Herpesviruses in Reptiles
- Division of Tropical Health and Medicine, College of Public Health, Medical and Veterinary Sciences, James Cook University, Townsville, QLD, Australia
Since the 1970s, several species of herpesviruses have been identified and associated with significant diseases in reptiles. Earlier discoveries placed these viruses into different taxonomic groups on the basis of morphological and biological characteristics, while advancements in molecular methods have led to more recent descriptions of novel reptilian herpesviruses, as well as providing insight into the phylogenetic relationship of these viruses. Herpesvirus infections in reptiles are often characterised by non-pathognomonic signs including stomatitis, encephalitis, conjunctivitis, hepatitis and proliferative lesions. With the exception of fibropapillomatosis in marine turtles, the absence of specific clinical signs has fostered misdiagnosis and underreporting of the actual disease burden in reptilian populations and hampered potential investigations that could lead to the effective control of these diseases. In addition, complex life histories, sampling bias and poor monitoring systems have limited the assessment of the impact of herpesvirus infections in wild populations and captive collections. Here we review the current published knowledge of the taxonomy, pathogenesis, pathology and epidemiology of reptilian herpesviruses.
Introduction
Reptiles are a group of vertebrates (class Reptilia) that are adapted to a broad range of terrestrial and aquatic environments (1, 2). The group comprises over 11,000 extant species placed in four orders, namely: Testudines (turtles, tortoises, and terrapins); Squamata (lizards, snakes, and worm lizards); Crocodilia (crocodiles, alligators, gharials, and caimans); and Rhynchocephalia (tuatara) (3). Reptiles constitute an integral part of the natural ecosystem and play the roles of both pollinators and predators, as well as environmental health indicators (4). In addition to their ecological services, reptiles have become desirable for food, medicinal products, pet trade, leather goods and research applications (5–7). However, their existence and well-being have constantly been threatened by several factors, such as hunting, environmental pollution, loss of habitat, destructive non-native species, climate change, and infectious diseases (4, 8–10). Disease surveillance and research in wild populations of reptiles are associated with numerous challenges including difficulties in accessing samples or field data, misleading epidemiological data and missing population data, as well as political and cultural restrictions (11). These challenges well explain the use of captive wildlife as models in many studies to acquire epidemiological information, since diseases are comparable in both wild and captive animals (12–15). Nonetheless, more robust and ideal epidemiological data are obtained when free ranging animals are surveyed. Recently, researchers have taken a renewed interest in reptilian viruses, partly due to the role played by reptiles as reservoir hosts for zoonotic viruses, as well as improvements in viral diagnostic methods that, in turn, have increased understanding of viruses in reptiles (16–20).
Herpesviruses (HVs) are members of the family Herpesviridae, a large taxon of DNA viruses that have been described in most vertebrate animals, including reptiles (16, 21). Herpesviruses are enveloped viruses with an icosahedral nucleocapsid and a linear double-stranded genome of varying length from ~124 to 259 kbp (22). Generally, HVs replicate within host cell nuclei and are able to remain latent in their natural hosts (17, 23). So far, reptilian HVs that have been identified and characterised all belong to the subfamily Alphaherpesvirinae (17, 24–26).
The occurrence of HV infections among reptiles has been widely documented and associated with stomatitis, tumors, encephalitis, conjunctivitis, hepatitis and mortalities (27, 28). Unfortunately, current treatment options of reptilian HVs are limited and the search for potent vaccines remains a herculean task; therefore, the adoption of preventative strategies is still the most efficient way of controlling these diseases. This review aims to assist in biosecurity planning as well as create a knowledge platform for decision makers and researchers by providing an overview of the taxonomy, pathogenesis, pathology and epidemiology of reptilian HVs.
Methods
Databases such as Medline (Ovid), PubMed, and Scopus were searched using specific keywords and phrases including Herpesviridae infections, herpesvirus infection, fibropapillomatosis, grey-patch disease, loggerhead genital-respiratory herpesvirus, herpesvirus disease, reptiles, turtles, tortoise, snakes, lizards, alligators, and crocodiles (Supplementary File 1). To ensure that relevant publications were not missed, each subheading was searched independently on PubMed. Also, an additional literature search was conducted by assessing references of articles selected from previous databases. A summary of the search results is shown in Figure 1. Furthermore, we read the abstracts and full texts of the selected articles, extracted and analysed information on the diagnostic methods used and the reptilian HVs investigated from 1972 to September 8, 2020 (Figures 2, 3; Supplementary File 2). Non-English, non-original research, guidelines, and review articles were excluded from the analysis.
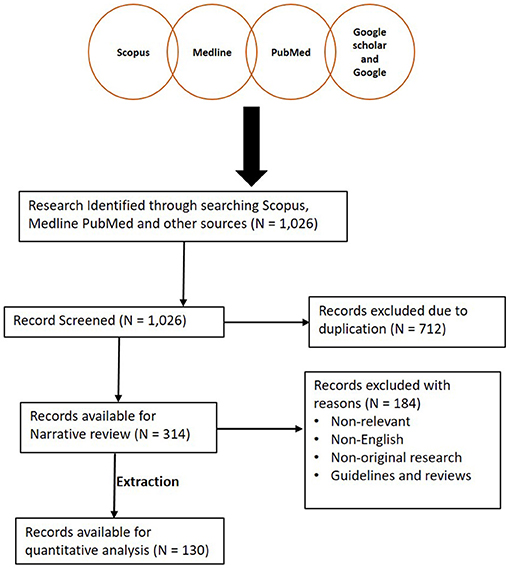
Figure 1. Summary of literature search conducted. A total of 1,026 articles were initially screened and 712 articles were later excluded due to duplication. A total of 314 articles were then reviewed for this study. One hundred and thirty studies were then extracted for quantitative analysis.
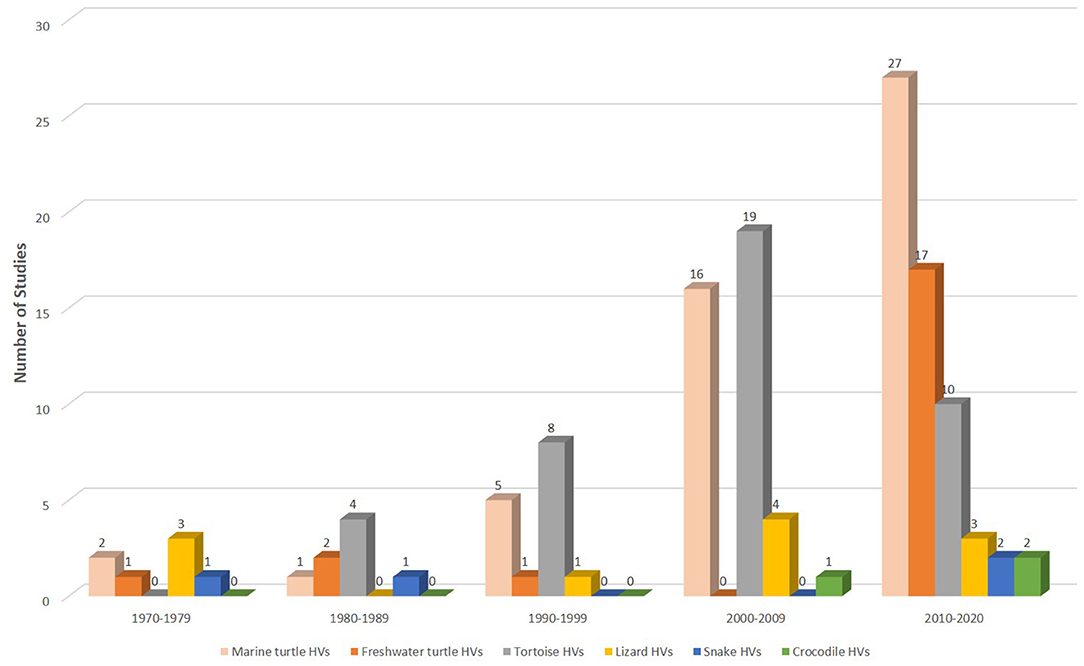
Figure 2. Studies on HVs in reptiles from 1972 to September 8, 2020. Overall, more than a quarter (n = 51; 39%) of the 130 extracted studies were conducted in marine turtles since the 1970s. Of the 60 publications between 2010 and 2020, 45% (n = 27) were ChHV-5 related studies.
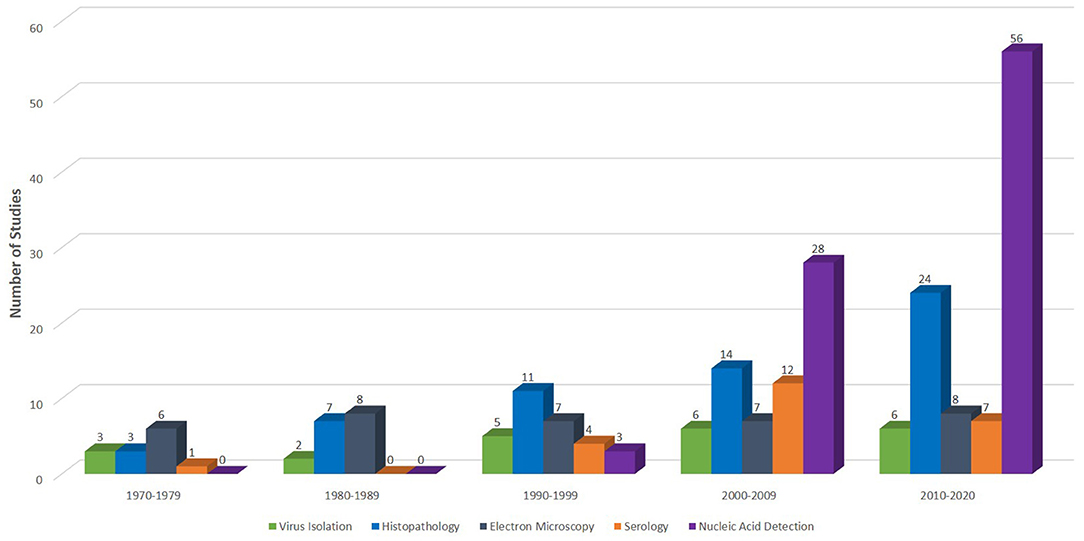
Figure 3. Diagnostic methods used in different studies from inception 1970 to September 8, 2020. Of the 130 studies extracted for this quantitative analysis, 22 (17%), 59 (45%), 36 (28%), 24 (18%), and 87 (67%) used virus isolation, histopathology, electron microscopy, serology, and nucleic acid detection assays, respectively, for various investigations of reptilian HVs.
Bibliometrics
We conducted bibliometric analyses of published articles on the topic of reptilian HVs using Vosviewer software (29) and the Web of Science Core Collection database. A total of 245 publications were downloaded from Web of Science Core Collection database using the following search terms; herpesvirus, turtle, lizard, snake, tortoise and crocodile. The strategy involved a combined use of the keywords, tags and Boolean operators to create query sets as follows: ALL= (herpesvirus) AND ALL= (turtle* OR lizard* OR snake* OR tortoise* OR crocodile*) with no limitations. USA had the highest number of research outputs with 149 (60.8%) articles. This was followed by Germany (28; 11.4%) and Australia (26; 10.6%) (Figure 4; Table 1). Using Vosviewer, we visualised the major keywords commonly used in the field of reptilian HVs and the link strengths between collaborating countries (Figure 4; Supplementary File 3). Of note, a low number of records on reptilian HVs were observed for some countries (Indonesia, Mexico and India) that have rich reptile diversity (Table 1) (3, 30, 31). Some of these countries also had little or no collaborations with the high research output countries (Figure 4), thus suggesting an under-reporting of reptilian HVs in these countries. Conversely, Germany has less reptile diversity with a higher number of records (Table 1). This observation could be attributed to the presence of established diagnostic resources or increased monitoring and reporting systems for reptilian diseases in the country.
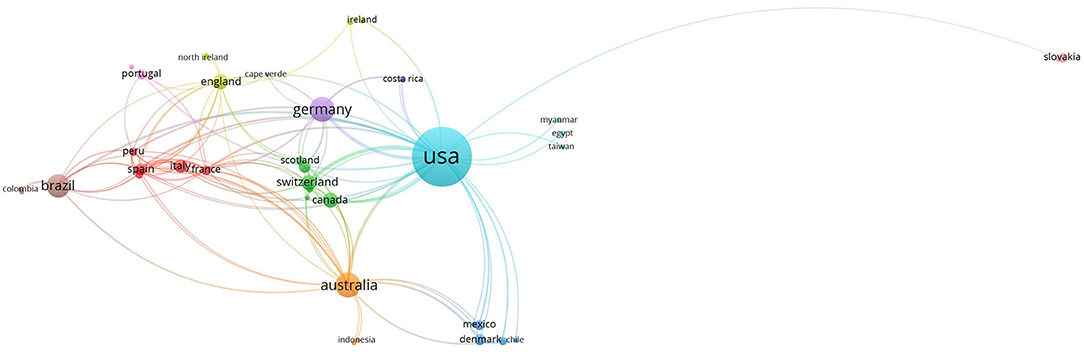
Figure 4. Links between collaborating authors from different countries with research output from inception to 2020. The circular coloured nodes represent countries and the node size indicates number of publications from the country. The lines between nodes indicate authorship collaborations between countries and the widths of these lines indicate the link strength.
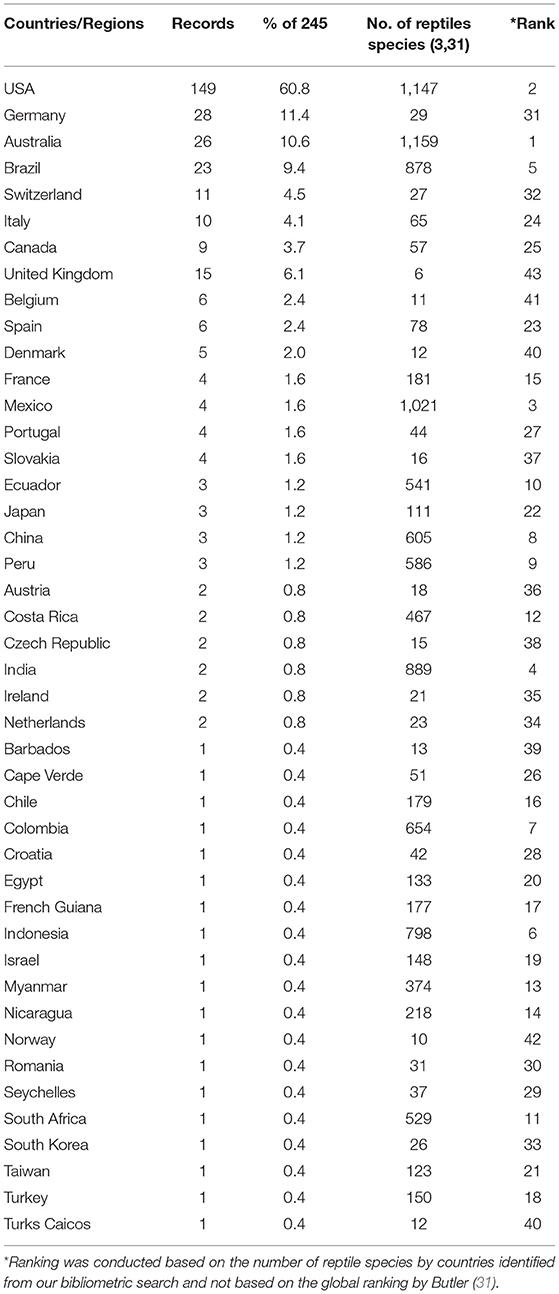
Table 1. Bibliographic data on reptilian herpesviruses based on the number of articles from different countries.
Taxonomy of Reptilian Herpesviruses
Reptilian HVs belong to the family Herpesviridae, a member of the order Herpesvirales (32). According to the 2019 International Committee on Taxonomy of Viruses (ICTV) classification, the subfamily Alphaherpesvirinae comprises five genera namely, IItovirus, Mardivirus, Scutavirus, Simplexvirus, and Varicellovirus. Only the genus Scutavirus contains species that cause HV diseases in reptiles and includes Chelonid alphaherpesvirus 5 (ChHV-5) and Testudinid alphaherpesvirus 3 (TeHV-3). The species Chelonid alphaherpesvirus 6 (ChHV-6) belongs to the subfamily Alphaherpesvirinae with unknown generic placement. The Iguanid herpesvirus 2 (IgHV-2) that causes cytopathic infection in iguanids is of unknown generic and subfamilial placement (32–35).
Since the end of the twentieth century, advances in molecular and phylogenetic analyses have made it possible for novel reptilian HVs to be identified with proposed taxonomic placements (36). Novel HVs have been detected in freshwater turtles, including Emydoidea herpesvirus 1 (EBHV-1), Pelomedusid herpesvirus 1, Glyptemys herpesvirus 1 and 2 (GlyHV-1 and−2), Emydid herpesvirus 1 and 2 (EmyHV-1 and−2), and Terrapene herpesvirus 1 and 2 (TerHV-1 and−2) (28, 37–41). Loggerhead genital-respiratory herpesvirus (LGRV) and loggerhead orocutaneous herpesvirus (LOCV) were detected in loggerhead turtles (Caretta caretta) and the genus Chelonivirus was proposed for these viruses and other related chelonian HVs (42). Also, tortoise HV species (TeHV-1,−2,−3,−4) have been identified and placed in the proposed genus Chelonivirus (43–46), although TeHV-3 has been formally assigned to the genus Scutavirus (32). Other unassigned reptilian HVs in the family Herpesviridae include the Iguanid herpesvirus 1 (IgHV-1), Gerrhosaurid herpesvirus 1-3, Varanid herpesvirus (VHV-1,−2,−3) and Helodermatid herpesvirus 1 (HeHV-1) in lizard species (25, 47–51), Opheodrys herpesvirus 1 in snakes (52), Crocodyline herpesvirus 1-3 (CrHV-1,−2,−3) in crocodiles (53), and, Chelonid herpesvirus 1-4 (ChHV-1,−2,−3,−4) in green turtles (Chelonia mydas-ChHV-1) (54), freshwater turtles (Clemmys marmorata-ChHV-2; Chrysemis picta-ChHV-3) (55, 56), and Argentine tortoise (Geochelone chilensis-ChHV-4) (57). Notably, some of these unassigned HVs were identified decades ago based on their morphological and biological characteristics using techniques (virus isolation, electron microscopy and histopathology) that were available at that time, thus making it challenging to place them taxonomically. We conducted a phylogenetic analysis to illustrate the relationship between the unassigned reptilian HVs and currently assigned HVs using amino acid sequences of HV-DNA-dependent DNA polymerase (37 complete and 17 partial sequences) from the NCBI website (https://www.ncbi.nlm.nih.gov/). As previously described (37, 58), the analysis showed that the unassigned reptilian HVs form a monophyletic group with members of the subfamily Alphaherpesvirinae. Freshwater HVs showed a close phylogenetic relationship with the tortoise HVs while the lizard HVs indicated high variations (Figure 5). Overall, given the variations shown by the unassigned reptilian HVs, it remains a matter of scientific deliberation whether these viruses should be assigned into one genus such as TeHV-3 and ChHV-5 or into different genera, although we envisage the latter would be the case.
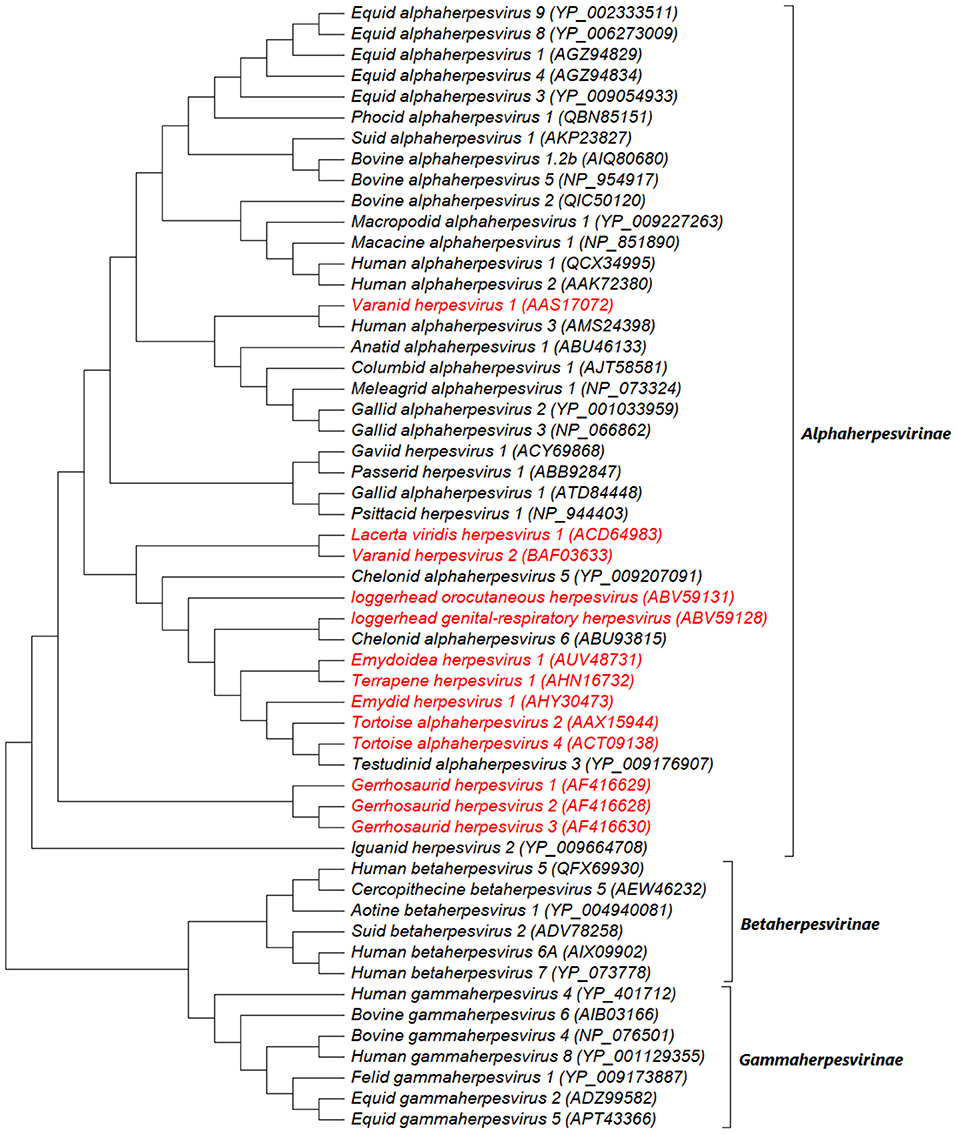
Figure 5. Midpoint rooted maximum likelihood phylogenetic tree of predicted amino acid sequences of HV-DNA-dependent DNA polymerase. The unassigned reptilian HVs are shown in red and cluster within the subfamily Alphaherpesvirinae. This analysis involved 54 amino acid sequences (37 complete and 17 partial sequences) downloaded from NCBI website (https://www.ncbi.nlm.nih.gov/) and aligned by ClustalW. There were a total of 1,443 positions in the final dataset. The evolutionary history was inferred by using the Maximum Likelihood method and JTT matrix-based model. The tree with the highest log likelihood (−60139.09) is shown. Initial tree(s) for the heuristic search were obtained automatically by applying Neighbor-Join and BioNJ algorithms to a matrix of pairwise distances estimated using a JTT model, and then selecting the topology with superior log likelihood value. The tree is drawn to scale, with branch lengths measured in the number of substitutions per site. Evolutionary analyses were conducted in MEGA X (59).
Virion and Genome Organization
All members of the family Herpesviridae share a common virion architecture, comprising a monopartite, linear, double stranded DNA core enclosed within an icosahedral capsid with a T = 16 symmetry (60–62). The capsid is tightly wrapped by a proteinaceous tegument, which, in turn, is surrounded by an envelope containing polyamines, lipids, and essential antigenic glycoproteins (62, 63). Unlike reptilian HVs, the atomic structures of human alphaherpesviruses have mostly been described. For instance, a cryo-electron microscopy (Cryo-EM) resolved the atomic structure of human simplexviruses (HSV-1 and−2), which comprise capsid organisation (hexons, pentons and triplexes), capsid proteins (VP5, VP19C, VP23, and VP26) and tegument proteins (pUL17, pUL25, and pUL36) (64–66). Although the atomic structures of reptilian HVs have not specifically been resolved, the resolved structures of other alphaherpesviruses provide insights, since their genomes have many similarities (36, 67). Consequently, the insights could guide future research in the atomic structure resolutions of reptilian HVs, which in turn could serve as a baseline for reptilian HV vaccinology.
Although the complete nucleotide sequences for most reptilian HVs are yet to be obtained, genomic features can be inferred from other fully sequenced alphaherpesviruses owing to sequence homology (36, 67). All alphaherpesvirus genome structures contain unique long (UL) and short (US) sequences and each are flanked by both terminal (TRL, TRS) and internal (IRL, IRS,) inverted repeat regions, giving the general configuration TRL-UL-IRL-IRS-US-TRS (68). The complete genome of two TeHV-3 strains (1976 and 4295) was recently sequenced. The 1976 strain was shown to have a novel inverted repeat (TRT, IRT) and unique (UT) regions (69). The genome is approximately 160 kbp, encodes at least 107 open reading frames (ORFs) and consists of UL (107,928 bp) and US (20,375 bp) regions. The UL is bound to its right by the US adjoined to inverted repeats (IRS and TRS; 8,536 bp) and to its left by a third unique region (UT; 12,595 bp), which is also bordered by inverted repeats (TRT and IRT; 1,194 bp) to give the overall layout TRT-UT-IRT-UL-IRS-US-TRS (69). However, this differs from the type D configuration earlier attributed to this species (70). In another study, the complete nucleotide sequence of a Bacterial Artificial Chromosome (BAC) containing the entire genome of ChHV-5 (cloned in pTARBAC2.1) was obtained and it showed a different configuration (UL-IRS-US-TRS) from that of TeHV-3, even though they both belong to the genus Scutavirus (71). Moreover, the genome characterisation of strain 4295 identified regions containing genes that could be involved in viral pathogenesis or virulence (69). This is an important finding as these regions could serve as therapeutic or diagnostic targets in future research. Similarly, evidence of recombination among strains of ChHV-5 documented by Morrison et al. could lead to increased virulence and transmission of ChHV-5 variants (72) and these events may remain undetected in sea turtle populations. Therefore, it is pertinent to strengthen current diagnostic approaches to allow for more comprehensive geographical surveys and characterisation of HVs. Also, as new and affordable diagnostic techniques are being developed and improved upon, we expect more novel structures of reptilian HVs to be reported.
Transmission and Pathogenesis
Several modes of reptilian HV transmission have been postulated including vertical, horizontal and mechanical transmissions (73–75). Marenzoni et al. reported the first evidence of vertical transmission of TeHV-3 in a captive breeding facility (14). In this study, one hatchling born in isolation from the egg laid by an infected tortoise (Testudo hermanni hermanni) presented with conjunctivitis and tested positive by specific polymerase chain reaction (PCR) targeting the partial sequence of the UL39 gene of TeHV-3 (14). In other studies, Jones and colleagues provided molecular evidence for the horizontal transmission of ChHV-5 in green turtles by demonstrating the molecular link between viral variants and foraging grounds (76, 77). Furthermore, experimental studies have revealed the possible transmission of reptilian HVs by direct contact with infectious secretions (78–80) or indirectly via vectors and water (81–84). By linking viral shedding patterns or frequency to disease occurrence, we could trace the most probable transmission mode of reptilian HVs. For instance, in captive enclosures, HVs could be easily transmitted via contact with secretions or contaminated materials, even at low shedding rates, and the removal of infected animals and infectious materials could stop the spread of the virus. In the wild, different transmission agents such as vectors, fomites and superspreaders could interplay to compensate for the low contact rate and infrequent shedding of some HVs (85). Because of the managerial implications, it is therefore important to further investigate the roles of these factors in the transmission of HVs in wild reptiles.
Four pathogenic mechanisms are highly conserved among all HVs and include: (1) intranuclear replication and capsid assembly; (2) expression of DNA metabolic and synthetic enzymes; (3) cell destruction following the release of viral progenies; and (4) the maintenance of latency in natural host cells (63, 86, 87). Generally, HV infections begin with viral entry, which is followed either by localisation or systemic spread (87). An experimental transmission study described the systemic dissemination of tortoise HVs (isolates HV 1976 and HV 4295/7R/95) (78). Following experimental infection via intranasal and intramuscular routes, the HVs elicited clinical signs and were detected by PCR in tissue samples from the respiratory, digestive and urogenital tracts, central nervous system (CNS), heart and spleen (78). Fibropapillomatosis (FP) and grey patch disease (GPD) are both associated with clinical signs that could be attributed to local destruction of infected cells due to replication and progeny release (54, 80, 88–90). Evidentially, two studies demonstrated the local replication of ChHV-5 by detecting certain biomarkers (eosinophilic intranuclear inclusions, F-VP26, DNA, and mRNA transcripts) within fibropapillomatous lesions (85, 91).
Unfortunately, the specific mechanisms involved in host cell invasion, immune evasion, localisation and spread of reptilian HVs have not been fully elucidated. However, recent molecular studies have provided insights into some virulence factors associated with reptilian HVs (69–71). Briefly, glycoproteins B (gB), gC, gD, gH, gK gL, gM, and gN have been hypothesised to function in host cell attachment and entry (69–71). gB and gC are capable of binding to heparan sulphate proteoglycans that are present on the surface of many cells, thus aiding viral adsorption and penetration into different cell types (69, 92). The interactions of gB, gD, gH, gK, gL, gM, and gN have also been postulated to mediate membrane fusion and viral entry into the cell (69, 70, 92). Glycoproteins B, E, H, and L are involved in viral cell to cell spread, which could occur through intercellular bridges or intra-axonal transport, thus circumventing humoral immune responses (69, 70). Similarly, the gC can bind to the third complement component (C3b) to block the alternative pathway complement activation (69, 93). The gE and gI in HSV-1 inhibit the normal function of antibodies by building up a complex that acts as an Fc-receptor (94). However, the immunosuppression mechanism of the gE homologue in reptilian HVs is not yet clear. Finally, the F-M04 and F-sial proteins were recently identified in ChHV-5 and thought to play a role in FP pathogenesis; however, the specific mechanisms involved are not yet understood (71). Future research should consolidate characterisation of reptilian HVs in order to increase the understanding of host-pathogen interactions and improve clinical interventions.
Clinical and Pathological Signs
Herpesvirus infections have been described in reptiles with a range of clinical manifestations (16). To provide an overview, the clinical signs and the gross and histological lesions associated with reptilian HVs are summarised in Table 2. Some of the more detailed descriptions are from sea turtles, tortoises and crocodiles. Grey patch disease and FP, characterised by coalescing greyish papular skin lesions (spreading patches) and branching papillary tumours (Figure 6), respectively, have been reported in sea turtles (42, 54, 108, 109). Lung-eye-trachea disease (LETD) with a clinical course of 2–3 weeks has been seen in green sea turtles (98). Lung-eye-trachea diseased turtles often present with pneumonia, stridor and caseation of the eyes, oropharynx and trachea (98). In freshwater turtles, HV infections are associated with hepatic necrosis, and proliferative and/or ulcerative lesions of the skin and shell (Figure 7) (37, 38, 110, 111). Infections in tortoises result in ulcerative to diphtheroid-necrotizing stomatitis, conjunctivitis, glossitis, rhinitis, dyspnoea, liver disease and neurological disease and could be accompanied by anorexia, regurgitation, neck oedema, lethargy and death (Figure 8) (112–115). Papillomas, stomatitis, and hepatitis are commonly described in lizards infected with HV (25, 47–49, 116, 117). Recently, five green snakes (Opheodrys vernalis) housed together presented with oropharyngeal squamous cell carcinoma and molecular analysis confirmed the presence of a novel Opheodrys herpesvirus-1 (Alphaherpesvirinae) (52). In another study, a lymphoid follicular cloacal inflammation in juvenile alligators was initially associated with tortoise HV. However, the HV (Genbank accession AY913769.1) was later determined to be a likely laboratory contamination and the actual causative agent is still unknown (118). Similarly, Hyndman et al. identified three novel HVs associated with conjunctivitis and/or pharyngitis (CP), systemic lymphoid proliferation with non-suppurative encephalitis (SLPE), and lymphonodular skin lesions (LNS) in farmed saltwater crocodiles (Crocodylus porosus) and captive freshwater crocodiles (Crocodylus johnstoni) (53). Obviously, HVs can induce significant diseases in both captive and wild reptiles; therefore, there is a need to develop rapid diagnostic tests that will aid disease surveillance and reporting in order to maintain safe biosecurity measures and reduce spread.
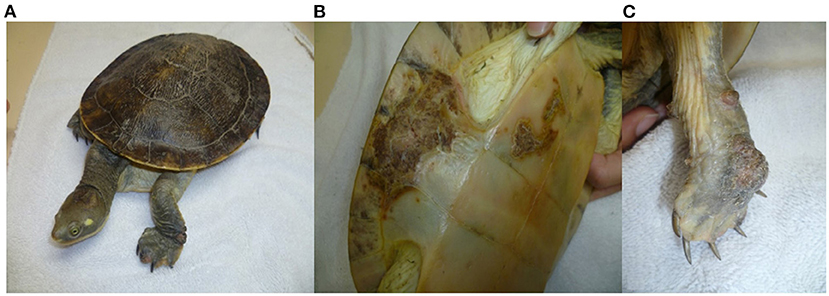
Figure 7. Herpesvirus infection in freshwater turtle (Emydura macquarii krefftii) presented with proliferative and ulcerative lesions of the skin (A), proliferative and crusted lesions on the bridge of the shell (B), and proliferative lesion on the palmar aspect of the right forefoot (C). “Adapted from Herpesvirus in a captive Australian Krefft's river turtle (Emydura macquarii krefftii)” by Cowan et al. (110). Copyright 2021 by John Wiley and Sons. Reprinted with permission.
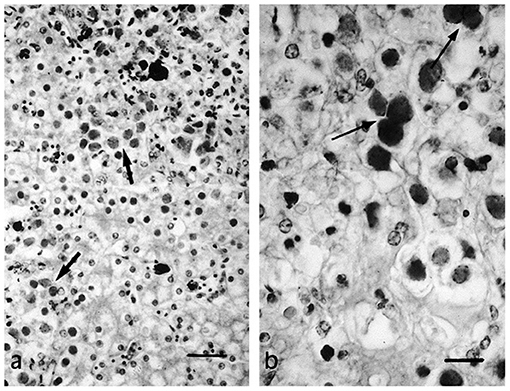
Figure 8. Necrotic foci (a) and syncytial formation (b) in HV infected hepatocytes of a tortoise (Testudo horsfieldii). “Adapted from Hepatitis Associated with Herpes Viral Infection in the Tortoise (Testudo horsfieldii)” by Hervás et al. (112). Copyright 2021 by John Wiley and Sons. Reprinted with permission.
Epidemiology
Epidemiological studies of HVs in wild reptiles could be challenging due to a lack of sensitive diagnostics for the detection of unknown or novel species, especially in resource-limited regions. HV infections are commonly characterised by non-specific clinical signs in most reptiles, thus making diagnoses on the basis of clinical signs alone difficult. An exception to this is FP, in which the presence of cutaneous tumours gives an indication of the disease; hence, more FP-associated HV data have been reported in recent decades (Figure 2; Supplementary File 2). Even so, the complete disease impact on wild populations could be currently underestimated due to the underreporting of outbreaks, sampling bias and poor monitoring systems. For instance, there is a paucity of information for FP epidemiology at the pelagic phase of life in sea turtles as most studies are biassed towards sampling nearshore juveniles and adult females at foraging grounds or nesting beaches. Nevertheless, wildlife workers and researchers who, despite numerous challenges, have provided considerable epidemiological data targeted at conservation efforts towards endangered species should be commended. An overview of some of the epidemiological information including the prevalence and demography of both wild and captive reptilian HVs is discussed in this section.
Herpesviruses are linked to different diseases of marine turtles, including FP, LETD and GPD (54, 82). FP is a debilitating disease characterised by the development of tumours (119, 120). Depending on the location of the tumours, FP can have detrimental effects (109, 121). On the basis of prevalence and distribution, Tagliolatto et al. reported a prevalence rate of 43% for FP in green turtles captured in a foraging area in south-eastern Brazil (121). Adnyana et al. recorded 22% overall prevalence in green turtles in Indonesia and also observed that the prevalence rate of FP was higher among turtles from waters adjacent to densely populated regions compared to those collected from waters remote from urbanised regions of Indonesia (122). These findings indicate that the epidemiology of FP in marine turtles vary between geographical regions and may be linked to anthropogenic activity. This theory is supported by the findings in another study, which attributed the variation of FP prevalence to environmental cofactors that vary among local habitats (123). A study associated the geographical distribution of FP with the genomic variation of HVs in marine turtles, and observed four forms of the virus corresponding to Atlantic Ocean, west Pacific, mid-Pacific, and east Pacific (124). A similar study conducted in Australian waters identified different genotypes along the east coast of Queensland. Such differences in strains may also effectuate different levels of pathogenicity between strains (76, 77) and account for variation in reported prevalence in different regions. Also, given that the immune system of reptiles is dependent on temperature (125, 126), the variation in the prevalence rates of reptilian HVs across regions could be associated with differences in regional climate types. Comprehensive reviews of the epidemiology of FP in marine turtles have been documented elsewhere (73, 97, 127–129). The host immune status influences the clinical course of a disease, as immuno-deficient populations are more likely to succumb to disease outbreaks. Serosurveys have been conducted to determine the immune status of populations and to provide evidence of past and ongoing HV infections (130, 131). Seroepidemiological studies in three localities in Florida revealed high anti-ChHV seroprevalences (up to 100%) in both FP and non-FP sea turtles (81, 132). Contrastingly, seropositivity to ChHV-5 was dependent on the tumour status in turtles from Hawaii (133). This variation was mainly attributed to differences in the pathogenicity of ChHV-5 subtypes from the two regions (133). In another report, an epizootic of LETD in confined juvenile green sea turtles resulted in 8 to 38% mortality, thus posing significant conservation and management concerns (98, 134). The LETD impact on free ranging sea turtles has not been investigated; however, seroprevalence rates of 13% and 22% were reported in two studies, respectively (134, 135).
Similarly, HV infections are causing increasingly significant concerns in non-marine chelonians (27, 39). Herpesviruses have been implicated as the cause of severe clinical signs and acute death in terrestrial and freshwater turtles (Table 2) (28, 40, 105, 110, 111, 136). Although, HVs have been associated with latent infections in their natural hosts, infections in young, immunosuppressed or non-adapted hosts could result in the development of significant diseases (137). Therefore, monitoring the disease impact on both wild and captive endangered species has become pertinent. In an epidemiological study conducted in Tennessee and Illinois, USA, 128 of 409 free-ranging eastern box turtles (Terrapene Carolina Carolina) tested positive for TeHV-1 using TaqMan quantitative PCR, and the detection rate varied widely between seasons (138). Another study reported 48.3% prevalence of HV infections in endangered populations of bog (Glyptemys muhlenbergii), wood (G. insculpta), and spotted (Clemmys guttata) turtles in the northeastern United States (37). Furthermore, tortoise HVs have been associated with high mortality and morbidity (104, 113, 139–141). Different HV species were identified to cause the death of a large number of pancake (Malacochersus tornieri), Horsfield (Testudo horsfieldii), Hermann's (Testudo hermanni), and Egyptian tortoises (Testudo kleinmanni) during spontaneous outbreaks in Japan, Italy and Germany, respectively (99, 102, 142). Species dependent susceptibility to HV was reported in a tortoise colony in which T. graeca and T. horsfieldii appeared to be unaffected by the HV species that caused the death of other tortoises in the same colony (113). A possible explanation could be that the causative HV species is well-adapted in these tortoises and they could be transmitting the virus to naïve or non-adapted tortoises. Of the four tortoise HV species (TeHV1-4), TeHV-3 appears to be the most pathogenic and frequently described, causing lethal disease in different tortoise species (14, 142–145). In a recent assessment of the incidence of chelonian HVs in Europe, more than half (54%) of all the detected chelonian viruses were TeHV-3 (146). Again, seroprevalence rates of 27% and 31% were reported for TeHV-1 and TeHV-3, respectively, in different populations of desert tortoises in California (147, 148). Despite the significance of HV infections, we observed that the disease is still grossly under-studied in some countries (Table 1). Thus, insufficient data and underreporting have made it difficult to assess the geographical patterns of the HV epidemiology in non-marine chelonians and other reptiles.
Herpesviruses have also been described in various species of squamates and crocodilians (25, 47, 48, 52, 106, 116–118, 149). A recent outbreak of a lethal HV infection in a private facility housing 127 snakes resulted in the death of all 71 horned vipers at the premises after a brief illness (150). An earlier study also implicated HV in the death of some boa constrictors within the first year of life (151). Herpesvirus-induced deaths have been reported in different species of lizards with case fatalities nearing 100% (33, 49, 152). As stated earlier, HV infections in crocodiles are associated with CP, SLPE and LNS syndromes (53). Another study strongly linked HV infection to SLPE and CP syndromes in farmed Australian saltwater crocodiles, with the highest prevalence rates of 94 and 54%, respectively (107). Crocodiles are intensively farmed for commercial purposes in Australia; therefore, the occurrence of HVs in crocodiles has both epidemiological and economic implications (53, 153).
Finally, we extracted a total of 130 articles, of which 39% (51 articles) and 32% (41 articles) were studies that investigated HVs in marine turtles and tortoises, respectively. A total of 21 (16%) studies investigated HVs in freshwater turtles. HVs were least studied in lizards (8%; 11 articles), snakes (3%; 4 articles) and crocodiles (2%; 3 articles) (Figure 2). The scant studies of HVs in some reptilian species since the 1970s could be attributed to the unavailability of reagents or sensitive diagnostic assays required to investigate reptilian diseases in remote areas or the lack of interest to investigate HVs in reptiles because of their relatively low socio-economic importance. Therefore, future efforts should be directed towards enhancing collaborations among government agencies, researchers and wildlife workers with a view to creating awareness, increasing access to reagents and sensitive assays, and ultimately conserving endangered reptiles.
Diagnosis
A timeline of reptilian HV diagnosis showed that traditional assays including histopathology, virus isolation (VI) and electron microscopy (EM) have been the mainstays in the diagnosis of reptilian HVs (Supplementary File 4). Many studies have reported the use of these techniques since the 1970s for the investigation of reptilian HVs. A breakdown of the number of studies that have used these methods to detect reptilian HVs is shown in Figure 3. Molecular diagnosis of reptilian HVs started two decades ago and has been used increasingly since then (Figure 3; Supplementary File 4). The advent of molecular diagnostic techniques has provided insight into the genetic characteristics and the phylogenetic relationship of most reptilian HVs. This section highlights some important characteristics of the various techniques used in the diagnosis of reptilian HVs.
Diagnosis of reptilian HVs is tentatively made on the basis of patient history, clinical signs, and gross and histological lesions (16). However, this is not always the case, as host-adapted HVs can cause subclinical, mild or latent infections in their natural hosts, and the demonstration of intranuclear inclusions is not pathognomonic of reptilian HV infections (154). Intranuclear inclusions are frequently associated with other reptilian viruses including adenoviruses and papillomaviruses (155–157). Earlier researchers used EM to confirm the presence of reptilian HV infections by demonstrating the ultrastructure of the viral particles in fixed, cut and stained sections of tissue samples (51, 54, 98, 106, 116, 136, 140, 158). More recently, EM has been used to confirm a necrotic hepatitis associated with HV infection in a tortoise with no clinical signs or lesions in the respiratory tract, oral cavity or other organs (112). The need for high technical capacities and the high cost of electron microscopes limit the use of EM for epidemiological and diagnostic purposes especially in resource-limited areas. Despite these limitations, EM remains a powerful detection tool in most high-class virology laboratories.
Reptilian HVs have been isolated in cell culture and identified on the basis of their cytopathic effects (50, 98, 107, 159). For instance, tortoise HVs were isolated from pharyngeal swabs, trachea, kidney, oesophagus, tongue, stomach, and intestine, and caused cytolysis and rounding of cells in terrapene heart cells (TH-1) (160). In another study, detachment and foci of enlarged, rounded, refractile cells were produced following inoculation of tissue and swab supernatants in turtle heart cells (142). ChHV-5, which historically has been resistant to replication in conventional cultures, produced de novo ballooning degeneration and eosinophilic intranuclear inclusion in plugs and organotypic skin cultures (89). This observation implies that ChHV-5 remains latent in conventional cultures and requires replication of the turtle skin to grow in vitro (89). Aside from the fact that CPE are not obtained for non-cytopathic viruses, cell culture is susceptible to both chemical and biological contaminations, which in turn affect its sensitivity and specificity. Also, diagnostic turnaround could be delayed for slow-growing viruses. Therefore, it should not be solely relied upon for the epidemiological investigations of HVs.
Following primary infections in reptiles, a strong non-specific (innate) immune response that includes lysozymes, leukocytes, natural antibodies (NAbs), antimicrobial peptides, and the complement pathway, is quickly stimulated (126, 161). No specific information is currently documented about adaptive cell mediated immunity to HV infections. Unlike mammals, in reptiles a less robust and slower humoral response (IgA, IgD, IgM, and IgY) is stimulated after the innate immune system is activated (126, 154). In tortoises, neutralising antibodies to HV infection were detectable in serum at least 4 weeks post-exposure (162). These serum neutralising antibodies did not appear to confer immunity to reinfection or recrudescence (78). Later seroconversion was observed (four months to one year) in green turtles (Chelonia mydas) that were experimentally infected with ChHV (81, 132). Generally, the detection of anti-herpesvirus antibodies in a single sample could indicate previous or latent infection, while rising antibody titre in paired samples collected at least 6 weeks apart indicates active infection (154, 163). Humoral antibodies are detected by serological assays such as serum neutralisation (SN) tests, ELISA, and immunoperoxidase (IP) assays (132, 164–166). The SN test is considered the reference test for anti-herpesvirus antibody detection but has limitations such as a delayed turnaround, inherent assay arduity and the requirement for standard isolates (162). ELISAs with high sensitivity and specificity have been developed and deployed in various seroepidemiological studies (44, 81, 132, 147, 162, 166). However, a high degree of cross-reactivity that potentially affects assay specificity has been demonstrated among different tortoise HV isolates used as antigens in the ELISA (147, 162). Cross-reactivity could also occur in other reptilian HVs that share similar antigenic epitopes, giving false positive results and, thus, leading to unnecessary post-exposure interventions. Overall, serological diagnostic techniques are not useful for the early diagnosis of reptilian HVs because of the delay in antibody response and the need for paired serum sample collection weeks apart with accurate timing. However, it can play an important role in retrospective studies and in the diagnosis of latent or asymptomatic patients.
Recent epidemiological studies have largely relied on molecular methods to identify potential genetic and environmental risk factors associated with reptilian HVs (24, 80, 138, 167–169). Species-specific PCR-based assays targeting specific gene segments of reptilian HVs have been developed and validated (78, 170). Lindeman et al. developed two quantitative PCR assays and recorded a detection limit as low as 1 viral copy per reaction using primers that targeted the EBHV-1 specific segment of DNA polymerase gene (UL30) (39). In another study, two TaqMan PCR assays developed to target the UL30 gene of TerHV-1 detected 10 viral copies per reaction (171). Conventional and heminested PCR assays using tortoise HV-specific primers have been developed with assay sensitivity of 103 and 101 DNA copies, respectively (172). Alternatively, consensus PCR techniques developed by VanDevanter et al. have been employed for the molecular screening and novel detection of reptilian HV species (39, 43, 100, 173–177). Although the molecular assays for the diagnosis of reptilian HVs have demonstrated excellent performance, their use still presents a major challenge in remote areas due to high cost, complexity of instrumentation, aseptic technique requirement and the need for electricity to operate PCR machines.
In order to accurately estimate the magnitude and scope of a disease outbreak or occurrence, case definition (that is, standard criteria for categorising diseases) would need to be established. One of the ways to achieve this is to make available rapid, sensitive and affordable assays for confirming the presence of diseases. Rapid diagnostic immunoassays that use lateral flow or chromatographic strategies should be developed for the rapid diagnosis of reptilian HV infections in the field or point of care (POC) settings. This approach could overcome some of the above-mentioned diagnostic challenges, especially in low resource areas. However, the use of lateral flow immunoassays for viral detection in other species have been marred by low and varying sensitivities (178–181). Sensitive molecular-based rapid assays are relatively expensive and yet to be employed for the diagnosis of reptilian HVs (182–185). We would propose an ultrasensitive format that combines PCR and immunoassay but then it can be argued that such a laboratory-based system is less rapid and has limited use in low-class laboratories (186, 187). Rapid detection techniques such as Microfluidic chip immunoassay and Smartphone-based rapid telemonitoring system (SBRTS) are fast becoming powerful tools in the diagnosis of viral infections (188–196). Of particular interest, is the SBRTS that combines biosensor and smartphone functionalities to produce a rapid, sensitive and cheap detection system (197). SBRTS has an average turnaround of 30 min, overcomes inherent problems associated with sample handling and preparation, and can remotely monitor and report data on disease occurrence, thus making it suitable for use in resource-limited countries (193, 197). This assay if employed could tick all the boxes for the epidemiological investigation and reporting of reptilian HVs.
Herpesvirus diagnostic and epidemiological data should be interpreted with prudence because of the possible influence of coinfection variables that could cause the reactivation of seemingly latent HV infections. For instance, some studies have reported the detection of co-pathogens in reptiles showing clinical signs, some of which are typical of HV infections (27, 38, 146, 168, 173, 198, 199). These observations imply that the detection of HVs may not be the actual cause of the current disease, but because the immune system is compromised by other pathogens, the HVs recrudesce and become easier to detect. Both latency (decreases apparent prevalence and significance) and coinfections (increase apparent prevalence and may also falsely assign the clinical signs to the HV) will have an influence on the disease picture. Therefore, we recommend that biosecurity and conservation measures should include a multiplex pathogen detection model whenever possible in order to fully assess the health of reptilian populations.
Treatment, Prevention, and Control
Surgical excision, carbon dioxide (CO2) laser surgery and cryosurgery are some of the commonly used therapeutic strategies for the management of HV-associated tumours (25, 110, 111, 120, 200–202). High rates of recurrence and the risk of secondary bacterial infections have greatly reduced the efficacy of surgical excision (200, 203). CO2 laser surgery, which combines laser excision and ablation of tumours, has shown improved intraoperative and postoperative outcomes and is therefore the treatment of choice (120, 201, 204). Non-surgical approaches including electrochemotherapy (ECT) and photodynamic therapy (PDT) with no known recurrence have recently been employed as alternatives in the treatment of FP (205, 206).
Several authors have recommended the use of acyclovir complemented by fluid and antibiotic therapies for the effective treatment of tortoise HV infection (143, 207–209). Marschang et al. showed that acyclovir and ganciclovir effectively inhibited HV replication in vitro at a single dose or repeated daily dose of 25 or 50 μg/mL (142). Similarly, the in vitro activities of acyclovir and ganciclovir were recently tested and shown to be effective against TeHV-3; however, the safety of these drugs is yet to be demonstrated in tortoises (210). Based on the toxicity (on liver and kidney cells) and other biochemical data, this same study showed that eprociclovir is not suitable for use as anti-TeHV-3 in Hermann's tortoises and further in vivo assessment of other potential antiviral drugs was recommended (210).
Recently, an autogenous vaccine therapy was proposed and used for the treatment of HV-associated papillomatosis in Williams' mud turtle (Pelusios williamsi) (111). The autogenous vaccine, which was aseptically prepared from excised fresh tissue induced substantial areas of necrosis of the papillomatous lesions, thus indicating the efficacy of the vaccine (111). Autogenous vaccines potentially contain relevant neoantigens that comparatively improve their efficacy (211). However, their use could be limited by lack of sufficient tumours (in patients) needed to produce adequate vaccine doses. Also, no standard protocol exists for autogenous vaccine production and delivery, and patients' tumours may progress beyond the intervention stage before the vaccine becomes ready for delivery. Allogeneic vaccines on the other hand, can overcome some of the aforementioned challenges; however, they may lack the advantageous self-neoantigens (211). In the past, an inactivated vaccine was evaluated against tortoise HV without success as no significant rise in antibody was detected in vaccinated tortoises after 369 days post vaccination (160). DNA or mRNA based vaccines have the capacity to induce both humoral and cellular immune responses and have shown promising outcomes against some animal and human diseases (212–215). Although vaccine research and development could be costly, laborious and time-consuming, the nucleic acid vaccines hold the potential to significantly reduce HV-associated losses in captive collections and wild reptiles of conservation concerns.
Prevention is of utmost importance in the management of reptilian HV infections, since death may still occur following therapeutic interventions and recovered animals remain latent carriers (143, 163). Unfortunately, there are no established preventive or control measures for HV infections in wild populations of reptiles (200), which consequently presents a major conservation challenge. Environmental factors including degraded water quality caused by pollutants, increased water temperature, natural biotoxins, and high dietary arginine concentrations due to microalgae bloom have arguably been linked as cofactors in the development of FP in sea turtles (73, 119, 216–221). Therefore, adopting conservation actions needed to regulate water and species management, as well as regulating human activities leading to climate change, would be sensible.
In captive reptiles, quarantine procedures and adequate testing of new acquisitions are strongly recommended (153, 163, 167). All previously infected or HV seropositive animals should be treated as latent carriers and potential shedders to naïve populations, as factors including stress, bad husbandry, illness or immunosuppression could reactivate the virus (14, 163, 167, 200). Generally, strict hygiene practises and adequate biosecurity should be followed in all facilities housing reptiles (162, 172, 222–224).
Author Contributions
GO and EA conceived and designed this review. GO wrote the manuscript, analysed the data, and prepared figures and tables. EA, PH, and DW contributed to the concept and reviewed drafts of the manuscript. All authors contributed to the article and approved the submitted version.
Funding
This review was supported by the College of Public Health, Medical and Veterinary Sciences Higher Degree Research Enhancement Scheme, James Cook University, Australia.
Conflict of Interest
The authors declare that the research was conducted in the absence of any commercial or financial relationships that could be construed as a potential conflict of interest.
Acknowledgments
The authors would like to especially thank Professor Elizabeth Tynan for providing editorial support to this review. Authors' special thanks also goes to Dr. Fredric Frye for providing us with the full text of some articles used in this review. Finally, we would like to thank James Cook University librarians for their technical support.
Supplementary Material
The Supplementary Material for this article can be found online at: https://www.frontiersin.org/articles/10.3389/fvets.2021.642894/full#supplementary-material
Supplementary File 1. Literature review search terms and strategies.
Supplementary File 2. Data extraction for literature review.
Supplementary File 3. Co-occurrence map of all keywords from 1975 to 2021. We found a total of 93 keywords with a minimum of 5 occurrences. Node size represents the number of occurrences of each keyword. The lines denote the total link strength between keywords, and it is proportional with the width. The line colours denote five different keyword clusters. The clusters gave an indication of the main areas research interest of reptilian HVs.
Supplementary File 4. A timeline of reptilian HV diagnosis. A review of articles conducted from 1972 to 2020 showed that VI, EM, histopathology, and serologic assays have been used for the detection of reptilian HVs since 1972. Nucleic acid detection assay (PCR) was first reported in 1998 for detection of ChHV-5 in fibropapillomas. Events were chosen based on information from the extracted articles (Supplementary File 2).
References
1. Rasmussen AR, Murphy JC, Ompi M, Gibbons JW, Uetz P. Marine reptiles. PLoS ONE. (2011) 6:e27373. doi: 10.1371/journal.pone.0027373
3. The Reptile Database [Internet]. editor [cited Febrruary 09, 2021]. Available from: http://www.reptile-database.org.
4. Gibbons JW, Scott DE, Ryan TJ, Buhlmann KA, Tuberville TD, Metts BS, et al. The Global Decline of Reptiles, Déjà Vu Amphibians: reptile species are declining on a global scale. Six significant threats to reptile populations are habitat loss and degradation, introduced invasive species, environmental pollution, disease, unsustainable use, and global climate change. BioScience. (2000) 50:653–66. doi: 10.1641/0006-3568(2000)050[0653:TGDORD]2.0.CO;2
5. Zug GR, Dowling HG. Reptile. Encyclopædia Britannica, Inc. (2020). Available online at: https://www.britannica.com/animal/reptile
6. González JA, Amich F, Postigo-Mota S, Vallejo JR. The use of wild vertebrates in contemporary Spanish ethnoveterinary medicine. J Ethnopharmacol. (2016) 191:135–51. doi: 10.1016/j.jep.2016.06.025
7. Robinson JE, Griffiths RA, John FAS, Roberts DL. Dynamics of the global trade in live reptiles: shifting trends in production and consequences for sustainability. Biol Conserv. (2015) 184:42–50. doi: 10.1016/j.biocon.2014.12.019
8. Todd BD, Willson JD, Gibbons JW. The global status of reptiles and causes of their decline. Ecotoxicol Amphibians Reptiles. (2010) 47:67. doi: 10.1201/EBK1420064162-c3
9. Van Der Ploeg JAN, Van Weerd M, Persoon GA. A cultural history of crocodiles in the Philippines: towards a new peace pact? Environ History. (2011) 17:229–64. doi: 10.3197/096734011X12997574043008
10. Reynolds J, Peres C. Overexploitation. In: Groom MJ, Meffe GK, Carroll CR, editors. Principles of Conservation Biology. Oxford, MA: Sinauer (2006). p. 253–77.
11. Ryser-Degiorgis M-P. Wildlife health investigations: needs, challenges and recommendations. BMC Vet Res. (2013) 9:223. doi: 10.1186/1746-6148-9-223
12. Munson L, Cook RA. Monitoring, investigation, and surveillance of diseases in captive wildlife. J Zoo Wildl Med. (1993) 24:281–90.
13. Haines H, Kleese WC. Effect of water temperature on a herpesvirus infection of sea turtles. Infect Immunity. (1977) 15:756–9. doi: 10.1128/IAI.15.3.756-759.1977
14. Marenzoni ML, Santoni L, Felici A, Maresca C, Stefanetti V, Sforna M, et al. Clinical, virological and epidemiological characterization of an outbreak of Testudinid Herpesvirus 3 in a chelonian captive breeding facility: lessons learned and first evidence of TeHV3 vertical transmission. PLoS ONE. (2018) 13:e0197169. doi: 10.1371/journal.pone.0197169
15. Hausmann JC, Wack AN, Allender MC, Cranfield MR, Murphy KJ, Barrett K, et al. Experimental challenge study of Fv3-like ranavirus infection in previously Fv3-like ranavirus infected eastern box turtles (Terrapene Carolina Carolina) to assess infection and survival. J Zoo Wildl Med. (2015) 46:732–46. doi: 10.1638/2015-0022.1
18. Dahlin CR, Hughes DF, Meshaka WE, Jr., Coleman C, Henning JD. Wild snakes harbor West Nile virus. One Health. (2016) 2:136–8. doi: 10.1016/j.onehlt.2016.09.003
19. Machain-Williams C, Padilla-Paz SE, Weber M, Cetina-Trejo R, Juarez-Ordaz JA, Loroño-Pino MA, et al. Antibodies to West Nile virus in wild and farmed crocodiles in southeastern Mexico. J Wildl Dis. (2013) 49:690–3. doi: 10.7589/2012-11-290
20. Habarugira G, Suen WW, Hobson-Peters J, Hall RA, Bielefeldt-Ohmann H. West Nile Virus: an update on pathobiology, epidemiology, diagnostics, control and “One Health” Implications. Pathogens. (2020) 9:589. doi: 10.3390/pathogens9070589
21. Mettenleiter TC, Keil GM, Fuchs W. Molecular Biology of Animal Herpesviruses. Animal Viruses: Molecular Biology. Norfolk: Caister Academic Press (2008) p. P531.
22. Payne S. Chapter 34 - family herpesviridae. In: Payne S, editor. Viruses. Cambridge: Academic Press (2017) p. 269–78.
23. Jacobson ER. Infectious Diseases and Pathology of Reptiles: Color Atlas and Text. CRC Press (2007).
24. Aplasca AC, Titus V, Ossiboff RJ, Murphy L, Seimon TA, Ingerman K, et al. Health assessment of free-ranging chelonians in an urban section of the Bronx river, New York, USA. J Wildl Dis. (2019) 55:352–62. doi: 10.7589/2017-12-304
25. Goe AM, Heard DJ, Abbott JR, de Mello Souza CH, Taylor KR, Sthay JN, et al. Surgical management of an odontogenic tumor in a banded Gila monster (Heloderma suspectum cinctum) with a novel herpesvirus. Vet Q. (2016) 36:109–14. doi: 10.1080/01652176.2016.1153169
26. Sharma V, Mobeen F, Prakash T. Comparative genomics of herpesviridae family to look for potential signatures of human infecting strains. Int J Genomics. (2016) 2016:9543274. doi: 10.1155/2016/9543274
27. Adamovicz L, Allender MC, Archer G, Rzadkowska M, Boers K, Phillips C, et al. Investigation of multiple mortality events in eastern box turtles (Terrapene carolina carolina). PLoS ONE. (2018) 13:e0195617. doi: 10.1371/journal.pone.0195617
28. Jungwirth N, Bodewes R, Osterhaus AD, Baumgartner W, Wohlsein P. First report of a new alphaherpesvirus in a freshwater turtle (Pseudemys concinna concinna) kept in Germany. Vet Microbiol. (2014) 170:403–7. doi: 10.1016/j.vetmic.2014.02.029
29. van Eck NJ, Waltman L. Software survey: VOSviewer, a computer program for bibliometric mapping. Scientometrics. (2010) 84:523–8. doi: 10.1007/s11192-009-0146-3
30. Fischetti M. Where the reptiles are. Sci Am. (2018) 318:80. doi: 10.1038/scientificamerican0418-80
31. Butler RA. Total Number of Reptile Species by Country. (2019). Available online at: https://rainforests.mongabay.com/03reptiles.htm.
32. International Committee on Taxonomy of Viruses. Virus Taxonomy. (2019). Available online at: https://talk.ictvonline.org/taxonomy/
33. Wilkinson M, Cline M, Jerome WG. Cytopathic herpesvirus infection in a green iguana (Iguana iguana). J Zoo Wildl Med. (2005) 36:724–6. doi: 10.1638/03-067.1
34. Wellehan JF, Jarchow JL, Reggiardo C, Jacobson ER. A novel herpesvirus associated with hepatic necrosis in a San Esteban Chuckwalla, Sauromalus varius. J Herpetol Med Surg. (2003) 13:15–9. doi: 10.5818/1529-9651.13.3.15
35. Gatherer D, Benkõ M, Brandt C, Bryant N, Dastjerdi A, Depledge D, et al. 18 New Species in the Family Herpesviridae, Order Herpesvirales (2018). Available online at: https://talk.ictvonline.org/ictv/proposals/2018.009D.A.v1.Herpesviridae_18sp.zip
36. McGeoch DJ, Gatherer D. Integrating reptilian herpesviruses into the family herpesviridae. J Virol. (2005) 79:725–31. doi: 10.1128/JVI.79.2.725-731.2005
37. Ossiboff RJ, Raphael BL, Ammazzalorso AD, Seimon TA, Newton AL, Chang TY, et al. Three novel herpesviruses of endangered Clemmys and Glyptemys turtles. PLoS ONE. (2015) 10:e0122901. doi: 10.1371/journal.pone.0122901
38. Yonkers SB, Schneider R, Reavill DR, Archer LL, Childress AL, Wellehan JF Jr. Coinfection with a novel fibropapilloma-associated herpesvirus and a novel Spirorchis sp. in an eastern box turtle (Terrapene carolina) in Florida. J Vet Diagn Investig. (2015) 27:408–13. doi: 10.1177/1040638715589612
39. Lindemann DM, Allender MC, Thompson D, Adamovicz L, Dzhaman E. Development and validation of a quantitative PCR assay for detection of Emydoidea herpesvirus 1 in free-ranging Blanding's turtles (Emydoidea blandingii). J Virol Methods. (2018) 254:40–5. doi: 10.1016/j.jviromet.2018.01.006
40. Sim RR, Norton TM, Bronson E, Allender MC, Stedman N, Childress AL, et al. Identification of a novel herpesvirus in captive Eastern box turtles (Terrapene carolina carolina). Veterinary Microbiology. (2015) 175:218–23. doi: 10.1016/j.vetmic.2014.11.029
41. Marschang RE, Heckers KO, Heynol V, Weider K, Behncke H. [Herpesvirus detection in clinically healthy West African mud turtles (Pelusios castaneus)]. Tierarztliche Praxis Ausgabe K, Kleintiere/Heimtiere. (2015) 43:166–9. doi: 10.15654/TPK-140575
42. Stacy BA, Wellehan JF, Foley AM, Coberley SS, Herbst LH, Manire CA, et al. Two herpesviruses associated with disease in wild Atlantic loggerhead sea turtles (Caretta caretta). Vet Microbiol. (2008) 126:63–73. doi: 10.1016/j.vetmic.2007.07.002
43. Bicknese EJ, Childress AL, Wellehan JF Jr. A novel herpesvirus of the proposed genus Chelonivirus from an asymptomatic bowsprit tortoise (Chersina angulata). J Zoo Wildl Med. (2010) 41:353–8. doi: 10.1638/2009-0214R.1
44. Johnson AJ, Pessier AP, Wellehan JF, Brown R, Jacobson ER. Identification of a novel herpesvirus from a California desert tortoise (Gopherus agassizii). Vet Microbiol. (2005) 111:107–16. doi: 10.1016/j.vetmic.2005.09.008
45. Marschang RE, Frost JW, Gravendyck M, Kaleta EF. Comparison of 16 chelonid herpesviruses by virus neutralization tests and restriction endonuclease digestion of viral DNA. J Vet Med Ser B. (2001) 48:393–9. doi: 10.1046/j.1439-0450.2001.00450.x
46. Marschang RE, Gleiser CB, Papp T, Pfitzner AJ, Bohm R, Roth BN. Comparison of 11 herpesvirus isolates from tortoises using partial sequences from three conserved genes. Vet Microbiol. (2006) 117:258–66. doi: 10.1016/j.vetmic.2006.06.009
47. Wellehan JF, Nichols DK, Li LL, Kapur V. Three novel herpesviruses associated with stomatitis in Sudan plated lizards (Gerrhosaurus major) and a black-lined plated lizard (Gerrhosaurus nigrolineatus). J Zoo Wildl Med. (2004) 35:50–4. doi: 10.1638/03-011
48. Wellehan JF, Johnson AJ, Latimer KS, Whiteside DP, Crawshaw GJ, Detrisac CJ, et al. Varanid herpesvirus 1: a novel herpesvirus associated with proliferative stomatitis in green tree monitors (Varanus prasinus). Vet Microbiol. (2005) 105:83–92. doi: 10.1016/j.vetmic.2004.10.012
49. Hughes-Hanks JM, Schommer SK, Mitchell WJ, Shaw DP. Hepatitis and enteritis caused by a novel herpesvirus in two monitor lizards (Varanus spp.). J Vet Diagn Investig. (2010) 22:295–9. doi: 10.1177/104063871002200224
50. Clark HF, Karzon DT. Iguana virus, a herpes-like virus isolated from cultured cells of a lizard, Iguana iguana. Infect Immunity. (1972) 5:559–69. doi: 10.1128/IAI.5.4.559-569.1972
51. Zeigel RF, Clark HF. Electron microscopy observations on a new herpes-type virus isolated from Iguana iguana and propagated in reptilian cells in vitro. Infect Immunity. (1972) 5:570–82. doi: 10.1128/IAI.5.4.570-582.1972
52. Lovstad JN, Ossiboff RJ, Kinsel MJ, Gamble KC. Novel herpesvirus associated with oropharyngeal squamous cell carcinoma in smooth green snakes (Opheodrys vernalis). Vet Pathol. (2019) 56:630–5. doi: 10.1177/0300985819837722
53. Hyndman TH, Shilton CM, Wellehan JF Jr., Davis S, Isberg SR, et al. Molecular identification of three novel herpesviruses found in Australian farmed saltwater crocodiles (Crocodylus porosus) and Australian captive freshwater crocodiles (Crocodylus johnstoni). Vet Microbiol. (2015) 181:183–9. doi: 10.1016/j.vetmic.2015.09.013
54. Rebell G, Rywlin A, Haines H. A herpesvirus-type agent associated with skin lesions of green sea turtles in aquaculture. Am J Vet Res. (1975) 36:1221–4.
55. Frye FL, Oshiro LS, Dutra FR, Carney JD. Herpesvirus-like infection in two Pacific pond turtles. J Am Vet Med Assoc. (1977) 171:882–4.
56. Cox WR, Rapley WA, Barker IK. Herpesvirus-like infection in a painted turtle (Chrysemys picta). J Wildl Dis. (1980) 16:445–9. doi: 10.7589/0090-3558-16.3.445
57. Jacobson ER, Clubb S, Gaskin JM, Gardiner C. Herpesvirus-like infection in Argentine tortoises. J Am Vet Med Assoc. (1985) 187:1227–9.
58. Davison AJ. Herpesviruses: general features. In: Mahy BWJ, Van Regenmortel MHV, editors. Encyclopedia of Virology. Oxford: Academic Press (2008). p. 430–6.
59. Kumar S, Stecher G, Li M, Knyaz C, Tamura K. MEGA X: molecular evolutionary genetics analysis across computing platforms. Mol Biol Evol. (2018) 35:1547–9. doi: 10.1093/molbev/msy096
60. Bowman BR, Baker ML, Rixon FJ, Chiu W, Quiocho FA. Structure of the herpesvirus major capsid protein. EMBO J. (2003) 22:757–65. doi: 10.1093/emboj/cdg086
61. Caspar DL, Klug A. Physical principles in the construction of regular viruses. Cold Spring Harbor Symp Quant Biol. (1962) 27:1–24. doi: 10.1101/SQB.1962.027.001.005
62. Richard J. Herpesviruses: Chapter 68. Whitley: University of Texas Medical Branch at Galveston (1996).
63. Roizmann B, Desrosiers R, Fleckenstein B, Lopez C, Minson A, Studdert M. The family Herpesviridae: an update. Arch Virol. (1992) 123:425–49. doi: 10.1007/BF01317276
64. Yuan S, Wang J, Zhu D, Wang N, Gao Q, Chen W, et al. Cryo-EM structure of a herpesvirus capsid at 3.1 Å. Science. (2018) 360:eaao7283. doi: 10.1126/science.aao7283
65. Brown JC, Newcomb WW. Herpesvirus capsid assembly: insights from structural analysis. Curr Opin Virol. (2011) 1:142–9. doi: 10.1016/j.coviro.2011.06.003
66. Dai X, Zhou ZH. Structure of the herpes simplex virus 1 capsid with associated tegument protein complexes. Science. (2018) 360:eaao7298. doi: 10.1126/science.aao7298
67. Mocarski ES Jr. Comparative analysis of herpesvirus-common proteins. In: Arvin A, Campadelli-Fiume G, Mocarski E, Moore PS, Roizman B, Whitley R, et al., editors. Human Herpesviruses: Biology, Therapy, and Immunoprophylaxis. Cambridge: Cambridge University Press (2007).
68. Hay J, Ruyechan WT. Alphaherpesvirus DNA replication. Human herpesviruses: Biology, Therapy, and Immunoprophylaxis. Cambridge: Cambridge University Press (2007).
69. Gandar F, Wilkie GS, Gatherer D, Kerr K, Marlier D, Diez M, et al. The genome of a tortoise herpesvirus (Testudinid Herpesvirus 3) has a novel structure and contains a large region that is not required for replication in vitro or virulence in vivo. J Virol. (2015) 89:11438–56. doi: 10.1128/JVI.01794-15
70. Origgi FC, Tecilla M, Pilo P, Aloisio F, Otten P, Aguilar-Bultet L, et al. A genomic approach to unravel host-pathogen interaction in chelonians: the example of testudinid herpesvirus 3. PLoS ONE. (2015) 10:e0134897. doi: 10.1371/journal.pone.0134897
71. Ackermann M, Koriabine M, Hartmann-Fritsch F, de Jong PJ, Lewis TD, Schetle N, et al. The genome of Chelonid herpesvirus 5 harbors atypical genes. PLoS ONE. (2012) 7:e46623. doi: 10.1371/journal.pone.0046623
72. Morrison CL, Iwanowicz L, Work TM, Fahsbender E, Breitbart M, Adams C, et al. Genomic evolution, recombination, and inter-strain diversity of chelonid alphaherpesvirus 5 from Florida and Hawaii green sea turtles with fibropapillomatosis. PeerJ. (2018) 2018:e4386. doi: 10.7717/peerj.4386
73. Jones K, Ariel E, Burgess G, Read M. A review of fibropapillomatosis in Green turtles (Chelonia mydas). Vet J. (2016) 212:48–57. doi: 10.1016/j.tvjl.2015.10.041
74. O'Rourke DP, Lertpiriyapong K. Chapter 19 - biology and diseases of reptiles. In: Fox JG, Anderson LC, Otto GM, Pritchett-Corning KR, Whary MT, editors. Laboratory Animal Medicine, 3rd ed. Boston, MA: Academic Press (2015) p. 967–1013.
75. Muller M, Sachsse W, Zangger N. [Herpesvirus epidemic in Greek (Testudo hermanni) and Moorish land tortoises (Testudo graeca) in Switzerland]. Schweizer Arch Tierheilkunde. (1990) 132:199–203.
76. Jones K, Burgess G, Budd AM, Huerlimann R, Mashkour N, Ariel E. Molecular evidence for horizontal transmission of chelonid alphaherpesvirus 5 at green turtle (Chelonia mydas) foraging grounds in Queensland, Australia. PLoS ONE. (2020) 15:e0227268. doi: 10.1371/journal.pone.0227268
77. Ariel E, Nainu F, Jones K, Juntunen K, Bell I, Gaston J, et al. Phylogenetic variation of chelonid alphaherpesvirus 5 (ChHV5) in Populations of Green Turtles Chelonia mydas along the Queensland Coast, Australia. J Aquat Anim Health. (2017) 29:150–7. doi: 10.1080/08997659.2017.1330783
78. Origgi FC, Romero CH, Bloom DC, Klein PA, Gaskin JM, Tucker SJ, et al. Experimental transmission of a herpesvirus in Greek tortoises (Testudo graeca). Vet Pathol. (2004) 41:50–61. doi: 10.1354/vp.41-1-50
79. Chaves A, Aguirre AA, Blanco-Peña K, Moreira-Soto A, Monge O, Torres AM, et al. Examining the role of transmission of chelonid alphaherpesvirus 5. EcoHealth. (2017) 14:530–41. doi: 10.1007/s10393-017-1248-7
80. Monezi TA, Mehnert DU, de Moura EM, Muller NM, Garrafa P, Matushima ER, et al. Chelonid herpesvirus 5 in secretions and tumor tissues from green turtles (Chelonia mydas) from Southeastern Brazil: a ten-year study. Vet Microbiol. (2016) 186:150–6. doi: 10.1016/j.vetmic.2016.02.020
81. Herbst LH, Greiner EC, Ehrhart LM, Bagley DA, Klein PA. Serological association between spirorchidiasis, herpesvirus infection, and fibropapillomatosis in green turtles from Florida. J Wildl Dis. (1998) 34:496–507. doi: 10.7589/0090-3558-34.3.496
82. Curry SS, Brown DR, Gaskin JM, Jacobson ER, Ehrhart LM, Blahak S, et al. Persistent infectivity of a disease-associated herpesvirus in green turtles after exposure to seawater. J Wildl Dis. (2000) 36:792–7. doi: 10.7589/0090-3558-36.4.792
83. Greenblatt RJ, Work TM, Balazs GH, Sutton CA, Casey RN, Casey JW. The Ozobranchus leech is a candidate mechanical vector for the fibropapilloma-associated turtle herpesvirus found latently infecting skin tumors on Hawaiian green turtles (Chelonia mydas). Virology. (2004) 321:101–10. doi: 10.1016/j.virol.2003.12.026
84. Lu Y, Yu Q, Zamzow JP, Wang Y, Losey GS, Balazs GH, et al. Detection of green turtle herpesviral sequence in Saddleback Wrasse Thalassoma Duperrey: a possible mode of transmission of green turtle fibropapilloma. J Aquat Anim Health. (2000) 12:58–63. doi: 10.1577/1548-8667(2000)012<0058:DOGTHS>2.0.CO;2
85. Work TM, Dagenais J, Balazs GH, Schettle N, Ackermann M. Dynamics of virus shedding and in situ confirmation of chelonid herpesvirus 5 in Hawaiian green turtles with fibropapillomatosis. Vet Pathol. (2015) 52:1195–201. doi: 10.1177/0300985814560236
86. Whitley R. Chapter 68: Herpesviruses. Medical Microbiology. Galveston, TX: University of Texas Medical Branch at Galveston (1996).
87. Davison AJ. Herpesviruses: General Features?. Reference Module in Biomedical Sciences. Elsevier (2014).
88. Page-Karjian A, Gottdenker NL, Whitfield J, Herbst L, Norton TM, Ritchie B. Potential noncutaneous sites of chelonid herpesvirus 5 persistence and shedding in green sea turtles Chelonia mydas. J Aquat Animal Health. (2017) 29:136–42. doi: 10.1080/08997659.2017.1321590
89. Work TM, Dagenais J, Weatherby TM, Balazs GH, Ackermann M. In vitro replication of chelonid herpesvirus 5 in organotypic skin cultures from Hawaiian green turtles (Chelonia mydas). J Virol. (2017) 91:01. doi: 10.1128/JVI.00404-17
90. Herbst LH, Jacobson ER, Klein PA, Balazs GH, Moretti R, Brown T, et al. Comparative pathology and pathogenesis of spontaneous and experimentally induced fibropapillomas of green turtles (Chelonia mydas). Vet Pathol. (1999) 36:551–64. doi: 10.1354/vp.36-6-551
91. Kang KI, Torres-Velez FJ, Zhang J, Moore PA, Moore DP, Rivera S, et al. Localization of fibropapilloma-associated turtle herpesvirus in green turtles (Chelonia mydas) by in-situ hybridization. J Comp Pathol. (2008) 139:218–25. doi: 10.1016/j.jcpa.2008.07.003
92. Engels M, Ackermann M. Pathogenesis of ruminant herpesvirus infections. Vet Microbiol. (1996) 53:3–15. doi: 10.1016/S0378-1135(96)01230-8
93. Huemer HP, Larcher C, van Drunen Littel-van den Hurk S, Babiuk LA. Species selective interaction of Alphaherpesvirinae with the “unspecific” immune system of the host. Arch Virol. (1993) 130:353–64. doi: 10.1007/BF01309666
94. Jacobs L. Glycoprotein E of pseudorabies virus and homologous proteins in other alphaherpesvirinae. Arch Virol. (1994) 137:209–28. doi: 10.1007/BF01309470
95. Haines H. A herpesvirus disease of green sea turtles in aquaculture. Mar Fish Rev. (1978) 40:330–37.
96. Haines HG, Rywlin A, Rebell G. A herpesvirus disease of farmed green turtles (Chelonia mydas). J World Aquac Soc. (1974) 5:183–95. doi: 10.1111/j.1749-7345.1974.tb00189.x
97. Herbst LH. Fibropapillomatosis of marine turtles. Ann Rev Fish Dis. (1994) 4:389–425. doi: 10.1016/0959-8030(94)90037-X
98. Jacobson ER, Gaskin JM, Roelke M, Greiner EC, Allen J. Conjunctivitis, tracheitis, and pneumonia associated with herpesvirus infection in green sea turtles. J Am Vet Med Assoc. (1986) 189:1020–3.
99. Une Y, Uemura K, Nakano Y, Kamiie J, Ishibashi T, Nomura Y. Herpesvirus infection in tortoises (Malacochersus tornieri and Testudo horsfieldii). Vet Pathol. (1999) 36:624–7. doi: 10.1354/vp.36-6-624
100. Une Y, Murakami M, Uemura K, Fujitani H, Ishibashi T, Nomura Y. Polymerase chain reaction (PCR) for the detection of herpesvirus in tortoises. J Vet Med Sci. (2000) 62:905–7. doi: 10.1292/jvms.62.905
101. Stöhr AC, Marschang RE. Detection of a tortoise herpesvirus type 1 in a Hermann's Tortoise (Testudo hermanni boettgeri) in Germany. J Herpetol Med Surg. (2010) 20:61–3. doi: 10.5818/1529-9651-20.2.61
102. Marschang RE, Papp T, Ferretti L, Hochscheid S, Bentivegna F. Detection and partial characterization of herpesviruses from Egyptian tortoises (Testudo kleinmanni) imported into Italy from Libya. J Zoo Wildl Med. (2009) 40:211–3. doi: 10.1638/2008-0045.1
103. Heldstab A, Bestetti G. Herpesviridae causing glossitis and meningoencephalitis in land tortoises (Testudo hermanni). Herpetopathologia. (1989) 1:5–9.
104. Kolesnik E, Mittenzwei F, Marschang RE. Detection of testudinid herpesvirus type 4 in a leopard tortoise (Stigmochelys pardalis). Tierarztliche Praxis Ausgabe K Kleintiere/Heimtiere. (2016) 44:283–6. doi: 10.15654/TPK-150843
105. Ossiboff RJ, Newton AL, Seimon TA, Moore RP, McAloose D. Emydid herpesvirus 1 infection in northern map turtles (Graptemys geographica) and painted turtles (Chrysemys picta). J Vet Diagn Investig. (2015) 27:392–5. doi: 10.1177/1040638715584793
106. Simpson CF, Jacobson ER, Gaskin JM. Herpesvirus-like infection of the venom gland of Siamese cobras. J Am Vet Med Assoc. (1979) 175:941–3.
107. Shilton CM, Jerrett IV, Davis S, Walsh S, Benedict S, Isberg SR, et al. Diagnostic investigation of new disease syndromes in farmed Australian saltwater crocodiles (Crocodylus porosus) reveals associations with herpesviral infection. J Vet Diagn Investig. (2016) 28:279–90. doi: 10.1177/1040638716642268
108. Aguirre AA, Spraker TR, Chaves A, Du Toit L, Eure W, Balazs GH. Pathology of fibropapillomatosis in olive ridley turtles lepidochelys olivacea nesting in costa rica. J Aquat Anim Health. (1999) 11:283–9. doi: 10.1577/1548-8667(1999)011<0283:POFIOR>2.0.CO;2
109. Cardenas DM, Cucalon RV, Medina-Magues LG, Jones K, Aleman RA, Alfaro-Nunez A, et al. Fibropapillomatosis in a Green Sea Turtle (Chelonia mydas) from the Southeastern Pacific. J Wildl Dis. (2019) 55:169–73. doi: 10.7589/2017-12-295
110. Cowan ML, Raidal SR, Peters A. Herpesvirus in a captive Australian Krefft's river turtle (Emydura macquarii krefftii). Aust Vet J. (2015) 93:46–9. doi: 10.1111/avj.12290
111. Siroky P, Frye FL, Dvorakova N, Hostovsky M, Prokop H, Kulich P. Herpesvirus associated dermal papillomatosis in Williams' mud turtle Pelusios williamsi with effects of autogenous vaccine therapy. J Vet Med Sci. (2018) 80:1248–54. doi: 10.1292/jvms.18-0126
112. Hervas J, Sanchez-Cordon PJ, de Chacon Lara F, Carrasco L, Gomez-Villamandos JC. Hepatitis associated with herpes viral infection in the tortoise (Testudo horsfieldii). J Vet Med Ser B. (2002) 49:111–4. doi: 10.1046/j.1439-0450.2002.00522.x
113. Hunt CJ. Herpesvirus outbreak in a group of mediterranean tortoises (Testudo spp). Vet Clin N Am Exot Anim Pract. (2006) 9:569–74. doi: 10.1016/j.cvex.2006.05.018
114. Braune S, Geiss V, Thiel W. A new herpesvirus-caused disease in tortoises. Tierarztliche Praxis. (1989) 17:416–9.
115. McArthur S, Blahak S, Köelle P, Jacobson ER, Marschang RE, Origgi F, et al. Chelonian herpesvirus. J Herpetol Med Surg. (2002) 12:14–31. doi: 10.5818/1529-9651.12.2.14
116. Raynaud A, Adrian M. [Cutaneous lesions with papillomatous structure associated with viruses in the green lizard (Lacerta viridis Laur.)]. Comptes Rendus Hebdomadaires Seances l'Academie Sciences - D: Sciences Naturelles. (1976) 283:845–7.
117. Literak I, Robesova B, Majlathova V, Majlath I, Kulich P, Fabian P, et al. Herpesvirus-associated papillomatosis in a green lizard. J Wildl Dis. (2010) 46:257–61. doi: 10.7589/0090-3558-46.1.257
118. Govett PD, Harms CA, Johnson AJ, Latimer KS, Wellehan JF, Fatzinger MH, et al. Lymphoid follicular cloacal inflammation associated with a novel herpesvirus in juvenile alligators (Alligator mississippiensis). J Vet Diagn Investig. (2005) 17:474–9. doi: 10.1177/104063870501700513
119. dos Santos RG, Martins AS, Torezani E, Baptistotte C, da Nóbrega FJ, Horta PA, et al. Relationship between fibropapillomatosis and environmental quality: a case study with Chelonia mydas of Brazil. Dis Aquat Organ. (2010) 89:87–95. doi: 10.3354/dao02178
120. Page-Karjian A, Norton TM, Krimer P, Groner M, Nelson SE, Jr., et al. Factors influencing survivorship of rehabilitating green sea turtles (Chelonia mydas) with fibropapillomatosis. J Zoo Wildl Med. (2014) 45:507–19. doi: 10.1638/2013-0132R1.1
121. Tagliolatto AB, Guimarães SM, Lobo-Hajdu G, Monteiro-Neto C. Characterization of fibropapillomatosis in green turtles Chelonia mydas (Cheloniidae) captured in a foraging area in southeastern Brazil. Dis Aquat Organ. (2016) 121:233–40. doi: 10.3354/dao03020
122. Adnyana W, Ladds PW, Blair D. Observations of fibropapillomatosis in green turtles (Chelonia mydas) in Indonesia. Aust Vet J. (1997) 75:736–42. doi: 10.1111/j.1751-0813.1997.tb12258.x
123. Ene A, Su M, Lemaire S, Rose C, Schaff S, Moretti R, et al. Distribution of chelonid fibropapillomatosis-associated herpesvirus variants in Florida: molecular genetic evidence for infection of turtles following recruitment to neritic developmental habitats. J Wildl Dis. (2005) 41:489–97. doi: 10.7589/0090-3558-41.3.489
124. Greenblatt RJ, Quackenbush SL, Casey RN, Rovnak J, Balazs GH, Work TM, et al. Genomic variation of the fibropapilloma-associated marine turtle herpesvirus across seven geographic areas and three host species. J Virol. (2005) 79:1125–32. doi: 10.1128/JVI.79.2.1125-1132.2005
125. Zimmerman LM, Vogel LA, Bowden RM. Understanding the vertebrate immune system: insights from the reptilian perspective. J Exp Biol. (2010) 213:661. doi: 10.1242/jeb.038315
126. Rios FM, Zimmerman LM. Immunology of Reptiles. eLS, Chichester: John Wiley & Sons, Ltd. (2015). p. 1–7.
127. Herbst LH, Klein PA. Green Turtle fibropapillomatosis: challenges to assessing the role of environmental cofactors. Environ Health Perspect. (1995) 103(Suppl 4):27–30. doi: 10.1289/ehp.95103s427
128. Herbst L, Ene A, Su M, Desalle R, Lenz J. Tumor outbreaks in marine turtles are not due to recent herpesvirus mutations. Curr Biol. (2004) 14:R697–9. doi: 10.1016/j.cub.2004.08.040
129. Jones AG. Sea turtles: old viruses and new tricks. Curr Biol. (2004) 14:R842–3. doi: 10.1016/j.cub.2004.09.038
130. Winter AK, Martinez ME, Cutts FT, Moss WJ, Ferrari MJ, McKee A, et al. Benefits and challenges in using seroprevalence data to inform models for measles and rubella elimination. J Infect Dis. (2018) 218:355–64. doi: 10.1093/infdis/jiy137
131. Willimann A. The prevalence of antibodies against envelope proteins of Chelonid herpesvirus 5 is inconsistent with the current understanding of the pathogenesis and epidemiology of fibropapillomatosis in marine turtles (Doctoral dissertation), University of Zurich, Zurich (2018).
132. Herbst LH, Lemaire S, Ene AR, Heslin DJ, Ehrhart LM, Bagley DA, et al. Use of baculovirus-expressed glycoprotein H in an enzyme-linked immunosorbent assay developed to assess exposure to chelonid fibropapillomatosis-associated herpesvirus and its relationship to the prevalence of fibropapillomatosis in sea turtles. Clin Vaccine Immunol. (2008) 15:843–51. doi: 10.1128/CVI.00438-07
133. Work TM, Dagenais J, Willimann A, Balazs G, Mansfield K, Ackermann M. Differences in antibody responses against chelonid alphaherpesvirus 5 (CHHV5) suggest differences in virus biology in CHHV5-seropositive green turtles from Hawaii and CHHV5-seropositive green turtles from Florida. J Virol. (2020) 94:e00404-17. doi: 10.1128/JVI.01658-19
134. Coberley SS, Herbst LH, Brown DR, Ehrhart LM, Bagley DA, Schaf SA, et al. Detection of antibodies to a disease-associated herpesvirus of the green turtle, Chelonia mydas. J Clin Microbiol. (2001) 39:3572–7. doi: 10.1128/JCM.39.10.3572-3577.2001
135. Coberley SS, Herbst LH, Ehrhart LM, Bagley DA, Hirama S, Jacobson ER, et al. Survey of Florida green turtles for exposure to a disease-associated herpesvirus. Dis Aquat Org. (2001) 47:159–67. doi: 10.3354/dao047159
136. Jacobson ER, Gaskin JM, Wahlquist H. Herpesvirus-like infection in map turtles. J Am Vet Med Assoc. (1982) 181:1322–4.
137. Divers SJ, Stahl SJ. Mader's Reptile and Amphibian Medicine and Surgery- E-Book. Amsterdam: Elsevier Health Sciences (2018).
138. Kane LP, Allender MC, Archer G, Dzhaman E, Pauley J, Moore AR, et al. Prevalence of terrapene herpesvirus 1 in free-ranging eastern box turtles (Terrapene carolina Carolina) in Tennessee and Illinois, USA. J Wildl Dis. (2017) 53:285–95. doi: 10.7589/2016-06-138
139. Pettan-Brewer KC, Drew ML, Ramsay E, Mohr FC, Lowenstine LJ. Herpesvirus particles associated with oral and respiratory lesions in a California desert tortoise (Gopherus agassizii). J Wildl Dis. (1996) 32:521–6. doi: 10.7589/0090-3558-32.3.521
140. Lange H, Herbst W, Wiechert JM, Schliesser T. [Electron microscopic detection of herpesviruses in a mass death of Greek tortoises (Testudo hermanni) and four-toed turtles (Agrionemys horsfieldii)]. Tierarztliche Praxis. (1989) 17:319–21.
141. Muro J, Ramis A, Pastor J, Velarde R, Tarres J, Lavin S. Chronic rhinitis associated with herpesviral infection in captive spur-thighed tortoises from Spain. J Wildl Dis. (1998) 34:487–95. doi: 10.7589/0090-3558-34.3.487
142. Marschang RE, Gravendyck M, Kaleta EF. Herpesviruses in tortoises: investigations into virus isolation and the treatment of viral stomatitis in Testudo hermanni and T. graeca. Zentralblatt Fuer Veterinaermed Reihe B. (1997) 44:385–94. doi: 10.1111/j.1439-0450.1997.tb00989.x
143. Origgi FC, Rigoni D, editors. A tortoise herpesvirus outbreak in Italy: history, diagnosis, therapy and follow up. In: Proceedings of the 10th Conference of the Association of Reptilian and Amphibian Veterinarians. Minneapolis, MI. (2003).
144. Origgi FC. Testudinid Herpesviruses: a review. J Herpetol Med Surg. (2012) 22:42–54. doi: 10.5818/1529-9651-22.1-2.42
145. Marschang RE. Emerging reptile viruses. Fowler's Zoo and Wild Animal Medicine. Curr Ther. (2019) 9:267. doi: 10.1016/B978-0-323-55228-8.00039-4
146. Kolesnik E, Obiegala A, Marschang RE. Detection of Mycoplasma spp., herpesviruses, topiviruses, and ferlaviruses in samples from chelonians in Europe. J Vet Diagn Investig. (2017) 29:820–32. doi: 10.1177/1040638717722387
147. Jacobson ER, Berry KH, Wellehan JF Jr., Origgi F, Childress AL, et al. Serologic and molecular evidence for Testudinid herpesvirus 2 infection in wild Agassiz's desert tortoises, Gopherus agassizii. J Wildl Dis. (2012) 48:747–57. doi: 10.7589/0090-3558-48.3.747
148. Johnson AJ, Morafka DJ, Jacobson ER. Seroprevalence of Mycoplasma agassizii and tortoise herpesvirus in captive desert tortoises (Gopherus agassizii) from the Greater Barstow Area, Mojave Desert, California. J Arid Environ. (2006) 67:192–201. doi: 10.1016/j.jaridenv.2006.09.025
149. McCowan C, Shepherdley C, Slocombe RF. Herpesvirus-like particles in the skin of a saltwater crocodile (Crocodylus porosus). Aust Vet J. (2004) 82:375–7. doi: 10.1111/j.1751-0813.2004.tb11109.x
150. Catoi C, Gal AF, Taulescu MA, Palmieri C, Catoi AF. Lethal herpesvirosis in 16 captive horned vipers (Vipera ammodytes ammodytes): pathological and ultrastructural findings. J Comp Pathol. (2014) 150:341–4. doi: 10.1016/j.jcpa.2013.10.002
151. Hauser B, Mettler F, Rubel A. Herpesvirus-like infection in two young Boas. J Comp Pathol. (1983) 93:515–9. doi: 10.1016/0021-9975(83)90057-9
152. Watson GL. Herpesvirus in red-headed (common) agamas (Agama agama). J Vet Diagn Investig. (1993) 5:444–5. doi: 10.1177/104063879300500326
153. Lott MJ, Moore RL, Milic NL, Power M, Shilton CM, Isberg SR. Dermatological conditions of farmed Crocodilians: a review of pathogenic agents and their proposed impact on skin quality. Vet Microbiol. (2018) 225:89–100. doi: 10.1016/j.vetmic.2018.09.022
154. Divers SJ, Mader DR. Reptile Medicine and Surgery-E-Book. Amsterdam: Elsevier Health Sciences (2005).
155. Farkas SL, Gál J. Adenovirus and mycoplasma infection in an ornate box turtle (Terrapene ornata ornata) in Hungary. Vet Microbiol. (2009) 138:169–73. doi: 10.1016/j.vetmic.2009.03.016
156. Manire CA, Stacy BA, Kinsel MJ, Daniel HT, Anderson ET, Wellehan JF Jr. Proliferative dermatitis in a loggerhead turtle, Caretta caretta, and a green turtle, Chelonia mydas, associated with novel papillomaviruses. Vet Microbiol. (2008) 130:227–37. doi: 10.1016/j.vetmic.2008.01.013
157. Hyndman T, Shilton CM. Molecular detection of two adenoviruses associated with disease in Australian lizards. Aust Vet J. (2011) 89:232–5. doi: 10.1111/j.1751-0813.2011.00712.x
158. Harper PA, Hammond DC, Heuschele WP. A herpesvirus-like agent associated with a pharyngeal abscess in a desert tortoise. J Wildl Dis. (1982) 18:491–4. doi: 10.7589/0090-3558-18.4.491
159. Drury SE, Gough RE, Kay AV, McArthur SD. Detection and isolation of a herpesvirus from a spur-thighed tortoise (Testudo graeca) in the UK. Vet Rec. (1999) 145:586–8. doi: 10.1136/vr.145.20.586
160. Marschang RE, Milde K, Bellavista M. Virus isolation and vaccination of Mediterranean tortoises against a chelonid herpesvirus in a chronically infected population in Italy. Deutsche Tierarztliche Wochenschrift. (2001) 108:376–9.
161. Zimmerman LM, Bowden RM, Vogel LA. Red-eared slider turtles lack response to immunization with keyhole limpet hemocyanin but have high levels of natural antibodies. ISRN Zool. (2013) 2013:858941. doi: 10.1155/2013/858941
162. Origgi FC, Klein PA, Mathes K, Blahak S, Marschang RE, Tucker SJ, et al. Enzyme-linked immunosorbent assay for detecting herpesvirus exposure in Mediterranean tortoises (spur-thighed tortoise [Testudo graeca] and Hermann's tortoise [Testudo hermanni]). J Clin Microbiol. (2001) 39:3156–63. doi: 10.1128/JCM.39.9.3156-3163.2001
163. Pasmans F, Blahak S, Martel A, Pantchev N. Introducing reptiles into a captive collection: the role of the veterinarian. Vet J. (2008) 175:53–68. doi: 10.1016/j.tvjl.2006.12.009
164. Origgi FC, Klein PA, Tucker SJ, Jacobson ER. Application of immunoperoxidase-based techniques to detect herpesvirus infection in tortoises. J Vet Diagn Investig. (2003) 15:133–40. doi: 10.1177/104063870301500207
165. Marschang RE, Schneider RM. Antibodies to viruses in wild-caught spur-thighed tortoises (Testudo graeca) in Turkey. Vet Rec. (2007) 161:102–3. doi: 10.1136/vr.161.3.102
166. Nie YC, Lu CP. Antibody against Testudo herpesvirus is not common in Chinese soft-shelled turtles. Zentralblatt Fuer Veterinaermed Reihe B. (1999) 46:731–4. doi: 10.1046/j.1439-0450.1999.00292.x
167. Martel A, Blahak S, Vissenaekens H, Pasmans F. Reintroduction of clinically healthy tortoises: the herpesvirus Trojan horse. J Wildl Dis. (2009) 45:218–20. doi: 10.7589/0090-3558-45.1.218
168. Sim RR, Allender MC, Crawford LK, Wack AN, Murphy KJ, Mankowski JL, et al. Ranavirus epizootic in captive eastern box turtles (Terrapene carolina carolina) with concurrent herpesvirus and mycoplasma infection: management and monitoring. J Zoo Wildl Med. (2016) 47:256–70. doi: 10.1638/2015-0048.1
169. Lawrance MF, Mansfield KL, Sutton E, Savage AE. Molecular evolution of fibropapilloma-associated herpesviruses infecting juvenile green and loggerhead sea turtles. Virology. (2018) 521:190–7. doi: 10.1016/j.virol.2018.06.012
170. Braun J, Schrenzel M, Witte C, Gokool L, Burchell J, Rideout BA. Molecular methods to detect Mycoplasma spp. and Testudinid herpesvirus 2 in desert tortoises (Gopherus agassizii) and implications for disease management. J Wildl Dis. (2014) 50:757–66. doi: 10.7589/2013-09-231
171. Kane LP, Bunick D, Abd-Eldaim M, Dzhaman E, Allender MC. Development and validation of quantitative PCR for detection of Terrapene herpesvirus 1 utilizing free-ranging eastern box turtles (Terrapene carolina carolina). J Virol Methods. (2016) 232:57–61. doi: 10.1016/j.jviromet.2016.02.002
172. Murakami M, Matsuba C, Une Y, Nomura Y, Fujitani H. Development of species-specific PCR techniques for the detection of tortoise herpesvirus. J Vet Diagn Investig. (2001) 13:513–6. doi: 10.1177/104063870101300610
173. Salinas M, Francino O, Sanchez A, Altet L. Mycoplasma and herpesvirus PCR detection in tortoises with rhinitis-stomatitis complex in Spain. J Wildl Dis. (2011) 47:195–200. doi: 10.7589/0090-3558-47.1.195
174. Lu Y, Wang Y, Yu Q, Aguirre AA, Balazs GH, Nerurkar VR, et al. Detection of herpesviral sequences in tissues of green turtles with fibropapilloma by polymerase chain reaction. Arch Virol. (2000) 145:1885–93. doi: 10.1007/s007050070063
175. Lackovich JK, Brown DR, Homer BL, Garber RL, Mader DR, Moretti RH, et al. Association of herpesvirus with fibropapillomatosis of the green turtle Chelonia mydas and the loggerhead turtle Caretta caretta in Florida. Dis Aquat Organ. (1999) 37:89–97. doi: 10.3354/dao037089
176. Quackenbush SL, Work TM, Balazs GH, Casey RN, Rovnak J, Chaves A, et al. Three closely related herpesviruses are associated with fibropapillomatosis in marine turtles. Virology. (1998) 246:392–9. doi: 10.1006/viro.1998.9207
177. VanDevanter DR, Warrener P, Bennett L, Schultz ER, Coulter S, Garber RL, et al. Detection and analysis of diverse herpesviral species by consensus primer PCR. J Clin Microbiol. (1996) 34:1666–71. doi: 10.1128/JCM.34.7.1666-1671.1996
178. Fedorko DP, Nelson NA, McAuliffe JM, Subbarao K. Performance of rapid tests for detection of avian influenza A virus types H5N1 and H9N2. J Clin Microbiol. (2006) 44:1596–7. doi: 10.1128/JCM.44.4.1596-1597.2006
179. Padgett KA, Cahoon-Young B, Carney R, Woods L, Read D, Husted S, et al. Field and laboratory evaluation of diagnostic assays for detecting West Nile virus in oropharyngeal swabs from California wild birds. Vector Borne Zoonot Dis. (2006) 6:183–91. doi: 10.1089/vbz.2006.6.183
180. Yu J, Lin Y, Cao Y, Li X, Liao D, Ye Y, et al. Development and application of a colloidal gold test strip for the rapid detection of the infectious laryngotracheitis virus. Poult Sci. (2020) 99:2407–15. doi: 10.1016/j.psj.2019.11.066
181. Chen H, Zhang X, Jin Z, Huang L, Dan H, Xiao W, et al. Differential diagnosis of PRV-infected versus vaccinated pigs using a novel EuNPs-virus antigen probe-based blocking fluorescent lateral flow immunoassay. Biosens Bioelectr. (2020) 155:112101. doi: 10.1016/j.bios.2020.112101
182. Lemieux B, Li Y, Kong H, Tang Y-W. Near instrument-free, simple molecular device for rapid detection of herpes simplex viruses. Expert Rev Mol Diagn. (2012) 12:437–43. doi: 10.1586/erm.12.34
183. Kohda C, Chiba N, Shimokoba K, Mizuno K, Negoro T, Nakano Y, et al. A simple smart amplification assay for the rapid detection of human cytomegalovirus in the urine of neonates. J Virol Methods. (2014) 208:160–5. doi: 10.1016/j.jviromet.2014.07.034
184. Shojaei TR, Tabatabaei M, Shawky S, Salleh MAM, Bald D. A review on emerging diagnostic assay for viral detection: the case of avian influenza virus. Mol Biol Rep. (2015) 42:187–99. doi: 10.1007/s11033-014-3758-5
185. Voermans JJC, Seven-Deniz S, Fraaij PLA, van der Eijk AA, Koopmans MPG, Pas SD. Performance evaluation of a rapid molecular diagnostic, MultiCode based, sample-to-answer assay for the simultaneous detection of Influenza A, B and respiratory syncytial viruses. J Clin Virol. (2016) 85:65–70. doi: 10.1016/j.jcv.2016.10.019
186. Loose FN, Breitbach A, Bertalan I, Rüster D, Truyen U, Speck S. Diagnostic validation of a rapid and field-applicable PCR-lateral flow test system for point-of-care detection of cyprinid herpesvirus 3 (CyHV-3). PLoS One. (2020) 15:e0241420. doi: 10.1371/journal.pone.0241420
187. Wang H, Sun M, Xu D, Podok P, Xie J, Jiang Y, et al. Rapid visual detection of cyprinid herpesvirus 2 by recombinase polymerase amplification combined with a lateral flow dipstick. J Fish Dis. (2018) 28:28. doi: 10.1111/jfd.12808
188. Sun F, Ganguli A, Nguyen J, Brisbin R, Shanmugam K, Hirschberg DL, et al. Smartphone-based multiplex 30-minute nucleic acid test of live virus from nasal swab extract. Lab Chip. (2020) 20:1621–7. doi: 10.1039/D0LC00304B
189. Zhu H, Fohlerová Z, Pekárek J, Basova E, NeuŽil P. Recent advances in lab-on-a-chip technologies for viral diagnosis. Biosens Bioelectr. (2020) 153:112041. doi: 10.1016/j.bios.2020.112041
190. Phillips EA, Moehling TJ, Ejendal KFK, Hoilett OS, Byers KM, Basing LA, et al. Microfluidic rapid and autonomous analytical device (microRAAD) to detect HIV from whole blood samples. Lab Chip. (2019) 19:3375–86. doi: 10.1039/C9LC00506D
191. Rodriguez-Moncayo R, Cedillo-Alcantar DF, Guevara-Pantoja PE, Chavez-Pineda OG, Hernandez-Ortiz JA, Amador-Hernandez JU, et al. A high-throughput multiplexed microfluidic device for COVID-19 serology assays. Lab Chip. (2021) 21:93–104. doi: 10.1039/D0LC01068E
192. Yu X, Xia Y, Tang Y, Zhang WL, Yeh YT, Lu H, et al. A nanostructured microfluidic immunoassay platform for highly sensitive infectious pathogen detection. Small. (2017) 13:1700425. doi: 10.1002/smll.201700425
193. Natesan M, Wu SW, Chen CI, Jensen SMR, Karlovac N, Dyas BK, et al. A smartphone-based rapid telemonitoring system for Ebola and Marburg disease surveillance. ACS Sens. (2019) 4:61–8. doi: 10.1021/acssensors.8b00842
194. Priye A, Bird SW, Light YK, Ball CS, Negrete OA, Meagher RJ. A smartphone-based diagnostic platform for rapid detection of Zika, Chikungunya, and Dengue viruses. Sci Rep. (2017) 7:44778. doi: 10.1038/srep44778
195. Yeo SJ, Cuc BT, Sung HW, Park H. Evaluation of a smartphone-based rapid fluorescent diagnostic system for H9N2 virus in specific-pathogen-free chickens. Arch Virol. (2016) 161:2249–56. doi: 10.1007/s00705-016-2922-8
196. Yeo SJ, Kang H, Dao TD, Cuc BT, Nguyen ATV, Tien TTT, et al. Development of a smartphone-based rapid dual fluorescent diagnostic system for the simultaneous detection of influenza A and H5 subtype in avian influenza A-infected patients. Theranostics. (2018) 8:6132–48. doi: 10.7150/thno.28027
197. Zhao W, Tian S, Huang L, Liu K, Dong L, Guo J. A smartphone-based biomedical sensory system. Analyst. (2020) 145:2873–91. doi: 10.1039/C9AN02294E
198. Soares JF, Chalker VJ, Erles K, Holtby S, Waters M, McArthur S. Prevalence of Mycoplasma agassizii and Chelonian herpesvirus in captive tortoises (Testudo sp.) in the United Kingdom. J Zoo Wildl Med. (2004) 35:25–33. doi: 10.1638/02-092
199. Archer GA, Phillips CA, Adamovicz L, Band M, Byrd J, Allender MC. Detection of copathogens in free-ranging Eastern Box Turtles (Terrapene carolina carolina) in Illinois and Tennessee. J Zoo Wildl Med. (2017) 48:1127–34. doi: 10.1638/2017-0148R.1
200. CABI. Fibropapillomatosis of Sea Turtles [original text by Chris A. Whitehouse]. Wallingford: CAB International (2019). Available online at: www.cabi.org/isc
201. Raiti P. Carbon Dioxide (CO2) Laser treatment of cutaneous papillomas in a common snapping turtle, Chelydra serpentina. J Zoo Wildl Med. (2008) 39:252–65. doi: 10.1638/2007-0055R.1
202. Schroeder BA, Witherington BE. Proceedings of the Thirteenth Annual Symposium on Sea Turtle Biology and Conservation. NOAA Technical Memorandum NMFS-SEFSC-341. Jekyll Island, GA (1994). p. 281.
203. Page-Karjian A, Perrault JR, Zirkelbach B, Pescatore J, Riley R, Stadler M, et al. Tumor re-growth, case outcome, and tumor scoring systems in rehabilitated green turtles with fibropapillomatosis. Dis Aquat Org. (2019) 137:101–8. doi: 10.3354/dao03426
204. Glazkova A. Treating sea turtle fibropapillomatosis with CO2 laser surgery. Veterinary Practice News. (2015). p. 34–5.
205. Brunner CH, Dutra G, Silva CB, Silveira LM, Martins Mde F. Electrochemotherapy for the treatment of fibropapillomas in Chelonia mydas. J Zoo Wildl Med. (2014) 45:213–8. doi: 10.1638/2010-0125.1
206. Sellera FP, Sabino CP, Fernandes LT, Pogliani FC, Teixeira CR, Dutra GH, et al. Green turtle (Chelonia mydas) cutaneous fibropapillomatosis treatment by photodynamic therapy. Mar Turt Newsl. (2014) 142:1–31.
207. McArthur SDJ. An acyclovir trial in Testudo sp. In: Proceedings of the BVZS, Spring Meeting, Emerging Diseases London (2000).
208. Origgi F. Herpesvirus in Tortoises. Reptile Medicine and Surgery, 2nd ed. St Louis, MO: Saunders (2006). p. 814–21.
209. Wright K, editor. How I treat herpesvirus in tortoises, small animal and exotics. In: Proceedings of the North American Veterinary Conference. Orlando, FL: The North American Veterinary Conference (2008).
210. Gandar F, Marlier D, Vanderplasschen A. In vitro and in vivo assessment of eprociclovir as antiviral treatment against testudinid herpesvirus 3 in Hermann's tortoise (Testudo hermanni). Res Vet Sci. (2019) 124:20–3. doi: 10.1016/j.rvsc.2019.02.001
211. Sondak VK, Sabel MS, Mulé JJ. Allogeneic and autologous melanoma vaccines: where have we been and where are we going? Clin Cancer Res. (2006) 12:2337s. doi: 10.1158/1078-0432.CCR-05-2555
212. Leal L, Guardo AC, Morón-López S, Salgado M, Mothe B, Heirman C, et al. Phase I clinical trial of an intranodally administered mRNA-based therapeutic vaccine against HIV-1 infection. AIDS. (2018) 32:2533–45. doi: 10.1097/QAD.0000000000002026
213. Deb R, Dey S, Madhan Mohan C, Gaikwad S, Kamble N, Khulape SA, et al. Development and evaluation of a Salmonella typhimurium flagellin based chimeric DNA vaccine against infectious bursal disease of poultry. Res Vet Sci. (2015) 102:7–14. doi: 10.1016/j.rvsc.2015.07.004
214. Brisse M, Vrba SM, Kirk N, Liang Y, Ly H. Emerging concepts and technologies in vaccine development. Front Immunol. (2020) 11:583077. doi: 10.3389/fimmu.2020.583077
215. Jackson LA, Anderson EJ, Rouphael NG, Roberts PC, Makhene M, Coler RN, et al. An mRNA vaccine against SARS-CoV-2 - preliminary report. N Engl J Med. (2020) 383:1920–31. doi: 10.1056/NEJMoa2022483
216. Foley AM, Schroeder BA, Redlow AE, Fick-Child KJ, Teas WG. Fibropapillomatosis in stranded green turtles (Chelonia mydas) from the eastern United States (1980-98): trends and associations with environmental factors. J Wildl Dis. (2005) 41:29–41. doi: 10.7589/0090-3558-41.1.29
217. Aguirre AA, Balazs GH, Zimmerman B, Galey FD. Organic contaminants and trace metals in the tissues of green turtles (Chelonia mydas) afflicted with fibropapillomas in the Hawaiian Islands. Mar Pollut Bull. (1994) 28:109–14. doi: 10.1016/0025-326X(94)90547-9
218. Van Houtan KS, Hargrove SK, Balazs GH. Land use, macroalgae, and a tumor-forming disease in marine turtles. PLoS ONE. (2010) 5:29. doi: 10.1371/journal.pone.0012900
219. Landsberg JH, Balazs GH, Steidinger KA, Baden DG, Work TM, Russell DJ. The potential role of natural tumor promoters in marine turtle fibropapillomatosis. J Aquat Anim Health. (1999) 11:199–210. doi: 10.1577/1548-8667(1999)011<0199:TPRONT>2.0.CO;2
220. Arthur K, Limpus C, Balazs G, Capper A, Udy J, Shaw G, et al. The exposure of green turtles (Chelonia mydas) to tumour promoting compounds produced by the cyanobacterium Lyngbya majuscula and their potential role in the aetiology of fibropapillomatosis. Harmful Algae. (2008) 7:114–25. doi: 10.1016/j.hal.2007.06.001
221. Van Houtan KS, Smith CM, Dailer ML, Kawachi M. Eutrophication and the dietary promotion of sea turtle tumors. PeerJ. (2014) 2:e602. doi: 10.7717/peerj.602
222. Stahl SJ, editor. Reptile production medicine. Semin Avian Exot Pet Med. (2001) 10:140–50. doi: 10.1053/saep.2001.24256
223. International Union for Conservation of Nature (IUCN). Red List of Threatened Species. (2020). Available online at: https://www.iucnredlist.org
Keywords: herpesviruses, reptiles, fibropapillomatosis, taxonomy, pathogenesis, pathology, epidemiology
Citation: Okoh GR, Horwood PF, Whitmore D and Ariel E (2021) Herpesviruses in Reptiles. Front. Vet. Sci. 8:642894. doi: 10.3389/fvets.2021.642894
Received: 17 December 2020; Accepted: 06 April 2021;
Published: 05 May 2021.
Edited by:
Amanda Linda Jean Duffus, Gordon State College, United StatesReviewed by:
Francisco Rivera-Benítez, Instituto Nacional de Investigaciones Forestales, Agrícolas y Pecuarias (INIFAP), MexicoAnnie Page-Karjian, Florida Atlantic University, United States
Copyright © 2021 Okoh, Horwood, Whitmore and Ariel. This is an open-access article distributed under the terms of the Creative Commons Attribution License (CC BY). The use, distribution or reproduction in other forums is permitted, provided the original author(s) and the copyright owner(s) are credited and that the original publication in this journal is cited, in accordance with accepted academic practice. No use, distribution or reproduction is permitted which does not comply with these terms.
*Correspondence: God'spower Richard Okoh, godspower.okoh@my.jcu.edu.au