- 1Department of Pathology and Microbiology, Faculty of Veterinary Medicine, Université de Montréal, Saint-Hyacinthe, QC, Canada
- 2Groupe de Recherche en Épidémiologie des Zoonoses et Santé Publique, Faculty of Veterinary Medicine, Université de Montréal, Saint-Hyacinthe, QC, Canada
- 3Groupe de Recherche sur les Maladies Infectieuses des Animaux de Production, Université de Montréal, Saint-Hyacinthe, QC, Canada
- 4Policy Integration and Zoonoses Division, Centre for Food-borne, Environmental and Zoonotic Infectious Diseases, Public Health Agency of Canada, Saint-Hyacinthe, QC, Canada
- 5Zoonotic Diseases and Special Pathogens Division, National Microbiology Laboratory, Public Health Agency of Canada, Winnipeg, MB, Canada
- 6Laboratoire de Santé Publique du Québec, Institut National de Santé Publique du Québec, Sainte-Anne-de-Bellevue, QC, Canada
- 7Institute of Parasitology, Faculty of Agricultural and Environmental Sciences, McGill University, Macdonald Campus, Sainte-Anne-de-Bellevue, QC, Canada
- 8Department of Microbiology and Immunology, Faculty of Medicine, McGill University, Montreal, QC, Canada
Cats that spend time outdoors and dogs are particularly at risk of exposure to ticks and the pathogens they transmit. A retrospective study on data collected through passive tick surveillance was conducted to estimate the risk of exposure to tick-borne pathogens in cats and dogs bitten by blacklegged ticks (Ixodes scapularis) in the province of Quebec, Canada, from 2010 to 2017. Blacklegged ticks collected from these host animals were tested by PCR for Borrelia burgdorferi sensu stricto, Borrelia miyamotoi, Anaplasma phagocytophilum, and Babesia microti. A total of 13,733 blacklegged ticks were collected from 12,547 animals. Most ticks were adult females and partially engorged. In total, 1,774 cats were infested with ticks and 22.6 and 2.7% of these animals were bitten by at least one tick infected with B. burgdorferi and A. phagocytophilum, respectively. For the 10,773 tick infested dogs, 18.4% were exposed to B. burgdorferi positive ticks while 1.9% of infested dogs were exposed to ticks infected with A. phagocytophilum. The risk of exposure of both cats and dogs to B. miyamotoi and B. microti was lower since only 1.2 and 0.1% of ticks removed were infected with these pathogens, respectively. Traveling outside of the province of Quebec prior to tick collection was significantly associated with exposure to at least one positive tick for B. burgdorferi, A. phagocytophilum and B. microti. Animals exposed to B. burgdorferi or B. miyamotoi positive tick(s) were at higher risk of being concurrently exposed to A. phagocytophilum; higher risk of exposure to B. microti was also observed in animals concurrently exposed to B. burgdorferi. The odds of dogs having B. burgdorferi antibodies were higher when multiple ticks were collected on an animal. The testing and treatment strategies used on dogs bitten by infected ticks were diverse, and misconceptions among veterinarians regarding the treatment of asymptomatic but B. burgdorferi-seropositive dogs were noted. In conclusion, our study demonstrates that cats and dogs throughout Quebec are exposed to blacklegged ticks infected with B. burgdorferi and A. phagocytophilum, and veterinarians across the province need to be aware of this potential threat to the health of pets and their owners.
Introduction
Given their habits, dogs and cats that spend time outdoors are particularly at risk of contracting tick-borne diseases (1, 2). In parts of eastern and central North America, blacklegged ticks, Ixodes scapularis, are the primary vector for the spirochete Borrelia burgdorferi sensu stricto (hereafter referred to as B. burgdorferi), which is the causative agent of Lyme disease (LD) (3). Infection with B. burgdorferi in dogs is most often asymptomatic, but may lead to febrile illness, inappetence, and arthropathy in some animals (4). Neurological signs have also been reported in dogs but are not well-understood, and neither is the fatal myocardial condition that has been documented in Boxer puppies (1). Other possible sequelae of B. burgdorferi infection include the ever-elusive Lyme nephritis, a protein-losing nephropathy, which is a dangerous condition that occurs in <1–2% of B. burgdorferi seropositive dogs (5). As erythema migrans is not known to occur in dogs (2), many canine cases of LD are overlooked until the onset of arthritis or nephritis (1, 6–8). In contrast, cats rarely develop clinical LD following natural infection (9, 10).
Blacklegged ticks can also transmit Anaplasma phagocytophilum, Babesia microti and Borrelia miyamotoi. A. phagocytophilum causes granulocytic anaplasmosis. Much like B. burgdorferi infections, most cases of granulocytic anaplasmosis in dogs are asymptomatic, although some animals may display non-specific signs including: fever, lethargy, lameness, hemolytic anemia, and thrombocytopenia (11–15). Although clinical disease as a result of exposure of cats to A. phagocytophilum has been reported, most feline exposures to infected ticks result in asymptomatic infection. The high seroprevalence of antibodies against A. phagocytophilum in healthy cats is further evidence that infections usually do not result in disease (16–18). Babesia microti causes a malaria-like illness in humans, but it is uncertain whether it can cause disease in companion animals and its taxonomic status is highly debated (19, 20). Nevertheless, this parasite may occasionally cause hematological abnormalities, azotemia, and death in dogs, but tends not to cause clinical signs in cats (21–23). In contrast, B. miyamotoi is not known to cause illness in dogs or cats (24–26).
Many of the aforementioned tick-borne diseases were long considered of low risk to Canadians and their pets given that blacklegged tick populations in North America were mostly limited to the United States (27). In recent years, however, this vector has extended its range to become prevalent in eastern and central portions of Canada, which led to an increased incidence of LD in humans and to serological evidence of exposure to A. phagocytophilum in humans and dogs (28, 29). Seroprevalence studies in dogs also suggest that the risk of exposure to B. burgdorferi has been increasing in Canada in recent years (29, 30).
Management of LD and granulocytic anaplasmosis in dogs is complicated and may vary greatly between veterinary practitioners. Currently, serology is the recommended method for detection of exposure to B. burgdorferi, using one of the existing commercial or reference laboratory tests (1). However, no consensus has been reached by the American College of Veterinary Internal Medicine (ACVIM) on whether asymptomatic B. burgdorferi seropositive dogs should be treated—though there is agreement that animals testing seropositive should be monitored for proteinuria (1, 4). Of note, although serological testing is considered useful evidence of exposure to B. burgdorferi, there is little evidence that antibody titers can reliably predict the onset of clinical signs (1). In dogs suffering from Lyme arthritis, a 4-week course of antibiotics is recommended with doxycycline as first-line choice (1). For granulocytic anaplasmosis, screening for infection by examining stained blood smears under the microscope is common, and diagnosis can be confirmed by detection of serum antibody or PCR on whole blood samples (31, 32). Doxycycline is generally the recommended course of treatment for clinical anaplasmosis, as with LD (31, 32). Tick control is advised for all at-risk dogs and cats for the prevention of tick-borne infections (1). Vaccines for B. burgdorferi are also available, but no consensus was reached among panelists of the ACVIM on their use in endemic areas (1). More research and veterinarian education to demystify the protocols for prevention and management of suspected cases of tick-borne disease are imperative as these diseases become more prevalent in Canada.
This study has several objectives, but the overarching aim is to provide crucial insight into the risk of exposure of companion animals in Quebec, Canada to tick-borne pathogens. Risk of exposure was estimated using I. scapularis ticks collected from cats and dogs and submitted to the Laboratoire de santé publique du Québec (LSPQ) from 2010 to 2017. Blacklegged ticks were tested for pathogens using PCR to (1) estimate the risk of exposure to ticks infected with B. burgdorferi, A. phagocytophilum, B. miyamotoi, or B. microti in cats and dogs bitten by at least one blacklegged tick (by year, administrative region, animal species (cat or dog) and based on the host's history of travel); (2) determine the presence of spatiotemporal clusters of infested cats and dogs exposed to pathogen infected ticks (by pathogen type); (3) assess the risk factors for exposure to infected ticks (by pathogen type) in infested animals; (4) determine the probability of coexposure to multiple pathogens from positive ticks; and (5) describe veterinarians' current practices for the management of animals bitten by ticks with known exposure to infected ticks. This unique bank of information provides a portrait of the recent evolution of tick-borne pathogens in ticks collected from cats and dogs in Quebec, as well as highlighting the management strategies implemented by veterinarians. As a whole, this study will contribute to the development of optimal prevention and management strategies as tick-borne infections become more prevalent in the province of Quebec.
Materials and Methods
Tick Collection and Laboratory Analyses
A passive surveillance program for blacklegged ticks has been ongoing in the province of Quebec since 1990. In the veterinary component of this surveillance system, ticks collected from companion animals (mostly cats and dogs) were submitted by participating veterinary clinics to the LSPQ. More than 500 veterinary clinics have participated in this surveillance program over time. All ticks received by the LSPQ were submitted for species identification and developmental stage evaluation following standard keys and taxonomic references (33–37). Three levels of engorgement were determined visually using semi-quantitative scores for most of the tick species and stages: not engorged, partially engorged and fully engorged. For I. scapularis females, specific size ranges were used to determine the level of engorgement: not engorged: <3.7 mm, partially engorged: 3.7 to 8 mm, fully engorged: >8 mm. For every tick received at the LSPQ, the date of tick collection, the travel history of the animal (with departure and return dates), as well as information on tick instars (larvae, nymph, adult male or adult female), tick condition (dead or alive), and degree of engorgement were compiled.
As part of the surveillance program, all ticks submitted to the LSPQ and identified as I. scapularis were then sent to the National Microbiology Laboratory (NML) of the Public Health Agency of Canada for polymerase chain reaction (PCR) testing. The NML routinely tests blacklegged ticks, submitted through passive tick surveillance, for the human pathogens B. burgdorferi, B. miyamotoi, A. phagocytophilum, and B. microti by real-time PCR (RT-PCR), as previously described (38, 39). Briefly, QIAGEN DNeasy 96 tissue kits (QIAGEN Inc., Mississauga, ON) were used according to the manufacturer's protocol for DNA extraction. A duplex RT-PCR assay was employed to screen samples for Borrelia spp. (including all members of the B. burgdorferi sensu lato group) and A. phagocytophilum by targeting the 23S rRNA and msp2 genes, respectively (39). Analysis for B. microti was conducted using the methods described by Nakajima et al. for the detection of the CCT eta gene (40), followed by an in-house RT-PCR assay targeting the 18S rRNA gene on positive samples. Subsequently, all Borrelia spp.-positive samples collected in 2014 were tested for B. burgdorferi using a confirmatory ospA RT-PCR assay, and for B. miyamotoi using an IGS real-time PCR assay. B. miyamotoi-positives were further verified using the glpQ RT-PCR assay (41). From 2014 onwards, Borrelia spp.-positive samples were confirmed by ospA and glpQ assays only. Samples were tested for A. phagocytophilum and B. burgdorferi throughout the study period, and PCR testing for B. microti and B. miyamotoi was added starting in 2013 and 2014, respectively. Most tick specimens were extracted and tested individually and most partially and fully engorged specimens were cut in half longitudinally, with DNA extracted from only one half of each specimen. If multiple ticks were collected from an animal, a pool of these ticks was tested by PCR.
In this study, data on blacklegged ticks collected from cats and dogs and submitted by veterinary clinics within the province of Quebec between 2010 and 2017, as well as the information gathered about these animals (see Section 2.6) were analyzed. All regions of Quebec were included, except for the administrative region of Montérégie. This region was excluded because, as of 2009, submissions from participating veterinary clinics located in this region were no longer accepted due to resource management issues. Also, data obtained from cats and dogs living in the Montérégie region from which ticks were collected in a clinic located in another region were excluded from our dataset since it would only represent partial data from this area. Similarly, data obtained from cats and dogs living outside the province of Quebec was also excluded.
Prevalence of Exposure of Hosts to Infected Ticks
The risk of exposure to at least one blacklegged tick infected with B. burgdorferi, A. phagocytophilum, B. microti, or B. miyamotoi for tick infested cats and dogs residing in the province of Quebec was estimated with 95% exact confidence intervals (CI) by year, administrative region, and according to the host animal's history of travel 14 days prior to tick collection. Risk of pathogen exposure in tick infested cats and dogs was also described according to the month of tick collection, aggregated overall years of the study.
Risk Mapping and Spatiotemporal Clusters of Exposure to Infected Ticks
All cats and dogs were first geolocated at their owner's municipality of residence and were then aggregated at the regional county municipality for mapping (Figure 1). Choropleth maps were used to illustrate the spatial distribution of cats and dogs exposed to PCR-positive ticks among all infested animals overall years. All mapping was performed in ArcGIS 10.5.1 (ESRI, Redlands, CA, USA) using geographic boundary files from the 2016 Statistics Canada census for administrative regions and population ecumene. All mapping was performed by animal species (cats, dogs) and for the two most frequent pathogens (B. burgdorferi, A. phagocytophilum), and was limited to animals that did not travel outside their municipality of residence within 14 days of tick collection.
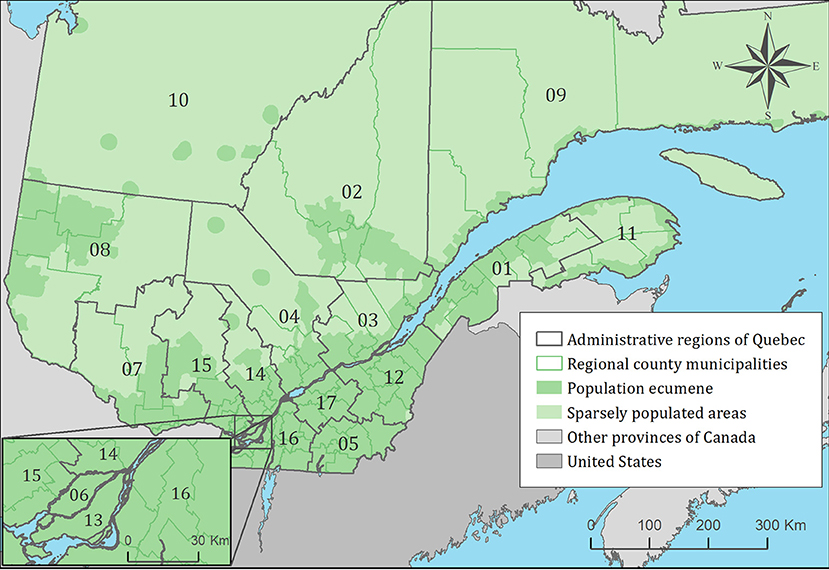
Figure 1. Administrative regions, regional county municipalities, population ecumene (i.e., land with population density ≥0.4 persons per km2) and sparsely populated areas (i.e., unpopulated land or land with population density <0.4 persons per km2) of the province of Quebec, Canada. Administrative regions are: 01: Bas-Saint-Laurent, 02: Saguenay-Lac-Saint-Jean, 03: Capitale-Nationale, 04: Mauricie, 05: Estrie, 06: Montréal, 07: Outaouais, 08: Abitibi-Témiscamingue, 09: Côte-Nord, 10: Nord-du-Québec, 11: Gaspésie-Îles-de-la-Madeleine, 12: Chaudière-Appalaches, 13: Laval, 14: Lanaudière, 15: Laurentides, 16: Montérégie, 17: Centre-du-Québec.
The spatiotemporal trends of the animal risk of exposure to at least one tick infected with B. burgdorferi and A. phagocytophilum was investigated using the Kulldorff scan test, performed separately for each animal species (cats, dogs) and pathogen. A Bernoulli model was used, scanning for the presence of spatiotemporal high-risk clusters of exposition to PCR-positive ticks, performed in SaTScan software version 9.6 (MA, USA) (42). Cases were defined as an animal bitten by at least one infected tick, and controls as an animal bitten by a tick or multiple ticks in which the pathogens of interest were not detected (i.e., negative controls). For this analysis, the spatial unit was the regional county municipality, and the temporal unit was the year (i.e., all cases and controls occurring within the same year and municipality were aggregated). Only cats and dogs that did not travel within 14 days of tick collection were used in this analysis. Statistical significance (p-value < 0.05) was determined based on 9999 Monte Carlo permutations.
Factors Associated With Exposure to Infected Ticks
Logistic regressions were used to model animal exposure to infected ticks for each pathogen based on the maximum level of engorgement of ticks collected from an animal (i.e., in the following categories: fully engorged, partially engorged, or not engorged), number of ticks pooled for PCR testing (one tick vs. two or more ticks), animal species (cat vs. dog), month of tick collection (i.e., in the following categories: January-March, April-June, July-September, October-December) and maximum distance animals traveled in the 14 days prior to tick collection (i.e., in the following categories: no travel, out of municipality, out of administrative region, out of province). The condition (at least one tick alive vs. all dead ticks) and the quality (at least one intact vs. all damaged) of the submitted ticks were also included as control variables and to assess the potential impact of these variables on the likelihood of pathogen detection in a surveillance context. For animals from which more than one tick was collected, the best condition (alive), the best quality (intact) and the maximum level of engorgement (fully engorged) were used for data description and analyses for all specimens. Variables with a p-value <0.20 in the univariable logistic regression models were retained for modeling. Selected variables were included in a full multivariable model and submitted to a manual backward selection procedure using a p-value >0.05 as criteria for rejection. However, for B. miyamotoi and B. microti, due to the limited number of animals exposed to ticks infected with these pathogens, only univariable exact logistic regressions were done. Odds ratios with 95% CI were used to present the results. Statistical analyses were performed on SAS version 9.4 (SAS Institute Inc., Cary, NC, USA).
Coexposure to Multiple Tick-Borne Pathogens
Coexposures of cats and dogs to the aforementioned agents through ticks were described, defined as an animal exposed to ticks coinfected with multiple pathogens or as an animal concurrently infested with multiple ticks infected with different pathogens. For each pairwise combination of pathogens, exact Chi-square tests were performed to evaluate if exposure to one pathogen increases the risk of being concurrently exposed to another one. Due to the limited number of coexposed hosts, cats and dogs were combined for these analyses.
Description of Veterinarian Management Practices and Serological Outcome
When ticks tested positive for B. burgdorferi and/or A. phagocytophilum by PCR, a questionnaire was sent to the veterinary clinics by the LSPQ to collect information on the animal's clinical manifestations and case management, including diagnostic testing and results, treatment, and vaccination. This questionnaire was usually sent 6 to 12 weeks after reception of the tick for identification at the LSPQ, and was completed on a voluntary basis by a veterinarian or animal health technician. Based on data collected from this questionnaire, the diagnostic procedures performed by a veterinarian for animals bitten by B. burgdorferi- and/or A. phagocytophilum-infected tick(s) were described. For dogs on which a SNAP 4DX was performed at least 4 weeks after tick collection and/or a Lyme quantitative C6 antibody assay was performed at least 3 weeks after tick collection, the prevalence of seropositive dogs was calculated. Logistic regression models were used to explore the impact of the level of engorgement (or maximum level of engorgement among ticks collected from an animal and pooled for testing) and the number of ticks collected from one dog on the probability that a dog bitten by at least one B. burgdorferi-infected tick tested seropositive for this agent. The model-building approach was the same as previously described. Finally, the treatment and vaccination of these dogs were described.
Results
Description of Ticks Collected
A total of 13,733 blacklegged ticks were collected from cats and dogs by participating veterinary clinics during the study. In total, 13,445 were adult females, 243 were adult males, 41 were nymphs, and four were larvae. Condition and quality of ticks upon receipt at the LSPQ was available for 7,755 and 7,754 ticks, respectively as these statistics were compiled from April 2014 to 2017. Only 5% (n = 387) of these ticks were alive but most specimens were intact (77%; n = 5,973). Level of engorgement was available for all years of the surveillance program and for 13,669 ticks: 23% (n = 3,134) were fully engorged, 73% (n = 10,040) were partially engorged, and 4% (n = 495) were not engorged.
Description of Animals Infested With Ticks
At least one blacklegged tick was collected from 12,547 cats or dogs over the course of this study. Information on the condition and quality of submitted ticks was available for 7,257 infested hosts, while level of tick engorgement was available for 11,026 infested animals. Among cats and dogs, 384 (5%) were bitten by at least one tick that was submitted alive and 5,547 (76.4%) were bitten by at least one tick that was submitted intact. In addition, 2,567 (23.3%), 8,084 (73.3%), and 375 (3.4%) of infested cats and dogs were bitten by at least one tick that was fully engorged, partially engorged, and not engorged, respectively.
The number of tick-infested cats reported to the surveillance program increased from 122 in 2010 to 438 in 2017 and overall, 1,774 cats were infested with 1,905 blacklegged ticks. As for dogs, 657 were tick-infested in 2010 and the reported number of infested dogs increased to 2,296 in 2017 with an overall total of 10,773 dogs infested with 11,828 ticks. Multiple tick specimens were collected from 5.2% (n = 92) of infested cats and 5.0% (n = 535) of infested dogs. Among animals infested by multiple ticks, the median number of ticks collected and pooled for testing was two in the 92 cats (minimum = 2 ticks, maximum = 12 ticks) and also two in the 535 dogs (minimum = 2 ticks, maximum = 25 ticks). Cats were most often infested with individual adult (n = 1,666 instances) or nymphal (n = 16) ticks, although multiple adult and nymphal ticks were detected on 91 cats and one cat, respectively. Similarly, of the 10,773 infested dogs, 10,223 had only one adult tick, 16 had only one nymphal tick, 530 dogs were infested with multiple adult ticks, four were infested with both adult and nymphal ticks, and one dog was infested with all tick stages. The seasonal distribution of blacklegged tick infestation of cats and dogs is presented in Figure 2. A bimodal distribution pattern of infestation was noted for both cats and dogs; however, fewer cats were infested during the spring peak compared to dogs. In contrast, 34 of 37 animals infested with nymphal ticks were observed between May and August inclusively.
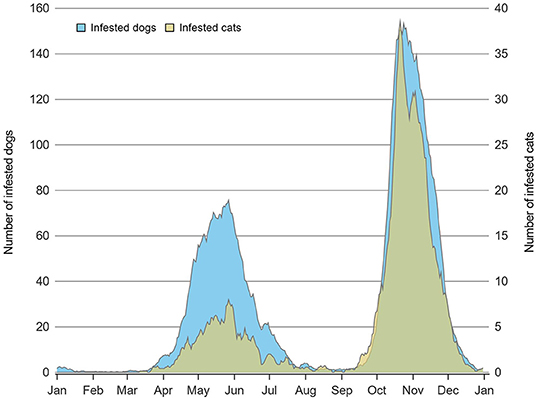
Figure 2. Daily number of dogs (n = 10,773) and cats (n = 1,774) infested with at least one adult tick in Quebec, Canada using a 7-day moving average on data from 2010 to 2017.
Prevalence of Exposure of Hosts to Infected Ticks
The proportion of cats infested with B. burgdorferi-infected blacklegged ticks varied from 14.8% in 2010 to 25.3% in 2017 with the highest prevalence (25.4%) observed in 2014. For infested dogs, the proportion of dogs infested with B. burgdorferi-infected ticks ranged from 16.6% in 2010 to 19.4% in 2017 (Table 1). The proportion of cats and dogs exposed to B. burgdorferi-infected ticks also varied within the administrative region of residence. For example, as few as 4.2% of infested cats in Abitibi-Témiscamingue to 38.5% of cats from Saguenay-Lac-Saint-Jean were exposed to B. burgdorferi-infected ticks. For dogs, the regional risk of exposure to infected ticks varied from 0% in Nord-du-Québec to 25.5% in Côte-Nord (Table 1). Of note, B. burgdorferi was only detected in adult ticks or in pools of ticks including at least one adult. The monthly proportions of cats and dogs infested with B. burgdorferi-infected ticks is presented in Supplementary Table 1.
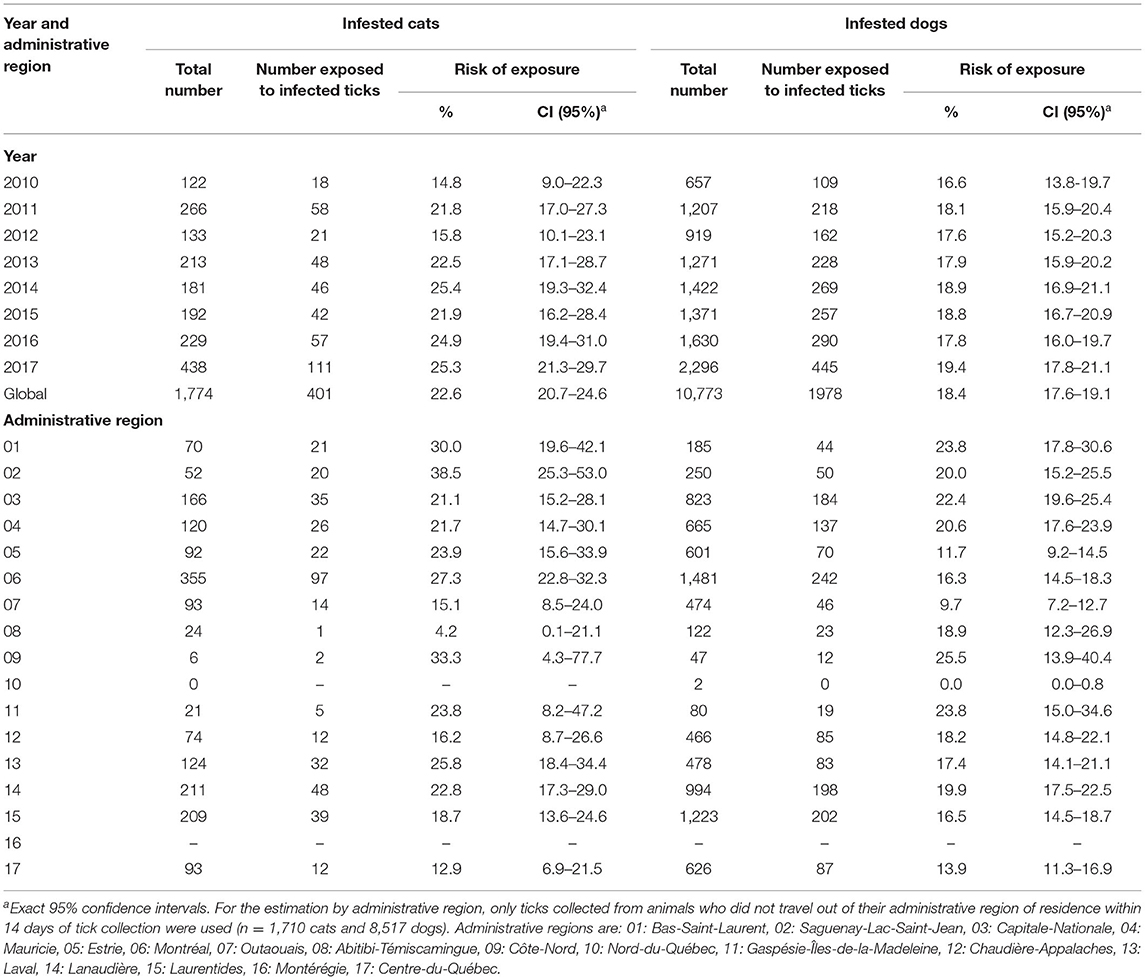
Table 1. Estimated risk of exposure to blacklegged ticks infected with Borrelia burgdorferi in infested cats and dogs per year and administrative region in Quebec, Canada from 2010 to 2017.
The proportion of cats and dogs infested with A. phagocytophilum-infected ticks was much less than those exposed to B. burgdorferi-infected ticks. For example, 0.8% to 5.2% of infested cats and 1.1% to 2.9% of infested dogs were bitten by A. phagocytophilum-infected ticks (Table 2). Regional differences were also noted. As few as 0% of cats in Côte-Nord and Saguenay-Lac-Saint-Jean to as many as 7.1% of cats in Bas-Saint-Laurent were exposed to A. phagocytophilum-infected ticks, although most confidence intervals were overlapping due to limited sample size. A similar range in proportion of infested dogs was noted in different regions (Table 2). The monthly proportions of cats and dogs infested with A. phagocytophilum-infected ticks is presented in Supplementary Table 1.
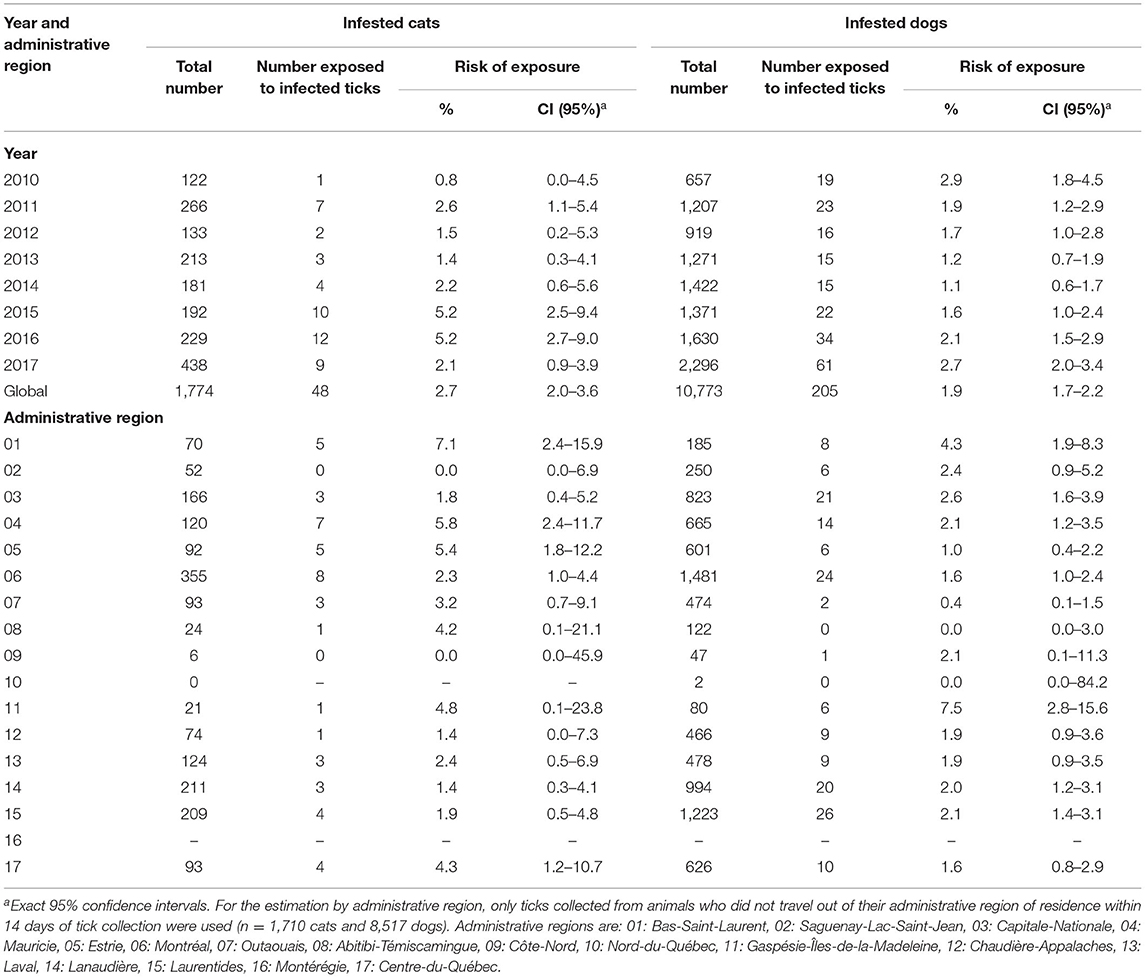
Table 2. Estimated risk of exposure to blacklegged ticks infected with Anaplasma phagocytophilum in infested cats and dogs per year and administrative region in Quebec, Canada from 2010 to 2017.
Ticks collected from a total of 1,239 cats and 7,797 dogs were tested for B. miyamotoi. The proportion of cats exposed to B. miyamotoi-infected ticks varied from 3.5% in 2014 to 0.2% in 2017, with the highest prevalence (8.0%) observed in 2015. A similar pattern was observed in dogs (Supplementary Table 2). Among companion animals that did not travel outside of their administrative region of residence within 14 days of tick collection, exposure to B. miyamotoi-infected ticks occurred in 6 and 11 administrative regions for cats and dogs, respectively (Supplementary Table 2).
Exposure of cats or dogs to ticks infected with B. microti was a very rare event. Only one infested cat and five infested dogs were bitten by ticks infected with this pathogen. The cat had traveled to the United States prior to tick collection while all but one of the dogs had travel histories, namely: one traveled in the Montérégie region, another to Ontario, and the other two to the United States (Supplementary Table 3).
Pets' risk of exposure to these four pathogens through ticks was also estimated for all resident cats and dogs of the surveillance program participative regions, no matter their travel history. For all three studied bacteria and the parasite, the absolute difference between the estimated regional risk of exposure among all resident animals and estimated regional risk of exposure among resident animals that did not travel varied between 0 and 2.5%. However, larger differences were observed between the risk in resident animals for a specific region vs. the risk in non-resident animals that traveled to this region within 14 days of tick collection (Supplementary Tables 4–7).
Risk Mapping and Spatiotemporal Clusters of Exposure to Infected Ticks
The spatial distribution of cats and dogs infested with blacklegged ticks infected with either B. burgdorferi or A. phagocytophilum are presented in Figures 3–8. In dogs, two spatiotemporal clusters were identified (Supplementary Figure 1 and Supplementary Table 8). The first cluster, which represents a higher risk area of exposure to positive ticks for B. burgdorferi, was located in the northern part of the province and was detected from 2011 to 2013. The other cluster of B. burgdorferi positivity was detected in 2015 in eastern Quebec, which only included 10 dogs. No statistically significant spatiotemporal high-risk clusters were identified for A. phagocytophilum or for cats.
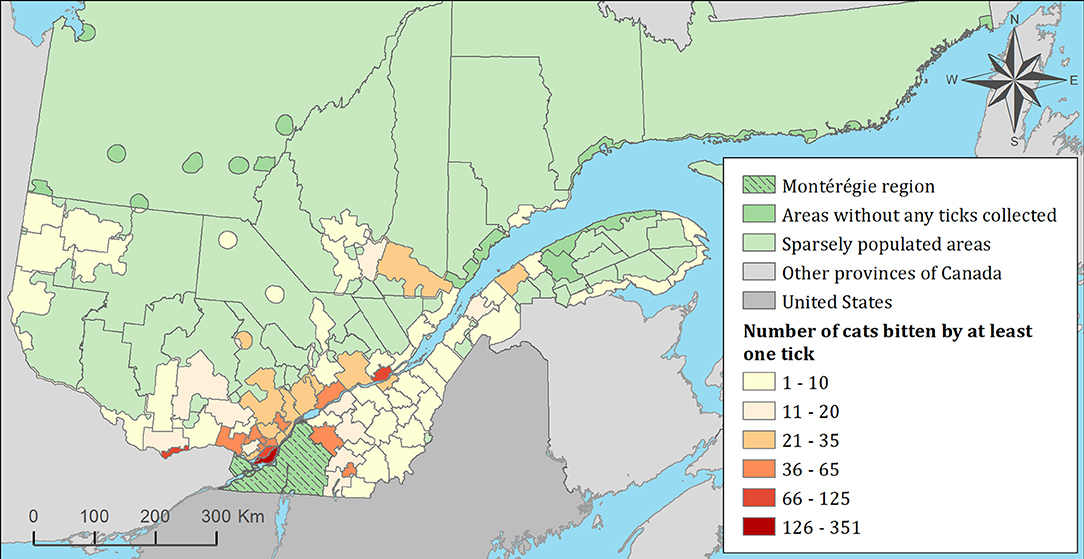
Figure 3. Number of cats infested with blacklegged ticks from 2010 to 2017 by regional county municipality considering the population ecumene (i.e., land with population density ≥0.4 persons per km2) and sparsely populated areas (i.e., unpopulated land or land with population density <0.4 persons per km2) of the province of Quebec, Canada. In total, 1,696 cats that did not travel out of their municipality of residence within 14 days of tick collection were included.
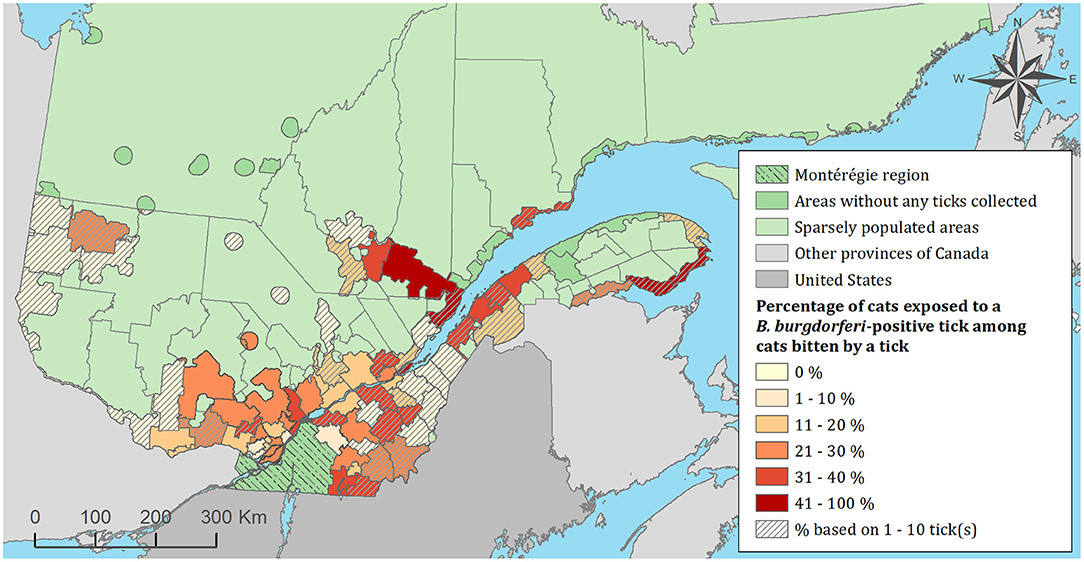
Figure 4. Percentage of cats exposed to Borrelia burgdorferi infected tick(s) among 1,696 cats infested with blacklegged ticks. Data covers from 2010 to 2017 by regional county municipality considering the population ecumene (i.e., land with population density ≥0.4 persons per km2) and sparsely populated areas (i.e., unpopulated land or land with population density <0.4 persons per km2) of the province of Quebec, Canada. Only cats that did not travel out of their municipality of residence within 14 days of tick collection were included.
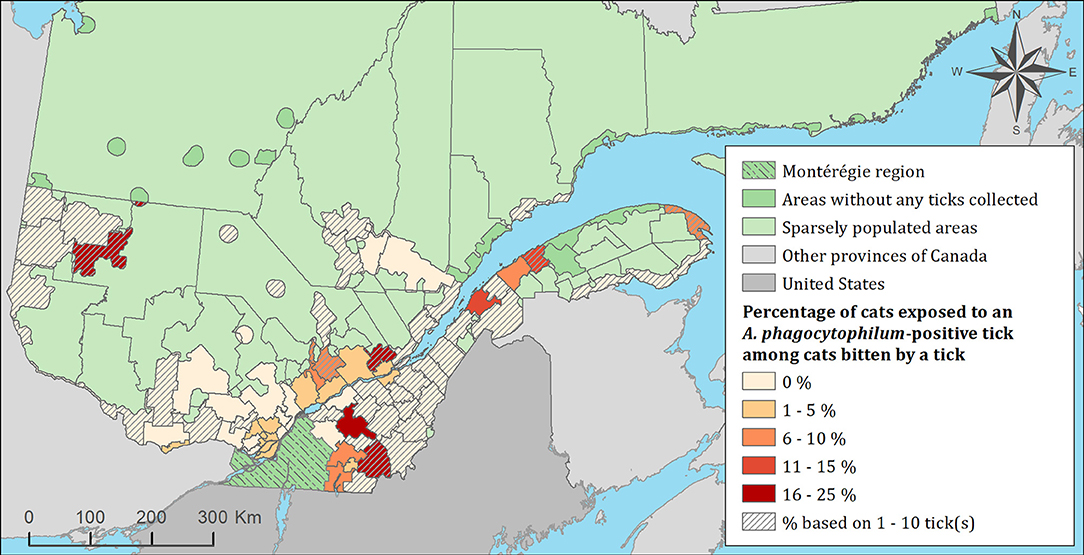
Figure 5. Percentage of cats exposed to Anaplasma phagocytophilum infected tick(s) among 1,696 cats infested with blacklegged ticks. Data covers from 2010 to 2017 by regional county municipality considering the population ecumene (i.e., land with population density ≥0.4 persons per km2) and sparsely populated areas (i.e., unpopulated land or land with population density <0.4 persons per km2) of the province of Quebec, Canada. Only cats that did not travel out of their municipality of residence within 14 days of tick collection were included.
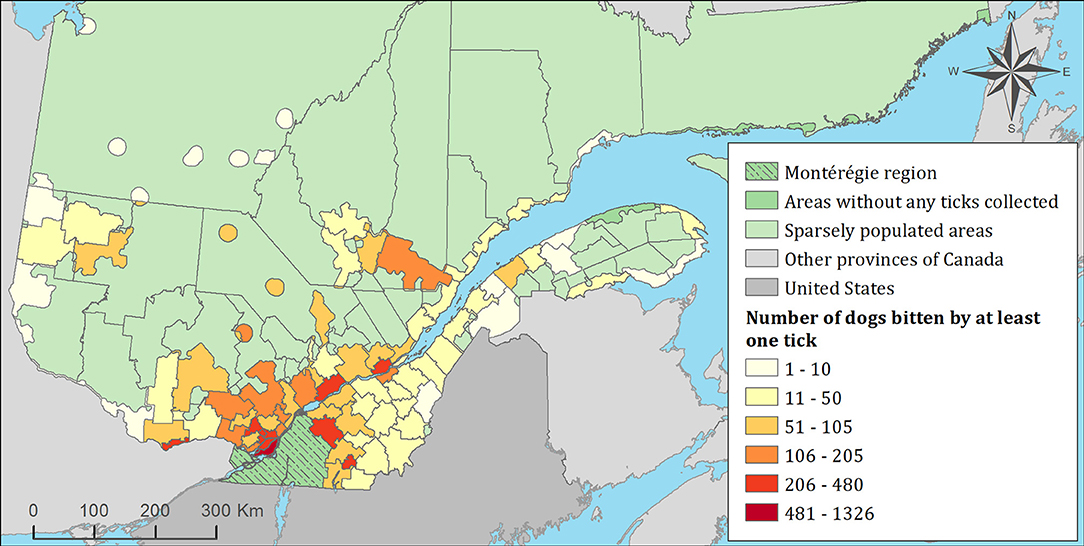
Figure 6. Number of dogs infested with blacklegged ticks from 2010 to 2017 by regional county municipality considering the population ecumene (i.e., land with population density ≥0.4 persons per km2) and sparsely populated areas (i.e., unpopulated land or land with population density <0.4 persons per km2) of the province of Quebec, Canada. In total, 7,644 dogs that did not travel out of their municipality of residence within 14 days of tick collection were included.
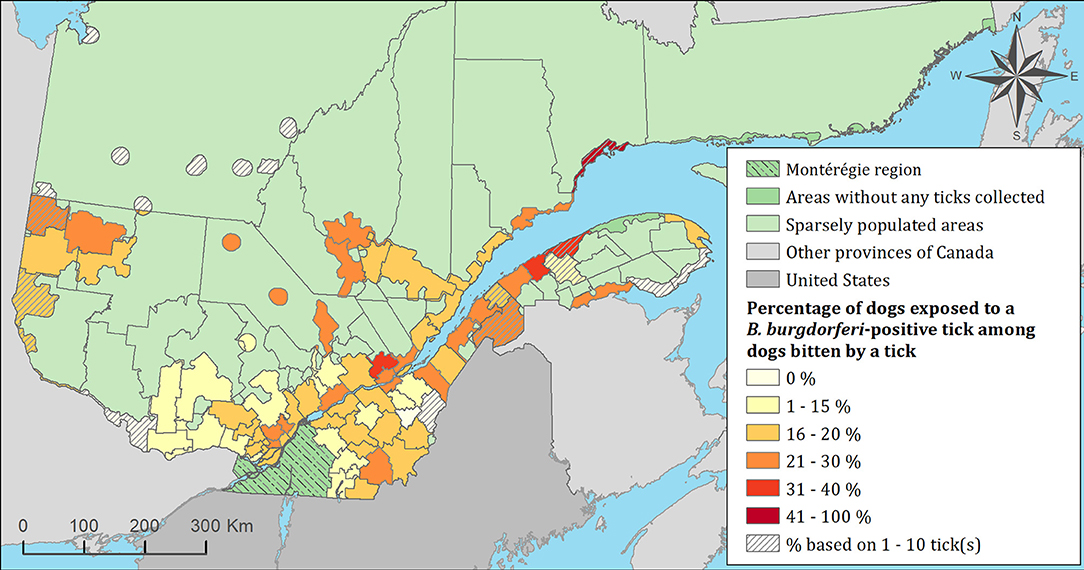
Figure 7. Percentage of dogs exposed to Borrelia burgdorferi infected tick(s) among 7,644 dogs infested with blacklegged ticks. Data cover from 2010 to 2017 by regional county municipality considering the population ecumene (i.e., land with population density ≥0.4 persons per km2) and sparsely populated areas (i.e., unpopulated land or land with population density <0.4 persons per km2) of the province of Quebec, Canada. Only dogs that did not travel out of their municipality of residence within 14 days of tick collection were included.
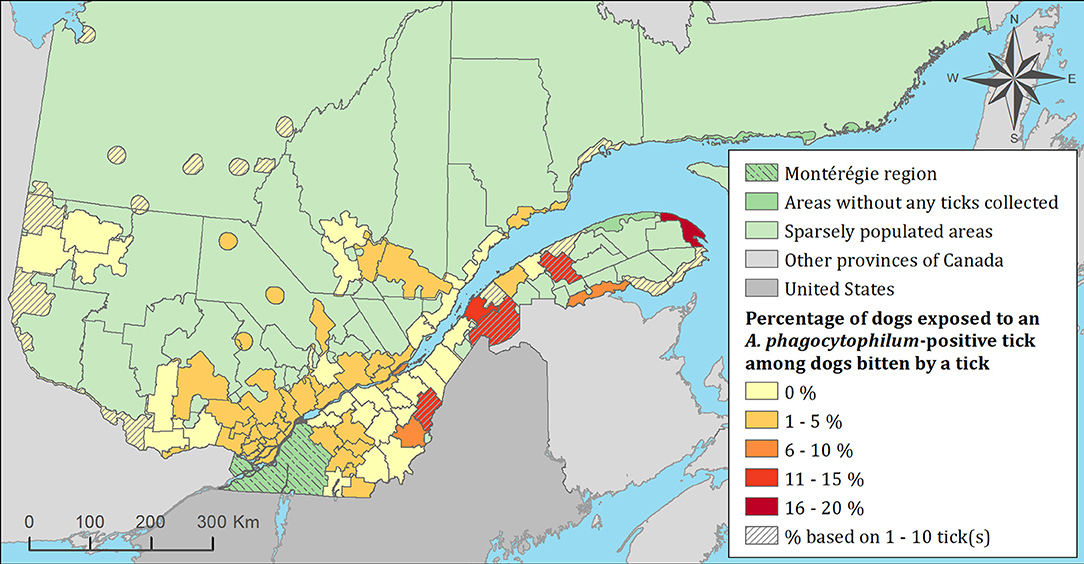
Figure 8. Percentage of dogs exposed to Anaplasma phagocytophilum infected tick(s) among 7,644 dogs infested with blacklegged ticks. Data cover from 2010 to 2017 by regional county municipality considering the population ecumene (i.e., land with population density ≥0.4 persons per km2) and sparsely populated areas (i.e., unpopulated land or land with population density <0.4 persons per km2) of the province of Quebec, Canada. Only dogs that did not travel out of their municipality of residence within 14 days of tick collection were included.
Factors Associated With Exposure of Hosts to Infected Ticks
For the analysis of exposure of cats and dogs to ticks infected with B. burgdorferi, the variables “condition of submitted ticks,” “quality of submitted ticks” and “month of tick collection” were not significant (p-value >0.05) and therefore excluded from the multivariable model. The final multivariable model demonstrated that the odds of exposure to tick(s) that were infected with B. burgdorferi was 2.3 (95% CI: 1.9–2.7) times higher for animals infested with multiple ticks (two or more ticks tested in pools) compared to animals infested with individual ticks, and 1.4 (95% CI: 1.2–1.5) times higher for cats than dogs (Table 3). Having traveled outside the province of Quebec within 14 days of tick collection was also identified as a risk factor for exposure to B. burgdorferi-infected ticks compared to cats and dogs that did not travel outside their municipality of residence, that traveled within their administrative region of residence, or outside their administrative region of residence but within the province (Table 3). Of note, of the 23 animals infested with B. burgdorferi-positive ticks collected from January to March, only six had traveled outside the province in the 14 days prior to tick collection, whereas 15 had not traveled outside the province, and travel histories were not available for two animals.
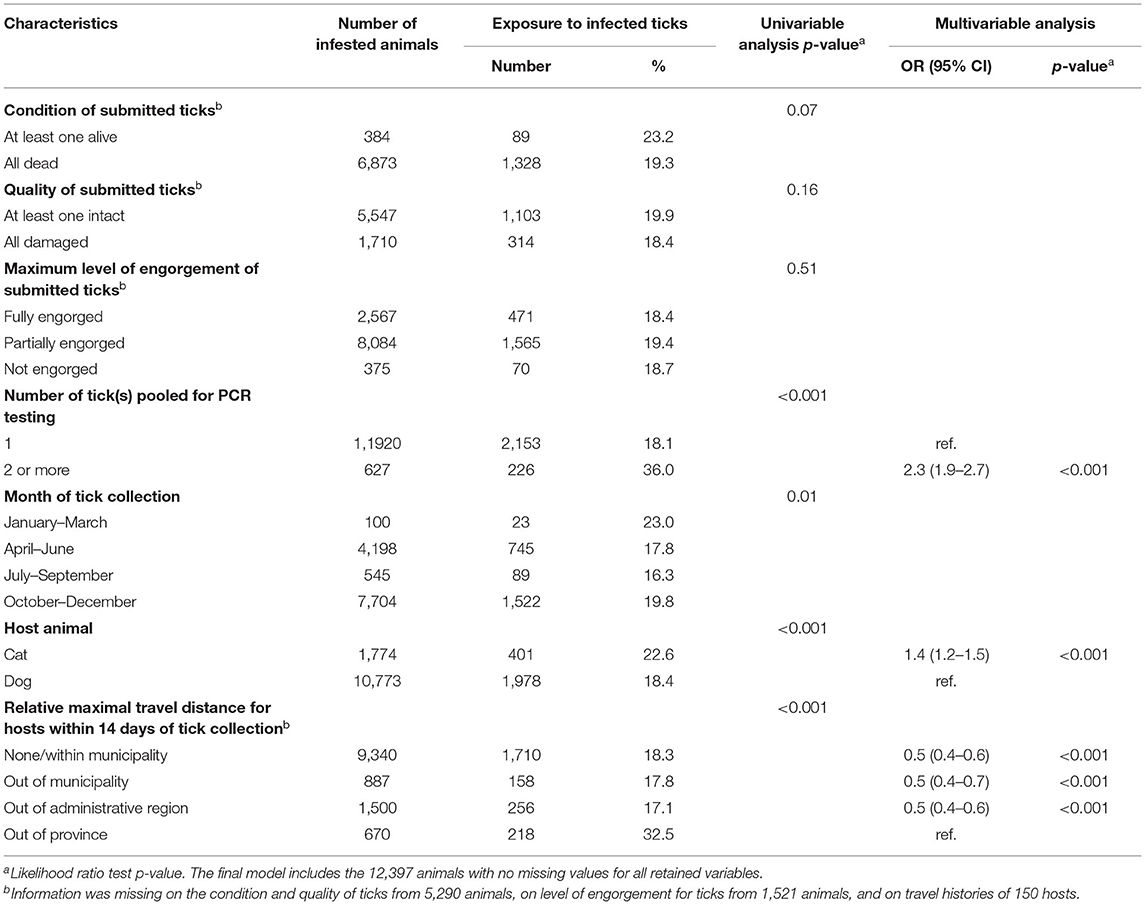
Table 3. Descriptive statistics of characteristics of submitted ticks, month of collection, host species and host travel history with p-value from univariable logistic regression and odd ratios (OR) with 95% confidence intervals (CI) and p-value from multivariable logistic regression modeling the exposure to blacklegged ticks infected with Borrelia burgdorferi in 12,547 infested animals in Quebec, Canada from 2010 to 2017.
For the analysis of exposure of cats and dogs to ticks infected with A. phagocytophilum, the variables “host animal” and “condition of submitted ticks” were excluded from the multivariable model since they were not significant (p-value >0.05). The final multivariable model showed that the odds of exposure to ticks infected with A. phagocytophilum were 1.9 (95% CI: 1.2–2.9) times higher for animals infested with multiple ticks compared to animals exposed to individual ticks. Moreover, the odds of exposure for animals that traveled outside of the province of Quebec within 14 days of tick collection were 5 (95% CI: 2–14) times higher in comparison to animals that only traveled within their administrative region of residence, and 2.5 (95% CI: 1.4–5) times higher compared to animals that traveled to other administrative region(s) but within the province (Table 4).
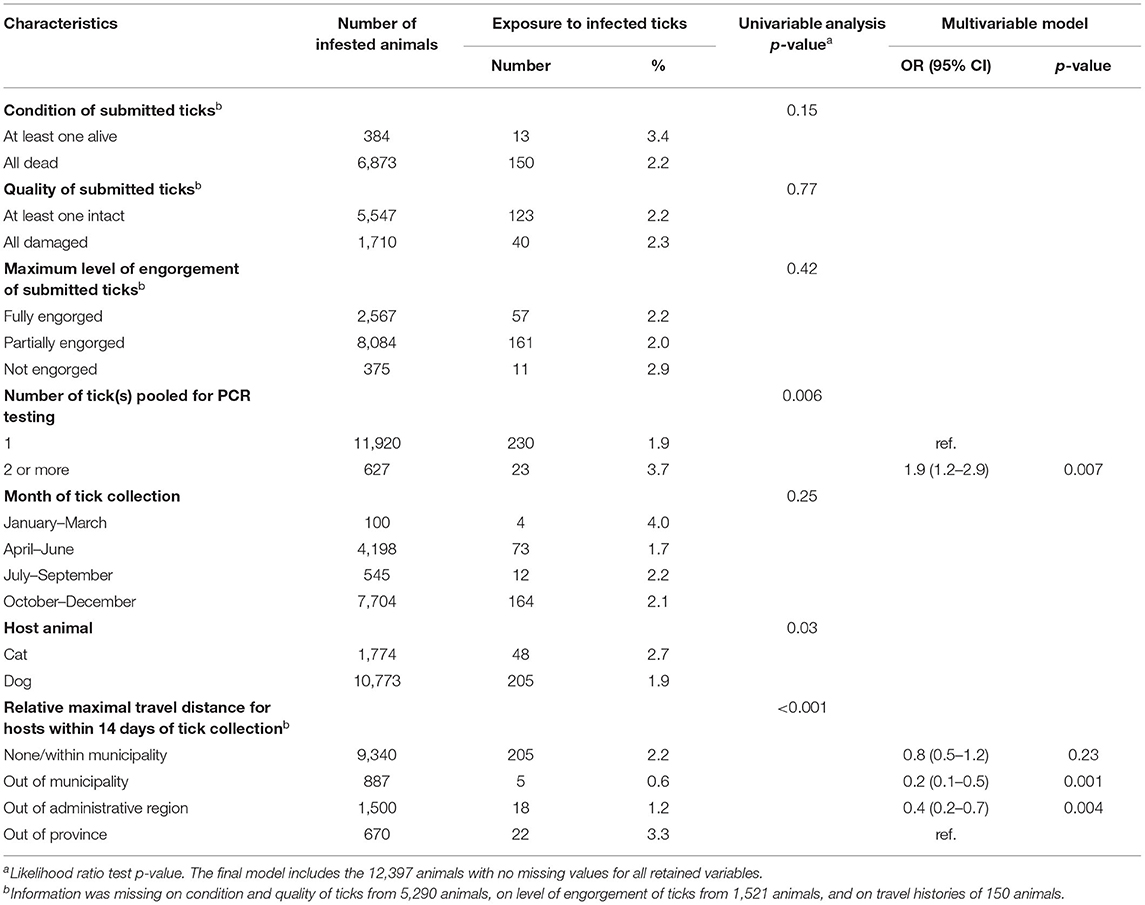
Table 4. Descriptive statistics of characteristics of submitted ticks, month of collection, host species, and host travel history with p-value from univariable logistic regression and odd ratios (OR) with 95% confidence intervals (CI) and p-value from multivariable logistic regression modeling the exposure to blacklegged ticks infected with Anaplasma phagocytophilum in 12,547 infested animals in Quebec, Canada from 2010 to 2017.
For B. miyamotoi, only the month of tick collection was statistically significant in the univariable analysis. The odds of exposure of cats or dogs to ticks infected with B. miyamotoi were 12.6 (95% CI: 2.8–57.0) times higher for animals exposed to tick(s) from January to March compared to animals exposed to ticks from October to December (Supplementary Table 9).
For B. microti, only the number of ticks collected per animal and the travel history were significant in the univariable analysis. The odds of cats or dogs being exposed to ticks infected with B. microti was 18.6 (95% CI: 3.8–92.6) times higher for pets that were infested with multiple ticks compared to animals bitten by a single tick. Animals that traveled out of the province of Quebec within 14 days of tick collection had 66.9 (95% CI: 7.5–599.7) times the odds of exposure to B. microti-infected ticks in comparison to animals that did not travel (Supplementary Table 10).
Coexposures of Hosts to Multiple Tick-Borne Pathogens
During this study, 2,581 (20.6%) of the 12,547 infested cats or dogs were bitten by ticks infected with at least one of the four pathogens of interest (Table 5). The various combinations of pathogens that hosts were exposed to are presented in Table 5. None of the cats or dogs were concurrently exposed to all four pathogens through the bites of one or multiple ticks.
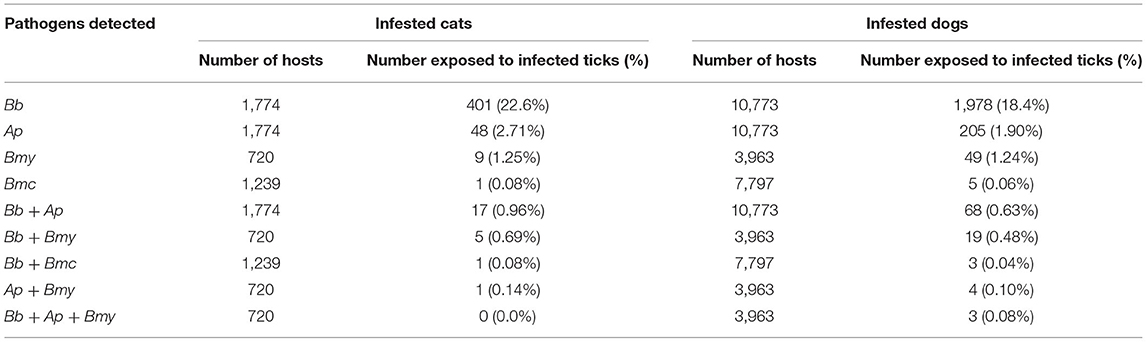
Table 5. Description of exposure and coexposures of cats and dogs to blacklegged ticks infected with Borrelia burgdorferi (Bb), Anaplasma phagocytophilum (Ap), Borrelia miyamotoi (Bmy), and/or Babesia microti (Bmc) in Quebec, Canada from 2010 to 2017.
In total, 1.7% of 10,168 animals bitten by B. burgdorferi PCR-negative ticks were exposed to A. phagocytophilum, compared to 3.6% of 2,379 animals bitten by B. burgdorferi PCR-positive ticks (p < 0.001). Similarly, the risk of exposure to A. phagocytophilum from a tick bite was 2.5% among the 4,625 animals bitten by B. miyamotoi PCR-negative ticks, compared to 8.6% among the 58 animals exposed to B. miyamotoi PCR-positive ticks (p = 0.02). Animals exposed to B. burgdorferi were also at higher risk of being concurrently exposed to B. microti (Supplementary Table 11).
Veterinary Practices and Serological Results
The information regarding testing was only available for 124 cats in the study; only four veterinarians reported performing a serological test (one immunofluorescence assay, one SNAP 4DX and two undetermined tests) on the aforementioned cats. For dogs among which the information was available (i.e., tick testing positive for B. burgdorferi or A. phagocytophilum and voluntary completion and return of a questionnaire by the veterinarian), veterinarians reported performing a serological diagnostic test for 47% (423/908) of dogs bitten by ticks, with information on the diagnostic test performed available for 42% (378/908). Only the SNAP 4DX or SNAP 4DX Plus was used for 75% of the dogs, whereas 16% of dogs had a SNAP 4DX test performed in combination with a Lyme quantitative C6 antibody assay, and 9% of dogs only had the latter test. The date of completion of serological testing extended from the day of tick collection to over a year later, and the median was 74 days after tick collection. Other tests performed by veterinarians in combination with serological testing (or not) were urine analysis (n = 17), especially protein-creatinine ratio (n = 10), a complete blood count (n = 10), serum biochemistry (n = 7), and blood smears (n = 1).
Of the 310 dogs exposed to one or more ticks infected with B. burgdorferi and tested with the SNAP 4DX at least 4 weeks after tick collection, 111 (36%) had evidence of B. burgdorferi antibodies. Of the 82 dogs bitten by at least one B. burgdorferi positive tick on which a Lyme quantitative C6 antibody assay was performed at least 3 weeks after tick collection, 62 (76%) obtained a positive result, and 9 of 54 of these dogs (with available information) developed clinical signs consistent with LD according to their veterinarian. Both the SNAP 4Dx and Lyme Quant C6 were performed on 51 dogs; a positive result was obtained for both diagnostic tests for 41 of these dogs, both tests were negative for three dogs, while for the remaining seven dogs SNAP 4DX was positive and the Lyme Quant C6 was negative. Antibodies against A. phagocytophilum were detected in 34% (16/47) of dogs bitten by ticks infected with A. phagocytophilum and tested with a SNAP 4DX. Of these dogs, one developed clinical signs consistent with anaplasmosis according to their veterinarian. No information was available on the B. burgdorferi or A. phagocytophilum antibody status of these animals prior to tick collection.
The probability of detecting antibodies against B. burgdorferi in dogs bitten by B. burgdorferi-infected ticks was not associated with the maximum level of tick engorgement (Table 6). However, the odds of seropositivity to B. burgdorferi were 2.5 (95% CI: 1.1–5.8) times higher for dogs from which multiple ticks were collected compared to dogs from which only one tick was collected (Table 6).
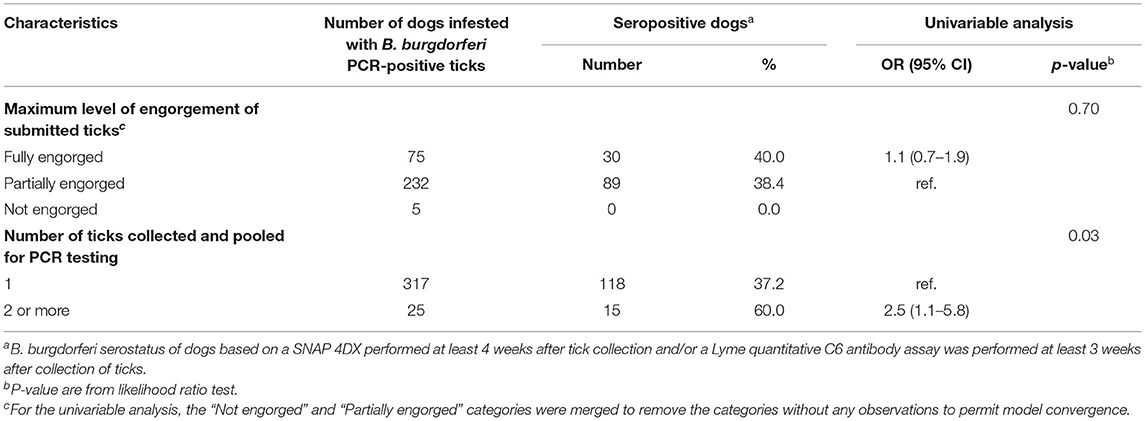
Table 6. Descriptive statistics of characteristics of ticks collected and p-value from univariable logistic regression modeling the seropositivity to Borrelia burgdorferi in dogs infested with blacklegged ticks infected with B. burgdorferi in Quebec, Canada from 2010 to 2017.
Out of 673 dogs bitten by ticks only infected with B. burgdorferi and with available information on treatment, 77 (11%) were treated; 27 within 2 months of tick collection, 45 between 2 and 5 months after tick collection and three at least 5 months after tick collection (date of treatment was missing for two dogs). Among veterinarians who indicated the antibiotic treatment they administered (n = 9), doxycycline (n = 8) was the most common antibiotic used. Information on treatment was available for 124 dogs bitten by ticks only infected with B. burgdorferi and who had positive serology to this agent. Among these dogs, 48 (39%) were treated; 12 within 2 months of tick collection, 35 between 2 to 5 months of tick collection, and the other one at least 5 months after tick collection. Information on the presence of clinical signs compatible with LD and treatment was available for 113 dogs bitten by a tick only infected with B. burgdorferi and seropositive for this pathogen. Among those, 65% (11/17) of dogs that displayed clinical signs of LD and 31% (30/96) of dogs that were asymptomatic were treated. The majority of the clinical signs compatible with LD were observed between 2 and 5 months after tick collection. Out of 38 dogs bitten by ticks only infected with A. phagocytophilum and with available information on treatment, five (13%) were treated; four within 2 months of tick collection and the other one between 2 and 5 months after tick collection. Only one veterinarian indicated the antibiotic treatment they administered, marbofloxacin and amoxicillin. Information on treatment was available for five dogs bitten by ticks only infected with A. phagocytophilum and who were seropositive to this agent. Among these dogs, two (40%) were treated; one within 2 months of tick collection, and the other one between 2 and 5 months after tick collection. For both dogs, no clinical signs compatible with anaplasmosis were reported by the veterinarians before they administered the treatment. Among the 32 dogs bitten by ticks concurrently infected with B. burgdorferi and A. phagocytophilum with available information on treatment, six (19%) were treated; three within 2 months of tick collection and two between 2 and 5 months after tick collection. Half of the treated dogs had a positive serological test for B. burgdorferi and/or A. phagocytophilum, and none presented clinical signs compatible with LD or anaplasmosis based on the available data.
Information on vaccination against LD was available for 712 dogs bitten by B. burgdorferi-positive ticks; 2% (n = 14) were vaccinated for LD within a year before tick collection, 1% (n = 8) had been vaccinated more than 1 year prior to tick collection, 1% (n = 8) were vaccinated but no dates were given, and 96% (n = 682) were not vaccinated. Among non-vaccinated dogs, 4.3% (n = 29) were vaccinated for LD after tick collection.
Discussion
Our findings represent a portrait of exposure to tick-borne pathogens in cats and dogs bitten by blacklegged ticks submitted to passive tick surveillance between 2010 and 2017 in the province of Quebec, Canada. The vast majority of ticks were collected from dogs, and most animals were infested with only one tick at the time of examination. By the time of removal, most ticks were partially or fully engorged, indicative of a recent blood meal (43). Most ticks were adult females, which is consistent with previous studies, and this is likely due in part to an increased ability to notice and remove adult females, which are much larger than nymphs (44, 45); differences in host preference between adult and immature stages of the blacklegged tick could also be involved as reported for other species (46). Interestingly, the bimodal seasonal pattern of infestations of cats and dogs was very similar to the pattern observed in humans from the same area and corresponds to the period of peak host-seeking for adult ticks in Quebec (45). Although we do not have a clear explanation for the lower amplitude of the spring seasonal peak in cats, it could be related to a hypothetical lower propensity for cats to spend time outdoors in risk areas in spring than dogs.
Interestingly, risk of exposure to B. burgdorferi in pets infested with blacklegged ticks in Quebec did not increase significantly between 2010 and 2017, nor did B. burgdorferi present a higher risk in southern areas which are more suitable for tick population establishment. Similarly, a temporal increase of the risk of exposure to blacklegged ticks infected with A. phagocytophilum and B. miyamotoi was not observed for infested cats and dogs. The absence of temporal increase of exposure to infected ticks is in marked contrast to earlier studies in which there was an increase in B. burgdorferi and A. phagocytophilum seropositivity reported in dogs between 2008 and 2015 in Quebec (30). However, a potential explanation is the exclusion of the Montérégie area from the study, which encompasses the large majority of municipalities considered at significant risk for LD during the study period in Quebec. Indeed, ticks collected in our study likely include adventitious ticks that have dropped off from migratory birds during seasonal patterns of spring migration (47). The overall risk of dogs being exposed to blacklegged ticks infected with B. burgdorferi was 18.4%, which is similar to the 15.4% infection rate reported in ticks collected from migratory birds in Canada (48), and is much lower than the >60% prevalence in ticks collected from sentinel surveillance sites from known LD-endemic areas in Quebec (49). Moreover, we do not have a clear explanation for the presence of the spatial and temporal cluster of B. burgdorferi-infected ticks observed in this study. However, considering that these clusters were either very limited in time and geographic space (i.e., one cluster was only present in 2015 and included 10 dogs) or located in northern areas, they are suggestive of a punctual increase in adventitious ticks, perhaps due to natural variation in migratory bird patterns and/or in the relative abundance of ticks infesting these hosts. Although infected adventitious ticks are not considered to constitute a risk for the establishment of an endemic transmission cycle of the pathogens of interest here (50), they do pose a health risk for the animals that they feed upon. Nevertheless, it should be noted that a high prevalence of pathogens in local ticks does not necessarily translate directly into a high regional risk of infection in pets, as this risk also depends on the likelihood of exposure to ticks. This could not be evaluated in our study as we did not have access to the regional pet population numbers of those actively involved in this tick surveillance program. However, our results are very likely representative of the regional risk of exposure to pathogens among animals that are bitten by blacklegged ticks. The very low risk of exposure to blacklegged ticks infected with B. microti during the study period suggests that this parasite did not constitute a significant risk in the province, and this is in agreement with a previous report (51).
The odds of exposure to blacklegged ticks infected with B. burgdorferi, A. phagocytophilum, B. microti and B. miyamotoi were always higher when ticks were pooled for testing and this is most likely due to an increased probability of detecting infected ticks in pooled samples. As an alternative hypothesis, animals with several ticks could be more likely to have picked them up from well-established tick populations with higher local infection rates. Interestingly, neither condition nor engorgement level had a significant effect on the prevalence of pathogens. Inconsistent findings have previously been reported on the impact of tick engorgement on the proportion of ticks infected with B. burgdorferi (43, 47, 52). Fewer damaged ticks were infected with B. burgdorferi but not the other pathogens and as a result, intact ticks should be prioritized for testing.
In our study, risk of exposure to blacklegged ticks infected with B. burgdorferi was significantly higher for infested dogs than cats. Ogden et al. observed a similar pattern and speculated that this may be due to anti-OspA present in vaccinated dogs clearing B. burgdorferi in a number of infected ticks (43, 53). That said, the data provided in our study does not allow us to draw conclusions regarding vaccine outcome. More studies are also warranted to draw definite conclusions on the effects of B. burgdorferi in cats.
Importantly, we observed that dogs which traveled outside of Quebec had a significantly higher risk of exposure to infected ticks (regardless of the pathogen) compared to dogs that did not travel. Tick populations have been well-established and extending their geographic range in Ontario and the United States for years (27, 44). It has been repeatedly demonstrated that B. burgdorferi infection prevalence increases in established tick populations, making areas with long-endemic tick population inherently of higher risk (43, 54). This information reinforces the importance of tick checks and the use of tick infestation preventative measures for animals traveling outside of Quebec. Within Quebec, we also explored the regional risk of exposure to infected ticks for resident animals that did not travel compared to the risk of exposure for non-resident animals that visited the same region, considering only travel history within the 14 days of tick collection. Interestingly, the risk was generally lower for non-resident animals, suggesting that in the context of a surveillance program, it might be important to consider the travel history of animals when presenting regional risk of exposure. This lower risk might be driven by a more frequent use of tick control products by dog owners used to traveling with their animals.
Given the diversity of pathogens with which blacklegged ticks can be infected, coinfections have been a theoretical concern for hosts exposed to multiple pathogens. For example, in humans, coinfections may result in a more serious illness and prolonged clinical signs (55). Likewise, infection with A. phagocytophilum has been reported to impair the immune system in infected mammals, possibly facilitating colonization with a second pathogen (56). Furthermore, these coinfections pose a diagnostic challenge for medical professionals (57). One meta-analysis reported rates of co-infection as high as 28% in some tick species (Ixodes pacificus and Ixodes ricinus were especially prone to coinfections), although coinfection and coexposure to pathogens was unpredictable across tick species and geographic regions (56). Our results demonstrate that coexposure to multiple pathogens occurred rather infrequently in Quebec. This observation seems logical given the overall low prevalence of pathogens other than B. burgdorferi in blacklegged ticks and this is consistent with results reported from other studies conducted in Canada (52). Despite this low prevalence, the probability of detecting a coexposure was higher than expected by chance for certain pathogen combinations, as also reported in other studies (52, 56, 58). The biological reason underlying this association is unclear. Of note, pets that were coexposed to B. burgdorferi and B. microti traveled outside the province of Quebec, mainly in the United-States, within 2 weeks of tick collection.
In our study, 47% of veterinarians that transmitted the information (on a voluntary basis) reported testing for antibodies in dogs bitten by ticks infected with B. burgdorferi and/or A. phagocytophilum. The vast majority were tested using the SNAP 4Dx (or SNAP 4Dx Plus) test, which is in accordance with the ACVIM 2018 guidelines as one of the validated serological tests for B. burgdorferi. Other tests performed included urine analysis and additional bloodwork; and these tests may be critically important to rule out proteinuria or hematological abnormalities in animals with antibodies against B. burgdorferi (1, 4). Information on serological testing was available for only a small number of cats (n = 124); however, four underwent serological tests; which is not surprising given the current lack of consensus on the impact of B. burgdorferi seropositivity in cats (1). Overall, 36% of dogs infested with B. burgdorferi-positive ticks were seropositive, suggesting that at most 36% seroconverted as we did not have access to serological status of dogs prior to tick collection. With respect to A. phagocytophilum, 34% of dogs infested with A. phagocytophilum-positive ticks were also seropositive for this pathogen. Cross-reactions among similar pathogens may play a role here. For example, positive results for A. phagocytophilum on serological testing may reflect exposure to Anaplasma platys, the widespread etiologic agent of cyclic thrombocytopenia, and consequently, results from serological testing need to be interpreted carefully (59). Data pertaining to clinical signs was limited given the voluntary nature of the questionnaire, so we could not use this information to account for possible cross-reactivity.
We observed that the odds of a dog testing seropositive for B. burgdorferi increased for dogs bitten by more than one tick, which could be due to exposure to an overall higher number of infected ticks. Another hypothesis is that dogs with multiple ticks are more likely to have had other attached ticks that went unnoticed in the past, increasing their chances of prior exposure to B. burgdorferi. Although owners may be more likely to notice ticks on their pets when more than one is present, their detection might take some time, especially in animals with thick fur. As B. burgdorferi transmission risk increases with increased tick attachment time, frequent tick checks and timely removal are critical (60). Management of LD is a complex topic that merits attention from general practitioners in the province. For example, recent studies involving family physicians in Quebec have revealed a number of misconceptions regarding the necessity of serological testing and antibiotic therapy in humans, as well as the value of tick testing in diagnosis and treatment of patients exposed to ticks (61, 62). These same issues are of concern among veterinarians in the province. Notably, the use of antibiotics in animals that are otherwise healthy but have antibodies against B. burgdorferi is still controversial. Recent ACVIM guidelines are clear on the value of regular B. burgdorferi screening for animals in endemic areas and testing B. burgdorferi-positive animals for proteinuria, but the treatment of healthy, seropositive dogs without clinical signs or proteinuria was a point of contention (1). The majority of panelists in the aforementioned paper do not recommend treatment in such cases. In our study, 11% of dogs infested with ticks infected only with B. burgdorferi received antibiotics, as well as 31% of dogs seropositive to B. burgdorferi that were bitten by ticks only infected with this pathogen but that did not present clinical signs of LD. This practice is counter to the current recommendations and likely has limited value since there is no evidence that antibiotics are effective in preventing future clinical signs. The ACVIM guidelines cite concerns over antibiotic overuse, lack of total parasite clearance, and possible reinfection as reasons not to treat a seropositive, clinically healthy animal. Furthermore, general practitioners are discouraged from prescribing antibiotics solely based on the outcomes of testing attached ticks (61). On the other hand, for animals clinically ill with LD or anaplasmosis, ACVIM guidelines recommend a 4-week course of antibiotics (1). In our study, 48% of dogs who developed clinical manifestations compatible with LD and/or anaplasmosis were treated, as were more specifically 65% of dogs bitten by ticks only infected with B. burgdorferi that also tested seropositive and that had clinical signs consistent with LD. Doxycycline was the most common antibiotic used, as recommended (1). Notably, some veterinarians reported the presence of clinical manifestations suggestive of LD <2 months after the tick collection, despite the experimental evidence of a long incubation period of 2 to 5 months in dogs (5). Although this might be reflective of veterinarian awareness regarding the large uncertainty surrounding the onset of LD or the knowledge gap on the onset of Lyme nephritis following infection in dogs, leading to a possibility that clinical signs can occur earlier, a misconception among veterinarians of LD evolution in dogs is also possible (5). Alternatively, veterinarians might have been aware of tick exposure in dogs earlier in the season, but this information was not reported to us or made available.
A LD vaccine has been available for dogs since 1990 and there are now numerous options available (1, 4). However, many of these vaccines have inconsistent efficacy and vaccination is also a highly debated topic. In our study, relatively few dogs were vaccinated, perhaps because of the uncertainty associated with their performance and utility. One systematic review and meta-analysis found that, although vaccines were significantly associated with lower odds of developing clinical LD in experimental studies, there are limitations in many studies including small sample size and potential bias (4, 63). Also, no information is available on the potential positive or negative impacts of vaccination on the risk of developing Lyme nephritis (5). Overall, half of 6 ACVIM panelists recommended routine use of LD vaccine in B. burgdorferi-endemic areas in North America (1, 4). In our study, information on vaccination against LD was unavailable for a large proportion of dogs; the data was therefore not substantial enough to draw significant conclusions with respect to vaccine efficacy. Interestingly, 4.3% of dogs were vaccinated after tick collection. This is in accordance with those ACVIM panelists who recommend vaccination against LD; they agreed that the vaccination of healthy, seropositive dogs may prove beneficial to decreasing the risk of reinfection (1). That being said, the use of tick infestation prevention products is to be prioritized (1, 4).
Some limitations should be taken into account in the interpretation of our results. First, underreporting of tick infestation is likely occurring, and this could be due in part to the voluntary nature of the participation by veterinary clinics and pet owners in our tick surveillance program. Representativeness may vary from one region of the province to another and across time depending on the proportion of participating clinics and owners, which may have been influenced by LD awareness campaigns; comparison between years and regions in the number of infested pets should therefore be interpreted cautiously. Considering that tick-borne diseases are evolving rapidly, the current portrait could also be different. Likewise, as no unique identification number for each pet was provided, we could not evaluate the likelihood of multiple submissions in time from the same animal, and thus each submission event had to be considered as independent. As most ticks were fully or partially engorged at time of collection, we cannot exclude that the detected pathogens originated from the host and not the tick. Veterinary management practices following the collection of a tick from cats or dogs could not be thoroughly described in this study because the questionnaire gathering this information was only distributed to clinics that submitted ticks that were found positive for B. burgdorferi or A. phagocytophilum by PCR. The information compiled in this study also does not thoroughly represent the management practices that veterinarians put in place when a tick is found positive, since the chronology of events could not be reliably determined. Indeed, we could not differentiate with confidence whether the serological testing or treatments were undertaken before or after the tick positive result was received by the clinic. Additionally, the completion of the questionnaire was done on a voluntary basis. This may have resulted in an overestimation of the proportion of veterinarians applying specific practices for LD management after tick removal, as these veterinarians may have been more inclined to fill out the questionnaire compared to others. Another limitation concerns the information from our study on clinical signs, since LD clinical signs are not specific, the ones reported in this study could not be definitively associated with LD.
In conclusion, our results support that from 2010 to 2017, cats and dogs throughout Quebec were at risk of exposure to A. phagocytophilum and B. burgdorferi through the bites of infected blacklegged ticks. Approximately 23% of cats and 18% of dogs were bitten by blacklegged ticks infected with B. burgdorferi, and a smaller percentage of hosts were bitten by ticks infected with A. phagocytophilum. As a result, veterinarians should be well aware of these risks and work to remain up-to-date on recommended case management strategies for animals that test positive for LD. Our results also highlight a potential misconception among some veterinarians regarding the need for treatment of healthy B. burgdorferi-seropositive dogs.
Data Availability Statement
The data analyzed in this study is subject to the following licenses/restrictions: The dataset was provided to the research team by the Laboratoire de santé publique du Québec (LSPQ) under a data sharing agreement, with permission limited to the sharing of analysis outcomes. The dataset can be made available upon request to the LSPQ. Requests to access these datasets should be directed to a2FyaW5lLnRoaXZpZXJnZUBpbnNwcS5xYy5jYQ==.
Author Contributions
KT, CF-P, and JA: conceptualization and supervision. LD, SG, and JA: data curation. LD and JA: formal analysis. LD and VW: writing—original draft preparation. LRL and AD provided oversight on all of the tick testing conducted in this study. All authors provided input as part of the manuscript preparation and editing and approved the submitted version.
Funding
This project was funded by Intervet Canada Corp., operating in Canada as Merck Animal Health. This research was supported by the Institut national de santé publique du Québec (INSPQ), the Ministère de la santé et des service sociaux (MSSS) and the Public Health Agency of Canada (PHAC).
Conflict of Interest
The authors declare that this study received funding from Intervet Canada Corp., operating in Canada as Merck Animal Health. The funder was not involved in the study design, collection, analysis, interpretation of data or the writing of this article.
Acknowledgments
We would like to thank all veterinary practitioners who participated in the surveillance program, as well as all the people who contributed to surveillance data collection, tick identification and testing. Special thanks are warranted for Brooks Waitt and Mahmood Iranpour from the NML for DNA extractions and PCR testing on the large number of ticks collected during this study.
Supplementary Material
The Supplementary Material for this article can be found online at: https://www.frontiersin.org/articles/10.3389/fvets.2021.696815/full#supplementary-material
Abbreviations
LD, Lyme disease.
References
1. Littman MP, Gerber B, Goldstein RE, Labato MA, Lappin MR, Moore GE, et al. consensus update on Lyme borreliosis in dogs and cats. J Vet Intern Med. (2018) 32:887–903. doi: 10.1111/jvim.15085
2. Little SE, Heise SR, Blagburn BL, Callister SM, Mead PS. Lyme borreliosis in dogs and humans in the USA. Trends Parasitol. (2010) 26:213–8. doi: 10.1016/j.pt.2010.01.006
3. Stanek G, Wormser GP, Gray J, Strle F. Lyme borreliosis. Lancet. (2012) 379:461–73. doi: 10.1016/S0140-6736(11)60103-7
4. Littman MP, Goldstein RE, Labato MA, Lappin MR, Moore GE. ACVIM small animal consensus statement on Lyme disease in dogs: diagnosis, treatment, and prevention. J Vet Intern Med. (2006) 20:422–34. doi: 10.1111/j.1939-1676.2006.tb02880.x
6. Fritz CL, Kjemtrup AM. Lyme borreliosis. J Am Vet Med Assoc. (2003) 223:1261–70. doi: 10.2460/javma.2003.223.1261
7. Bacon RM, Kugeler KJ, Mead PS, Centers for Disease Control and Prevention. Surveillance for Lyme disease–United States, 1992-2006. MMWR Surveill Summ. (2008) 57:1–9. Available online at: https://www.cdc.gov/mmwr/preview/mmwrhtml/ss5710a1.htm
8. Nadelman RB, Nowakowski J, Forseter G, Goldberg NS, Bittker S, Cooper D, et al. The clinical spectrum of early lyme borreliosis in patients with culture-confirmed erythema migrans. Am J Med. (1996) 100:502–8. doi: 10.1016/S0002-9343(95)99915-9
9. Krupka I, Straubinger RK. Lyme borreliosis in dogs and cats: background, diagnosis, treatment and prevention of infections with Borrelia burgdorferi sensu stricto. Vet Clin North Am Small Anim Pract. (2010) 40:1103–19. doi: 10.1016/j.cvsm.2010.07.011
10. Hoyt K, Chandrashekar R, Beall M, Leutenegger C, Lappin MR. Evidence for clinical anaplasmosis and borreliosis in cats in Maine. Top Companion Anim Med. (2018) 33:40–4. doi: 10.1053/j.tcam.2018.05.002
11. Carrade DD, Foley JE, Borjesson DL, Sykes JE. Canine granulocytic anaplasmosis: a review. J Vet Intern Med. (2009) 23:1129–41. doi: 10.1111/j.1939-1676.2009.0384.x
12. Beall MJ, Chandrashekar R, Eberts MD, Cyr KE, Diniz PP, Mainville C, et al. Serological and molecular prevalence of Borrelia burgdorferi, Anaplasma phagocytophilum, and Ehrlichia species in dogs from Minnesota. Vector Borne Zoonotic Dis. (2008) 8:455–64. doi: 10.1089/vbz.2007.0236
13. Bexfield NH, Villiers EJ, Herrtage ME. Immune-mediated haemolytic anaemia and thrombocytopenia associated with Anaplasma phagocytophilum in a dog. J Small Anim Pract. (2005) 46:543–8. doi: 10.1111/j.1748-5827.2005.tb00284.x
14. Lester SJ, Breitschwerdt EB, Collis CD, Hegarty BC. Anaplasma phagocytophilum infection (granulocytic anaplasmosis) in a dog from Vancouver Island. Can Vet J. (2005) 46:825–7.
15. Poitout FM, Shinozaki JK, Stockwell PJ, Holland CJ, Shukla SK. Genetic variants of Anaplasma phagocytophilum infecting dogs in Western Washington State. J Clin Microbiol. (2005) 43:796–801. doi: 10.1128/JCM.43.2.796-801.2005
16. Bjoersdorff A, Svendenius L, Owens JH, Massung RF. Feline granulocytic ehrlichiosis–a report of a new clinical entity and characterisation of the infectious agent. J Small Anim Pract. (1999) 40:20–4. doi: 10.1111/j.1748-5827.1999.tb03249.x
17. Lappin MR, Breitschwerdt EB, Jensen WA, Dunnigan B, Rha JY, Williams CR, et al. Molecular and serologic evidence of Anaplasma phagocytophilum infection in cats in North America. J Am Vet Med Assoc. (2004) 225: 893–6. doi: 10.2460/javma.2004.225.893
18. Magnarelli LA, Bushmich SL, Ijdo JW, Fikrig E. Seroprevalence of antibodies against Borrelia burgdorferi and Anaplasma phagocytophilum in cats. Am J Vet Res. (2005) 66:1895–9. doi: 10.2460/ajvr.2005.66.1895
19. Homer MJ, Aguilar-Delfin I, Telford SR, Krause PJ, Persing DH. Babesiosis. Clin Microbiol Rev. (2000) 13:451. doi: 10.1128/CMR.13.3.451
20. Baneth G, Florin-Christensen M, Cardoso L, Schnittger L. Reclassification of Theileria annae as Babesia vulpes sp. nov. Parasit Vectors. (2015) 8:207. doi: 10.1186/s13071-015-0830-5
21. Camacho AT, Guitian EJ, Pallas E, Gestal JJ, Olmeda AS, Goethert HK, et al. Azotemia and mortality among Babesia microti-Like infected dogs. J Vet Intern Med. (2004) 18:141–6. doi: 10.1111/j.1939-1676.2004.tb00152.x
22. Simoes PB, Cardoso L, Araujo M, Yisaschar-Mekuzas Y, Baneth G. Babesiosis due to the canine Babesia microti-like small piroplasm in dogs-first report from Portugal and possible vertical transmission. Parasit Vectors. (2011) 4:50. doi: 10.1186/1756-3305-4-50
23. Spada E, Proverbio D, Galluzzo P, Perego R, Bagnagatti De Giorgi G, Roggero N, et al. Frequency of piroplasms Babesia microti and Cytauxzoon felis in stray cats from Northern Italy. Biomed Res Int. (2014) 2014:943754. doi: 10.1155/2014/943754
24. Siński E, Welc-Faleciak R, Zajkowska J. Borrelia miyamotoi: a human tick-borne relapsing fever spirochete in Europe and its potential impact on public health. Adv Med Sci. (2016) 61:255–60. doi: 10.1016/j.advms.2016.03.001
25. Telford SRI, Goethert HK, Molloy PJ, Berardi VP, Chowdri HR, Gugliotta JL, et al. Borrelia miyamotoi disease: neither Lyme disease nor relapsing fever. Clin Lab Med. (2015) 35:867–82. doi: 10.1016/j.cll.2015.08.002
26. Schreiber C, Krücken J, Beck S, Maaz D, Pachnicke S, Krieger K, et al. Pathogens in ticks collected from dogs in Berlin/Brandenburg, Germany. Parasit Vectors. (2014) 7:535. doi: 10.1186/s13071-014-0535-1
27. Hoen AG, Margos G, Bent SJ, Diuk-Wasser MA, Barbour A, Kurtenbach K, et al. Phylogeography of Borrelia burgdorferi in the eastern United States reflects multiple independent Lyme disease emergence events. Proc Natl Acad Sci USA. (2009) 106:15013–8. doi: 10.1073/pnas.0903810106
28. Nelder MP, Russell CB, Lindsay LR, Dibernardo A, Brandon NC, Pritchard J, et al. Recent emergence of Anaplasma phagocytophilum in Ontario, Canada: early serological and entomological Indicators. Am J Trop Med Hyg. (2019) 101:1249–58. doi: 10.4269/ajtmh.19-0166
29. Ogden NH, Bouchard C, Badcock J, Drebot MA, Elias SP, Hatchette TF, et al. What is the real number of Lyme disease cases in Canada? BMC Public Health. (2019) 19:849. doi: 10.1186/s12889-019-7219-x
30. Evason M, Stull JW, Pearl DL, Peregrine AS, Jardine C, Buch JS, et al. Prevalence of Borrelia burgdorferi, Anaplasma spp., Ehrlichia spp. and Dirofilaria immitis in Canadian dogs, 2008 to 2015: a repeat cross-sectional study. Parasit Vectors. (2019) 12:64. doi: 10.1186/s13071-019-3299-9
31. Kohn B, Silaghi C, Galke D, Arndt G, Pfister K. Infections with Anaplasma phagocytophilum in dogs in Germany. Res Vet Sci. (2011) 91:71–6. doi: 10.1016/j.rvsc.2010.08.008
32. Cockwill KR, Taylor SM, Snead EC, Dickinson R, Cosford K, Malek S, et al. Granulocytic anaplasmosis in three dogs from Saskatoon, Saskatchewan. Can Vet J. (2009) 50:835–40.
33. Lindquist EE, Galloway TD, Artsob H, Lindsay LR, Drebot M, Wood H, et al. A Handbook to the Ticks of Canada (Ixodida: Ixodidae, Argasidae). Monograph series No. 7. Biological Survey of Canada, (2016) doi: 10.3752/9780968932186
34. Durden LA, Keirans JE. Nymphs of the Genus Ixodes (Acari: Ixodidae) of the United States : Taxonomy, Identification Key, Distribution, Hosts, and Medical/Veterinary Importance. Lanham, MD: Entomological Society of America, (1996).
35. Keirans JE, Litwak TR. Pictorial key to the adults of hard ticks, family Ixodidae (Ixodida: Ixodoidea), east of the Mississippi River. J Med Entomol. (1989) 26:435–48. doi: 10.1093/jmedent/26.5.435
36. Cooley RA, Kohls GM. The Genus Ixodes in North America. National Institute of Health Bulletin No.184. Washington, DC: United States Government Printing Office (1945).
37. Clifford CM, Anastos G, Elbl A. The larval ixodid ticks of the eastern United States (Acarina-Ixodidae). Misc Publ Entomol Soc Am. (1961) 2:215–44.
38. Smith KA, Oesterle PT, Jardine CM, Dibernardo A, Huynh C, Lindsay R, et al. Tick infestations of wildlife and companion animals in Ontario, Canada, with detection of human pathogens in Ixodes scapularis ticks. Ticks Tick Borne Dis. (2019) 10:72–6. doi: 10.1016/j.ttbdis.2018.08.018
39. Courtney JW, Kostelnik LM, Zeidner NS, Massung RF. Multiplex real-time PCR for detection of Anaplasma phagocytophilum and Borrelia burgdorferi. J Clin Microbiol. (2004) 42:3164–8. doi: 10.1128/JCM.42.7.3164-3168.2004
40. Nakajima R, Tsuji M, Oda K, Zamoto-Niikura A, Wei Q, Kawabuchi-Kurata T, et al. Babesia microti-group parasites compared phylogenetically by complete sequencing of the CCTeta gene in 36 isolates. J Vet Med Sci. (2009) 71:55–68. doi: 10.1292/jvms.71.55
41. Ullmann AJ, Gabitzsch ES, Schulze TL, Zeidner NS, Piesman J. Three multiplex assays for detection of Borrelia burgdorferi sensu lato and Borrelia miyamotoi sensu lato in field-collected Ixodes nymphs in North America. J Med Entomol. (2005) 42:1057–62. doi: 10.1603/0022-2585(2005)0421057:TMAFDO2.0.CO;2
42. Kulldorff M. A spatial scan statistic. Commun Stat Theory Methods. (1997) 26:1481–96. doi: 10.1080/03610929708831995
43. Ogden NH, Bouchard C, Kurtenbach K, Margos G, Lindsay LR, Trudel L, et al. Active and passive surveillance and phylogenetic analysis of Borrelia burgdorferi elucidate the process of Lyme disease risk emergence in Canada. Environ Health Perspect. (2010) 118:909–14. doi: 10.1289/ehp.0901766
44. Nelder MP, Russell C, Lindsay LR, Dhar B, Patel SN, Johnson S, et al. Population-based passive tick surveillance and detection of expanding foci of blacklegged ticks Ixodes scapularis and the Lyme disease agent Borrelia burgdorferi in Ontario, Canada. PLoS ONE. (2014) 9:e105358. doi: 10.1371/journal.pone.0105358
45. Gasmi S, Ogden NH, Leighton PA, Lindsay LR, Thivierge K. Analysis of the human population bitten by Ixodes scapularis ticks in Quebec, Canada: Increasing risk of Lyme disease. Ticks Tick Borne Dis. (2016) 7:1075–81. doi: 10.1016/j.ttbdis.2016.09.006
46. Kollars TM. Jr., Oliver JH, Jr., Kollars PG, Durden LA. Seasonal activity and host associations of Ixodes scapularis (Acari: Ixodidae) in southeastern Missouri. J Med Entomol. (1999) 36:720–6. doi: 10.1093/jmedent/36.6.720
47. Ogden NH, Trudel L, Artsob H, Barker IK, Beauchamp G, Charron DF, et al. Ixodes scapularis ticks collected by passive surveillance in Canada: analysis of geographic distribution and infection with Lyme borreliosis agent Borrelia burgdorferi. J Med Entomol. (2006) 43:600–9. doi: 10.1603/0022-2585(2006)43600:ISTCBP2.0.CO;2
48. Ogden NH, Lindsay LR, Hanincova K, Barker IK, Bigras-Poulin M, Charron DF, et al. Role of migratory birds in introduction and range expansion of Ixodes scapularis ticks and of Borrelia burgdorferi and Anaplasma phagocytophilum in Canada. Appl Environ Microbiol. (2008) 74:1780–90. doi: 10.1128/AEM.01982-07
49. Guillot C, Badcock J, Clow K, Cram J, Dergousoff S, Dibernardo A, et al. Sentinel surveillance of Lyme disease risk in Canada, 2019: Results from the first year of the Canadian Lyme Sentinel Network (CaLSeN). Can Commun Dis Rep. (2020) 46:354–61. doi: 10.14745/ccdr.v46i10a08
50. Hasle G. Transport of ixodid ticks and tick-borne pathogens by migratory birds. Front Cell Infect Microbiol. (2013) 3:48. doi: 10.3389/fcimb.2013.00048
51. O'brien SF, Delage G, Scalia V, Lindsay R, Bernier F, Dubuc S, et al. Seroprevalence of Babesia microti infection in Canadian blood donors. Transfusion. (2016) 56:237–43. doi: 10.1111/trf.13339
52. Dibernardo A, Cote T, Ogden NH, Lindsay LR. The prevalence of Borrelia miyamotoi infection, and co-infections with other Borrelia spp. in Ixodes scapularis ticks collected in Canada. Parasit Vectors. (2014) 7:183. doi: 10.1186/1756-3305-7-183
53. Schwan TG, Piesman J. Vector interactions and molecular adaptations of lyme disease and relapsing fever spirochetes associated with transmission by ticks. Emerg Infect Dis. (2002) 8:115–21. doi: 10.3201/eid0802.010198
54. Leighton PA, Koffi JK, Pelcat Y, Lindsay LR, Ogden NH. Predicting the speed of tick invasion: an empirical model of range expansion for the Lyme disease vector Ixodes scapularis in Canada. J Appl Ecol. (2012) 49:457–64. doi: 10.1111/j.1365-2664.2012.02112.x
55. Dos Santos CC, Kain KC. Two tick-borne diseases in one: a case report of concurrent babesiosis and Lyme disease in Ontario. CMAJ. (1999) 160:1851–3.
56. Nieto NC, Foley JE. Meta-analysis of coinfection and coexposure with Borrelia burgdorferi and Anaplasma phagocytophilum in humans, domestic animals, wildlife, and Ixodes ricinus-complex ticks. Vector Borne Zoonotic Dis. (2009) 9:93–102. doi: 10.1089/vbz.2008.0072
57. Lyme Disease Co-Infection. National Institute of Allergy and Infectious Diseases. Available online at: https://www.niaid.nih.gov/diseases-conditions/lyme-disease-co-infection (accessed October 2019).
58. Sun J, Liu Q, Lu L, Ding G, Guo J, Fu G, et al. Coinfection with four genera of bacteria (Borrelia, Bartonella, Anaplasma, and Ehrlichia) in Haemaphysalis longicornis and Ixodes sinensis ticks from China. Vector Borne Zoonotic Dis. (2008) 8:791–6. doi: 10.1089/vbz.2008.0005
59. Abarca K, Lopez J, Perret C, Guerrero J, Godoy P, Veloz A, et al. Anaplasma platys in dogs, Chile. Emerg Infect Dis. (2007) 13:1392–5. doi: 10.3201/eid1309.070021
60. Cook MJ. Lyme borreliosis: a review of data on transmission time after tick attachment. Int J Gen Med. (2015) 8:1–8. doi: 10.2147/IJGM.S73791
61. Ferrouillet C, Milord F, Lambert L, Vibien A, Ravel A. Lyme disease: Knowledge and practices of family practitioners in southern Quebec. Can J Infect Dis Med Microbiol. (2015) 26:151–6. doi: 10.1155/2015/846963
62. Gasmi S, Ogden NH, Leighton PA, Adam-Poupart A, Milord F, Lindsay LR, et al. Practices of Lyme disease diagnosis and treatment by general practitioners in Quebec, 2008-2015. BMC Fam Pract. (2017) 18:65. doi: 10.1186/s12875-017-0636-y
Keywords: Anaplasma phagocytophilum, Babesia microti, Borrelia burgdorferi, cat, dog, Ixodes scapularis, vector-borne, zoonosis
Citation: Duplaix L, Wagner V, Gasmi S, Lindsay LR, Dibernardo A, Thivierge K, Fernandez-Prada C and Arsenault J (2021) Exposure to Tick-Borne Pathogens in Cats and Dogs Infested With Ixodes scapularis in Quebec: An 8-Year Surveillance Study. Front. Vet. Sci. 8:696815. doi: 10.3389/fvets.2021.696815
Received: 17 April 2021; Accepted: 15 June 2021;
Published: 15 July 2021.
Edited by:
Andrei Daniel Mihalca, University of Agricultural Sciences and Veterinary Medicine of Cluj-Napoca, RomaniaReviewed by:
Ana M. Palomar, Hospital San Pedro de La Rioja, SpainKevin Bown, University of Salford, United Kingdom
Mirabela Dumitrache, University of Agricultural Sciences and Veterinary Medicine of Cluj-Napoca, Romania
Copyright © 2021 Duplaix, Wagner, Gasmi, Lindsay, Dibernardo, Thivierge, Fernandez-Prada and Arsenault. This is an open-access article distributed under the terms of the Creative Commons Attribution License (CC BY). The use, distribution or reproduction in other forums is permitted, provided the original author(s) and the copyright owner(s) are credited and that the original publication in this journal is cited, in accordance with accepted academic practice. No use, distribution or reproduction is permitted which does not comply with these terms.
*Correspondence: Karine Thivierge, a2FyaW5lLnRoaXZpZXJnZUBpbnNwcS5xYy5jYQ==; Christopher Fernandez-Prada, Y2hyaXN0b3BoZXIuZmVybmFuZGV6LnByYWRhQHVtb250cmVhbC5jYQ==; Julie Arsenault, anVsaWUuYXJzZW5hdWx0QHVtb250cmVhbC5jYQ==
†These authors have contributed equally to this work and share first authorship