- 1Department of Poultry, Faculty of Agriculture, Zagazig University, Zagazig, Egypt
- 2Agriculture Department, Faculty of Environmental Sciences, King Abdulaziz University, Jeddah, Saudi Arabia
- 3The Strategic Center to Kingdom Vision Realization, King Abdulaziz University, Jeddah, Saudi Arabia
- 4Animal and Poultry Production Department, Faculty of Agriculture, Damanhour University, Damanhour, Egypt
- 5Forensic Medicine and Toxicology Department, Faculty of Veterinary Medicine, Zagazig University, Zagazig, Egypt
- 6Department of Poultry Production, Faculty of Agriculture, Fayoum University, Fayoum, Egypt
- 7Department of Biological Sciences, Zoology, King Abdulaziz University, Jeddah, Saudi Arabia
- 8Department of Pathology, Faculty of Veterinary Medicine, Alexandria University, Alexandria, Egypt
- 9Department of Physiology, Veterinary Faculty, University of Sarajevo, Sarajevo, Bosnia and Herzegovina
- 10Department of Animal and Fish Production, King Faisal University, Al-Hufof, Saudi Arabia
The novel coronavirus (SARS-CoV-2) infection (COVID-19) has raised considerable concern on the entire planet. On March 11, 2020, COVID-19 was categorized by the World Health Organization (WHO) as a pandemic infection, and by March 18, 2020, it has spread to 146 countries. The first internal defense line against numerous diseases is personalized immunity. Although it cannot be claimed that personalized nutrition will have an immediate impact on a global pandemic, as the nutritional interventions required a long time to induce beneficial outcomes on immunity development, nutritional strategies are still able to clarify and have a beneficial influence on the interplay between physiology and diet, which could make a positive contribution to the condition in the next period. As such, a specific goal for every practitioner is to evaluate different tests to perceive the status of the patient, such as markers of inflammation, insulin regulation, and nutrient status, and to detect possible imbalances or deficiencies. During the process of disease development, the supplementation and addition of different nutrients and nutraceuticals can influence not only the viral replication but also the cellular mechanisms. It is essential to understand that every patient has its individual needs. Even though many nutrients, nutraceuticals, and drugs have beneficial effects on the immune response and can prevent or ameliorate viral infections, it is essential to detect at what stage in COVID-19 progression the patient is at the moment and decide what kind of nutrition intervention is necessary. Furthermore, understanding the pathogenesis of coronavirus infection is critical to make proper recommendations.
Introduction
Coronaviridae is one of the families of viruses that are known to cause diseases in birds and mammals ranging from the common cold to severe acute respiratory syndrome (SRAS). A new species that belongs to this family emerged in late 2019, causing an outbreak of a respiratory disease in the eastern part of Asia. The first species of coronavirus infecting humans (229E) was observed in 1931 and isolated first in 1965. Ultimately, the virus has evolved into the novel coronavirus (2019-n-CoV).
Regarding the current situation of the COVID-19 pandemic, in which there is no optimal vaccination or cure, the functionality and efficiency of the immune response is the key factor in the defense against viral infections. Several nutrients, especially vitamins and microelements, are crucial for the immune system to function normally (1). Moreover, dietary supplementations of such nutrients have beneficial impacts on the immune responses to viral infections. Studies showed that after influenza vaccination, the supplementation of vitamins A and D raised the humoral immunity of pediatric patients (2). In patients with torque teno virus (TTV), dietary supplementation with high levels of zinc resulted in improved immunity (3). Also, high doses of selenium had positive effects on the immune response after influenza vaccination (4).
In the literature, several micronutrients, herbal therapeutics, and probiotics were reported to have positive effects in both treatment and prophylaxis of viral infections (5). Also, various nutraceuticals were found to have immunomodulatory effects (6, 7). Malnutrition, as a major cause of increased morbidity and mortality, also has a remarkable economic influence on the healthcare system, depending on the economic situations of each country (8). Such repercussions of malnutrition are reflections of the increased infection rates and delayed recovery as well. Also, in infection, the requirements for various nutrients become higher (9). Nutrition is an essential regulator of the immune homeostasis, and even small deficiencies of some micronutrients could disturb the immune response (10). Lately, Calder et al. reported that balanced nutrition is of crucial importance in the protection from viral infection (11), while Jayawardena et al. recommended that some nutraceuticals and probiotics could be useful in possible prevention and management of COVID-19 (12).
Regarding the COVID-19, it is fundamental to demonstrate the data on increasing the immune response to viral infections. The present review paper principally subjects influenza-like viral infections. Nonetheless, other viral infections have also been taken into consideration. Therefore, practical recommendations on using nutrients for the prevention and therapy of COVID-19 are given. Accordingly, the aim of this review was to provide a complementary overview of the recently available scientific literature on the strategy of using nutraceuticals in boosting the immune system under the COVID-19 pandemic. Emphasis is placed on the supplementations of nutraceuticals, probiotics, vitamins, and trace elements.
Symptoms and Risk Factors of COVID-19
Two to 14 days after exposure to the virus, the usual clinical signs are dry cough and shortness of breath. The Centers for Disease Control and Prevention (CDC) reported that the appearance of fever, chills, myalgia, headache, as well as the loss of smell and taste, could indicate an infection. The majority of humans have mild symptoms if any occur, and it has been reported that ca. 80% of infected people do not need hospitalization (13).
Nevertheless, in some individuals, the infection can cause severe problems. With aging, the immune response becomes weaker (immunosenescence), and the levels of inflammatory mediators in the blood increases (inflammageing). The high-risk group of COVID-19 that are clinically vulnerable includes primarily elderly individuals and those with inflammation-associated conditions such as overweight and obesity, chronic obstructive pulmonary disease, cardiovascular diseases, diabetes, kidney disease, etc. The majority of severe COVID-19 cases and mortalities were within the abovementioned group of individuals, although it is worrisome that younger and healthy individuals are showing up in this cohort (14, 15).
The virus rapidly multiplies and infects the surrounding cells throughout the respiratory system. At the time the virus gets to the lungs, an inflammatory process starts in the mucous membranes and damages the alveoli, which have difficulties in supplying oxygen, resulting in breathing difficulties. This can cause swelling in the lungs, which can result in the accumulation of fluids and dead cells and, finally, severe pneumonia. The infection can spread through mucous membranes of the body, such as the digestive system. In some cases, gastrointestinal symptoms like diarrhea, indigestion, and vomiting have been reported along with respiratory symptoms, and studies propose the possibility of fecal–oral transmission (16). The typical symptoms of COVID-19 and their incidence percentage in 55,924 laboratory confirmed cases are illustrated in Figure 1 (according to the report of the WHO-China Joint Mission; 20 Feb., 2020).
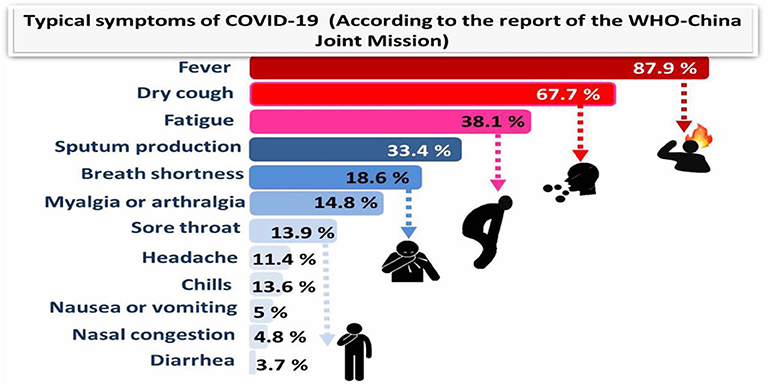
Figure 1. Typical symptoms of COVID-19 (according to the report of the WHO-China Joint Mission) relied on 55,924 COVID-19 confirmed cases (February 2020).
SARS-CoV-2 has been isolated from the brainstems of positive patients, which indicates that this virus has common pathways of transmission with other coronaviruses that can spread through synapse-connected routes from the lung and airways. The virus can cause problems in different parts of the body, such as the heart, liver, and kidneys, particularly in the later stage of the disease. Fatal cases of COVID-19 can be caused by multiple organ failure, especially in individuals with genetic immune factors (17, 18).
The Role of Nutraceuticals in Raising the Immunity for Confrontation of the COVID-19 Pandemic
This review highlights the nutritional interventions to increase the immune response in the body during viral infections, especially considering the novel coronavirus pandemic. Due to their beneficial effects on general healthcare and disease prophylaxis, nutraceuticals have been gaining more and more importance lately (19). Nutraceutical is composed of two words: nutrient and pharmaceutical. It has a vital role in maintaining the healthy body and thus prevents the body from diseases. Several nutraceuticals have been reported to have a significant impact on enhancing the immune system and aid in the treatment and/or protection of viral infections, particularly influenza-like diseases (19, 20). In the current COVID-19 pandemic situation and unavailability of the drugs, these safe alternatives such as nutraceuticals can provide a significant value against the combat of COVID-19.
Over an extended period, obesity has been correlated with a higher occurrence of chronic diseases. Research findings indicate that obesity increases infectious diseases risk (21). The latest evidences in patients suffering from COVID-19 reported that the occurrence and severity of the disease are related to BMI ≥ 28 kg m−2 (22). Based on translational data, it could be a result of metabolic alterations.
In obese patients, the profile of T cells showed inhibition of the function and activation of these crucial immune cells (23). El-Kader and Al-Jiffri (24) conducted a study on 100 obese individuals with chronic infection of HIV and demonstrated that leukocytes, monocytes, total count of neutrophils, CD3, CD4, and CD8 lymphocytes showed significantly lower mean values in the individuals that went through a weight loss program, compared with the control group (24). However, there are two apparent limitations of this review. Firstly, due to the heterogenic nature of the studies, a meta-analysis was not carried out. Secondly, the review did not include studies on the impact of nutrient supplementation in hepatitis C virus (HCV) patients. Despite this, we think that clinical trials on HCV may enrich the aim of this review, discussing the respiratory infections such as COVID-19.
Qualitative evidences by the Jadad scale identified trials (<30%) with a score of <3 points, showing low qualities of methodology. Such studies were not ignored, mainly since a meta-analysis was not done for pooled estimates. On the other side, 24 types of research (>54%) had a score >3, which suggests a valuable methodology. Although it is well-known that exercise is a very active booster of immunity (25), whereas stress strongly alters the immune response (26), this review did not consider such studies as they are outside the scope of this paper.
Several in vivo and in vitro researches on animals have investigated the antiviral properties of trace elements, vitamins, and other nutraceuticals (27, 28). Nevertheless, it is not easy to give conclusions or make recommendations from these studies, and there is a need for further human clinical trials regarding COVID-19.
Micronutrient deficiencies have to be determined in the early stages to set the right therapeutic dose. If the individual micronutrient deficiencies are absent, each malnourished individual should be provided with a multivitamin and mineral (MVM) supplement (29). To increase the immune response of the body, an obese individual (BMI > 25 kg m−2) should reduce at least 5% of the weight of the body (30). Diabetes mellitus patients must have a balanced food to keep normal glucose levels and increase immunity (31) by having diets with the low glycemic index, limiting the consumptions of high fat and sugary or starchy diets, and choosing lean protein varieties (31).
The Role of Vitamins
Numerous vitamins are crucial for the normal functions of the immune response (1). For maintaining the vitamin homeostasis in the body, it is vital to have a varied and balanced diet (32). The dietary supplementation of vitamin D may have positive effects on individuals who are either insufficient or deficient. Evidence supporting the role of vitamin D in reducing the risk of COVID-19 includes the fact that the outbreak occurred in winter, a time when 25-hydroxyvitamin D (25(OH)D) concentrations are lowest; that the number of cases in the Southern Hemisphere near the end of summer are low; that vitamin D deficiency has been found to contribute to acute respiratory distress syndrome; and that case-fatality rates increase with age and with chronic disease comorbidity, both of which are associated with lower 25(OH)D concentration (33). Vitamin E, as a well-known antioxidant, also has functions in regulating the immune response. Meanwhile, numerous studies showed that supplementation with vitamin E could have harmful effects on the immune system, especially in cancer and cardiovascular diseases. There was no conclusive evidence of the role of vitamin E in the treatment of COVID-19, but it is believed that vitamin E protects the integrity of cell membranes from damage caused by free radicals and has the potential to influence both innate and adaptive immunity.
Moreover, excessive amounts of vitamin E could have fatal consequences. A recent study reported that dietary supplementation with high concentrations of micronutrients and vitamins C and D is an effective and low-cost method to intensify the immune response to COVID-19 and similar respiratory diseases (33, 34). It is well-known that vitamins C and D are essential for the immune system. Vitamin C takes part in the development and functionality of various immune cells and the production of antibodies. The contribution of vitamin C in immune response has been suggested due to the enhancement of different cellular functions of innate and adaptive immunity. Vitamin C enhances the function of epithelial barrier against pathogens and stimulates skin scavenging activity to protect against the environmental oxidative stress. In addition, it could accumulate in neutrophils to promote chemotaxis phagocytosis and with subsequent microbial killing. It is also required for apoptosis and neutrophil clearance from the infection sites, which resulted in a reduction of necrosis and possible tissue damage. In B and T lymphocytes, vitamin C might promote the cellular differentiation and proliferation due to its gene-regulating activities. Therefore, the deficiency of vitamin C may result in immunity impairment and increased susceptibility to infections. Therefore, infections may have a significant effect on level of vitamin C because of inflammation enhancement. Interestingly, vitamin C supplementation seems to be able to prevent and treat the respiratory and systemic infections (35) (Figure 2).
The function of immune cells is also affected by vitamin D receptors, which indicates that this vitamin deeply influences the immune response to infections. The deficiency of vitamin D, mostly caused by insufficient intake, could result in weak resistance to infections and a higher incidence of various viral diseases. Concerning the COVID-19 pandemic, Ghavideldarestani et al. (36) reported that vitamin D deficiency may have resulted in increased lung injury risk, acute respiratory distress syndrome, diabetes, and cardiovascular symptoms, which are the major risks in COVID-19 patients. The protective role of vitamin D is via enhancement of both innate and adaptive immunity and blocking of the renin–angiotensin system (RAS). Therefore, supplementation of vitamin D could boost the immunity against COVID-19 with subsequent reduction of disease severity in vitamin D-deficient patients. In addition, Panfili et al. (37) reviewed the role of vitamin D supplementation for COVID-19 patients; they concluded that vitamin D is able to interact with innate immune system via activating the Toll-like receptors (TLRs) or upregulating the cathelicidin and β-defensin levels. However, it may interact with adaptive immunity through reduction of immunoglobulin secretion from plasma cells and production of pro-inflammatory cytokines, which alter the function of T cells (Figure 3). The goal should be to raise 25(OH)D concentrations above 40–60 ng/ml (100–150 nmol/L). For treatment of people who become infected with COVID-19, higher vitamin D3 doses might be useful (33).
Concerning the global deficiency of vitamin D, particularly in the Northern Hemisphere populations during the winter, dietary vitamin D supplementation (5,000 IU daily) could have positive outcomes for high-risk groups of individuals (diabetes and obesity), but also during quarantine (38). The occurrence of adverse effects is rare in vitamin D, and modestly high amounts, 2,000–5,000 IU daily, can be consumed for an extended period (39). High amounts of vitamins C and E were found to be ineffective in increasing the immune response, except for vitamin E for viral hepatitis (40, 41). Before the dietary supplementation of vitamin D, it is important to test for possible deficiencies of micronutrients. According to local guidelines, insufficient patients must administer vitamin D at therapeutic doses (39). Other vitamin deficiencies have to be also treated. In patients with infections caused by viruses, the MVM (1 × RDI) supplementation has been suggested (29). In several countries in South Asia, the deficiency of micronutrients, especially vitamin D and B12, has been reported (42).
Such defects take place even in high-income countries and are the most common among children and infants, adolescents, the elderly, and during lactation and pregnancy (43). Groups of people with limited diets, such as vegetarians, food allergies, and chronic diseases, are very susceptible to deficiencies of micronutrients (44). To enhance immunity and optimize the nutritional status, it is recommended to consume MVM supplementation daily (29). The immune functions and mechanisms of vitamins are summarized in Table 1.
The Role of Minerals
Minerals are crucial for a potent immune response, and improving the mineral status of the body could be very efficient in boosting the immune system in the defense against the COVID-19 infection (67). The immune functions and mechanisms of minerals are displayed in Table 2. Many studies have proven that many microelements, especially zinc, intensify the immune response (78). Zinc regulates the signaling pathways in the cells of both specific and non-specific immunity (79). Disorders in the homeostasis of zinc alter the immune response in several ways, resulting in abnormal lymphopoiesis, disturbed intercellular cytokine signaling, and weakening the innate immune response through phagocytosis and oxidative burst (80). Zinc deficiency is a very common disorder, and almost 1/5 of the global population is at risk (81). The lack of zinc impedes the immune response, reduces pathogenic resistance, and extends the duration and incidence of pneumonia (82). In the elderly population, dietary supplementation of zinc increases the IL-2 and IL-2R-α mRNA expressions in peripheral blood mononuclear cells (PBMCs) (83). Recent studies showed that zinc affects the polarization of CD4+ T cells on behalf of Th1. In contrast, the up-regulation of IL-12 signaling and transcription factor T-bet activity leads to an increase of IFN-γ (84). Barnett et al. (82) investigated the effects of dietary supplementation with zinc (30 mg/day for 3 months). They reported an increase in zinc concentrations in serum, which was associated with peripheral T cell numbers (85). High intracellular concentrations of zinc with zinc ionophores such as pyrithione can harm the replications of several RNA viruses (86). Concerning the global crisis of the COVID-19 pandemic, the use of zinc as possible supportive treatment in COVID-19 patients could be attributed to its immune enhancement effect and direct antiviral activity (87). In particular, Zn2+ cations combined with Zn ionophore pyrithione have a role in inhibition of SARS-coronavirus RNA polymerase (RNA-dependent RNA polymerase, RdRp) activity via reduction of viral replication (86). Interestingly, recent trials concluded the antiviral activity of chloroquine against COVID-19 (88), where they suggested that chloroquine has increased flux of zinc into the cell (89). In continuous context, it was suggested that increased concentration of zinc inside cells by chloroquine could enhance the antiviral effect of zinc against SARS-CoV-2 (90). Similarly, supplementation of zinc without chloroquine may have similar desired effects without the deleterious side effects of chloroquine (91). Another theory for COVID-19 treatment includes targeting Zn ions in the viral protein structure; for instance, in MERS-CoV and SARS-CoV, the antiviral drug disulfiram induced release of zinc from papain-like protease leading to destabilization of viral protein (92). Based on such findings, dietary supplementation with zinc could have positive effects not only on the relief of symptoms related to COVID-19 but also on the virus itself (87).
Another risk factor that can cause acute infections in the respiratory system is iron deficiency (87). Both hosts and pathogens use iron. Iron deficiency can weaken the immune system, while excessive amounts of iron can cause oxidative stress that promotes mutations of viruses (93). Hemoglobinopathy, hypoxia, and cell iron overload might have a potential additional role. Scientific reports have stated two possible pathophysiological mechanisms: (i) severe acute respiratory syndrome–coronavirus-2 interaction with hemoglobin molecule, through CD26, CD147, and other receptors located on erythrocyte and/or blood cell precursors; (ii) hepcidin-mimetic action of a viral spike protein, inducing ferroportin blockage (94). Selenium has essential roles in the immune response, mostly by incorporating it into selenoproteins (95). Selenium may have a significant place in COVID-19 management, particularly in vulnerable elderly, and might represent a game changer in the global response to COVID-19. Nuclear factor kappaB (NF-kB) signaling pathway plays a role in COVID-19 progression and selenium is a NF-kB inhibitor. Selenium might reduce the effect of SARS-CoV-2 on vascular endothelial cells and aggregation of platelets (96). Supplementing COVID-19-affected patients with selenium might be an efficient way to treat and prevent the novel coronavirus (97). The synergistic use of selenium with saponins from ginseng stem–leaf could activate the immune responses to a live bivalent vaccine of infectious bronchitis coronavirus in chickens (98). The dietary supplementations of selenium could represent an effective method for treating the novel virus of COVID-19 (87). Regarding the aforementioned effects of zinc and selenium, we suggest that dietary supplementation with these two microelements may improve the immune response to viral infections. The suggested dosage for zinc is 20 mg per day while that for selenium is 50 μg per day (4, 11, 99).
Phosphorus (P) is an essential constituent of many important primary cell metabolites such as nucleic acids, phospholipids, lipopolysaccharides, and different cytoplasmic solutes (100–102). P has been suggested in various studies with different species to perform an important role in the immune system and the modulation of P level in the diet could modulate the function and migration of immune cells (100, 101). Similarly, Ca is a normal body constituent and is critical for the immune responses (102).
Calcium phosphate (CaP) in the form of microparticle or nanoparticle has been used as potential adjuvants or vaccine carrier (delivery system) for DNA and peptide vaccines for humans and mammals. CaP nanoparticles have a number of advantages over other inorganic particles. Their biodegradability and biocompatibility are excellent (103); they are native to the body, well-tolerated and absorbed in the body, non-toxic, cost-effective, and easily manufactured and have a high affinity to protein, DNA, antigens, and chemotherapy drugs (104). Calcium phosphate has been used for many years as the adjuvant for diphtheria–tetanus–pertussis (DTP) vaccine and other vaccines (105). CaP nanoparticles have been used to couple several viruses like Epstein–Barr virus and Herpes simplex virus-2, a potent mucosal adjuvant, and induce both mucosal and cell-mediated immunity to nucleotide vaccines in humans and were used in glycoprotein vaccine (106, 107). Additionally, CaP nanoparticles have also been reported to possess better stimulation of both innate and adaptive immunity in mice (108).
The Role of Probiotics
The utilization of probiotics has been determined to be very beneficial for improving immunity. Lactobacillus-based probiotics are recommended for the prevention of influenza-like viral infections (109, 110). Those patients who had been positively tested for COVID-19 have to undergo a validated screening test for malnutrition (e.g., NRS-2002) (111). Probiotics have important roles in the immune response through the regulation of immune cells in the mucosa and epithelial cells of the intestines (112). However, not every type of probiotics exhibits the same effects in the organism, and to obtain the optimal immunostimulatory results, probiotics should be selected thoughtfully based on the clinical state of the disease (113). Lactobacillus-based probiotics have been reported to have beneficial effects in viral infection, and they may be used along with proper energy and nutritional intake (109, 114). A small case series from China revealed that some patients with COVID-19 showed microbial dysbiosis with decreased Lactobacillus and Bifidobacterium (115). Mak et al. (116) stated that the rationale for using probiotics in COVID-19 is derived from indirect evidence. Blind use of conventional probiotics for COVID-19 is not suggested until we have more understanding of the pathogenesis of SARS-CoV-2 and its impact on the gut microbiota. It is likely that a novel and more targeted approach to modulation of the gut microbiota as one of the therapeutic approaches of COVID-19 and its comorbidities will be required.
The Role of Dietary Guidelines
In addition to hygienic measures and lifestyle behaviors, dietary guidelines are crucial in preventing and treating viral diseases, such as COVID-19. Every patient should follow the dietary recommendations given by the governing bodies (117). The majority of the guidelines state that the diets should predominately consist of fruits, vegetables, and starchy carbohydrates. The diet should consist of meat or a protein-rich equivalent for vegetarians, at least two portions per day (118). Due to the possible economic and logistical difficulties regarding such protein diets, the supplementation with MVM should be mandatory during the pandemic period. In particular, malnourished individuals and those belonging to risk groups should continuously monitor their nutrient status and should be very careful when composing meals (119). It would be advisable to consult a nutritionist regarding the diets, but due to the current socio-economic situation, this could be difficult.
The Role of Protein-Rich Foods
Protein-rich foods such as meat, milk, egg, bioactive peptides, and others are important for enhancing the immune systems and the body health. Proteins represent the framework of body cells, defense system, hormones, and enzymes that control the functions of the different body systems (120). Proteins are important nutriments, and their chemical structure is composed mainly of nitrogen, hydrogen, carbon, and oxygen. Protein metabolism has been reported to have important functions in formation of both natural and acquired immunities against different infectious agents. The idea that deficiency of proteins could decrease immune functions may be due to the deficiency of essential amino acids connected with the regulation of the immune system (121). Therefore, some amino acids with beneficial immune modulatory effects have been used for fortifying foods such as “immunity regulators” to enhance the immune system functions (122).
Arginine could be act as a vital regulator of immune system when taken with some cofactors like vitamin B. It could stimulate the secretion of growth hormone by pituitary gland, increase T cell production through enlargement of thymus gland, increase the healing activity of the body, and help cancer prevention (123).
Glutamine is an important amino acid that acts as an oxidative fuel for cell proliferation (blood, intestinal, cancer cells, and others) and rapidly replicating cells such as mucosal cells of gastrointestinal tract, colonocytes, enterocytes, macrophages, and lymphocytes. It is a glutathione precursor and could help in regulation of acid–base balance and transportation of nitrogen between body organs. It was found to increase the number of CD8+, CD4+, and T lymphocytes after bone marrow transplantation (124, 125). Other amino acids such as aspartate, glutamate, and arginine cannot be used as substitution for glutamine for supporting the proliferation of lymphocytes (126, 127).
Supplementary nucleotides have been reported to influence a considerable number of immune functions such as enhancing the maturation of T cells and the activity of natural killer cells. Nucleotides could also improve the delayed hypersensitivity in skin, reverse the immunosuppression induced by starvation and malnutrition, increase the resistance against some infections such as Candida albicans and Staphylococcus aureus, accelerate the immune responses to vaccines, and increase the titers of antibodies (128–130). Additionally, nucleotides could be included in infant formulas to enhance the function of their immune systems (122).
Fish is a rich source of omega-3 fatty acids, such as docosahexaenoic acid (DHA), which is essential for the immune system. Alagawany et al. (131) illustrated that omega-3 fatty acids are important nutritional factors that modulate immune functions. Therefore, it makes sense to consider the use of n-3 PUFAs for clinical management of COVID-19 patients (132). Nutraceuticals in various combinations and forms have been proven to be very useful in the enhancement of the immune system during infections caused by viruses. Several nutraceuticals have immunostimulatory effects, and among them, fish oil, garlic, cranberries, and broccoli are practical and accessible options (133–136). On the contrary, Rogero et al. (137) reported that although the inflammatory resolution improved by eicosapentaenoic acid (EPA) and DHA could contribute to the recovery of patients infected with SARS-CoV-2, omega-3 fatty acid supplementation cannot be recommended before randomized controlled trials are carried out.
The diets of patients under intensive care should also be recommended by a dietician/nutritionist. Besides, to maintain the homeostasis of nutrient intake, some patients will require an oral nutrition supplement (ONS). During viral infections, the resting energy expenditure was elevated by 10%, and due to such circumstances, the energy intake has to be risen by 10% throughout the disease progression (138). Diabetes patients, as members of the risk group, could have severe disease progress and higher mortality (22), and consequently, they should also have specific diet programs and should be monitored by a nutritionist (139). Regarding diabetes patients with COVID-19, Gupta et al. recently demonstrated clinical considerations for such patients (140).
Phytogenic Feed Additives
Phytogenic feed additives exert their favorable health improving effects owing to their content of active substances such as polyphenols and secondary metabolites. Polyphenols are found in vegetables, grains, fruits, and green and black tea in different parts of the plant (leaves, roots, flowers, seeds, and fruits) to protect the plant immune system against UV radiation and pests (141–151).
Dietary polyphenols have been reported to have various biological activities including polyphenols, which have immunomodulatory, antioxidant, anti-inflammatory, anti-allergic antimicrobial, ant- mutagenic, and detoxification activities (152). Recent studies showed that polyphenols can modulate the immune functions through binding to cellular receptors and altering the cell pathways, thereby regulating the host immune response (153). For instance, curcumin and resveratrol have been evidenced to inhibit the signaling pathway of nuclear factor-kappa β (NF-κβ) and thereby inhibit the release of the proinflammatory cytokine TNF-α (154). Curcumin has been reported to have a role in activation and proliferation of B and T lymphocytes, increase the antibody titer against some viruses, enhance innate immunity in challenged animals, improve the immune response by increasing leucocyte count and phagocytic activates, and stimulate the function of plasma lysozyme and increased immunoglobulin (146, 155, 156). Curcumin could also improve the functions of T and B lymphocytes, monocytes, heterophils, macrophages, and dendritic cells and reduce the inflammatory responses (157).
Another example of polyphenols is resveratrol, which has been reported to have various biological properties such as immunomodulation, antioxidation, hepatoprotection, anti-inflammation, regulation of lipid metabolism, and others (158–160). Resveratrol could strongly inhibit the generation of ROS in mammalian macrophages and neutrophils, suppress the extracellular and intracellular myeloperoxidase (MPO) activity, and reduce the expression of MPO mRNA in neutrophils (161). Moreover, some polyphenols can modulate the expression of some proinflammatory genes and the production of cytokines and the population of immune cells. Dietary polyphenols can alleviate the inflammatory diseases related to field infections via modulating pathogen recognition receptor-mediated signaling pathways (162).
In addition to the immunomodulatory properties of polyphenol compounds, they also showed effective antioxidant properties. Polyphenols could neutralize the free radicals of both reactive oxygen species (ROS) and reactive nitrogen species (RNS), which can damage the chromosomes and consequently modify the encoded amino acid and the associated biological process (163). Zhong and Zhou (164) similarly reported that exogenous antioxidants, including polyphenols, can act as the initial line of defense in the cell that could protect it from oxidative damage caused by excessive production of free radicals. Among all polyphenols, flavonoids such as naringin and naringenin are the most effective in removing generated free radicals and the injuries caused by them (165).
They exhibited robust antioxidant properties in several in vivo disease models and were noticed to dramatically prevent the xanthine oxidase activity in in vitro studies (166). In general, polyphenols protect the cells from generated free radicals through some action mechanisms such as activation of antioxidant enzymes; scavenging of ROS by participation as electron donor; restriction of hydroxyl radicals (HO·) formation through chelation of transition metals; inhibition of activities of pro-oxidant enzymes such as protein kinase C, xanthine oxidase, and membrane-associated β-nicotin-amide adenine dinucleotide [NAD(P)H] oxidase; alleviation of nitric oxide (NO·) oxidative stress; reduction of α-tocopherol radicals; and enhancing the antioxidant activities of low-molecular antioxidants such as ascorbate and tocopherols by preventing their oxidation (152, 163, 165).
Animal Coronaviruses
Animal modeling for the newly emerged human coronavirus (2019-nCoV/SARS-CoV-19) is necessary to investigate the virus pathogenicity and to perform the preclinical testing of the possible vaccines and antiviral agents. Factors that have to be considered when selecting/inventing an animal model of human coronaviruses have been reviewed (167). Ideally, the animal model should express the same kind of virus receptors, and the receptors should be distributed in similar anatomical locations to those of humans, such that they get infection via a similar route of transmission as do human beings (168). The modeled animals should allow the virus to replicate and disseminate to the organs and tissues as in the infected human. Moreover, the infected modeled animals should develop clinical features/morbidity (pathophysiology and clinical manifestations, i.e., signs and symptoms) that resemble human disease and the symptom severity should correlate with the viral load and demographic conditions in human (elderly and immunocompromised subjects are vulnerable to severe disease and mortality).
Numerous animal prototypes have been developed for SARS and MERS caused by the respective coronaviruses (166, 169). These include several strains of inbred mice (young vs. aged) and mice with targeted immune defect/specific gene knockout (170, 171), Syrian golden hamsters (172, 173), cats, ferrets (174, 175), and non-human primates including macaques and African green monkeys (176). These models may be used in the study of SARS-CoV-19 infections, an immune correlate of protection, the protective efficacy of vaccines, and the effectiveness of new therapeutics.
Coronavirus spike glycoprotein (S1 domain) binds to the cognate receptor, and the S2 domain mediates viral envelope fusion with the cell membrane for the genome release into cytosol (virus uncoating process). Antibodies targeting the spike glycoprotein of coronaviruses play a critical role in inhibiting viral entry and uncoating. They have clinical potential in conferring passive immunity in the coronavirus-infected individuals.
Currently, several monoclonal antibodies (both fully human molecules or humanized-version) that object the S1 receptor-binding domain (RBD) and non-RBD and S2 regions of coronaviruses have been produced and examined in cell cultures to determine the neutralizing capacity of viruses and animal models as well to assess the efficiency in prophylaxis and post-exposure stage (177). Such antibodies can be utilized as reagents to alleviate the generation of therapeutic drugs/antiviral inhibitors and vaccines.
Many compliant cell lines to human coronaviruses, including the epithelial cells of monkeys (Vero-B4 and LLC-MK2), are being utilized in the neutralization assays [inhibition of virally induced cytopathic effects (CPE) by different antibody concentrations or plaque assays] for determining the levels of neutralizing titers of the antibodies. The lung cells of goats, kidney cells of alpaca, and dromedary umbilical cord cells were identified to be permissive for MERS-CoV (178). Moreover, pseudo-typed viral particles [virus-like particles (VLPs)] that display spike protein on the surface from SARS-CoV were capable for entry to transfected or permissive cells that overexpress the receptors of virus (179–181). The pseudo-typed virions/VLPs with a genome coding for reporting systems, like green fluorescent proteins (GFP) or luciferase could be utilized for quantitative measurement and assessment of inhibitors/monoclonal antibodies that inhibit the cellular entry of the coronaviruses (182). The assays using the pseudo-typed virions/VLPs are safe and can be done in a BSL-2 facility, as they do not involve infectious viruses. A safety attention on the passive immunization using intact antibodies is the possibility of the antibody-based enrichment of the virus replication. Antibody molecules with an altered Fc fragment (cannot be fixed to the Fc receptors on host cells) or without Fc fragment, e.g., human single-chain antibodies (scFv), Fab, or F(ab′)2, should be a harmless alternative.
Data from animal coronavirus vaccine development suggested that systemic cell-mediated or humoral immune reactions induced by parenteral management may not be enough to inhibit the virus invasion in the respiratory system (183). Since the mucosa in the respiratory system is the first target in coronavirus (SARS-, MERS-, and 2019-nCoV/SARS-CoV-19) transmission and infection, the mucosal immunization such as using intranasal vaccine (184) may be a beneficial method for prophylaxis by inducing the immune response in the mucosa. There are severe differences between the molecular mechanisms of mucosal and systemic immunological response; therefore, at the time being, it is rather hard to propose the surrogate indicator for protective ability against the coronavirus diseases. The best surrogate assay for prevention, as well as the herd immunity toward the various coronavirus diseases, warrants investigation.
Past Experience, Current Situation, and Future Prospective
Immune systems do great work in fighting foreign cells to protect the body from disease. Of course, when a global pandemic strikes the whole world, humans will feel anxious until they conquer it and be healthy. When the COVID-19 epidemic appeared, all sought to find a vaccine to counter this virus. Given a vaccine if provided, immune systems will need to adapt to the COVID-19-infected body (40, 41). Proper nutrition can help in maintaining immune systems as the frontline of defense. Researchers have believed that the deficiency of a particular nutritional element is implicated in the impaired immune responses (6, 11, 185, 186). Nutrition principles based on using some dietary substances such as trace elements, vitamins, probiotics, and nutraceuticals may be helpful in the possible prevention and management of COVID-19 (187). Control of the COVID-19 outbreak and future epidemics requires global efforts among clinicians, immunologists, nutritionists, researchers, veterinarians, and pharmacists (11, 19, 108, 109). Also, public health awareness should be increased about the role of nutrition in eliminating the virus by boosting the immune system. Finally, better understanding the transmission dynamics, incubation period, and replications of COVID-19, along with finding and developing specific vaccines and therapeutics, will pave the way to end this infection soon (188–190).
The lessons learned from this review clarified the common shared scientific knowledge between human and animal nutrition, and that knowledge gained from animal model experiences can be used as a guide in human experiments. This indicates that vitamins such as vitamins C, E, and D and some micro-minerals such as zinc, iron, and selenium and probiotics are candidates to enhance the immunity to viral infections with its specification for each virus.
In conclusion, the strategies of containment have turned out not to be so effective. Such an approach did not stop the spreading of the virus. Social distancing and hygiene measures were an attempt to inhibit the transmission of the virus to give healthcare workers more time to handle the infected patients and “flatten the curve” to lower the peak and meanwhile buy time as researchers all around the world are trying to find efficient therapeutics. Even though the exact intracellular mechanisms of the immunostimulatory effects of nutraceuticals are not entirely explored, a possible beneficial effect is their anti-oxidative and anti-inflammatory activity. This review paper highlighted numerous favorable outcomes of nutraceutical usage in viral infections for both human and animal application, but based on their composition and extraction methods.
Author Contributions
All authors listed have made a substantial, direct and intellectual contribution to the work, and approved it for publication.
Conflict of Interest
The authors declare that the research was conducted in the absence of any commercial or financial relationships that could be construed as a potential conflict of interest.
Acknowledgments
All authors acknowledge and thank their respective Institutes and Universities.
References
1. Wintergerst ES, Maggini S, Hornig DH. Contribution of selected vitamins and trace elements to immune function. Ann Nutr Metab. (2007) 51:301–23. doi: 10.1159/000107673
2. Patel N, Penkert RR, Jones BG, Sealy RE, Surman, SL, Sun Y, et al. Baseline serum vitamin A and D levels determine benefit of oral vitamin A, D supplements to humoral immune responses following pediatric influenza vaccination. Viruses. (2019) 1:907. doi: 10.3390/v11100907
3. Iovino L, Mazziotta F, Carulli G, Guerrini F, Morganti R, Mazzotti V, et al. High-dose zinc oral supplementation after stem cell transplantation causes an increase of TRECs and CD4+ naïve lymphocytes and prevents TTV reactivation. Leukemia Res. (2018) 70:20–4.doi: 10.1016/j.leukres.2018.04.016
4. Ivory K, Prieto E, Spinks C, Armah CN, Goldson AJ, Dainty JR, et al. Selenium supplementation has beneficial and detrimental effects on immunity to influenza vaccine in older adults. Clinical Nut. (2017) 36:407–15. doi: 10.1016/j.clnu.2015.12.003
5. Mousa HA. Prevention and treatment of influenza, influenza-like illness, andcommon cold by herbal, complementary, and natural therapies. J Evid Based Complementary Altern Med. (2017) 22:166–74. doi: 10.1177/2156587216641831
6. McCarty MF, DiNicolantonio JJ. Nutraceuticals have potential for boosting the type 1 interferon response to RNA viruses, including influenza and coronavirus. Prog Cardiovasc Dis. (2020) 63:383–5. doi: 10.1016/j.pcad.2020.02.007
7. Kang EJ, Kim SY, Hwang IH, Ji YJ. The effect of probiotics on prevention of common cold, a meta-analysis of randomized controlled trial studies. Korean J Fam Med. (2013) 34:2–10. doi: 10.4082/kjfm.2013.34.1.2
8. Curtis LJ, Bernier P, Jeejeebhoy K, Allard J, Duerksen D, Gramlich L, et al. Costs of hospital malnutrition. Clinical Nut. (2017) 36:1391–6. doi: 10.1016/j.clnu.2016.09.009
9. Rytter MJH, Kolte L, Briend A, Friis H, Christensen VB. The immune system in children with malnutrition—a systematic review. PLoS ONE. (2014) 9:e105017. doi: 10.1371/journal.pone.0105017
10. Bhaskaram P. Immunobiology of mild micronutrient deficiencies. Br J Nutr. (2001) 85:S75–80. doi: 10.1079/BJN2000297
11. Calder PC, Carr AC, Gombart AF, Eggersdorfer M. Optimal nutritional status for a well-functioning immune system is an important factor to protect against viral infections. Nutrients. (2020) 12:1181. doi: 10.3390/nu12041181
12. Jayawardena R, Sooriyaarachchi P, Chourdakis M, Jeewandara C, Ranasinghe P. Enhancing immunity in viral infections, with special emphasis on COVID-19: a review. Diabetes Metab Syndrome. (2020) 14:367–82. doi: 10.1016/j.dsx.2020.04.015
13. Leung WK, To KF, Chan PK, Chan HL, Wu AK, Lee N, et al. Enteric involvement of severe acute respiratory syndrome-associated coronavirus infection. Gastroenterology. (2003) 125:1011–7. doi: 10.1016/S0016-5085(03)01215-0
14. To KK, Tsang OT, Yip CCY, Chan KH, Wu TC, Chan JMC, et al. Consistent detection of 2019 novel coronavirus in saliva. Clin Infect Dis. (2020) 71:841–3. doi: 10.1093/cid/ciaa149
15. Lu R, Zhao X, Li J, Niu P, Yang B, Wu H, et al. Genomic characterisation and epidemiology of 2019 novel coronavirus, implications for virus origins and receptor binding. Lancet. (2020) 395:565–74. doi: 10.1016/S0140-6736(20)30251-8
16. Gu J, Han B, Wang J. COVID-19: gastrointestinal manifestations and potential fecal–oral transmission. Gastroenterology. (2020) 158:1518–9. doi: 10.1053/j.gastro.2020.02.054
17. Chau TN, Lee KC, Yao H, Tsang TY, Chow TC, Yeung YC, et al. SARS-associated viral hepatitis caused by a novel coronavirus, report of three cases. Hepatology. (2004) 39:302–10. doi: 10.1002/hep.20111
18. Zhou P, Yang XL, Wang XG, Hu B, Zhang L, Zhang W, et al. A pneumonia outbreak associated with a new coronavirus of probable bat origin. Nature. (2020) 579:270–3. doi: 10.1038/s41586-020-2012-7
19. Nasri H, Baradaran A, Shirzad H, Rafieian-Kopaei M. New concepts in nutraceuticals as alternative for pharmaceuticals. Int J Prev Med. (2014) 5:1487–99.
20. Chen YH, Chang GK, Kuo SM, Huang SY, Hu IC, Lo YL, et al. Well-tolerated Spirulina extract inhibits influenza virus replication and reduces virus-induced mortality. Sci Rep. (2016) 6:24253. doi: 10.1038/srep24253
21. Milner JJ, Beck MA. The impact of obesity on the immune response to infection. Proc Nut. Soc. (2012) 71:298–6. doi: 10.1017/S0029665112000158
22. Huang C, Wang Y, Li X, Ren L, Zhao J, Hu Y, et al. Clinical features of patients infected with 2019 novel coronavirus in Wuhan, China. Lancet. (2020) 395:497–506. doi: 10.1016/S0140-6736(20)30183-5
23. Green WD, Beck MA. Obesity impairs the adaptive immune response to influenza virus. Ann Am Thorac Soc. (2017) 14:S406–9. doi: 10.1513/AnnalsATS.201706-447AW
24. Abd El-Kader SM, Al-Jiffri OH. Impact of weight reduction on selected immune system response among Hepatitis C virus Saudi patients. Afr Health Sci. (2018) 18:417–27. doi: 10.4314/ahs.v18i2.27
25. Martin SA, Pence BD, Woods JA. Exercise and respiratory tract viral infections. Exe Sport Sci Rev. (2009) 37:157–64. doi: 10.1097/JES.0b013e3181b7b57b
26. Morey JN, Boggero IA, Scott AB, Segerstrom SC. Current directions in stress and human immune function. Curr Opin Psychol. (2015) 5:13–7. doi: 10.1016/j.copsyc.2015.03.007
27. Bouvier NM, Lowen AC. Animal models for influenza virus pathogenesis and transmission. Viruses. (2010) 2:1530–63. doi: 10.3390/v20801530
28. Bodewes R, Geelhoed-Mieras MM, Wrammert J, Ahmed R, Wilson PC, Fouchier RA, et al. In vitro assessment of the immunological significance of a human monoclonal antibody directed to the influenza a virus nucleoprotein. Cli Vac Imm. CVI. (2013) 20:1333–7. doi: 10.1128/CVI.00339-13
29. Ward E. Addressing nutritional gaps with multivitamin and mineral supplements. Nut J. (2014) 13:72. doi: 10.1186/1475-2891-13-72
30. Santos MS, Lichtenstein AH, Leka LS, Goldin B, Schaefer EJ, Meydani SN. Immunological effects of low-fat diets with and without weight loss. J Am Coll Nutr. (2003) 22:174–82. doi: 10.1080/07315724.2003.10719291
31. IDF. COVID-19 and Diabetes 2020 9/4/2020. Available online at: https//www.idf.org/aboutdiabetes/what-is-diabetes/covid-19-and-diabetes.html (accessed April 11, 2020).
32. Miller ER, Pastor-Barriuso R, Dalal D, Riemersma RA, Appel LJ, Guallar E. Meta-analysis, high-dosage vitamin E supplementation may increase all-cause mortality. Ann Intern Med. (2005) 142:37–46. doi: 10.7326/0003-4819-142-1-200501040-00110
33. Grant WB, Lahore H, McDonnell SL, Baggerly CA, French CB, Aliano JL, et al. Evidence that vitamin D supplementation could reduce risk of influenza and COVID-19 infections and deaths. Nutrients. (2020) 12:988. doi: 10.3390/nu12040988
34. Cheng RZ. Can early and high intravenous dose of vitamin C prevent and treat coronavirus disease 2019 (COVID-19)? Med Drug Discov. (2020) 5:100028. doi: 10.1016/j.medidd.2020.100028
35. Carr AC, Maggini S. Vitamin C and immune function. Nutrients. (2017) 9:1211. doi: 10.3390/nu9111211
36. Ghavideldarestani M, Honardoost M, Khamseh ME. Role of vitamin D in pathogenesis and severity of COVID-19 infection. Preprints. (2020). doi: 10.20944/preprints202004.0355.v1. [Epub ahead of print].
37. Panfili FM, Roversi M, D'Argenio P, Rossi P, Cappa M, Fintini D. Possible role of vitamin D in Covid-19 infection in pediatric population. J Endocrinol Investig. (2020) 15:1–9. doi: 10.1007/s40618-020-01327-0
39. Marcinowska-Suchowierska E, Kupisz-Urbańska M, Łukaszkiewicz J, Płudowski P, Jones G. Vitamin D toxicity-A clinical perspective. Front Endoc. (2018) 9:550. doi: 10.3389/fendo.2018.00550
40. Hemila H, Chalker E. Vitamin C for preventing and treating the common cold. Cochrane Database Syst Rev. (2013) 1:CD000980. doi: 10.1002/14651858.CD000980.pub4
41. Graat JM, Schouten EG, Kok FJ. Effect of daily vitamin E and multivitamin-mineral supplementation on acute respiratory tract infections in elderly persons: a randomized controlled trial. JAMA. (2002) 288:715–21. doi: 10.1001/jama.288.6.715
42. Gopalan HS, Misra A, Jayawardena R. Nutrition and diabetes in South Asia. Eur J Clin Nut. (2018) 72:1267–73. doi: 10.1038/s41430-018-0219-6
43. Bruins MJ, Bird JK, Aebischer CP, Eggersdorfer M. Considerations for secondary prevention of nutritional deficiencies in high-risk groups in high-income countries. Nutrients. (2018) 10:47. doi: 10.3390/nu10010047
44. Kirby M, Danner E. Nutritional deficiencies in children on restricted diets. Pediatr Clin North Am. (2009) 56:1085–103. doi: 10.1016/j.pcl.2009.07.003
45. Haryanto B, Suksmasari T, Wintergerst E, Maggini S. Multivitamin supplementation supports immune function and ameliorates conditions triggered by reduced air quality. Vitam Miner. (2015) 4:1–15. doi: 10.4172/2376-1318.1000128
46. Micronutrient Information Center. Immunity in Depth. Linus Pauling Institute. (2016). Available online at: http://lpi.oregonstate.edu/mic/health-disease/immunity (accessed May 10, 2019).
47. Maggini S, Beveridge S, Sorbara JP, Senatore G. Feeding the immune system: the role of micronutrients in restoring resistance to infections. CAB Rev. (2008) 3:1–21. doi: 10.1079/PAVSNNR20083098
48. Biesalski HK. Nutrition meets the microbiome: micronutrients and the microbiota. Ann N Y Acad Sci. (2016) 1372:53–64. doi: 10.1111/nyas.13145
49. Levy M, Thaiss CA, Elinav E. Metabolites: messengers between the microbiota and the immune system. Genes Dev. (2016) 30:1589–97. doi: 10.1101/gad.284091.116
50. Sirisinha S. The pleiotropic role of vitamin A in regulating mucosal immunity. Asian Pac J Allergy Immunol. (2015) 33:71–89.
51. Chew BP, Park JS. Carotenoid action on the immune response. J Nutr. (2004) 134:257S−61S. doi: 10.1093/jn/134.1.257S
52. Yin Z, Pintea V, Lin Y, Hammock BD, Watsky MA. Vitamin D enhances corneal epithelial barrier function. Investig Ophthalmol Vis Sci. (2011) 52:7359–64. doi: 10.1167/iovs.11-7605
53. Wu D, Lewis ED, Pae M, Meydani SN. Nutritional modulation of immune function: analysis of evidence, mechanisms, and clinical relevance. Front Immunol. (2019) 9:3160. doi: 10.3389/fimmu.2018.03160
54. Saeed F, Nadeem M, Ahmed R, Nadeem M, Arshad M, Ullah A. Studying the impact of nutritional immunology underlying the modulation of immune responses by nutritional compounds—a review. Food Agric Immunol. (2016) 27:205–29. doi: 10.1080/09540105.2015.1079600
55. Sakakeeny L, Roubeno R, Obin M, Fontes JD, Benjamin EJ, Bujanover Y, et al. Plasma pyridoxal-5-phosphate is inverselyassociatedwith systemicmarkers of inflammation in a population of US adults. J Nutr. (2012) 142:1280–15. doi: 10.3945/jn.111.153056
56. Ueland PM, McCann A, Midttun O, Ulvik A. Inflammation, vitamin B6 and related pathways. Mol Asp Med. (2017) 53:10–27. doi: 10.1016/j.mam.2016.08.001
57. Bozonet SM, Carr AC. The role of physiological vitamin C Concentrations on key functions ofneutrophils isolated from healthy individuals. Nutrients. (2019) 11:1363. doi: 10.3390/nu11061363
58. Wintergerst E, Maggini S, Hornig D. Immune-enhancing role of vitamin C and zinc and effect on clinical conditions. Ann Nutr Metab. (2006) 50:85–94. doi: 10.1159/000090495
59. Micronutrient Information Center. Immunity in Depth. Linus Pauling Institute. (2016). Available online at: http://lpi.oregonstate.edu/mic/health-disease/immunity (accessed May 10, 2019).
60. Clark A, Mach N. Role of vitamin D in the hygiene hypothesis: the interplay between Vitamin D, Vitamin D receptors, gut microbiota, and immune response. Front Immunol. (2016) 7:627. doi: 10.3389/fimmu.2016.00627
61. Gombart AF. The vitamin D–antimicrobial peptide pathway and its role in protection against infection. Future Microbiol. (2009) 4:1151. doi: 10.2217/fmb.09.87
62. Clairmont A, Tessman D, Stock A, Nicolai S, Stahl W, Sies H. Induction of gap junctional intercellularcommunication by vitamin D in human skin fibroblasts is dependent on the nuclear Induction of gap junctional intercellular communication by vitamin D in human skin fibroblasts is dependent on the nuclearvitamin D receptor. Carcinogenesis. (1996) 17:1389–91. doi: 10.1093/carcin/17.6.1389
63. Palmer HG, Gonzalez-Sancho JM, Espada J, Berciano MT, Puig I, Baulida J, et al. Vitamin D3 promotes the di erentiation of colon carcinoma cells by the induction of E-cadherin and the inhibition of beta-catenin signaling. J Cell Biol. (2001) 154:369–87. doi: 10.1083/jcb.200102028
64. Mihajlovic M, Fedecostante M, Oost MJ, Steenhuis SKP, Lentjes E, Maitimu-Smeele I, et al. Role of Vitamin D in maintaining renal epithelial barrier function in uremic conditions. Int J Mol Sci. (2017) 18:2531. doi: 10.3390/ijms18122531
65. Lee GY, Han SN. The role of Vitamin E in immunity. Nutrients. (2018) 10:1614. doi: 10.3390/nu10111614
66. Han SN, Adolfsson O, Lee CK, Prolla TA, Ordovas J, Meydani SN. Vitamin E and gene expression in immune cells. Ann N Y Acad Sci. (2004) 1031:96–101. doi: 10.1196/annals.1331.010
67. Khedmat L. New coronavirus (2019-nCoV) An insight toward preventive actions and natural medicine. Int J Travel Med Glob Health. (2020) 8:44–5. doi: 10.34172/ijtmgh.2020.07
68. Wessels I, Rink L. Micronutrients in autoimmune diseases: possible therapeutic benefits of zinc and vitamin D. J Nutr Biochem. (2019) 77:108240. doi: 10.1016/j.jnutbio.2019.108240
69. Besold AN, Culbertson EM, Culotta VC. The Yin and Yang of copper during infection. J Biol Inorg Chem. (2016) 21:137–44. doi: 10.1007/s00775-016-1335-1
70. Attia YA, Abd El-Hamid AE, Zeweil HS, Qota EM, Bovera F, Monastra M, et al. Effect of dietary amounts of organic and inorganic Zinc on productive and physiological traits of white peckin ducks. Animal. (2013) 7:695–700. doi: 10.1017/S1751731113000050
71. Beck MA. Trace minerals, immune function, and viral evolution. In: Military Strategies for Sustainment of Nutrition and Immune Function in the Field, Washington, DC: National Academy Press (1999). p. 339.
72. Hurwitz BE, Klaus JR, Llabre MM, Gonzalez A, Lawrence PJ, Maher KJ, et al. Suppression of human immunodeficiency virus type 1 viral load with selenium supplementation: a randomized controlled trial. Arch Intern Med. (2007) 167:148–54. doi: 10.1001/archinte.167.2.148
73. Attia YA, Abdalah AA, Zeweil HS, Bovera F, Tag El-Din AA, Araft MA. Effect of inorganic or organic copper additions on reproductive performance, lipid metabolism and morphology of organs of dual-purpose breeding hens. Archiv Für Geflügelk. (2011) 75:169–78. doi: 10.1080/00071668.2011.650151
74. Attia YA, Abdalah AA, Zeweil HS, Bovera F, Tag El-Din AA, Araft MA. Effect of inorganic or organic selenium supplementation on productive performance, egg quality and some physiological traits of dual purpose breeding hens. Cezh J Animal Sci. (2010) 55:505–19. doi: 10.17221/1702-CJAS
75. Laires MJ, Monteiro C. Exercise, magnesium and immune function. Magnes Res. (2008) 21:92–6. doi: 10.1684/mrh.2008.0136
76. Bussiere FI, Mazur A, Fauquert JL, Labbe A, Rayssiguier Y, Tridon A. High magnesium concentration in vitro decreases human leukocyte activation. Magnes Res. (2002) 15:43–8. 159.
77. Hafez HM, Attia YA. Challenges to the poultry industry: current perspectives and strategic future after the COVID-19 outbreak. Front. Vet. Sci. 7:516. doi: 10.3389/fvets.2020.00516
78. Derbyshire E, Delange J. COVID-19, is there a role for immunonutrition, particularly in the over 65s? BMJ Nut Pre Health. (2020). doi: 10.1136/bmjnph-2020-000071. [Epub ahead of print].
79. Wessels I, Maywald M, Rink L. Zinc as a gatekeeper of immune function. Nutrients. (2017) 9:1286. doi: 10.3390/nu9121286
80. Maares M, Haase H. Zinc and immunity: an essential interrelation. Arch Biochem Biophys. (2016) 611:58–65. doi: 10.1016/j.abb.2016.03.022
81. Wessells KR, Brown KH. Estimating the global prevalence of zinc deficiency, results based on zinc availability in national food supplies and the prevalence of stunting. PLoS ONE. (2012) 7:e50568. doi: 10.1371/journal.pone.0050568
82. Barnett JB, Hamer DH, Meydan SN. Low zinc status, a new risk factor for pneumonia in the elderly? Nut Rev. (2010) 68:30–7. doi: 10.1111/j.1753-4887.2009.00253.x
83. Prasad AS, Bao B, Beck FW, Sarkar FH. Correction of interleukin-2 gene expression by in vitro zinc addition to mononuclear cells from zinc-deficient human subjects, a specific test for zinc deficiency in humans. Transl Res. (2006) 148:325–33. doi: 10.1016/j.trsl.2006.07.008
84. Bao B, Prasad AS, Beck FW, Bao GW, Singh T, Ali S, et al. Intracellular free zinc up-regulates IFN-gamma and T-bet essential for Th1 differentiation in Con-A stimulated HUT-78 cells. Biochem Biophys Res Commun. (2011) 407:703–7. doi: 10.1016/j.bbrc.2011.03.084
85. Barnett JB, Dao MC, Hamer DH, Kandel R, Brandeis G, Wu D, et al. Effect of zinc supplementation on serum zinc concentration and T cell proliferation in nursing home elderly, a randomized, double-blind, placebo-controlled trial. Am J Clin Nut. (2016) 103:942–51. doi: 10.3945/ajcn.115.115188
86. Te Velthuis AJ, van den Worm SH, Sims AC, Baric RS, Snijder EJ, van Hemert MJ. Zn2+ inhibits coronavirus and arterivirus RNA polymerase activity in vitro and zinc ionophores block the replication of these viruses in cell culture. PLoS Pathogens. (2010) 6:e1001176. doi: 10.1371/journal.ppat.1001176
87. Zhang L, Liu Y. Potential interventions for novel coronavirus in China, a systematic review. J Med Virol. (2020) 92:479–90. doi: 10.1002/jmv.25707
88. Wang M, Cao R, Zhang L, Yang X, Liu J, Xu M, et al. Remdesivir and chloroquine effectively inhibit the recently emerged novel coronavirus (2019-nCoV) in vitro. Cell Res. (2020) 30:269–71. doi: 10.1038/s41422-020-0282-0
89. Xue J, Moyer A, Peng B, Wu J, Hannafon BN, Ding WQ. Chloroquine is a zinc ionophore. PLoS ONE. (2014) 9:e1091802014. doi: 10.1371/journal.pone.0109180
90. Skalny AV, Rink L, Ajsuvakova OP, Aschner M, Gritsenko VA, Alekseenko S, et al. Zinc and respiratory tract infections: perspectives for COVID 19 (Review). Int J Mol Med. (2020) 46:17–26. doi: 10.3892/ijmm.2020.4575
91. Guastalegname M, Vallone A. Could chloroquine/hydroxy-chloroquine be harmful in Coronavirus disease 2019 (COVID-19) treatment? Clin Infect Dis. (2020) 71:888–9 doi: 10.1093/cid/ciaa321
92. Lin MH, Moses DC, Hsieh CH, Cheng SC, Chen YH, Sun CY, et al. Disulfiram can inhibit MERS and SARS coro-navirus papain-like proteases via different modes. Antiviral Res. (2018) 150:155–63. doi: 10.1016/j.antiviral.2017.12.015
93. Wessling-Resnick M. Crossing the iron gate, why and how transferrin receptors mediate viral entry. Annu Rev Nutr. (2018) 38:431–58. doi: 10.1146/annurev-nutr-082117-051749
94. Cavezzi A, Troiani E, Corrao S. COVID-19: hemoglobin, iron, and hypoxia beyond inflammation. A narrative review. Clin Pract. (2020)10:1271. doi: 10.4081/cp.2020.1271
95. Hoffmann PR, Berry MJ. The influence of selenium on immune responses. Mol Nutr Food Res. (2008) 52:1273–80. doi: 10.1002/mnfr.200700330
96. Hiffler L, Rakotoambinina B. Selenium and RNA viruses interactions: potential implications for SARS-Cov-2 infection (Covid-19). Front Nutr. (2020) 7. doi: 10.31219/osf.io/vaqz6
97. Guillin OM, Vindry C, Ohlmann T, Chavatte L. Selenium, selenoproteins and viral infection. Nutrients. (2019) 1:2101. doi: 10.3390/nu11092101
98. Ma X, Bi S, Wang Y, Chi X, Hu S. Combined adjuvant effect of ginseng stem-leaf saponins and selenium on immune responses to a live bivalent vaccine of Newcastle disease virus and infectious bronchitis virus in chickens. Poult Sci. (2019) 98:3548–56. doi: 10.3382/ps/pez207
99. Acevedo-Murillo JA, Garcia-Leon ML, Firo-Reyes V, Santiago Cordova JL, Gonzalez-Rodriguez AP, Wong-Chew RM. Zinc supplementation promotes a Th1 response and improves clinical symptoms in fewer hours in children with pneumonia younger than 5 years old. A randomized controlled clinical trial. Front Pediatr. (2019) 7:431. doi: 10.3389/fped.2019.00431
100. Liu N, Ru YJ, Cowieson AJ, Li FD, Cheng XCh. Effects of phytate and phytase on the performance and immune function of broilers fed nutritionally marginal diets. Poult Sci. (2008) 87:1105–11. doi: 10.3382/ps.2007-00517
101. Chen K, Jiang WD, Wu P, Liu Y, Kuang SY, Tang L, et al. Effect of dietary phosphorus deficiency on the growth, immune function and structural integrity of head kidney, spleen and skin in young grass carp (Ctenopharyngodon idella). Fish Shellfish Immunol. (2017) 63:103–26. doi: 10.1016/j.fsi.2017.02.007
102. Chen K, Zhou XQ, Jianga WD, Wu P, Liu Y, Jiang J, et al. Dietary phosphorus deficiency caused alteration of gill immune and physicalbarrier function in the grass carp (Ctenopharyngodon idella) after infectionwith Flavobacterium columnare Aquaculture. (2019) 506:1–13. doi: 10.1016/j.aquaculture.2019.03.018
103. Sokolova VV, Radtke I, Heumann R, Epple M. effective transfection of cells with multi-shell calcium phosphate–DNA nanoparticles. Biomaterials. (2006) 27:3147–53. doi: 10.1016/j.biomaterials.2005.12.030
104. Behera T. Priyabrat Swain-antigen adsorbed calcium phosphate nanoparticles stimulate both innateand adaptive immune response in fish, Labeo rohita H. Cell Immunol. (2011) 271:350–9. doi: 10.1016/j.cellimm.2011.07.015
105. Goto N, Kato H, Maeyama J, Eto K, Yoshihara S. Studies on the toxicities of aluminium hydroxide and calcium phosphate as immunological adjuvants for vaccines. Vaccine. (1993) 11:914–8. doi: 10.1016/0264-410X(93)90377-A
106. He Q, Mitchell AR, Johnson SL, Bartak CW, Morcol T, Bell SJ. Calcium phosphate nanoparticle adjuvant. Clin Diagn Lab Immunol. (2000) 7: 899–903. doi: 10.1128/CDLI.7.6.899-903.2000
107. He Q, Mitchell AR, Morcol T, Bell Steve JD. Calcium phosphate nanoparticles induce mucosal immunity and protection against herpes simplex virus type 2. Clin Diagn Lab Immunol. (2002) 9:1021–4. doi: 10.1128/CDLI.9.5.1021-1024.2002
108. Sokolova V, Knuschke T, Kovtun A, Buer J, Epple M, Maria AW. The use of calcium phosphate nanoparticles encapsulating Toll-like receptor ligands and the antigen hemagglutinin to induce dendritic cell maturation and T cell activation. Biomaterials. (2010) 31:5627–33. doi: 10.1016/j.biomaterials.2010.03.067
109. Berggren A, Ahrén IL, Larsson N, Önning G. Randomised, double-blind and placebo-controlled study using new probiotic lactobacilli for strengthening the body immune defence against viral infections. Eur J Nutr. (2011) 50:203–10. doi: 10.1007/s00394-010-0127-6
110. Boge T, Rémigy M, Vaudaine S, Tanguy J, Bourdet-Sicard R, van Der Werf S. A probiotic fermented dairy drink improves antibody response to influenza vaccination in the elderly in two randomised controlled trials. Vaccine. (2009) 27:5677–84. doi: 10.1016/j.vaccine.2009.06.094
111. Reber E, Gomes F, Vasiloglou MF, Schuetz P, Stanga Z. Nutritional risk screening and assessment. J Clin Med. (2019) 8:1065. doi: 10.3390/jcm8071065
112. Yan F, Polk DB. Probiotics and immune health. Curr Opin Gastroenterol. (2011) 27:496–501. doi: 10.1097/MOG.0b013e32834baa4d
113. Lin JS, Chiu YH, Lin NT, Chu CH, Huang KC, Liao KW, et al. Different effects of probiotic species/strains on infections in preschool children, a double-blind, randomized, controlled study. Vaccine. (2009) 27:1073–9. doi: 10.1016/j.vaccine.2008.11.114
114. de Vrese M, Winkler P, Rautenberg P, Harder T, Noah C, Laue C, et al. Probiotic bacteria reduced duration and severity but not the incidence of common cold episodes in a double blind, randomized, controlled trial. Vaccine. (2006) 24:6670–74. doi: 10.1016/j.vaccine.2006.05.048
115. Xu K, Cai H, Shen Y, Ni Q, Chen Y, Hu S, et al. Management of corona virus disease-19 (COVID-19): the Zhejiang experience. J Zhejiang Univ. (2020) 49:147–57. doi: 10.3785/j.issn.1008-9292.2020.02.02
116. Mak JW, Chan FK, Ng SC. Probiotics and COVID-19: one size does not fit all. Lancet Gastroenterol Hepatol. (2020) 5:644–5. doi: 10.1016/S2468-1253(20)30122-9
117. WHO. Food and Nutrition Tips During Self-Quarantine. Copenhagen: The WHO Regional Office for Europe (WHO/Europe) (2020).
118. BDA. COVID-19 / Coronavirus - Advice for the General Public. (2020). Available online at: https//www.bda.uk.com/resource/covid-19-corona-virus-advice-for-the-general-public.html (accessed March 29, 2020).
119. Alagawany M, Farag MR, Dhama K, Patra A. A review on nutritional significance and health benefits of designer eggs. World's Poultr Sci J. (2018) 74:317–30. doi: 10.1017/S0043933918000041
120. Reddy G, Altaf MD, Naveena BJ, Venkateshwar M, Kumar EV. Amylolytic bacterial lactic acid fermentation—a review. Biotechnol Adv. (2008) 26:22–34. doi: 10.1016/j.biotechadv.2007.07.004
121. Hurley WL, Theil PK. Perspectives on immunoglobulins in colostrum and milk. Nutrients. (2011) 3:442–74. doi: 10.3390/nu3040442
122. Schloerb PR. Immune enhancing diets: products, components, and their rationales. J Parent Enteral Nutr. (2001) 25:S3–7. doi: 10.1177/014860710102500202
123. Ogilvie GK. Nutrition and cancer: exciting advances for 2002. In: Proceedings of 27th WSAVA Congress. Granada (2002).
124. Anderson RM, May RM. Vaccination and herd immunity to infectious diseases. Nature. (1985) 318:323–9. doi: 10.1038/318323a0
125. Kaetzel CS. Cooperativity among secretory IgA, the polymeric immunoglobulin receptor, and the gut microbiota promotes host–microbial mutualism. Immunol Lett. (2014) 162:10–21. doi: 10.1016/j.imlet.2014.05.008
126. Ardawi MSM, Newsholme EA. Glutamine metabolism in lymphocytes of the rat. Biochem J. (1983) 212:835–42. doi: 10.1042/bj2120835
127. Calder PC. Requirement for both glutamine and arginine by proliferating lymphocytes. Proc Nutr Soc. (1995) 54:123A.
131. Alagawany M, Elnesr SS, Farag MR, Abd El-Hack ME, Khafaga AF, Taha AE, et al. Omega-3 and omega-6 fatty acids in poultry nutrition: effect on production performance and health. Animals. (2019) 9:573. doi: 10.3390/ani9080573
132. Torrinhas RS, Calder PC, Lemos GO, Waitzberg DL. Parenteral fish oil, an adjuvant pharmacotherapy for COVID-19? Nutrition. (2020) 2020:110900. doi: 10.1016/j.nut.2020.110900
133. Müller L, Meyer M, Bauer RN, Zhou H, Zhang H, Jones S, et al. Effect of broccoli sprouts and live attenuated influenza virus on peripheral blood natural killer cells, a randomized, double-blind study. PLoS ONE. (2016) 11:e0147742. doi: 10.1371/journal.pone.0147742
134. Nabeshima S, Kashiwagi K, Ajisaka K, Masui S, Takeoka H, Ikematsu H, et al. A randomized, controlled trial comparing traditional herbal medicine and neuraminidase inhibitors in the treatment of seasonal influenza. J Infect Chemother. (2012) 18:534–43. doi: 10.1007/s10156-012-0378-7
135. Nantz MP, Rowe CA, Muller C, Creasy R, Colee J, Khoo C, et al. Consumption of cranberry polyphenols enhances human γδ-T cell proliferation and reduces the number of symptoms associated with colds andinfluenza, a randomized, placebo-controlled intervention study. Nutr J. (2013) 12:161. doi: 10.1186/1475-2891-12-161
136. Thies F, Nebe-von-Caron G, Powell JR, Yaqoob P, Newsholme EA, Calder PC. Dietary supplementation with eicosapentaenoic acid, but not with other long-chain n– 3 or n– 6 polyunsaturated fatty acids, decreases natural killer cell activity in healthy subjects aged> 55 y. Am J Clin Nutr. (2001) 73:539–48. doi: 10.1093/ajcn/73.3.539
137. Rogero MM, Leão MDC, Santana TM, de MB, Pimentel MV, Carlini GC, da Silveira TF, et al. Potential benefits and risks of omega-3 fatty acids supplementation to patients with COVID-19. Free Radic Biol Med. (2020) 156:190–9. doi: 10.1016/j.freeradbiomed.2020.07.005
138. Kosmiski L. Energy expenditure in HIV infection. Am J Clin Nutr. (2011) 94:1677S−82S. doi: 10.3945/ajcn.111.012625
139. Zhou J, Tan J. Diabetes patients with COVID-19 need better blood glucose management in Wuhan, China. Metab Clin Exp. (2020) 107:154216. doi: 10.1016/j.metabol.2020.154216
140. Gupta R, Ghosh A, Singh AK, Misra A. Clinical considerations for patients with diabetes in times of COVID-19 epidemic. Diabetes Metab Syndr. (2020) 14:211–2. doi: 10.1016/j.dsx.2020.03.002
141. Dhama K, Karthik K, Khandia R, Munjal A, Tiwari R, Rana R, et al. Medicinal and therapeutic potential of herbs and plant metabolites / extracts countering viral pathogens - current knowledge and future prospects. Curr Drug Metab. (2018) 19:236–63. doi: 10.2174/1389200219666180129145252
142. Alagawany M, Farag MR, Salah AS, Mahmoud MA. The role of oregano herb and its derivatives as immunomodulators in fish. Rev Aquac. (2020). doi: 10.1111/raq.12453. [Epub ahead of print].
143. Mohamed LA, El-Hindawy MM, Alagawany M, Salah AS, El-Sayed SA. Effect of low- or high-CP diet with cold-pressed oil supplementation on growth, immunity and antioxidant indices of growing quail. J Anim Physiol Anim Nutr. (2019) 3:1380–7. doi: 10.1111/jpn.13121
144. Alagawany M, Nasr M, Al-Abdullatif A, Alhotan RA, Azzam MMM, Reda FM. Impact of dietary cold-pressed chia oil on growth, blood chemistry, hematology, and antioxidant and immunity status of growing Japanese quail. Italian J Anim Sci. (2020) 19:896–904. doi: 10.1080/1828051X.2020.1807420
145. Ismail IE, Alagawany M, Taha AE, Puvača N, Laudadio V, Tufarelli V. Effect of dietary supplementation of garlic powder and phenyl acetic acid on productive performance, blood haematology, immunity and antioxidant status of broiler chickens. Asian Australas J Anim Sci. (2020). doi: 10.5713/ajas.20.0140. [Epub ahead of print].
146. Reda FM, El-Saadony MT, Elnesr SS, Alagawany M, Tufarelli V. Effect of dietary supplementation of biological curcumin nanoparticles on growth and carcass traits, antioxidant status, immunity and caecal microbiota of Japanese quails. Animals. (2020) 10:754. doi: 10.3390/ani10050754
147. Farag MR, Alagawany M. The role of Astragalus membranaceus as immunomodulator in poultry. World Poult Sci J. (2019) 75:43–54. doi: 10.1017/S0043933918000739
148. Tiwari R, Latheef SK, Ahmed I, Iqbal HMN, Bule MH, Dhama K, et al. Herbal immunomodulators, a remedial panacea for the designing and developing effective drugs and medicines: current scenario and future prospects. Curr Drug Metab. (2018) 19:264–301. doi: 10.2174/1389200219666180129125436
149. Abd El-Hack ME, Alagawany M, Farag MR, Tiwari R, Karthik K, Dhama K. Nutritional, healthical and therapeutic efficacy of black cumin (Nigella sativa) in animals, poultry and humans. Int J Pharmacol. (2016) 12:232–48. doi: 10.3923/ijp.2016.232.248
150. Hashemi SR, Davoodi H. Herbal plants and their derivatives as growth and health promoters in animal nutrition. Vet Res Communications. (2011) 35:169–80. doi: 10.1007/s11259-010-9458-2
151. Petti S, Scully C. Polyphenols, oral health and disease: a review. J Dentist. (2009) 37:413–23. doi: 10.1016/j.jdent.2009.02.003
152. Surai P. Polyphenol compounds in the chicken/animal diet: from the past to the future. J Anim Physiol Anim Nutr. (2014) 98:19–31. doi: 10.1111/jpn.12070
153. Sobhani M, Farzaei MH, Kiani S, Khodarahmi R. Immunomodulatory; anti- inflammatory/antioxidant effects of polyphenols: a comparative review on the parental compounds and their metabolites. Food Rev Int. (2020) 1–53. doi: 10.1080/87559129.2020.1717523
154. Liang Y, Zhou J, Ji K, Liu H, Degen A, Zhai M, et al. Protective effect of resveratrol improves systemic inflammation responses in LPS-injected lambs. Animals. (2019) 9:872. doi: 10.3390/ani9110872
155. Yonar ME, Yonar SM, Ispir Ü, Ural MS. Effects of curcumin on haematological values, immunity, antioxidant status and resistance of rainbow trout (Oncorhynchus mykiss) against Aeromonas salmonicida subsp. Achromogenes. Fish Shellfish Immunol. (2019) 89:83–90. doi: 10.1016/j.fsi.2019.03.038
156. Badran AM, Basuony AH, Elsayed AM, Abdel-Moneim A-ME. Effect of dietary curcumin and curcumin nanoparticles supplementation on growth performance, immune response and antioxidant of broilers chickens. Egypt Poult Sci J. (2020) 40:325–43. doi: 10.21608/epsj.2020.81756
157. Srivastava RM, Singh S, Dubey SK, Misra K, Khar A. Immunomodulatory and therapeutic activity of curcumin. Int Immunopharmacol. (2011) 11:331–41. doi: 10.1016/j.intimp.2010.08.014
158. Alagawany M, Farag MR, El-Kholy MS, El-Sayed SAA, Dhama K. Effect of resveratrol, cinnamaldehyde and their combinations on the antioxidant defense system and ATP release of rabbit erythrocytes: in vitro study. Asian J Anim Sci Vet Advances. (2016) 12:1–9. doi: 10.3923/ajava.2017.1.9
159. Farag MR, Alagawany M, Abd El-Hack ME, Tufarelli V. Alleviative effect of some phytochemicals on cyadox-induced oxidative damage in rabbit erythrocytes. Jpn J Vet Res. (2016) 64:171–82. doi: 10.14943/jjvr.64.3.171
160. Jia R, Li Y, Cao L, Du J, Zheng T, Qian H, et al. Antioxidative, anti- inflammatory and hepatoprotective effects of resveratrol on oxidative stress-induced liver damage in tilapia (Oreochromis niloticus). Comp Biochem Physiol Part C Toxicol Pharm. (2019) 215:56–66. doi: 10.1016/j.cbpc.2018.10.002
161. Chang CY, Choi DK, Lee DK, Hong YJ, Park EJ. Resveratrol confers protection against rotenone-induced neurotoxicity by modulating myeloperoxidase levels in glial cells. PLoS ONE. (2013) 8:e60654. doi: 10.1371/journal.pone.0060654
162. Lillehoj HS, Lee KW. Immune modulation of innate immunity as alternatives- to-antibiotics strategies to mitigate the use of drugs in poultry production. Poult Sci. (2012) 91:1286–91. doi: 10.3382/ps.2012-02374
163. Lipiński K, Mazur M, Antoszkiewicz Z, Purwin C. Polyphenols in monogastric nutrition–a review. Ann Anim Sci. (2017) 17:41–58. doi: 10.1515/aoas-2016-0042
164. Zhong RZ, Zhou DW. Oxidative stress and role of natural plant derived antioxidants in animal reproduction. J Integr Agri. (2013) 12:1826–38. doi: 10.1016/S2095-3119(13)60412-8
165. Procházková D, Boušová I, Wilhelmová N. Antioxidant and prooxidant properties of flavonoids. Fitoterapia. (2011) 82:513–23. doi: 10.1016/j.fitote.2011.01.018
166. Cavia-Saiz M, Busto MD, Pilar-Izquierdo MC, Ortega N, Perez-Mateos M, Muñiz P. Antioxidant properties, radical scavenging activity and biomolecule protection capacity of flavonoid naringenin and its glycoside naringin: a comparative study. J Sci Food Agri. (2010) 90:1238–44. doi: 10.1002/jsfa.3959
167. Gretebeck LM, Subbarao K. Animal models for SARS and MERS coronaviruses. Curr Opin Virol. (2015) 13:123–29. doi: 10.1016/j.coviro.2015.06.009
168. Tseng CTK, Huang C, Newman P, Wang N, Narayanan K, Watts DM, et al. Severe acute respiratory syndrome coronavirus infection of mice transgenic for the human Angiotensin-converting enzyme 2 virus receptor. J Virol. (2007) 81:1162–73. doi: 10.1128/JVI.01702-06
169. Zhao X, Guo F, Liu F, Cuconati A, Chang J, Block TM, et al. Interferon induction of IFITM proteins promotes infection by human coronavirus OC43. Proc Natl Acad Sci USA. (2014) 111:6756–61. doi: 10.1073/pnas.1320856111
170. McCray PB, Pewe L, Wohlford-Lenane C, Hickey M, Manzel L, Shi L, et al. Lethal infection of K18-hACE2 mice infected with severe acute respiratory syndrome coronavirus. J Virol. (2007) 8:813–21. doi: 10.1128/JVI.02012-06
171. Frieman M, Yount B, Agnihothram S, Page C, Donaldson E, Roberts A, et al. Molecular determinants of severe acute respiratory syndrome coronavirus pathogenesis and virulence in young and aged mouse models of human disease. J Virol. (2012) 86:884–97. doi: 10.1128/JVI.05957-11
172. Roberts A, Thomas WD, Guarner J, Lamirande EW, Babcock GJ, Greenough TC, et al. Therapy with a severe acute respiratory syndrome–associated coronavirus–neutralizing human monoclonal antibody reduces disease severity and viral burden in golden Syrian Hamsters. J Infect Dis. (2006) 193:685–92. doi: 10.1086/500143
173. Lamirande EW, DeDiego ML, Roberts A, Jackson JP, Alvarez E, Sheahan T, et al. A live attenuated severe acute respiratory syndrome coronavirus is immunogenic and efficacious in golden Syrian hamsters. J Virol. (2008) 82:7721–24. doi: 10.1128/JVI.00304-08
174. Martina BE, Haagmans BL, Kuiken T, Fouchier RA, Rimmelzwaan GF, van Amerongen G, et al. SARS virus infection of cats and ferrets. Nature. (2003) 425:915. doi: 10.1038/425915a
175. Weingartl H, Czub M, Czub S, Neufeld J, Marszal P, Gren J, et al. Immunization with modified vaccinia virus Ankara-based recombinant vaccine against severe acute respiratory syndrome is associated with enhanced hepatitis in ferrets. J Virol. (2004) 78:12672–76. doi: 10.1128/JVI.78.22.12672-12676.2004
176. McAuliffe J, Vogel L, Roberts A, Fahle G, Fischer S, Shieh WJ, et al. Replication of SARS coronavirus administered into the respiratory tract of African Green, rhesus and cynomolgus monkeys. Virology. (2004) 330:8–15. doi: 10.1016/j.virol.2004.09.030
177. Xu J, Jia W, Wang P, Zhang S, Shi X, Wang X, Zhang L. Antibodies and vaccines against Middle East respiratory syndrome coronavirus. Emerg Infect Dis. (2019) 8:841–56. doi: 10.1080/22221751.2019.1624482
178. Eckerle I, Corman VM, Müller MA, Lenk M, Ulrich RG, Drosten C. Replicative capacity of MERS coronavirus in livestock cell lines. Emerg Infect Dis. (2014) 20:276. doi: 10.3201/eid2002.131182
179. Giroglou T, Cinatl J, Rabenau H, Drosten C, Schwalbe H, Doerr HW, et al. Retroviral vectors pseudotyped with severe acute respiratory syndrome coronavirus S protein. J Virolog. (2004) 78:9007–15. doi: 10.1128/JVI.78.17.9007-9015.2004
180. Moore MJ, Dorfman T, Li W, Wong SK, Li Y, Kuhn JH, et al. Retroviruses pseudotyped with the severe acute respiratory syndrome coronavirus spike protein efficiently infect cells expressing angiotensin-converting enzyme 2. J Virol. (2004) 78:10628–35. doi: 10.1128/JVI.78.19.10628-10635.2004
181. Fukushi S, Mizutani T, Saijo M, Matsuyama S, Miyajima N, Taguchi F, et al. Vesicular stomatitis virus pseudotyped with severe acute respiratory syndrome coronavirus spike protein. J Gen Virol. (2005) 86:2269–74. doi: 10.1099/vir.0.80955-0
182. Kilianski A, Baker SC. Cell-based antiviral screening against coronaviruses, developing virus-specific and broad-spectrum inhibitors. Antivir Res. (2014) 101:105–12. doi: 10.1016/j.antiviral.2013.11.004
183. Qu D, Zheng B, Yao X, Guan Y, Yuan ZH, Zhong NS, et al. Intranasal immunization with inactivated SARS-CoV (SARS-associated coronavirus) induced local and serum antibodies in mice. Vaccine. (2005) 23:924–31. doi: 10.1016/j.vaccine.2004.07.031
184. Lee JS, Poo H, Han DP, Hong SP, Kim K, Cho MW, et al. Mucosal immunization with surface-displayed severe acute respiratory syndrome coronavirus spike protein on Lactobacillus casei induces neutralizing antibodies in mice. J Virol. (2006) 80:4079–87. doi: 10.1128/JVI.80.8.4079-4087.2006
185. Attia YA, El-Naggar AS, Abou-Shehema BM, Abdella AA. Effect of supplementation with trimethylglycine (betaine) and/or vitamins on semen quality, fertility, antioxidant status, DNA repair and welfare of roosters exposed to chronic heat stress. Animals. (2019) 9:547. doi: 10.3390/ani9080547
186. Attia, YA, Al-Harthi MA, El-Shafey AS, Rehab YA, Kim WK. Enhancing tolerance of broiler chickens to heat stress by supplementation with vitamin E, vitamin C and/or probiotics. Ann Anim Sci. (2017) 17:1–15. doi: 10.1515/aoas-2017-0012
187. Petrovic J, Stanic D, Dmitrasinovic G, Plecas-Solarovic B, Ignjatovic S, Batinic B, et al. Magnesium supplementation diminishes peripheral blood lymphocyte DNA oxidative damage in athletes and sedentary young man. Oxidat Med Cell Longev. (2016) 2016:2019643. doi: 10.1155/2016/2019643
188. Chen G, Wu D, Guo W, Cao Y, Huang D, Wang H, et al. Clinical and immunologic features in severe and moderate coronavirus disease 2019. J Clin Investig. (2020) 130:2620–9. doi: 10.1101/2020.02.16.20023903
189. Qin C, Zhou L, Hu Z, Zhang S, Yang S, Tao Y, et al. Dysregulation of immune response in patients with coronavirus 2019 (COVID-19) in Wuhan, China. Clin Infect Dis. (2020) 2020:ciaa248. doi: 10.1093/cid/ciaa248
Keywords: COVID-19, SARS—CoV-2, vitamins, minerals, probiotics
Citation: Alagawany M, Attia YA, Farag MR, Elnesr SS, Nagadi SA, Shafi ME, Khafaga AF, Ohran H, Alaqil AA and Abd El-Hack ME (2021) The Strategy of Boosting the Immune System Under the COVID-19 Pandemic. Front. Vet. Sci. 7:570748. doi: 10.3389/fvets.2020.570748
Received: 08 June 2020; Accepted: 24 August 2020;
Published: 08 January 2021.
Edited by:
Xiaolun Sun, University of Arkansas, United StatesReviewed by:
Mohit Bansal, University of Arkansas, United StatesYing Fu, University of Arkansas, United States
Shad Mahfuz, Sylhet Agricultural University, Bangladesh
Copyright © 2021 Alagawany, Attia, Farag, Elnesr, Nagadi, Shafi, Khafaga, Ohran, Alaqil and Abd El-Hack. This is an open-access article distributed under the terms of the Creative Commons Attribution License (CC BY). The use, distribution or reproduction in other forums is permitted, provided the original author(s) and the copyright owner(s) are credited and that the original publication in this journal is cited, in accordance with accepted academic practice. No use, distribution or reproduction is permitted which does not comply with these terms.
*Correspondence: Youssef A. Attia, eWFhdHRpYUBrYXUuZWR1LnNh; Mohamed E. Abd El-Hack, ZHIubW9oYW1lZC5lLmFiZGFsaGFxQGdtYWlsLmNvbQ==