- 1Key Laboratory of Animal Genetics, Breeding and Reproduction of Shaanxi Province, College of Animal Science and Technology, Northwest A&F University, Yangling, China
- 2College of Veterinary Medicine, Northwest A&F University, Yangling, China
- 3Shaanxi Provincial Engineering and Technology Research Center of Cashmere Goats, Yulin University, Yulin, China
- 4Life Science Research Center, Yulin University, Yulin, China
Growth differentiation factor 9 (GDF9) is a high-fertility candidate gene that plays a crucial role in early folliculogenesis in female mammals. In this study, direct sequencing was used to screen possible SNP loci in the goat GDF9 gene. Three SNP loci, p.proline27alanine (P27R), p.leucine61leucine (L61L), and p.alanine85glycine (A85G), were identified in Shaanbei white cashmere (SBWC) goats. Among the three SNPs, two rare missense SNP loci (P27R and A85G) were discovered to be strongly linked with each other (D′ value = 0.926, r2 value = 0.703). Both P27R and A85G loci had two genotypes: wild type and heterozygous type. A85G exerted a significant effect on litter size (P = 0.029) in SBWC goats, and the heterozygous genotype was superior in comparison with the wild type. The heterozygous genotype was also superior in P27R but no significant association was found. However, the combination genotypes of P27R and A85G were identified to have superior effects on litter size (P = 3.8E−15). This information suggested that these two SNPs influenced litter size in goats synergistically. Combining this information with our previous studies, we propose that the GDF9 gene is the principal high-fertility candidate gene and that the A85G locus is a promising SNP that affects litter size in goats. These results may fill a research gap regarding rare mutations as well as provide crucial molecular markers that could be useful in marker-assisted selection (MAS) goat rearing when selecting superior individuals.
Introduction
The growth differentiation factor 9 (GDF9) gene is a unique member of the transforming growth factor β (TGFβ) superfamily (1), as its protein has six Cys, being different from others in this superfamily that have seven or nine Cys (2, 3). Moreover, its greatest expression is in the ovary while it is widely expressed in 20 different tissues such as the hypothalamus, pituitary, and uterus (4, 5); this indicates that it affects different physiological pathways and metabolism, as well as phenotypic expression to some degree (6). Along with being a powerful intra-ovarian regulator during early folliculogenesis, the GDF9 gene is expressed throughout follicle development, and its mutations may contribute to increased ovulation rates or infertility in female mammals (7, 8).
Based on relevant study and data from the National Center for Biotechnology Information Search database (NCBI), 45 SNP loci have been identified in the goat GDF9 gene (9). Among these, 15 SNPs were identified to have significant associations with litter size in more than 30 goat breeds (10–12). Furthermore, there were some controversial and promising SNPs that had different impacts in different goat breeds (13–16). For instance, three missense mutations, A240V (17), Q320P (18–20), and V397I (21, 22), and three synonymous mutations, L61L (23), N121N (24), and L141L (25, 26), were found to have high mutant frequencies and be significantly associated with litter size in different breeds. However, due to breed-specific effects, several of the above results were not consistent. For instance, in Shaanbei white cashmere (SBWC), Lubei White, Jining Gray, Inner Mongolia cashmere, and Laiwu black goat breeds, the G allele is the major allele in V397I (22, 23, 26), but among the Xinong Saanen dairy goat, Big foot black goat, Guanzhong dairy goat, Jintang black goat, and Yimeng black goat, the A allele is associated with larger litter sizes (27, 28). However, almost all the abovementioned studies focused on a single SNP locus and neglected the fact that quantitative traits are controlled by multiple loci. Therefore, the gap in research regarding the combined effects of multiple loci needed to be addressed.
A combination of whole-genome sequencing and marker-assisted selection (MAS) could satisfy the demand to screen pivotal genes accurately and rapidly (29–31), as well as to assess relationships between their variations and growth and reproductive traits (32–35).
As a powerful high-fertility candidate gene, our group previously found that two strongly linked SNPs, Q320P and V397I, and a 12-bp indel within the GDF9 gene were significant associated with litter size in goats (20, 36). Furthermore, we summarized all reported SNPs within the GDF9 gene (9). Hence, based on our preliminary work, this study aimed to verify a greater number of SNP loci within the GDF9 gene as well as to analyze their relationships with goat litter size, thus providing more information for selecting a population with a high fertility using MAS method in goat rearing.
Materials and Methods
All experiments were approved by the International Animal Care and Use Committee of the Northwest A&F University (IACUC-NWAFU; protocol number NWAFAC1008) and followed local animal welfare guidelines, laws, and policies. The care and use of animals complied with local animal welfare laws and policies.
Sample Collection and DNA Isolation
For this study, 309 ear tissue samples were randomly collected from female SBWC goats (2–3 years) at a goat-breeding farm in Yulin City, Shaanxi Province, China. All selected goats had the same diet and rearing conditions after weaning (37, 38), were healthy, and had records for their first-born litter size and growth traits (e.g., body height, body length, heart girth, body weight, and cannon bone circumference index). Additionally, random selection ensured that individuals were as unrelated as possible (39, 40).
DNA was extracted from ear tissue samples, diluted to 50 ng/μl, and stored at −20°C according to Aljanabi's method (41). The DNA extraction protocol was as follows: 400 μl of buffer (0.4 M NaCl, 10 mM Tris–HCl at pH 8.0, and 2 mM EDTA at pH 8.0), 40 μl of 20% SDS (2% final concentration), and 8 μl of 20 mg/ml proteinase K (400 μg/ml final concentration) were added to the fresh tissue and mixed well. The samples were kept at a constant temperature of 65°C in a water bath shaker for 12–16 h, followed by the addition of 300 μl of 6 M NaCl (NaCl saturated H2O) and subsequent centrifugation for 30 min at 10,000 r/min. The supernatant was then transferred to fresh tubes. An equal volume of isopropanol was added to each sample, mixed well, and samples were incubated at 20°C for 1 h. Samples were then centrifuged for 20 min at 4°C and at 10,000 r/min. The pellet was washed with 70% ethanol, dried, and finally resuspended in 300–500 μl sterile dH2O.
Primer Design, PCR Amplification, and Genotyping
Based on the sequence of Capra hircus species (GenBank Accession No. NC_030814.1), a pair of primers (F: 5′-TTTGGTTTTGCTGCTTTGCCT-3′; R: 5′-TCTTTCTTCTTCCCTCCACCCA-3′), which covered P27R, L61L, A85G, L50P, G40G, N112N, and D129D loci, were designed to amplify exon 3 of the goat GDF9 gene using Primer Premier software (Version 6.0). The PCR was carried out in a 25-μl reaction condition containing 1.0 μl of genomic DNA, 0.5 μl of forward and reverse primer separately, 12.5 μl of 2 × MIX (Tsingke, Xi'an, China), and 10.5 μl of ddH2O. The PCR amplification protocol contained a pre-denaturation at 95°C for 5 min and denaturation at 94°C for 30 s, followed by 18 cycles of denaturation for 30 s at 95°C, annealing for 30 s at 68°C (with a decrease of 1°C per cycle), 30 cycles of elongation at 72°C for 30 s, and a final extension at 72°C for 10 min with subsequent cooling to 4°C (42, 43). Subsequently, PCR products were genotyped by electrophoresis using 2.0% agarose gel, which was stained with ethidium bromide. The PCR product was then directly sequenced by the Tsingke Biotechnology Company (Xi'an, China) using Sanger sequencing technology. Finally, sequence alignment was conducted using BioXM 2.6 (College of Agriculture, Nanjing Agricultural University, Nanjing, China) and Chromas 2.4.1 (Technelysium Pty Ltd, South Brisbane, Queensland, Australia).
Statistical Analyses
Genotype and allele frequencies, Hardy–Weinberg equilibrium (HWE), homozygosity (Ho), heterozygosity (He), effective allele numbers (Ne), and polymorphism information content (PIC) were calculated using PopGene version 1.3.1 (Molecular Biology and Biotechnology Center, University of Alberta, Edmonton, Canada) (44).
Linkage disequilibrium (LD) analysis was conducted on the SHEsis online platform (http://analysis.bio-x.cn) (45). The case of D′ = 1 or r2 = 1 indicated a complete LD. Values of D′ < 1; r2 > 0.33 indicated strong LD (46, 47).
The general linear models were established to analyze correlations between SNP loci and litter size and growth traits using R3.2.0 software. For litter size, model I: Yijlm = μ + Ki +HYSj + Gl + εijlm, where Yijlm is the litter size phenotypic value, μ is the mean of the overall population, Ki is the effect of kidding years, HYSj is the mean of population, Gl is the fixed effect of the genotype, and εijlm is the random error (36).
Considering that growth traits had a positive correlation with litter size (39), association between SNP loci and growth traits were analyzed. The association between SNPs and growth traits was considered. Model II: Yklm = μ2 + Ak + Gl + εklm, where Yklm is the observation of growth traits on each of the klmth animal, μ2 is the population mean, Ak is the fixed effect of age of the kth animal, Gl is the fixed effect of genotypes of the lth animal, and εklm is the random error.
t-test and the analysis of variance (ANOVA) were conducted to analyze the association between SNP loci and quantitative traits. Moreover, the t-test directed to two group analyses and ANOVA were available for >2 group analyses.
Function Prediction of P27R and A85G Within the Goat GDF9 Gene
As P27R and A85G are missense SNP loci, they may contribute to amino acid type change during encoding of the goat GDF9 gene. Herein, potential effects of SNP loci on protein structures and functions were predicted using three prediction tools, SIFT, PolyPhen-2, and PROVEAN (48–50).
Results
Genotyping of SNP Loci Within the Goat GDF9 Gene
A total of three SNP loci (Table 1; Figure 1) in exon 3 of the GDF9 gene were detected in this study. Based on sequence chromatograms, three SNP loci (P27R, L61L, and A85G) were genotyped in the analyzed SBWC goat population. For both P27R—-where TGC (proline) transformed to TGG (alanine)—-and L61L loci, two genotypes, CC and CG, were identified. For the A85G locus where CCT (alanine) transformed to CCG (glycine), three genotypes (CC, CA, and AA) were verified.
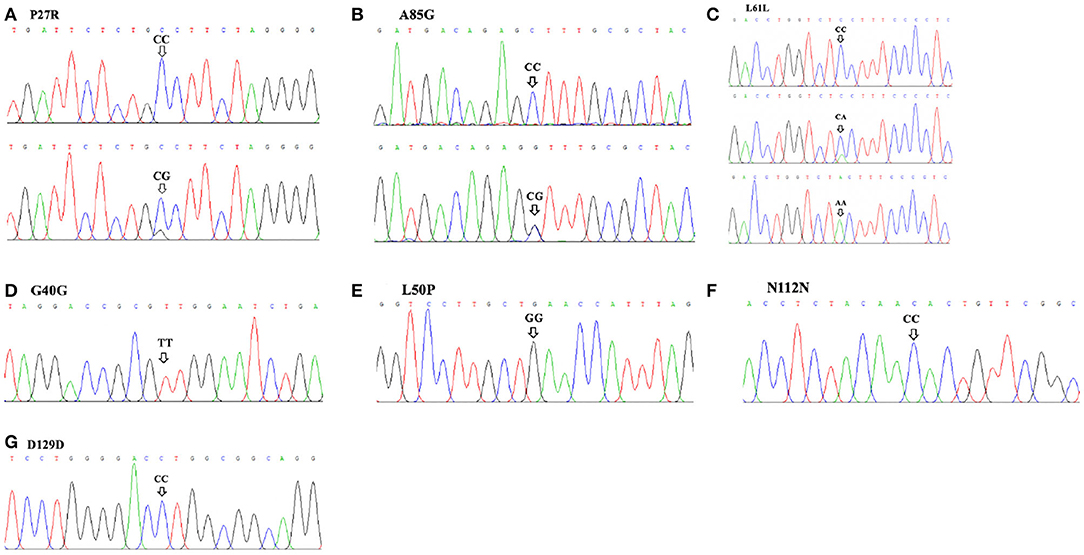
Figure 1. Sequence chromatograms of seven SNPs in the goat GDF9 gene run on Chromas. (A) P27R; (B) A85G; (C) L61L; (D) G40G; (E) L50P; (F) N112N; (G) D129D.
Genotypic and Allelic Frequencies of SNP Loci of the GDF9 Gene
Based on PIC values, P27R and A85G displayed low genetic diversity and PIC values were 0.047 and 0.019, respectively (Table 2). The PIC value of L61L was 0.355, demonstrating a medium genetic diversity. Additionally, CC and CG genotypes were identified in the P27R locus, and frequencies of C and G alleles were 0.976 and 0.024, respectively. For the L61L locus, three genotypes (CC, CA, and AA) were identified, and frequencies of C and A alleles were 0.639 and 0.361, respectively. For the A85G locus, in which CC and CG genotypes were detected, the frequency of C and G alleles were 0.990 and 0.010, respectively. Furthermore, both P27R and A85G loci met the HWE principle, whereas L61L did not (Table 2).
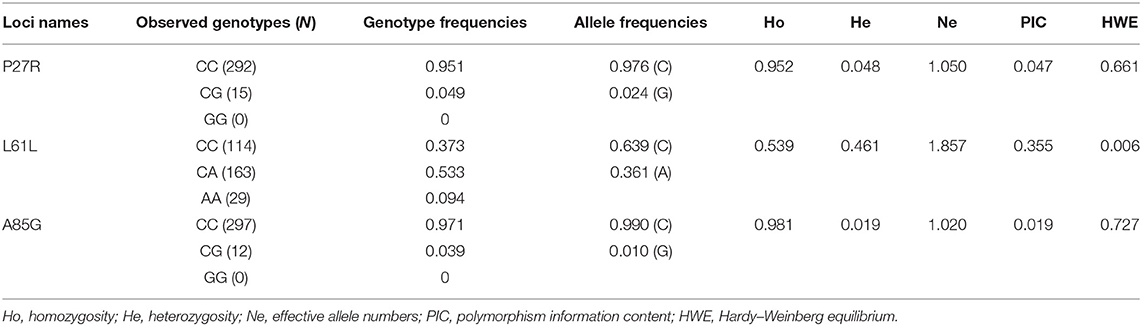
Table 2. Genotypic and allelic frequencies of seven SNPs within the GDF9 gene in Shaanbei white cashmere (SBWC) goats.
Linkage Disequilibrium Analyses
Based on LD analysis results (Table 3; Figure 2), the P27R and A85G loci were discovered to be strongly linked; the D′ and r2 values were 0.926 and 0.703, respectively. For L61L with P27R locus, and L61L with A85G locus, D′ values were 0.985 and 0.973, respectively, and r2 values were 0.016 and 0.012, respectively.
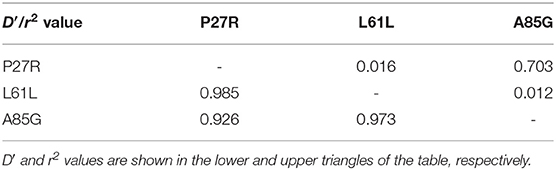
Table 3. Linkage disequilibrium parameters (D′ and r2) among P27R, L61L, and A85G loci of the GDF9 gene in Shaanbei white cashmere (SBWC) goats.
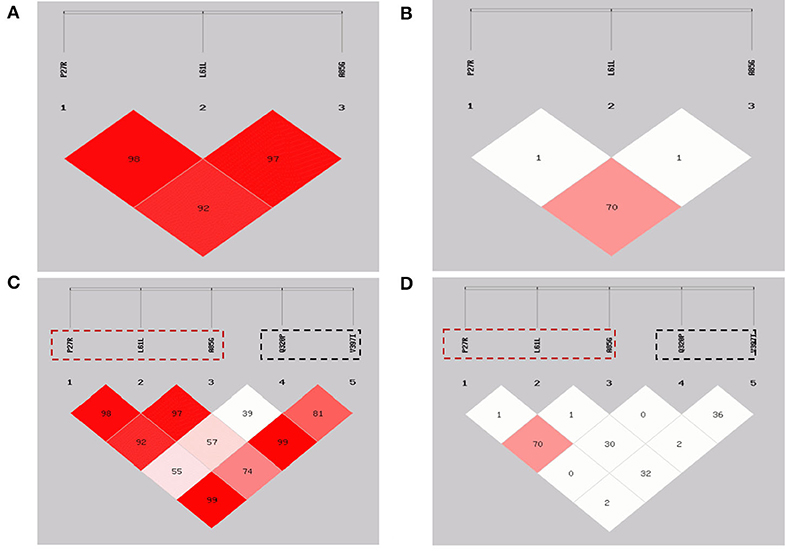
Figure 2. Linkage disequilibrium plots of the GDF9 gene in Shaanbei white cashmere (SBWC) goats. (A,C): D′ of SBWC goats; (B,D): r2 of SBWC goats. The three loci in the red dotted box were detected in this study and the two loci in the black dotted box were detected in our previous study.
Furthermore, in our previous study, two strongly linked SNPs, Q320P and V397I, were identified to be significantly associated with litter size, using the same population as those in this study (20). Combining our previous data regarding two missense SNPs (Q320P and V397I) within the goat GDF9 gene (20), the LD analysis of P27R, L61L, A85G, Q320P, and V397I (Table 4; Figure 2) revealed that neither P27R nor A85G was strongly linked to Q320P or V397I; our previous study verified that these were strongly linked, and significantly affected litter size. Interestingly, L61L was almost found to be strongly linked to both the Q320P and V397I loci. For L61L with Q320P, and L61L with V397I, D′ values were 0.573 and 0.741, respectively, and r2 values were 0.306 and 0.324, respectively.
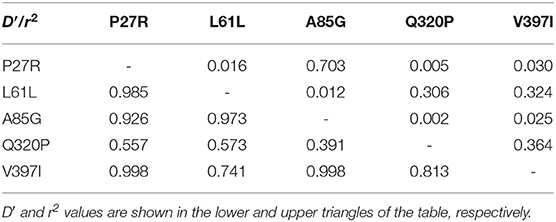
Table 4. Linkage disequilibrium parameters (D′ and r2) among P27R, L61L, A85G, Q320P, and V397I loci of the GDF9 gene in Shaanbei white cashmere (SBWC) goats.
Association Analyses Between SNP Loci and Litter Size
The association analysis between SNP loci and litter size (Table 5) illustrated that the A85G locus was significantly associated with litter size (P = 0.029), with the heterozygosity genotype displaying a superior phenotype over the homozygosity genotype. However, the P27R locus did not exert a remarkable effect on litter size. In combination genotype analysis of the P27R and A85G loci (Table 6), combination genotypes were significantly correlated to litter size (P = 3.8E−15) and the CG-CG produced the largest litter size. Furthermore, based on our previous data of Q320P and V397I (20), we did a combination genotype analysis, which showed that combination genotypes of P27R, A85G, Q320P, and V397I also exerted significant impacts on litter size (P = 0.001) with the CC-CC-CC-AA being dominant.
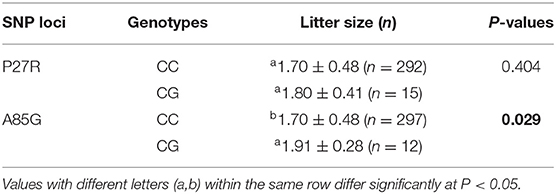
Table 5. Relationship between SNP loci of the GDF9 gene and litter size in Shaanbei white cashmere (SBWC) goats.
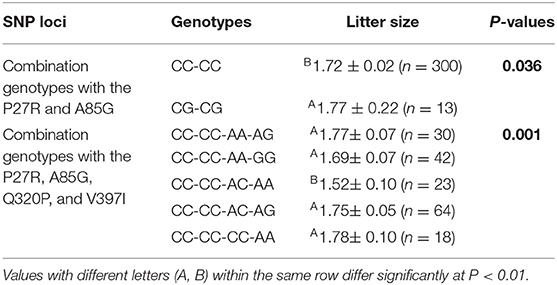
Table 6. Least squares mean and standard error for litter size of different combination genotypes of the GDF9 gene in Shaanbei white cashmere (SBWC) goats.
Association Analyses Between SNP Loci and Growth Traits
Previous studies by our group report a significant positive correlation between litter size and growth traits in our SBWC goat cohorts (20, 36); therefore, the relationship between these two missense SNPs (P27R and A85G) was addressed here. Association analyses between P27R and A85G loci as well as growth traits of SBWC goats revealed that the A85G locus was significantly related to body length (P = 0.001) and heart girth (P = 0.002). Furthermore, the heterozygosity genotype was superior when compared with the wild type (Table 7). No significance was found for P27R and growth traits. However, combination genotypes of P27R and A85G were proved to be significantly associated with hip width (P = 6.9E−8) and the cannon circumference index (P = 1.0E−6), with the phenotype of the homozygosity genotype showing a superior performance over the heterozygosity genotype (P = 6.9E−8; Table 8).
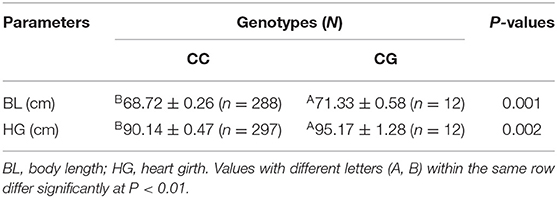
Table 7. Relationship between the A85G locus of the GDF9 gene and growth parameters in Shaanbei white cashmere (SBWC) goats.
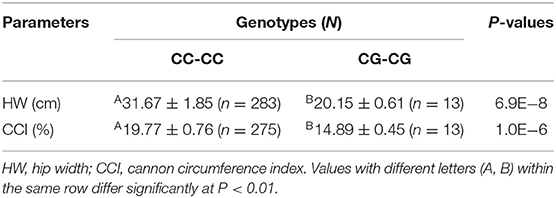
Table 8. Least squares mean and standard error for growth parameters of different combination genotypes of the SNPs P27R and A85G within the GDF9 gene in Shaanbei white cashmere (SBWC) goats.
Protein Function Prediction of SNP Loci
For protein function prediction of the P27R and A85G loci, the scores of SIFT, PolyPhen-2, and PROVEAN suggested that their influence on the GDF9 protein was benign and probably damaging, respectively. The scores implied that A85G had an impact on protein structure change of the goat GDF9 gene.
Discussion
The GDF9 gene plays a considerable role in the control of somatic cell functions such as follicular proliferation, ovulation, and fertilization, as well as enhancing oocyte development in females (51, 52). Therefore, study of the GDF9 gene and its mutations is worthwhile (53, 54). In the current study, three SNP loci (P27R, L61L, and A85G) were identified within the goat GDF9 gene. The L61L locus is reported to have a negative association with litter size in Jining Gray and Yimeng Black goat breeds (55). However, no significant association has been noted in Wendeng dairy, Liaoning cashmere, Beijing native, Boer, and Lubei goat breeds (23), which was consistent with the findings of this study. This may be due to a breed-specific effect or some degree of linkage between L61L and other SNP loci (22). Furthermore, two novel SNP loci, P27R and A85G, were detected to have low mutation frequencies and be strongly linked. The A85G locus was found to be significantly associated with litter size in SBWC goats while the P27R locus was not. However, combination genotypes of P27R and A85G showed significant association with litter size of SBWC goats with the heterozygosity genotype having a larger litter size. This could imply that these two SNP loci may exert a synergistic effect on litter size in goats. Considering that the SIFT, PolyOhen-2, and PROVEAN scores of P27R and A85G were benign and probably damaging, respectively, A85G might be responsible for changes in protein structure as well as DNA-binding ability. Additionally, this variation may alter GDF9 gene outcomes in terms of post-transcriptional regulation, such as RNA modification (56, 57), alternative splicing (58), and tRNA processing (56). For P27R, which is suggested to have no effect on changes in protein structure, it might be linked with other major polymorphisms exerting indirect effects (20).
In our previous studies, a 12-base pair indel (36) and two strongly linked SNP loci, Q320P and V397I, were significantly associated with litter size in goats (20). Q320P&V397I and P27R&A85G were regarded as block1 and block2, respectively. To investigate the relationship between block1 and block2, LD analyses were performed. Results illustrated that no strong linkage existed between the two blocks, but the combination genotype of the Q320P&V397I&P27R&A85G loci was significantly associated with litter size in goats, and it could be speculated that the two blocks affected litter size synchronously. Given the fact that quantitative traits are controlled by multiple loci, and only two strongly linked blocks have been uncovered to date (20), the identification of novel strongly linked loci, as well as their combined effects, would be promising for MAS breeding.
Already knowing that growth traits are positively correlated with reproductive traits (39), our study set out to determine whether the two strongly linked SNPs had a significant effect on growth traits. Association analyses showed that A85G was significantly associated with growth traits, but this was not true of P27R. However, combination genotype analyses further verified that combination genotypes of P27R and A85G exerted a significant effect on growth traits. These findings hinted that P27R and A85G might have a synergistic effect on growth performance. Therefore, both A85G and P27R could be considered as growth-related loci to some degree.
Conclusion
In conclusion, two novel rare missense SNPs were verified to be strongly linked in this study. Moreover, A85G was significantly associated with litter size, and P27R could have a simultaneous effect. The present study succeeded in establishing a clear and significant correlation between two SNPs (P27R and A85G) within the GDF9 gene with litter size in SBWC goat. Further studies are needed to identify sire effect before the commercial use of these SNPs in MAS.
Data Availability Statement
The datasets analyzed for this study can be found in the Ensembl database under accession numbers listed in Table 1 [http://www.ensembl.org/index.html].
Ethics Statement
The animal study was reviewed and approved by The International Animal Care and Use Committee of the Northwest A&F University (IACUC- NWAFU) (protocol number NWAFAC1008). Written informed consent was obtained from the owners for the participation of their animals in this study.
Author Contributions
YB, JL, and XW came with idea and wrote manuscript. LH, KL, XS, and XL collected the goat samples and isolated of genomic DNA. YB, JL, XW, and LH performed the experiments. YB, LQ, XS, XL, and CP analyzed the data. All authors approved the final version of the manuscript for submission. All authors contributed to the article and approved the submitted version.
Funding
This work was funded by the Shaanxi Provincial Scientific and Technological Innovation Project for Undergraduates of Northwest A&F University (S201910712052).
Conflict of Interest
The authors declare that the research was conducted in the absence of any commercial or financial relationships that could be construed as a potential conflict of interest.
Acknowledgments
Sincere thanks go to all the staff at the Shaanbei White Cashmere goat breeding farm, who made sample collection possible. We would also like to thank the Agricultural Technical Station of Veterinary and Animal Husbandry of Yulin City, Shaanxi Province, P.R. China and the Life Science Research Core Services (LSRCS) of Northwest A&F University (Northern Campus), for providing us with the platform.
Abbreviations
GDF9, growth differentiation factor 9; SNPs, single-nucleotide polymorphisms; PCR, polymerase chain reaction; SBWC, Shaanbei white cashmere goat; P27R, p.proline27alanine; L61L, p.leucine61leucine; A85G, p.alanine85glycine; Q320P, p.glutamine320proline; V397I, p.valine397isoleucine; A240V, p.alanine240valine; N121N, p.asparagine336asparagine; L141L, p.leucine141leucine; LD, linkage disequilibrium; HWE, Hardy–Weinberg equilibrium; Ho, homozygosity; He, heterozygosity; Ne, effective allele number; PIC, polymorphism information content; MAS, marker-assisted selection.
References
1. McPherron AC, Lee SJ. GDF-3 and GDF-9: two new members of the transforming growth factor-beta superfamily containing a novel pattern of cysteines. J Biol Chem. (1993) 268:3444–9.
2. Incerti B, Dong J, Borsani G, Matzuk MM. Structure of the mouse growth/differentiation factor 9 gene. Biochim Biophys Acta. (1994) 1222:125–8. doi: 10.1016/0167-4889(94)90034-5
3. Ahmad HI, Liu G, Jiang X, Edallew SG, Wassie T, Tesema B, et al. Maximum-likelihood approaches reveal signatures of positive selection in BMP15 and GDF9 genes modulating ovarian function in mammalian female fertility. Ecol Evol. (2017) 7:8895–902. doi: 10.1002/ece3.3336
4. Silva JR, van den Hurk R, van Tol HT, Roelen BA, Figueiredo JR. Expression of growth differentiation factor 9 (GDF9), bone morphogenetic protein 15 (BMP15), and bmp receptors in the ovaries of goats. Mol Reprod Dev. (2005) 70:11–9. doi: 10.1002/mrd.20127
5. Pramod RK, Sharma SK, Singhi A, Pan S, Mitra A. Differential ovarian morphometry and follicular expression of BMP15, GDF9 and BMPR1B influence the prolificacy in goat. Reprod Domest Anim. (2013) 48:803–9. doi: 10.1111/rda.12165
6. Pan ZY, Di R, Tang QQ, Jin HH, Chu MX, Huang DW, et al. Tissue-specific mRNA expression profiles of GDF9, BMP15, and BMPR1B genes in prolific and non-prolific goat breeds. Czech J Anim Sci. (2015) 60:452–8. doi: 10.17221/8525-CJAS
7. Simpson CM, Robertson DM, Al-Musawi SL, Heath DA, McNatty KP, Ritter LJ, et al. Aberrant GDF9 expression and activation are associated with common human ovarian disorders. J Clin Endocrinol Metab. (2014) 99:E615–24. doi: 10.1210/jc.2013-3949
8. Ahlawat S, Sharma R, Maitra A, Tantia MS. Current status of molecular genetics research of goat fecundity. Small Ruminant Res. (2015) 125:34–42. doi: 10.1016/j.smallrumres.2015.01.027
9. Wang XY, Yang Q, Zhang SH, Zhang XY, Pan CY, Chen H, et al. Genetic effects of single nucleotide polymorphisms in the goat GFD9 gene on prolificacy: true or false positive? Animals. (2019) 9:886. doi: 10.3390/ani9110886
10. Polley S, De S, Batabyal S, Kaushik R, Yadav P, Arora JS, et al. Polymorphism of fecundity genes (BMPR1B, BMP15 and GDF9) in the indian prolific black bengal goat. Small Rumin Res. (2009) 85:122–9. doi: 10.1016/j.smallrumres.2009.08.004
11. Ahlawat S, Sharma R, Roy M, Tantia MS, Prakash V. Association analysis of novel SNPs in BMPR1B, BMP15 and GDF9 genes with reproductive traits in black bengal goats. Small Rumin Res. (2015) 132:92–8. doi: 10.1016/j.smallrumres.2015.10.010
12. Ahlawat S, Sharma R, Roy M, Mandakmale S, Prakash V, Tantia MS. Genotyping of novel SNPs in BMPR1B, BMP15, and GDF9 genes for association with prolificacy in seven Indian goat breeds. Anim Biotechnol. (2016) 27:199–207. doi: 10.1080/10495398.2016.1167706
13. Shokrollahi B, Morammazi S. Polymorphism of GDF9 and BMPR1B genes and their association with litter size in markhoz goats. Repro Domest Anim. (2018) 53:971–8. doi: 10.1111/rda.13196
14. Hourad G, Sadegh FY, Jalal S, Abolfazl B. Identification of mutation in BMP15 and GDF9 gene association with prolificacy of markhoz goats. Arch Anim Breed. (2019) 62:565–70. doi: 10.5194/aab-62-565-2019
15. Peyman M, Amir R, Jalal R, Mohammad R. Association between c.1189G>A single nucleotide polymorphism of GDF9 and litter size in goats: a meta-analysis. Anim Reprod Sci. (2019) 209:106140. doi: 10.1016/j.anireprosci.2019.106140
16. Yue C, Bai WL, Zheng YY, Hui TY, Sun JM, Guo D, et al. Correlation analysis of candidate gene SNP for high- yield in liaoning cashmere goats with litter size and cashmere performance. Anim Biotechnol. (2019) 1–8. doi: 10.1080/10495398.2019.1652188
17. Wang YC, Zhang XH, Chu MX. Polymorphism of caprine GDF9 gene and their association with litter size in henan dairy goat. J Anim Vet Adv. (2013) 12:1590–6. doi: 10.3923/javaa.2013.1590.1596
18. Zhang HY, Ding XL, Ying SJ, Wang ZY, Pan XS, Wang RF, et al. SSCP analysis on exon2 of GDF9 gene in local of jiangsu province. Jiangsu Agric Sci. (2008) 5:51–3. doi: 10.3969/j.issn.1002-1302.2008.05.018
19. Feng T, Geng X, Lang XZ, Chu MX, Cao GL, Di R, et al. Polymorphisms of caprine GDF9 gene and their association with litter size in jining grey goats. Mol Biol Rep. (2011) 38:5189–97. doi: 10.1007/s11033-010-0669-y
20. Wang XY, Yang Q, Wang K, Yan HL, Pan CY, Chen H, et al. Two strongly linked single nucleotide polymorphisms (Q320P and V397I) in GDF9 gene are associated with litter size in cashmere goats. Theriogenology. (2019) 125:115–21. doi: 10.1016/j.theriogenology.2018.10.013
21. An XP, Hou JX, Zhao HB, Li G, Bai L, Peng JY, et al. Polymorphism identification in goat GNRH1 and GDF9 genes and their association analysis with litter size. Anim Genet. (2013) 44:234–8. doi: 10.1111/j.1365-2052.2012.02394.x
22. Zhao J, Liu SQ, Zhou XB, Zhuang R, Li Y, Wang XL, et al. A non-synonymous mutation in GDF9 is highly associated with litter size in cashmere goats. Anim Genet. (2016) 47:628–33. doi: 10.1111/age.12447
23. Dong CH, Du LX. Research on polymorphism analysis of GDF9 gene related to reproduction trait of goat. J Shandong Agric Univ. (2011) 42:227–37.
24. Tang JS, Zhang W, Zhu DJ, Hui WQ, Su SG, Chen S. Effects of GDF9 gene polymorphisms on serum levels of FSH and LH in goat ewes. Anim. Husb Vet Med. (2017) 49:13–8.
25. Wu ZH, Chu XM, Li XW, Fang L, Ye SC, Liu ZH, et al. PCR-SSCP analysis on exon 2 of growth differentiation factor 9 gene in goats. Sci Agric Sin. (2006) 39:802–8.
26. Wang BC, Zhang QK, Xu JJ, Chen M, Liu KD, Li Z, et al. Polymorphism detection of GDF9 gene exon 2 in three China goat breeds. China Herbiv Sci Collect Pap. (2014) 37:41–6.
27. Hanrahan JP, Gregan SM, Mulsant P, Mullen M, Davis GH, Powell R, et al. Mutations in the genes for oocyte-derived growth factors GDF9 and BMP15 are associated with both increased ovulation rate and sterility in Cambridge and belclare sheep (Ovis aries). Biol Reprod. (2004) 70:900–9. doi: 10.1095/biolreprod.103.023093
28. Zhu GQ, Wang QI, Kang YG, Lv YZ, Cao BY. Polymorphisms in GDF9 gene and its relationship with litter size in five breeds of black goats. Iran J Appl Anim Sci. (2013) 3:625−8. doi: 10.9774/GLEAF.978-1-909493-38-4_2
29. Chen MY, Wang J, Liu N, Cui WB, Dong WZ, Xing BS, et al. Pig SOX9: expression profiles of sertoli cell (SCs) and a functional 18bp indel affecting testis weight. Theriogenology. (2019) 138:94–101. doi: 10.1016/j.theriogenology.2019.07.008
30. Cui Y, Chen R, Lv XY, Pan CY. Detection of coding sequence, mRNA expression and three insertions/deletions (indels) of KDM6A gene in male pig. Theriogenology. (2019) 133:10–21. doi: 10.1016/j.theriogenology.2019.04.023
31. Kang ZH, Jiang EH, Wang K, Pan CY, Chen H, Yan HL, et al. Goat membrane associated ring-CH-type finger 1 (MARCH1) mRNA expression and association with litter size. Theriogenology. (2019) 128:8–16. doi: 10.1016/j.theriogenology.2019.01.014
32. Chen MY, Yan HL, Wang K, Cui Y, Chen R, Liu JW, et al. Goat SPEF2: expression profile, indel variations identification and association analysis with litter size. Theriogenology. (2019) 139:147–55. doi: 10.1016/j.theriogenology.2019.08.007
33. Kang ZH, Zhang SH, Zhu HJ, Wang Z, Yan HL, Huang YZ, et al. A 14-bp functional deletion within the CMTM2 gene is significantly associated with litter size in goat. Theriogenology. (2019) 139:49–57. doi: 10.1016/j.theriogenology.2019.07.026
34. Wang K, Kang ZH, Jiang EH, Yan HL, Qu Lei, Lan XY, et al. Genetic effects of DSCAML1 identified in genome-wide association study revealing strong associations with litter size and semen quality in goat (Capra hircus). Theriogenology. (2020) 146:20–5. doi: 10.1016/j.theriogenology.2020.01.079
35. Zhang YH, Cui WB, Yang H, Wang M, Yan HL, Zhu HJ, et al. A novel missense mutation (L280V) within POU1F1 gene strongly affects litter size and growth traits in goat. Theriogenology. (2019) 135:198–203. doi: 10.1016/j.theriogenology.2019.06.021
36. Wang XY, Yang Q, Wang K, Zhang SH, Pan CY, Chen H, et al. A novel 12-bp indel polymorphism within the GDF9 gene is significantly associated with litter size and growth traits in goats. Anim Genet. (2017) 48:735–6. doi: 10.1111/age.12617
37. Cui Y, Yan H, Wang K, Xu H, Zhang X, Zhu H, et al. Insertion/deletion within the KDM6A gene is significantly associated with litter size in goat. Front Genet. (2018) 9:91. doi: 10.3389/fgene.2018.00091
38. Zhang XL, Yan HL, Wang K, Zhou T, Chen MY, Zhu HJ, et al. Goat CTNNB1: mRNA expression profile of alternative splicing in testis and association analysis with litter size. Gene. (2018) 679:297–304. doi: 10.1016/j.gene.2018.08.061
39. Yang Q, Yan HL, Li J, Xu H, Wang K, Zhu H, et al. A novel 14-bp duplicated deletion within goat GHR gene is significantly associated with growth traits and litter size. Anim Genet. (2017) 48:499–500. doi: 10.1111/age.12551
40. Wang K, Yan HL, Xu H, Yang Q, Zhang SH, Pan CY, et al. A novel indel within goat casein alpha s1 gene is significantly associated with litter size. Gene. (2018) 671:161–9. doi: 10.1016/j.gene.2018.05.119
41. Aljanabi SM, Martinez I. Universal and rapid salt-extraction of high quality genomic DNA for PCR-based techniques. Nucleic Acids Res. (1997) 25:4692–3. doi: 10.1093/nar/25.22.4692
42. Yang Q, Zhang SH, Liu LL, Cao XK, Lei CZ, Qi XL, et al. Application of mathematical expectation (ME) strategy for detecting low frequency mutations: an example for evaluating 14-bp insertion/deletion (indel) within the bovine PRNP gene. Prion. (2016) 10:409–19. doi: 10.1080/19336896.2016.1211593
43. Li J, Erdenee S, Zhang SL, Wei ZY, Zhang M, Jin YY, et al. Genetic effects of PRNP gene insertion/deletion (indel) on phenotypic traits in sheep. Prion. (2018) 12:42–53. doi: 10.1080/19336896.2017.1405886
44. Chen F, Shi J, Luo YQ, Sun SY, Pu M. Genetic characterization of the gypsy moth from China (Lepidoptera, Lymantriidae) using inter simple sequence repeats markers. PLoS ONE. (2013) 8:873017. doi: 10.1371/journal.pone.0073017
45. Li Z, Zhang Z, He Z, Tang W, Li T, Zeng Z, et al. A partition-ligation-combination-subdivision EM algorithm for haplotype inference with multiallelic markers: update of the SHEsis. Cell Res. (2009) 19:519–23. doi: 10.1038/cr.2009.33
46. Reich DE, Cargill M, Bolk S, Ireland J, Sabeti PC, Richter DJ, et al. Linkage disequilibrium in the human genome. Nature. (2001) 411:199–204. doi: 10.1038/35075590
47. Ardlie KG, Kruglyak L, Seielstad M. Patterns of linkage disequilibrium in the human genome. Nat Rev Genet. (2002) 3:299–309. doi: 10.1038/nrg777
48. Ng PC, Henikoff S. Accounting for human polymorphisms predicted to affect protein function. Genome Res. (2002) 12:436–46. doi: 10.1101/gr.212802
49. Adzhubei IA, Schmidt S, Peshkin L, Ramensky VE, Gerasimova A, Bork P, et al. A method and server for predicting damaging missense mutations. Nat Methods. (2010) 7:248–9. doi: 10.1038/nmeth0410-248
50. Choi Y, Chan AP. Provean web server: a tool to predict the functional effect of amino acid substitutions and indels. Bioinformatics. (2015) 31:2745–7. doi: 10.1093/bioinformatics/btv195
51. Spicer LJ, Aad PY, Allen D, Mazerbourg S, Hsueh AJ. Growth differentiation factor-9 has divergent effects on proliferation and steroidogenesis of bovine granulosa cells. J Endocrinol. (2006) 189:329–39. doi: 10.1677/joe.1.06503
52. Otsuka F, McTavish KJ, Shimasaki S. Integral role of GDF-9 and BMP-15 in ovarian function. Mol Reprod Dev. (2011) 78:9–21. doi: 10.1002/mrd.21265
53. Yang CX, Zi XD, Wang Y, Yang DQ, Ma L, Lu JY, et al. Cloning and mRNA expression levels of GDF9, BMP15, and BMPR1B genes in prolific and non-prolific goat breeds. Mol Reprod Dev. (2012) 79:2. doi: 10.1002/mrd.21386
54. Dutta R, Das B, Laskar S, Kalita DJ, Borah P, Zaman G, et al. Polymorphism, sequencing and phylogenetic characterization of growth differentiation factor 9 (GDF9) gene in assam hill goat. Afr J Biotechnol. (2013) 12:6894–6900.
55. Chu MX, Wu ZH, Feng T, Cao G, Fang L, Di R, et al. Polymorphism of GDF9 gene and its association with litter size in goats. Vet Res Commun. (2011) 35:329–36. doi: 10.1007/s11259-011-9476-8
56. Oerum S, Roovers M, Leichsenring M, Acquaviva-Bourdain C, Beermann F, Gemperle-Britschgi C, et al. Novel patient missense mutations in the HSD17B10 gene affect dehydrogenase and mitochondrial tRNA modification functions of the encoded protein. Biochim Biophys Acta Mol Basis Dis. (2017) 1863:3294–302. doi: 10.1016/j.bbadis.2017.09.002
57. Shaheen R, Tasak M, Maddirevula S, Abdel-Salam GMH, Sayed ISMS, Alazami AM, et al. PUS7 mutations impair pseudouridylation in humans and cause intellectual disability and microcephaly. Hum Genet. (2019) 138:231–9. doi: 10.1007/s00439-019-01980-3
Keywords: goat, growth differentiation factor 9 (GDF9) gene, litter size, association, linkage disequilibrium
Citation: Bi Y, Li J, Wang X, He L, Lan K, Qu L, Lan X, Song X and Pan C (2020) Two Novel Rare Strongly Linked Missense SNPs (P27R and A85G) Within the GDF9 Gene Were Significantly Associated With Litter Size in Shaanbei White Cashmere (SBWC) Goats. Front. Vet. Sci. 7:406. doi: 10.3389/fvets.2020.00406
Received: 27 February 2020; Accepted: 08 June 2020;
Published: 30 July 2020.
Edited by:
Khalid El Allali, Agronomic and Veterinary Institute Hassan II, MoroccoReviewed by:
Mohammed Piro, Agronomic and Veterinary Institute Hassan II, MoroccoYong-Fu Huang, Southwest University, China
Copyright © 2020 Bi, Li, Wang, He, Lan, Qu, Lan, Song and Pan. This is an open-access article distributed under the terms of the Creative Commons Attribution License (CC BY). The use, distribution or reproduction in other forums is permitted, provided the original author(s) and the copyright owner(s) are credited and that the original publication in this journal is cited, in accordance with accepted academic practice. No use, distribution or reproduction is permitted which does not comply with these terms.
*Correspondence: Xianyong Lan, bGFueGlhbnlvbmc3OUAxMjYuY29t; Chuanying Pan, Y2h1YW55aW5ncGFuQDEyNi5jb20=
†These authors have contributed equally to this work