- 1Instituto Superior de Investigaciones Biológicas (INSIBIO), Consejo Nacional de Investigaciones Científicas y Técnicas - Universidad Nacional de Tucumán (CONICET-UNT), and Instituto de Biología “Dr. Francisco D. Barbieri”, Facultad de Bioquímica, Química y Farmacia, UNT, San Miguel de Tucumán, Argentina
- 2Cátedra de Zootecnia General I, Facultad de Agronomía y Zootecnia, UNT, San Miguel de Tucumán, Argentina
- 3Facultad de Ciencias Veterinarias, Universidad Austral de Chile, Valdivia, Chile
- 4Cátedra de Biología Celular y Molecular, Facultad de Bioquímica, Química y Farmacia, UNT, San Miguel de Tucumán, Argentina
One striking reproductive feature in South American camelids is that more than 90% of gestations are established in the left uterine horn (LUH). This phenomenon could be related to a differential vascular irrigation of the LUH. An increase of vascularization in llama endometrium was observed after systemic administration of Beta Nerve Growth Factor (β-NGF), a neurotrophin present in the uterus and placenta of various mammals that is involved in pregnancy development. We hypothesized that the β-NGF signaling pathway is related to embryo implantation in the LUH in camelids. The aim of this study was to characterize the spatial expression of β-NGF and its high-affinity receptor, TrKA, between LUH and right uterine horn (RUH) of non-pregnant (NP) and early pregnant alpacas (15 and 30 days of gestation, 15 and 30P, respectively). In addition, β-NGF, TrKA, and Vascular Endothelium Growth Factor A (VEGFA) temporal gene expression patterns and counting of blood vessels were evaluated among groups. The β-NGF and TrKA were localized in the luminal, glandular, and vascular epithelium of the alpaca uterus and in the embryonic membranes of the 30-days-old conceptus. β-NGF and TrKA immunosignal were stronger in 15P females than that of NP and 30P. In addition, TrKA signal was higher in the LUH luminal epithelium of NP and 15P alpacas than that of NP-RUH and 15P-RUH. β-NGF mRNA relative abundance was higher in the 30P-RUH than that of NP-RUH; whereas TrKA mRNA abundance only differed between 15P-RUH and NP-LUH. VEGFA mRNA relative abundance was higher in NP females compared to the LUH of 15P and 30P alpacas, and lower to their right counterparts. The number of vessels per field was higher in 15P than that of 30P. A positive correlation was observed between the number of vessels per field and β-NGF immunosignal in 15P-LUH. In contrast, the area occupied by vessels was higher in 30P alpacas than of NP and 15P females. The changes of β-NGF/TrKA expression pattern in the peri-implantation endometria between LUH and RUH and their localization in the extraembryonic membranes support the implication of the neurotrophin during implantation and pregnancy development in South American Camelids.
Introduction
In the reproductive physiology of mammals, critical events related to embryo survival occur during early pregnancy, with the majority of embryo losses occurring in the first month of pregnancy (1, 2). In South American camelids (SACs), the pregnancy rate 30 days after mating is <50% (3), indicating that embryo losses are much higher in SACs than in other small ruminants (4). Particularly, in SACs, the embryos formed in the right oviduct must first migrate to the left uterine horn (LUH) to subsequently achieve their successful implantation. In alpacas, embryos reach the uterus on 6 days post ovulation (dpo) and by 9 dpo, 83% of embryos derived from right-ovary ovulations have arrived to the LUH. Maternal recognition of pregnancy occurs between 8 and 10 dpo, conceptus elongation between 10 and 15 dpo, and the apposition and implantation start around 20 dpo (5–7). If an embryo implants in the right uterine horn (RUH) gestation probably would not continue, since RUH would seem to be inadequate to sustain pregnancy (8, 9), (since 98% are carried out in LUH, a 2% do actually get to term in RUH) (10, 11). It has been proposed that this unique pattern of early embryo migration and implantation is influenced by a vascular asymmetry favoring the blood vessels that irrigate the LUH (12). Interestingly, Urra et al. (13) reported an increase in endometrial vascularization in the llama uterus, after the intrauterine administration of beta nerve growth factor (β-NGF).
The β-NGF, a member of the neurotrophin family, would seem to be a key factor for the success of pregnancy since it constitutes a functional link between the nervous, immune, and endocrine systems (14). The multiple effects of β-NGF are mediated through its receptors, the high-affinity membrane receptor tyrosine kinase A (TrkA) and the low-affinity receptor, the nerve growth factor receptor (NGFR or p75NTR) (15–17). The expression of β-NGF and its receptors in a wide variety of reproductive tissues, including different organs of the male and female genital tract, enables it to perform key reproductive functions.
This system is present in the uterus of several species such as rat (18), squirrel (15), ewe (19), horse, pig, human (20), and induced ovulatory species such as rabbits and camelids (17). Several reports suggest that this factor would participate in the endometrial prostaglandin synthesis during the maternal recognition of pregnancy, early development of the embryo, and conceptus immunotolerance (21, 22). Furthermore, in humans and rats, β-NGF has been shown to be a potent angiogenic factor, increasing the expression of vascular endothelial growth factor (VEGF) and promoting vascular cell proliferation in female reproductive organs (23, 24). The VEGFA, a member of the VEGF family, is a marker of uterine receptivity for implantation in humans and bovines. It mediates the establishment of an appropriate uterine environment through the regulation of endothelial cell growth, angiogenesis, and vascular permeability (25).
In camelids, β-NGF is present in the seminal plasma acting as an ovulation-inducing factor (26, 27). The factor is absorbed from the endometrium surface after copulation, rapidly entering systemic circulation to elicit GnRH secretion from the hypothalamus (28). Moreover, the factor increases uterus vessel formation and corpus luteum vascularization. In fact, β-NGF addition to llama cultured granulosa cells increased the expression of VEGFA after 48 h in vitro culture (29).
The mechanisms leading to embryo implantation and survival in SACs is far from being explained. Given this scenario, associated with the reports on the roles of β-NGF in the uterus during pregnancy, the objectives of this study were: (1) Characterize the spatial localization and expression pattern of β-NGF and TrkA in the endometrium of LUH and RUH in alpacas during the first month of gestation. (2) Describe the VEGFA gene expression pattern and count the number of associated blood vessels, and its association with β-NGF expression.
Materials and Methods
Animals and Sampling
Twelve adult female Huacaya breed alpacas (Vicugna pacos), >2 years of age, weighing 65–70 kg and that were destined for meat production, were used in this study. According to their reproductive status, females were divided into 3 experimental groups: non-pregnant (NP; n = 4), 15 days of pregnancy (15P; n = 4), and 1 month of pregnancy (30P; n = 4).
Non-pregnant and 15 days-pregnant alpacas were obtained from the Veterinary Research Center (IVITA), Universidad Nacional Mayor de San Marcos (UNMSM) in the province of Canchis, Perú (14°S, 71°W; 3698 m altitude). Virgin females, between 2 and 3 years of age, were mated once with a fertile adult male and they were slaughtered fifteen days after mating, in line with standards set by the local Committee of Animal Ethics and Welfare (Comité de Ética y Bienestar Animal; CEBA from the School of Veterinary Medicine of the UNMSM). Females with follicles of <7 mm in diameter in their ovaries were considered NP; while, those with a corpus luteum and embryos in the uterus were considered pregnant (Table 1).
Alpacas around 1 month of pregnancy (30P) were obtained from a slaughterhouse at Huancavelica, Perú (12°S, 74°W, 3676 m altitude). The gestational age (GA) of these animals was calculated using embryo crown-rump length (CRL) and the formula GA= (10.328*CRL)0.4427 (Table 1) as it was previously reported (6, 7, 30, 31). Based on previous studies maternal recognition of pregnancy occurs between 8 and 10 dpo and embryo apposition happens around 20 dpo (5, 30). Therefore, 15P samples corresponded to the pre-implantation stage; while in 30P samples the embryo was already implanted in the uterus.
Endometria from the midsection of the LUH and RUH were dissected in two segments of 10 mm, which were subsequently placed in 4% formaldehyde-PBS solution (pH 7.4) for immunohistochemical assays or RNAlater® (Ambion, Austin, USA) for RT-PCR assays. RNAlater®-embedded samples were transported on dry ice to the laboratory at INSIBIO, Argentina and stored at −80°C until further analysis.
RNA Isolation and cDNA Synthesis
Total RNA from endometria was extracted using the Spin Vacuum total RNA Isolation System™ (Promega, Madison, USA) according to the manufacturer's instructions. The RNA samples were quantified at 260 nm on a Shimadzu UV- spectrophotometer 1800 (Shimadzu Corporation, Japan) and its integrity was examined by electrophoresis on 1.5% agarose gels stained with Invitrogen™ SYBR™ Safe DNA Gel Stain (Carlsbad, USA). Finally, total RNA was stored at −80°C.
Reverse transcription of all of the RNA samples was carried out with M-MLV reverse transcriptase™ (Promega) and Oligo(dT)15-primer™ (Promega) in a 25 μl reaction mixture. Reactions were performed by incubating the mixture in a thermocycler (Techne™ TC-512 Gradient Thermal Cycler, Burlington, USA) at 42°C for 90 min followed by a reverse-transcriptase inactivation at 94°C for 5 min.
Semi-quantitative PCR of β-NGF, TrKA, and VEGFA
The relative abundance of β-NGF, TrKA, and VEGFA mRNA was analyzed by semi-quantitative PCR in NP (n = 4), 15P (n = 4), and 30P (n = 4) endometria using ACTB as reference gene as was described by Barraza et al. (32).
PCR amplifications were carried out in a final volume of 10 μl containing 0.5 μL of cDNA, 2 μL of 5X Green GoTaq® Reaction Buffer (Promega), 0.2 mM of dATP, dTTP, dCTP and dGTP (Promega), 2.5 units of GoTaq® DNA polymerase (Promega), and 1 μM of each primer pair: β-NGF (5′-TGCTGGGAGAGGTGAACATT-3′, 5′-CGAAGGTGTGGGTTGTGGTA-3′), TrKA (5′-GCTTCATCTTCACCGAGTTCCT-3, 5′-TAGCCAGCAGCGTGTAGTTG-3′), VEGFA (5′-CGGTATAAATCCTGGAGCGT-3′, 5′-GCCTCGGCTTGTCACATCT-3′) and ACTB (5′-GCGGGACCACCATGTACC-3′, 5′-ACTCCTGCTTGCTGATCCAC-3′). Oligonucleotides were synthetized at Thermo Fisher Scientific Custom Standard DNA Oligos Service (Buenos Aires, Argentina).
Different amplification settings were assayed to determine optimal PCR conditions: 94°C for 3 min, followed by 40 cycles at 94°C for 10 s, 60°C for 5 s, 72°C for 5 s, and a final extension at 72°C for 5 min. PCR products were analyzed with 1.5% agarose gel electrophoresis.
For semi-quantitative expression analysis, the 4 samples of each uterine horn and physiological status were amplified in duplicate (n = 4, r = 2). The amplification products were visualized in agarose gels and documented with a Pentax™Optio™ M90 digital camera (Milan, Italy), and the optical density of PCR products was quantified using ImageJ 1.42q software (National Institutes of Health, Bethesda USA). Data represent the average of the 2 PCR amplifications. The relative abundance of each transcript was normalized against that of ACTB, and the transcript/ACTB ratio was calculated.
Immunohistochemistry
Immune assays were performed as described by Sari et al. (33). Fixed tissues were dehydrated in ethanol, cleared in chloroform, and embedded in paraffin blocks. Five-micron sections were cut and mounted on Biotraza positively charged slides (Huida Medical Instruments CO., Jiangsu, China). After deparaffinization with xylene and rehydration, sections were subjected to antigen retrieval by incubating in Proteinase K (Sigma-Aldrich, St. Louis, USA) solution (10 μg/μL in Tris EDTA (TE) Buffer, 50 mM Tris Base, 1 mM EDTA, 0.5% Triton X-100, pH 8.0) for 20 min at 37°C in a humid chamber, followed by incubation in TE Buffer at room temperature (RT) for 10 min. The slides were then blocked with 1% Bovine Serum Albumin (Sigma-Aldrich) in PBS at room temperature for 30 min. Then, they were incubated at 37°C for 1 h with polyclonal antibodies against β-NGF (dilution 1:1,500, sc-548) or TrKA (dilution 1:100, sc-118, Santa Cruz Biotechnology, Santa Cruz, USA). After washing in 0.02% Tween-PBS, slides were incubated for 20 min at RT in a humidified chamber with a 1:200 dilution of the biotinylated anti-rabbit IgG antibody (B8895, Sigma-Aldrich). Subsequently, samples were incubated at RT for 30 min with a solution 1:500 ExtraAvidin®-Alkaline Phosphatase (Sigma-Aldrich). Following three washes with 0.02% Tween in PBS, the sections were incubated with SIGMAFAST™ BCIP®/NBT (B5655, Sigma-Aldrich) until color was developed.
Finally, the sections (n = 4) were counterstained with Nuclear Fast Red (N3020, Sigma-Aldrich), then dehydrated and mounted with Entellan® (Merck, Darmstadt, Germany). Negative controls were performed by replacing the primary antibody with blocking buffer with 1% BSA-PBS with 0.02% Tween under the same experimental conditions.
Samples were observed under a Leica DM500TM light microscope, and images were captured with a Leica ICC50 TM HD camera using LASZ Leica Inc. Software.
The ImageJ 1.42q software (National Institutes of Health, Bethesda USA) was used to measure the immunolabeled area of each sample according to Jensen (34). For this analysis, three different photographs (100X) of each section were used. Three rectangular regions of interest (ROI) were randomly selected in each image for its evaluation. First, the images were converted to 8 bits, then a specific threshold was determined and quantification was performed. Data were expressed as pixels/μm2.
Histological Evaluation of Blood Vessels
The number and area occupied by vascular vessels were determined as follows: endometrial sections of 5 μm were stained with hematoxylin and eosin (BIOPUR, Rosario, Argentina). Then, images were captured with a DM500TM microscope coupled to a Leica ICC50TM HD camera, and the images were converted to 8 bits. Each vessel was marked in black and ImageJ v1.42q (National Institutes of Health, Bethesda USA) was used to analyze the number of marked vessels/field and the total area of vascularization (35). Nine representative microscopic fields of each uterine horn (n = 4) were randomly selected for all measurements. Data represent the average of the nine measurements at 100X magnification. Data were expressed as a percentage per field of endometrium.
Statistical Analysis
Statistical analysis was performed with InfoStat software (Universidad Nacional de Córdoba, Córdoba, Argentina, see http://infostat.com.ar). Gene relative abundance as well as immunohistochemistry staining were analyzed with One-way Analysis of Variance (ANOVA) followed by Tukey post-hoc test, which is a multiple comparison test. The power of each performed test with α = 0.050 was between 1.0 and 0.8; 0.80 is the lowest desired power value. Less than desired power indicates there are less likely possibilities to detect a difference when one actually exists. On the other hand, the number of vessels and the vascularized area were evaluated using the Kruskal–Wallis test (a non-parametric, distribution free test). Results are expressed as the mean ± standard error (SE). In addition, correlation analysis between the variables was performed for each uterine horn, without discriminating between physiological status. Pearson Correlation Coefficient was calculated. In all the analyses, data were considered statistically significant at p < 0.05.
Results
Relative Abundance of β-NGF and TrKA mRNA in Alpaca Endometrium
The presence of β-NGF and TrKA transcripts was found in the uterine horns of alpacas in both pregnant and non-pregnant females.
The relative abundance of β-NGF showed similar levels between the left and right uterine horns in all the experimental groups. When the physiological status was compared, the RUH of 30P animals displayed higher relative abundance levels than the RUH of NP females, p = 0.008. While β-NGF relative abundance in the LUH presented steady levels in all the groups (Figure 1A).
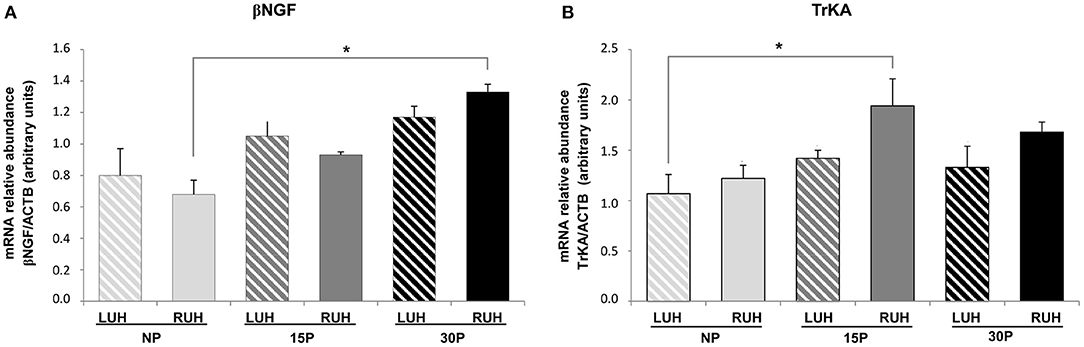
Figure 1. Relative abundance of β-NGF (A) and TrKA (B) transcripts in the endometrium of non-pregnant (NP), 15 days-pregnant (15P), and 30 days-pregnant (30P) alpacas by RT-PCR. Histograms illustrate mean values ± SE. Asterisks denote significant differences (*p < 0.05). LUH (left uterine horn) and RUH (right uterine horn).
Regarding TrKA, transcript relative abundance was higher in the right uterine horn of 15P females compared to NP-LUH, p = 0.025 (Figure 1B).
Immunolocalization of β-NGF and TrKA in Alpaca Endometrium
β-NGF tissue localization and immunolabeling intensity changed during the peri-implantation period; the signal was more intense in the uterine horns of 15P alpacas compared to the uterine horns of NP and 30P females. In non-pregnant females, the β-NGF mark was faint and located in the luminal and glandular epithelium as well as the endothelium of both uterine horns. NP-LUH showed a stronger immunosignal compared to NP-RUH at the luminal epithelium. In 15 day-pregnant alpacas, an intense immunolabel was observed mainly in the luminal epithelium. A mark was also present in the glandular epithelium and the endothelium. No differences were observed between the left and right uterine horns. In 30 day-pregnant animals, β-NGF staining was weak in the endometrium, no differences between LUH and RUH were detected (Figure 2 and Table 2).
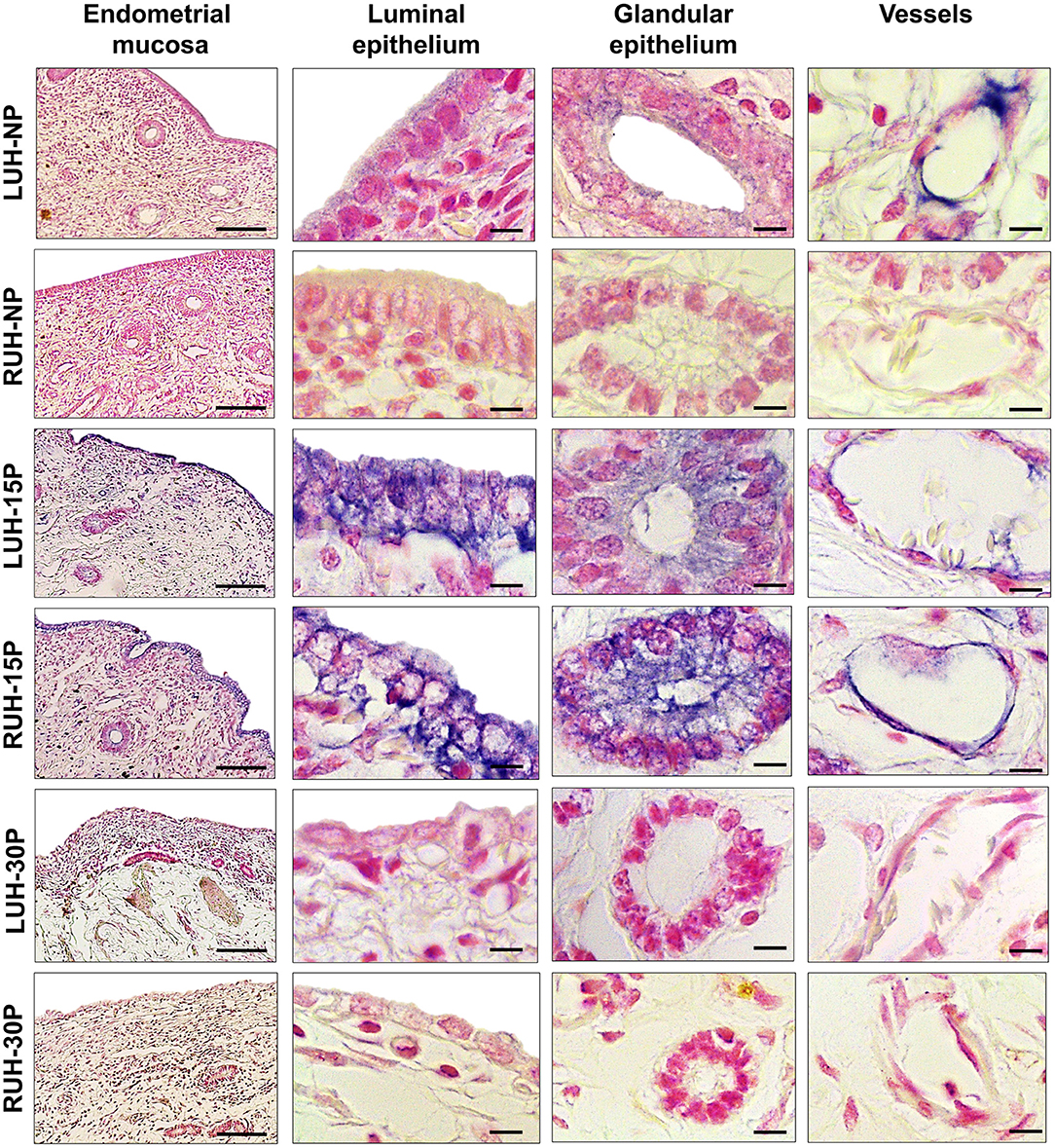
Figure 2. Immunohistochemical localization of β-NGF in the endometrial mucosa of alpacas, positive labeling gives a blue-violaceous coloration. A 10X magnification of endometria of the left uterine horn (LUH) and right uterine horn (RUH) of non-pregnant females (NP); 15 days-pregnant (15P); and 30 days-pregnant females (30P) is presented (scale bar = 100 μm). Also, a 100X magnification of the luminal, glandular, and vascular endothelium of the different physiological status is shown (scale bar = 10 μm).
TrKA mark was co-localized with β-NGF. The receptor immunolabel was detected in the luminal, glandular, and vascular epithelium. The same distribution was observed in pregnant and non-pregnant alpacas and between left and right uterine horns (Figure 3). However, the mark was stronger in the uterine horns of 15P females compared to NP and 30P animals. Statistically significant differences between LUH and RUH were observed only at the luminal epithelium of NP and 15P alpacas, where the signal was more intense in the LUH (Table 3).
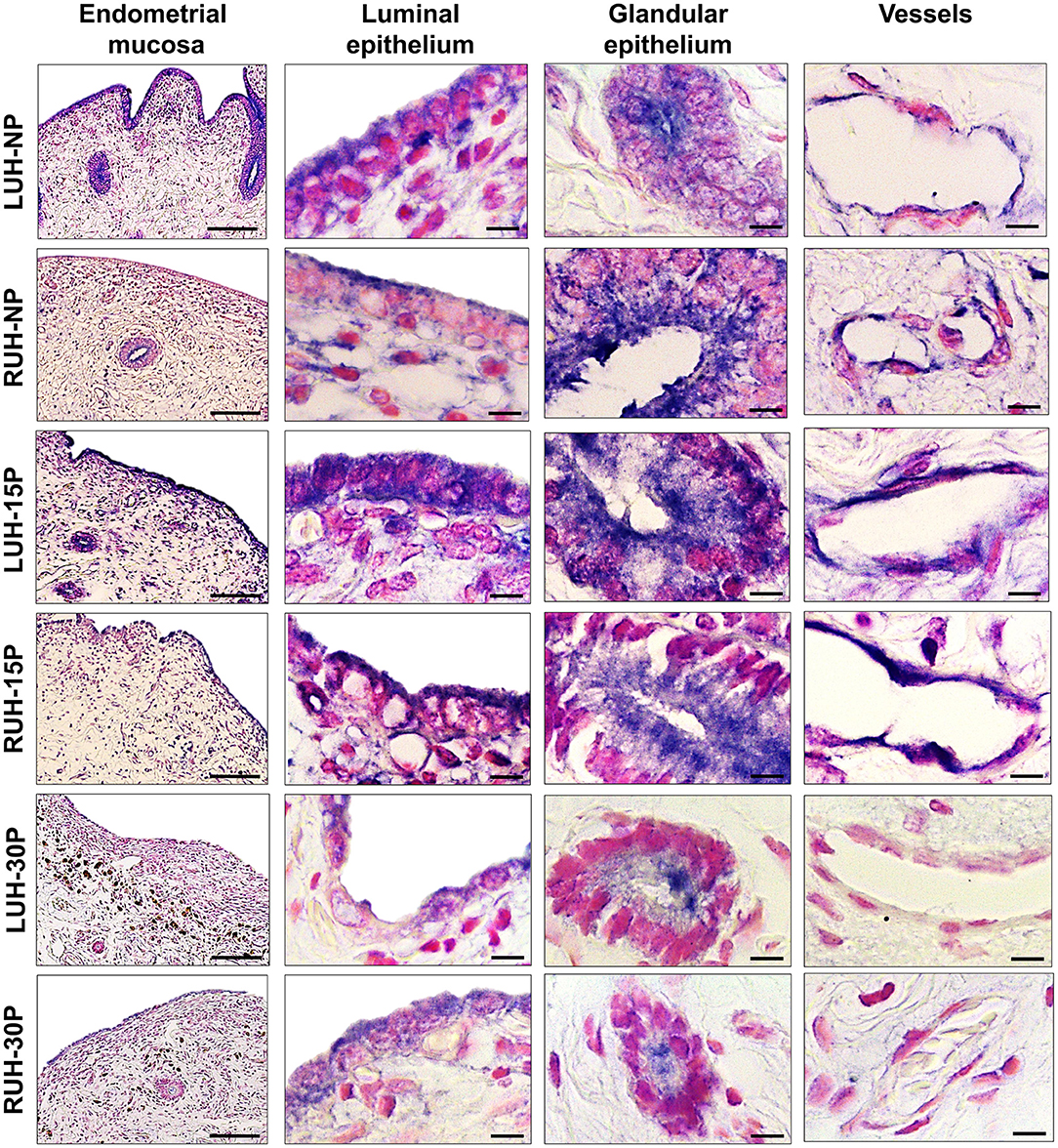
Figure 3. Immunohistochemical localization of TrKA in the endometrial mucosa of alpacas, positive labeling gives a blue-violaceous coloration. A 10X magnification of endometria of the left uterine horn (LUH) and right uterine horn (RUH) of non-pregnant females (NP); 15 days-pregnant (15P); and 30 days-pregnant females (30P) is presented (scale bar = 100 μm). Also, a 100X magnification of the luminal, glandular and vascular endothelium of the different physiological status is shown (scale bar = 10 μm).
In 30 days-pregnant alpacas, a strong immunosignal was observed in the extraembryonic membranes of the conceptus for β-NGF (Figures 4A,B) and TrKA (Figures 4C,D).
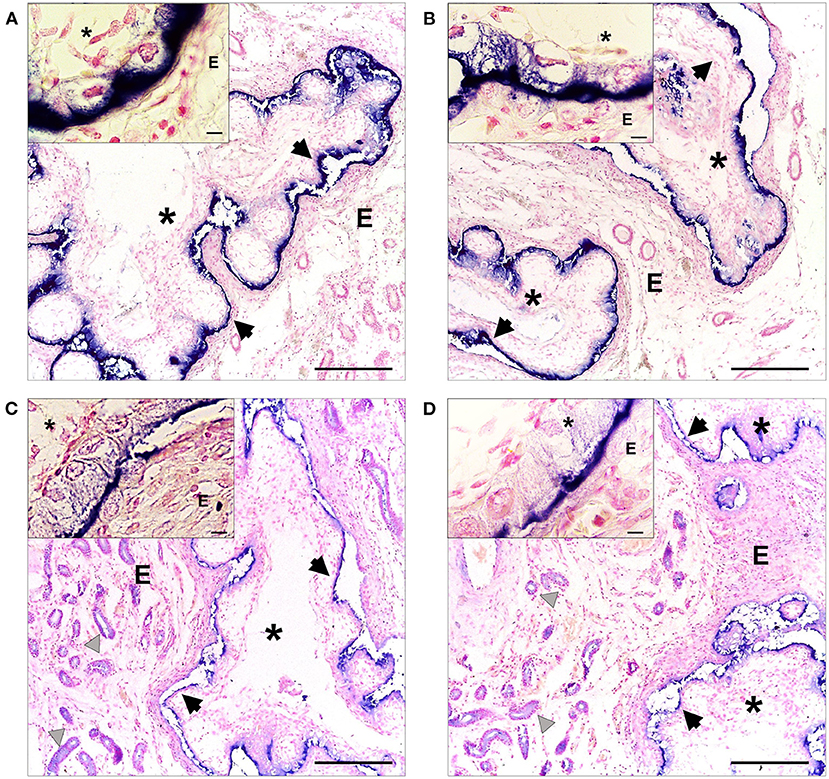
Figure 4. Immunohistochemical localization of β-NGF (A,B) and TrKA (C,D) in the endometrial mucosa of 30 days-pregnant alpacas, positive labeling gives a blue-violaceous coloration. 100-fold magnification (bar = 100 μm) of left uterine horn (A,C) and right uterine horn (B,D). In addition, inset shows a magnification of the extraembryonic membranes (bar = 10 μm). E: endometrium, asterisks: extraembryonic membranes, black arrows indicate the positive immunolabeling on the extraembryonic membranes, gray triangles indicate positive TrKA immunostaining in glandular epithelium and vessels.
Relative Abundance of VEGFA in Alpaca Endometrium
VEGFA transcripts were present in the endometrium of uterine horns from non-pregnant and pregnant alpacas (Figure 5). Differences between left and right uterine horns were observed only in pregnant alpacas (15 and 30P), in all cases, the RUH showed a higher relative abundance than the LUH (p ≤ 0.01).
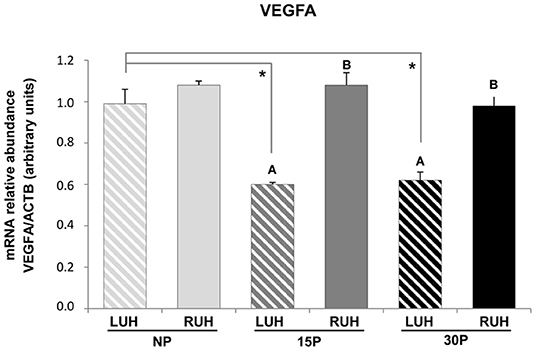
Figure 5. Relative abundance of VEGFA mRNA in the endometrium of non-pregnant (NP), 15 days-pregnant (15P), and 30 days-pregnant (30P) alpacas by RT-PCR. Histograms illustrate mean values ± SE. Different uppercase letters (A,B) indicate significant differences between LUH (left uterine horn) and RUH (right uterine horn) within NP, 15P, and 30P status (p < 0.05). Asterisks indicate significant differences (*p < 0.05) between the physiological status.
When the different physiological status were compared, only the LUH of non-pregnant females displayed a higher relative abundance of VEGFA than that of LUH of pregnant alpacas. The RUH presented similar relative abundance in all the experimental groups. No statistically significant association was observed between β-NGF expression and VEGFA relative abundance (Table 4).
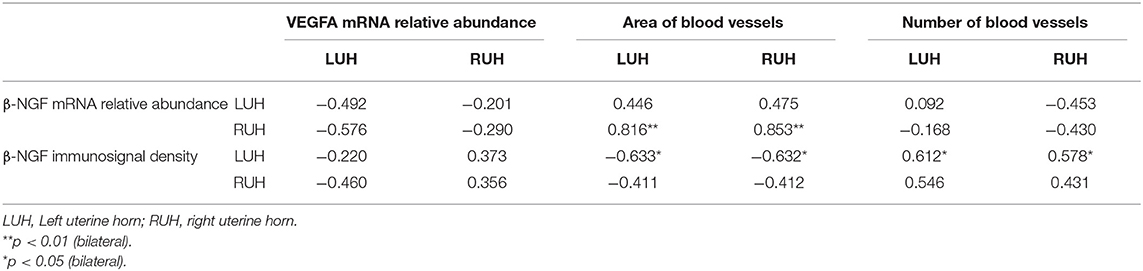
Table 4. Pearson correlation coefficient between β-NGF and VEGFA, blood vessel area and number for left and right uterine horns.
Vessels Abundance and Distribution
The number of blood vessels was similar for LUH and RUH in the 3 groups studied. The endometrium of 30 days-pregnant alpacas showed fewer vessels than 15 day-pregnant females. 30P-RUH was significantly different from both 15P-RUH and 15P-LUH. Whereas, 30P-LUH presented a smaller number of vessels than 15-LUH (Figure 6A).
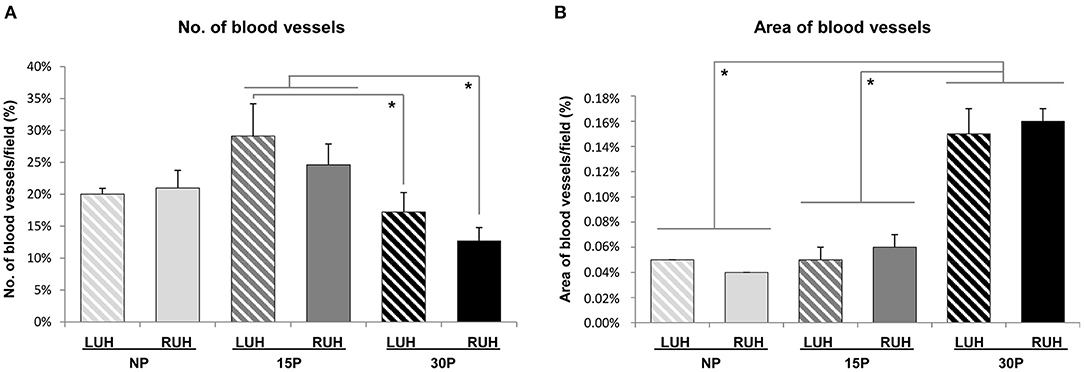
Figure 6. Effect of pregnancy status and stage of pregnancy on the blood vessel number and area in the endometrium of alpacas. (A) Number of blood vessels in LUH (left uterine horn) and RUH (right uterine horn) of non-pregnant (NP), 15 days-pregnant (15P), and 30 days-pregnant (30P) alpacas. (B) Percentage of the endometrial area occupied by blood vessels in LUH and RUH. Histograms illustrate mean values ± SE. Asterisks indicate significant differences (*p < 0.05).
The area occupied by the vessels was similar between non-pregnant and 15 days-pregnant females. While 30 days-pregnant animals presented significantly higher values of the vascularized area compared to non-pregnant and 15 day-pregnant alpacas. No differences between left and right uterine horns were detected (Figure 6B).
LUH displayed a positive association between β-NGF immunolabelling and the number of vessels (p = 0.034); however, the correlation between β-NGF immunolabelling and the vascularized area of the endometrium was negative (p = 0.027). Regarding RUH, no statistically significant association was observed between β-NGF immunosignal and the number or area of vessels (Table 4).
Discussion
The current study reveals for the first time the gene expression patterns and the protein localization of β-NGF and TrKA in the alpaca uterus during the peri-implantation period along with VEGFA gene expression patterns and the features of endometrium vasculature. These results support the implication of the β-NGF system during the early pregnancy period of South American camelids.
β-NGF and TrKA presence has been reported in the uterus of spontaneous and reflex ovulatory species, as well as in species with different placentation, such as humans, rodents, and rabbits with hemochorial placentation, goats and bovines with synepitheliochorial placentation, and pigs and horses with epitheliochorial placentation (17, 20, 36, 37). In all the species assayed, β-NGF immunoreactivity was detected in the luminal epithelium, glandular epithelium, and vascular tissue, this latter especially in pig and horse. The uterine expression of TrKA mirrored that of its ligand, it is mainly localized in the luminal, glandular epithelium, and vessels of the above-mentioned species (17, 20, 36, 37). Coincidently, the same distribution was observed for β-NGF system in alpaca oviducts (38), and uterine horns. In camelids, the establishment of pregnancies occurs almost exclusively in the LUH; at 15 days of pregnancy elongated embryos, that reach a length of 21 cm, are free within the uterine lumen until day 22–26 of gestation when the trophoblast becomes apposed to the epithelial surface of the uterus (6, 7). Striking differences were determined in alpacas for β-NGF and TrKA immunolabeling among the different physiological status analyzed. The signal was remarkably strong in the uterus of 15 days-pregnant females when the embryo elongates and the endometrium prepares for implantation. In contrast, it was faint in non-pregnant and 30 days-pregnant alpacas. In squirrel (Citellus dauricus Brandt), β-NGF and TrKA proteins reached the highest immunoreactivity at the time of early pregnancy (15). In coincidence, Lobos et al. (18) reported higher amounts of mature β-NGF in the uterus from early pregnant rats compared to non-pregnant, middle, and late pregnant females. Interestingly, although β-NGF protein was almost imperceptible in the uterus of 30 days-pregnant alpacas, a strong signal was ascertained in the extraembryonic membranes, as well as for TrKA signal. According to this, in humans, β-NGF was found in the cyto and syncytial trophoblast, chorionic mesodermic cells, and in decidua during the first trimester of gestation (39). Moreover, Coassin et al. (40) also reported the presence of β-NGF and TrKA in human amniotic membranes. In alpacas, even though no differences were observed in the β-NGF immunohistochemical patterns between the LUH and the RUH during pregnancy, TrKA immunolabeling was stronger in the LUH of non-pregnant and 15 days-pregnant alpacas. Maranesi et al. (17), demonstrated that treatment with TrKA inhibitor reduced the NGF-induced pathway in rabbit uterus. In addition, gene expression studies of matrix metalloproteinases (MMPs) during early pregnancy in alpacas (Vicugna pacos), demonstrated higher mRNA levels of MMP-2, which play an important role during embryo implantation in the uterine horn, in the LUH compared to the RUH of non-pregnant and 15 days-pregnant alpacas (41). Interestingly, MMP-2 activates pro-NGF into NGF (42). All these data would suggest that the NGF pathway is more active in the luminal epithelium of the left uterine horn, especially in the pre-implantation period, when the immunolabelling of β-NGF and TrKA was increased.
It has been suggested that β-NGF function in the uterus and placenta during pregnancy is to ensure adequate maternal immunomodulatory and developmental processes at the fetal-maternal interface. A well-balanced level of β-NGF is required for a successful pregnancy outcome as both insufficient or elevated levels of the factor may provoke fetal rejection (2, 21, 43). In alpacas the β-NGF system was not only detected in embryo membranes when the implantation has already occurred but also in the pre-implantation endometrium, during embryo elongation, suggesting a role during this period.
Intriguingly, in alpaca endometrium β-NGF and TrKA mRNA and protein expression were dissimilar, analogous observations were reported by Sari et al. (38) when analyzing gene and protein expression pattern in the llama oviduct. Many authors have postulated that regulation of β-NGF production does not occur solely at the level of transcription and that post-transcriptional mechanisms operate as well. Even more, there is a diverse post-transcriptional regulation between different cell types (44–46). For example, in rat uterus, alterations in NGF isoforms during pregnancy, accumulation of proNGF, and decreased ratios of mature β-NGF to proNGF were reported (18).
A rise in endometrial vascularization during pregnancy has been described in other mammals. Mares that were pregnant exhibited a greater area of the endometrium occupied by blood vessels compared with non-pregnant females and mares at 21 days of pregnancy exhibited the greatest area of endometrium occupied by blood vessels (47). Something similar occurs in cows, in which the number of blood vessels in the endometrium increased from day 15 to 18 of gestation, and pregnant cows had more blood vessels than non-pregnant cows on day 18 (48, 49). Likewise, in alpacas, a larger area of the endometrium was occupied by blood vessels at 30 days of gestation. It is known that intrauterine administration of β-NGF induces an increase of endometrial vascularization in both uterine horns (13) and it has been demonstrated that β-NGF acts as a pro-angiogenic factor that increases the expression of VEGFA in cultures of llama granulosa cells (29). In 15 days-pregnant alpacas, the increased β-NGF protein intensity was in correspondence with the elevated number of vessels in the endometrium. At 15 days of pregnancy, embryos occupied the entire uterine cavity and on day 45 the entire extent of the trophoblast was closely attached to the left and right uterine epithelium (6, 7). This could explain the vascular similarities between both uterine horns; nevertheless, a positive correspondence between β-NGF and the number of vessels was only observed in the left uterine horn.
The VEGF family is known to regulate vascular functions such as angiogenesis, vasculogenesis, vascular permeability, and lymphangiogenesis; and is composed of several subtypes including VEGFA, VEGFB, VEGFC, VEGFD, and placental growth factor (50). VEGFA mRNA can be alternatively spliced into five iso-forms representing proteins of 121, 145, 165, 189, and 206 amino acids (51). In alpacas, VEGFA mRNA was present in the uterus of pregnant and non-pregnant females, showing a higher expression in the endometrium of non-pregnant animals and differences between horns in pregnant females. In bovines, gene expression of VEGFA transcript variant 1 was higher in non-pregnant females (18th day of estrous cycle) compared with pregnant ones (18th day of pregnancy). Even more, VEGFA levels did not show changes during early pregnancy or during the peri-implantation period (49). Hayashi et al. (48) speculated that the lower peripheral estradiol concentrations in pregnant heifers explain these results. In addition to this, Johnson et al. (52) determined that the expression of VEGFA in the endometrium of ovariectomized sheep was stimulated by estradiol. Moreover, estradiol receptor, mRNA expression was lower in the ipsilateral uterine horn of non-pregnant sheep, cows and horses, and in pregnant mares (day 13), indicating changes in the sensitivity of the endometrium to estradiol (53). These findings could also explain the differences of VEGFA expression between uterine horns and physiological status in alpacas. In mares and pigs, VEGFA was upregulated during early pregnancy. In pigs VEGFA isoform 188 aa was specifically assayed (46); while in horses, the VEGFA transcript variant X1 was amplified (54). In alpacas, primers amplified VEGFA transcript variant X1(186 aa), X2 (180 aa), and X3 (162 aa), a fact that could also explain the differences between alpacas and other livestock species. Although the VEGFA gene expression pattern did not show drastic changes between pregnancy periods and was even decreased compared to non-pregnant alpacas, other members of the VEGF system could be involved in the angiogenesis changes observed in alpacas. Hayashi et al. (48), reported an increase of VEGFB on day 15 compared to days 18 and 27 of pregnancy, suggesting the possible involvement of VEGFB in endometrial receptivity for successful implantation at earlier stages in Japanese Black cows.
In summary, the endometrium expresses β-NGF, TrKA, and VEGFA in pregnant and non-pregnant alpacas. β-NGF differential protein pattern in alpaca endometrium during the peri-implantation period of pregnancy implies a precise regulation, which suggests a possible role in embryo development and implantation. The proangiogenic function of β-NGF could be responsible for the increase of endometrial vasculature, thereby supporting embryo implantation and survival. Nevertheless, further investigation on the β-NGF and VEGF signaling pathway is necessary to fully understand the differential implantation in South American camelids.
Data Availability Statement
The raw data supporting the conclusions of this article will be made available by the authors, without undue reservation.
Ethics Statement
The animal study was reviewed and approved by Committee of Animal Ethics and Welfare (Comité de Ética y Bienestar Animal (CEBA) from the School of Veterinary Medicine of the UNMSM. Written informed consent for participation was not obtained from the owners because the animals were destined for meat production and they were sent to a slaughterhouse, where samples were collected following the regulations of the abattoir.
Author Contributions
DB and MA analyzed the data and wrote the manuscript. MA, LS, and DB performed the laboratory analysis and participated in the acquisition of the data. MA and SA designed the study. SA and MR discussed and revised the manuscript. All authors read and approved the manuscript for publication.
Funding
This work was partially supported by Universidad Nacional de Tucumán [Grant number PIUNT A616/3], Fondo para la Investigación Científica y Tecnológica, [Grant number BID-PICT 2017-0363 and BID-PICT 2016-0145].
Conflict of Interest
The authors declare that the research was conducted in the absence of any commercial or financial relationships that could be construed as a potential conflict of interest.
Acknowledgments
The authors of this manuscript thank Prof. Joel Pacheco (Instituto Veterinario de Investigaciones Tropicales y de Altura, Universidad Nacional Mayor de San Marcos) and Dr. Renato Zampini (Instituto Superior de Investigaciones Biológicas, CONICET-UNT) for their collaboration.
References
1. Galina CY, Valencia J. Reproducción de Animales Domésticos. 2, ed Limusa, Noeniega editors. México (2006). p. 128, 130, 135, 136.
2. Tometten M, Blois S, Kuhlmei A, Stretz A, Klapp BF, Arck PC. Nerve growth factor translates stress response and subsequent murine abortion via adhesion molecule-dependent pathways. Biol Reproduct. (2006) 74:674–83. doi: 10.1095/biolreprod.105.044651
3. Sumar J. Removal of the ovaries or ablation of the corpus luteum and its effect on the maintenance of gestation in the alpaca and llama. Acta Vet Scand Suppl. (1988) 83:133–41.
4. Diskin MG, Morris DG. Embryonic and early foetal losses in cattle and other ruminants. Reproduct Domestic Animals. (2008) 43:260–7. doi: 10.1111/j.1439-0531.2008.01171.x
5. Aba MA, Kindahl H, Forsberg M, Quiroga M, Auza N. Levels of progesterone and changes in prostaglandin F(2alpha) release during luteolysis and early pregnancy in llamas and the effect of treatment with flunixin meglumine. Anim Reprod Sci. (2000) 59:87e97. doi: 10.1016/S0378-4320(00)00068-3
6. Olivera LVM, Zago DA, Jones CJP, Bevilacqua E. Develpmental changes at the materno-embryonic interface in early pregnancy of the alpaca, Lamos pacos. Anat Embryol. (2003) 207:317–31. doi: 10.1007/s00429-003-0346-1
7. Picha Y, Tibary A, Memon M, Kasimanickam R, Sumar J. Chronology of early embryonic development and embryo uterine migration in alpacas. Theriogenology. (2013) 79:702–8. doi: 10.1016/j.theriogenology.2012.11.027
8. Brown BW. A review on reproduction in South American camelids. Animal Reproduct Sci. (2000) 58:169–95. doi: 10.1016/S0378-4320(99)00081-0
9. Powell SA, Smith BB, Timm KI, Menino AR. Estradiol production by preimplantation blastocysts and increased serum progesterone following estradiol treatment in llamas. Animal Reproduct Sci. (2007) 102:66–75. doi: 10.1016/j.anireprosci.2006.10.002
10. Fernandez-Baca S, Hansel W, Saatman R, Sumar J, Novoa C. Differential luteolytic effects of right and left uterine horns in the alpaca. Biol Reproduct. (1979) 20:586–95. doi: 10.1095/biolreprod20.3.586
11. Vaughan J, Mihm M, Wittek T. Factors influencing embryo transfer success in alpacas—a retrospective study. Animal Reproduct Sci. (2013) 136:194–204. doi: 10.1016/j.anireprosci.2012.10.010
12. Del Campo MR, Del Campo CH, Ginther OJ. Vascular provisions for a local utero-ovarian cross-over pathway in new world camelids. Theriogenology. (1996) 46:983–91. doi: 10.1016/S0093-691X(96)00262-2
13. Urra F, Ratto MH, Silva M. Evaluation of the effect of mating, intrauterine deposition of raw seminal plasma or seminal plasma purified β-NGF on endometrial vascularization in llamas. Theriogenology. (2019) 125:18–23. doi: 10.1016/j.theriogenology.2018.10.007
14. Vandita D, Kilari A, Pisal H, Patil V, Mehendale S, Wagh G, et al. Maternal nerve growth factor levels during pregnancy in women with preeclampsia: a longitudinal study. Int J Dev Neurosci. (2015) 47:340–6. doi: 10.1016/j.ijdevneu.2015.08.003
15. Li B, Sheng X, Song M, Zhang H, Weng J, Zhang M, et al. Expression of nerve growth factor and its receptors TrkA and p75 in the uterus of wild female ground squirrel (Citellus dauricus Brandt). General Comparat Endocrinol. (2012) 176:62–9. doi: 10.1016/j.ygcen.2011.12.024
16. Mohamed R, El-Remessy AB. Imbalance of the nerve growth factor and its precursor: implication in diabetic retinopathy. J Clin Exp Ophthalmol. (2015) 6:483. doi: 10.4172/2155-9570.1000483
17. Maranesi M, Parillo F, Leonardi L, Rebollar PG, Alonso B, Petrucci L, et al. Expression of nerve growth factor and its receptors in the uterus of rabbits: functional involvement in prostaglandin synthesis. Domestic Animal Endocrinol. (2016) 56:20–8. doi: 10.1016/j.domaniend.2016.02.001
18. Lobos E, Gebhardt C, Kluge A, Spanel-Borowski K. Expression of nerve growth factor (NGF) isoforms in the rat uterus during pregnancy: accumulation of precursor proNGF. Endocrinology. (2005) 146:1922–9. doi: 10.1210/en.2004-0925
19. Mirshokraei P, Hassanpour H, Rahnama A, Foster WG. Gene expression of BDNF and its receptors, TrkB and p75 in the uterus and oviduct of pregnant and non-pregnant ewes. Res Vet Sci. (2013) 95:164–8. doi: 10.1016/j.rvsc.2013.03.009
20. Wessels JM, Wu L, Leyland NA, Wang H, Foster WG. The brain-uterus connection: brain derived neurotrophic factor (BDNF) and its receptor (Ntrk2) are conserved in the mammalian uterus. PLoS ONE. (2014) 9:e94036. doi.org/10.1371/journal.pone.0094036 doi: 10.1371/journal.pone.0094036
21. Tometten M, Blois S, Arck PC. Nerve growth factor in reproductive biology: link between the immune, endocrine and nervous system? Chem Immunol Allergy. (2005) 89:135–48. doi: 10.1159/000087962
22. Garcia-Garcia RM, Arias-Alvarez M, Sanchez-Rodriguez A, Lorenzo PL, Rebollar PG. Role of nerve growth factor in the reproductive physiology of female rabbits: a review. Theriogenology. (2020) 150:321–8. doi: 10.1016/j.theriogenology.2020.01.070
23. Julio-Pieper M, Lara HE, Bravo JA, Romero C. Effects of nerve growth factor (NGF) on blood vessels area and expression of the angiogenic factors VEGF and TGFbeta1 in the rat ovary. Reproduct Biol Endocrinol. (2006) 4:1–11. doi: 10.1186/1477-7827-4-57
24. Julio-Pieper M, Lozada P, Tapia V, Vega M, Miranda C, Vantman D, et al. Nerve growth factor induces vascular endothelial growth factor expression in granulosa cells via a trkA receptor/mitogen-activated protein kinase-extracellularly regulated kinase 2-dependent pathway. J Clin Endocrinol Metabol. (2009) 94:3065–71. doi: 10.1210/jc.2009-0542
25. Mona e Pinto J, Pavanelo V, Alves de Fátima L, Medeiros de Carvalho Sousa LM, Pacheco Mendes G, Machado Ferreira R, et al. Treatment with eCG decreases the vascular density and increases the glandular density of the bovine uterus. Reproduct Domestic Animals. (2014) 49:453–62. doi: 10.1111/rda.12307
26. Kershaw-Young CM, Druart X, Vaughan J, Maxwell WMC. β-Nerve growth factor is a major component of alpaca seminal plasma and induces ovulation in female alpacas. Reproduct Fertility Dev. (2012) 24:1093–7. doi: 10.1071/RD12039
27. Ratto MH, Leduc YA, Valderrama XP, Van Straaten KE, Delbaere LT, Pierson RA, et al. The nerve of ovulation-inducing factor in semen. Proc Natl Acad Sci USA. (2012) 109:15042–7. doi: 10.1073/pnas.1206273109
28. El Allali K, El Bousmaki N, Ainani H, Simonneaux V. Effect of the camelid's seminal plasma ovulation-inducing factor/β-NGF: a kisspeptin target hypothesis. Front Vet Sci. (2017) 4:99. doi.org/10.3389/fvets.2017.00099 doi: 10.3389/fvets.2017.00099
29. Valderrama XP, Goicochea JF, Silva ME, Ratto MH. The effect of seminal plasma β-NGF on follicular fluid hormone concentration and gene expression of steroidogenic enzymes in llama granulosa cells. Reproduct Biol Endocrinol. (2019) 17:60. doi: 10.1186/s12958-019-0504-9
30. Catone G, Basile M, Barbato O, Ayala C. Transvaginal embryo biometry in alpaca (Lama pacos): Preliminary report. In: Gerken M, Renieri C, Editors. South American Camelids Research. (2006) 1:99–105.
31. Argañaraz ME, Apichela SA, Zampini R, Vencato J, Stelletta C. Biochemical and protein profile of alpaca (Vicugna pacos) uterine horn fluid during early pregnancy. Reprod Domest Anim. (2015) 50:121–8. doi: 10.1111/rda.12460
32. Barraza DE, Zampini R, Apichela SA, Pacheco JI, Argañaraz ME. Changes in mucins and matrix metalloproteases in the endometrium of early pregnant alpacas (Vicugna pacos). Acta Histochem. (2018) 120:438–45. doi: 10.1016/j.acthis.2018.05.009
33. Sari LM, Zampini R, Argañaraz ME, Carretero MI, Fumuso FG, Barraza DE, et al. Expression of β-NGF and high-affinity NGF receptor (TrKA) in llama (Lama glama) male reproductive tract and spermatozoa. Mol Reproduct Dev. (2018) 85:934–44. doi: 10.1002/mrd.23075
34. Jensen EC. Quantitative analysis of histological staining and fluorescence using ImageJ. Anatomical Record. (2013) 296:378–81. doi: 10.1002/ar.22641
35. Woloszyk A, Buschmann J, Waschkies C, Stadlinger B, Mitsiadis TA. Human dental pulp stem cells and gingival fibroblasts seeded into silk fibroin scaffolds have the same ability in attracting vessels. Front Physiol. (2016) 7:140. doi: 10.3389/fphys.2016.00140
36. Ren L, Medan MS, Weng Q, Jin W, Li C, Watanabe G, et al. Immunolocalization of nerve growth factor (NGF) and its receptors (TrkA and p75LNGFR) in the reproductive organs of Shiba goats. J Reproduct Dev. (2005) 51:399–404. doi: 10.1262/jrd.16082
37. Jana B, Andronowska A. Expression of nerve growth factor and its receptors in the uterus of gilts with endometritis induced by infection with Escherichia coli. J Comparat Pathol. (2012) 147:522–32. doi: 10.1016/j.jcpa.2012.03.001
38. Sari LM, Zampini R, Argañaraz ME, Ratto MH, Apichela SA. Effect of mating on mRNA and protein expression of beta nerve growth factor and its receptor, TrKA, in the oviduct of llama (Lama glama). Mol Reprod Dev. (2020) 87:1133–40. doi: 10.1002/mrd.23426
39. Toti P, Ciarmela P, Florio P, Volpi N, Occhini R, Petraglia F. Human placenta and fetal membranes express nerve growth factor mRNA and protein. J Endocrinol Invest. (2006) 29:337–41. doi: 10.1007/BF03344105
40. Coassin M, Lambiase A, Micera A, Tirassa P, Aloe L, Bonini S. Nerve growth factor modulates in vitro the expression and release of TGF-β1 by amniotic membrane. Graefe's Arch Clin Exp Ophthalmol. (2006) 244:485–91. doi: 10.1007/s00417-005-0098-0
41. Barraza DE, Zampini R, Apichela SA, Pacheco JI, Argañaraz ME. Modifications of extracellular matrix features in the left and right uterine horns during the embryo pre-implantation period in Vicugna pacos. Theriogenology. (2020) 157:440–8. doi: 10.1016/j.theriogenology.2020.07.001
42. Saygili E, Schauerte P, Pekassa M, Saygili E, Rackauskas G, Schwinger RH, et al. Sympathetic neurons express and secrete MMP-2 and MT1-MMP to control nerve sprouting via pro-NGF conversion. Cell Mol Neurobiol. (2011) 31:17–25. doi: 10.1007/s10571-010-9548-2
43. Frank P, Barrientos GL, Tirado González I, Cohen M, Moschansky P, Peters EM, et al. Balanced levels of nerve growth factor are requiredfor normal pregnancy progression. Reproduction. (2014) 148:179–89. doi: 10.1530/REP-14-0112
44. Gonzalez D, Les Dees W, Hiney JK, Ojeda SR, Saneto RP. Expression of β-nerve growth factor in cultured cells derived from the hypothalamus and cerebral cortex. Brain Res. (1990) 511:249–58. doi: 10.1016/0006-8993(90)90169-C
45. Colangelo AM, Pani L, Mocchetti I. Correlation between increased AP-1?-NGF binding activity and induction of nerve growth factor transcription by multiple signal transduction pathways in C6-2B glioma cells. Mol Brain Res. (1996) 35:1–10. doi: 10.1016/0169-328X(95)00171-N
46. Sherer TB, Neff PS, Tuttle JB. Increased nerve growth factor mRNA stability may underlie elevated nerve growth factor secretion from hypertensive vascular smooth muscle cells. Mol Brain Res. (1998) 62:167–74. doi: 10.1016/S0169-328X(98)00247-2
47. Silva LA, Klein C, Ealy AD, Sharp DC. Conceptus-mediated endometrial vascular changes during early pregnancy in mares: an anatomic, histomorphometric, and vascular endothelial growth factor receptor system immunolocalization and gene expression study. Reproduction. (2011) 142:593–603. doi: 10.1530/REP-11-0149
48. Hayashi KG, Hosoe M, Fujii S, Kanahara H, Sakumoto R. Temporal expression and localization of vascular endothelial growth factor family members in the bovine uterus during peri-implantation period. Theriogenology. (2019) 133:56–64. doi: 10.1016/j.theriogenology.2019.04.021
49. Silva LA, Ginther OJ. Local effect of the conceptus on uterine vascular perfusion during early pregnancy in heifers. Reproduction. (2010) 139:453–63. doi: 10.1530/REP-09-0363
50. Girling JE, Rogers PA. Regulation of endometrial vascular remodelling: role of the vascular endothelial growth factor family and the angiopoietin-TIE signalling system. Reproduction. (2009) 138:883–93. doi: 10.1530/REP-09-0147
51. Zygmunt M, Herr F, Münstedt K, Lang U, Liang OD. Angiogenesis and vasculogenesis in pregnancy. Eur J Obstetr Gynecol Reproduct Biol. (2003) 110:S10–8. doi: 10.1016/S0301-2115(03)00168-4
52. Johnson ML, Grazul-Bilska AT, Redmer DA, Reynolds LP. Effects of estradiol-17beta on expression of mRNA for seven angiogenic factors and their receptors in the endometrium of ovariectomized (OVX) ewes. Endocrine. (2006) 30:333–42. doi: 10.1007/s12020-006-0012-5
53. Kalpokas I, Mattos RC, Cavestany D, Martínez MN, Perdigón F, Meikle A. Effect of side of the corpus luteum and pregnancy on estrogen and progesterone receptor expression and localization in the endometrium of mares. Theriogenology. (2018) 114:221–8. doi: 10.1016/j.theriogenology.2017.12.034
Keywords: alpacas, embryo implantation, uterus, β-NGF, VEGF
Citation: Barraza DE, Sari LM, Apichela SA, Ratto MH and Argañaraz ME (2021) New Insights Into the Role of β-NGF/TrKA System in the Endometrium of Alpacas During Early Pregnancy. Front. Vet. Sci. 7:583369. doi: 10.3389/fvets.2020.583369
Received: 14 July 2020; Accepted: 07 December 2020;
Published: 22 January 2021.
Edited by:
Khalid El Allali, Agronomic and Veterinary Institute Hassan II, MoroccoReviewed by:
Rosa Maria Garcia-Garcia, Complutense University of Madrid, SpainDeborah Neild, University of Buenos Aires, Argentina
Martin G. Maquivar, Washington State University, United States
Fabio S. Lima, University of California, Davis, United States
Copyright © 2021 Barraza, Sari, Apichela, Ratto and Argañaraz. This is an open-access article distributed under the terms of the Creative Commons Attribution License (CC BY). The use, distribution or reproduction in other forums is permitted, provided the original author(s) and the copyright owner(s) are credited and that the original publication in this journal is cited, in accordance with accepted academic practice. No use, distribution or reproduction is permitted which does not comply with these terms.
*Correspondence: Martin E. Argañaraz, bWFydGluLmFyZ2FuYXJhekBmYnFmLnVudC5lZHUuYXI=