstriction, and reduction of vasogenic edema (19). Although rare, possible complications related to increased atmospheric pressure, increased partial pressure of alveolar oxygen and hyperoxia include pneumothorax and other forms of barotrauma, oxygen lung toxicity and seizures, respectively (20).
The current understanding of the physiology and mechanisms of action of HBOT results primarily from investigations in people and laboratory animal (21). Prospective randomized controlled studies investigating the HBOT-mediated cellular response to oxidative stress in companion animals are lacking.
The goal of this study was to investigate the physiological effects of HBOT on surgically induced systemic inflammation and oxidation in dogs by comparing the expression of inflammatory biomarkers, pro-inflammatory and anti-inflammatory cytokines, total oxidant status, total antioxidant status, and oxidative stress index before and after postoperative HBOT. We hypothesized that HBOT would be safe under the proposed conditions and that it has anti-inflammatory and antioxidant properties in dogs undergoing ovariohysterectomy.
Materials and Methods
Study Population
Twelve 11–12-month-old intact female purpose-bred beagle dogs were found to be healthy based on their medical history, physical examination, hematological, and biochemistry profiles, urine analysis, vaginal swab, ZnSO4/I2 fecal flotation and SNAP 4Dx® assay (IDEXX Laboratories, Westbrook, ME, USA). Exclusion criteria are listed in Table 1. All animals were housed in a facility accredited by the United States Department of Agriculture and the Association for Assessment and Accreditation of Laboratory Animal Care International for the duration of the study. The dogs were cared for in compliance with the Animal Welfare Acts and fed the same diet (Pro Plan Adult Chicken & Rice Formula, Nestle/Purina, St. Louis, MO, USA) based on their daily energy requirement. The study was approved by the University Institutional Animal Care and Use Committee.
Anesthesia, Surgery, and Post-operative Care
All dogs were pre-medicated with hydromorphone (0.1 mg/kg) and dexmedetomidine (200 mcg/m2) intra-muscularly, and a peripheral intravenous catheter was placed. Anesthesia was induced with midazolam (0.2 mg/kg) and ketamine (5 mg/kg) intravenously, and maintained with isoflurane in 100% oxygen. During anesthesia, the dogs received Lactated Ringer's Solution (Vetivex®, Dechra, Overland Park, KS, USA) at a constant rate (5 mL/kg/h) intravenously. Intraoperative monitoring consisted of electrocardiography, capnography, body temperature, pulse oximetry (SurgiVet Advisor® 3 parameter vital signs monitor, Smiths Medical Inc., Minneapolis, MI, USA) and Doppler blood pressure (Doppler ultrasound, Parks Medical Electronics Inc., Aloha, OR, USA).
Ovariohysterectomies (OVE) were performed via ventral midline laparotomy by a single board-certified surgeon using a standardized surgical protocol. The length of the abdominal incision was consistent at ~13 cm. Duration of surgery, estimated blood loss, doses and time of drugs administered were recorded. After surgery, the dexmedetomidine was antagonized with 0.5 μg/m2 atipamezole administered intramuscularly.
Mentation was monitored hourly and a physical examination was performed every 6 h for the first 30 h, and once daily for 13 additional days. Post-operative care included administration of hydromorphone (0.05 mg/kg) intravenously every 6 h for the first 18 h. After collection of the final blood samples, the dogs received carprofen (2.2 mg/kg) by mouth every 12 h for 4 days for analgesia. Incision sites were uncovered, and the sutures were removed 14 days after surgery.
Hyperbaric Oxygen Therapy
The dogs were randomly divided into a control group (control group) and HBOT group using a random integer set generator (www.random.org). The HBOT group received 2 hyperbaric treatments, 6 and 18 h after surgery (Figure 1). During hyperbaric treatments, the dogs were administered 100% oxygen at 2.0 ATA for 35 min in a purpose-built veterinary hyperbaric chamber (Hvm model 3200 veterinary hyperbaric chamber, Hyperbaric veterinary medicine®, Boca Raton, FL, USA), with a compression and decompression time of 10 min each. A veterinarian who completed an introductory course approved by the National Board of Diving and Hyperbaric Medical Technology (Hyperbaric medicine team training for animal applications, International ATMO Inc., San Antonio, TX, USA) supervised all hyperbaric treatments. Treatments were performed at least 4 h after the last administration of hydromorphone to decrease the risk for respiratory depression. Through the windows of the chamber, the dogs were monitored for inappropriate mentation, respiratory distress, seizures, signs of barotrauma (head shaking, discomfort, vocalization), and confinement anxiety. A full physical examination was performed immediately prior and after completion of the treatments.
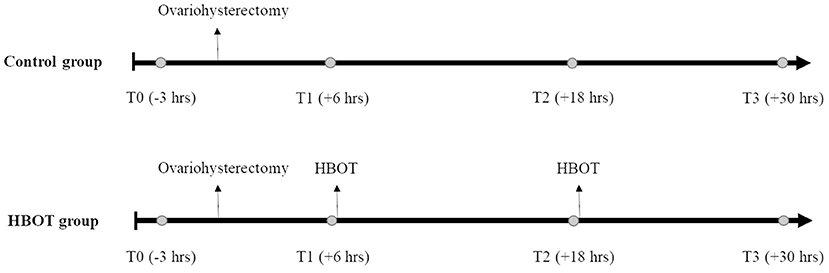
Figure 1. Experiment timeline. Whole blood (circle) is collected 3 h prior to ovariohysterectomy (T0), 6, 18, and 30 h after ovariohysterectomy (T1, T2, and T3, respectively), and prior to hyperbaric oxygen therapy (HBOT) for the HBOT group.
Clinical Outcomes
Post-operative wound healing and pain were evaluated by two non-blinded observers every 6 h for the first 30 h, and once daily for 13 additional days using the incision scoring system described by (22), and the pain scoring system developed by (23), respectively (22, 23).
Inflammatory and Oxidative Biomarkers
Five milliliters of whole blood were collected by direct venipuncture of a jugular vein 3 h prior to surgery (T0) and then 6 h (T1), 18 h (T2), and 30 h (T3) after surgery (Figure 1). In the HBOT group, samples were collected prior to the hyperbaric treatments. Blood was allowed to clot for 30 min and centrifuged at 3,000 rpm for 9 min. All serum samples were stored at −80°C until use. Inflammatory biomarkers and oxidative stress were measured at all time-points, in a blinded fashion and in duplicate.
Serum cytokines, chemokines and growth factors concentrations were measured using commercially available enzyme-linked immunosorbent assays and Luminex multiplex technology. Milliplex MAP Canine Cytokine/Chemokine Magnetic Bead Panels (EMD Millipore Corporation, Burlington, MA, USA) were used to measure the following cytokines: interleukin (IL)-2, IL-6, IL-7, IL-8, IL-10, IL-15, IL-18, interferon γ (IFN-γ), interferon-γ-induced protein (IP-10), tumor necrosis factor alpha (TNF-α), monocyte chemoattractant protein 1 (MCP-1), granulocyte-macrophage colony-stimulating factor (GM-CSF), and keratinocyte chemotactic-like (KC-like). The results were read with a Luminex MAP 96-well-microplate reader (Luminex Corporation, Austin, TX, USA) at 511 nm and the data were analyzed using xPONENT® (Luminex Corporation, Austin, TX, USA).
Serum samples were sent to the University of Miami Acute Phase Protein Laboratory for serum CRP levels measurements. Randox immunoturbidimetric Canine CRP assays (Randox, Kearneysville, WV, USA) were ran with a Daytona Rx analyzer (Randox, Kearneysville, WV, USA).
Serum iron (S. iron) and unsaturated iron-binding capacity (UIBC) were measured using the colorimetric Ferrozine method with the Roche Cobas 311 chemistry analyzer (Roche/Hitachi Ltd., Indianapolis, IN, USA). Total iron binding capacity (TIBC) and transferrin saturation (TSAT) were calculated by the following:
Serum total oxidant status (TOS) and total antioxidant status (TAS) levels were determined by the colorimetric methods described by Erel et al. in 2004 and 2005 using commercially available kits (Total oxidant status and total antioxidant status assay kits, Rel Assay Diagnostics, Gaziantep, Turkey) (24, 25). Oxidative stress index (OSI) was calculated by the following:
Icteric Index, Hemolytic Index, Lipemic Index, Progesterone Concentration
Icteric, hemolytic and lipemic indices were measured by spectrophotometry in all samples using a Roche Cobas 311 chemistry analyzer (Roche/Hitachi Ltd., Indianapolis, IN, USA). Serum progesterone levels were measured at T0 using an enzyme-labeled chemiluminescent technology and Immulite 1000 (Siemens Healthcare Diagnostics Inc., Tarrytown, NY, USA).
Statistical Analysis
All analyses were performed using a commercially available software SAS V 9.4 (SAS Institute Inc., Cary, NC, USA). Unpaired Student t-tests were used to identify differences in body weight and time of the surgical procedure between groups. Cytokine concentrations below the limit of detection were substituted with the limit of detection divided by the square root of 2 (26). For all inflammatory and oxidative stress biomarkers, outliers were identified prior to surgery at T0 using the median absolute deviation and a threshold of 3.5 (27). This allowed the identification of two dogs in the control group with pre-existing abnormal cytokine profile. No underlying cause was identified, and the dogs were excluded from the cytokine statistical analysis.
Model residuals were examined to evaluate the assumption of normality for all continuous data. Considering all serum concentrations were normally distributed, a linear mixed model was used to analyze the biomarker concentrations with inclusion of fixed factors such as group, time, and group by time interaction for each biomarker. Alternatively, to control for baseline differences and therefore possible differences in relative change, baseline biomarker concentration was added as a covariate. Lipemic index (at each time), hemolytic index (at each time) and progesterone (at first time) were initially included as fixed covariates for each biomarker, and backwards elimination allowed the identification of significant covariates. A random intercept for each dog was included in the mixed model to account for within dog repeated measures correlation over time. Satterthwaite degrees of freedom method allowed the comparison of means from two normal distributions with small samples and possibly different variances. All pairwise comparisons were corrected for multiple comparisons with Tukey's method. A p-value of <0.05 was defined as the significance cut-off. Results are presented as mean ± standard deviation.
Results
Study Population and Clinical Outcome
The dogs' mean body condition score was 4.6/9, with no statistical difference between the control and HBOT groups. The control dogs weighed significantly more than the dogs treated with HBOT with a body weight of 10.3 (±0.462) kg, and 8.98 (±1.01) kg, respectively. This difference was statistically significant (p = 0.017) with no influence detected on other variables. Nine dogs were in anestrus and 3 dogs were more than 30 days into diestrus by the time of surgery. Among the 3 dogs in diestrus, 1 control dog and 1 HBOT dog had elevated blood progesterone level at T0 (25 and 7.05 ng/mL, respectively). The range of surgical times was 27–40 min, with a median time of 30.5 min and no significant difference between groups. There were no surgical complications observed. All dogs in the HBOT group completed the hyperbaric oxygen sessions without any observed adverse effects. Physical examinations were unremarkable before and after hyperbaric treatments. No dog required rescue analgesia during hospitalization in any of the groups. Pain and incision scores did not differ at any time between the control and HBOT groups. All incisions were healed without complication 14 days post-surgery by the time of suture removal, and all dogs were determined clinically healthy 2 months after the study.
Significant covariates for each biomarker are shown in Table 2.
Acute Phase Protein and Iron Status
Mean and standard deviation for CRP, S. iron, UIBC, TIBC, and TSAT by time and group are presented in Figures 2, 3. Ovariohysterectomy induced a significant increase in CRP concentration at T2 and T3 (p < 0.01), and UIBC at T1 and T2 (p < 0.01) in both control and HBOT groups. Ovariohysterectomy induced a significant decrease in S. iron concentrations and TSAT at T1, T2, and T3 (p < 0.01), and TIBC at T2 and T3 (p = 0.01 and p < 0.01, respectively in the control group, and p = 0.04 and p < 0.01, respectively in the HBOT group). Total iron binding capacity was significantly increased at T3 compared to T1 in the HBOT group (p = 0.01) with no significant difference between control and HBOT groups. There was no effect of HBOT for any of the variables at any time point.
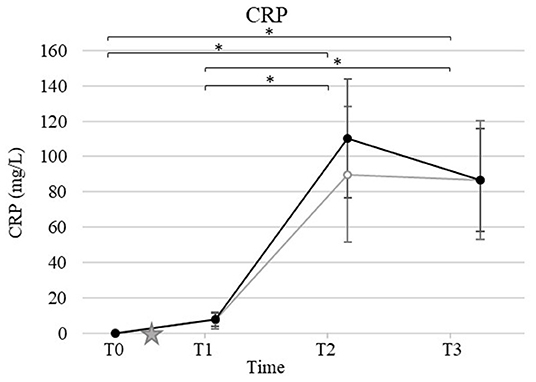
Figure 2. Mean and standard deviation of serum C-reactive protein (CRP) concentrations prior to ovariohysterectomy (T0), 6, 18, and 30 h after ovariohysterectomy (T1, T2, and T3, respectively) for the control group (gray line) and the hyperbaric oxygen therapy (HBOT) group (black line). The star indicates the time of surgery. *indicates a significant difference (p < 0.05) with time in both control and HBOT groups.
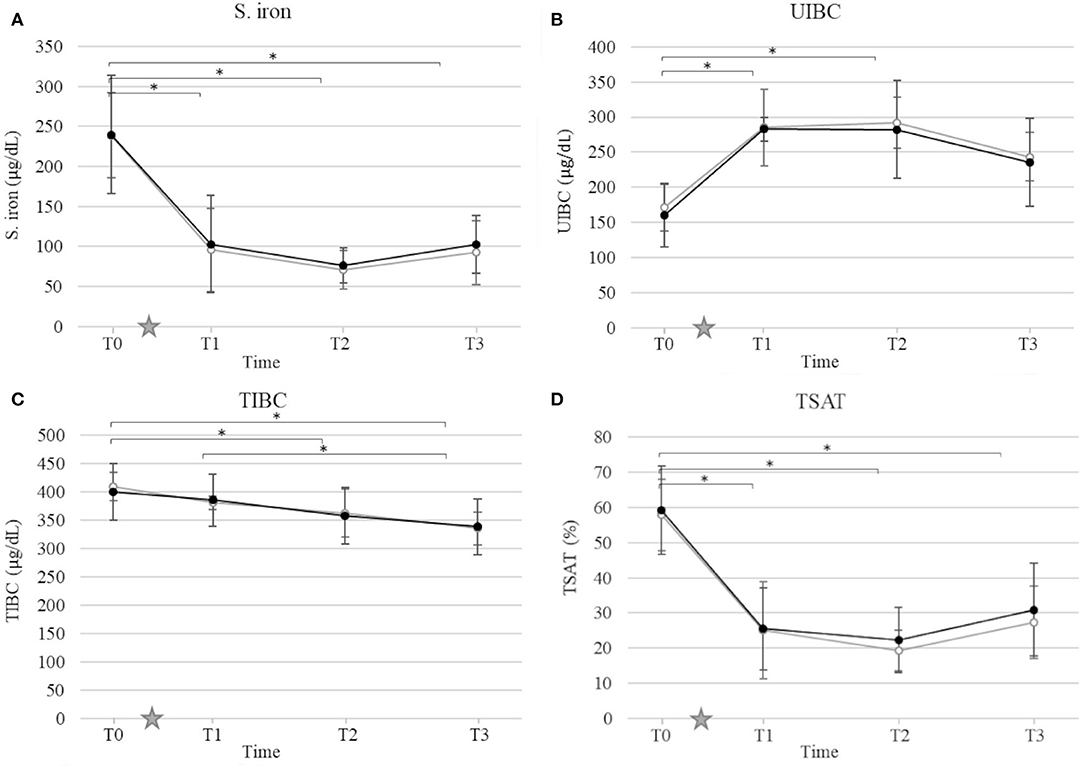
Figure 3. Mean and standard deviation of serum iron concentration (S. iron) (A), unsaturated iron-binding capacity (UIBC) (B), total iron-binding capacity (TIBC) (C), and transferrin saturation (TSAT) (D) prior to ovariohysterectomy (T0), 6, 18, and 30 h after ovariohysterectomy (T1, T2, and T3, respectively) for the control group (gray line) and the hyperbaric oxygen therapy (HBOT) group (black line). The star indicates the time of surgery. *indicates a significant difference (p < 0.05) with time in both control and HBOT groups.
Cytokines Profile
Ovariohysterectomy induced a ~4-fold increase in the serum concentration of KC-like in both control and HBOT groups at T1 (p < 0.01), and a ~2-fold increase in the IL-6 serum concentration in the HBOT group at T1 (p < 0.01) with no significant difference between control and HBOT groups (Figure 4). There was no significant effect of either OHE or HBOT on the remaining 11 cytokines.
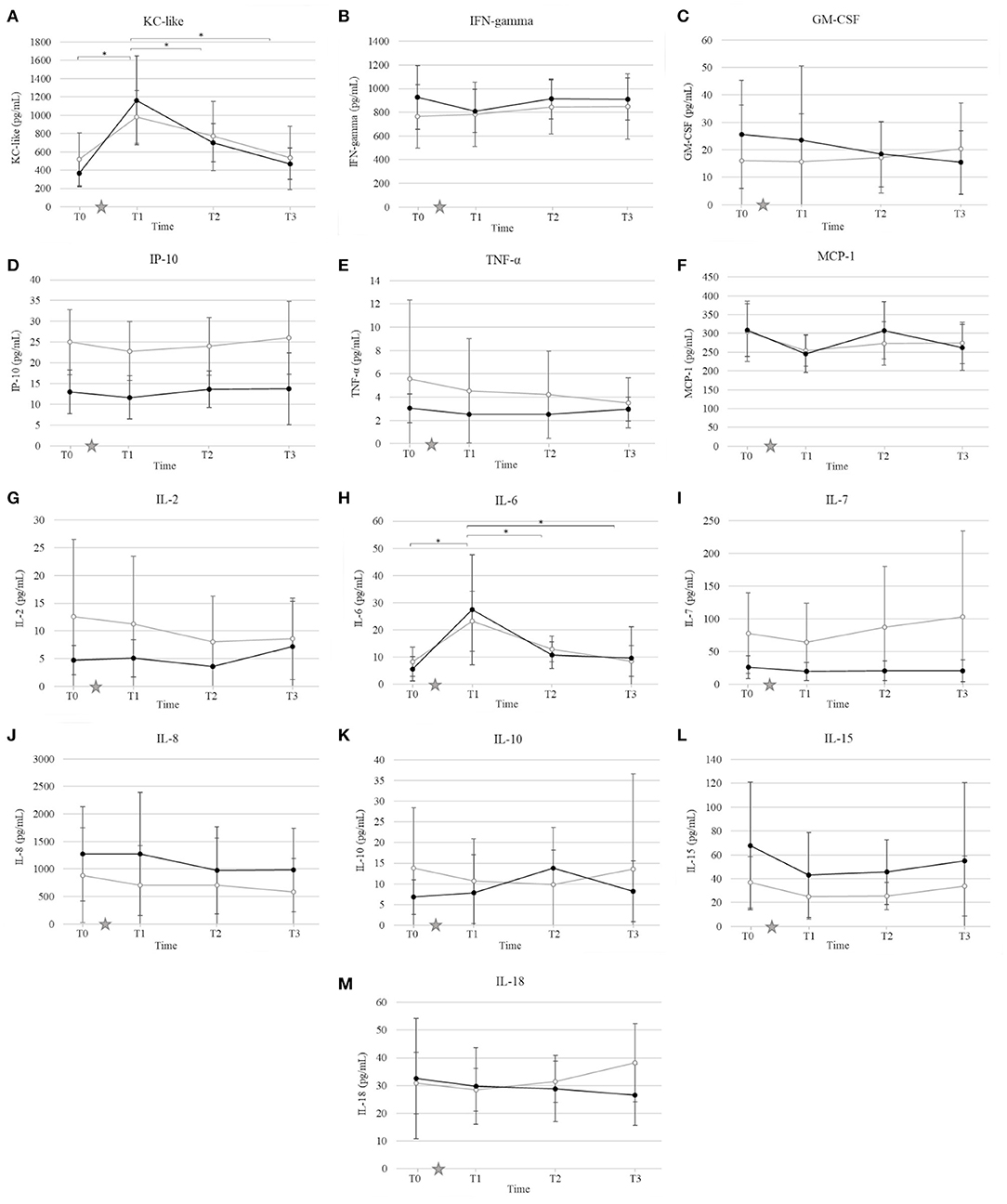
Figure 4. Mean and standard deviation of serum concentrations of keratinocyte chemotactic-like (KC-like) (A), interferon γ (IFN-γ) (B), granulocyte-macrophage colony-stimulating factor (GM-CSF) (C), interferon-γ-induced protein (IP-10) (D), tumor necrosis factor alpha (TNF-α) (E), monocyte chemoattractant protein 1 (MCP-1) (F), interleukin (IL) IL-2 (G), IL-6 (H), IL-7 (I), IL-8 (J), IL-10 (K), IL-15 (L), IL-18 (M) prior to ovariohysterectomy (T0), 6, 18, and 30 h after ovariohysterectomy (T1, T2, and T3, respectively) for the control group (gray line) and the hyperbaric oxygen therapy (HBOT) group (black line). The star indicates the time of surgery. *indicates a significant difference (p < 0.05) with time in both control and HBOT groups.
Oxidation Status
There was no significant effect of either OHE or HBOT on TOS, TAS and OSI (Figure 5).
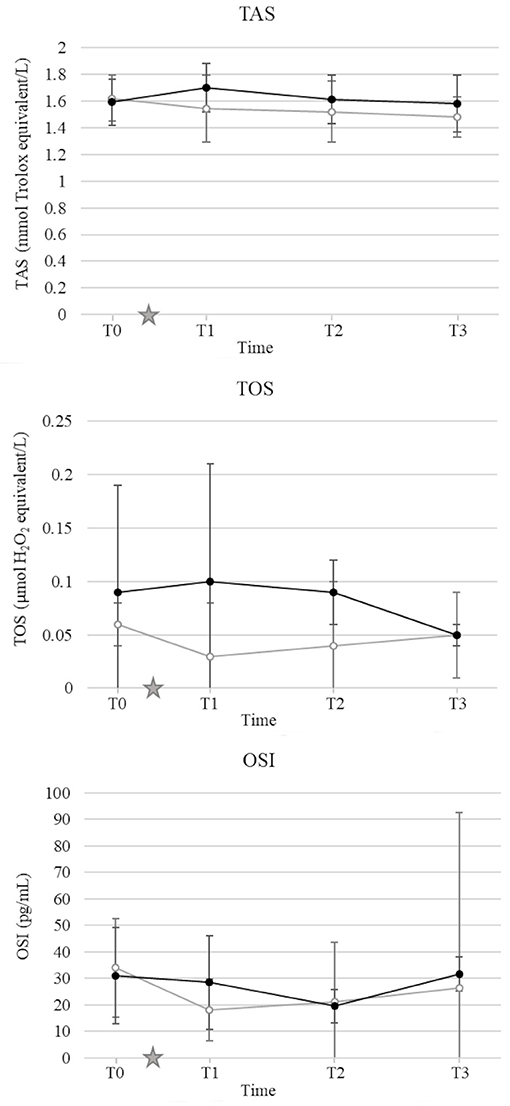
Figure 5. Mean and standard deviation of serum total antioxidant status (TAS) (A), total oxidant status (TOS) (B), oxidative stress index (OSI) (C) prior to ovariohysterectomy (T0), 6, 18, and 30 h after ovariohysterectomy (T1, T2, and T3, respectively) for the control group (gray line) and the hyperbaric oxygen therapy (HBOT) group (black line). The star indicates the time of surgery.
Discussion
Ovariohysterectomy induced a mild inflammatory response in dogs with no change detected in oxidative status at any sampling time. HBOT did not affect any of the variables. There was no difference detected between the control group and the HBOT group for any of the variables, suggesting no benefit nor harm of this HBOT protocol in dogs following OVE. HBOT appeared to be well-tolerated and portrayed no outward adverse effects at the dosage and duration used in the present study.
Since HBO is typically utilized as an adjunctive therapy in veterinary medicine, designing a study to evaluate its therapeutic effects with adequate power and minimal bias remains challenging. Ideally, HBOT would be the only treatment administered to a large population affected by a disease comparable in severity and measurable with specific and sensitive biomarkers, and comparable to a placebo. While these conditions are sometimes met with experimental laboratory animal models (28), they are difficult to achieve in a clinical setting. Additionally, most diseases that are reported to have potentially benefited from HBOT have varying degrees of severity with no disease specific biomarkers identified (i.e., pancreatitis, brain injury, sepsis, lung injury, wounds, and cardiopulmonary bypass surgery) (3–8, 13, 29, 30). For this study, an elective OHE performed by a skilled surgeon induced a mild inflammatory response as indicated by a short, but significant increase in CRP, UIBC, IL-6, and KC-like cytokine with a concurrent decrease in S. iron, TSAT, and TIBC in all dogs. Ovariohysterectomy is performed in a controlled environment with relatively comparable amounts of stress and tissue injury between patients, and limited medical intervention outside of analgesics. In order to limit the variability of inflammation induced in this study, a single surgeon performed the procedure using the same anesthetic and surgical protocols. Additionally, non-steroidal anti-inflammatory drugs were not initiated until after the last sampling time. Our results showed no statistical difference in biomarker concentrations, incision score, and pain score between groups prior to surgery, and prior to HBOT. Therefore, the clinical outcome, inflammation and oxidative stress induced by surgery were comparable immediately after OHE, and we speculated that differences between groups would be attributable to HBOT.
Interestingly, progesterone was identified as a significant covariate for IL-8, S. iron, TSAT, and TOS in this study. While it is known that the canine corpus luteum expresses various cytokines including IL-8 throughout diestrus (31), few data are available regarding the relationship between iron homeostasis, oxidative stress, and estrous phases in dogs. Studies with more dogs in different phases of the estrous cycle are warranted for further investigation. All dogs displayed a significant increase in CRP 18 h after surgery. These results are consistent with previous studies reporting an increase in CRP within the first 24 h after OHE with a subsequent reduction in the absence of ongoing trauma (32–36). HBOT has been shown, previously, to reduce CRP concentration in people with traumatic brain injury treated with 4 hyperbaric oxygen treatments at 3-day intervals (1 h on 100% oxygen at 2.0 ATA) (5), and in children with autism after 40 hyperbaric oxygen treatments at 1- to 2-day intervals (45 min on 100% oxygen at 1.3 and 1.5 ATA) (37). In our study, HBOT did not affect the concentration of CRP. This finding may indicate a lack of effect of HBOT in dogs at the lower dose used in this study, namely 2 treatments at 12-h interval for 35 min on 100% oxygen at 2.0 ATA. The small population and mild inflammation induced could also lead to a type II error, resulting in the absence of difference detected in CRP concentration between the control and HBOT groups. Further studies involving greater inflammatory stimuli and/or chronic inflammation (i.e., orthopedic surgery, pancreatitis, dermatitis), exposure to more and/or longer hyperbaric oxygen treatments, and measurements of CRP over a longer period are needed before determining the lack of effects of HBOT on inflammatory acute phase proteins in dogs.
Hypoferremia is a sensitive marker of systemic inflammation, including surgically induced inflammation (36, 38–41). Changes in iron homeostasis occur within the first 24 h after surgery, progressively normalize as inflammation subsides (36, 41), correlate with the extent of surgery (38), and serve as a valuable biomarker to monitor systemic inflammation and predict clinical outcome (39, 40). In this study, S. iron concentration and TSAT significantly decreased as early as 6 h after OHE, while TIBC decreased 18 to 32 h after surgery, and UIBC significantly increased 6 h after surgery in both the control and HBOT groups. Inflammation-related hypoferremia is multi-factorial. Upregulation of acute phase proteins such as ferritin and hepcidin leads to the sequestration of iron by the sarcoplasmic reticulum and the reduction of dietary iron absorption, resulting in increased UIBC (41–45). Simultaneously, the downregulation of the main iron binding transporter protein, transferrin, decreases TIBC (46). As the blood level of iron continues to decline, the amount of transferrin saturated with iron decreases, reflected by a lower TSAT.
To the author's knowledge, there are no published reports on the effects of HBOT on iron homeostasis. Iron and oxygen homeostasis are known to be strongly interconnected. Hypoxia is associated with decreased production of hepcidin and increased expression of transferrin. This results in an increase in dietary iron absorption and iron transport to erythroid tissues, ultimately leading to an increase in the capacity of red blood cells to transport oxygen (47). In the present study, the hyperoxemic state induced by HBOT did not have a detectable effect on iron homeostasis 32 h after surgery. Further studies are warranted to evaluate the effect of HBOT on the expression of hepcidin, transferrin and ferroportin in patients with systemic inflammation and/or ischemic injury.
Ovariohysterectomy induced a significant increase in KC-like and IL-6 serum concentration 6 h after surgery, with no significant change in the remaining cytokines and chemokines measured. Cytokines are key modulators of the inflammatory response to trauma and proper healing. Under physiologic conditions, a dynamic balance between pro- and anti-inflammatory cytokines serve as immunomodulatory elements to limit potential injury or excessive inflammatory reactions (48). Studies in human medicine yield insights into potential applications of cytokines in the diagnosis, monitoring, prognostication, and therapy of a variety of diseases (48), but much remains to be elucidated regarding cytokine regulation, synergistic or antagonistic interactions, and roles in either development, progression, or control of pathologic conditions in veterinary medicine. Previous studies report an increase in serum concentrations of IL-6 and IL-10 in healthy dogs 3 days after ovariohysterectomy (49) and identified KC-like as being significantly higher in dogs with pyometra and sepsis compared to dogs without sepsis (50). Additionally, IL-6 and KC-like have been shown to correlate with the severity of disease and surgical trauma (51, 52). The temporary and mild increase limited to IL-6 and KC-like identified in our study suggests that the inflammation induced by OHE was minimal. The absence of significant change between the control and HBOT groups suggests that HBOT does not induce alterations in pro- and anti-inflammatory cytokines in dogs after OHE. These findings are in contrast with previous studies that report an anti-inflammatory effect of HBOT in people and rodents treated for pancreatitis, traumatic brain injury, sepsis, and acute lung injury (4, 5, 13, 29, 53). In this study, the absence of detectable changes in cytokines levels after HBOT may be related to the nature of the surgery that induced a relatively minimal inflammatory response, and/or the inclusion of cytokines that are not specifically stimulated by OHE in dogs. Additionally, given the high inter-individual variability in the cytokine concentrations and response to stimulus, the small sample size may be a limitation to detect significant changes after OHE and HBOT. As previously mentioned, further studies evaluating the effects of HBOT on chronic and/or more severe inflammation are warranted in dogs to complement our investigation.
It is well-accepted that HBOT increases the production of reactive oxygen species at a sub-lethal concentration (12). At a low dose, via non-cytotoxic oxidative stimuli, increased reactive oxygen species levels activate a negative feedback loop, which leads to downregulation of oxidant enzymes and upregulation of antioxidant enzymes (54–57). One of the primary goals of this study was to determine the effects of HBOT on oxidative stress in dogs after OHE. For this purpose, TAS and TOS were measured to estimate the global antioxidant and oxidant status, respectively. To demonstrate the antioxidant effect of HBOT, most studies used individual antioxidant enzymes such as superoxide dismutase-1, catalase, and glutathione peroxidase, or oxidant enzymes such as malondialdehyde and F2-isoprostane (3–5, 58, 59). However, because the antioxidant and oxidant effects of each individual enzymes are additive, and their measurement can be technically challenging, none of these are ideal surrogate biomarker of oxidative stress (60). More recently, TAS and TOS have been suggested to assess the antioxidant and oxidant status of biological samples (24, 25, 61). In 2014, Lee et al. demonstrated an increase in plasma TOS and OSI levels and decrease in TAS levels after ovariectomy in dogs, suggesting the oxidative stress induced by the surgery (62). Total antioxidant and oxidant status were also used to demonstrate the antioxidant effects of HBOT in rats with induced acute lung injury after 5 daily treatments at 2.0 ATA for 1 h (53). In this study, OHE and HBOT did not induce any change in TOS, TAS, or OSI. These results may be related to the absence of oxidative stress induced by OHE performed by a board-certified surgeon and/or the lack of antioxidant effect of HBOT in dogs with no oxidative stress. A transient change in oxidation status after OHE or HBOT may be detected with collection of blood samples immediately after the procedures. The technique used to measure the total antioxidant and oxidant capacity may also be a source of error. To this point, the ideal reference method to measure TAS and TOS in dogs has not been established and further studies are needed to validate the technique used in this study (61). Finally, TAS and TOS estimate the antioxidant and oxidant capacity of the serum but provide limited information regarding the intracellular oxidative stress, and do not evaluate the role of important enzymes such as superoxide dismutase, glutathione peroxidase, and catalases (63, 64). Ideally, individual antioxidants and oxidants can be measured in complement to provide a wider picture of the antioxidant status.
A considerable number of studies suggests that short exposure to hyperoxia during HBOT can have therapeutic benefits. However, oxygen, particularly at supraphysiological pressures, also has the potential to increase systemic oxidative stress and induce hyperoxic cellular damage (65). Studies have shown that long-term exposure to HBOT (66, 67), and hyperbaric treatments at more than 3.0 ATA (29) results in cumulative oxidative stress and worsens clinical outcome in people and laboratory animals. This study identified a safe HBOT protocol for healthy dogs undergoing an elective surgical procedure.
In this study, HBOT did not improve wound healing or pain scores. The absence of effects on incision score after OHE is consistent with one study in which HBOT did not influence the healing of uncomplicated open and incisional wounds surgically created in dogs (68). There was no difference at any time for contraction, epithelialization, subjective wound scores, histopathology scores, or bacterial loads between the control group and the group that received HBOT (68). A Cochrane review including 12 randomized trials (577 human patients) concluded that HBOT is more likely to benefit ischemic wounds, or chronic wounds characterized by hypoxic tissues and associated with venous diseases or diabetes (69). Numerous studies have demonstrated an antinociceptive effect of HBOT for relief of chronic pain (70–72) and acute inflammatory pain in people (73, 74). The mechanism of the reported analgesic effect of HBOT is unknown, although some have attributed this effect to its anti-inflammatory properties and decreased expressions of inducible nitric oxide synthase and neuronal nitric oxide synthase (72, 73). In this study, there was no difference in pain scores or need for rescue analgesia between the control and HBOT groups. This is likely related to appropriate analgesia provided by the administration of opioids.
Conclusion
This study identified a well-tolerated HBOT protocol with no adverse effects detected in dogs following elective surgery with minimal inflammation. The absence of significant changes in circulating inflammatory markers suggests that there are no added benefits of this HBOT protocol in elective procedure such as OHE. However, these findings do not apply to more severe or chronic diseases processes. Additional investigation into various sources and types of inflammation is required to identify appropriate markers to quantify the response to HBOT, determine its role as an adjunctive therapy in dogs, and optimal dose. The present study, however, provides an important prospective study on the safety and efficacy of HBOT.
Data Availability Statement
All datasets generated for this study are included in the article/supplementary material.
Ethics Statement
The animal study was reviewed and approved by Auburn University Institutional Animal Care and Use Committee.
Author Contributions
AG, EG, LB, AW, LP, and KK contributed to the conception and design of the study. AG and KK supervised the hyperbaric oxygen treatments. AG, EG, and EF collected the data, contributed to the laboratory work, and analyzed the results. AG wrote the first draft of the manuscript. AG, EG, LB, EF, AW, LP, and KK contributed to the manuscript revision, read and approved the submitted version.
Funding
The present study was financially supported by Hyperbaric Veterinary Medicine®, Boca Raton, FL, USA. Fund/Org: 242485 - 139400. Grant: G00011243.
Conflict of Interest
AG and KK received a research grant from Hyperbaric Veterinary Medicine®, Boca Raton, FL, USA. The funder was not involved in the study design, collection, analysis, interpretation of data, the writing of this article or the decision to submit it for publication.
The remaining authors declare that the research was conducted in the absence of any commercial or financial relationships that could be construed as a potential conflict of interest.
Acknowledgments
We thank Dr. Michael Tillson (Auburn University) for performing the Ovariohysterectomies, and Dr. Rie Watanabe (Auburn University) for her assistance with the benchtop experiments.
Abbreviations
ATA, atmosphere of absolute pressure; CRP, C-reactive protein; GM-CSF, granulocyte-macrophage colony-stimulating factor; HBOT, hyperbaric oxygen therapy; IL, interleukin; IFN-γ, interferon γ; IP-10, interferon-γ-induced protein; KC-like, keratinocyte chemotactic-like; MCP-1, monocyte chemoattractant protein 1; OHE, ovariohysterectomy; OSI, oxidative stress index; S. iron, serum iron; TAS, total antioxidant status; TIBC, total iron binding capacity; TOS, total oxidant status; TNF-α, tumor necrosis factor α; TSAT, transferrin saturation; UIBC, unsaturated iron-binding capacity.
References
1. Bitterman H. Bench-to-bedside review: oxygen as a drug. Crit Care. (2009) 13:205–12. doi: 10.1186/cc7151
2. Edwards ML. Hyperbaric oxygen therapy. Part 1: history and principles. J Vet Emerg Crit Care. (2010) 20:284–8. doi: 10.1111/j.1476-4431.2010.00535.x
3. Sureda A, Batle JM, Martorell M, Capo X, Tjada S, Tur JA, et al. Antioxidant response of chronic wounds to hyperbaric oxygen therapy. PLoS ONE. (2016) 11:1–14. doi: 10.1371/journal.pone.0163371
4. Gao ZX, Rao J, Li YH. Hyperbaric oxygen preconditioning improves postoperative cognitive dysfunction by reducing oxidant stress and inflammation. Neural Regen Res. (2017) 12:329–36. doi: 10.4103/1673-5374.200816
5. Liu D, Yang H. Effects of hyperbaric oxygen on serum inflammatory factors, oxidative stress, endothelin and intracranial pressure in patients with severe head injury. J Hainan Med Univ. (2017) 23:83–6.
6. Alex J, Laden G, Cale ARJ, Bennett S, Flowers K, Madden L, et al. Pretreatment with hyperbaric oxygen and its effect on neuropsychometric dysfunction and systemic inflammatory response after cardiopulmonary bypass: a prospective randomized double-blind trial. J Thorac Cardiovasc Surg. (2005) 130:1623–30. doi: 10.1016/j.jtcvs.2005.08.018
7. Karadurmus N, Sahin M, Tasci C, Naharci I, Ozturk C, Ilbasmis S, et al. Potential benefits of hyperbaric oxygen therapy on atherosclerosis and glycaemic control in patients with diabetic foot. Polish J Endocrinol. (2010) 61:275–9.
8. Dundar TT, Seyithanoglu MH, Korpinar S, Atar R, Evran S. The effectiveness of hyperbaric oxygen therapy in an established intracerebral tumor model. Bezmialem Sci. (2015) 3:1–4. doi: 10.14235/bs.2015.449
10. Dennog C, Radermacher P, Barnett YA, Speit G. Antioxidant status in humans after exposure to hyperbaric oxygen. Mutat Res. (1999) 428:83–9. doi: 10.1016/S1383-5742(99)00034-4
11. van Hulst RA, Drenthen J, Haitsma JJ, Lameris TW, Visser GH, Klein J, et al. Effects of hyperbaric treatment in cerebral air embolism on intracranial pressure, brain oxygenation, and brain glucose metabolism in the pig. Crit Care Med. (2005) 33:841–6. doi: 10.1097/01.CCM.0000159529.26114.CA
12. Thom SRS. Oxidative stress is fundamental to hyperbaric oxygen therapy. J Appl Physiol. (2009) 106:988–95. doi: 10.1152/japplphysiol.91004.2008
13. Bai X, Song Z, Zhou Y, Pan S, Wang F, Guo Z, et al. The apoptosis of peripheral blood lymphocytes promoted by hyperbaric oxygen treatment contributes to attenuate the severity of early stage acute pancreatitis in rats. Apoptosis. (2014) 19:58–75. doi: 10.1007/s10495-013-0911-x
14. Luongo C, Imperatore F, Matera MG, Mangoni G, Marmo M, Baroni A, et al. Effect of hyperbaric oxygen therapy in experimental subcutaneous and pulmonary infections due to Pseudomonas aeruginosa. Undersea Hyperb Med. (1999) 26:21–5.
15. Mendel V, Reichert B, Simanowski HJ, Scholz HC. Therapy with hyperbaric oxygen and cefazolin for experimental osteomyelitis due to Staphylococcus aureus in rats. Undersea Hyperb Med. (1999) 26:169–74.
16. Muhonen A, Haaparanta M, Grönroos T, Bergman J, Knuuti J, Hinkka S, et al. Osteoblastic activity and neoangiogenesis in distracted bone of irradiated rabbit mandible with or without hyperbaric oxygen treatment. Int J Oral Maxillofac Surg. (2004) 33:173–8. doi: 10.1054/ijom.2003.0489
17. Kang TS, Gorti GK, Quan SY, Ho M, Koch RJ. Effect of hyperbaric oxygen on the growth factor profile of fibroblasts. Arch Facial Plast Surg. (2004) 6:31–5. doi: 10.1001/archfaci.6.1.31
18. Asano T, Kaneko E, Shinozaki S, Imai Y, Shibayama M, Chiba T, et al. Hyperbaric oxygen induces basic fibroblast growth factor and hepatocyte growth factor expression, and enhances blood perfusion and muscle regeneration in mouse ischemic hind limbs. Circ J. (2007) 71:405–11. doi: 10.1253/circj.71.405
19. Skyhar MJ, Hargens AR, Strauss MB, Gershuni DH, Hart GB, Akeson WH. Hyperbaric oxygen reduces edema and necrosis of skeletal muscle in compartment syndromes associated with hemorrhagic hypotension. J Bone Joint Surg Am. (1986) 68:1218–24. doi: 10.2106/00004623-198668080-00011
20. Heyboer M, Sharma D, Santiago W, McCulloch N. Hyperbaric oxygen therapy: side effects defined and quantified. Adv Wound Care. (2017) 6:210–24. doi: 10.1089/wound.2016.0718
21. Edwards ML. Hyperbaric oxygen therapy. Part 2: application in disease. J Vet Emerg Crit Care. (2010) 20:289–97. doi: 10.1111/j.1476-4431.2010.00535_1.x
22. Sylvestre A, Wilson J, Hare J. A comparison of 2 different suture patterns for skin closure of canine ovariohysterectomy. Can Vet J. (2002) 43:699–702.
23. Davidson EB, Moll HD, Payton ME. Comparison of laparoscopic ovariohysterectomy and ovariohysterectomy in dogs. Vet Surg. (2004) 33:62–9. doi: 10.1111/j.1532-950X.2004.04003.x
24. Erel O. A novel automated direct measurement method for total antioxidant capacity using a new generation, more stable ABTS radical cation. Clin Biochem. (2004) 37:277–85. doi: 10.1016/j.clinbiochem.2003.11.015
25. Erel O. A new automated colorimetric method for measuring total oxidant status. Clin Biochem. (2005) 38:1103–11. doi: 10.1016/j.clinbiochem.2005.08.008
26. Croghan C, Egeghy PP. Methods of Dealing With Values Below the Limit of Detection Using SAS. Abstract presented at: Southern SAS User Group, St. Petersburg, FL (2003).
27. Leys C, Ley C, Klein O, Bernard P, Licata L. Detecting outliers: do not use standard deviation around the mean, use absolute deviation around the median. J Exp Soc Psychol. (2013) 49:764–6. doi: 10.1016/j.jesp.2013.03.013
28. Nie H, Xiong L, Lao N, Chen S, Xu N, Zhu Z. Hyperbaric oxygen preconditioning induces tolerance against spinal cord ischemia by upregulation of antioxidant enzymes in rabbits. J Cereb Blood Flow Metab. (2006) 26:666–74. doi: 10.1038/sj.jcbfm.9600221
29. Buras J, Holt D, Orlow D, Belikoff B, Pavlides S, Reenstra WR. Hyperbaric oxygen protects from sepsis mortality via an interleukin-10-dependent mechanism. Crit Care Med. (2006) 34:2624–9. doi: 10.1097/01.CCM.0000239438.22758.E0
30. Meher S, Mishra TS, Sasmal PK, Rath S, Sharma R, Rout B, et al. Role of biomarkers in diagnosis and prognostic evaluation of acute pancreatitis. J biomarkers. (2015) 2015:519–34. doi: 10.1155/2015/519534
31. Engel E, Klein R, Baumgärtner W, Hoffmann B. Investigations on the expression of cytokines in the canine corpus luteum in relation to dioestrus. Ani Repro Sci. (2005) 87:163–76. doi: 10.1016/j.anireprosci.2004.10.002
32. Yamamoto S, Shida T, Miyaji S, Santsuka H, Fujise H, Mukawa K, et al. Changes in serum C-reactive protein levels in dogs with various disorders and surgical traumas. Vet Res Commun. (1993) 17:85–93. doi: 10.1007/BF01839236
33. Dabrowski R, Wawron W, Kostro K. Changes in CRP, SAA and haptoglobin produced in response to ovariohysterectomy in healthy bitches and those with pyometra. Theriogenology. (2007) 67:321–7. doi: 10.1016/j.theriogenology.2006.07.019
34. Dabrowski R, Wawron W. Acute-phase response in monitoring postoperative recovery in bitches after ovariohysterectomy. Ann Anim Sci. (2014) 14:287–95. doi: 10.2478/aoas-2014-0015
35. Liao PY, Chang SC, Chen KS, Wang HC. Decreased postoperative C-reactive protein production in dogs with pyometra through the use of low-dose ketamine. J Vet Emerg Crit Care. (2014) 24:286–90. doi: 10.1111/vec.12178
36. Moldal ER, Kjelgaard-Hansen MJ, Peeters ME, Nødtvedt A, Kirpensteijn J. C-reactive protein, glucose and iron concentrations are significantly altered in dogs undergoing open ovariohysterectomy or ovariectomy. Acta Vet Scand. (2018) 60:32–9. doi: 10.1186/s13028-018-0384-6
37. Rossignol DA, Rossignol LW, James SJ, Melnyk S, Mumper E. The effects of hyperbaric oxygen therapy on oxidative stress, inflammation, and symptoms in children with autism: an open-label pilot study. BMC Pediatr. (2007) 7:36–48. doi: 10.1186/1471-2431-7-36
38. van Iperen CE, Kraaijenhagen RJ, Biesma DH, Beguin Y, Marx JJ, van de Wiel A. Iron metabolism and erythropoiesis after surgery. Br J Surg. (1998) 85:41–5. doi: 10.1046/j.1365-2168.1998.00571.x
39. Jitpean S, Holst BS, Höglund OV, Pettersson A, Olsson U, Strage E, et al. Serum insulin-like growth factor-I, iron, C-reactive protein, and serum amyloid A for prediction of outcome in dogs with pyometra. Theriogenology. (2014) 82:43–8. doi: 10.1016/j.theriogenology.2014.02.014
40. Silvestrini P, Zoia A, Planellas M, Roura X, Pastor J, Cerón JJ, et al. Iron status and C-reactive protein in canine leishmaniasis. JSAP. (2014) 55:95–101. doi: 10.1111/jsap.12172
41. Torrente C, Manzanilla EG, Bosch L, Fresno L, Rivera Del Alamo M, Andaluz A, et al. Plasma iron, C-reactive protein, albumin, and plasma fibrinogen concentrations in dogs with systemic inflammatory response syndrome. J Vet Emerg Crit Care. (2015) 25:611–9. doi: 10.1111/vec.12340
42. Nemeth E, Valore EV, Territo M, Schiller G, Lichtenstein A, Ganz T. Hepcidin, a putative mediator of anemia of inflammation, is a type II acute-phase protein. Blood. (2003) 101:2461–3. doi: 10.1182/blood-2002-10-3235
43. Nemeth E, Rivera S, Gabayan V, Keller C, Taudorf S, Pedersen BK, et al. IL-6 mediates hypoferremia of inflammation by inducing the synthesis of the iron regulatory hormone hepcidin. J Clin Invest. (2004) 113:1271–6. doi: 10.1172/JCI200420945
44. Wessling-Resnick M. Iron homeostasis and the inflammatory response. Annu Rev Nutr. (2010) 30:105–22. doi: 10.1146/annurev.nutr.012809.104804
45. Kunireddy N, Jacob R, Khan SA, Yadagiri B, Sai Baba KSS, Rajendra Vara Prasad I, et al. Hepcidin and ferritin: important mediators in inflammation associated anemia in systemic lupus erythematosus patients. Indian J Clin Biochem. (2018) 33:406–13. doi: 10.1007/s12291-017-0702-1
46. Ceron JJ, Eckersall PD, Martinez-Subiela S. Acute phase proteins in dogs and cats: current knowledge and future perspectives. Vet Clin Pathol. (2005) 34:85–99. doi: 10.1111/j.1939-165X.2005.tb00019.x
47. Peyssonaux C, Nizet V, Johnson RS. Role of the hypoxia inducible factors in iron metabolism. Cell Cycle. (2008) 7:28–32. doi: 10.4161/cc.7.1.5145
48. Hsing CH, Wang JJ. Clinical implication of perioperative inflammatory cytokine alteration. Acta Anaesthesiol Taiwan. (2015) 53:23–8. doi: 10.1016/j.aat.2015.03.002
49. Dabrowski R, Pastor J, Szczubiał M, Piech T, Bochniarz M, Wawron W, et al. Serum IL-6 and IL-10 concentrations in bitches with pyometra undergoing ovariohysterectomy. Acta Vet Scand. (2015) 57:61–4. doi: 10.1186/s13028-015-0153-8
50. Karlsson I, Hagman R, Johannisson A, Wang L, Södersten F, Wernersson S. Multiplex cytokine analyses in dogs with pyometra suggest involvement of KC-like chemokine in canine bacterial sepsis. Vet Immunol Immunopathol. (2015) 170:41–6. doi: 10.1016/j.vetimm.2016.01.005
51. Watt DG, Horgan PG, McMillan DC. Routine clinical markers of the magnitude of the systemic inflammatory response after elective operation: a systematic review. Surgery. (2015) 157:362–80. doi: 10.1016/j.surg.2014.09.009
52. Galán A, Mayer I, Rafaj RB, Bendelja K, Sušić V, Cerón JJ, et al. MCP-1, KC-like and IL-8 as critical mediators of pathogenesis caused by Babesia canis. PLoS ONE. (2018) 13:1–21. doi: 10.1371/journal.pone.0190474
53. Akcilar R, Akcilar A, Simşek H, Koçak FE, Koçak C, Yümün G, et al. Hyperbaric oxygen treatment ameliorates lung injury in paraquat intoxicated rats. Int J Clin Exp Pathol. (2015) 8:13034–42.
54. Zhang Q, Gould LJ. Hyperbaric oxygen reduces matrix metallo-proteinases in ischemic wounds through a redox-dependent mechanism. J Invest Dermatol. (2014) 134:237–46. doi: 10.1038/jid.2013.301
55. Godman CA, Joshi R, Giardina C, Perdrizet G, Hightower LE. Hyperbaric oxygen treatment induces antioxidant gene expression. Ann N Y Acad Sci. (2010) 1197:178–83. doi: 10.1111/j.1749-6632.2009.05393.x
56. Matsunami T, Sato Y, Sato T, Ariga S, Shimomura T, Yukawa M. Oxidative stress and gene expression of antioxidant enzymes in the streptozotocin-induced diabetic rats under hyperbaric oxygen exposure. Int J Clin Exp Pathol. (2010) 3:177–88.
57. He X, Xu X, Fan M, Chen X, Sun X, Luo G, et al. Preconditioning with hyperbaric oxygen induces tolerance against renal ischemia-reperfusion injury via increased expression of heme oxygenase-1. J Surg Res. (2011) 170:e271–7. doi: 10.1016/j.jss.2011.06.008
58. Niu KC, Lin MT, Chang CP. Hyperbaric oxygen improves survival in heatstroke rats by reducing multiorgan dysfunction and brain oxidative stress. Eur J Pharmacol. (2007) 569:94–102. doi: 10.1016/j.ejphar.2007.04.037
59. Rockswold SB, Rockswold GL, Zaun DA, Liu J. A prospective, randomized Phase II clinical trial to evaluate the effect of combined hyperbaric and normobaric hyperoxia on cerebral metabolism, intracranial pressure, oxygen toxicity, and clinical outcome in severe traumatic brain injury. J Neurosurg. (2013) 118:1317–28. doi: 10.3171/2013.2.JNS121468
60. Marrocco I, Altieri F, Peluso I. Measurement and clinical significance of biomarkers of oxidative stress in humans. Oxid Med Cell Longev. (2017) 2017:6501046. doi: 10.1155/2017/6501046
61. Rubio CP, Hernández-Ruiz J, Martinez-Subiela S, Tvarijonaviciute A, Ceron JJ. Spectrophotometric assays for total antioxidant capacity (TAC) in dog serum: an update. BMC Vet Res. (2016) 12:166–72. doi: 10.1186/s12917-016-0792-7
62. Lee JY, Kim MC. Comparison of oxidative stress status in dogs undergoing laparoscopic and open ovariectomy. J Vet Med Sci. (2014) 76:273–6. doi: 10.1292/jvms.13-0062
63. Sies H. Total antioxidant capacity: appraisal of a concept. J Nutr. (2007) 137:1493–5. doi: 10.1093/jn/137.6.1493
64. Bartosz G. Non-enzymatic antioxidant capacity assays: limitations of use in biomedicine. Free Radic Res. (2010) 44:711–20. doi: 10.3109/10715761003758114
65. Jamieson D, Chance B, Cadenas E, Boveris A. The relation of free radical production to hyperoxia. Annu Rev Physiol. (1986) 48:703–19. doi: 10.1146/annurev.ph.48.030186.003415
66. Benedetti S, Lamorgese A, Piersantelli M, Pagliarani S, Benvenuti F, Canestrari F. Oxidative stress and antioxidant status in patients undergoing prolonged exposure to hyperbaric oxygen. Clin Biochem. (2004) 37:312–7. doi: 10.1016/j.clinbiochem.2003.12.001
67. Simsek K, Ay H, Topal T, Ozler M, Uysal B, Ucar E, et al. Long-term exposure to repetitive hyperbaric oxygen results in cumulative oxidative stress in rat lung tissue. Inhal Toxicol. (2011) 23:166–72. doi: 10.3109/08958378.2011.558528
68. Latimer C, Lux CN, Roberts S, Drum MG, Braswell C, Sula MJM. Effects of hyperbaric oxygen therapy on uncomplicated incisional and open wound healing in dogs. Vet Surg. (2018) 47:827–36. doi: 10.1111/vsu.12931
69. Kranke P, Bennett MH, Martyn-St James M, Schnabel A, Debus SE, Weibel S. Hyperbaric oxygen therapy for treating chronic wounds. Cochrane Database Syst Rev. (2015) 6:CD004123. doi: 10.1002/14651858.CD004123.pub4
70. Kiralp MZ, Yildiz S, Vural D, Keskin I, Ay H, Dursun H. Effectiveness of hyperbaric oxygen therapy in the treatment of complex regional pain syndrome. J Int Med Res. (2004) 32:258–62. doi: 10.1177/147323000403200304
71. Yildiz S, Uzun G, Kiralp MZ. Hyperbaric oxygen therapy in chronic pain management. Curr Pain Headache Rep. (2006) 10:95–100. doi: 10.1007/s11916-006-0019-x
72. Ding Y, Yao P, Hong T, Li H, Zhu Y, Han Z, et al. The analgesic effect of early hyperbaric oxygen treatment in chronic constriction injury rats and its influence on nNOS and iNOS expression and inflammatory factor production. Mol Pain. (2018) 14:1–11. doi: 10.1177/1744806918765837
73. Wilson JR, Foresman BH, Gamber RG, Wright T. Hyperbaric oxygen in the treatment of migraine with aura. Headache. (1998) 38:112–5. doi: 10.1046/j.1526-4610.1998.3802112.x
Keywords: canine, hyperbaric oxygen therapy, ovariohysterectomy, inflammation, cytokines, oxidation, iron
Citation: Gautier A, Graff EC, Bacek L, Fish EJ, White A, Palmer L and Kuo K (2020) Effects of Ovariohysterectomy and Hyperbaric Oxygen Therapy on Systemic Inflammation and Oxidation in Dogs. Front. Vet. Sci. 6:506. doi: 10.3389/fvets.2019.00506
Received: 20 October 2019; Accepted: 20 December 2019;
Published: 15 January 2020.
Edited by:
Peter James O'Brien, University College Dublin, IrelandReviewed by:
Michela Pugliese, University of Messina, ItalyAnnamaria Passantino, University of Messina, Italy
Copyright © 2020 Gautier, Graff, Bacek, Fish, White, Palmer and Kuo. This is an open-access article distributed under the terms of the Creative Commons Attribution License (CC BY). The use, distribution or reproduction in other forums is permitted, provided the original author(s) and the copyright owner(s) are credited and that the original publication in this journal is cited, in accordance with accepted academic practice. No use, distribution or reproduction is permitted which does not comply with these terms.
*Correspondence: Anais Gautier, YXpnMDA3NUBhdWJ1cm4uZWR1