- 1Interdepartmental Center of Veterinary Radiology, University of Naples Federico II, Naples, Italy
- 2Institute of Biostructures and Bioimaging of the National Council of Research, Naples, Italy
- 3Department of Veterinary Medicine and Animal Production, University of Naples Federico II, Naples, Italy
- 4Department of Pharmacy, University of Salerno, Fisciano, Italy
- 5Department of Public Sanity, University of Naples Federico II, Naples, Italy
- 6Universidad Nacional Mayor San Marcos, Lima, Peru
Background: Tetrahydrocannabinol (THC) administration is associated with testicular damage and reduced semen quality. Oral administration of Lepidium Meyenii (maca) improves spermatogenesis and sperm motility and count and reduces spermatogenic damage.
Objectives: The aim of this study was to evaluate the effect of administration of THC, maca, and their combination on testicular tissue and semen parameters.
Materials and Methods: Thirty-six-week-old male mice were classified into control, THC, Maca, and THC + Maca groups. The mice were subjected to Eco Color Doppler ultrasound examination of the testicles before and after treatment. After euthanasia, the epididymis, testes, liver, and kidney were collected for histological examination. For morphometry of the testis, tubular diameters and seminiferous epithelium height were measured. Sperm concentration and sperm motilities were assessed. Differences among the groups were assessed using the Kruskal–Wallis and Dunn's post-hoc test.
Results: In all the groups, there were no significant changes in testicular morphology before and after treatment. Histological assessment of the testes showed no alterations in control, no significant alterations in Maca, mild to moderate alterations in THC, and mild alterations in THC + Maca groups. Histological examination of the other organs showed no significant differences among the groups. Tubular diameter showed significantly increased thickening for THC and THC + Maca compared with that for Maca and control. Moreover, seminiferous epithelium height decreased for THC compared with that in the control, Maca, and THC + Maca groups. No statistically significant reduction in the spermatogenic index was observed for THC compared with that for Maca and THC + Maca. Epididymal cross-sections of the groups showed no significant alterations. Sperm concentration and motility were higher for control and THC + Maca groups than in group THC and Maca.
Conclusion: In vivo maca administration reduced the deleterious effect of THC on testicular parenchyma and semen production.
Introduction
The medical properties of marijuana and cannabinoids have been widely recognized (1, 2). Cannabis-based medicines have proven useful in alleviating autoimmune disorders such as multiple sclerosis, rheumatoid arthritis, and other inflammatory diseases and also play an important role in the treatment of certain neurological diseases such as Alzheimer's disease and amyotrophic sclerosis lateral (2–5). More recent research has shown the ability of Cannabis-based medicine to reduce the spread of neoplastic cells (4). These cannabinoids have a high safety profile in relation to the risks of acute toxicity, but not in chronic use (4). Chronic toxic effects have been recognized in reproductive performance (6, 7), and it has been scientifically demonstrated that Cannabis sativa and Ruta Graveolens induce hypofertility (8). Currently, the illegal use of Cannabis sativa and cannabinoids is widespread and growing, especially in individuals of reproductive age, for recreational, social, medical, and spiritual reasons. Simultaneously, the problems of couple hypofertility is increasing, one-third of which are due to male factors (9). Numerous studies have shown the negative effect of daily marijuana intake on male fertility in both laboratory animals (10) and humans (7).
The direct effect of prolonged exposure to cannabinoids on reproductive organs in various animal species, which interferes with the normal anatomy, histology, and function of male reproductive organs, has been evaluated. Few studies have examined the physical (morphological and histological) effects of the use of exogenous cannabinoids on reproductive organs in humans. Although Kolodny et al. (11) concluded that the chronic use of marijuana in humans does not induce changes in testicular size and histological features of the testicles themselves, numerous studies have shown the opposite. Since endocannabinoid receptor endocannabinoid signaling system (ECSs) are involved in the regulation of the male reproductive system, numerous studies have been conducted to evaluate the effect of cannabinoids on various quality parameters of the semen (7–11). The correlation between cannabinoid exposure and sperm morphological alterations has been poorly studied (7); only one study has shown that this exposure represents a risk factor for the decay of the morphological characteristics of sperm (12).
Both in humans and animals, regular exposure to Cannabis induces reduction in sperm concentration (SC) in the ejaculate. Furthermore, the reduction in the number of spermatozoa per ejaculate is dose-dependent (7, 13, 14).
Finally, the literature clearly demonstrates the correlation between exposure to cannabinoids and motility and sperm vitality, both in vivo in men and animals and in vitro.
Therefore, exogenous cannabinoids, which disturb the physiological homeostasis of ECS receptors, induce harmful energy-dependent effects capable of affecting potential sperm fertility (7, 13, 14).
It is widely documented that oxidative stress plays an important role in the development of hypofertility. A recent study in rats showed that the administration of antioxidants such as melatonin and vitamin C together with the intake of cannabinoids reduces the spermiotoxic effect of the latter (15).
Among the various phytotherapeutics, a tuber, Lepidium meyenii, known in common parlance as maca, is recognized by the Andean people and used by the Inca people for its antioxidant power and ability to improve both male and female reproductive functions (16). The aphrodisiac effect of maca as well as its power to increase the reproductive capacity of those who consume it have been scientifically verified (7, 17). Maca has been consumed in Peru for 400 years, both as food and as a medicine. In fact, it has found use in the treatment of rheumatism, respiratory problems, and hormonal imbalances, in the stimulation of metabolism and memory, as a laxative, and finally for the treatment of depression, anemia, leukemia, aids, cancer, alcoholism, and reproduction (18, 19). Other studies have shown that maca can improve the quality of Stallone seed and its refrigerability (9, 20). In addition, maca counteracts the spermiotoxic effects induced by lead acetate in rats (21). The scientific recognition of its properties has led scientists and clinicians to officially include maca as a drug for the treatment of human male hypofertility (7). The aim of this study was to explore in vivo the effects of Δ-9-tetrahydrocannabinol (THC) in inducing morphological and histological changes in mouse testes, evaluate sperm motility and concentration, and explore the use of maca in mitigating or boosting the in vivo effect of cannabinoids in mice fertility.
Materials and Methods
Animal Procedures
The animal protocols used in this work were evaluated and approved by the Animal Use and Ethical Committee (OPBA) of CEINGE, Biotecnologie Avanzate s.c.a.r.l. (Naples, Italy) and by the Italian Ministery of Health [number of authorization 659 del 31.08.17, in accordance with FELASA guidelines and the guidelines defined by the European Communities Council Directive (2010/63/EU)]. Twenty-four C57BL/6 male mice at 6 weeks of age were purchased from Charles River Laboratories International, Inc. and were allowed to acclimate for 2 weeks before the experiments. Mice were divided into four groups: control group (six mice) without any treatment, the first group (nine mice) received 10 mg/kg di Δ9-THC in 0.1 ml of sesame oil subcutaneously for 30 days; the second group (10 mice) received 50 mg/kg maca via oral administration for 30 days, and the third group (5 mice) received 10 mg/kg di Δ9-THC subcutaneously and 50 mg/kg maca by oral administration.
High-Frequency Ultrasound
High-frequency ultrasound equipment (Vevo 2100, VisualSonics Inc., Toronto, Ontario, Canada) with a multifrequency (30–50 MHz) probe (MicroScan™ MS550D, VisualSonics Inc., Toronto, Ontario, Canada) was used in all procedures.
Mice were divided into three groups based on the established treatment and subjected to ultrasound examination of the testicles before and after treatment. Ultrasound examination was performed under general anesthesia with isoflurane in oxygen (induction phase: 5% isoflurane in 2 L/min oxygen; maintenance phase: 2% isoflurane in 2 L/min oxygen). All ultrasound examinations were performed before treatment and one day after treatment.
Each examined animal was placed in a dorsal decubitus position on the handling table of the Vevo imaging station (Vevo Integrated Rail System III; VisualSonics Inc., Toronto, Ontario, Canada), and vital signs (temperature, heart rate, and respiratory rate) were recorded using a dedicated monitoring system. Body temperature was maintained at 36 ± 5°C via an infrared lamp. After positioning, the animal was tricotomized in the pubic and abdominal regions. Each ultrasound session lasted ~45 min. For each testicle, the mediolateral, dorsoventral, and craniocaudal diameters were measured. The volume (mm3) of each testicle was calculated using the ellipsoid formula (width × depth × length × xπ/6). Thereafter, a 3D acquisition of mouse testes was performed: a set of consecutive 2D image planes of the testicles were acquired and then automatically reconstructed into 3D views.
Vascularization of tissues within the testicles was assessed using 2D and 3D color-Doppler (36.1 mm/s velocity, 25 dB Doppler gain), and a percent vascularity value (PV%) was provided after the volume had been created (mm3). The PV% provides the percentage of the volume that contains flow detected from the color Doppler image. All ultrasonographic assessments were performed by the same trained physician (S.A.), who was unaware of the results obtained in the previous evaluation and blinded to the mice group and pathological results.
Histopathology and Morphometry
After treatment and the last ultrasound examination, mice were euthanized with overdose of Isoflurane: Isoflurane (Iso-vet®, 1,000 mg/ml, EDRA S.p.A., Italy) were delivered via a custom fitted anesthetic machine (Vet-Equipe, Inc., Livermore, CA, USA) that allowed the direct introduction of the gas into the anesthetic chamber. Afterwards mice were subjected to cervical dislocation according to the European rules about animal experimentation.
The testes, liver, kidney, and colon were harvested and preserved in 10% neutral buffered formalin (code no. 05-01007Q, Bio-Optica, Milan, Italy), dehydrated, and embedded in paraffin (code no. 06-7920, Bio-Optica, Milan, Italy). Paraffin blocks were cut into 4-μm-thick sections and stained with hematoxylin and eosin for analysis of morphology.
For the liver, kidney, and colon histologic assessment, several parameters were semiquantitatively evaluated separately by two independent, experienced pathologists (O.P. and D.DB.) in a blinded fashion, with good concordance (Cohen's κ = 0.913, P < 0.001).
For the liver histological examination, three main broad categories of histological features were analyzed: steatosis, inflammation, and necrosis. The grading system was adapted from Kleiner et al. (22), as previously described (23). Kleiner's grading system considers the following histological variables: severity of steatosis (quantified by the evaluation of parenchymal involvement by steatosis): score 0, <5%; score 1, 5–33%; score 2, >33–66%, score 3, >66%; location (predominant distribution pattern): zone 3, score 0; zone 1, score 1; azonal, score 2; inflammation: lobular inflammation (overall assessment of all inflammatory foci): score 0, no foci; score 1, <2 foci per ×200 magnification field; score 2, 2–4 foci per ×200 magnification field; score 3, >4 foci per ×200 magnification field; necrosis: score 0, present; score 1, absent.
For the kidney, the examined histologic features were: (1) epithelial degeneration, (2) glomerular atrophy, (3) vascular changes, (4) stromal fibrosis, and (5) tubular atrophy. When present, the damage was evaluated semiquantitatively as 0: none, 1: mild, 2: moderate, or 3: severe (24).
For the colon, the histologic scoring system was adapted from Coretti et al. (25) as follows: (a) the severity of inflammatory cell infiltration was evaluated based on the percentage of leukocyte density in the lamina propria area and estimated in a high-power field representative of the section (0 for no signs of inflammation, 1 for minimal <10%, 2 for mild 10–25% with scattered neutrophils, 3 for moderate 26–50%, 4 for marked >51% with dense infiltrate); (b) The extent of the inflammation was estimated as expansion of leukocyte infiltration (0 for none, 1 for mucosal, 2 for mucosal and submucosal, and 3 for mucosal, submucosal, and transmural levels).
Morphometry of the testis was carried out as previously described by other authors (26, 27), with modifications. Micrographs of experimental and control animals were acquired under a light microscope (Nikon Eclipse E600) attached to a microphotography system (Nikon digital camera DMX1200). For morphometric analysis, setting scale and conversion of values from pixels to micrometers were obtained from a picture with known distance in micrometer. Transverse sections of testes with at least 20 round or nearly round seminiferous tubules were chosen randomly to measure tubular diameters and seminiferous epithelium height for each animal regardless of the stage of the seminiferous epithelium cycle (26) using images obtained at ×100 magnification. The diameter (D) of the seminiferous tubules was measured across the minor and major axes of the tubules by calculating the average of two diameters, D1 and D2. The same tissue section used for measuring tubular diameters was used to measure the seminiferous epithelium height. For this analysis, two perpendicular lines in each field were drawn from the basement membrane (tunica propria) to the tubule lumen (luminal border). The mean of these two values was considered as the height of the seminiferous tubule.
For tubular spermatogenesis index evaluation and quantification, we applied a ten-point scoring system formulated by Johnsen (28) and used both in human and experimental pathology because of its good reproducibility (29). The Johnsen criteria were established according to the profile of the cells encountered along the seminiferous tubules, ranging from no cells to complete spermatogenesis.
Semen Collection and Evaluation
Immediately after euthanasia, the cauda epididymis and the vasa deferentia were excised. The tissues were incised and placed into a 2-ml Eppendorf with 500 μL of pre-warmed Dulbecco's phosphate-buffered saline solution (Sigma-Aldrich, Milan, Italy). Spermatozoa were allowed to swim up into the medium for at least 30 min at 35°C.
SC was determined using a Bürker chamber at phase contrast (400 × magnification), and the results are presented in sperm cells/mL. Sperm motility was evaluated by placing 10 μL of pre-warmed (37°C) semen suspension between a pre-warmed slide and a coverslip. The slides were examined for total motility (%), as well as rapid and slow progressive motile sperm (%) by a blinded investigator using a phase contrast microscope (Leitz Laborlux K Microscope, Leitz, Italy) at 100 × magnification and heating stage (37°C). For each sample, 10 different randomly selected fields were evaluated.
Numerical variables are reported as medians with interquartile ranges (25th, 75 percentile). Differences among groups were assessed using the Kruskal–Wallis test, followed by Dunn's post-hoc test. Statistical significance was set at p < 0.05. All analyses were conducted using the statistical platform R (ver. 4.0.1).
Results
B-mode acquisition in the transverse and longitudinal planes, followed by a motor 3D-B-mode and -Color Doppler Mode reconstruction of both testicles was performed in all 24 mice before and after treatment (Figure 1). Mice imaged before treatment were considered as controls.
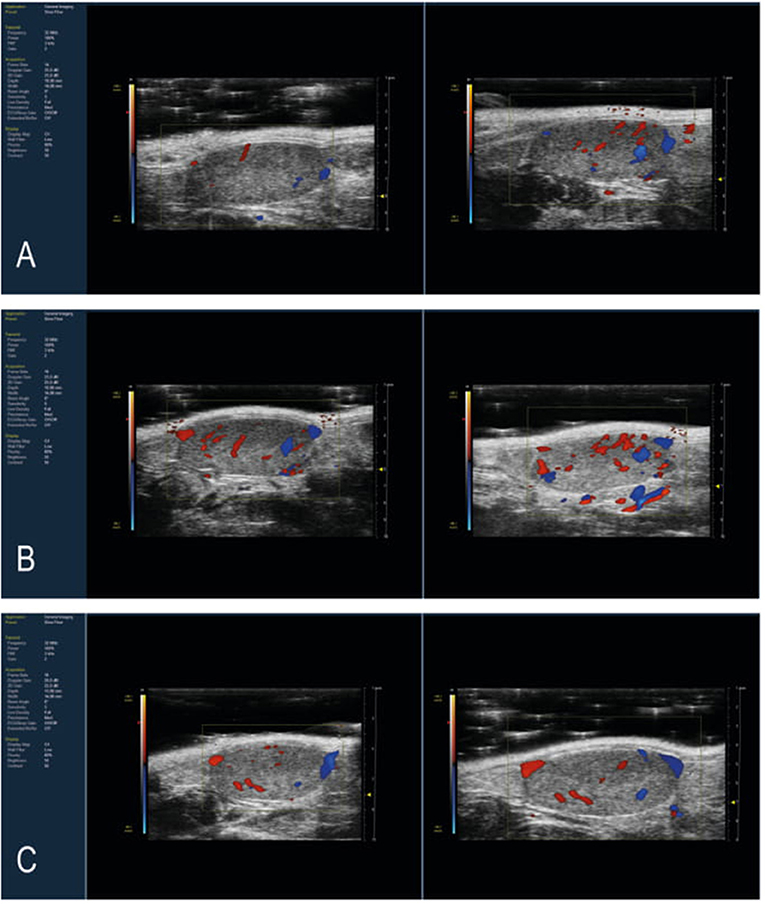
Figure 1. Representative Longitudinal Scan of mouse testis with color Doppler HFUS image. Images of pre-treatment and post-treatment mice testis with (A) 10 mg/kg THC, (B) 50 mg/Kg maca, and (C) 10 mg/kg Δ9-THC and 50 mg/Kg maca. After 30 days of treatment, the THC group showed more intense vascularization than the baseline. In contrast, the Maca and Maca + THC groups showed less differences in pre- and post-treatment vascularization.
In all the groups, there were no significant changes in testicular morphology before and after treatment, nor in the echogenic structures evaluated. The testicles had a testicular volume ranging from 58.157 to 84.205 mm3 pre-treatment and a volume of 54.959 to 85.883 mm3 post-treatment. No significant differences were evident among the groups and between all mice pre- and post-treatment.
Similar to the tridimensional analysis, we reported the percentage of vascularization (PV%) in all the groups pre- and post-treatment (Figure 2). No significant differences were found among pre-treatment groups (Figure 2). After treatment, the THC group showed a significantly higher PV% than the Maca (p = 0.032) and Maca + THC group (p = 0.004; Figure 2).
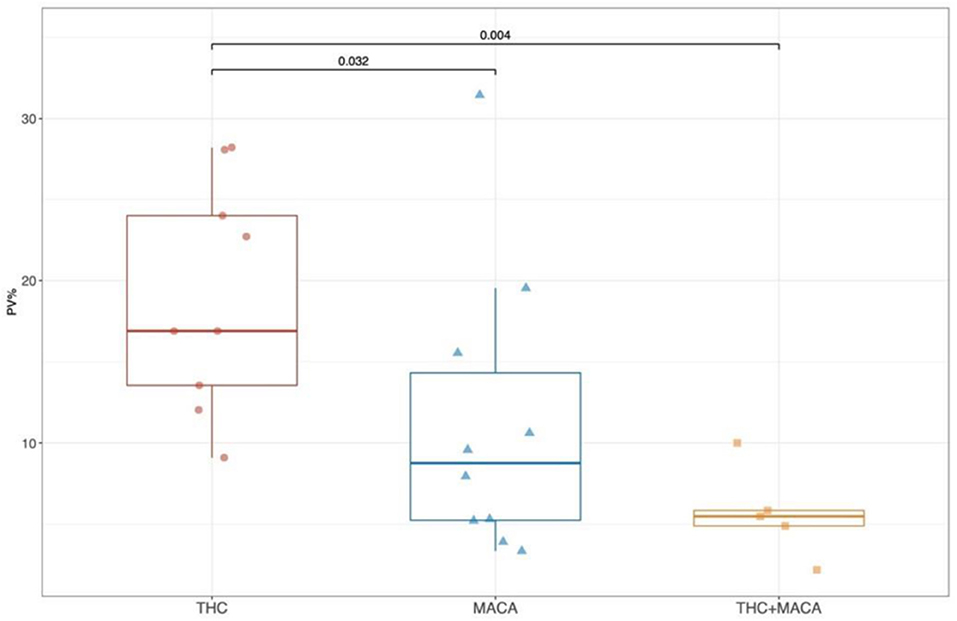
Figure 2. Percentage of vascularization from color Doppler images. Boxplot showing the distribution of post-treatment PV (%) stratified by experimental groups. Boxes represent the 25th to 75th percentile, the line represents the median and the whisker represents the min and max aside outliers that are reported outside the whiskers.
Histopathology and Morphometry
Morphological analysis was performed for the 24 treated and 4 untreated C57BL/6 mice. Histological examination of formalin-fixed and paraffin-embedded sections of the liver, kidney, and cecum showed no evident histopathological changes for the selected parameters and no statistically significant difference among mice groups.
Histological assessment of testes from mice of the control group showed no alterations with a normal histoarchitecture that consisted of uniform, well-organized seminiferous tubules with complete spermatogenesis and normal interstitial connective tissue. Seminiferous tubules had an intact epithelium with a full complement of spermatogenic cells. Mature spermatozoa filled with tubule lumens and interstitial tissue had a normal distribution of Leydig cells.
In the control group and experimental group 3 (THC + Maca), no severe and significant alterations were observed in testicular parenchyma or spermatogenesis. In experimental group 1 (THC), transverse sections of the testis showed mild to moderate pathologic modifications accounting for almost 45% of the testicular parenchyma. Pathologic findings consisted mostly of multifocal detachment of the germinal epithelium, irregular and buckled basement membrane, tubular deformation and degeneration, several shrunken seminiferous tubules, and multifocally increased luminal diameter. In experimental group 2 (Maca), transverse sections of the testis showed an overall normal histoarchitecture of the testicular parenchyma with scattered seminiferous tubules lined by intact epithelium and normal spermatogenesis. A small number of seminiferous tubules, accounting for ~25% of the testicular parenchyma, showed mild alterations such as detachment of the germinal epithelium and a reduced population of mature spermatozoa. Representative pictures of testicular morphology in the control and experimental groups are shown in Figures 3A–D.
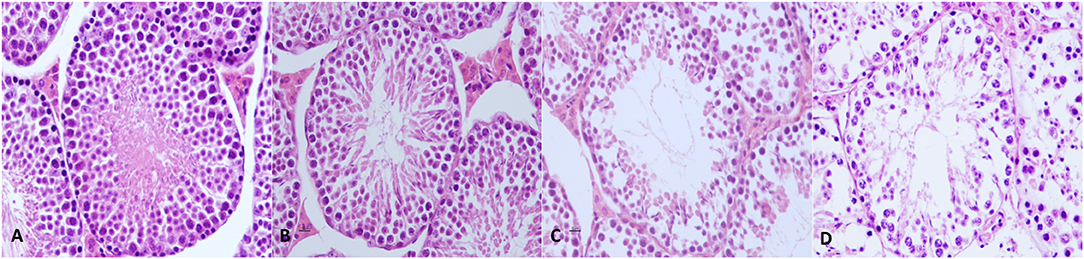
Figure 3. Mouse testis from control and experimental groups. (A) Testes from mice of the control group showed normal histoarchitecture with uniform, well-organized seminiferous tubules, and complete spermatogenesis. (B) In experimental group 1, no severe and significant alterations were observed in testicular parenchyma nor in spermatogenesis. (C) In experimental group 2, transverse sections of the testis showed scattered mild to moderate alterations, which were present mostly in the multifocal detachment of germinal epithelium, irregular and buckled basement membrane, tubular deformation and degeneration, shrunken seminiferous tubules, and increased luminal diameter. (D) In experimental group 3, mild alterations such as the detachment of germinal epithelium and reduced population of mature spermatozoa are shown. Hematoxylin and eosin, original magnification 40×.
Morphometric results are summarized in Table 1. Morphometric measurements showed that tubular diameter significantly decreased in experimental groups 1 and 2 compared with control group and experimental group 3 (p < 0.05). Moreover, seminiferous epithelium height decreased significantly in experimental group 1 compared with control group and experimental groups 2 and 3 (p < 0.01). The spermatogenic index had a level of 10 (complete spermatogenesis with many spermatozoa) in the control group and experimental group 3, but shifted from 10 to 9 (many spermatozoa present, but germinal epithelium disorganized with marked sloughing or obliteration of lumen) in experimental groups 1 and 2. Therefore, a slight but not statistically significant reduction in the spermatogenic index was observed in experimental group 1 (p < 0.001) compared with experimental groups 2 and 3.

Table 1. Morphometry of the testis of control and experimental group 1 (THC), group 2 (Maca), and group 3 (THC + Maca).
Epididymal cross-sections of control groups, as well as experimental groups 1, 2, and 3, showed no significant alterations. The epididymal lumen was filled with spermatozoa, and the epithelium showed an intact basement membrane, epididymal tubules, pseudostratified columnar epithelium, and interstitial areas.
Semen Parameters
Semen evaluation was performed on the 24 treated mice and in 6 untreated c57/BL/6 mice used as controls. Significant differences in all semen-related variables were found among the groups (Figure 4). The THC group showed a significantly lower semen concentration (23 [20; 26.5] × 106 spz/ml) than the Maca group (36.5 [31.5; 43.2] × 106 spz/ml; p = 0.015), THC + Maca (52 [46.5; 62.5] × 106 spz/ml; p < 0.01) and control groups (53 [43.5; 56.2] × 106 spz/ml; p < 0.001). Maca administration resulted in lower semen concentrations in the THC + Maca group (p = 0.032).
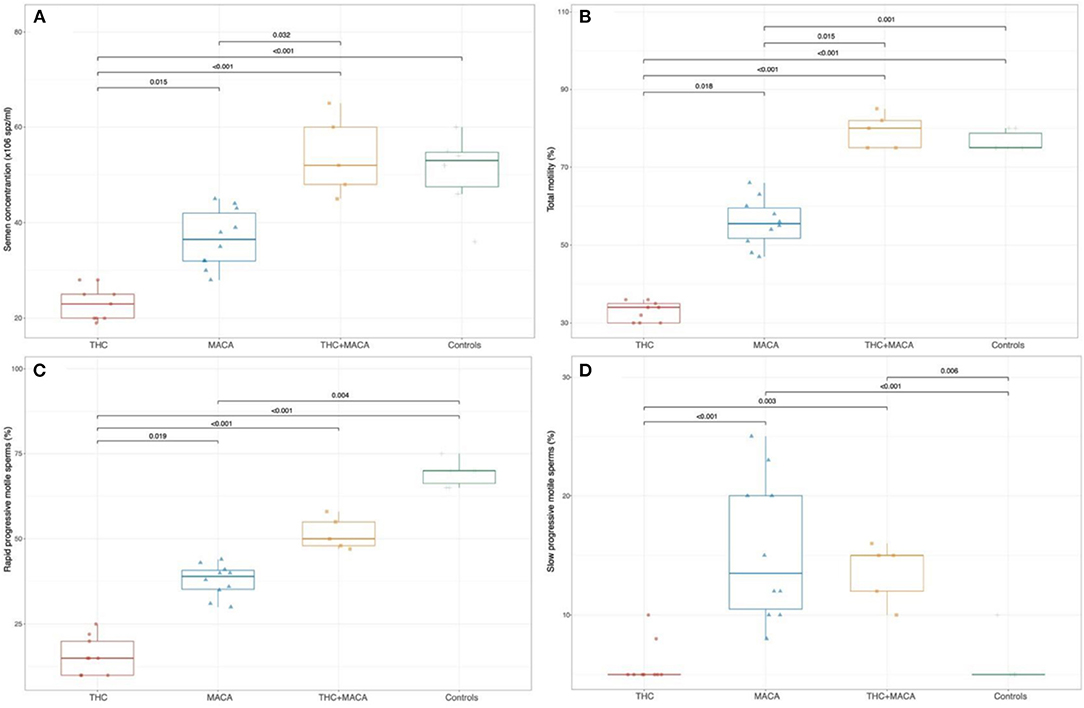
Figure 4. Semen parameters in control and experimental groups. Boxplot showing the distribution of semen parameters stratified by experimental groups. Semen concentration (A), total motility (B), the percentage of rapid and slow progressive motile sperm (C,D). Boxes represent the 25th−75th percentile, the line represents the median and the whisker represents the min and max aside outliers that are reported outside the whiskers.
Total motility was significantly reduced in the THC group [34 (30; 35.5)] and Maca group [55.5 (50.2; 60.8)] compared with that in the control group (75 [75; 80]; p < 0.001 and p = 0.036, respectively). The THC group also showed a significantly reduced total motility compared to the Maca group (p = 0.018) and THC + Maca group (80 [75; 83.5]; p < 0.001).
The percentage of rapid progressive motile sperms was significantly reduced in the THC group (15 (10, 23)) and Maca group (39 [34; 41.5]) than in the control group (70 [65; 71.2]; p < 0.001 and p = 0.004, respectively). The THC group showed a significantly reduced percentage of rapid progressive motile sperm with respect to the Maca (p = 0.019) and THC + Maca group (50 [47.5; 56.5]; p < 0.001).
With respect to slow progressive motile sperms (%), both the control (5 [5; 6.2]) and THC groups (5 [5; 6.5]) showed significantly reduced percentages when compared with the Maca (13.5 [10; 20.8]; p < 0.001 for both) and THC + Maca (15 [11; 15.5]) groups (p = 0.006 and 0.003, respectively).
Discussion
Studies on human reproduction are challenging, given ethical considerations, and the results on the impact of marijuana are confounded by socioeconomic factors and drug variability (9). Strong efforts have been made during the years to elucidate the effect of marijuana on reproduction in human and animal models (9, 30). One of the purposes of this study was to verify the effect of THC administration in vivo on the characteristics of epidydimal mouse sperm cells.
Furthermore, studies in humans have suggested that dietary supplementation with antioxidants reduces seminal oxidative stress and improves semen quality, particularly in subfertile males (9, 31–33). Many studies have focused on the use of natural antioxidants from terrestrial plants to prevent sperm damage caused by reactive oxygen species (ROS) (34–36). Maca is a traditional Andean crop used as a nutraceutical for the fertility-enhancing properties associated with its antioxidant activity (37, 38). Moreover, horses with maca dietary supplementation showed an improvement in semen quality during cooling by protecting testicular cell membranes and mitochondria from oxidative stress (9). To date, the in vivo effects of THC combined with maca have not been explored. Ultrasound examination is the imaging technique of choice to explore in vivo morphology and vascularization of soft tissue (39), including the testis, in experimental animal models of disease (7, 40).
In our study, in vivo ultrasound examination showed the absence of grossly morphologic alterations in mice treated with THC, maca, and the combination of the two treatments. However, we found a significant improvement in the percentage of vascularization in mice treated with THC. This could be attributed to the vascular congestion in the seminiferous tubules of testes, also reported by others (41). We speculate that the administration of THC in animal models leads to an early stage of vascular congestion and subsequently, to vascular damage, especially for a prolonged administration of up to 6 months. However, the increase in the percentage of vascularization by itself can induce hyperthermia and consecutively hypofertility. The PV % decreases when mice are treated with maca, and this is even more evident when mice are treated with THC and maca. Maca is confirmed to have a beneficial effect related to the decrease in oxidative stress, which could explain the improvement of the fertility of mice treated with both maca and THC. Finally, maca used alone did not demonstrate the same effect in improving vascularization in vivo (42).
Histological evaluations was performed in order to identify possible alterations subsequent to the oral administration of THC and/or maca. The analysis of the selected organs other than testis did not reveal any abnormalities. The effect of THC and Maca on spermatogenesis were evaluated by morphometric parameters and morphological evaluation of testis histology using tubular spermatogenesis index, already reported in literature (26, 27). Those analysis revealed very mild to moderate alterations in parenchymal cytoarchitecture and spermatogenesis in experimental groups compared to the controls. The administration of THC affected the spermatogenesis mostly at the stage of spermiation, showing detachment of the germinal epithelium, exfoliation of spermatocytes, multifocally increased luminal diameter, and a slight reduction in spermatogenesis. However, maca administration seems to reverse the effect of THC on spermatogenesis. Similar results were found after administration of lead acetate plus maca (21).
In line with the morphometric evaluation of seminiferous tubules, the in vitro semen evaluation showed a drastic reduction in semen concentration and a loss of sperm motility, confirming the negative effect of THC on male fertility. Despite a large number of recent studies, the results of whether THC affects the ability of sperm to fertilize and generate embryos remains unclear, and the effects of cannabinoids are controversial. The association between the chronic use of THC and abnormalities in sperm count, concentration, motility, and morphology, as well as structural changes in the testis in humans, has been widely reported and reviewed in the literature (9, 43, 44). However, a recent study conducted in male mice showed opposite results, with no negative effect of THC on the male reproduction process (45). Furthermore, the reduction in motility and ATP in sperm treated with THC was dose-dependent (45–48). The mechanism by which THC induces sperm damage is still under investigation. THC activates cannabinoid receptors, which are part of the endogenous endocannabinoid system. This system is a relatively novel system located in the hypothalamus, pituitary, and gonads in both sexes and is involved in spermatogenesis and sperm function (44, 49). The negative effects of THC on testicular morphology and spermatogenesis may depend on the modulation of cannabinoid receptors that are present on Sertoli and Leydig cells and that modulate the balance of molecular signaling and nurturing the microenvironment (50). Modulation of cannabinoid receptors such as CB1 (on Leydig cells) and CB2 (on Sertoli cells) have been suggested to induce local reduction of testosterone production and apoptosis of Sertoli cells, respectively, hence affecting sperm development (51, 52). In the last few years, a growing amount of data has underlined the potential role of oxidative stress in the mechanism of action of THC (53, 54). The risk of stroke in young Cannabis users has recently been correlated with the generation of reactive ROS, leading to oxidative stress (53). Moreover, a recent study conducted in vivo on rats showed that THC induced cerebral mitochondrial dysfunction and increased hydrogen peroxide production (54). Since oxidative stress is involved in male infertility, different studies have examined the role of this stress in Cannabis-associated sperm alterations (55, 56). These studies confirmed the implication of oxidative stress in Cannabis-induced spermatotoxicity (55, 56). In our study, oral administration of maca (group 2) and the combination of THC and maca (group 3) interestingly showed little to no pathologic effect on testis and spermatogenesis. However, these data were not completely corroborated by the in vitro evaluation of semen, which revealed a harmful effect of maca on SC and sperm motility. Indeed, other investigators have observed a beneficial effect of maca administration on spermatogenesis in mice, improving sperm count and motility (7, 9, 16, 57). Meanwhile, the use of only maca reduced sperm motility and concentration, and the supplementation of mice receiving THC with maca improved sperm characteristics.
Based on results, authors can hypothesize different mechanisms used by Maca to reverse the deleterious effect of THC. Since mice treated with THC plus Maca have similar histological results to control group, maca could protect the testis from spermatogenic disruption caused by THC preventing apoptosis of the developing germ cells and improving the number of cells progress through the spermatogenesis. Previous study suggests that Maca reversed deleterious effects due lead acetate on spermatogenesis by protecting onset of mitosis and spermiation (21).
Positive effect of Maca is correlated with its antioxidant effect that reduces THC-associated sperm damage caused by oxidative stress. Similar ameliorative effects in Cannabis sativa-associated spermiotoxicity were reported with the use of other antioxidants, such as a combination of melatonin and vitamin C (15, 55). The negative effect of the administration of maca on semen can be due to an alteration of the endogenous antioxidant systems by this antioxidant. Oxidative stress caused by ROS is physiologically balanced by endogenous antioxidant systems. The authors' hypothesis is that in these mice, under physiological conditions, antioxidant supplementation is not necessary because the balance between pro-oxidants and antioxidants is already in place for the natural evolution of sperm physiology. The addition of antioxidants in the diet or in the semen-targeted improvement of semen production and quality should take into account the endogenous production of antioxidants, which varies greatly between individuals (9, 58, 59). There is a limitation of the study and potential bias caused by the subjective evaluation of sperm motility.
In conclusion, this study confirmed that the oral administration of maca prevents the harmful effect of THC on mouse spermatogenesis and spermatozoa features, and it lends further credibility to the hypothesis that Maca could be an alternative treatment for male infertility. In order to evaluate the biological activity of maca during oral supplementation, successive studies should be carried out on redox status measurements and reproductive hormonal modifications in treated mice.
Data Availability Statement
The original contributions presented in the study are included in the article/supplementary material, further inquiries can be directed to the corresponding author/s.
Ethics Statement
The animal study was reviewed and approved by Animal Use and Ethical Committee (OPBA) of CEINGE, Biotecnologie Avanzate s.c.a.r.l. (Na-ples, Italy) and by the Italian Ministry of Health [number of authorization 659 del 31.08.17, in accordance with FELASA guidelines and the guidelines defined by the European Communities Council Directive (2010/63/EU)].
Author Contributions
All authors listed have made a substantial, direct, and intellectual contribution to the work and approved it for publication.
Funding
This study was supported by a grant from the Department of Veterinary Medicine and Animal Productions University of Naples Federico II, Italy, in partnership with the Interdepartmental Center of Veterinary Radiology, University of Naples Federico II, and CEINGE, Advanced Biotechnology, University of Naples Federico II.
Conflict of Interest
The authors declare that the research was conducted in the absence of any commercial or financial relationships that could be construed as a potential conflict of interest.
Publisher's Note
All claims expressed in this article are solely those of the authors and do not necessarily represent those of their affiliated organizations, or those of the publisher, the editors and the reviewers. Any product that may be evaluated in this article, or claim that may be made by its manufacturer, is not guaranteed or endorsed by the publisher.
Acknowledgments
We thank the Euro-BioImaging facility at the Institute of Biostructures and Bioimaging (CNR), Naples for help with Imaging experiments.
References
1. Birdsall SM, Birdsall TC, Tims LA. The use of medical marijuana in cancer. Curr Oncol Rep. (2016) 18:40. doi: 10.1007/s11912-016-0530-0
2. Hill KP. Medical marijuana for treatment of chronic pain and other medical and psychiatric problems: a clinical review. JAMA. (2015) 313:2474–83. doi: 10.1001/jama.2015.6199
3. Solimini R, Rotolo MC, Pichini S, Pacifici R. Neurological disorders in medical use of Cannabis: an update. CNS Neurol Disord Drug Targets. (2017) 16:527–33. doi: 10.2174/1871527316666170413105421
4. Tkaczyk M, Florek E, Piekoszewski W. Marihuana and cannabinoids as medicaments. Przegladlekarski. (2011) 69:1095–7.
5. Whiting PF, Wolff RF, Deshpande S, Di Nisio M, Duffy S, Hernandez AV, et al. Cannabinoids for medical use: a systematic review and meta-analysis. JAMA. (2015) 313:2456–73. doi: 10.1001/jama.2015.6358
6. Brents LK. Marijuana, the endocannabinoid system and the female reproductive system. Yale J Biol Med. (2016) 89:175–91.
7. Gonzales GF, Gonzales-Castañeda C, Gasco M. A mixture of extracts from Peruvian plants (black maca and yacon) improves sperm count and reduced glycemia in mice with streptozotocin-induced diabetes. Toxicol Mech Methods. (2013) 23:509–18. doi: 10.3109/15376516.2013.785656
8. Sailani MR, Moeini H. Effect of Ruta graveolens and Cannabis sativa alcoholic extract on spermatogenesis in the adult wistar male rats. Indian J Urol. (2007) 23:257–60. doi: 10.4103/0970-1591.33720
9. Fronczak CM, Kim ED, Barqawi AB. The insults of illicit drug use on male fertility. J Androl. (2012) 33:515–28. doi: 10.2164/jandrol.110.011874
10. Park B, McPartland JM, Glass M. Cannabis, cannabinoids and reproduction. Prostaglandins Leukot Essent Fatty Acids. (2004) 70:189–97. doi: 10.1016/j.plefa.2003.04.007
11. Kolodny RC Masters WH Kolodner RM Toro G Depression Depression of plasma testosterone levels after chronic intensive marihuana use. N Engl J Med. (1974) 290:872–4. doi: 10.1056/NEJM197404182901602
12. Amoako AA, Marczylo TH, Marczylo EL, Elson J, Willets JM, Taylor AH, et al. Anandamide modulates human sperm motility: implications for men with asthenozoospermia and oligoasthenoteratozoospermia. Hum Reprod. (2013) 28:2058–66. doi: 10.1093/humrep/det232
13. Hsiao P, Clavijo RI. Adverse effects of cannabis on male reproduction. Eur Urol Focus. (2018) 4:324–8. doi: 10.1016/j.euf.2018.08.006
14. Bari M, Battista N, Pirazzi V, Maccarrone M. The manifold actions of endocannabinoids on female and male reproductive events. Front Biosci (Landmark Ed). (2011) 16:498–516. doi: 10.2741/3701
15. Alagbonsi IA, Olayaki LA, Salman TM. Melatonin and vitamin C exacerbate Cannabis sativa-induced testicular damage when administered separately but ameliorate it when combined in rats. J Basic Clin Physiol Pharmacol. (2016) 27:277–87. doi: 10.1515/jbcpp-2015-0061
16. Gonzales GF, Cordova A, Vega K, Chung A, Villena A, Gonez C. Effect of Lepidium meyenii (Maca) a root with aphrodisiac and fertility-enhancing properties, on serum reproductive hormone levels in adult healthy men. J Endocrinol. (2003) 176:163–8. doi: 10.1677/joe.0.1760163
17. Valentová K, Frcek J, Ulrichová J. Yacon (Small anthussonchifolius) and MACA (Lepidium meyenii), traditional Andean crops as new functional foods on the European market. Feedback. (1997) 91.
19. Wang Y, Wang Y, McNeil B, Harvey LM. Maca: an Andean crop with multi-pharmacological functions. Food Res Int. (2007) 40:783–92. doi: 10.1016/j.foodres.2007.02.005
20. Del Prete C, Tafuri S, Ciani F, Pasolini MP, Ciotola F, Albarella S, et al. Influences of dietary supplementation with Lepidium meyenii (Maca) on stallion sperm production and on preservation of sperm quality during storage at 5°C. Andrology. (2018) 6:351–61. doi: 10.1111/andr.12463
21. Rubio J, Riqueros MI, Gasco M, Yucra S, Miranda S, Gonzales GF. Lepidium meyenii (Maca) reversed the lead acetate induced-damage on reproductive function in male rats. Food Chem Toxicol. (2006) 44:1114–22. doi: 10.1016/j.fct.2006.01.007
22. Kleiner DE, Brunt EM, Van Natta M, Behling C, Contos MJ, Cummings OW, et al. Design and validation of a histological scoring system for nonalcoholic fatty liver disease. Hepatology. (2005) 41:1313–21. doi: 10.1002/hep.20701
23. Annunziata C, Lama A, Pirozzi C, Cavaliere G, Trinchese G, Di Guida F, et al. Palmitoylethanolamide counteracts hepatic metabolic inflexibility modulating mitochondrial function and efficiency in diet-induced obese mice. FASEB J. (2020) 34:350–64. doi: 10.1096/fj.201901510RR
24. Aktoz T, Durmus-Altun G, Usta U, Torun N, Ergulen A, Atakan IH. Radioiodine-induced kidney damage and protective effect of amifostine: an experimental study. Hippokratia. (2012) 16:40–5.
25. Coretti L, Cristiano C, Florio E, Scala G, Lama A, Keller S. Sex-related alterations of gut microbiota composition in the BTBR mouse model of autism spectrum disorder. Sci Rep. (2017) 7:45356. doi: 10.1038/srep45356
26. Mehraein F, Negahdar F. Morphometric evaluation of seminiferous tubules in aged mice testes after melatonin administration. Cell J (Yakhteh). (2011) 13:1.
27. Abarikwu SO, Wokoma AF, Mgbudom-Okah CJ, Omeodu SI, Ohanador R. Effect of Fe and Cd co-exposure on testicular steroid metabolism, morphometry, and spermatogenesis in mice. Biol Trace Elem Res. (2019) 190:109–23. doi: 10.1007/s12011-018-1536-2
28. Johnsen SG. Testicular biopsy score count-a method for registration of spermatogenesis in human testes: normal values and results in 335 hypogonadal males. Horm Res Paediatr. (1970) 1:2–25. doi: 10.1159/000178170
29. Teixeira TA, Pariz JR, Dutra RT, Saldiva PH, Costa E, Hallak J. Cut-off values of the Johnsen score and Copenhagen index as histopathological prognostic factors for postoperative semen quality in selected infertile patients undergoing microsurgical correction of bilateral subclinical varicocele. Transl Androl Urol. (2019) 8:346. doi: 10.21037/tau.2019.06.23
30. D'Angelo D, Ciani F, Zaccherini A, Tafuri S, Avallone L, D'Ingeo S, et al. Human-animal relationship dysfunction: a case study of animal hoarding in Italy. Animals. (2020) 10:1501. doi: 10.3390/ani10091501
31. Esposito L, Auletta L, Ciani F, Pelagalli A, Pasolini MP, Lamagna B, et al. Hair cortisol levels in captive brown hare (Lepus europaeus): potential effect of sex, age, and breeding technology. Eur J Wildl Res. (2017) 63:62. doi: 10.1007/s10344-017-1121-6
32. Ross C, Morriss A, Khairy M, Khalaf Y, Braude P, Coomarasamy A, et al. A systematic review of the effect of oral antioxidants on male infertility. Reprod Biomed Online. (2010) 20:711–23. doi: 10.1016/j.rbmo.2010.03.008
33. Wong WY, Thomas CM, Merkus JM, Zielhuis GA, Steegers-Theunissen RP. Male factor subfertility: possible causes and the impact of nutritional factors. Fertil Steril. (2000) 73:435–42. doi: 10.1016/S0015-0282(99)00551-8
34. Patil RB, Vora SR, Pillai MM. Antioxidant effect of plant extracts on phospholipids levels in oxidatively stressed male reproductive organs in mice. Int J Reprod Biomed. (2009) 7:35.
35. Tempest HG, Homa ST, Routledge EJ, Garner A, Zhai XP, Griffin DK. Plants used in Chinese medicine for the treatment of male infertility possess antioxidant and anti-oestrogenic activity. Syst Biol Reprod Med. (2008) 54:185–95. doi: 10.1080/19396360802379073
36. Zhong RZ, Zhou DW. Oxidative stress and role of natural plant derived antioxidants in animal reproduction. J Integr Agric. (2013) 12:1826–38. doi: 10.1016/S2095-3119(13)60412-8
37. Tafuri S, Cocchia N, Carotenuto D, Vassetti A, Staropoli A, Mastellone V, et al. Chemical analysis of Lepidium meyenii (Maca) and its effects on redox status and on reproductive biology in stallions. Molecules. (2019) 24:1981. doi: 10.3390/molecules24101981
38. Tafuri S, Cocchia N, Vassetti A, Carotenuto D, Esposito L, Maruccio L, et al. Lepidium meyenii (Maca) in male reproduction. Nat Prod Res. (2019) 35:4550–9. doi: 10.1080/14786419.2019.1698572
39. Greco A, Mancini M, Gargiulo S, Gramanzini M, Claudio PP, Brunetti A, et al. Ultrasound biomicroscopy in small animal research: applications in molecular and preclinical imaging. J Biomed Biotechnol. (2012) 2012:519238. doi: 10.1155/2012/519238
40. Celebi M, Paul AG. Assessment of ischaemia-reperfusion injury in the mice testis by using contrast ultrasound molecular imaging. Andrologia. (2016) 48:907–13. doi: 10.1111/and.12531
41. Nosarieme OA. Cannabis sativa (Marijuana) alters blood chemistry and the cytoarchitecture of some organs in Sprague Dawley rat models. Food Chem Toxicol. (2018) 116:292–7. doi: 10.1016/j.fct.2018.04.023
42. Solinas M, Massi P, Cantelmo AR, Cattaneo MG, Cammarota R, Bartolini D, et al. Cannabidiol inhibits angiogenesis by multiple mechanisms. Br J Pharmacol. (2012) 167:1218–31. doi: 10.1111/j.1476-5381.2012.02050.x
43. Carroll K, Pottinger AM, Wynter S, DaCosta V. Marijuana use and its influence on sperm morphology and motility: identified risk for fertility among Jamaican men. Andrologia. (2020) 8:136–42. doi: 10.1111/andr.12670
44. Gundersen TD, Jorgensen N, Andersson AM, Bang AK, Nordkap L, Skakkebæk NE, et al. Association between use of marijuana and male reproductive hormones and semen quality: a study among 1,215 healthy young men. J. Epidemiol. (2015) 182:473–81. doi: 10.1093/aje/kwv135
45. López-Cardona AP, Ibarra-Lecue I, Laguna-Barraza R, Pérez-Cerezales S, Urigüen L, Agirregoitia N, et al. Effect of chronic THC administration in the reproductive organs of male mice, spermatozoa and in vitro fertilization. Biochem Pharmacol. (2018) 157:294–303. doi: 10.1016/j.bcp.2018.07.045
46. Aktoz T, Durmus-Altun G, Usta U, Torun N, Ergulen A, Atakan IH. Radioiodine-induced kidney damage and protective effect of amifostine: an experimental study. Hippokratia. (2012) 16:40–5.
47. Badawy ZS. Cannabinoids inhibit the respiration of human sperm. Fertil Steril. (2009) 91:2471–6. doi: 10.1016/j.fertnstert.2008.03.075
48. Morgan DJ, Muller CH, Murataeva NA, Davis BJ, Mackie K. Δ9-Tetrahydrocannabinol (Δ9-THC) attenuates mouse sperm motility and male fecundity. Br J Pharmacol. (2012) 165:2575–83. doi: 10.1111/j.1476-5381.2011.01506.x
49. Karasu T, Marczylo TH, Maccarrone M, Konje IC. The role of sex steroid hormones, cytokines and the endocannabinoid system in female fertility. Hum Reprod Update. (2011) 17:347–61. doi: 10.1093/humupd/dmq058
50. Rajanahally S, Raheem O, Rogers M. The relationship between cannabis and male infertility, sexual health, and neoplasm: a systematic review. Andrology. (2019) 7:139–47. doi: 10.1111/andr.12585
51. Maccarrone M, Finazzi-Agro A. The endocannabinoid system, anandamide and the regulation of mammalian cell apoptosis. Cell Death Differ. (2003) 10:946–55. doi: 10.1038/sj.cdd.4401284
52. Gye MC, Kang HH, Kang HJ. Expression of cannabinoid receptor 1 in mouse testes. Arch Androl. (2005) 51:247–55. doi: 10.1080/014850190898845
53. Archie SR, Cucullo L. Harmful effects of smoking cannabis: a cerebrovascular and neurological perspective. Front Pharmacol. (2019) 10:1481. doi: 10.3389/fphar.2019.01481
54. Wolff V, Schlagowski AI, Rouyer O, Charles A-L, Singh F, Auger C, et al. Tetrahydrocannabinol induces brain mitochondrial respiratory chain dysfunction and increases oxidative stress: a potential mechanism involved in cannabis-related stroke. Biomed Res Int. (2015) 2015:323706. doi: 10.1155/2015/323706
55. Alagbonsi IA, Olayaki LA. Role of oxidative stress in Cannabis sativa-associated spermatotoxicity: evidence for ameliorative effect of combined but not separate melatonin and vitamin C. Middle East Fertil Soc J. (2017) 22:136–44. doi: 10.1016/j.mefs.2016.12.004
56. Mandal TK, Das NS. Testicular toxicity in cannabis extract treated mice: association with oxidative stress and role of antioxidant enzyme systems. Toxicol Ind Health. (2010) 26:11–23. doi: 10.1177/0748233709354553
57. Yucra S, Gasco M, Rubio J, Nieto J, Gonzales GF. Effect of different fractions from hydroalcoholic extract of Black Maca (Lepidium meyenii) on testicular function in adult male rats. Fertil Steril. (2008) 89:1461–7. doi: 10.1016/j.fertnstert.2007.04.052
58. Del Prete C, Stout T, Montagnaro S, Pagnini U, Uccello M, Florio P, et al. Combined addition of superoxide dismutase, catalase and glutathione peroxidase improves quality of cooled stored stallion semen. Anim Reprod Sci. (2019) 210:106195. doi: 10.1016/j.anireprosci.2019.106195
Keywords: Lepidium meyenii (maca), sperm cells, THC, antioxidant, ultrasound color Doppler
Citation: Greco A, Del Prete C, De Biase D, Palumbo V, Albanese S, Bruzzese D, Carotenuto D, Ciani F, Tafuri S, Meomartino L, Mancini M, Paciello O and Cocchia N (2021) Effects of Oral Administration of Lepidium meyenii on Morphology of Mice Testis and Motility of Epididymal Sperm Cells After Tetrahydrocannabinol Exposure. Front. Vet. Sci. 8:692874. doi: 10.3389/fvets.2021.692874
Received: 09 April 2021; Accepted: 17 November 2021;
Published: 09 December 2021.
Edited by:
Manuel Alvarez Rodriguez, Linköping University, SwedenReviewed by:
Pier Paolo Claudio, University of Mississippi, United StatesAli Shalizar-Jalali, Urmia University, Iran
Copyright © 2021 Greco, Del Prete, De Biase, Palumbo, Albanese, Bruzzese, Carotenuto, Ciani, Tafuri, Meomartino, Mancini, Paciello and Cocchia. This is an open-access article distributed under the terms of the Creative Commons Attribution License (CC BY). The use, distribution or reproduction in other forums is permitted, provided the original author(s) and the copyright owner(s) are credited and that the original publication in this journal is cited, in accordance with accepted academic practice. No use, distribution or reproduction is permitted which does not comply with these terms.
*Correspondence: Veronica Palumbo, veronica.palumbo@unina.it