- 1Department of Veterinary Clinical Sciences, College of Veterinary Medicine, Iowa State University, Ames, IA, United States
- 2Veterinary Diagnostic Laboratory, College of Veterinary Medicine, Iowa State University, Ames, IA, United States
The objective of this study was to describe bacterial culture and antibiotic susceptibility results in 476 dogs presenting with suspected bacterial keratitis in Iowa and surrounding Midwestern states, further detailing trends in patient characteristics, seasonality, and antimicrobial resistance. Corneal swabs yielded 465 bacterial isolates and 220 cultures (46.2%) with no apparent growth (0–5 isolates per culture). The most frequent bacterial genera were Staphylococcus (32.3%), Streptococcus (19.1%), and Pseudomonas (12.5%), while the most common bacterial species were Staphylococcus pseudintermedius (26.7%), Streptococcus canis (12%), and Pseudomonas aeruginosa (7.5%). Compared to mixed-breed dogs, canine breeds most likely to be examined for ulcerative keratitis included Boston terrier, Cavalier King Charles spaniel, miniature pinscher, pug, rat terrier, Saint Bernard, shih tzu, and silky terriers. In summer, the likelihood to yield a negative culture was reduced while the likelihood to culture Pseudomonas species was increased. Bacteria considered multidrug resistant (MDR, resistant to ≥ 3 antibiotic classes) represented 20% of all canine isolates and were most prevalent for Staphylococcus species (33%). An alarming, escalating trend of MDR prevalence was noted between 2016 (5%) and 2020 (34%). Individual ophthalmic preparations (i.e., single antibiotics or commercially available antibiotic combinations) with highest efficacy against all bacterial isolates included chloramphenicol (83%), ceftiofur (79%), amikacin (77%), neomycin-polymyxin B-bacitracin (77%), and gentamicin (74%). Efficacy of systemic antibiotics and combinations of ophthalmic preparations was also evaluated. Based on the present findings, triple antibiotic (Neo-Poly-Bac) is recommended as empirical monotherapy for prophylactic antibiotic therapy in dogs with simple corneal ulcers, while a chloramphenicol-ciprofloxacin combination is empirically recommended for therapeutic management of infected corneal ulcers. Pending culture and susceptibility results, appropriate selection of empiric antibiotic therapy is important to enhance therapeutic outcome and reduce antibacterial resistance in dogs with corneal ulceration.
Introduction
Bacterial keratitis is a major global cause of ocular discomfort and visual impairment in dogs and other species. Following an injury to the eye from trauma or other causes, corneal wounds in dogs have a high tendency toward infection given the presence of indigenous microflora on the corneal and conjunctival surfaces, including Staphylococcus species, Streptococcus species, Pseudomonas species, and gram-positive bacilli (1–3). Rapid and appropriate use of antibiotics is critical in mitigating the potential devastating effects of bacterial keratitis in dogs, including keratomalacia (corneal melting), corneal perforation, scarring, and loss of vision or the entire eye (4, 5). Successful management requires the clinician to be aware of the most common bacterial isolates and their susceptibility profiles to antibiotics, as there is often a lag period of several days until corneal samples collected from the patient provide results for bacterial culture and sensitivity testing.
The distribution of bacterial populations has been described previously for several geographic regions of the world including Asia (3, 6, 7), Australia (1, 8), Europe (9), North America (10, 11), and South America (2). While there are distinct similarities between these regional investigations, there are notable differences in bacterial prevalence and susceptibilities across the world. Further, differences within separate geographic regions of even a single country (10, 11) demonstrate that more targeted evaluation of local or regional populations is needed. Most previous reports in veterinary literature are limited to describing the prevalence of bacterial isolates and susceptibility profiles for individual antibiotics (6, 7, 10). Such information is useful but is generally insufficient to provide optimized recommendations for clinicians managing bacterial keratitis in dogs. For instance, it is common practice to use two different ophthalmic antibiotics in veterinary patients with an infected corneal ulcer (12–15) or reach for systemic antibiotics in patients with vascularized corneal lesions or corneal perforations. However, to our knowledge, specific comparisons of antibiotic combination efficacies on bacterial isolates has not been described in dogs or other species, and only selected canine reports have described the efficacy of systemic antibiotics for corneal disease (16, 17). Using fewer, more effective drugs for an appropriate timeframe allows for better patient outcomes and less antibiotic resistance. Less antibiotic resistance in the environment will benefit both veterinary and human medicine.
The primary goal of the study was to describe results of bacterial cultures and susceptibility testing (ophthalmic and systemic profiles) in canine patients that presented with bacterial keratitis from Iowa and surrounding Midwestern states of the United States. A secondary objective was to report the prevalence and trends over time (2014–2020) of antibacterial resistance in corneal isolates, as well as risk factors associated with positive bacterial growth from corneal samples. We hope this information will provide clinically relevant data for managing bacterial keratitis in dogs and help mitigate the alarming rise of antibacterial resistance in the species.
Materials and Methods
Data Collection
The database of Iowa State University's Veterinary Diagnostic Laboratory (ISU VDL) was searched for results of bacterial cultures collected from canine corneas, as well as associated antibiotic susceptibility testing when available. The search covered a period from March 2014—when an ophthalmic-specific susceptibility profile was introduced—to the date of manuscript writing (June 2020). Corneal cultures processed by ISU VDL originated from two sources: (i) In-house submissions from the ISU Lloyd Veterinary Medical Center (ISU LVMC), for which information about patients characteristics (i.e., age, breed) was available; and (ii) Mail-in submissions from veterinary practices in Iowa and surrounding states (Minnesota, Missouri, South Dakota).
Sample Identification and Susceptibility Testing
At the ISU LVMC, samples were collected with sterile culturette swabs (BBLTM CultureSwabTM, Becton Dickinson and Company, Sparks, MD) that were pre-moistened prior to contact with the corneal wound and processed for aerobic microbiologic assessment using a non-selective medium (tryptic soy agar with 5% sheep blood [blood agar]) and a Gram-negative selective medium (MacConkey). The blood agar was incubated at 35 ± 2°C with 5–10% CO2 for a total length of 4 days while the MacConkey agar was incubated 35 ± 2°C without CO2 for a total length of 2 days. Both agar plates were observed for growth every 24 h. Organisms were then identified using Matrix-Assisted Laser Desorption Ionization Time-of-Flight mass-spectrometry (MALDI-TOF MS, Bruker) or conventional microbiology methods when necessary. Minimum inhibitory concentration (MIC) susceptibility testing was performed using an automated broth microdilution system (Sensititre AIM, Trek Diagnostic System Inc.) and susceptibility panels (Thermo Fisher Scientific). Interpretations were determined by the MIC breakpoints, which are based on the VET08 and M100 Clinical and Laboratory Standards Institute (CLSI) documents (18, 19). Depending on the clinician's request, susceptibility testing performed by the ISU VDL included an ophthalmic susceptibility profile (JOEYE2 plate, Thermo Scientific Inc.) and/or a systemic susceptibility profile. Antibiotics and drug concentrations evaluated in JOEYE2 plates can be found on the manufacturer's website (assets.thermofisher.com/TFS-Assets/MBD/Specification-Sheets/Sensititre-Plate-Layout-JOEYE2.pdf).
Data Analysis
Results considered “non-interpretable” by CLSI guidelines were excluded from data analysis, that is, not classified as susceptible nor resistant in calculations of percent sensitivity and multidrug resistance. An isolate was considered susceptible to a combination therapy (e.g., chloramphenicol-ciprofloxacin) if one or both antibiotics yielded a “susceptible” result for the given isolate. Bacterial isolates were considered multidrug resistant (MDR) if resistant to three or more classes of antibiotics (20), removing all known intrinsic resistances from the MDR definition as described by Sweeney et al. (21).
Odds ratios were calculated with SigmaPlot 14.0 (Systat software, Point Richmond, CA), and P < 0.05 were considered significant unless another α-value is described: (i) Likelihood of a pure breed vs. mixed breed dog to present to the ISU LVMC Ophthalmology service with a corneal infection—as compared to other reasons for the visit such as keratoconjunctivitis sicca, glaucoma, or cataracts—evaluating the canine population presented to Ophthalmology over the 6-years study period; (ii) Likelihood of a pure breed vs. mixed breed dog with a corneal infection (i.e., positive corneal culture) to yield a bacterial isolate classified as MDR; (iii) Likelihood of selected antibiotics vs. all others to provide higher efficacy against all bacterial isolates, adjusting the α-value to 0.0026 (0.05/19) to account for multiple pairwise comparisons with the Bonferroni correction; and (iii) Likelihood of not detecting bacteria (i.e., negative corneal culture) or detecting selected bacterial genera in different seasons. For the latter, a corneal culture performed between March and May was considered as the spring season, June to August for summer, September to November for fall, and December to February for winter.
Results
Patients Characteristics
Between March 2014 and June 2020, corneal swabs were obtained from 476 dogs with suspected bacterial keratitis and submitted in-house (ISU LVMC, n = 317) or as mail-in (referring clinics, n = 159) to ISU VDL for aerobic bacterial culture and susceptibility testing. The population was comprised of dogs age 2 months to 17 years old, including 57 (8%) intact male, 285 (41%) castrated male, 56 (8%) intact female, 263 (38%) spayed female dogs, and 30 (4%) patients with no sex listed. Mixed breed dogs represented 17% (79/476) of the study population, while the most common pure breeds were shih tzu (18%, 86/476), Boston terriers (9%, 41/476), and Yorkshire terriers (4%, 18/476). Compared to mixed breed dogs, Labrador retrievers had a significantly lower likelihood (OR = 0.10, 95% CI = 0.03–0.28, P < 0.001) to present to the ISU LVMC Ophthalmology service with a suspected bacterial infection, while the likelihood was significantly higher in the following canine breeds: Boston terriers (OR = 4.16, 95% CI = 2.14–8.09, P < 0.001), Cavalier King Charles spaniels (OR = 4.55, 95% CI = 1.63–12.65, P = 0.006), miniature pinschers (OR = 6.77, 95% CI = 2.56–17.90, P < 0.001), pugs (OR = 3.93, 95% CI = 1.75–8.80, P = 0.001), rat terriers (OR = 5.31, 95% CI = 2.04–13.83, P < 0.001), Saint Bernards (OR = 16.37, 95% CI = 2.85–93.95, P = 0.002), shih tzus (OR = 6.61, 95% CI = 3.92–11.13, P < 0.001), and silky terriers (OR = 10.91, 95% CI = 2.09–57.01, P = 0.012).
Bacterial Isolate Characteristics
The 476 aerobic cultures resulted in 465 bacterial isolates, six fungal growths (data not shown), and 220 cultures (46.2%) with no apparent growth (0–5 isolates per culture). The proportion of negative bacterial cultures did not differ between in-house samples (147/317, 46.4%) and mail-in samples (73/159, 45.9%). Table 1 provides a summary of the bacterial genera and species isolated from canine corneas. The most common bacterial genera were Staphylococcus species (150/465, 32.3%), Streptococcus species (89/465, 19.1%), Pseudomonas species (58/465, 12.5%), and Corynebacterium species (39/465, 8.4%), while the most common bacterial species included Staphylococcus pseudintermedius (124/465, 26.7%), Streptococcus canis (56/465, 12.0%), and Pseudomonas aeruginosa (35/465, 7.5%). Further information detailing the species of bacteria isolated from canine corneas is summarized in Table 1. The number of isolates cultured in the spring (n = 134), summer (n = 153), fall (n = 107), and winter (n = 71) are described by genera in Figure 1. Compared to all bacterial isolates, Pseudomonas species were significantly less likely to be isolated in the spring (OR = 0.34, 95% CI = 0.15–0.77, P = 0.011), but were significantly more likely to be isolated in the summer (OR = 2.04, 95% CI = 1.18–3.53, P = 0.015). Compared to all bacterial cultures, a negative culture result (no growth) was significantly less likely to occur in the summer (OR = 0.57, 95% CI = 0.39–0.83, P = 0.004).
Topical and Systemic Antibiotic Susceptibility Testing by MIC
Ophthalmic and systemic susceptibility profiles were available for 352 and 308 of the isolates, respectively. Table 2 summarizes the findings from the ophthalmic susceptibility profiles, describing results of individual antibiotics as well as multi-drug formulations commonly used in veterinary practice (neomycin-polymyxin B-bacitracin, oxytetracycline-polymyxin B, and polymyxin B-trimethoprim). Except for erythromycin (30%), all antibiotics tested had a significantly (P ≤ 0.0026) greater percentage of susceptible isolates compared to polymyxin B (0%), bacitracin (7%), cefazolin (8%), and moxifloxacin (29%). Further, bacterial isolates were significantly (P < 0.0026) more susceptible to gentamicin (74%), neomycin (76%), amikacin (77%), ceftiofur (79%), and chloramphenicol (83%) compared to all other ophthalmic antibiotics tested except ciprofloxacin (69%). Minimum inhibitory concentrations required to inhibit the growth of 50 or 90% of organisms (MIC50 and MIC90, respectively) are described in Table 3. For the systemic susceptibility profile, the highest efficacy was seen with vancomycin (90%), imipenem (86%), and amikacin (80%), while the lowest efficacy was seen with pradofloxacin (0%), cephalexin (23%), and orbifloxacin (30%) (Table 4).
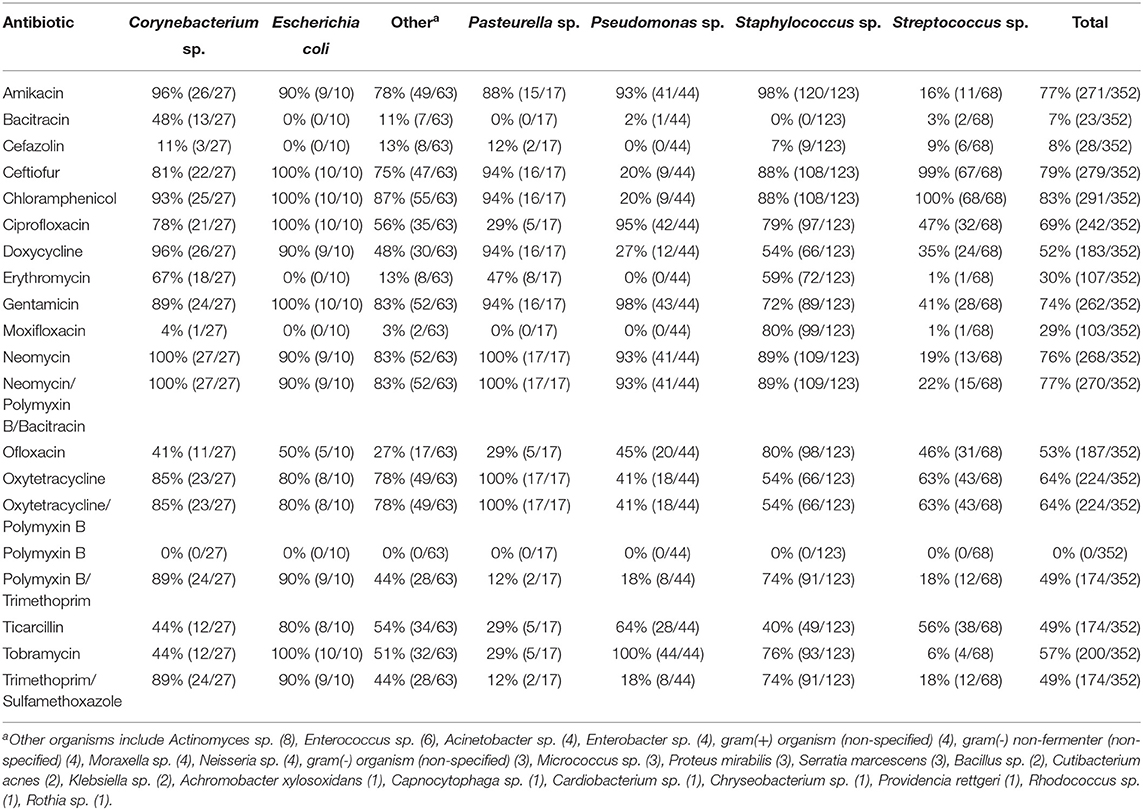
Table 2. In vitro susceptibility of 352 bacterial isolates to potential topical ophthalmic antibiotics.
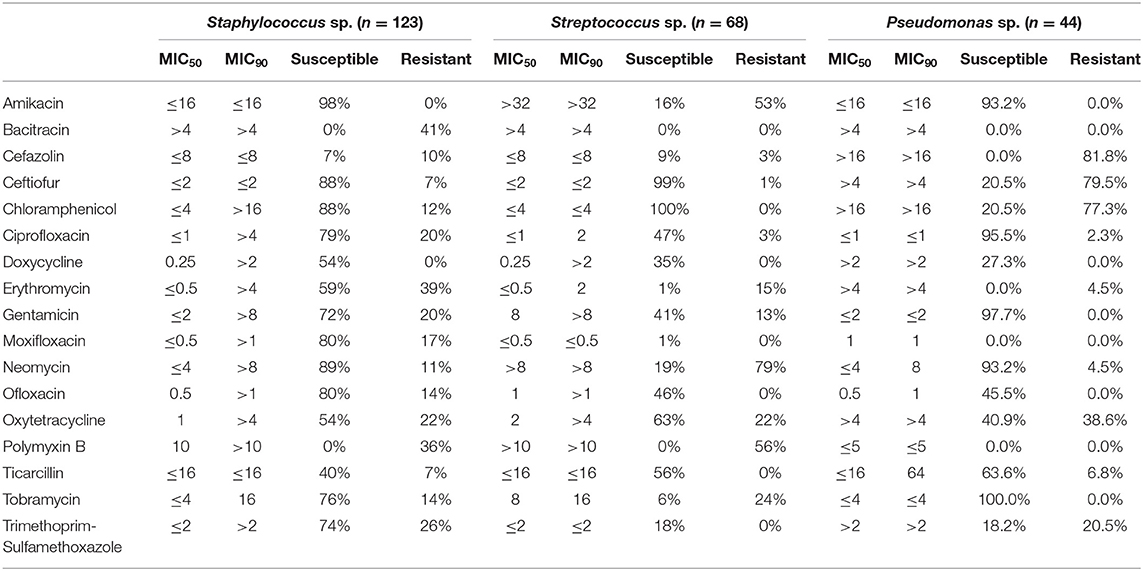
Table 3. Minimal inhibitory concentrations (MIC50 and MIC90) of ophthalmic antibiotics for the most prevalent bacterial genera isolated in dogs with bacterial keratitis.
Combination Therapies
Table 5 describes the efficacy of combining two antibiotic formulations (reported in the ophthalmic susceptibility) against all bacterial isolates identified in the study for which an ophthalmic panel was available (n = 352), considering individual antibiotics as well as commercially available combination formulations. The most effective combinations were amikacin-chloramphenicol (98% susceptible), chloramphenicol-neomycin (98%), and amikacin-ceftiofur (97%), while the least effective combinations were bacitracin-polymyxin B (7% susceptible), cefazolin-polymyxin B (8%), and bacitracin-cefazolin (13%).
Multi-Drug Resistant Isolate Characteristics
Bacteria considered MDR represented 20% (69/352) of all canine isolates. Specifically, MDR strains were identified in 33% (40/123) of Staphylococcus sp., 25% (17/68) of Streptococcus sp., 19% (5/27) of Corynebacterium sp., 10% (1/10) of E. coli, 0% (0/44) of Pseudomonas sp., 0% (0/17) of Pasteurella sp., and 10% (6/63) of all other isolates. Notably, the incidence of MDR increased over the last 5 years of data collection, with MDR strains representing 5% (2/42) of all bacteria isolated in 2016, 10% (13/126) in 2017, 22% (12/54) in 2018, 32% (17/53) in 2019, and 34% (11/32) in 2020. Dogs with a high prevalence of MDR isolates included shih tzus (13/46, 28%), mixed breed (10/23, 43%), pugs (3/9, 33%), and Boston terriers (2/16, 13%). Compared to mixed breed dogs, Pomeranians and Saint Bernards were significantly more likely to yield a MDR isolate when bacterial growth was present, with 3/3 isolates classified as MDR for Pomeranians (OR = ∞, P = 0.049) and 4/4 isolates classified as MDR for Saint Bernards (OR = ∞, P = 0.019).
Discussion
In the present study, the three most common bacterial genera isolated from dog corneas were Staphylococcus (32.3%), Streptococcus (19.1%), and Pseudomonas (12.5%). These findings are generally in agreement with other canine studies across the globe (2, 3, 9–11), with subtle geographic differences such as a lower prevalence of Staphylococcus species in Australia (8) or a relatively higher prevalence of Streptococcus species in the Midwestern United States (present study) compared to other locations (6, 7). Staphylococcus pseudintermedius was the most common Staphylococcus species (26.7% of all isolates), as recognized in most canine reports (2, 3, 7, 10, 11), followed by non-specified Coagulase-negative Staphylococcus species (3%) and Staphylococcus epidermidis (0.6%). Surprisingly, Staphylococcus aureus only accounted for 0.4% of isolates in the present study, while it had previously been reported as more prevalent in canine bacterial keratitis (6, 9). Streptococcus canis (12%) and Pseudomonas aeruginosa (7.5%) were the most common species cultured for each respective genus, consistent with previous reports (3, 8, 10, 11).
As compared to mixed-breed dogs, one canine breed (Labrador retriever) was found to be less likely to present to the Ophthalmology service with bacterial keratitis—a finding presumably related to the higher proportion of eye certification exams in Labradors—while eight canine breeds were found to be at higher risk. Over-represented dogs included brachycephalic breeds (Boston terrier, Cavalier King Charles spaniel, pug, and shih tzu), as previously reported (2, 8, 9), but also non-brachycephalic breeds such as Saint Bernard, miniature pinscher, rat terrier, and silky terrier. Further, the study assessed the seasonality of bacterial isolates and found that summer increased the risk of yielding a positive bacterial culture from canine corneas, notably Pseudomonas species. Summer was also shown to affect the prevalence of selected bacterial isolates in humans (22). UV light is a known risk factor for potentiating infections in bovine eyes (23), resulting in degenerative changes to corneal epithelial cells that allow for easier bacterial colonization (24, 25), and the same may be true in dogs. Awareness of seasonal variation might inform clinical recommendations and prevention strategies, and it is therefore advised to report seasonality in bacteriological studies.
Appropriate management of corneal ulcers requires an understanding of common bacterial isolates and associated susceptibilities to antibiotics. Monotherapy (i.e., individual drug or combination drug such as triple antibiotic) is generally advised for ulcers characterized as “simple” (superficial, no gross signs of infection), while combination therapy with 2 antibiotic formulations is generally suggested for “complicated” corneal ulcers with active signs of infection (e.g., stromal loss, keratomalacia, cellular infiltrates) to provide broad spectrum coverage for both gram-positive and gram-negative bacteria (12–15). Further, the complementary use of a systemic antibiotic can be considered in well-vascularized corneal lesions and corneal perforations (at risk for endophthalmitis), or when systemic administration achieves tear film concentrations above minimal inhibitory concentrations for common bacterial isolates. The latter was demonstrated for various antibiotics administered parenterally to cows (i.e., oxytetracycline, chloramphenicol, gentamicin, and erythromycin) (26) but has not been documented in companion animals to date. The summary information detailed in the manuscript's tables provide a quick reference for clinicians that manage bacterial keratitis in canine patients.
Individual antibiotics with the highest efficacy rates included chloramphenicol (83%), ceftiofur (79%), amikacin (77%), neomycin (76%), and gentamicin (74%). When considering commercially available formulations, the best option remains triple antibiotic (neomycin-polymxin B-bacitracin) with 77% efficacy, consistent with general guidelines to use this medication for prophylactic use in non-infected ulcers (4, 5, 13, 27), followed by gentamicin (4, 13). Other options for empirical monotherapy include chloramphenicol, ceftiofur, and amikacin (≥77% efficacy), however chloramphenicol and amikacin require compounding in the US, while ceftiofur is only marketed for horses and production animals as an injectable formulation for parenteral use, with proven clinical efficacy for cattle with infectious bovine keratoconjunctivitis (28). Chloramphenicol and ceftiofur were highly effective against Streptococcus and Staphylococcus species (≥88%), but their efficacy against Pseudomonas was limited (20%). Preferred antibiotics for Pseudomonas species included ciprofloxacin (95%) and aminoglycosides (amikacin, gentamicin, neomycin) with ≥93% efficacy. It is important to note that another 2nd-generation fluoroquinolone (ofloxacin) had relatively limited efficacy against Pseudomonas species in the present study (45%), consistent with the trend described in some veterinary and human studies (29, 30). As expected, the MIC50 and MIC90 values for Pseudomonas sp. and cephalosporins (cefazolin and ceftiofur) were higher than MIC values detected for Staphylococcus and Streptococcus species; further, MIC50 and MIC90 values for Streptococcus sp. and aminoglycosides (amikacin, gentamicin, neomycin, and tobramycin) were higher than MIC values identified for Staphylococcus or Pseudomonas isolates, as recently described in canine and equine patients (31).
Combination antibiotic therapies are routinely used in practice to provide broad-spectrum coverage for suspected infected corneal ulcers (12–15), yet the efficacy of combined therapy is not addressed in previous bacteriological studies. A strong combination therapy highlighted in the present study is chloramphenicol (Staphylococcus, Streptococcus, Corynebacterium, and “others” coverage) and ciprofloxacin (Pseudomonas coverage), two drugs with good penetration into corneal tissues (32) and excellent efficacy against common bacterial isolates in dogs when used together (97%). Other strong combinations include chloramphenicol-amikacin (98%), chloramphenicol-tobramycin (97%), amikacin-ceftiofur (97%), chloramphenicol-gentamicin (96%), and ciprofloxacin-gentamicin (96%). Importantly, adjustments to combination therapies should be considered when culture and sensitivity results are obtained, avoiding the unnecessary use of antibiotics, and reducing ocular surface toxicity from excessive use of preservative-containing ophthalmic solutions.
Systemic antibiotics can be used to complement topical antibiotic therapy in dogs with bacterial keratitis if the lesion is vascularized or the cornea is perforated. Amoxicillin-clavulanic acid was very effective for gram-positive organisms (≥83% efficacy) (16), while aminoglycosides and other selected antibiotics (ceftazidime, marbofloxacin, and piperacillin) were most effective against gram-negative organisms (≥76% efficacy). Antibiotics generally considered “last-line” therapies such as vancomycin (90%) and imipenem (86%) were highly effective against most bacterial isolates.
Antimicrobial resistance is a serious concern in veterinary ophthalmology (33). The overall prevalence of MDR isolates was relatively high (20%) in the present study, with Pomeranians and Saint Bernards being significantly more likely to yield MDR isolates when presented to our Ophthalmology service with corneal ulcers. The high prevalence of MDR isolates in these two canine breeds is puzzling and may simply be related to low sample size; alternatively, it is possible the microbiome differs among canine breeds due to peculiarities in ocular surface anatomy and physiology. Interestingly, none (0%) of Pseudomonas isolates were considered MDR in this study, likely due to extensive intrinsic resistance profile for this bacterial genera in veterinary medicine (18, 21); of note, most (>90%) Pseudomonas isolates were susceptible to aminoglycosides and ciprofloxacin as previously described (7, 34). Further, we noted an alarming, escalating trend of multi-drug resistance over the last 5 years of the study (5% in 2016 to 34% in 2020). MDR is also prevalent in human patients with bacterial keratitis (35, 36). The “Antibiotic Resistance Monitoring in Ocular Microorganisms” (ARMOR) studies in humans have provided invaluable information for clinicians managing bacterial keratitis in practice, and the same collaborative effort is critically needed in veterinary medicine to mitigate the rise of MDR in animals. Such work could promote antimicrobial stewardship at a regional- or clinic-level, decreasing the rate of MDR through judicious use of ophthalmic antibiotics in practice.
The present work focused on aerobic bacterial cultures in dogs with ulcerative keratitis. Depending on the clinical appearance of the lesion, clinicians should also consider anaerobic and/or fungal cultures to increase the likelihood of identifying the causative agent (37, 38). In fact, diagnostic tests other than culture-based methods will soon become the gold standard for microbial species identification as the field of clinical microbiology is rapidly evolving. Unlike culture-based methods, technologies such as mass spectrometry and nucleic acid sequencing provide rapid and sensitive tools to probe the microbiome in clinical patients (39)—as recently described for the ocular surface of veterinary species (40, 41)—enabling clinicians to optimize the antibiotic treatment sooner and thereby improve clinical outcomes (42).
Similar to previous reports of antibiotic susceptibility profiles in veterinary medicine, the main limitation of the present study is the reliance on incomplete veterinary specific CLSI guidelines to determine whether a bacterial isolate is susceptible or resistant to a given antibiotic. In veterinary CLSI guidelines, the lack of interpretative breakpoints for selected bacteria/antibiotic combinations yields a “non-interpretable” clinical interpretation in the antibiogram. Such missing information may be due to intrinsic resistances, or the absence of pharmacokinetics-pharmacodynamics studies that assess the concentration of a given antibiotic at the target tissue and determine whether such levels are above the minimal inhibitory concentration (MIC) of a given bacterial species. Findings presented herein likely underestimate the true susceptibility rates as some of the “non-interpretable” results may in fact be sensitive in clinical practice. For instance, moxifloxacin susceptibility rates were generally low in the present study because 64% of moxifloxacin results were reported as “non-interpretable” by the CLSI guidelines, while in practice this fourth-generation fluoroquinolone is considered superior to ofloxacin and ciprofloxacin for most bacterial isolates (43). Similarly, polymyxin B, cefazolin, and bacitracin also had high numbers of “non-interpretable” reports (72, 71, and 55%, respectively). On the other hand, caution must be exercised when clinical guidelines provide an actual interpretation of “susceptible” or “resistant.” A closer look at the MIC data provided by the ophthalmic panel (JOEYE2 plate) shows that the concentration tested for selected antibiotics (e.g., 4 μg/mL for chloramphenicol/neomycin/tobramycin, 16 μg/mL for amikacin/ticarcillin) is relatively high when compared to drug levels achieved in canine tear film. Indeed, topical drug delivery achieves high concentrations in the short-term but concentrations on the canine ocular surface rapidly decrease due to efficient drainage through the nasolacrimal duct (44–46).
In conclusion, the bacterial profile from corneal cultures in Iowa and surrounding Midwestern United States followed world-wide trends with high proportion of Staphylococcus, Streptococcus, and Pseudomonas species. Characteristics of bacterial keratitis in dogs were influenced by season and canine breeds. The rate of multi-drug resistance was relatively high, notably for Staphylococcus isolates, with an alarming escalating trend over time. Appropriate selection of empiric antibiotic therapy is important to enhance therapeutic outcome and reduce antibacterial resistance in dogs with corneal ulceration, whether using individual or combination drug therapy. Subsequently, clinicians' selection of antibiotics should be guided by the antibiogram received for each given patient, requiring adjustments to the empirical therapy initiated earlier in selected cases.
Data Availability Statement
The raw data supporting the conclusions of this article will be made available by the authors, without undue reservation.
Ethics Statement
Ethical review and approval was not required for the animal study because the work was a retrospective analysis of bacterial cultures collected in dogs as part of the patients' standard of care. Written informed consent for participation was not obtained from the owners because the work was a retrospective analysis of bacterial cultures collected in dogs as part of the patients' standard of care.
Author Contributions
LS and RA conceptualized and designed the study in consultation with JH and DK. LS and JH analyzed the data. All authors wrote the manuscript.
Conflict of Interest
The authors declare that the research was conducted in the absence of any commercial or financial relationships that could be construed as a potential conflict of interest.
Acknowledgments
The authors would like to thank Poonam Dubey for her assistance with data retrieval, as well as Dr. Orhan Sahin for his aid in microbiological interpretations.
References
1. McDonald PJ, Watson ADJ. Microbial-flora of normal canine conjunctivae. J Small Anim Practice. (1976) 17:809–12. doi: 10.1111/j.1748-5827.1976.tb06947.x
2. Prado MR, Rocha MEG, Brito EHS, Girao MD, Monteiro AJ, Teixeira MES, et al. Survey of bacterial microorganisms in the conjunctival sac of clinically normal dogs and dogs with ulcerative keratitis in Fortaleza, Ceara, Brazil. Vet Ophthalmol. (2005) 8:33–7. doi: 10.1111/j.1463-5224.2005.04061.x
3. Wang L, Pan QS, Zhang LB, Xue Q, Cui J, Qi CM. Investigation of bacterial microorganisms in the conjunctival sac of clinically normal dogs and dogs with ulcerative keratitis in Beijing, China. Vet Ophthalmol. (2008) 11:145–9. doi: 10.1111/j.1463-5224.2008.00579.x
4. Ollivier FJ. Bacterial corneal diseases in dogs and cats. Clin Techniq Small Anim Practice. (2003) 18:193–8. doi: 10.1016/S1096-2867(03)90016-8
5. Belknap EB. Corneal emergencies. Top Compan Anim Med. (2015) 30:74–80. doi: 10.1053/j.tcam.2015.07.006
6. Lin CT, Petersen-Jones SM. Antibiotic susceptibility of bacterial isolates from corneal ulcers of dogs in Taiwan. J Small Anim Practice. (2007) 48:271–4. doi: 10.1111/j.1748-5827.2007.00348.x
7. Ekapopphan D, Srisutthakarn A, Moonarmart W, Buddhirongawatr R, Bangphoomi N. Identification and antimicrobial susceptibility of microorganisms isolated from severe corneal ulcers of dogs in Thailand. J Vet Med Sci. (2018) 80:1259–65. doi: 10.1292/jvms.18-0045
8. Hindley KE, Groth AD, King M, Graham K, Billson FM. Bacterial isolates, antimicrobial susceptibility, and clinical characteristics of bacterial keratitis in dogs presenting to referral practice in Australia. Vet Ophthalmol. (2016) 19:418–26. doi: 10.1111/vop.12325
9. Suter A, Voelter K, Hartnack S, Spiess BM, Pot SA. Septic keratitis in dogs, cats, and horses in Switzerland: associated bacteria and antibiotic susceptibility. Vet Ophthalmol. (2018) 21:66–75. doi: 10.1111/vop.12480
10. Tolar EL, Hendrix DVH, Rohrbach BW, Plummer CE, Brooks DE, Gelatt KN. Evaluation of clinical characteristics and bacterial isolates in dogs with bacterial keratitis: 97 cases (1993–2003). JAVMA. (2006) 228:80–5. doi: 10.2460/javma.228.1.80
11. Auten CR, Urbanz JL, Dees DD. Comparison of bacterial culture results collected via direct corneal ulcer vs. conjunctival fornix sampling in canine eyes with presumed bacterial ulcerative keratitis. Vet Ophthalmol. (2020) 23:135–40. doi: 10.1111/vop.12698
12. Baum JL. Initial therapy of suspected microbial corneal ulcers 1. Broad Antibiotic Ther Based Prevalence Organ Survey Ophthalmol. (1979) 24:97–105. doi: 10.1016/0039-6257(79)90127-9
13. Kern TJ. Ulcerative keratitis. Vet Clin North Am Small Anim Practice. (1990) 20:643–66. doi: 10.1016/S0195-5616(90)50055-8
14. de Bustamante MGM, Good KL, Leonard BC, Hollingsworth SR, Edwards SG, Knickelbein KE, et al. Medical management of deep ulcerative keratitis in cats: 13 cases. J Feline Med Surg. (2019) 21:387–93. doi: 10.1177/1098612X18770514
15. Guyonnet A, Desquilbet L, Faure J, Bourguet A, Donzel E, Chahory S. Outcome of medical therapy for keratomalacia in dogs. J Small Anim Practice. (2020) 61:253–8. doi: 10.1111/jsap.13118
16. Kim JY, Won HJ, Jeong SW. A retrospective study of ulcerative keratitis in 32 dogs. Int J Appl Res Vet Med. (2009) 7:27–31.
17. Ion L, Ionascu I, Birtoiu A. Melting keratitis in dogs and cats. Conference Agri Life Life Agri. (2015) 6:342–9. doi: 10.1016/j.aaspro.2015.08.090
18. CLSI. Performance Standards for Antimicrobial Disk and Dilution Susceptibility Tests for Bacteria Isolated From Animals; 4th Informational Supplement. (Wayne, PA: CLSI). (2018).
19. CLSI. Performance Standards for Antimicrobial Susceptibility Testing, 28th Edn. (Wayne, PA: CLSI). (2019).
20. Schwarz S, Silley P, Simjee S, Woodford N, van Duijkeren E, Johnson AP, et al. Editorial: assessing the antimicrobial susceptibility of bacteria obtained from animals. J Antimicrob Chemother. (2010) 65:601–4. doi: 10.1093/jac/dkq037
21. Sweeney MT, Lubbers BV, Schwarz S, Watts JL. Applying definitions for multidrug resistance, extensive drug resistance and pandrug resistance to clinically significant livestock and companion animal bacterial pathogens. J Antimicrob Chemother. (2018) 73:1460–3. doi: 10.1093/jac/dky043
22. Perencevich EN, McGregor JC, Shardell M, Furuno JP, Harris AD, Morris JG, et al. Summer peaks in the incidences of gram-negative bacterial infection among hospitalized patients. Infect Contr Hosp Epidemiol. (2008) 29:1124–31. doi: 10.1086/592698
23. Lepper AWD, Barton IJ. Infectious bovine keratoconjunctivitis—seasonal-variation in cultural, biochemical and immunoreactive properties of moraxella-bovis isolated from the eyes of cattle. Austr Vet J. (1987) 64:33–9. doi: 10.1111/j.1751-0813.1987.tb16125.x
24. Vogelweid CM, Miller RB, Berg JN, Kinden DA. Scanning electron-microscopy of bovine corneas irradiated with sun lamps and challenge exposed with moraxella-bovis. Am J Vet Res. (1986) 47:378–84.
25. Postma GC, Carfagnini JC, Minatel L. Moraxella bovis pathogenicity: an update. Comp Immunol Microbiol Infect Dis. (2008) 31:449–58. doi: 10.1016/j.cimid.2008.04.001
26. Punch PI, Costa ND, Chambers ED, Slatter DH, Wilcox GE. Plasma and tear concentrations of antibiotics administered parenterally to cattle. Res Vet Sci. (1985) 39:179–87. doi: 10.1016/S0034-5288(18)31742-9
27. Kern TJ. Antibacterial agents for ocular therapeutics. Vet Clin North Am Small Anim Practice. (2004) 34:655. doi: 10.1016/j.cvsm.2003.12.010
28. Dueger EL, George LW, Angelos JA, Tankersley NS, Luiz KM, Meyer JA, et al. Efficacy of a long-acting formulation of ceftiofur crystalline-free acid for the treatment of naturally occurring infectious bovine keratoconjunctivitis. Am J Vet Res. (2004) 65:1185–8. doi: 10.2460/ajvr.2004.65.1185
29. Peterson LR, Postelnick M, Pozdol TL, Reisberg B, Noskin GA. Management of fluoroquinolone resistance in Pseudomonas aeruginosa—outcome of monitored use in a referral hospital. Int J Antimicrob Agents. (1998) 10:207–14. doi: 10.1016/S0924-8579(98)00045-4
30. LaBorwit SE, Katz HR, Hirschbein MJ, Oswald MR, Snyder LS, Schwartz KS, et al. Topical 0.3% ciprofloxacin vs. topical 0.3% ofloxacin in early treatment of Pseudomonas aeruginosa keratitis in a rabbit model. Ann Ophthalmol. (2001) 33:48–52. doi: 10.1007/s12009-001-0055-4
31. Jinks MR, Miller EJ, Diaz-Campos D, Mollenkopf DF, Newbold G, Gemensky-Metzler A, et al. Using minimum inhibitory concentration values of common topical antibiotics to investigate emerging antibiotic resistance: A retrospective study of 134 dogs and 20 horses with ulcerative keratitis. Vet Ophthalmol. (2020). doi: 10.1111/vop.12801. [Epub ahead of print].
33. Soimala T, Lübke-Becker A, Hanke D, Eichhorn I, Feßler AT, Schwarz S, et al. Molecular and phenotypic characterization of methicillin-resistant Staphylococcus pseudintermedius from ocular surfaces of dogs and cats suffering from ophthalmological diseases. Vet Microbiol. (2020) 244:108687. doi: 10.1016/j.vetmic.2020.108687
34. Thomas RK, Melton R, Asbell PA. Antibiotic resistance among ocular pathogens: current trends from the ARMOR surveillance study (2009–2016). Clin Optometry. (2019) 11:15–26. doi: 10.2147/OPTO.S189115
35. Oldenburg CE, Lalitha P, Srinivasan M, Rajaraman R, Ravindran M, Mascarenhas J, et al. Emerging moxifloxacin resistance in Pseudomonas aeruginosa keratitis isolates in South India. Ophthal Epidemiol. (2013) 20:155–8. doi: 10.3109/09286586.2013.790978
36. Asbell PA, Sanfilippo CM, Pillar CM, DeCory HH, Sahm DF, Morris TW. Antibiotic resistance among ocular pathogens in the United States five-year results from the antibiotic resistance monitoring in ocular microorganisms (ARMOR) surveillance study. Jama Ophthalmol. (2015) 133:1445–54. doi: 10.1001/jamaophthalmol.2015.3888
37. Ledbetter EC, Scarlett JM. Isolation of obligate anaerobic bacteria from ulcerative keratitis in domestic animals. Vet Ophthalmol. (2008) 11:114–22. doi: 10.1111/j.1463-5224.2008.00610.x
38. Scott EM, Carter RT. Canine keratomycosis in 11 dogs: a case series (2000–2011). J Am Anim Hosp Assoc. (2014) 50:112–8. doi: 10.5326/JAAHA-MS-6012
39. van Belkum A, Durand G, Peyret M, Chatellier S, Zambardi G, Schrenzel J, et al. Rapid clinical bacteriology and its future impact. Ann Lab Med. (2013) 33:14–27. doi: 10.3343/alm.2013.33.1.14
40. Leis ML, Costa MO. Initial description of the core ocular surface microbiome in dogs: bacterial community diversity and composition in a defined canine population. Vet Ophthalmol. (2019) 22:337–44. doi: 10.1111/vop.12599
41. Rogers CM, Scott EM, Sarawichitr B, Arnold C, Suchodolski JS. Evaluation of the bacterial ocular surface microbiome in ophthalmologically normal dogs prior to and following treatment with topical neomycin-polymyxin-bacitracin. PLoS ONE. (2020) 15:e0234313. doi: 10.1371/journal.pone.0234313
42. Galar A, Leiva J, Espinosa M, Guillén-Grima F, Hernáez S, Yuste JR. Clinical and economic evaluation of the impact of rapid microbiological diagnostic testing. J Infect. (2012) 65:302–9. doi: 10.1016/j.jinf.2012.06.006
43. Kowalski RP, Dhaliwal DK, Karenchak LM, Romanowski EG, Mah FS, Ritterband DC, et al. Gatifloxacin and moxifloxacin: an in vitro susceptibility comparison to levofloxacin, ciprofloxacin, and ofloxacin using bacterial keratitis isolates. Am J Ophthalmol. (2003) 136:500–5. doi: 10.1016/S0002-9394(03)00294-0
44. Sebbag L, Allbaugh RA, Wehrman RF, Uhl LK, Ben-Shlomo G, Chen T, et al. Fluorophotometric assessment of tear volume and turnover rate in healthy dogs and cats. J Ocular Pharmacol Therap. (2019) 35:497–502. doi: 10.1089/jop.2019.0038
45. Sebbag L, Kirner NS, Allbaugh RA, Reis A, Mochel JP. Kinetics of fluorescein in tear film after eye drop instillation in beagle dogs: does size really matter? Front Vet Sci. (2019) 6:9. doi: 10.3389/fvets.2019.00457
Keywords: bacterial keratitis, canine, topical antibiotic, systemic antibiotic, antibiotic resistance, multidrug resistance
Citation: Hewitt JS, Allbaugh RA, Kenne DE and Sebbag L (2020) Prevalence and Antibiotic Susceptibility of Bacterial Isolates From Dogs With Ulcerative Keratitis in Midwestern United States. Front. Vet. Sci. 7:583965. doi: 10.3389/fvets.2020.583965
Received: 16 July 2020; Accepted: 12 October 2020;
Published: 20 November 2020.
Edited by:
Roswitha Merle, Freie Universität Berlin, GermanyReviewed by:
Johanna Corinna Eule, Freie Universität Berlin, GermanyFabrizio Passamonti, University of Perugia, Italy
Copyright © 2020 Hewitt, Allbaugh, Kenne and Sebbag. This is an open-access article distributed under the terms of the Creative Commons Attribution License (CC BY). The use, distribution or reproduction in other forums is permitted, provided the original author(s) and the copyright owner(s) are credited and that the original publication in this journal is cited, in accordance with accepted academic practice. No use, distribution or reproduction is permitted which does not comply with these terms.
*Correspondence: Lionel Sebbag, bHNlYmJhZ0BpYXN0YXRlLmVkdQ==