- 1Key Laboratory of Buffalo Genetics, Breeding and Reproduction Technology, Ministry of Agriculture and Guangxi Buffalo Research Institute, Chinese Academy of Agricultural Sciences, Nanning, China
- 2Institute of Animal and Dairy Sciences, University of Agriculture, Faisalabad, Pakistan
Hot and humid weather exposes animals to high temperature and relative humidity that ultimately reduce their ability to disperse body heat. To avoid serious consequences of heat stress, it is imperative to understand animal physiological responses and biochemical changes during a state of altered body homeostasis across different seasons of the year. This study evaluated seasonal dynamics of physiological, oxidative, and metabolic responses of Nili-Ravi buffaloes to hot and humid climate. Twenty non-lactating multiparous buffaloes were enrolled for this 1-year study. Meteorological data were recorded twice daily to calculate temperature humidity index (THI). Physiological parameters including rectal temperature (RT), body surface temperature (BST), and respiratory rate (RR) were measured weekly. Blood samples were collected once in each season (spring, summer, autumn, and winter) to analyze biochemical and antioxidant parameters. We also measured activities of liver enzymes including alanine aminotransferase (ALT) and aspartate aminotransferase (AST). The results revealed a significantly higher THI value (82) during summer which resulted in a significant increase in RR and BST as compared to winter. Higher oxidative stress was observed in summer owing to significantly higher malondialdehyde (MDA) content and lower levels of serum antioxidant enzymes (GPx, SOD, and CAT) as compared to other seasons. Moreover, serum cortisol was also significantly higher while adrenocorticotropic hormone (ACTH), Triiodothyronine (T3), insulin, and growth hormone contents were significantly lower in summer. Contrarily, plasma thyroxin (T4) level was higher in summer. THI showed a positive correlation with physiological responses but a negative correlation with antioxidant parameters. Our study provides practical insights on the adaptive physiology of buffaloes and has several implications regarding the alleviation of heat stress in buffaloes to enhance the efficiency of production and reproduction under tropical climate. Our study suggests the use of appropriate cooling strategies to effectively manage the non-lactating buffaloes to avoid performance losses and animal welfare issues in summer season.
Introduction
Climate change imposes adverse effects on animal physiology, leading to an overall decrease in efficiency of production and reproduction. Moreover, it also raises animal health and welfare concerns, sometimes with serious consequences. Heat stress is one of the major climatic effects faced by animals, especially in tropical and sub-tropical countries. Heat stress in the tropics is generally associated with animal welfare issues and significant economic losses resulting from reduced performance but increased morbidity and mortality of livestock (1). The impact of heat stress is expected to become worse in the recent climate change scenario as it will increase the potential intensity of hot and humid conditions in the future, leading to an increased frequency of heat stress episodes (2). At higher ambient temperatures (above 30°C), excessive heat load hampers the ability of animals to dissipate their body heat. Heat stress occurs usually when animals are unable to maintain the balance between heat produced/stored and heat dissipated (3, 4). To manage this excessive heat load, animals attempt to reduce metabolic heat production while increasing heat dissipation to maintain euthermia. This is accompanied by a series of physiological, metabolic, and behavioral manifestations to thrive and mitigate adverse effects of heat stress. The first line of response to excessive heat load mainly includes accelerated respiratory rate (RR), increased water intake but reduced feed intake (5). Physiological parameters like body surface temperature (BST), rectal temperature (RT), respiratory rate, and pulse rate (PR) are the quick, ultimate responses of animals to climatic stress and eventually the level of discomfort or comfort of animals in a given environment (6).
Heat stress exposes dairy animals, especially buffaloes, to an excessive load of reactive oxygen species (ROS), leading to severe oxidative stress that subsequently reduces metabolic activity and immune response (7). Antioxidant system of animals fails to scavenge a large number of free radicals in time due to reduced activities of antioxidant enzymes (CAT, SOD, and GPx) under chronic heat stress (8). Therefore, levels of oxidative and antioxidant enzymes in ruminants can be used as markers to assess the degree of oxidative stress (9). Exposure to high ambient temperature also adversely affects the activity of the thyroid gland owing to its high sensitivity to environmental heat variations, subsequently leading to reduced levels of T3 and T4 (10). This decline in thyroid activity is coupled with a decrease in growth hormone to reduce metabolic activity and body heat increment. Moreover, stimulation of the hypothalamus pituitary–adrenal axis leads to the secretion of adrenocorticotropic hormone (ACTH) from the pituitary gland and then triggers the synthesis and secretion of glucocorticoids like cortisol (11). Hence, serum cortisol has been used extensively as a biomarker of heat stress in cattle (12, 13) and buffalo (14, 15).
These physiological manifestations in response to heat stress, significantly affect biological rhythm (diurnal and seasonal) in dairy animals. Compared to Zebu cattle, these effects are more pronounced in buffaloes owing to their dark skin color and lack of sweat glands. Heat stress triggers many physiological changes in the body of buffaloes, including a decrease in feed intake, shift in hormonal and metabolic secretions but an increase in BST, RT, RR, and oxidative stress (16, 17). Thermal comfort is generally measured by temperature-humidity index (THI) and has been applied to different livestock species, to understand the physiological stages of thermo-neutral and heat stress zones. It is imperative to better understand the changes in physiological and metabolic rhythms in buffaloes during different seasons, to devise strategies to mitigate the adverse effects of heat stress so that to avoid economic losses and animal welfare issues. Moreover, the association of THI with various physiological and biochemical parameters in buffaloes is a precondition to predict environmental effects during different seasons of the year. It is pertinent to mention that tropical climate of Southern China is quite different from other South Asian countries due to quite higher humidity which substantially increases average THI. Moreover, to best of our knowledge, no study is available regarding the seasonal variations in the oxidative physiology of non-lactating buffaloes under hot and humid climate of south China. Therefore, this study was planned to explore variations in biological rhythms of physiological, metabolic, hormonal, and oxidative responses of non-lactating Nili-Ravi buffaloes to tropical climatic conditions in Southern China.
Materials and Methods
Animal Welfare Statement
All procedures used in this experiment were approved by the Ethics Committee of the Guangxi Buffalo Research Institute, Chinese Academy of Agriculture Sciences China (Approval No. BRI2017006). All steps of animal rearing, recording of physiological parameters and blood sample collection were conducted strictly according to the guidelines of the committee. We confirm that we followed the rules of the Declaration of Helsinki for recording biological rhythms in buffaloes during this experiment.
Geographical Location and Climate
This study was conducted at the Guangxi Buffalo Research Institute (22°53′22.59″ N; 108°21′51.19″ E; 122 m above sea level) located in Nanning, China. The overall climate of this area is characterized as hot and humid as it is located in the subtropical monsoon climate zone. This area receives sufficient sunshine with abundant rainfall (average 1,304 mm) with longer summer and short winter seasons. We used an online dust monitoring system (Shenzhen Greenford Environmental Technology Co., Ltd.) to record weather data in real-time, mainly including air temperature (AT, °C) and relative humidity (RH, %), with an interval at 30 min and at an installation height of buffalo's body.
Environmental variables were recorded twice daily in the morning (at 8:00 A.M.) and in the afternoon (at 2:30 P.M.) and used to depict monthly meteorological variations during the whole study period (Figure 1). Average daily temperature and relative humidity were recorded during the study period from April 2017 to March 2018 (for 1 year) and Temperature Humidity Index (THI) was calculated by using the following formula (18):
Where, AT is the air temperature and DPT the dew point temperature of the buffalo shed. Four seasons of the year were defined as; spring (March–May), summer (June–August), autumn (September–November), and winter (December–February).
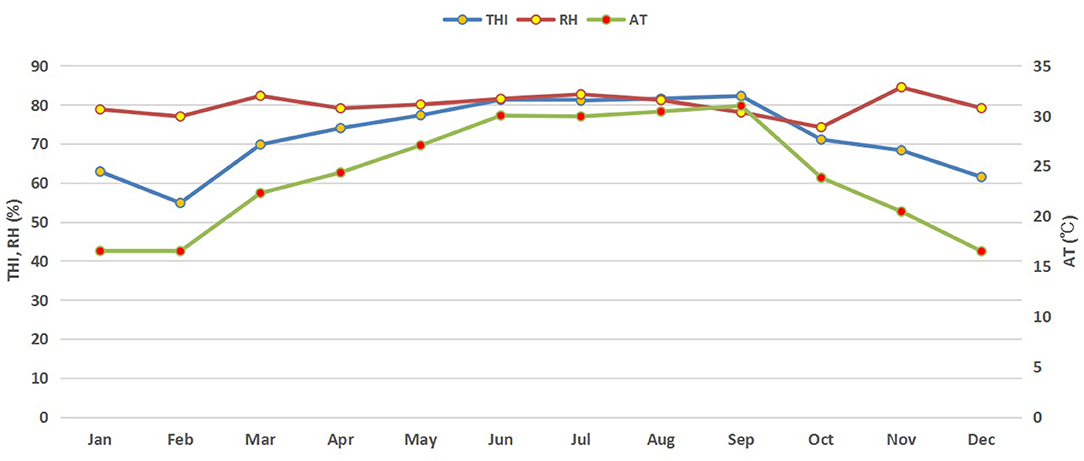
Figure 1. Monthly trends of THI, AT, and RH during the study period. THI, temperature humidity index; RH, relative humidity (%); AT, air temperature (°C).
Selection and Management of Buffaloes
Twenty non-lactating Nili-Ravi buffaloes were randomly selected for this experiment. All buffaloes were multiparous (3–5 lactations) with an average body weight of 550 ± 50 Kg. Buffaloes were housed in an open uncovered area with a stocking density of 15 m2/head throughout the study period. Free access to water was provided to all buffaloes throughout the day. Mist spraying was performed on buffaloes whenever the temperature exceeded 28°C throughout the year. All buffaloes were fed a total mix ration (TMR) consisting of grass (Pennisetum purpureum schum), corn silage and concentrate mixture (corn, soybean meal, and wheat bran). The same quantity of TMR was fed daily to each animal to meet its dietary requirement as per the general routine of the buffalo farm. The formulation and chemical composition of total mix ration are presented in Table 1.
Recording of Physiological Parameters and Blood Sampling
Physiological parameters of buffaloes were recorded weekly as follows:
Body Surface Temperature (BST)
Average BST was recorded each Tuesday at 8:00 A.M. and 2:30 P.M. using an animal infrared thermometer from three different body sites, including forehead, left chest, and abdomen. Three respective values were averaged to get weekly BST for each buffalo.
Rectal Temperature (RT)
At the same time, RT was also recorded by inserting a veterinary rectal thermometer in the rectum of buffalo for 2 min.
Respiratory Rate (RR)
Weekly RR was recorded as times/min by observing and counting thoracic movements using a stopwatch and a counter for 2 min.
Blood Sampling and Determination of Biochemical Parameters
Blood samples (10 mL) from each buffalo were collected from jugular vein before morning feeding once during each season viz: spring (April 17th), summer (July 22nd), autumn (October 18th), and winter (January 28th). These samples were used to determine serum hormones and antioxidant enzymes as follows:
Serum Hormones
Blood samples were put on ice after the collection and immediately transferred to the laboratory for serum isolation. Blood samples were centrifuged at 3,000 rpm for 15 min, and serum was harvested according to standard methods (19). Isolated serum was stored at −20°C until further analysis. Serum hormones including Adrenocorticotropic hormone (ACTH), Insulin, Cortisol, Triiodothyronine (T3), Thyroxine (T4), and growth hormone (GH) were analyzed using commercial ELISA kits according to manufacturer's instructions (CUSABIO BIOTECH CO., Wuhan, China). The coefficient of variation (inter and intra-assay) of these kits was <10% for each assay.
Serum Antioxidant Enzymes
Concentrations of serum antioxidant enzymes including total antioxidant capacity (TAC), malondialdehyde (MDA), superoxide dismutase (SOD), Glutathione peroxidase (GPx), and catalase (CAT) were determined through spectrophotometer using the Nanjing Built-in Kits (www.njjcbio.com) according to manufacturer's instructions. Average values for each enzyme in each season for all buffaloes are expressed as Mean ± s.e. The coefficient of variations (inter and intra-assay) of these kits was in the same range (<10%).
Plasma Biochemical Parameters
Plasma biochemical parameters including total protein (TP), albumin (ALB), globulin (GLB), blood urea nitrogen, and glucose were determined using respective commercially available kits according to manufacturer's instructions. Activities of liver enzymes ALT and AST were also determined using standard kits according to manufacturer's instructions.
Statistical Analysis
Data were analyzed using the general linear model by analysis of variance in SAS software version 9.2 (SAS Institute Inc., Cary, NC, USA). Pearson correlation coefficients between THI and different physiological and biochemical parameters were calculated using the CORR command in SAS.
Results
Monthly Trend of Meteorological Data
Monthly variations in THI, ambient temperature and relative humidity of the buffalo house are presented in Figure 1. Higher average THI values (above 80) were observed in summer (June–September) but started to decrease in autumn and winter. It started increasing again in spring. Similar trends in RR and BST of lactating buffaloes were also observed (Figure 2).
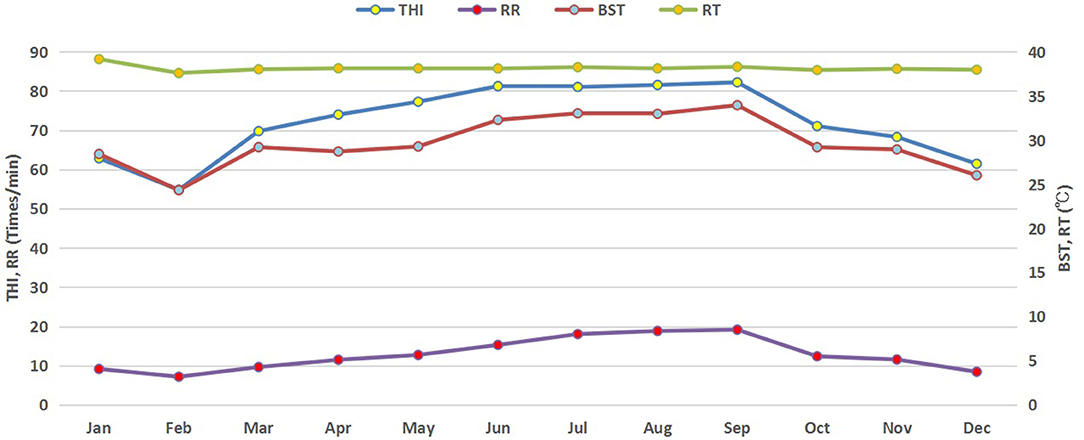
Figure 2. Monthly trends of physiological parameters of buffaloes in response to THI. THI, temperature humidity index; RT, rectal temperature (°C); RR, respiratory rate (times/minute); BST, body surface temperature (°C).
Effect of THI on Physiological Parameters in Different Seasons
The average values of THI and physiological parameters of non-lactating buffaloes in different seasons are provided in Table 2. The THI indices in spring, summer, and autumn were significantly higher than that in winter (p < 0.05), with the highest value in summer, followed by autumn and spring. Moreover, RR, BST, and RT were all the highest in summer, while RT did not change across different seasons (p < 0.05).
Effect of Season on Serum Antioxidant Enzymes
MDA concentrations were significantly (p < 0.05) higher in summer and autumn than in winter and spring (Figure 3). TAC was significantly higher in summer than in other seasons (p < 0.05) while the differences between spring, autumn, and winter were not significant (p > 0.05). However, serum concentrations of GSH, SOD, and CAT were significantly lower in summer but significantly higher in winter compared to other seasons (p < 0.05) as presented in Figure 3.
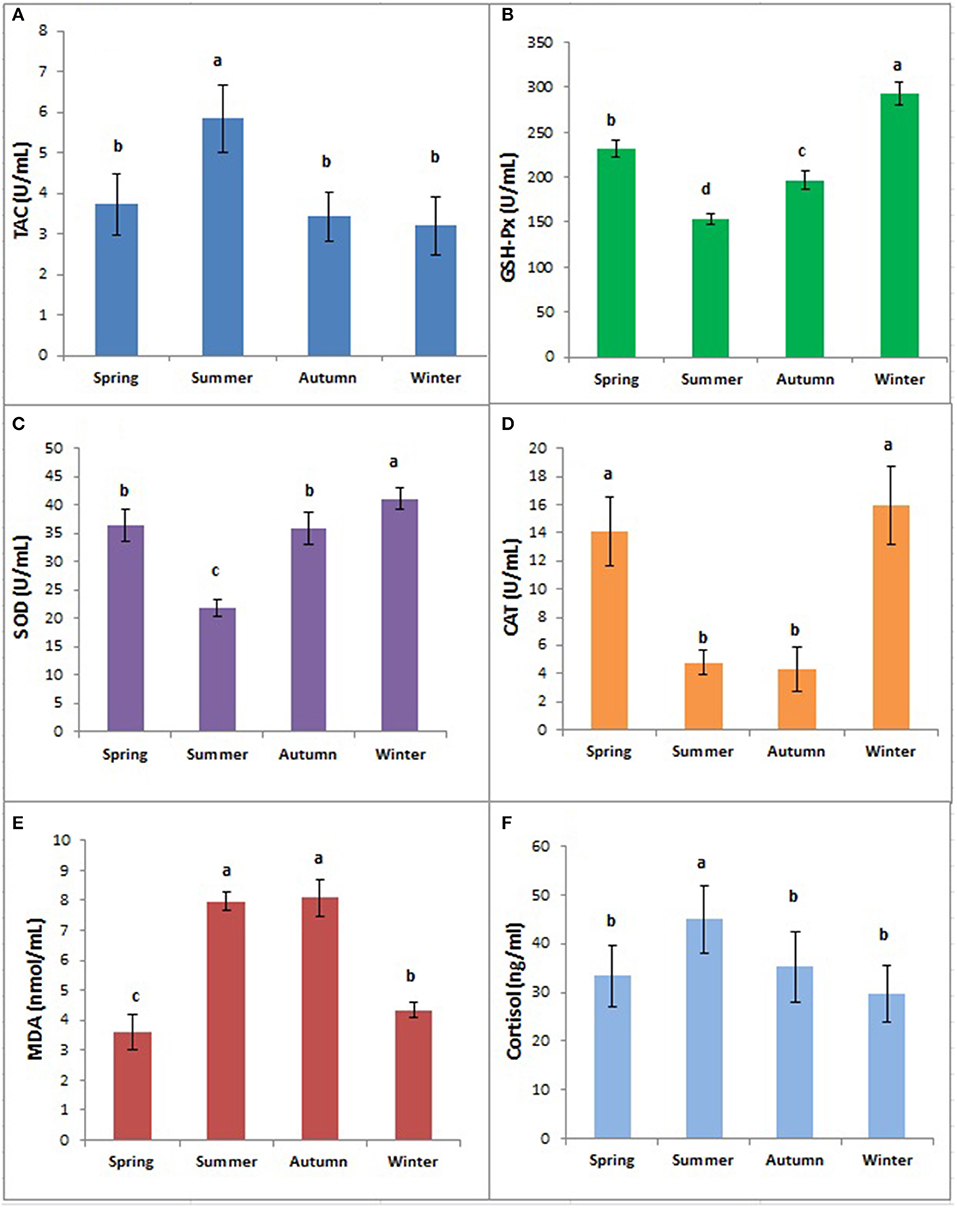
Figure 3. Concentrations of TAC (A), GPx (B), SOD (C), CAT (D), MDA (E), and Cortisol (F) in buffaloes in different seasons. Bars marked with different letters differ significantly (p < 0.05). TAC, total antioxidant capacity; GPx, glutathione peroxidase; SOD, superoxide dismutase; CAT, catalase; MDA, malondialdehyde.
Effect of Season on Serum Hormones
Significantly higher ACTH was observed in winter than other seasons (p < 0.05) (Table 3). However, cortisol was significantly higher in summer than in other seasons (p < 0.05). The insulin concentration in summer was significantly lower than other seasons (p < 0.05). Furthermore, GH and T3 concentrations were significantly lower in summer and autumn as compared to spring and winter (p < 0.05). Contrarily, T4 concentration was significantly higher in summer and autumn than in spring and winter (p < 0.05).
Effect of Season on Serum Biochemical Parameters
Total protein contents were higher in summer followed by spring, autumn, and winter while albumin was significantly higher (p < 0.05) in winter than other seasons (Table 4). Globulin was significantly higher in summer than spring and autumn followed by winter at the end (p < 0.05). Alanine aminotransferase (ALT) enzyme activity was significantly higher in summer than in other seasons (p < 0.05). Aspartate aminotransferase (AST) enzyme activity was significantly higher in spring than in other seasons (p < 0.05). Urea nitrogen was significantly lower in autumn than in other seasons (p < 0.05). Glucose was significantly higher in winter and autumn followed by summer and then winter at the end (p < 0.05) (Table 4).
Correlation of THI With Physiological and Biochemical Parameters
At significant levels (p < 0.05 or 0.01), Pearson correlation analysis showed positive associations of THI with all three physiological parameters of the non-lactating buffaloes, while RT, BST, and RR also positively correlated between each other. Negative correlations (p < 0.05) of THI with ACTH and cortisol were also observed. Both cortisol and T4 were positively correlated each other first and then with all three physiological parameters. Cortisol was negatively correlated with ACTH while T3 showed a positive correlation with GH (Table 5).
Correlation of THI With Serum Antioxidant Enzymes
THI showed highly negative correlations (p < 0.05 or 0.01) with GPx, SOD, and CAT but a positive correlation (P < 0.05) with TAC in the non-lactating buffaloes (Table 6). TAC content showed negative correlations (p < 0.05 or 0.01) with GPx and SOD and MDA content was negatively associated with GPx and CAT (p < 0.01). However, positive collections (P < 0.05 or 0.01) were observed between GPx, SOD, and CAT (Table 6).
Discussion
Climate is one of the major factors that cause oxidative stress and challenge the defense system of an animal, subsequently leading to many physiological manifestations. These physiological changes are the immediate responses of an animal to climatic stress and eventually reflect the level of discomfort or comfort of an animal in a given environment (6). The most obvious physiological effects of heat stress on animals include pronounced changes in their heart and respiratory rates. This metabolic and physiological load induced by heat stress also significantly affects their diurnal and seasonal rhythms (1).
Effect of THI on Physiological Parameters
Our study revealed significant increases in BST and RR of non-lactating buffaloes in summer when THI value went up to 82. Increased RR and pulse rate (PR) reflected the response of animals, as an adaptive strategy, to lower down their body temperatures through evaporative cooling. Studies have reported higher RR and RT but lower PR in buffaloes exposed to high temperatures in a climatic chamber (3) and under pasture grazing system (20). Similarly, dramatic increases in RR, RT, and PR have been observed in cattle under heat stress conditions (21). However, average RT showed no significant difference among different seasons in the present study. Similar findings have been reported in swamp buffaloes at an ambient temperature of 30°C (22, 23). Exposure to high ambient temperatures (>30°C) has shown to stimulate a remarkable increase in RR and subsequent the initiation of panting without any change in RT in buffaloes (22). In our study, despite the high THI in summer, no severe response was found in physiological parameters of the non-lactating buffaloes, including RT as an index of heat storage in the body. This shows that thermo-tolerant ability of the non-lactating buffaloes along with management practices like mist spraying facilitated the mitigation of adverse effects of heat stress under tropical weather.
Serum Antioxidant Indices of the Non-lactating Buffaloes in Different Seasons
Concentrations of antioxidant enzymes and heat shock proteins are one of the most important stress markers reflecting animals' comfort and climatic adaptability (24). We observed a significant increase in serum TAC in the non-lactating buffaloes in summer, indicating their ultimate response of antioxidant enzyme system to oxidative stress to remove excessive ROS. However, activities of CAT, SOD, and GPx were decreased significantly in summer, owing to the inability of antioxidant defense system to combat excessive ROS load (8). That's why such serum antioxidant enzymes have been used as physiological biomarkers to reveal the extent of oxidative stress in animals (9). Similarly, we observed significantly high concentrations of MDA in summer and autumn, revealing a higher degree of oxidative stress as it is a major end product of lipid peroxidation in cells to damage cell membranes. Similar findings regarding high MDA levels in summer have been reported earlier in Murrah buffaloes (25). In our study, a combination of oxidant (MDA) and antioxidant (TAC, GHS-Px, SOD, and CAT) enzymes revealed the high level of oxidative stress in non-lactating buffaloes during summer. These data indicate the need to exploit some cooling practices more effective than mist spraying to completely eliminate adverse effects of heat stress in buffaloes, especially during summer when THI value exceeds 80.
Effect of THI on Hormonal and Biochemical Parameters of the Non-lactating Buffaloes
Exposure of an animal to high ambient temperature adversely affects the activity of its thyroid gland owing its great sensitivity to body temperature and metabolic heat increment (26). In our study, GH concentrations were decreased significantly in summer and autumn, revealing the most obvious sign of heat stress in the non-lactating buffaloes. Reduced plasma GH in summer was in agreement with earlier studies on buffaloes (27, 28). Plasma T3 levels were also decreased in summer and autumn which is in agreement with earlier findings regarding the depression of T3 in buffaloes during the summer stress period (29). Decreases in plasma T3 and T4 during heat stress were considered as an adaptive strategy to reduce basal metabolic rate and then net body heat production (30, 31). Contrary to our findings, no difference in plasma T3 and T4 in Nili-Ravi buffaloes was observed between hot humid and hot dry seasons (32). Fluctuations in T3 and T4 concentrations in response to heat stress are not abrupt as it takes time to change them and subsequently it requires several days to stabilize them at a new steady state (6).
In contrast to T3, plasma T4 was significantly higher in summer and autumn than in spring and winter. Similar findings have been reported earlier as a significantly higher level of T4 was observed in Khuzestan buffalo bulls during the summer season as compared to winter (33). Moreover, it was reported that the concentration of T3, TSH, and free T4 index did not differ between summer and winter seasons in same study. Additionally, no difference between T3 and T4 levels and their ratio has been observed in different seasons (summer, monsoon, and winter) in Murrah buffalo bulls (34). Similarly, no difference in T3 uptake (%) was observed in the summer and winter season in Friesian calves (35). But heat stress significantly reduced the T4 level in summer as compared to winter season while T3 uptake (%) showed no difference between summer and winter seasons as reported in buffaloes and Friesian cows (36). But in the same study, animals that were treated with water spray and a diaphoretic showed a 32.4% increase in T4 levels (42% in free T4 index) making its concentration significantly higher than observed in the summer season (35). Interestingly, T3 concentrations (% T3 uptake) showed a comparatively lower response to this treatment as only a 11% increase in its concentration was observed. Many other studies have also reported that use of evaporative cooling strategies (such as mist spraying or water sprinkling over animal) can significantly alleviate the adverse effects of heat stress and mitigate the decline in T4 levels as observed in crossbred cows (37) and buffaloes (38). Keeping in view of above findings the possible reason for higher plasma T4 levels observed in the present study during summer and autumn is the use of mist spraying over buffaloes whenever the ambient temperature exceeds 28°C. The same trend was not observed for T3 levels because T3 is generally seen as the most metabolically active thyroid hormone and it has a shorter half-life in the blood than T4 (39). The provision of mist spraying or water sprinkling showed no effect on plasma T3 levels in summer season (40). Additionally, wallowing has also shown to decrease free T3 in swamp buffaloes (41). That's why we observed lower plasma T3 in the summer and autumn while higher T4 in Nili-Ravi buffaloes in the present study.
The liver enzymes ALT and AST are the most reliable markers of liver damage or necrosis. The ALT primarily exists in the liver, but AST exists in various tissues like heart, liver, kidney, and so on. In the present study, we observed similar ALT levels during spring, summer, and winter except for autumn during which higher ALT level was observed. However, a higher AST level was found in spring with no change in other seasons. These findings revealed no adverse effect of heat stress on liver health in buffaloes in the present study as values of ALT and AST were within normal physiological limits (42). Animal exposure to ambient temperature up to 30°C, have not shown any significant change in ALT and AST levels in crossbred cattle (43). These observations are in line with our findings as we observed no rise in levels of both liver enzymes during the summer season in buffaloes as the average ambient temperature did not exceed above 30°C in the present study. No changes in the concentration of AST have been observed in the Surti buffalo during summer and winter seasons. However, a significant increase in ALT content was observed in the summer (under hot dry and hot humid conditions when THI increased from 68 to 81) as compared to winter season (44). Similar findings regarding the increase in ALT in the summer season (hot periods of the year) have been reported in cattle (45, 46). Heat stress during metabolically demanding conditions including lactation, pregnancy, or growth can put adverse effects on liver physiology, which is not the case with dry buffaloes as no changes in liver enzymes were observed during the summer season in the present study. Both AST and gamma-glutamyl transferase (GGT) have shown excellent predictive value for liver damage in cattle but in case of buffalo GGT is reported to be a more reliable indicator of liver damage (47). As we did not measure GGT in this study which is a limitation of the present study and should be considered in future studies.
Significantly high plasma cortisol was observed in summer, suggesting a high level of oxidative stress. Stressful conditions like heat stress result in activation of hypothalamic–pituitary–adrenal axis (HPA) which results in increased secretion of hypothalamic peptides including corticotrophin releasing hormone (CRH) and arginine vasopressin (AVP) into pituitary portal circulation, where they stimulate ACTH secretion. This, in turn, triggers an increase in cortisol release from the adrenal gland to act on multiple targets to restore homeostasis (48). Increased plasma cortisol levels observed in summer in the present study, indicated enhanced activity of HPA to facilitate homeostasis and mitigate the adverse effects of heat stress. Similar findings regarding high cortisol contents in summer have been reported earlier in Egyptian buffaloes (1), Murrah buffaloes (17, 29), and Sahiwal cows (13). Moreover, elevated cortisol levels have been observed in crossbred buffaloes (Nili-Ravi × Murrah) as compared to purebred Mediterranean buffaloes (49).
Contrary to cortisol, we observed significantly higher level of ACTH in buffaloes during summer. Regulation of ACTH secretion within the HPA axis is a multifactorial process, with the hypothalamic peptides CRH and AVP being important physiological stimulators, and cortisol along with other adrenal glucocorticoids as a major inhibitory factor by feedback loops (50, 51). Inhibition of the ACTH response to CRH and AVP by cortisol depends on the length of exposure: fast (within seconds to minutes), intermediate (2–10 h) and slow (hours to days) feedback (50). Both fast and intermediate feedbacks inhibit ACTH release rather than synthesis, while the slow feedback appears to affect both ACTH release and synthesis. The higher plasma cortisol levels results in negative feedback to subsequently lower the plasma ACTH levels through mediating complex interaction with CRH and AVP (52). It has also been reported earlier that ACTH increases in response to the onset of stress and then decreases in spite of continuing heat stimulus, indicating a negative glucocorticoid feedback and a decrease in the glucocorticoid-binding transcortin (53). This is the possible reason for lower ACTH levels observed in buffaloes during summer when plasma cortisol was quite higher in the present study.
We observed significantly low plasma insulin level in summer, in agreement with an early report (54). However, contrary findings like increase (23) or no change in insulin concentration (55) have been reported in cows under heat stress. Such variations are mainly attributed to different metabolic status, nutrition, physiological stage and thermo-tolerance ability of the animals.
Association of THI With Physiological and Biochemical Parameters
THI has been efficiently used to evaluate the level of heat stress in animals (56, 57). Our study showed positive associations of THI with physiological parameters (BST, RR, and RT) and serum TAC content. However, moderately negative correlations of THI with ACTH and cortisol were observed, a bit strange as early study has reported a positive association of THI with cortisol content (29). However, a negative correlation of THI with serum antioxidant enzymes was in agreement with earlier studies on dairy cows (57, 58) and buffaloes (29). The decrease in activity of antioxidant enzymes during summer revealed a reduced total antioxidant capacity to manage the excessive load of ROS which has been associated with reproductive acyclicity in buffaloes, owing to severe damage to follicular cells (59). Usually non-lactating buffaloes are neglected in terms of management of heat stress as compared to lactating animals, which subsequently affects their reproductive performance. In our study, we observed average THI above 80 in summer which was well-correlated with the physiological and oxidative responses of non-lactating buffaloes. Therefore, it is recommended that in summer, buffaloes should be allowed to swimming for some period, especially during the hot period of the day, to alleviate adverse effects of heat stress. Earlier studies have also suggested the use of cooling strategies during months from April to September to alleviate heat stress in dairy animals (60). To best of our knowledge, present study is the first report on the seasonal dynamics of physiological and metabolic responses of non-lactating Nili-Ravi buffaloes to oxidative stress under the tropical climate in South China. It provides practical insights on the adaptive physiology of buffaloes and has several implications regarding the alleviation of heat stress in buffaloes to enhance the efficiency of performance and avoid animal welfare issues under tropical climate. However, a relatively small sample size is a limitation of this study that needs to be accounted for in future studies.
Conclusions
High THI in summer was associated with significant increases in physiological parameters, serum MDA and cortisol concentrations in non-lactating buffaloes. Moreover, THI showed a highly negative association with serum total antioxidant capacity as it significantly reduced serum contents of GSH, SOD, and CAT in summer. Plasma T3 and GH concentrations were also reduced in summer. Our study reveals that summer months (from June to September) are critical in buffalo management as THI value exceeds 80, so cooling practices are required to alleviate heat stress to avoid performance losses and animal welfare issues. However, further studies are required on a larger cohort of samples to corroborate the association of THI thresholds with physiological and biochemical parameters in the non-lactating buffaloes under tropical climatic conditions.
Data Availability Statement
All datasets generated for this study are included in the article/supplementary material.
Ethics Statement
The animal study was reviewed and approved by Ethics Committee of the Guangxi Buffalo Research Institute, Chinese Academy of Agriculture Sciences China (Approval No. BRI2017006). All animals studied were the property of the Guangxi Buffalo Research Institute.
Author Contributions
ML, ZT, and CY: conceptualization. FH: data curation. ML: formal analysis. CY: funding acquisition. ML, ZT, FX, and LP: investigation. ML, YG, FX, and LP: methodology. XL: project administration. YG, ZT, XL, FX, LP, and CY: resources. FH: software and writing—original draft. CY: supervision and validation. FH and XL: visualization. ML and CY: writing—review and editing. All authors contributed to the article and approved the submitted version.
Funding
This work was supported by grants from the National Key Research and Development Program of China (2016YFD0500507 and 2018YFD0501602). The APC will be covered by the same grant.
Conflict of Interest
The authors declare that the research was conducted in the absence of any commercial or financial relationships that could be construed as a potential conflict of interest.
References
1. Marai I, Haeeb A. Buffalo's biological functions as affected by heat stress—a review. Livestock Sci. (2010) 127:89–109. doi: 10.1016/j.livsci.2009.08.001
2. Gauly M, Bollwein H, Breves G, Brügemann K, Dänicke S, Daş G, et al. Future consequences and challenges for dairy cow production systems arising from climate change in Central Europe–a review. Animal. (2013) 7:843–59. doi: 10.1017/S1751731112002352
3. Wankar AK, Singh G, Yadav B. Thermoregulatory and adaptive responses of adult buffaloes (Bubalus bubalis) during hyperthermia: physiological, behavioral, and metabolic approach. Vet World. (2014) 7:825–30. doi: 10.14202/vetworld.2014.825-830
4. Alhussien MN, Dang AK. Diurnal rhythm in the counts and types of milk somatic cells, neutrophil phagocytosis and plasma cortisol levels in Karan Fries cows during different seasons and parity. Biol Rhythm Res. (2018) 49:187–99. doi: 10.1080/09291016.2017.1350442
5. Bernabucci U, Lacetera N, Baumgard LH, Rhoads RP, Ronchi B, Nardone A. Metabolic and hormonal acclimation to heat stress in domesticated ruminants. Animal. (2010) 4:1167–83. doi: 10.1017/S175173111000090X
6. Silanikove N. Effects of heat stress on the welfare of extensively managed domestic ruminants. Livestock Production Sci. (2000) 67:1–18. doi: 10.1016/S0301-6226(00)00162-7
7. Tanaka M, Kamiya Y, Kamiya M, Nakai Y. Effect of high environmental temperatures on ascorbic acid, sulfhydryl residue and oxidized lipid concentrations in plasma of dairy cows. Anim Sci J. (2007) 78:301–6. doi: 10.1111/j.1740-0929.2007.00439.x
8. Aréchiga C, Vázquez-Flores S, Ortiz O, Hernandez-Ceron J, Porras A, McDowell L, et al. Effect of injection of β-carotene or vitamin E and selenium on fertility of lactating dairy cows. Theriogenology. (1998) 50:65–76. doi: 10.1016/S0093-691X(98)00114-9
9. Celi P. Biomarkers of oxidative stress in ruminant medicine. Immunopharmacol Immunotoxicol. (2011) 33:233–40. doi: 10.3109/08923973.2010.514917
10. Sejian V, Srivastava R. Interrelationship of endocrine glands under thermal stress: effect of exogenous glucocorticoids on mineral, enzyme, thyroid hormone profiles and phagocytosis index of Indian goats. Endocr Regul. (2010) 44:101–7. doi: 10.4149/endo_2010_03_101
11. Tilbrook AJ, Turner AI, Clarke IJ. Effects of stress on reproduction in non-rodent mammals: the role of glucocorticoids and sex differences. Rev Reprod. (2000) 5:105–13. doi: 10.1530/revreprod/5.2.105
12. Chaiyabutr N, Chanpongsang S, Suadsong S. Effects of evaporative cooling on the regulation of body water and milk production in crossbred Holstein cattle in a tropical environment. Int J Biometeorol. (2008) 52:575–85. doi: 10.1007/s00484-008-0151-x
13. Alhussien MN, Dang AK. Impact of different seasons on the milk somatic and differential cell counts, milk cortisol and neutrophils functionality of three Indian native breeds of cattle. J Thermal Biol. (2018) 78:27–35. doi: 10.1016/j.jtherbio.2018.08.020
14. Zhengkang H, Zhenzhong C, Shaohua Z, Vale W, Barnabe V, Mattos J. Rumen metabolism, blood cortisol and T3, T4 levels and other physiological parameters of swamp buffalo subjected to solar radiation. Proas World Buffalo Cong. (1994) 2:39–40.
15. Dimri U, Ranjan R, Sharma MC, Varshney V. Effect of vitamin E and selenium supplementation on oxidative stress indices and cortisol level in blood in water buffaloes during pregnancy and early postpartum period. Trop Anim Health Prod. (2010) 42:405–10. doi: 10.1007/s11250-009-9434-4
16. Hooda O, Singh G. Effect of thermal stress on feed intake, plasma enzymes and blood biochemicals in buffalo heifers. Indian J Anim Nutr. (2010) 27:122–7.
17. Bombade K, Kamboj A, Alhussien MN, Mohanty AK, Dang AK. Diurnal variation of milk somatic and differential leukocyte counts of Murrah buffaloes as influenced by different milk fractions, seasons and parities. Biol Rhythm Res. (2018) 49:151–63. doi: 10.1080/09291016.2017.1345472
19. Coles EH. Veterinary Clinical Pathology, 4th Edn. Philadelphia, PA: W.B. Saunders Company (1986).
20. Carvalho BC, Rodrigues dSJA, Guerreiro ML, Alves VR, Souza NBd, Maciele SAG, et al. Infrared thermography to assess thermoregulatory reactions of female buffaloes in a humid tropical environment. Front Vet Sci. (2020) 7:180. doi: 10.3389/fvets.2020.00180
21. Banerjee D, Ashutosh. Circadian changes in physiological responses and blood ionized sodium and potassium concentrations under thermal exposure in Tharparkar and Karan Fries heifers. Biol Rhythm Res. (2011) 42:131–9. doi: 10.1080/09291011003729411
22. Chikamune T. Effect of environmental temperature on thermoregulatory responses and oxygen consumption in swamp buffalo and Holstein cattle. Buffalo J. (1986) 2:151–60.
23. Chaiyabutr N, Buranakarl C, Muangcharoen V, Loypetjra P, Pichaicharnarong A. Effects of acute heat stress on changes in the rate of liquid flow from the rumen and turnover of body water of swamp buffalo. J Agric Sci. (1987) 108:549–53. doi: 10.1017/S0021859600079934
24. Lacetera N, Bernabucci U, Scalia D, Basiricò L, Morera P, Nardone A. Heat stress elicits different responses in peripheral blood mononuclear cells from Brown Swiss and Holstein cows. J Dairy Sci. (2006) 89:4606–12. doi: 10.3168/jds.S0022-0302(06)72510-3
25. Lallawmkimi MC, Singh S, Upadhyay R, De S. Impact of vitamin E supplementation on heat shock protein 72 and antioxidant enzymes in different stages of Murrah buffaloes during seasonal stress. Indian J Anim Sci. (2013) 83:909–15.
26. Pereira AM, Baccari F, Titto EA, Almeida JA. Effect of thermal stress on physiological parameters, feed intake and plasma thyroid hormones concentration in Alentejana, Mertolenga, Frisian and Limousine cattle breeds. Int J Biometeorol. (2008) 52:199–208. doi: 10.1007/s00484-007-0111-x
27. Mitra R, Christison G, Johnson H. Effect of prolonged thermal exposure on growth hormone (GH) secretion in cattle. J Anim Sci. (1972) 34:776–9. doi: 10.2527/jas1972.345776x
28. Igono M, Johnson H, Steevens B, Hainen W, Shanklin M. Effect of season on milk temperature, milk growth hormone, prolactin, and somatic cell counts of lactating cattle. Int J Biometeorol. (1988) 32:194–200. doi: 10.1007/BF01045279
29. Lakhani P, Alhussien MN, Lakhani N, Jindal R, Nayyar S. Seasonal variation in physiological responses, stress and metabolic-related hormones, and oxidative status of Murrah buffaloes. Biol Rhythm Res. (2018) 49:844–52. doi: 10.1080/09291016.2018.1424775
30. Collier RJ, Collier J, Rhoads R, Baumgard L. Invited review: genes involved in the bovine heat stress response. J Dairy Sci. (2008) 91:445–54. doi: 10.3168/jds.2007-0540
31. Farooq U, Samad H, Shehzad F, Qayyum A. Physiological responses of cattle to heat stress. World Appl Sci J. (2010) 8:38–43.
32. Das K, Singh J, Singh G, Upadhyay R, Malik R, Oberoi P. Heat stress alleviation in lactating buffaloes: effect on physiological response, metabolic hormone, milk production and composition. Indian J Anim Sci. (2014) 84:275–80.
33. Mayahi S, Mamouei M, Tabatabaei S, Mirzadeh K. Reproductive characteristics and thyroidal function in relation with season in Khuzestan buffalo (Bubalus bubalis) bulls. Vet Res Forum Int Q J. (2014) 5:201–5.
34. Dixit NK, Agrmal SP, Agarml VK, Dwarkna PK. Seasonal vabiations in serum levels of thyroid hormones and their relation with seminal quality and libido in buffalo bulls. Theriogenology. (1984) 22:497–507. doi: 10.1016/0093-691X(84)90049-9
35. Marai IFM, Habeeb AA, Daader AH, Yousef HM. Effects of Egyptian subtropical summer conditions and the heat-stress alleviation technique of water spray and a diaphoretic on the growth and physiological functions of Friesian calves. J Arid Environ. (1995) 30:219–25. doi: 10.1016/S0140-1963(05)80073-4
36. E1-Masry KA, Habeeb AA. Thyroid function in lactating Friesian cows and water buffaloes in winter and summer Egyptian conditions. In: 3rd Egyptian-British Conference on Animal, Fish and Poultry Production. Alexandria University, Alexandria (1989). p. 613–20.
37. Aggarwal A, Singh M. Changes in hormonal levels during early lactation in summer calving cows kept under mist cooling system. Indian J Anim Nutr. (2009) 26:337−40.
38. Aggarwal A, Singh M. Hormonal changes in heat-stressed Murrah buffaloes under two different cooling systems. Buffalo Bull. (2010) 29:1–6.
39. Anderson RR. Secretion rates of thyroxine and triiodothyronine in dairy cattle. J Dairy Sci. (1971) 54:1195–9. doi: 10.3168/jds.S0022-0302(71)85999-4
40. Aggarwal A, Upadhyay R. Heat Stress and Animal Productivity. New Delhi: Springer (2013). p. 27–44.
41. Khongdee T, Sripoon S, Vajrabukka C. The effects of high temperature and wallow on physiological responses of swamp buffaloes (Bubalus bubalis) during winter season in Thailand. J Thermal Biol. (2011) 36:417–21. doi: 10.1016/j.jtherbio.2011.07.006
42. Duncan JR, Prasse KW, Mahaffey EA. Veterinary Laboratory Medicine, Clinical Pathology. 4th ed. Ames, IA: Iowa State University Press (2003).
43. Yadav B, Singh G, Wankar A, Dutta N, Chaturvedi VB, Verma MR. Effect of simulated heat stress on digestibility, methane emission and metabolic adaptability in crossbred cattle. Asian Austral J Anim Sci. (2016) 29:1585–92. doi: 10.5713/ajas.15.0693
44. Chaudhary SS, Singh VK, Upadhyay RC, Puri G, Odedara AB, Patel PA. Evaluation of physiological and biochemical responses in different seasons in Surti buffaloes. Vet World. (2015) 8:727–31. doi: 10.14202/vetworld.2015.727-731
45. Singh K, Bhattacharyya NK. Cardio-respiratory activity in zebu and their F1 crosses with European breeds of dairy cattle at different ambient temperatures. Livestock Production Sci. (1990) 24:119–28. doi: 10.1016/0301-6226(90)90072-E
46. Koubkova M, Knizkova I, Kunc P. Influence of high environmental temperatures and evaporative cooling on some physiological, hematological and biochemical parameters in high-yielding dairy cows. Czech J Anim Sci. (2002) 47:309–18.
47. Minervino AHH, Barrêto RA, Queiroz GF, Headley SA, Ortolani EL. Predictive values of aspartate aminotransferase and gamma-glutamyl transferase for the hepatic accumulation of copper in cattle and buffalo. J Vet Diagnostic Investig. (2008) 20:791–5. doi: 10.1177/104063870802000613
48. Buckingham J, Cowell A, Gillies G, Herbison A, Steel J. Stress and the hypothalamo-pituitary-adrenal (HPA) axis. In: Buckingham JC, Cowell A-M, Gillies GE, editors. Stress, Stress Hormones, and the Immune System. New York, NY: John Wiley & Sons (1997). p. 26–36.
49. Shenhe L, Jun L, Zipeng L, Tingxian D, ur Rehman Z, Zichao Z, et al. Effect of season and breed on physiological and blood parameters in buffaloes. J Dairy Res. (2018) 85:181–4. doi: 10.1017/S0022029918000286
50. Kellerwood M, Dallman MF. Corticosteroid inhibition of ACTH secretion. Endocrine Rev. (1984) 5:1–24. doi: 10.1210/edrv-5-1-1
51. Aguilera G. Regulation of pituitary ACTH secretion during chronic stress. Front Neuroendocrinol. (1994) 15:321–50. doi: 10.1006/frne.1994.1013
52. Evans MJ, Mulligan RS, Livesey JH, Donald RA. The integrative control of adrenocorticotrophin secretion: a critical role for corticotrophin-releasing hormone. J Endocrinol. (1996) 148:475–83. doi: 10.1677/joe.0.1480475
53. Lindner HR. Comparative aspects of cortisol transport: lack of firm binding to plasma proteins in domestic ruminants. J Endocrinol. (1964) 28:301–20. doi: 10.1677/joe.0.0280301
54. Abdel-Samee A, Habeeb A, Kamal T, Abdel-Razik M. The role of urea and mineral mixture supplementation in improving productivity of heat stressed Friesian calves in the subtropics [Animals, fish, and poultry production]. In: Proceedings of 3rd Egyptian-British Conference on Animal, Fish and Poultry Production, Vol. 2. Egypt: Alexandria University (1989). p. 637–41.
55. McVeigh J, Tarrant P, Harrington M. Behavioral stress and skeletal muscle glycogen metabolism in young bulls. J Anim Sci. (1982) 54:790–5. doi: 10.2527/jas1982.544790x
56. Gantner V, Mijić P, Kuterovac K, Solić D, Gantner R. Temperature-humidity index values and their significance on the daily production of dairy cattle. Mljekarstvo. (2011) 61:56–63.
57. Alhussien MN, Dang AK. Integrated effect of seasons and lactation stages on the plasma inflammatory cytokines, function and receptor expression of milk neutrophils in Sahiwal (Bos indicus) cows. Vet Immunol Immunopathol. (2017) 191:14–21. doi: 10.1016/j.vetimm.2017.07.010
58. Polsky L, von Keyserlingk MA. Invited review: effects of heat stress on dairy cattle welfare. J Dairy Sci. (2017) 100:8645–57. doi: 10.3168/jds.2017-12651
59. Jan M, Das G, Khan F, Singh J, Bashir S, Khan S, et al. Evaluation of follicular oxidant-antioxidant balance and oxidative damage during reproductive acyclicity in water buffalo (Bubalus bubalis). Asian Pac J Reprod. (2014) 3:35–40. doi: 10.1016/S2305-0500(13)60182-7
Keywords: temperature humidity index, heat stress, physiological response, oxidative stress, serum metabolites, buffalo
Citation: Li M, Hassan F, Guo Y, Tang Z, Liang X, Xie F, Peng L and Yang C (2020) Seasonal Dynamics of Physiological, Oxidative and Metabolic Responses in Non-lactating Nili-Ravi Buffaloes Under Hot and Humid Climate. Front. Vet. Sci. 7:622. doi: 10.3389/fvets.2020.00622
Received: 16 April 2020; Accepted: 31 July 2020;
Published: 08 September 2020.
Edited by:
Antonio Minervino, Federal University of Western Pará, BrazilReviewed by:
Veerasamy Sejian, Indian Council of Agricultural Research (ICAR), IndiaPreeti Lakhani, Lala Lajpat Rai University of Veterinary and Animal Sciences, India
Copyright © 2020 Li, Hassan, Guo, Tang, Liang, Xie, Peng and Yang. This is an open-access article distributed under the terms of the Creative Commons Attribution License (CC BY). The use, distribution or reproduction in other forums is permitted, provided the original author(s) and the copyright owner(s) are credited and that the original publication in this journal is cited, in accordance with accepted academic practice. No use, distribution or reproduction is permitted which does not comply with these terms.
*Correspondence: Chengjian Yang, eWNqMDc0NkBzaW5hLmNvbQ==
†These authors have contributed equally to this work