Chytridiomycosis Outbreak in a Chilean Giant Frog (Calyptocephalella gayi) Captive Breeding Program: Genomic Characterization and Pathological Findings
- 1Sustainability Research Centre & PhD Program in Conservation Medicine, Faculty of Life Sciences, Universidad Andres Bello, Santiago, Chile
- 2Institute of Zoology, Zoological Society of London, London, United Kingdom
- 3Núcleo de Ciencias Aplicadas en Ciencias Veterinarias y Agronómicas, Universidad de las Américas, Santiago, Chile
- 4Criadero y Centro de Exhibición de la Rana Chilena Calyptocephalella gayi, Santiago, Chile
- 5Department of Infectious Disease Epidemiology, MRC Centre for Global Infectious Disease Analysis, School of Public Health, Imperial College London, London, United Kingdom
- 6ONG Ranita de Darwin, Valdivia, Chile
- 7Instituto de Conservación, Biodiversidad y Territorio, Facultad de Ciencias Forestales y Recursos Naturales, Universidad Austral de Chile, Valdivia, Chile
Emerging infectious diseases in wildlife are increasingly associated with animal mortality and species declines, but their source and genetic characterization often remains elusive. Amphibian chytridiomycosis, caused by the fungus Batrachochytrium dendrobatidis (Bd), has been associated with catastrophic and well-documented amphibian population declines and extinctions at the global scale. We used histology and whole-genome sequencing to describe the lesions caused by, and the genetic variability of, two Bd isolates obtained from a mass mortality event in a captive population of the threatened Chilean giant frog (Calyptocephalella gayi). This was the first time an association between Bd and high mortality had been detected in this charismatic and declining frog species. Pathological examinations revealed that 30 dead metamorphosed frogs presented agnathia or brachygnathia, a condition that is reported for the first time in association with chytridiomycosis. Phylogenomic analyses revealed that Bd isolates (PA1 and PA2) from captive C. gayi group with other Bd isolates (AVS2, AVS4, and AVS7) forming a single highly supported Chilean Bd clade within the global panzootic lineage of Bd (BdGPL). These findings are important to inform the strengthening of biosecurity measures to prevent the impacts of chytridiomycosis in captive breeding programs elsewhere.
Introduction
Although amphibian enigmatic declines had been identified by herpetologists as early as the 1970s, they were only recognized two decades later as a global phenomenon that in some cases could not be explained by environmental changes or other expected anthropogenic factors alone (1–3). The discovery of the amphibian-killing fungus Batrachochytrium dendrobatidis [hereafter Bd (4, 5)] was a turning point in understanding why many amphibian species have been in steep decline. The emergence of Bd, which causes the lethal disease, amphibian chytridiomycosis, has been associated with amphibian population declines of more than 500 species, including the presumed extinction of at least 90 species (6). Evidence suggests that Bd recently spread across the globe from an endemic focus, with East Asia as the most likely source from where it expanded to other continents during the past century (7–10). Its global spread has been mainly facilitated by the international trade of amphibians, particularly the North American bullfrog (Lithobates catesbeianus), the most intensively farmed frog worldwide (11–15).
Novel genomic techniques, including whole-genome sequencing and multilocus sequence typing have shown the existence of at least five major lineages of Bd: BdGPL, BdCAPE, BdASIA-1 (including BdCH), BdASIA-2/BdBRAZIL and BdASIA-3 (9, 10, 16). Of these, the global panzootic lineage (BdGPL) is the most widespread variant of Bd and is responsible for most known cases of amphibian population declines due to chytridiomycosis (9). Although BdGPL is highly virulent, its impacts are context-dependent (17), and under some conditions other lineages may be responsible for lethal disease and population declines (18). Additionally, multiple introductions of Bd have led to different lineages of Bd coming into contact, resulting in the formation of interlineage recombinants (e.g., through the co-infection of amphibians) that may have higher pathogenicity or transmissibility (9, 10, 14, 16, 19, 20). For instance, interlineage recombinants have been reported for BdGPL with BdASIA-2/BdBRAZIL, and for BdGPL with BdCAPE (9, 10, 14, 19).
Despite South America being the region with the greatest loss of biodiversity due to Bd (6), only low numbers of Bd isolates from this region have been genetically characterized (14, 21–26). This limits our capacity to adequately understand the epidemiological processes that have led to impacts of chytridiomycosis on South American native amphibians. Our focus here, the Chilean giant frog (Calyptocephalella gayi), is endemic to Chile and is considered as a living fossil since its family represents an old neobatrachian clade that diverged during the Cretaceous around 100–120 Mya (27). With females reaching up to 2 kg, this is the second largest anuran species worldwide, which has led this species to be of economic interest as a food source (28). This highly aquatic species is considered Vulnerable by the IUCN Red List, and is threatened by overconsumption, habitat loss due to agriculture, and invasive species including several introduced fish and the African clawed frog [Xenopus laevis (29)]. Chytridiomycosis has been suspected to be a contributing factor in its steep decline (30), but to date, no evidence has been found linking Bd to lethal effects in C. gayi. Based on histology and whole-genome sequencing, the aim of this study is to describe the lesions of chytridiomycosis in C. gayi and the genetic characterization of Bd isolates obtained from a chytridiomycosis outbreak that occurred in a C. gayi captive breeding program in Chile. In addition, the genomics of Bd isolates from captive C. gayi together with previously obtained Chilean Bd isolates are compared with a global panel of Bd.
Materials and Methods
Chilean Giant Frog Captive Breeding Center
The Chilean giant frog captive breeding center (Resolution N°2358/2013 by the Chilean Agriculture and Livestock Service) in Santiago has been functioning since 2013, with its objective to generate reproductive knowledge and to support the conservation of C. gayi. The center was built in an area of 70 m2 and was composed of 10 large and 10 small tanks for tadpoles (100 and 30 L each, respectively), 10 small tanks for recently metamorphosed frogs (30 L) and 20 medium size tanks for adult frogs (50 L). Water used in tanks was from the mains supply but had been left to stand for 2 days to allow chlorine evaporation. Around 50% of water in the tanks was changed twice a week. Tadpoles were fed on spirulina algae supplemented with lettuce given ad libitum, while postmetamorphs and adults were fed twice a day with dried amphipod crustaceans (Orchistoidea spp.) supplemented with chicken protein, vitamins, and minerals. By August 2016, the captive breeding program comprised ~400 1-year-old tadpoles, six juveniles, and 86 breeding adults (43 females and 43 males).
Mortality and Pathological Analyses
In September 2016, the program received new individuals from a separate C. gayi captive breeding program that had been terminated. The newly incoming individuals consisted of 800 2-year-old tadpoles and 18 breeding adults (9 females, 9 males), which were maintained in separate tanks from the resident animals. By early November 2016, 40 of the new tadpoles completed metamorphosis. From December 2016 to January 2017, 75 of the new individuals died: 37 tadpoles, 35 postmetamorphs and three reproductive adults. All dead postmetamorphs had not consumed any food after metamorphosis, and some of the tadpoles were observed to be lethargic prior to death and exhibiting partial depigmentation of the mouthparts, a finding consistent with amphibian chytridiomycosis (31). Freshly dead animals (4 tadpoles and 29 postmetamorphs) were transported in refrigerated conditions to the laboratory for postmortem examination and Bd isolation. Necropsies of the 29 postmetamorphs were performed according to standard protocol (32). Tissue sections of postmetamorphs were collected in neutral-buffered 10% formaldehyde from any organ displaying gross lesions and from lung, liver, spleen, kidney, skeletal muscle, heart, skin, stomach, small and large intestines. For histopathological analyses, tissues were embedded in paraffin wax, sectioned (4–5 μm) and stained with hematoxylin and eosin (H&E).
Bd Sampling and qPCR Assay
Non-invasive skin swabs (MW100, Medical & Wire Equipment Co.) were obtained from postmetamorphic amphibians (n = 29) by firmly running the swabs five times each over the ventral abdomen and pelvis, each ventral hind limb (femur and tibia) and the plantar surface of each hind foot (33). Also, from dead tadpoles (n = 4), samples were obtained from the oral disc by rotating the swab 10 times around the mouth opening. Swabs were kept in a cool box until freezing at −80°C once back at the laboratory. Briefly, DNA extraction from skin and oral swabs and subsequent detection of Bd DNA using a specific real time qPCR assay was done following Soto-Azat et al. (34). For each sample, diagnostic assays were performed in duplicate, and standards of known zoospore concentration (obtained from a previous Bd culture) were included within each PCR plate as positive controls. We assumed that a Bd-positive swab was indicative of Bd infection. By including known concentrations of Bd DNA in serial diluted positive control wells on each PCR plate, we were able to quantify infection intensity, which we defined as the number of zoospore equivalents/swab (ZE). To quantify and correct the infection intensity per swab, each genomic value obtained from the qPCR assay was multiplied by 120 to account for sample dilution (35).
Bd Isolation
Freshly dead tadpoles with suspected Bd infection (n = 4) were used for Bd isolation following Longcore et al. (5) and Fisher et al. (36). Subsequent confirmation of Bd infection status and load by qPCR, served to guide Bd isolation efforts. Within 8 h. after death, the whole mouthparts of Bd-positive dead tadpoles were removed and sectioned into small pieces and deposited in a fungal growth TGhL medium (8 g. tryptone, 2 g. gelatin hydrolysate, 4 g. lactose, 10 g. agar). Cultured sections were first cleaned using an agar plate with antibiotics (200 mg/L penicillin-G and 400 mg/L streptomycin sulfate), and then placed singly into TGhL agar plate with antibiotics incubated at 15–20°C. Because zoospore release may occur immediately, especially from tadpole mouthparts, cultures were examined with an inverted microscope for the presence of active zoospores every day for up to 1 week. Once growth of zoospores and/or zoosporangia was observed, part of the agar was transferred to a new TGhL agar plate without antibiotics and incubated at 15–20°C up to 1 week. Isolates were then passaged no more than three times in order to lessen the chance of genomic change due to prolonged laboratory culture (37).
DNA Extraction, Sequence Library Preparation and Phylogenomic Analyses
We performed DNA extraction using the MasterPureTM Yeast DNA Purification Kit (Epicentre, Wisconsin, USA) from all obtained purified Bd cultures. DNA extractions were first quantified using a Tapestation 2200 (Agilent Technologies, California, USA) and Qubit 2.0 fluorimeter (Thermo Fisher Scientific, Massachusetts, USA), and then sequenced using an Illumina HiSeq 2000 (Illumina, California, USA). Subsequently, TruSeq Nano 350 gel-free sequencing libraries were prepared for 125 + 125 bp paired-end sequencing using Illumina HiSeq high output v4 chemistry, and sequencing reads cleaned of adapter sequences and quality trimmed using cutadapt v1.10 (38). Reads of the JEL423 reference genome (GenBank assembly accession: GCA_000149865.1) were mapped using Burrows-Wheeler Aligner v0.7.8 (39).
We processed the resulting sequence alignment map (SAM) files using SAMtools v1.3.1 with the “fixmate” and “sort” programs to read the files for variants discovery. We performed the variant detection in a two-step process using Freebayes version dbb6160 (40). First, sorted SAM files for each isolate in the phylogeny were independently called to find variant positions and merged into a single variant call format (VCF) file. Second, each of the samples was re-called using the positions in VCF file, to produce a squared-off call set (a genotype call was made at every locus for each isolate, including for missing data). All VCF files were processed using vcflib (41) to break complex variants into allelic primitives and vt (42) to normalize short insertion and deletion sequences. VCF files were then quality filtered using bcftools v1.3.1 (43) to accept only variants with sufficient supporting evidence. Potentially polymorphic sites were filtered using the settings in the bcbio.variation.recall squaring-off pipeline (44), with sites not passing these filters set to homozygous reference (there was not enough evidence to call a variant at that position). Then, the processed VCF files were merged into a single multisample VCF and extracted a FASTA file of the SNP variant calls. Phylogenomic analyses were conducted using RAxML v8.2.9 with GTRCAT model with 500 bootstrap runs. Weir and Cockerham's estimator was performed using a sliding-window comparison of FST of Chilean Bd isolates against a global panel of representative global diversity of Bd in vcftools. Single nucleotide polymorphisms (SNPs) that were in high linkage disequilibrium were pruned from the dataset using the SNPRelate package version 1.10.2 in R v3.4.0 (45). After pruning using a sliding-window based analysis and a linkage disequilibrium threshold of 0.125, 3,900 SNPs positions remained which were analyzed using SNPRelate and plotted with ggplot2 (46). Finally, the clustering of Chilean Bd isolates against a global panel of Bd was investigated using principal component analysis (PCA) with adegenet package (47) and plotted with ggplot2 (46).
Results
Pathology and Bd qPCR Assay
During December 2016 and January 2017, we observed a mass mortality event in a C. gayi captive breeding program, killing 87.5% (35/40) of metamorphosed frogs in the newly acquired group of animals. Of these, 30 individuals presented: jaw deformation (n = 21) or absence (n = 9) of oral structures, dying a few weeks after completing metamorphosis as they could not feed properly (Figure 1A). PCR Bd-positive samples were detected in 100% of sampled tadpoles, and postmetamorphic individuals (n = 33). The infection load in Bd-positive amphibians ranged from 95 to 147,366 ZE (median = 9,842). Of the total number of infected frogs, 33.3% (11/33) had more than 10,000 ZE. At gross necropsy a distended gallbladder (with bile) and the absence of gastrointestinal content was observed in all individuals with jaw absence/deformation (30/35). We confirmed Bd infection microscopically, as we observed hyperkeratosis within the superficial layer of the skin with distinct stages of developing zoosporangia that are morphologically typical of Bd (Figure 1B). No other macroscopic or microscopic findings were observed in the other analyzed tissues.
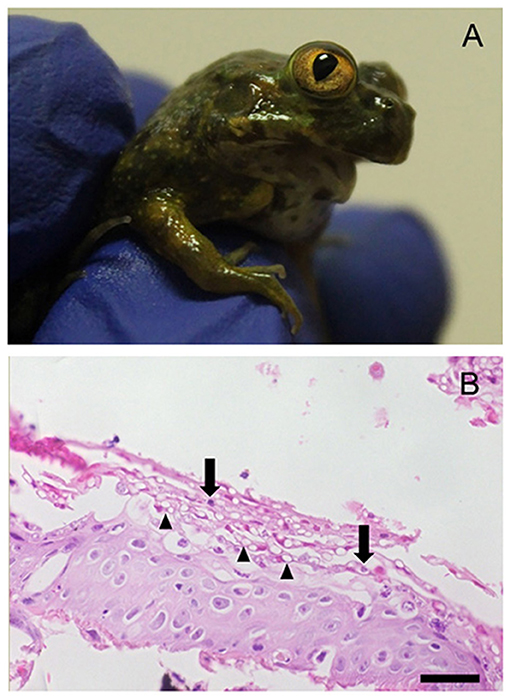
Figure 1. A Chilean giant frog (Calyptocephalella gayi) postmetamorph showing signs of disease. (A) Absence of jaw (agnathia). (B) Histological section of hind limb skin. Note distinct stages of developing zoosporangia (arrows) and multiple empty spaces (arrowheads) within the superficial keratinized layer, morphologically typical of Batrachochytrium dendrobatidis infection. Stained with hematoxylin and eosin. Bar = 24 μm (bottom).
Bd Isolation and Phylogenomic Analyses
From our attempts to culture Bd from four freshly dead C. gayi tadpoles, we obtained two isolates (PA1 and PA2, WGS read data available at the NCBI Sequence Read Archive: https://www.ncbi.nlm.nih.gov/sra under accession numbersSRS8215364 and SRS8216816, respectively). Phylogenomic analyses showed that our C. gayi isolates grouped within BdGPL forming a single and highly supported clade (100% bootstrap support; Figure 2). The Bd isolates were fixed for 99.4% of the segregating sites that were observed in BdGPL after filtering for missing positions. There were only 2,257 variable sites exclusive to the C. gayi Bd isolates. Although we compared the genomes of C. gayi isolates (PA1 and PA2) with an extensive global panel of Bd, they were shown to be highly divergent from the only known regional potentially endemic lineage in South America (BdAsia2/BdBrazil). Within BdGPL, the Bd isolates from captive C. gayi clustered with other isolates obtained from wild amphibians in Chile (AVS2 from Batrachyla antartandica, AVS4 from Xenopus laevis and AVS7 from C. gayi) and an isolate from the UK (UKTvB), collected from a smooth newt (Lissotriton vulgaris) in 2009 in Kent, United Kingdom, to form a well-supported clade (100% bootstrap support; Figure 2).
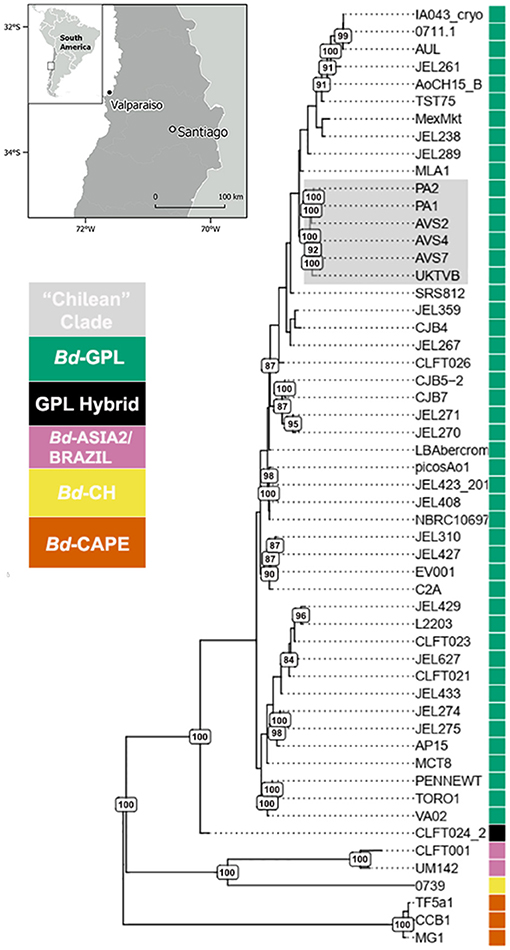
Figure 2. Global phylogeny of 54 isolates of Batrachochytrium dendrobatidis (Bd) based on 363,497 segregating sites obtained by whole genome sequencing. The clade containing the Bd isolates PA1 and PA2 from captive C. gayi grouping with other Bd isolates from Chile and the United Kingdom is highlighted in gray. The branches of the tree are weighted (thickness) by bootstrap support (500 replicates), with branches with 80% of support and above labeled.
We used the Weir and Cockerham's estimator to perform a sliding-window comparison of FST of Bd isolates (PA1 and PA1) against all the other BdGPL isolates. In this analysis, we identified several stretches of genome where the FST estimator was more than two standard deviations greater than the mean of all FST values, indicating differentiation due to positive selection or reduced rates of recombination (Figure 3). Finally, we analyzed isolate clustering using PCA on a filtered subset of 3,900 SNPs in linkage equilibrium, revealing an overall population structure that is consistent with our phylogenetic analyses (Figure 4).
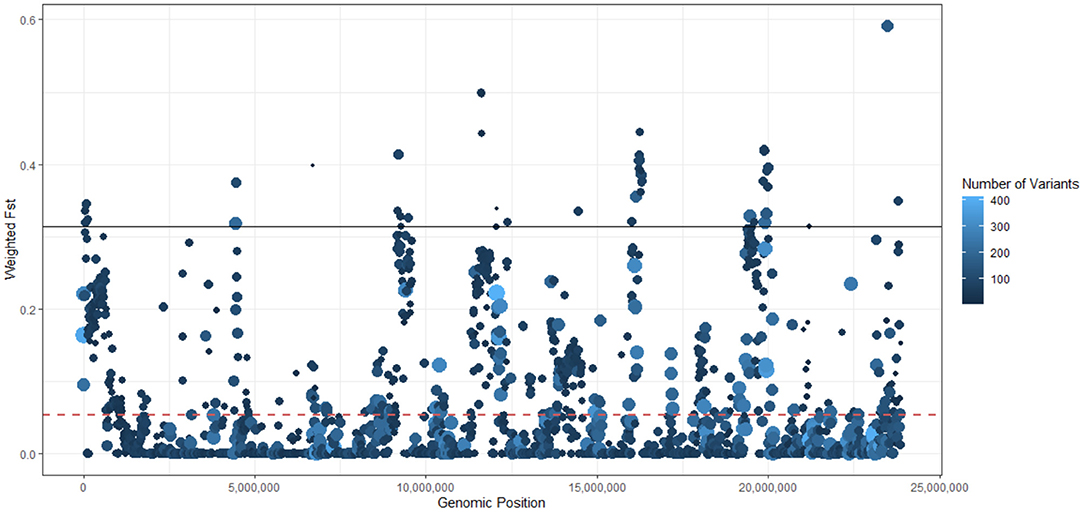
Figure 3. Sliding window analysis of population differentiation of Batrachochytrium dendrobatidis (Bd) isolates (PA1 and PA2) against other 45 global panzootic lineage of Bd (BdGPL) isolates using Weir and Cockerham's FST estimator. Each point represents a 10 Kb genomic window, with a 5 kb step-size. The dashed red line represents the mean FST (0.0538). The solid black line represents the 95% quantile threshold of the FST estimator (0.3141). Each point is sized and colored on a log scale by the number of variants in each window. The legend indicates the color scale (the number of SNPs included in each window varied from 1 to 364, with a median of 31). Point size from small to large is scaled from low to high numbers of variants.
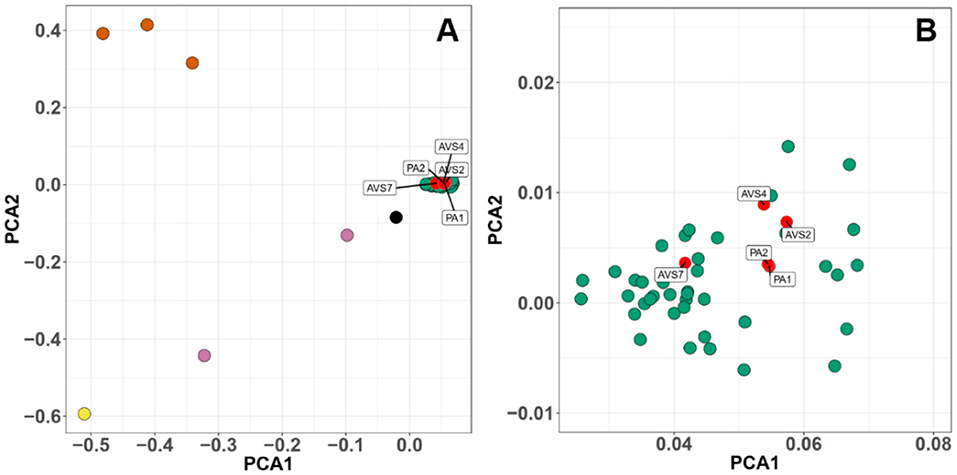
Figure 4. Principal components analysis (PCA) of 3,900 SNPs in linkage equilibrium of a global panel of 54 Batrachochytrium dendrobatidis (Bd) isolates. Each point represents an isolate, colored by phylogenetic lineage. (A) Bd isolates separate into clearly defined clusters. (B) Higher resolution of the global panzootic lineage of Bd (BdGPL) cluster showing the position of the Chilean isolates within the cluster. The axes plot the first and second principal components, PCA1 and PCA2. Chilean isolates are clustered within BdGPL. Chilean isolates (red), other BdGPL (green), BdASIA2/BdBRAZIL (pink), hybrids between the previous two groups (black), BdCH (yellow), BdCAPE (orange).
Discussion
The now globalized Bd has caused the greatest loss of biodiversity known due to a single pathogen (6). The impacts of chytridiomycosis have likely been underestimated, as the affected amphibian species are often difficult to study, particularly in endangered cryptic species that occur in remote locations (48). In addition, not all Bd lineages have the same impact on infected amphibian populations and species, therefore a better understanding of the genetic diversity of Bd is critical to understanding the risk presented by this pathogen and to informing mitigation actions (16). In this study, we describe a mass mortality event due to chytridiomycosis in an endangered species of amphibian in a captive breeding program. We genetically characterized two Bd isolates from this outbreak, showing that they nested within the BdGPL clade and were highly related to Bd genotypes previously isolated from wild amphibians in Chile.
The presence of agnathia and brachygnathia associated with Bd infection in postmetamorphic amphibians has not been reported before. Tadpole oral malformations have been associated with low temperatures (49), water contamination (50), nutrition (51), or ecological factors (52, 53). Although, this malformation might have been due to an unknown environmental or other cause, it is likely associated with Bd infection of the oral discs of tadpoles (54–56). Absence or reduction in development of the lower jaw may have had a profound impact on the ability of postmetamorphic amphibians (and tadpoles) to acquire food, contributing to death along with chytridiomycosis. The presence of distended gall bladders and the absence of gastrointestinal content in all the animals examined suggests a lack of feeding. Although oral deformations in postmetamorphic amphibians have not been used before as an indication of chytridiomycosis, they might be still important and may indicate an unknown sequela of Bd infection that may have been overlooked previously. This study highlights the need to use accurate diagnostic techniques such as qPCR or histology to be able to complement these observations (57).
Captive breeding has increasingly been used as a tool for amphibian conservation, but for these initiatives to be successful, several aspects must be considered, including genetic management and biosecurity protocols (58, 59). In our case, newly admitted individuals of C. gayi came from a semi-open captive breeding program that was supplied with water from an agricultural canal, in which X. laevis had previously been recorded (34). Despite the implementation of quarantine, this was not enough to prevent the introduction of Bd to the captive breeding program, causing mortality in the newly admitted animals. This highlights the importance of implementing strict biosecurity protocols against Bd (and other pathogens), such as Bd testing prior to admittance, preventive antifungal treatment or disinfection of water and materials (59).
Susceptibility to Bd is also influenced by environmental factors, such as climate (20). Immune function in amphibians is closely dependent on environmental temperature (60). For instance, low temperature has been associated with a lower survival in Bd-exposed frogs under laboratory conditions (60, 61) and chytridiomycosis die-offs have been often associated with higher elevation, lower temperature and winter season (62–64). It is possible that in our case, stress associated with transportation might have induced immunosuppression, facilitating the development of chytridiomycosis. Ramsey et al. (65) demonstrated that natural resistance to Bd in X. laevis can be reversed with the implementation of sub-lethal immunosuppressive treatments, such as exposure to X-irradiation or norepinephrine injections.
The Bd lineage that we isolated from infected individuals in this chytridiomycosis outbreak, BdGPL, has been associated with catastrophic mass mortalities and population declines in multiple continents (22, 48, 66, 67). Over the last four decades, a severe population decline of two species of Darwin's frogs (Rhinoderma darwinii and R. rufum) populations has occurred across central and south Chile. Retrospective and cross-sectional Bd studies and population monitoring data suggest that chytridiomycosis has contributed to these extirpations (33, 48) and that Bd has been present in Chile at least since 1970 (33). Although characterization of Bd isolates infecting R. darwinii has not been achieved so far, the identification of BdGPL over a large area of Chile, provides support to the hypothesis that BdGPL is causing these declines (25).
In our phylogenomic whole-genome analysis, all the Chilean Bd isolates (including the two isolates from captive C. gayi) grouped together with a genotype isolated in 2009 from the United Kingdom (UKTvB). A similar phylogenetic relationship was observed when restricting the analysis to a subset of the genome spanning a heterozygosity loss event shared by all BdGPL isolates, but in this case, isolates from other European countries and a Canadian isolate also group with the Chilean isolates (25). While there has been no report of mortality in the wild caused by UKTvB, a challenge with this isolate in the Mallorcan midwife toad (Alytes muletensis) under laboratory conditions caused a 73% mortality rate (18). It is likely, therefore, that the BdGPL genotypes in Chile are virulent and have caused amphibian mortalities in nature (25). However, detecting mortalities in the wild is often difficult, particularly in cryptic species, for which better surveillance is needed. In addition, we also evaluated the presence of Frog virus 3 (FV3) ranavirus in the same individuals as a possible cause of mortality, however all tested negative using a qPCR assay. Previously, a mass mortality of adult C. gayi, allegedly due to a drought, was described in central Chile, however whether Bd was involved in this mortality event is unknown as no fresh carcasses were available for necropsy, Bd detection or other diagnostics to be performed (68).
The low number of segregating sites exclusive to the Chilean Bd isolates, compared to the total number of sites where the BdGPL isolates are polymorphic, suggests a single and recent introduction of Bd into Chile, possibly through the international movement of amphibians, other aquatic animals, fomites, or tourism (9, 14, 25, 66). Molecular characterization of further isolates from non-surveyed areas in Chile and neighboring countries (e.g., Argentina and Peru), along with the calibration of a genome-wide molecular clock, is required to confirm this hypothesis. The existence of an apparently unique and recently introduced lineage of Bd in Chile differs with the known history of this pathogen in Brazil, where both BdGPL and BdAsia2/BdBrazil coexist, with evidence of multiple interlineage recombination events between them (9, 24). Therefore, together with the potential introduction of novel Bd genotypes, interlineage recombination can potentially arise facilitated by the globalization of human and animal transport (16). This highlights the importance of biosecurity measures at the national and local level, to prevent the introduction and establishment of further pathogenic Bd lineages, as this pathogen has the capability to increase its genomic diversity through the exchange of haplotypes among lineages (25).
Our work describes for the first time a mass mortality event in the endangered giant Chilean frog from a captive breeding program. We also provide new data on the potential susceptibility of C. gayi to the impacts of chytridiomycosis, a species that has been declining fast across its distribution in Chile. The high mortality observed in C. gayi with postmetamorphs exhibiting agnathia or brachygnathia as a possible consequence of oral infection with Bd in tadpoles has not been described previously and is a condition that can be considered in the monitoring of amphibians maintained in captivity, such as farms, zoos and ex situ conservation programs. We described two new isolates of Bd in Chile, belonging to BdGPL and clustering in a single group with another three previously isolated Bd isolates from central and south Chile (9, 25), for which evidence as a cause of amphibian mortality and population declines is growing.
Data Availability Statement
The datasets presented in this study can be found in online repositories. The names of the repository/repositories and accession number(s) can be found at: NCBI SRA; SRS8215364 and SRS8216816.
Ethics Statement
The animal study was reviewed and approved by Bioethics Committee Universidad Andres Bello and by the Zoological Society of London's Ethics Committee.
Author Contributions
MA-R and CA led the manuscript writing. MA-R, PA, and AP-R collected the data. MA-R and AP-R completed the PCR analysis, post-mortem investigation, and Bd isolation. TS, SO'H, and MF competed the whole genome analyses. AV-S, AC, and CA supported data analyses and pathological research. All authors contributed to the manuscript.
Funding
This study was funded by FONDECYT Regular number 1211587 (to CA), and the Dirección General de Investigación y Doctorados, Universidad Andres Bello, grant N ° DI-582-14/I (to MA-R). TS and MF were supported by a grant from Natural Environmental Research Council (NERC; NE/S000844/1) and the UK Medical Research Council (MRC; MR/R015600/1). MF is a fellow in the CIFAR Fungal Kingdoms program.
Conflict of Interest
The authors declare that the research was conducted in the absence of any commercial or financial relationships that could be construed as a potential conflict of interest.
Publisher's Note
All claims expressed in this article are solely those of the authors and do not necessarily represent those of their affiliated organizations, or those of the publisher, the editors and the reviewers. Any product that may be evaluated in this article, or claim that may be made by its manufacturer, is not guaranteed or endorsed by the publisher.
References
1. Blaustein AR, Wake DB. Declining amphibian populations: a global phenomenon? Trends Ecol. Evol. (1990) 5:203–4. doi: 10.1016/0169-5347(90)90129-2
2. Stuart SN, Chanson JS, Cox NA, Young BE, Rodrigues A, Fishman D, et al. Status and Trends of amphibian declines and extinctions worldwide. Science. (2004) 306:1783–6. doi: 10.1126/science.1103538
3. Collins JP, Crump ML. Extinction in Our Times: Global Amphibian Decline. New York, NY: Oxford University Press (2009).
4. Berger L, Speare R, Daszak P, Green DE, Cunningham AA, Goggin CL, et al. Chytridiomycosis causes amphibian mortality associated with population declines in the rain forests of Australia and Central America. PNAS. (1998) 95:9031–6. doi: 10.1073/pnas.95.15.9031
5. Longcore JE, Pessier AP, Nichols DK. Batrachochytrium dendrobatidis gen. et sp nov, a chytrid pathogenic to amphibians. Mycologia. (1999) 91:219–27. doi: 10.1080/00275514.1999.12061011
6. Scheele BC, Pasmans F, Skerratt LF, Berger L, Martel A, Beukema W, et al. Amphibian fungal panzootic causes catastrophic and ongoing loss of biodiversity. Science. (2019) 363:1459–63. doi: 10.1126/science.aav0379
7. Goka K, Yokoyama JUN, Une Y, Kuroki T, Suzuki K, Nakahara M, et al. Amphibian chytridiomycosis in Japan: distribution, haplotypes and possible route of entry into Japan. Mol Ecol. (2009) 18:4757–74. doi: 10.1111/j.1365-294X.2009.04384.x
8. Bataille A, Fong JJ, Cha M, Wogan GO, Baek HJ, Lee H, et al. Genetic evidence for a high diversity and wide distribution of endemic strains of the pathogenic chytrid fungus Batrachochytrium dendrobatidis in wild Asian amphibians. Mol Ecol. (2013) 22:4196–209. doi: 10.1111/mec.12385
9. O'hanlon S, Rieux A, Farrer RA, Rosa GM, Waldman B, Bataille A, et al. Recent Asian origin of chytrid fungi causing global amphibian declines. Science. (2018) 360:621–7. doi: 10.1126/science.aar1965
10. Byrne AQ, Vredenburg VT, Martel AN, Pasmans F, Bell RC, Blackburn DC, et al. Cryptic diversity of a widespread global pathogen reveals expanded threats to amphibian conservation. PNAS. (2019) 116:20382–7. doi: 10.1073/pnas.1908289116
11. Garner TW, Perkins MW, Govindarajulu P, Seglie D, Walker S, Cunningham A, et al. The emerging amphibian pathogen Batrachochytrium dendrobatidis globally infects introduced populations of the North American bullfrog, Rana catesbeiana. Biol Lett. (2006) 2:455–9. doi: 10.1098/rsbl.2006.0494
12. Fisher MC, Garner TW. The relationship between the emergence of Batrachochytrium dendrobatidis, the international trade in amphibians and introduced amphibian species. Fungal Biol Rev. (2007) 21:2–98. doi: 10.1016/j.fbr.2007.02.002
13. Schloegel LM, Picco AM, Kilpatrick AM, Davies AJ, Hyatt AD, Daszak P. Magnitude of the US trade in amphibians and presence of Batrachochytrium dendrobatidis and ranavirus infection in imported North American bullfrogs (Rana catesbeiana). Biol Conserv. (2009) 142:1420–6. doi: 10.1016/j.biocon.2009.02.007
14. Schloegel LM, Toledo LF, Longcore JE, Greenspan SE, Vieira CA, Lee M, et al. Novel, panzootic and hybrid genotypes of amphibia chytridiomycosis associated with the bullfrog trade. Mol Ecol. (2012) 21:5162–77. doi: 10.1111/j.1365-294X.2012.05710.x
15. Kolby JE, Smith KM, Berger L, Karesh WB, Preston A, Pessier A, et al. First evidence of amphibian chytrid fungus (Batrachochytrium dendrobatidis) and Ranavirus in Hong Kong amphibian trade. PLoS ONE. (2014) 9:e90750. doi: 10.1371/journal.pone.0090750
16. Azat C. Not just a pathogen: the importance of recognizing genetic variability to mitigate a wildlife pandemic. Mol Ecol Resour. (2021) 21:1410–2. doi: 10.1111/1755-0998.13348
17. Bates KA, Clare FC, O'Hanlon S, Bosch J, Brookes L, Hopkins K, et al. Amphibian chytridiomycosis outbreak dynamics are linked with host skin bacterial community structure. Nat Commun. (2018) 9:693. doi: 10.1038/s41467-018-02967-w
18. Doddington BJ, Bosch J, Oliver JA, Grassly NC, Garcia G, Schmidt BR, et al. Context-dependent amphibian host population response to an invading pathogen. Ecology. (2013) 94:1795–804. doi: 10.1890/12-1270.1
19. Greenspan SE, Lambertini C, Carvalho T, James TY, Toledo LF, Haddad CFB, et al. Hybrids of amphibian chytrid show high virulence in native hosts. Sci Rep. (2018) 8:1–10. doi: 10.1038/s41598-018-27828-w
20. Fisher MC, Garner TW. Chytrid fungi and global amphibian declines. Nat Rev Microbiol. (2020) 18:332–43. doi: 10.1038/s41579-020-0335-x
21. Flechas SV, Medina EM, Crawford AJ, Sarmiento C, Cárdenas ME, Amézquita A, et al. Characterization of the first Batrachochytrium dendrobatidis isolate from the Colombian Andes, an amphibian biodiversity hotspot. Ecohealth. (2013) 10:72–6. doi: 10.1007/s10393-013-0823-9
22. Rosenblum EB, James TY, Zamudio KR, Poorten TJ, Ilut D, Rodriguez D, et al. Complex history of the amphibian-killing chytrid fungus revealed with genome resequencing data. PNAS. (2013) 110:9385–90. doi: 10.1073/pnas.1300130110
23. James T, Toledo F, Rödder D, da Silva D, Belasen A, Betancourt-Roman C, et al. Disentangling host, pathogen, and environmental determinants of a recently emerged wildlife disease: lessons from the first 15 years of amphibian chytridiomycosis research. Ecol Evol. (2015) 5:4079–97. doi: 10.1002/ece3.1672
24. Jenkinson TS, Betancourt CM, Lambertini C, Valencia-Aguilar A, Rodriguez D, Nunes-de-Almeida CH, et al. Amphibian-killing chytrid in Brazil comprises both locally endemic and globally expanding populations. Mol Ecol. (2016) 25:2978–96. doi: 10.1111/mec.13599
25. Valenzuela-Sánchez A, O'Hanlon SJ, Alvarado-Rybak M, Uribe-Rivera DE, Cunningham AA, Fisher MC, et al. Genomic epidemiology of the emerging pathogen Batrachochytrium dendrobatidis from native and invasive amphibian species in Chile. Transbound Emerg Dis. (2018) 65:309–14. doi: 10.1111/tbed.12775
26. Burrowes PA, James TY, Jenkinson TS, De la Riva I. Genetic analysis of post-epizootic amphibian chytrid strains in Bolivia: adding a piece to the puzzle. Transb Emerg Dis. (2020) 67:2163–71. doi: 10.1111/tbed.13568
27. Feng Y-J, Blackburn DC, Liang D, Hillis DM, Wake DB, Cannatella DC, et al. Phylogenomics reveals rapid, simultaneous diversification of three major clades of Gondwanan frogs at the Cretaceous-Paleogene boundary. PNAS. (2017). 114:E5864E5870. doi: 10.1073/pnas.1704632114
28. Vélez CM. Manejo en cautiverio de la Rana Grande Chilena Capytocephalella gayi (Duméril and Bibron, 1841). Santiago: Ediciones Universidad Santo Tomás (2014).
29. IUCNSSC Amphibian Specialist Group. Calyptocephalella gayi. The IUCN Red List of Threatened Species 2019:e.T4055A85633603. Downloaded on 21 May 2021 (2019).
30. Bacigalupe LD, Soto-Azat C, García-Vera C, Barría-Oyarzo I, Rezende EL. Effects of amphibian phylogeny, climate and human impact on the occurrence of the amphibian-killing chytrid fungus. Glob Change Biol. (2017) 23:3543–53. doi: 10.1111/gcb.13610
31. Knapp RA, Morgan JAT. Tadpole mouthpart depigmentation as an accurate indicator of chytridiomycosis, an emerging disease of amphibians. Copeia. (2006) 2:188–97. doi: 10.1643/0045-8511(2006)6[188:TMDAAA]2.0.CO;2
32. Pessier AP, Pinkerton M. Practical gross necropsy of amphibians. Semin Avian Exot Pet Med. (2003) 12:81–8. doi: 10.1053/saep.2003.127884
33. Soto-Azat C, Valenzuela-Sánchez A, Clarke BT, Busse K, Ortiz JC, Barrientos C, et al. Is chytridiomycosis driving Darwin's frogs to extinction? PLoS ONE. (2013) 8:e79862. doi: 10.1371/journal.pone.0079862
34. Soto-Azat C, Peñafiel-Ricaurte A, Price SJ, Sallaberry-Pincheira N, García MP, Alvarado-Rybak M, et al. Xenopus laevis and emerging amphibian pathogens in Chile. Ecohealth. (2016) 13:775. doi: 10.1007/s10393-016-1186-9
35. Hudson M, Young R, Jackson J, Orozco-terWengel P, Martin L, James A, et al. Dynamics and genetics of a disease-driven species decline to near extinction: lessons for conservation. Sci Rep. (2016) 6:30772. doi: 10.1038/srep30772
36. Fisher M, Ghosh P, Shelton J, Bates K, Brookes L, Wierzbicki C, et al. Development and worldwide use of non-lethal, and minimal population-level impact, protocols for the isolation of amphibian chytrid fungi. Sci Rep. (2018) 8:7772. doi: 10.1038/s41598-018-24472-2
37. Voyles J, Johnson LR, Briggs CJ, Cashins SD, Alford RA, Berger L, et al. Experimental evolution alters the rate and temporal pattern of population growth in Batrachochytrium dendrobatidis, a lethal fungal pathogen of amphibians. Ecol Evol. (2014) 4:3633–41. doi: 10.1002/ece3.1199
38. Martin M. Cutadapt removes adapter sequences from highthroughput sequencing reads. EMBnet J. (2011) 17:10–2. doi: 10.14806/ej.17.1.200
39. Li H, Durbin R. Fast and accurate short read alignment with Burrows-Wheeler Transform. Bioinformatics. (2009) 25:1754–60. doi: 10.1093/bioinformatics/btp324
40. Garrison E, Marth G. Haplotype-based variant detection from short-read sequencing. arXiv. 1207.3907v2 [q-bio.GN]. (2012).
41. Garrison E. vcflib: A C++ Library for Parsing and Manipulating VCF Files. Retrieved from: https://github.com/vcflib/vcflib (accessed November 12, 2016) (2016).
42. Tan A, Abecasis GR, Kang HM. Unified representation of genetic variants. Bioinformatics. (2015) 31:2202–4. doi: 10.1093/bioinformatics/btv112
43. Li H, Handsaker B, Wysoker A, Fennell T, Rua J, Homer N, et al. The sequence alignment/map format and SAMtools. Bioinformatics. (2009) 25:1–2. doi: 10.1093/bioinformatics/btp352
44. Chapman B. v0.1.7: Avoid FreeBayes Error. Retrieved from: https://github.com/chapmanb/bcbio.variation.recall/blob/master/src/bcbio/variation/recall/square (accessed November 12, 2016) (2016).
45. Zheng X, Levine D, Shen J, Gogarten S, Laurie C, Weir B. A high-performance computing toolset for relatedness and principal component analysis of SNP data. Bioinformatics. (2012) 28:3326–8. doi: 10.1093/bioinformatics/bts606
47. Jombart T. Adegenet: a R package for the multivariate analysis of genetic markers. Bioinformatics. (2008) 24:1403–5. doi: 10.1093/bioinformatics/btn129
48. Valenzuela-Sánchez A, Schmidt BR, Uribe-Rivera DE, Costas F, Cunningham AA, Soto-Azat C. Cryptic disease-induced mortality may cause host extinction in an apparently stable host–parasite system. Proc Roy Soc B. (2017) 284:20171176. doi: 10.1098/rspb.2017.1176
49. Rachowicz LJ. Mouthpart pigmentation in Rana muscosa tadpoles: seasonal changes without chytridiomycosis. Herpetol Rev. (2002) 33:263–5.
50. Rowe CL, Kinney OM, Congdon JD. Oral deformities in tadpoles of the bullfrog (Rana catesbeiana) caused by conditions in a polluted habitat. Copeia. (1998) 1:244–6. doi: 10.2307/1447729
51. McDiarmid RW, Altig R. Tadpoles: The Biology of Anuran Larvae. Chicago, IL: The University of Chicago Press (1999).
52. Brett MA, Gouchie GM, Wassersug RJ. Can visual stimulation alone induce phenotypically plastic responses in Rana sylvatica tadpole oral structures? J. Herpetol. (2009) 43:165–8. doi: 10.1670/07-197R3.1
53. Relyea RA, Auld JR. Predator and competitor induced plasticity: how changes in foraging morphology affect phenotypic trade-offs. Ecology. (2005) 86:1723–9. doi: 10.1890/04-1920
54. Drake DL, Altig R, Grace JB, Walls SC. Occurrence of oral deformities in larval anurans. Copeia. (2007) 2007:449–58. doi: 10.1643/0045-8511(2007)7[449:OOODIL]2.0.CO;2
55. Venesky MD, Parris MJ, Storfer A. Impacts of Batrachochytrium dendrobatidis infection on tadpole foraging performance. Ecohealth. (2009) 6:565–75. doi: 10.1007/s10393-009-0272-7
56. Patel YA, Cavin JN, Moore MK. Morphological anomalies as indicators of chytrid infection in Bufo marinus from Trinidad, West Indies. Bios. (2012) 83:75–80. doi: 10.1893/0005-3155-83.3.75
57. Navarro-Lozano A, Sánchez-Domene D, Rossa-Feres DC, Bosch J, Sawaya RJ. Are oral deformities in tadpoles accurate indicators of anuran chytridiomycosis?. PloS ONE. (2018) 13:e0190955. doi: 10.1371/journal.pone.0190955
58. Harding G, Griffiths RA, Pavajeau L. Developments in amphibian captive breeding and reintroduction programs. Conserv Biol. (2016) 30:340–9. doi: 10.1111/cobi.12612
59. Linhoff LJ, Soorae PS, Harding G, Donnelly MA, Germano JM, Hunter DA, et al. IUCN Guidelines for Amphibian Reintroductions and Other Conservation Translocations. 1st ed. Gland: IUCN (2021).
60. Murphy PJ, St-Hilaire S, Corn PS. Temperature, hydric environment, and prior pathogen exposure alter the experimental severity of chytridiomycosis in boreal toads. Dis Aquat Organ. (2011) 95:31–42. doi: 10.3354/dao02336
61. Andre SE, Parker J, Briggs CJ. Effect of temperature on host response to Batrachochytrium dendrobatidis infection in the mountain yellow-legged frog (Rana muscosa). J Wildl Dis. (2008) 44:716–20. doi: 10.7589/0090-3558-44.3.716
62. Berger L, Speare R, Hines H, Marantelli G, Hyatt AD, Skerratt LF, et al. Effect of season and temperature on mortality in amphibians due to chytridiomycosis. Aust Vet J. (2004) 82:31–6. doi: 10.1111/j.1751-0813.2004.tb11137.x
63. Kriger KM, Pereoglou F, Hero JM. Latitudinal variation in the prevalence and intensity of chytrid (Batrachochytrium dendrobatidis) infection in eastern Australia. Conserv Biol. (2007) 21:1280–90. doi: 10.1111/j.1523-1739.2007.00777.x
64. Savage AE, Sredl MJ, Zamudio KR. Disease dynamics vary spatially and temporally in a North American amphibian. Biol Conserv. (2011) 144:1910–5. doi: 10.1016/j.biocon.2011.03.018
65. Ramsey JP, Reinert LK, Harper LK, Woodhams DC, Rollins-Smith LA. Immune defenses against Batrachochytrium dendrobatidis, a fungus linked to global amphibian declines, in the South African clawed frog, Xenopus laevis. Infect Immun. (2010) 78:3981–92. doi: 10.1128/IAI.00402-10
66. Farrer RA, Weinert LA, Bielby J, Garner TWJ, Balloux F, Clare F, et al. Multiple emergences of genetically diverse amphibian-infecting chytrids include a globalized hypervirulent recombinant lineage. PNAS. (2011) 108:18732–6. doi: 10.1073/pnas.1111915108
67. Vredenburg VT, Knapp RA, Tunstall TS, Briggs CJ. Dynamics of an emerging disease drive large-scale amphibian population extinctions. PNAS. (2010) 107:9689–94. doi: 10.1073/pnas.0914111107
Keywords: agnathia, amphibians, Batrachochytrium dendrobatidis, BdGPL, brachygnathia, Chile, emerging infectious disease, whole-genome sequencing
Citation: Alvarado-Rybak M, Acuña P, Peñafiel-Ricaurte A, Sewell TR, O'Hanlon SJ, Fisher MC, Valenzuela-Sánchez A, Cunningham AA and Azat C (2021) Chytridiomycosis Outbreak in a Chilean Giant Frog (Calyptocephalella gayi) Captive Breeding Program: Genomic Characterization and Pathological Findings. Front. Vet. Sci. 8:733357. doi: 10.3389/fvets.2021.733357
Received: 30 June 2021; Accepted: 27 August 2021;
Published: 24 September 2021.
Edited by:
Amanda Linda Jean Duffus, Gordon State College, United StatesReviewed by:
Rebecca Hardman, The University of Tennessee, Knoxville, United StatesAn Martel, Ghent University, Belgium
Copyright © 2021 Alvarado-Rybak, Acuña, Peñafiel-Ricaurte, Sewell, O'Hanlon, Fisher, Valenzuela-Sánchez, Cunningham and Azat. This is an open-access article distributed under the terms of the Creative Commons Attribution License (CC BY). The use, distribution or reproduction in other forums is permitted, provided the original author(s) and the copyright owner(s) are credited and that the original publication in this journal is cited, in accordance with accepted academic practice. No use, distribution or reproduction is permitted which does not comply with these terms.
*Correspondence: Mario Alvarado-Rybak, maalry@gmail.com; Claudio Azat, claudio.azat@unab.cl