- 1Livestock Production Sciences Branch, Agri-Food and Biosciences Institute, Hillsborough, United Kingdom
- 2Institute for Global Food Security, School of Biological Sciences, Queens University Belfast, Belfast, United Kingdom
The UK dairy herd is predominantly of the Holstein-Friesian (HF) breed, with a major emphasis placed on milk yield. Subsequently, following years of continued single-trait selection, the beef production potential of dairy bred calves has declined. Thus, male HF calves are commonly seen as a by-product of the dairy industry. Limited markets, perceived low economic value and high rearing costs mean that these surplus calves are often euthanised shortly after birth or exported to the EU for further production. Welfare concerns have been raised regarding both euthanasia and long distance transportation of these calves. Furthermore, total UK beef consumption increased by 8.5% from 2009 to 2019. Thus, in light of this growing demand, beef from the dairy herd could be better utilized within the UK. Therefore, the potential for these calves to be used in a sustainable, cost-effective beef production system with high welfare standards within the UK requires investigation. Thus, the aim of this review was to evaluate both steer and bull beef production systems, examining the impact on performance, health, welfare, and economic potential to enable a sustainable farming practice, while meeting UK market requirements. The principal conclusions from this review indicate that there is the potential for these calves to be used in UK based production systems and meet market requirements. Of the steer production systems, a 24 month system appears to achieve a balance between input costs, growth from pasture and carcass output, albeit the literature is undecided on the optimum system. The situation is similar for bull beef production systems, high input systems do achieve the greatest gain in the shortest period of time, however, these systems are not sustainable in volatile markets with fluctuating concentrate prices. Thus, again the inclusion of a grazing period, may increase the resilience of these systems. Furthermore, production systems incorporating a period at pasture are seen to have animal welfare benefits. The main welfare concern for surplus dairy bred calves is often poor colostrum management at birth. While in steer systems, consideration needs to be given to welfare regarding castration, with the negative impacts being minimized by completing this procedure soon after birth.
Introduction
Currently the UK dairy herd consists of 1.867 million cows (1) and is predominantly of the Holstein-Friesian (HF) breed. The main focus of the HF breed is maximizing milk yield and subsequently, following years of continued single-trait selection (2), annual milk yield per cow has increased by 14.1% in the 10 year period to 2018 (3). However, this has had a detrimental effect on other traits (e.g. functional traits, reproduction and health) and thus the beef production potential of dairy bred calves has declined, particularly in terms of carcass conformation (4, 5). Therefore, male HF calves are commonly seen as a by-product of the dairy industry (6, 7) due to limited markets and perceived low economic value (8). As a result the neonatal care of these surplus male calves is often inferior to that which heifer calves receive. Heifer calves are seen as the future of the dairy herd, and therefore, are often given priority to ensure their future productivity (9). Thus, dairy bred bull calves are at a greater risk of not receiving a sufficient quantity of quality colostrum within 24 h of birth, resulting in increased risk of failure of passive transfer (FPT) of antibodies (10). High levels of pre-wean mortality are associated with FPT, in addition to increased morbidity post-weaning, and reduced live weight gain (LWG) (11, 12).
Euthanasia soon after birth is occasionally used as a means of removing these calves from the herd, for example, in 2018 it was reported that 15% of male dairy sired calves were euthanised at birth in Great Britain (13). The high labor requirement associated with rearing, together with high rearing costs, which have been reported at £195.19 per calf from birth to weaning, mean there is little economic incentive to rear these calves when the monetary return is minimal (14, 15). This is a problem that exists worldwide. For example in New Zealand and Australia, surplus dairy calves are referred to as bobby calves and it is estimated that 2.2 million bobby calves are slaughtered for meat processing between 4 and 7 days of age in New Zealand each year (16). In the UK an alternative outlet for a large number of male dairy calves is the export market to the EU for further production such as intensive veal or bull beef. For example, in Northern Ireland a 3 year average of 19,863 calves of under 42 days of age were exported to the EU from 2015 to 2017. The vast majority of these were exported to Spain (17), where they may be finished as bull beef for the domestic market, or further exported as store cattle to non-EU countries (18). This is considered a valuable market for the industry and vastly reduces the need for euthanasia (17, 19). The live export of cattle goes beyond the UK and is a common occurrence across Europe, for example in 2017 the intra-EU live cattle trade consisted of over 3.86 million cattle, 53% of which were intended for immediate slaughter. While live cattle exported from the EU to non-EU countries was estimated to be 0.80 million cattle in 2017 (20). Unsurprisingly, animal welfare and ethical considerations have been raised regarding such live animal exports. Concerns such as journey times, animals not being given rest periods, overcrowding and poor provision of feed and water have all been highlighted (21). Research has shown that long-haul transport with deprivation of food and water can result in an increased heart rate together with implications for liver function (22). In contrast Grigor et al. (23) reported that there was no major detrimental welfare impact when calves were transported under good conditions with access to feed, water, milk replacer, although the authors did suggest that there was some negative impact on calf health status post transport. Within the UK the exporting of live animals is regulated under the Welfare of Animals (Transport) Order 2006 (24). Inspections are conducted regularly and compliance rates within the UK are reported to be high. For example in 2015 and 2016 the mean non-compliance rate for cattle was 0.06% (17). Nevertheless, live exports have received a great deal of media attention, with calls for this practice to be banned (21). Furthermore, as a result of Brexit, the UK government may now have the opportunity to impose a ban on live animal exports. Alternatively, live exports may become more heavily regulated or see additional challenges associated with market or trade agreements for transporting animals across borders (25). However, the true impact that Brexit will have is yet to be determined. In addition, the environmental impact of transporting live animals such long distances has raised concerns, with one study showing that it is more environmentally sustainable, due to reduced CO2 and NOx emissions, to produce and slaughter lambs in their country of origin and export the carcasses, rather than operating live exports (26).
By definition in the UK, veal is meat from bovine animals slaughtered under 8 months of age. Whereas, beef is from bovine animals slaughtered over 8 months of age (27). However, in the UK the market for veal meat is small, with only 143,000 calves being slaughtered for veal in 2018 (28). Furthermore, public awareness of the product is limited and perceptions are often influenced by welfare concerns such as slaughter age (29). Therefore, the potential for these calves to be used in a sustainable, cost-effective beef production system with high welfare standards [regulated under the Welfare of Farmed Animals Regulations 2007 (30)] within their country of origin requires investigation. Here we review the literature, including examples from our own research, on the beef potential of these surplus male calves. This includes an evaluation of different production systems examining the animal performance and economic potential to enable a sustainable farming practice, while meeting the market requirements of the UK beef industry. In addition, the animal health and welfare implications are also evaluated.
Steer Beef Production
Of the prime beef (cattle produced for the sole purpose of beef production) slaughtered in the UK in 2019, 50.9% were steers, while only 9.6% were young bulls, while the remaining 39.5% were heifers (31). A 24 months steer system, where cattle spend two seasons at pasture and are slaughtered following an indoor finishing period (32, 33), is thought to be a relatively low cost system, that gains a high proportion of growth from pasture (34). For example, Murphy et al. (35) reported HF steers growing at 0.80 and 0.93 kg/d during their first and second season at grass, respectively. These steers were finished indoors on ad libitum grass silage, supplemented with 5 kg dry matter (DM) of concentrate per head daily, achieving a slaughter weight, carcass weight and fat classification (on a 15 point scale) of 603 kg, 307 kg and 7.86, respectively. Similar levels of performance were achieved by McNamee et al. (36) with a LWG of 0.92 kg/d during the second grazing season and 1.45 kg/d during the finishing period [concentrates fed at 67% of dry matter intake (DMI) during finishing]. A summary of the key findings on steer finishing performance from published literature are presented in Table 1 however, the literature assessing Holstein steer production systems is relatively limited.
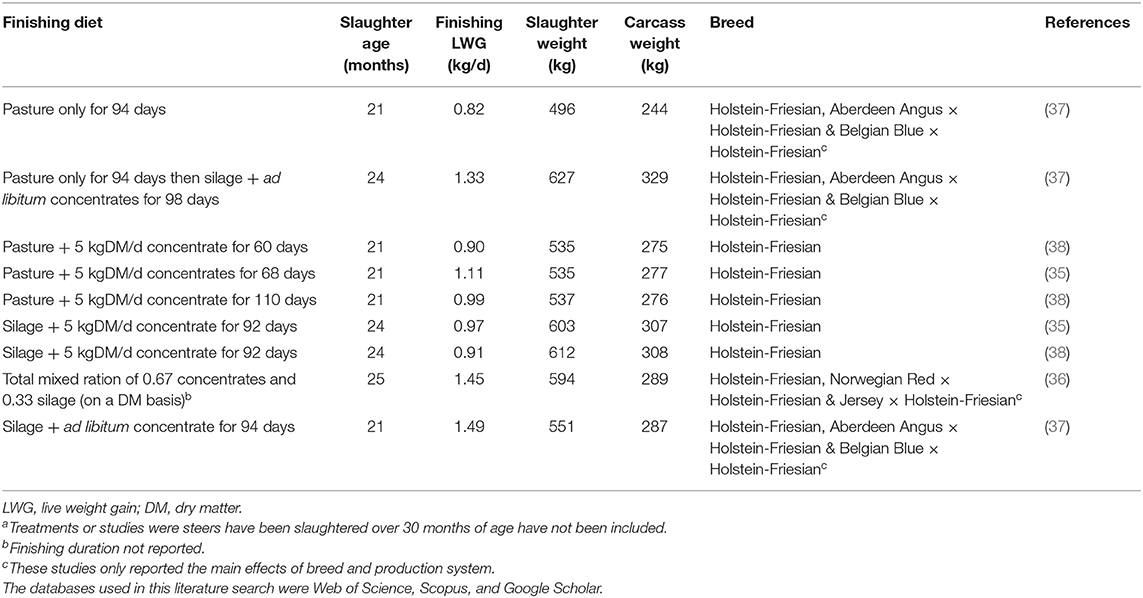
Table 1. Summary of key published researcha addressing the effect of finishing diet on steer performance.
Alternative production systems for steers involve earlier finishing, usually off pasture at the end of the second grazing season. Keane and Moloney (37) compared two early (~20 months) finishing strategies to the traditional 24 months system. The authors reported that finishing steers early lead to insufficient carcass weights and fat cover; while, ad libitum concentrates during the finishing period of the early strategy proved to be uneconomical. Thus, the most successful of the three systems was deemed to be the 24 months system when the grazing period was followed by a 3 months ad libitum finishing period; this system resulted in a lower concentrate intake per kg live weight (LW) than early ad libitum finishing (37). Murphy et al. (38), conducted a similar study and also found fat cover to be lower in a 21 months pasture finishing systems compared to a 24 months system. The reduced fat deposition at 21 months may be a limitation of implementing this system with the HF breed, due to their later maturing nature. Thus, a 21 months system may be more suitable for early maturing × dairy bred cattle such as Aberdeen Angus (37). Murphy et al. (38) also reported that slaughtering at 21 months following either a 60 or 120 days 5 kgDM concentrate supplementation period produced similar carcass weights of 275 and 276 kg, respectively, in comparison to 308 kg for a 24 months system (also offered 5 kgDM concentrate supplementation during indoor finishing). However, in contrast to Keane and Moloney (37) and Murphy et al. (38) reported that a 21 months system with a 60 days concentrate finishing period at pasture resulted in the greatest net profit margin (€55/head), while the 24 months steer system actually resulted in a net loss (–€29/head). Murphy et al. (38) took into consideration the additional land charge associated with keeping 24 months steers for an additional 3 months. The authors reported that the additional carcass gain achieved during this time was not sufficient to sustain the additional costs associated with land and feed. With feed costs accounting for a substantial proportion (75%) of the variable costs in beef production (39), it is important that a balance is reached between production costs and carcass output. Overall, the literature indicates that carcass output, concentrate costs, and stocking rate will largely determine the most economical steer beef production system. The environmental impact of a shorter steer system has also been considered. In the case of Murphy et al. (38) finishing steers at pasture with 60 days of concentrates resulted in an 11% reduction in GHG emissions per kg beef produced compared to a 24 months system. Yet, when the unit of measure is changed to GHG emissions per hectare that of the 21 months system is greater due to the greater stocking rate associated with the shorter production cycle.
UK steer market requirements dictate a maximum age of 30 months. However, the literature on Holstein steer systems largely focuses on slaughtering cattle at 21 and 24 months, and thus does not consider more extensive systems. Keane and Allen (40) compared an extensive (29 months) system to a conventional (24 months) system with Charolais × Friesian steers. Due to the study design, cattle were slaughtered at a target live weight and thus, carcass weights were consistent across the two systems. Interestingly, the authors summarized the inputs and outputs of the two systems, reporting that the extensive system had a greater gross profit margin per head [156 vs. 34 European Currency Unit (ECU)] and per hectare (229 vs. 71 ECU) than the conventional system. The lower production costs associated with the reduced concentrate intake in the extensive system was the main contributing factor (40). Thus, extensive production systems may have some merit as a Holstein beef system. However, from an environmental perspective further increasing the age at slaughter would be expected to result in a greater GHG output per head and per kg beef (35). With recent Net-Zero Carbon targets outlined, the livestock sector will have to consider both the economic and environmental sustainability of its production systems going forward (41).
Bull Beef Production
Characteristically, bulls have a much higher growth rate potential and improved feed efficiency than steers (6, 42, 43). Under UK market requirements, bulls are to be slaughtered under 16 months of age, with research demonstrating that the slaughter age of bulls will directly impact on a number of meat eating qualities (44). Bulls slaughtered outside of market specification will be subject to reduction in the price paid per kg carcass. These market requirements create both challenges and opportunities for bull beef production. In order to achieve desired carcass weights at 16 months, there is little room for setbacks in LWG during the production cycle (45). Bearing in mind that male dairy bred calves often have an inferior health status (10), this can result in longer term indirect effects associated with reduced lung function, feed efficiency and LWG (46). Therefore, ensuring target weights are achieved throughout the production system can often be a challenge for producers. One potential advantage of the current market requirements is that the shorter production cycle for bull beef results in a faster throughput of cattle on farm. This is particularly advantageous where the availability of land or housing are limiting factors. Furthermore, a 16 months bull beef system has been reported to result in lower greenhouse gas emissions, than a steer system, due to slaughtering animals at a younger age (35, 47). However, if the bull beef system relies on imported feed this can substantially increase greenhouse gas emissions in comparison to one which uses nationally or home grown feedstuffs (48). The key findings from published bull beef research are summarized in Table 2 and these will be discussed in the following three sub-sections.
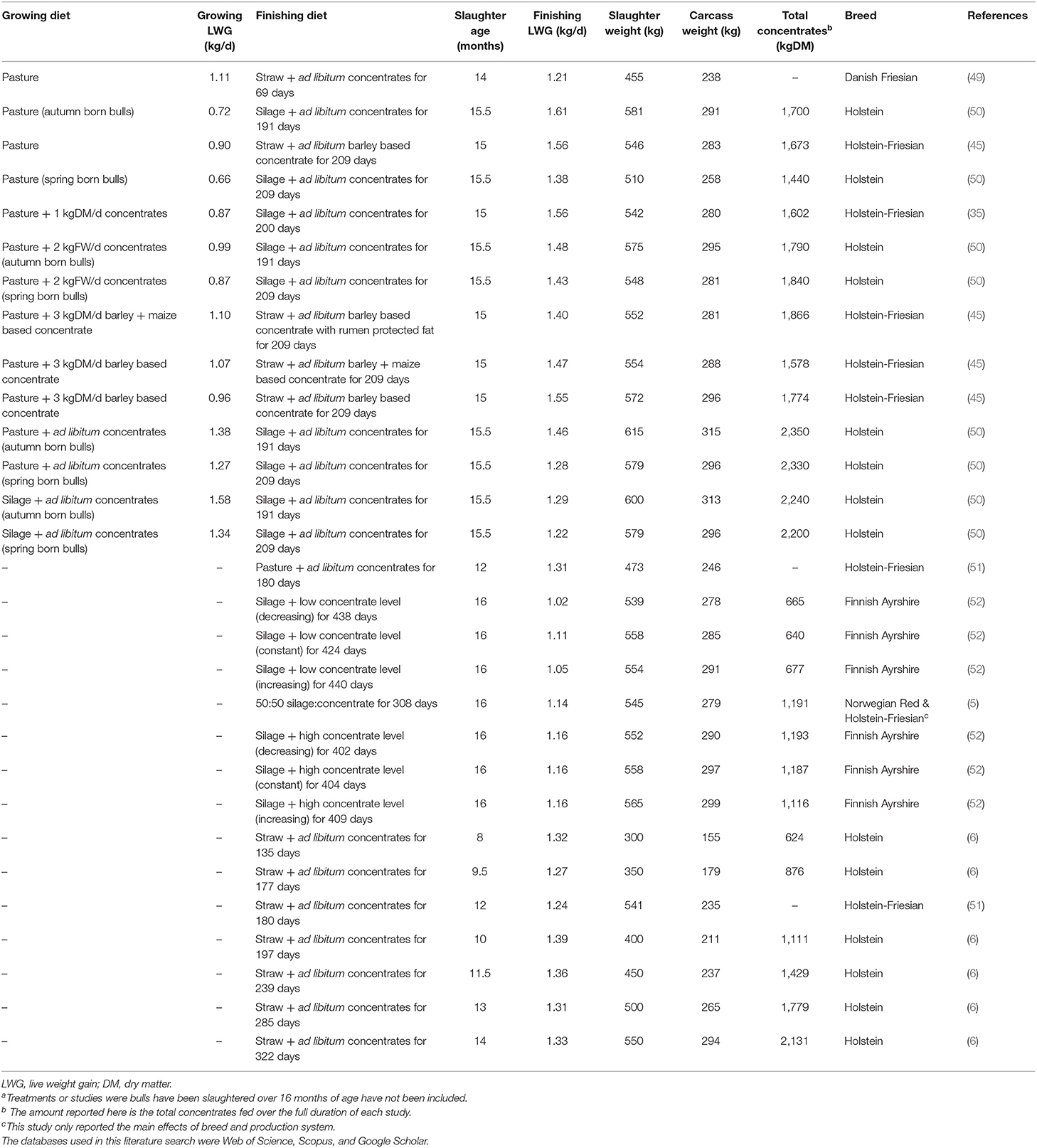
Table 2. Summary of key published researcha addressing the effect of production system on bull performance and concentrate intake.
High Input Bull Beef Production Systems
Bull beef production is traditionally an intensive ad libitum concentrate, indoor system (6, 53). Ad libitum feeding has the potential to lead to superior weight gains by supporting the genetic potential of the animal. Supplementing concentrates at 95% of DMI from 3 months of age until slaughter has been shown to result in a mean LWG of 1.33 kg/d, with bulls reaching target slaughter weight (550 kgLW) at 14 months of age (6). Rutherford et al. (50) offered housed autumn born Holstein bulls ad libitum concentrates from 6 months until slaughter at 15.5 months and achieved a mean LWG of 1.39 kg and carcass weight of 313.3 kg. These results are in agreement with previous ad libitum studies (49, 51) and show the potential for bulls to achieve a high level of performance when offered an intensive diet.
The literature has also explored the effect of ad libitum concentrates at grass. Rutherford et al. (50) offered bulls ad libitum concentrates at grass during the summer followed by a housed ad libitum finishing period. This system resulted in a 1.44 kg/d LWG and 314.5 kg carcass weight at 15.5 months. Similarly, Moloney et al. (51) slaughtered bulls at ~12 months of age, following a 6 months period of ad libitum concentrates at grass achieving a LWG and carcass weight of 1.31 kg/d and 245.6 kg, respectively. The lighter carcass weight here, being a result of the younger age at slaughter. It was also reported that total concentrate intake did not differ between bulls that were housed or grazed with ad libitum concentrates (50). Furthermore, in both studies meat quality parameters were unaffected by ad libitum production system (50, 51). Although the forage component of the diet differed, both production systems were heavily dependent on concentrates and thus the energy dense diet resulted in sufficient muscle and liver glycogen stores for anaerobic glycolysis to occur post-mortem (54, 55).
One limitation of an ad libitum concentrate system, is that it often results in a high cost of production due to the high input nature of the diet. For example, total concentrate consumption from ad libitum production systems has been reported at 2.13 tDM (6) and 2.34 tDM (50) during the study period. This therefore creates a system that is subject to fluctuating concentrate prices and beef market volatility (34) and thus profit margins can vary substantially from year to year. In addition, a high input, housed system, may also raise animal health and welfare concerns which are discussed in section Effect of Beef Production System on Health and Welfare.
Housed Low Input Bull Beef Production Systems
When combined with good quality silage, moderate (56) or no (57) concentrate supplementation can support high levels of performance. Moving away from offering ad libitum concentrates and therefore, reducing the dependence on them, has the potential to lessen the cost of production. Kirkland et al. (5) offered concentrates at 50% of DMI (mean of 3.87 kgDM/d) from 6 to 16 months of age; achieving a LWG of 1.14 kg/d carcass weight of 278.8 kg. During the 249 d experimental period, total concentrate intake equated to 1.14 tDM (5); considerably lower than that of ad libitum fed bulls (6, 50).
Manni et al. (52) achieved a LWG of 1.16 kg/d from Finnish Ayrshire bulls when supplementing concentrates at 42% DMI. While a concentrate inclusion rate of 23% DMI resulted in a slightly lower LWG of 1.11 kg/d. The lower concentrate also meant that these bulls required an additional 20 days to reach target slaughter weight. Even though both groups were slaughtered at the same LW (558 kg), differences were observed in carcass fatness, with the bulls on the higher concentrate inclusion rate being fatter. This indicates that the greater proportion of metabolisable energy in the diet from concentrates, not only supported a greater LWG but resulted in increased fat deposition (52), which as previously stated can have a detrimental effect on feed efficiency (58). However, fat cover is considered an important component of meat eating quality, as intramuscular fat can lead to increased flavor and tenderness of the meat (59).
Research has also investigated finishing dairy bulls up to 20 months of age. Under the current UK market requirements, these systems would not produce beef that is within market specification, however, some interesting trends were reported that could be used to inform a 16 months system. Although increasing slaughter age often results in a heavier carcass weight, growth rates have been shown to slow in the final months prior to slaughter. This was evident in the study conducted by Kirkland et al. (5) where increasing slaughter age from 16 to 20 months resulted in an increased LW at slaughter of 111 kg. However, growth rates were compromised during this additional 4 months of finishing. Bulls slaughtered at 20 months had a mean LWG of 1.14 kg/d up to 16 months of age; following this, mean LWG decreased to 0.88 kg/d; a substantial 30% reduction (5). This trend had previously been reported (60, 61) and reflects the sigmoidal growth curve of cattle (62).
Grazed Bull Beef Production Systems
Profitability in a dairy-origin beef production system is reported to be determined by two main factors; carcass output per hectare and the proportion of grazed grass in the diet (63). Grazed grass is known to be the cheapest form of feed for ruminant production (33, 64). Thus, the inclusion of a grazing period during the production system, may have the potential to reduce production costs considerably. However, managing cattle in a rotational pasture system can be more labor intensive than a housed system, particularly if the grazing infrastructure is suboptimal. The most suitable time for a grazing period in bull beef production is during the grower period, to allow for an indoor finishing period so that carcass traits are not compromised.
A LWG from grass of 0.72 and 0.80 kg/d have been reported during a 203 and 134 d grazing period, respectively for HF bulls (65). Therkildsen et al. (49) observed a greater LWG of 1.1 kg/d from grass for Danish Friesian bulls from 7 to 12 months of age. However, this was still 37% lower than that of housed ad libitum concentrate bulls. Supplementing concentrates during the grazing period may aid in maintaining a greater level of performance. Offering bulls 2 kg/d concentrates while at grass has been shown to support a LWG of 0.99 kg/d for autumn born bulls and 0.87 kg/d for spring born bulls. This was greater than that achieved from grass alone in the same study; 0.72 kg/d for autumn born bulls and 0.66 kg/d for spring born bulls (50).
Herbage quality (45) and pre-grazing height (66) are said to be two of the main factors influencing the LWG achievable from grass. Reducing sward-surface height from 10.0 to 6.5 cm resulted in 0.33 kg/d reduction in LWG in Continental × Friesian bulls (66). In addition, grazing weaned Friesian (FR) bulls on herb-legume swards has been reported to result in greater performance in comparison to grazing pasture or pasture with concentrate supplementation (67). However, this study focused purely on the summer grazing period, and thus it is unknown as to whether the live weight advantage continued throughout the finishing period. Therefore, the literature is limited on the effect of different pasture types in Holstein bull beef production systems from post-weaning to slaughter, thus this is an area that warrants further research.
One of the challenges of grazing is that a post-turnout LW loss can occur when calves are turned out to pasture. In the case of Steen and Kilpatrick (66) this resulted in a reduction in LW of up to 15 kg over a period of 2 weeks. This reduces the efficiency and LWG achieved from grass, as the period of loss and subsequent recovery time result in a period of unproductiveness. However, the extent of this can be reduced if cattle are fed a high forage diet pre-turnout, as opposed to having a high level of concentrate supplementation (68) and grazing and weather conditions are good at turnout. Other problems can arise with bulls at pasture, particularly if weather conditions are not optimal. Bulls are renowned for agonistic and sexual behavior (69), and being generally more unsettled that steers. The likelihood of which is amplified if conditions are wet, bulls are grazed in large groups or have a lack of shelter in a paddock grazing system (70). This can lead to increased poaching and a reduction in the grazable area. Thus, early housing of bulls in the autumn can reduce setbacks in LWG (65) and sward damage. Adverse grazing conditions were thought to be largely responsible for the poor LWG (0.58 kg/d) of bulls during the grazing season observed by McNamee et al. (36). Keane and Fallon (65) reported that bulls housed in early September were of better body condition than those housed in late November. This was confirmed by a reduction in mean LWG from grass of 0.08 kg/d when the grazing season was extended. Considering the relatively short duration for finishing following a summer grazing period (particularly for autumn born bulls) it is vital that a balance is reached between maximizing the inclusion of grass in the diet and LW performance.
Once housed, the level of concentrate feeding during the finishing period must be carefully considered. Depending on the length of the grazing period; bulls are likely to be considerably lighter than if they had been housed on an ad libitum system (6, 49, 50, 65). Thus, in order to ensure carcass weight is not substantially compromised, the aim should be to maximize carcass gain during the subsequent finishing period. Where concentrate supplementation is not offered during the grazing period, a finishing period of at least 200 days is said to be required (71).
Following a grazing period, Rutherford et al. (50) offered autumn born bulls ad libitum concentrates during finishing, achieving a LWG of 1.32 kg/d and carcass weight of 291.3 kg, which was 22 kg less than that achieved by lifetime ad libitum bulls. Thus, it was suggested that these bulls expressed compensatory growth during the finishing period (72). Furthermore, Rutherford et al. (50) reported similar carcass weights between bulls offered 0 and 2 kg/d concentrates at grass during the grower period. This was in agreement with Murphy et al. (45) who also reported similar finishing LWGs and carcass weights for bulls supplemented and unsupplemented at grass. Furthermore, mean carcass fat classification did not differ between these groups. Yet when the authors considered the proportion of bulls in each treatment that achieved the target fat classification, those that had received 3 kg/d concentrates at grass, were proportionately 40% more likely to reach target fat classification than those unsupplemented (45).
Keane and Fallon (65) evaluated three levels of concentrate feeding (3, 6 kg/d and ad libitum) for finishing HF bulls following a grazing period. Ad libitum concentrate feeding achieved a mean LWG of 1.36 kg/d; leading to a LW at slaughter of 610 kg. These bulls obtained a carcass weight of 338 kg, and fat classification of 3.2 following a mean finishing period of 225 days. Whereas, those on 3 kg/d concentrates had a LWG of 0.91 kg/d, and LW at slaughter of 514 kg. Slaughter traits such as dressing proportion, carcass conformation, fat classification were also reduced as a result of the lighter slaughter weight (65). Although not specified, it is estimated that these bulls were approaching 18 months at slaughter, and thus alterations would need to be made to meet current market requirements.
The finishing diet must take into account the total feed input, the duration of finishing and the desired level of carcass finish. Keane and Fallon (65) concluded that commencing a finishing period at 300 kgLW following a grazing period, would require 1.9 tDM of concentrates and 0.2 tDM of silage over a 7 months period when concentrates were supplemented ad libitum, to achieve a carcass weight of 320 kg. A further 1.5 months of finishing was required when concentrates were supplemented at 6 kgDM/d; equalling a total concentrate intake during the finishing period of 1.3 tDM along with a 1.1 tDM silage intake. Thus, a balance between feed input and length of finishing period needs to be considered when identifying the optimum production system for bull beef. This will largely be determined by the age and weight of the bulls at the start of the finishing period. If carcass weights are to be lighter as a result of including a grazing period, then a lower cost of production must be guaranteed. Rutherford et al. (50) demonstrated that the inclusion of a grazing period could reduce total concentrate usage by 890 kg in spring born bulls compared to a housed ad libitum concentrate system, thus substantially reducing production costs. Therefore, although careful management is required to ensure market requirements are achieved (45), the literature does suggest that the inclusion of a grazing period in a bull beef system is a potential method of operating a sustainable bull beef production system.
Effect of Beef Production System on Health and Welfare
One of the main welfare concerns in a steer production system is the age and method of castration. Castration is well-known to cause pain and elicit a stress response, illustrated by a rise in plasma cortisol concentration and changes in calf behavior (73). Furthermore, neurohormonal and electroencephalographic stress responses to castration have been shown to be age-specific (74). Therefore, the method and age of castration of calves is controlled under UK legislation [the Protection of Animals (Anesthetic) Act 1954 and the Veterinary Surgeons Act 1966] (75), in order to minimize the negative impact on animal welfare. For example, the use of rubber ring castration can only be completed up to 7 days of age, whereas for calves up to 2 months of age, bloodless castration (crushing the spermatic cords) is the recommended method. After 2 months of age castration should be carried out be a veterinary surgeon (75). From a production perspective, it is recommended that calves are castrated at birth or close to birth, as live weight loss increases quadratically as the age of castration increases, regardless of the method used (76).
Behavioral freedom is an important component of animal welfare, and pasture based production systems are perceived to offer this to a greater extent than continuously housed systems (77). Research in dairy cows has shown that cows at pasture will spend a greater amount of time lying (78) and feeding (79) than those housed. Furthermore, bulls that have been housed throughout their lifetime have been observed to be more aggressive toward humans and one another, compared to those that have been at pasture (66). Therefore, a pasture based steer system or bull beef system with the inclusion of a grazing period, would be considered best in providing behavioral freedom for beef cattle, as this way cattle spend a lesser proportion of their lifetime housed. However, the handling and feeding of bulls at pasture can be a potential safety risk for the farmer, and thus care must be taken when carrying out these tasks. In contrast, the use of well-designed housing and handling facilities, make feeding and routine procedures such as weighing easier and safer to complete in a housed system. Yet regardless of whether bulls are produced indoors or at pasture there will always be an increased safety risk when working with bulls as opposed to steers.
Another consideration of continuously housed beef systems is the impact on lameness. Lameness has a multifactorial and complex etiology (77), and research on beef production systems has largely focused on the impacts of different floor surfaces (concrete, rubber, and straw) in housed systems (80, 81). Slatted concrete flooring has been documented to be a contributing factor, increase the incidence and severity of sole hemorrhages in dairy bred calves in comparison to straw bedding (80). Yet little is known about the impacts of a fully housed beef system in comparison to a pasture based beef system.
Subacute ruminal acidosis (SARA) is a common nutritional disease in beef production, which can have a considerable detrimental effect on animal health, welfare, and performance (82–84). The primary cause of SARA is the consumption of excessive amounts of rapidly fermentable carbohydrates in conjunction with inadequate fiber (85); resulting in an increased accumulation of organic acids within the rumen. The effects of which are compounded if the rumen environment is not sufficiently adapted to concentrate feeding (85). Thus, SARA is a health risk of intensive production systems, such as an ad libitum concentrate bull beef systems, or those where an appropriate transition period is not adhered to at the beginning of the finishing period. Liver abscesses are often a secondary outcome of SARA, and may result in a further reduction in DMI, LWG, feed efficiency and carcass yield (86). However, in the case of Rutherford et al. (50) bull beef production system had no impact on the post-mortem incidence of liver abscesses, thus indicating that with the appropriate management, this can be avoided.
Other parameters such as clinical pneumonia incidence, together with the post-mortem incidence of pneumonia and pericarditis have also been considered and found to be unaffected by production system (50). Similarly, Manni et al. (87) also reported no clinical signs of disease during a bull beef production system study in Finland.
Bulls are reported to be more susceptible to stress than steers (88). Thus, pre-slaughter stressors associated with handling, re-grouping, transportation, and lairage (89, 90) should be minimized in order to reduce the risk to both animal welfare and meat quality (91, 92). Through the use of rumen temperature boluses, Rutherford et al. (93) demonstrated that bulls that displayed stress induced hyperthermia during the pre-slaughter phase also had the poorest meat quality. Suboptimal meat quality can result in a substantial cost to the red meat industry (94), thus bull beef systems may require better pre-slaughter management than that of steer systems.
The literature on beef production systems rarely evaluates the impacts on animal health and/or welfare, as the primary focus of these studies are intakes, performance, and carcass characteristics. Thus, direct comparisons of the welfare of beef production systems are particularly limited within the scientific literature. Therefore, future research evaluating these systems should involve a much wider assessment, beyond simply the impacts on animal productivity.
Conclusion
This review demonstrates that there is the potential for surplus male dairy bred calves to be used in UK beef production and meet market requirements. Whether the system implemented involves steers or bulls is something that will vary from farm to farm. Profitability is highly subject to fluctuations in calf, concentrate and beef price, while a high carcass output per hectare combined with a low total concentrate intake, have been shown to be key measures within a production system that can be farmer managed to maximize the sustainability of a production system (34, 35). Thus, it would be expected that the most suitable systems are those that achieve a high proportion of growth from pasture, such as a 24 months steer system, or a bull beef system that involves a grazing period.
A number of health and welfare challenges associated with beef production systems were highlighted within this review. However, the castration of calves is regulated within the UK, while health issues such as SARA can be prevented with appropriate nutritional management. Thus, these are challenges that can be overcome and do not raise the same welfare concerns as the current methods of exporting or euthanising surplus calves.
The issue of surplus male dairy calves may also be addressed via breeding programmes within the UK dairy herd. The increased use of sexed semen for targeted breeding of dairy replacements (95), now means that more beef genetics are being used within dairy herds (96). Beef sired dairy calves are well-known to have improved carcass gain and carcass characteristics while also having a lower DMI than their dairy sired counterparts (97) and thus are better suited for beef production.
Beef from the dairy herd has also been shown to have significantly less GHG emissions than that from the suckler herd (35, 98) and therefore from an environmental perspective surplus male calves should be utilized to their full potential within the UK. However, one consideration of using surplus dairy bred calves in UK beef production is to ensure that this additional beef production would not flood the market and drive prices down. However, the UK produces beef to some of the highest welfare standards in the world, in addition to a large proportion of beef being grass fed (33), and thus the marketing potential of this product should assist in sourcing and expanding markets.
Author Contributions
NR: writing—original draft preparation. NR, GA, and FL: writing—review and editing. All authors: contributed to the article and approved the submitted version.
Funding
This work was jointly funded by the Department of Agriculture, Environment and Rural Affairs for Northern Ireland and AgriSearchNI.
Conflict of Interest
The authors declare that the research was conducted in the absence of any commercial or financial relationships that could be construed as a potential conflict of interest.
References
2. Veerkamp RF, Koenan EPC, de Jong G. Genetic correlations among body condition score, yield and fertility in first parity cows estimated by random regression models. J Dairy Sci. (2001) 84:2327–35. doi: 10.3168/jds.S0022-0302(01)74681-4
3. AHDB. UK Milk Yield. (2019). Available online at: https://ahdb.org.uk/dairy/uk-milk-yield (accessed September 06, 2020).
4. Keane MG, Neilan R, Moloney AP, Allen P. Comparison of high genetic merit, standard genetic merit, Friesian and CharolaisxFriesian male cattle for beef production. In: End of Project Report – Beef Production Series No. 24. Dunsany: Grange Research Centre (2001).
5. Kirkland RM, Patterson DC, Keady TWJ, Moss BW, Steen RWJ. Beef production potential of Norwegian Red and Holstein-Friesian bulls slaughtered at two ages. Animal. (2007) 1:1506–14. doi: 10.1017/S1751731107000791
6. Kirkland RM, Keady TWJ, Patterson DC, Kilpatrick DJ, Steen RWJ. The effect of slaughter weight and sexual status on performance characteristics of male Holstein-Friesian cattle offered a cereal-based diet. Anim Sci. (2006) 82:397–404. doi: 10.1079/ASC200642
7. Chever T, Cogoluenhès C, Lardier F, Renault S, Renault C, Romieu V, et al. Evaluation of the Market Implications of Veal and Young Cattle Meat Standards. (2014). Available online at: https://ec.europa.eu/agriculture/sites/agriculture/files/evaluation/market-and-income-reports/2014/veal-marketingstandards/fulltext_en.pdf (accessed September 07, 2020).
8. Holden SA, Butler ST. Review: applications and benefits of sexed semen in dairy and beef herds. Animal. (2018) 12:S97–103. doi: 10.1017/S1751731118000721
9. Wilson DJ, Pempek JA, Roche SM, Creutzinger KC, Locke SR, Habing G, et al. A focus group study of Ontario dairy producer perspectives on neonatal care of male and female calves. J Dairy Sci. (2021) 104:P6080–6095. doi: 10.3168/jds.2020-19507
10. Hulbert LE, Moisa SJ. Stress, immunity, and the management of calves. J Dairy Sci. (2016) 99:3199–216. doi: 10.3168/jds.2015-10198
11. Robison JD, Stott GH, DeNise SK. Effects of passive immunity on growth and survival in the dairy heifer. J Dairy Sci. (1988) 71:1283–7. doi: 10.3168/jds.S0022-0302(88)79684-8
12. DeNise SK, Robison JD, Stott GH, Armstrong DV. Effects of passive immunity on subsequent production in dairy heifers. J Dairy Sci. (1989) 72:552–4. doi: 10.3168/jds.S0022-0302(89)79140-2
13. CHAWG. Fifth Report. Great Britain (2020). Available online at: https://projectblue.blob.core.windows.net/media/Default/Beef%20&%20Lamb/CHAWG2020-Report5_3613_171120_WEB.pdf (accessed March 18, 2021).
14. Boulton AC, Rushton J, Wathes DC. A study of dairy heifer rearing practices from birth to weaning and their associated costs on UK dairy farms. Open J Anim Sci. (2015) 5:185–97. doi: 10.4236/ojas.2015.52021
15. Hawkins A, Burdine K, Amaral-Phillips D, Costa JHC. An economic analysis of the costs associated with pre-weaning management strategies for dairy heifers. Animals. (2019) 9:11. doi: 10.3390/ani9070471
16. Boulton AC, Kells NJ, Cogger N, Johnson CB, O'Connor C, Webster J, et al. Risk factors for bobby calf mortality across the New Zealand dairy supply chain. Prev Vet Med. (2020) 174:10. doi: 10.1016/j.prevetmed.2019.104836
17. Ulster Farmers' Union (UFU). Ulster Farmers' Union- Submission to Consultation on “A Call for Evidence on Controlling Live Exports for Slaughter and to Improve Animal Welfare During Transport After the UK Leaves the EU. Belfast (2018).
18. Bord Bia. The Spanish Livestock Trade. (2017). Available online at: https://www.bordbia.ie/industry/news/food-alerts/the-spanish-livestock-trade/ (accessed November 10, 2020).
19. Ares E. Briefing Paper Number 8031: Live Animal Exports. House of Commons Library, London. (2019). p. 1–19
20. Eurogroup for Animals. A Strategy to Reduce and Replace Live Animal Transport Towards a Meat and Carcasses Only Trade. Brussels (2019).
21. Compassion in World Farming C. Ban Live Exports. (2020). Available online at: https://www.ciwf.org.uk/our-campaigns/ban-live-exports/ (accessed November 10, 2020).
22. Uetake K, Ishiwata T, Tanaka T, Sato S. Physiological responses of young cross-bred calves immediately after long-haul road transportation and after one week of habituation. Anim Sci J. (2009) 80:705–8. doi: 10.1111/j.1740-0929.2009.00693.x
23. Grigor PN, Cockram MS, Steele WB, Le Sueur CJ, Forsyth RE, Guthrie JA, et al. Effects of space allowance during transport and duration of mid-journey lairage period on the physiological, behavioural and immunological responses of young calves during and after transport. Anim Sci. (2001) 73:341–60. doi: 10.1017/S135772980005832X
24. Department for Environment Food and Rural Affairs (DEFRA). Welfare of Animals During Transport. Council Regulation (EC) No 1/2005 on the Protection of Animals During Transport and related Operations. The Welfare of Animals (Transport) (England) Order 2006 and Parallel National Legislation in Scotland, Wales and Northern Ireland. London: DEFRA (2011).
25. Department for Environment Food Rural Affairs (DEFRA). Export or Move Live Animals Animal Products to the EU or Northern Ireland From 1 January 2021. Welfare A, editor. London: DEFRA (2020).
26. Baltussen W, Nuhoff-Isakhanyan G, van Wagenberg C. Transport of Live Animals Versus Meat: Case Studies of Spent Hens and Lambs, Using Newly Developed Calculation Model. Wageningen Economic Research Report, no. 2017-065. Wageningen: Wageningen Economic Research (2017). doi: 10.18174/420339
27. European Parliament and Council of European Union. Regulation (EU) No 1308/2013 of the european parliament and of the council of 17 December 2013 - establishing a common organisation of the markets in agricultural products and repealing Council Regulations (EEC) No 922/72, (EEC) No 234/79, (EC) No 1037/2001 and (EC) No 1234/2007. Off J Euro Union. (2013) 347:671–854.
28. DEFRA. Agriculture in the United Kingdom 2019. (2019). Available online at: https://assets.publishing.service.gov.uk/government/uploads/system/uploads/attachment_data/file/950618/AUK-2019-07jan21.pdf (accessed March 18, 2021).
29. Skelhorn EPG, Garcia-Ara A, Nova RJ, Kinston H, Wapenaar W. Public opinion and perception of rose veal in the UK. Meat Sci. (2020) 167:8. doi: 10.1016/j.meatsci.2019.108032
30. DEFRA. Welfare of Farmed Animals Regulations. (2007). Available online at: https://www.legislation.gov.uk/uksi/2007/2078/regulation/1/made (accessed March 18, 2021).
31. Department for Environment Food and Rural Affairs (DEFRA). Monthly UK Statistics on Cattle, Sheep and Pig Slaughter and Meat Production–Dataset. (2020). Available online at: https://www.gov.uk/government/statistics/cattle-sheep-and-pig-slaughter
32. Keane MG, Drennan MJ. Two-year-old beef production from Friesian and Friesian cross steers. In: Beef Series No. 9. Dublin: Teagasc (1991).
33. O'Donovan M, Lewis E, O'Kiely P. Requirements of future grass-based ruminant production systems in Ireland. Irish J Agric Food Res. (2011) 50:1–21.
34. Ashfield A, Wallace M, Prendiville R, Crosson P. Bioeconomic modeling of male Holstein-Friesian dairy calf-to-beef production systems on Irish farms. Irish J Agric Food Res. (2014) 53:133–47.
35. Murphy B, Crosson P, Kelly AK, Prendiville R. An economic and greenhouse gas emissions evaluation of pasture-based dairy calf-to-beef production systems. Agric Syst. (2017) 154:124–32. doi: 10.1016/j.agsy.2017.03.007
36. McNamee A, Keane MG, Kenny DA, Moloney AP, Buckley F, O'Riordan EG. Beef production from Holstein–Friesian, Norwegian Red × Holstein–Friesian and Jersey × Holstein–Friesian male cattle reared as bulls or steers. Livest Sci. (2015) 173:95–105. doi: 10.1016/j.livsci.2014.12.009
37. Keane MG, Moloney AP. Comparison of pasture and concentrate finishing of Holstein Friesian, Aberdeen Angus x Holstein Friesian and Belgian Blue x Holstein Friesian steers. Irish J Agric Food Res. (2010) 49:11–26.
38. Murphy B, Crosson P, Kelly AK, Prendiville R. Performance, profitability and greenhouse gas emissions of alternative finishing strategies for Holstein-Friesian bulls and steers. Animal. (2018) 12:2391–400. doi: 10.1017/S1751731118000034
39. Finneran E, Crosson P, O'Kiely P, Shalloo L, Forristal D, Wallace M. Simulation modeling of the cost of producing and utilising feeds for ruminants on Irish farms. J Farm Manage. (2010) 14:95–116.
40. Keane MG, Allen P. Effects of production system intensity on performance, carcass composition and meat quality of beef cattle. Livest Prod Sci. (1998) 56:203–14. doi: 10.1016/S0301-6226(98)00155-9
42. Steen RWJ. Beef production from bulls. In: Recent Research on Beef, Occasional Publication No. 20. Agricultural Research Institute of Northern Ireland (1991). p. 45–53
43. Steen RWJ. The effect of plane of nutrition and slaughter weight on growth and food efficiency in bulls, steers and heifers of 3 breed crosses. Livest Prod Sci. (1995) 42:1–11. doi: 10.1016/0301-6226(95)00002-3
44. Nian Y, Kerry JP, Prendiville DJ, Allen P. The eating quality of beef from young dairy bulls derived from two breed types at three ages from two different production systems. Irish J Agric Food Res. (2017) 56:31–44. doi: 10.1515/ijafr-2017-0003
45. Murphy B, Kelly A, Prendiville R. Alternative finishing strategies for Holstein-Friesian bulls slaughtered at 15 months of age. Agric Food Sci. (2018) 27:28–37. doi: 10.23986/afsci.66719
46. Potter T, Aldridge B. Systematic approach to calf pneumonia. Livestock. (2010) 15:31–4. doi: 10.1111/j.2044-3870.2010.tb00317.x
47. Kamilaris C, Dewhurst RJ, Sykes AJ, Alexander P. Modeling alternative management scenarios of economic and environmental sustainability of beef finishing systems. J Clean Prod. (2020) 253:11. doi: 10.1016/j.jclepro.2019.119888
48. Crosson P, Shalloo L, O'Brien D, Lanigan GJ, Foley PA, Boland TM, et al. A review of whole farm systems models of greenhouse gas emissions from beef and dairy cattle production systems. Anim Feed Sci Technol. (2011) 166–7:29–45. doi: 10.1016/j.anifeedsci.2011.04.001
49. Therkildsen M, Vestergaard M, Jensen LR, Andersen HR, Sejrsen K. Effect of feeding level, grazing and finishing on growth and carcass quality of young Friesian bulls. Acta Agric Scand Sect A Anim Sci. (1998) 48:193–201. doi: 10.1080/09064709809362420
50. Rutherford NH, Gordon AW, Arnott G, Lively FO. The effect of beef production system on the health, performance, carcass characteristics and meat quality of Holstein bulls. Animals. (2020) 10:1922. doi: 10.3390/ani10101922
51. Moloney AP, Fallon RJ, Mooney MT, Troy DJ. The quality of meat and fatness of bulls offered ad libitum concentrates, indoors or at pasture. Livest Prod Sci. (2004) 87:271–6. doi: 10.1016/j.livprodsci.2003.07.009
52. Manni K, Rinne M, Huhtanen P. Comparison of concentrate feeding strategies for growing dairy bulls. Livest Sci. (2013) 152:21–30. doi: 10.1016/j.livsci.2012.12.006
54. Immonen K, Ruusunen M, Hissa K, Puolanne E. Bovine muscle glycogen concentration in relation to finishing diet, slaughter and ultimate pH. Meat Sci. (2000) 55:25–31. doi: 10.1016/S0309-1740(99)00121-7
55. Ponnampalam EN, Hopkins DL, Bruce H, Li D, Baldi G, El-din Bekhit A. Causes and contributing factors to “dark cutting” meat: current trends and future directions: a review. Comprehens Rev Food Sci Food Safety. (2017) 16:400–30. doi: 10.1111/1541-4337.12258
56. Steen RWJ, Kilpatrick DJ, Porter MG. Effects of the proportions of high or medium digestibility grass silage and concentrates in the diet of beef cattle on live weight gain, carcass composition and fatty acid composition of muscle. Grass Forage Sci. (2002) 57:279–91. doi: 10.1046/j.1365-2494.2002.00326.x
57. Randby AT, Nørgaard P, Weisbjerg MR. Effect of increasing plant maturity in timothy-dominated grass silage on the performance of growing/finishing Norwegian Red bulls. Grass Forage Sci. (2010) 65:273–86. doi: 10.1111/j.1365-2494.2010.00745.x
58. Murphy TA, Loerch SC. Effects of restricted feeding of growing steers on performance, carcass characteristics and composition. J Agric Sci. (1994) 72:2497–507. doi: 10.2527/1994.7292497x
60. Andersen HR, Ingvartsen KL. The influence of energy-level, weight at slaughter and castration on growth and feed-efficiency in cattle. Livest Prod Sci. (1984) 11:559–69. doi: 10.1016/0301-6226(84)90071-X
61. Patterson DC, Moore CA, Steen RWJ. The effects of plane of nutrition and slaughter weight on the performance and carcass composition of continental beef bulls given high forage diets. Anim Prod. (1994) 58:41–7. doi: 10.1017/S0003356100007066
63. Ashfield A, Crosson P, Wallace M. Simulation modeling of temperate grassland based dairy calf to beef production systems. Agric Syst. (2013) 115:41–50. doi: 10.1016/j.agsy.2012.10.001
64. O'Riordan EG, O'Kiely P. Potential of beef production systems based on grass. Irish Grassland Anim Prod Assoc J. (1996) 30:185–217.
65. Keane MG, Fallon RJ. Effects of feeding level and duration on finishing performance and slaughter traits of Holstein-Friesian young bulls. Irish J Agric Food Res. (2001) 40:145–60. doi: 10.1017/S175275620000497X
66. Steen RWJ, Kilpatrick DJ. Effects of pasture grazing or storage feeding and concentrate input between 5.5 and 11 months of age on the performance and carcass composition of bulls and on subsequent growth and carcass composition at 620 kg live weight. Anim Sci. (1998) 66:129–41. doi: 10.1017/S1357729800008900
67. Pettigrew EJ, Morris ST, Back PJ, Kenyon PR, Berry J, Donald AJ, et al. Growth of weaned Friesian bull calves on a herb sward or with concentrate supplementation during late summer and early autumn. N Zeal J Agric Res. (2017) 60:70–9. doi: 10.1080/00288233.2016.1259641
68. Danielsson D-A, Johnsson S, Lindell L. Ungtjursuppfödning på bete och vallfoder - en fältstudie i västra Sverige. Report 208. Swedish University of Agricultural Sciences, Department of Animal Nutrition and Management (1992).
69. Timsit E, Assié S, Quiniou R, Seegers H, Bareille N. Early detection of bovine respiratory disease in young bulls using reticulo-rumen temperature boluses. Vet J. (2011) 190:136–42. doi: 10.1016/j.tvjl.2010.09.012
70. Harte FJ. Performance of Bulls and Steers Under Similar Feeding and Management Conditions. The Hague: Martinus Nijhoff Publishers (1984).
71. Murphy B, Crosson P, Kelly AK, Prendiville R. Animal performance and economic implications of alternative production systems for dairy bulls slaughtered at 15 months of age. Irish J Agric Food Res. (2017) 56:93–103. doi: 10.1515/ijafr-2017-0010
72. Hornick JL, Van Eenaeme C, Gerard O, Dufrasne I, Istasse L. Mechanisms of reduced and compensatory growth. Domest Anim Endocrinol. (2000) 19:121–32. doi: 10.1016/S0739-7240(00)00072-2
73. Sutherland MA, Ballou MA, Davis BL, Brooks TA. Effect of castration and dehorning singularly or combined on the behavior and physiology of Holstein calves. J Anim Sci. (2013) 91:935–42. doi: 10.2527/jas.2012-5190
74. Dockweiler JC, Coetzee JF, Edwards-Callaway LN, Bello NM, Glynn HD, Allen KA, et al. Effect of castration method on neurohormonal and electroencephalographic stress indicators in Holstein calves of different ages. J Dairy Sci. (2013) 96:4340–54. doi: 10.3168/jds.2012-6274
75. Department for Environment Food Rural Affairs (DEFRA). Code of Recommendations for the Welfare of Livestock: Cattle. Division AW, editor. London: DEFRA Publications (2003).
76. Bretschneider G. Effects of age and method of castration on performance and stress response of beef male cattle: a review. Livest Prod Sci. (2005) 97:89–100. doi: 10.1016/j.livprodsci.2005.04.006
77. Arnott G, Ferris CP, O'Connell NE. Review: welfare of dairy cows in continuously housed and pasture-based production systems. Animal. (2017) 11:261–73. doi: 10.1017/S1751731116001336
78. Olmos G, Boyle L, Hanlon A, Patton J, Murphy JJ, Mee JF. Hoof disorders, locomotion ability and lying times of cubicle-housed compared to pasture-based dairy cows. Livest Sci. (2009) 125:199–207. doi: 10.1016/j.livsci.2009.04.009
79. Roca-Fernandez AI, Ferris CP, Gonzalez-Rodriguez A. Short communication. Behavioural activities of two dairy cow genotypes (Holstein-Friesian vs Jersey x Holstein-Friesian) in two milk production systems (grazing vs confinement). Spanish J Agric Res. (2013) 11:120–6. doi: 10.5424/sjar/2013111-2682
80. Thomsen PT, Hansen I, Martin HL, Kudahl AB. Sole haemorrhages in Danish bull calves: prevalence and risk factors. Vet J. (2017) 224:44–5. doi: 10.1016/j.tvjl.2017.06.003
81. Murphy VS, Lowe DE, Lively FO, Gordon AW. The impact of floor type on lameness and hoof health of dairy origin bulls. Animal. (2018) 12:2382–90. doi: 10.1017/S1751731118000095
82. Fulton WR, Klopfenstein TJ, Britton RA. Adaptation to high concentrate diets by beef cattle. I Adaptation to corn and wheat diets. J Anim Sci. (1979) 49:775–84. doi: 10.2527/jas1979.493775x
83. Nagaraja TG, Titgemeyer EC. Ruminal acidosis in beef cattle: the current microbiological nutritional outlook. J Dairy Sci. (2007) 90(Suppl.):E17–38. doi: 10.3168/jds.2006-478
84. Mohammed R, Hunerberg M, McAllister TA, Beauchemin KA. Characterization of ruminal temperature and its relationship with ruminal pH in beef heifers fed growing and finishing diets. J Anim Sci. (2014) 92:4650–60. doi: 10.2527/jas.2014-7859
85. Kleen JL, Hooijer GA, Rehage J, Noordhuizen J. Subacute ruminal acidosis (SARA): a review. J Vet Med Ser A Physiol Pathol Clin Med. (2003) 50:406–14. doi: 10.1046/j.1439-0442.2003.00569.x
86. Nagaraja TG, Chengappa MM. Liver abscesses in feedlot cattle: a review. J Anim Sci. (1998) 76:287–98. doi: 10.2527/1998.761287x
87. Manni K, Rinne M, Joki-Tokola E, Huuskonen A. Effects of different restricted feeding strategies on performance of growing and finishing dairy bulls offered grass silage and barley based diets. Agric Food Sci. (2017) 26:91–101. doi: 10.23986/afsci.63053
88. Bonny SP, Hocquette JF, Pethick DW, Farmer LJ, Legrand I, Wierzbicki J, et al. The variation in the eating quality of beef from different sexes and breed classes cannot be completely explained by carcass measurements. Animal. (2016) 10:987–95. doi: 10.1017/S175173111500292X
89. Ferguson DM, Warner RD. Have we underestimated the impact of pre-slaughter stress on meat quality in ruminants? Meat Sci. (2008) 80:12–9. doi: 10.1016/j.meatsci.2008.05.004
90. Miranda-de la Lama GC, Villarroel M, Maria GA. Livestock transport from the perspective of the pre-slaughter logistic chain: a review. Meat Sci. (2014) 98:9–20. doi: 10.1016/j.meatsci.2014.04.005
91. Hemsworth PH, Rice M, Karlen MG, Calleja L, Barnett JL, Nash J, et al. Human–animal interactions at abattoirs: relationships between handling and animal stress in sheep and cattle. Appl Anim Behav Sci. (2011) 135:24–33. doi: 10.1016/j.applanim.2011.09.007
92. Lomiwes D, Farouk MM, Wu G, Young OA. The development of meat tenderness is likely to be compartmentalised by ultimate pH. Meat Sci. (2014) 96:646–51. doi: 10.1016/j.meatsci.2013.08.022
93. Rutherford NH, Lively FO, Arnott G. Evaluation of rumen temperature as a novel indicator of meat quality: rumen temperature, and haematological indicators of stress during the pre-slaughter period as predictors of instrumental meat quality in bulls. Meat Sci. (2019) 158:107913. doi: 10.1016/j.meatsci.2019.107913
94. Teke B, Akdag F, Ekiz B, Ugurlu M. Effects of different lairage times after long distance transportation on carcass and meat quality characteristics of Hungarian Simmental bulls. Meat Sci. (2014) 96:224–9. doi: 10.1016/j.meatsci.2013.07.009
95. Livestock and Meat Commission (LMC), editor. Bulletin Saturday 24 August 2019. In: Weekly Publication of the Livestock and Meat Commission for Northern Ireland. Belfast (2019).
96. Livestock and Meat Commission (LMC), editor. Bulletin Saturday 05 January 2019. In: Weekly Publication of the Livestock and Meat Commission for Northern Ireland. Belfast (2019).
97. Keane MG. A comparison of finishing strategies to fixed slaughter weights for Holstein Friesian and Belgian Blue x Holstein Friesian steers. Irish J Agric Food Res. (2010) 49:41–54.
Keywords: Holstein, steer, bull, concentrates, welfare, high input, low input, pasture
Citation: Rutherford NH, Lively FO and Arnott G (2021) A Review of Beef Production Systems for the Sustainable Use of Surplus Male Dairy-Origin Calves Within the UK. Front. Vet. Sci. 8:635497. doi: 10.3389/fvets.2021.635497
Received: 30 November 2020; Accepted: 29 March 2021;
Published: 27 April 2021.
Edited by:
Nicole Kemper, University of Veterinary Medicine Hannover, GermanyReviewed by:
Ruth Rodríguez-Bermúdez, University of Santiago de Compostela, SpainMelissa Cantor, University of Kentucky, United States
Peter Tozer, Massey University, New Zealand
Copyright © 2021 Rutherford, Lively and Arnott. This is an open-access article distributed under the terms of the Creative Commons Attribution License (CC BY). The use, distribution or reproduction in other forums is permitted, provided the original author(s) and the copyright owner(s) are credited and that the original publication in this journal is cited, in accordance with accepted academic practice. No use, distribution or reproduction is permitted which does not comply with these terms.
*Correspondence: Naomi H. Rutherford, bmFvbWkucnV0aGVyZm9yZEBhZmJpbmkuZ292LnVr