- 1Teagasc Animal and Grassland Research and Innovation Centre, Cork, Ireland
- 2School of Natural and Environmental Sciences, Newcastle University, Newcastle upon Tyne, United Kingdom
- 3Adaptation Physiology Group, Department of Animal Sciences, Wageningen University & Research, Wageningen, Netherlands
- 4ONIRIS, Nantes, France
- 5Biotechnical Faculty, Department of Animal Science, University of Ljubljana, Ljubljana, Slovenia
- 6Chamber of Agriculture of North Rhine-Westphalia, Animal Health Services, Bad Sassendorf, Germany
- 7Faculty of Veterinary Medicine, Department of Paraclinical Sciences, Norwegian University of Life Sciences, Oslo, Norway
- 8Faculty of Veterinary Medicine, Trakia University, Stara Zagora, Bulgaria
- 9Ellinikos Georgikos Organismos-DIMITRA (ELGO-DIMITRA), Veterinary Research Institute, Thessaloniki, Greece
- 10Department of Production Animal Medicine, Research Centre for Animal Welfare, University of Helsinki, Helsinki, Finland
Damaging behaviors (DB) such as tail and ear biting are prevalent in pig production and reduce welfare and performance. Anecdotal reports suggest that health challenges increase the risk of tail-biting. The prevalence of tail damage and health problems show high correlations across batches within and between farms. There are many common risk factors for tail-biting and health problems, notably respiratory, enteric and locomotory diseases. These include suboptimal thermal climate, hygiene, stocking density and feed quality. The prevalence of tail damage and health problems also show high correlations across batches within and between farms. However, limited evidence supports two likely causal mechanisms for a direct link between DB and health problems. The first is that generalized poor health (e.g., enzootic pneumonia) on farm poses an increased risk of pigs performing DB. Recent studies indicate a possible causal link between an experimental inflammation and an increase in DB, and suggest a link between cytokines and tail-biting. The negative effects of poor health on the ingestion and processing of nutrients means that immune-stimulated pigs may develop specific nutrient deficiencies, increasing DB. The second causal mechanism involves tail-biting causing poor health. Indirectly, pathogens enter the body via the tail lesion and once infected, systemic spread of infection may occur. This occurs mainly via the venous route targeting the lungs, and to a lesser extent via cerebrospinal fluid and the lymphatic system. In carcasses with tail lesions, there is an increase in lung lesions, abscessation, arthritis and osteomyelitis. There is also evidence for the direct spread of pathogens between biters and victims. In summary, the literature supports the association between poor health and DB, particularly tail-biting. However, there is insufficient evidence to confirm causality in either direction. Nevertheless, the limited evidence is compelling enough to suggest that improvements to management and housing to enhance pig health will reduce DB. In the same way, improvements to housing and management designed to address DB, are likely to result in benefits to pig health. While most of the available literature relates to tail-biting, we suggest that similar mechanisms are responsible for links between health and other DB.
Introduction
The health and welfare of intensively farmed pigs is an important topic of research for decades. Currently the drivers for work in this area include (1) the threat of antimicrobial resistance and the associated mis/overuse of antibiotics in animal production, (2) unprecedented levels of societal concern and interest in animal production practices (3) and the related renewed impetus to implement legislation on animal welfare in the EU [e.g., on tail docking—(1)]. These share a cross cutting theme of the interplay between animal health and animal welfare, which is a growing area of research interest [e.g., (2)]. In this review animal health is defined as the absence of disease in the main physiological (i.e., respiratory, enteric and locomotory) systems and animal welfare is defined as “the physical and mental state of an animal in relation to the conditions in which it lives and dies” (3).
Damaging behaviors (DB) involve oral manipulation of a body part of another pig with the outcome being tissue damage at the targeted body part (referred to as “lesions” in this paper, unless otherwise indicated). DB include tail, ear and flank biting (4–6) with leg and penis biting also reported (7), albeit much less commonly. DB are commonly performed by growing pigs but sows also perform vulva biting (8). Furthermore, there are cases of piglets directing DB toward sows in the farrowing crate (9, 10). Only DB performed by grower-finisher pigs will be considered in this review. Furthermore, although aggression also causes lesions (11) it is not included as it is not considered a DB but forms a normal part of the agonistic behavioral repertoire of the pig (12).
The high prevalence and widespread nature of tail-biting and the contentious way it is controlled (i.e., tail docking) means that it is the most widely studied of all DB [e.g., (13–16)]. Whilst most prevalent in intensive housing systems, tail lesions, usually attributed to tail biting behavior, are also found in straw based (17) and free range/organic production systems (18–20). Tail-biting has negative economic implications as it increases days to slaughter (21) due to reduced growth rates (22, 23), and is related to poor carcass characteristics and an increased risk of carcass condemnation (24–26). It is associated with poor welfare because of the stress and the pain experienced by the bitten pig (27, 28). Such lesions cause additional suffering because of the risk of systemic spread of infection to various organs including the lungs (29–31). There is also some evidence suggesting that pigs performing tail-biting are suffering from stress (28).
The reported incidence and prevalence of tail-biting varies widely between and within farms (25, 32), reflecting the multifactorial etiology of tail-biting (33) and possibly all DB. The risk of tail-biting is increased by management and housing practices that fail to meet the basic needs of pigs, thereby causing stress (28, 34). In addition, acute stress (e.g., caused by a blocked feeder) may cause a sudden outbreak of DB even on a farm where the tail-biting risk is otherwise well-managed (35). Management and housing practices present numerous risk factors relating to diet, regrouping strategy, group composition, space allowance, climate and enrichment. These interact with animal factors such as genetics, sex, age/weight and health. As risk factors differ for every farm, a farm specific solution is required, thereby complicating the development of more generalized prevention strategies (13). Further complication arises from the fact that there are at least three different types of tail-biting, which may have a different motivational background and therefore potentially different risk factors (33).
Ear lesions are a growing problem on pig farms, with a recent study reporting a 100% farm level prevalence on 31 farms with a median of 6.97% animals affected (16). Ear lesions result from factors that compromise skin defenses, allowing the entry of infective agents [e.g., (36)]. High levels of oral behavior (nibbling and chewing) directed toward the ears (37) suggest that, in some cases, DB is the cause of ear lesions. This etiology is well-accepted for tail lesions, where an outbreak of tail-biting is often preceded by a period of tail manipulation without any injured tails (38) and once the tails are damaged and blood is present the problem escalates (39, 40). However, certainty about the etiology of ear lesions is complicated by the relative lack of research on ear biting behavior and on related conditions such as ear necrosis (“dead ear tissue”). Furthermore, similar to tail-biting, there may be three different types of ear biting (37) which further complicates the issue.
Different forms of DB may have some causal factors in common or indeed may be causally linked. Several studies report that individual animals performing more tail-biting also perform more of other DB [e.g., (4, 10)], and treatments that influence tail-biting often influence ear biting and other DB [e.g., (10, 41–43)]. Furthermore, on farms where tails are docked short there is more ear biting (44). Taken together this suggests that at least part of the animal- and environment-based risk factors may overlap for tail-biting and the “less-studied” DB.
There is much anecdotal evidence supporting the putative role that health plays in DB (45, 46). Indeed, disease and tail lesion prevalence correlate at the farm (14) and abattoir (47) level. This may be related to the commonality of risk factors between DB and health problems, for example the absence of straw is associated with a higher risk of tail biting and lameness (48). However, evidence of direct causal links also exist. Animals that feel unwell and/or stressed, experience an immune reaction and altered metabolic state (49), and this may lead them to increase manipulatory behavior of their penmates (50–52). On the other hand, sickness also causes pigs to respond less to general manipulation by their pen mates, thereby placing them at higher risk of injury (51, 52). The potential mechanism for the role sickness plays in DB (52) was explored recently (53). The objective of the current paper is to review links between health and DB in pigs, to assess the evidence for a causal relationship in both directions and to elucidate potential mechanistic pathways.
Correlations Between the Prevalence of Disease or Lesions and Damaging Behavior
The first evidence for links between health and DB comes from studies showing correlations between disease or disease lesions and DB or the associated lesions at farm and abattoir level. Studies describing such associations almost all focus on tail lesions. There are few studies showing links between ear lesions and disease other than those showing the obvious association between ear lesions and ear necrosis [see Malika et al. for review (54)]. Though recently Pessoa et al. (55) reported an association between ear lesions during the grower phase and pericarditis at slaughter. They suggest possible mediation via Streptococcus suis in the saliva. Schroder-Petersen and Simonsen (38) also cite a Dutch study by Elst et al. (56) in which a correlation of 0.25 was found between the percentage of weaned piglets with respiratory disease and ear (and tail) biting problems at farm level.
Tail Data From Live Animals On-Farm and Disease Data
At farm level, authors report an association (14) or a tendency for one between the presence of respiratory diseases and tail-biting (57). Furthermore, Pandolfi et al. (58) showed that the prevalence of severe tail-biting on farms was associated with the prevalence of enzootic pneumonia-like lesions at slaughter. In a controlled farm and abattoir-based study, Marques et al. (59) found an association between tail lesions measured on farm and post-mortem lung lesions (pleuritis and embolic pneumonia). However, at individual pig level the study found no association between tail lesions and new cases of respiratory disease on farm. Indeed, it is difficult to detect respiratory disease in individual pigs in a group on-farm and it often goes unnoticed. Also at individual level, Marques et al. (59) reported that pigs with severe tail lesions had higher odds of presenting locomotion disorders than pigs with no lesion. Similarly, Vom Brocke et al. (60) found a correlation between tail lesions of any severity and leg inflammation and Niemi et al. (61) found more lameness in victims of tail-biting (20% affected) compared to non-victims (9% affected). Interestingly, in the study by Niemi et al. (61) lameness was diagnosed 3.7 days before the pig was diagnosed as a victim of tail-biting suggesting a causal relationship. These authors also reported a 1.8-fold higher risk of generalized health disorders in victims of tail-biting compared to non-victims.
Tail and Disease Data Collected at the Abattoir
As with live animals, data are available on correlations between disease lesions and tail lesions in the slaughtered pig (Table 1). Observed lesions are localized on viscera, mainly reflecting pathologies in the respiratory system or on the carcass (mainly arthritis or abscessation). Potential outcomes of the latter include trimming and partial or entire condemnation. Potentially important associations between tail lesions and disorders of other systems e.g., the digestive tract, cannot be evaluated at the abattoir because the intestines are not routinely inspected.
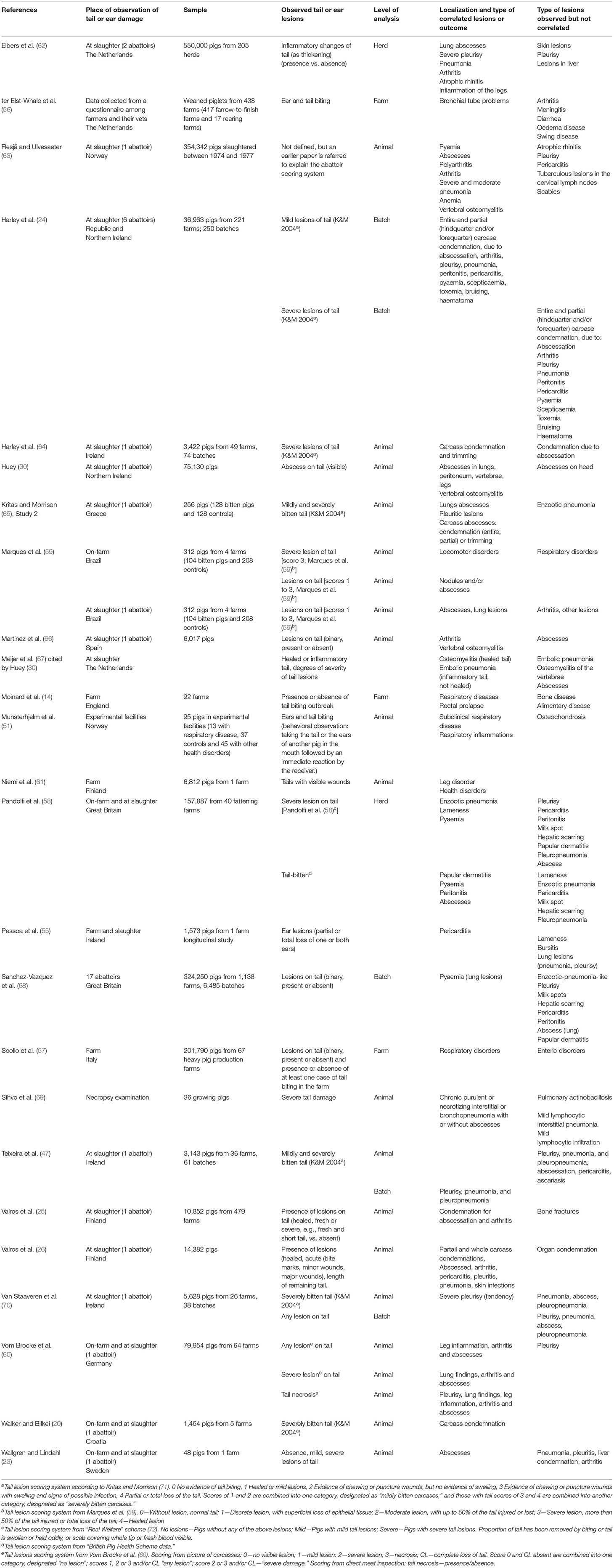
Table 1. Overview of studies showing correlations between tail and ear lesions on farm or at slaughter and carcass findings at slaughter.
Studies look at associations between the presence or absence [e.g., (59)] or severity [e.g., (65)] of tail lesions and viscera and/or carcass lesions in the slaughterhouse. The latter data are either routinely collected as part of meat inspection [e.g., (47)] or involve more detailed measurements using lesion scoring schemes such as that developed by BPEX [e.g., (70)]. Studies based on official meat inspection data are difficult to compare because of inconsistencies between meat inspectors (47, 62) between slaughterhouses (73) and between countries (24, 74). Standardized scoring systems also differ between studies and definitions used of both lung and tail lesions are often vague.
Respiratory Lesions
Numerous studies describe an association at herd or batch level between tail lesions and different lesions of the respiratory tract; pleurisy (47, 62), pneumonia (47, 58, 62), and lung abscesses (58, 62, 68) (Table 1). In contrast, van Staaveren et al. (70) did not find a batch-level association between any lesions of the respiratory tract and tail lesions.
The three studies (47, 58, 62) showing batch or herd level associations between lesions related to (enzootic) pneumonia and tail lesions suggest that generalized poor respiratory health on farm might increase the risk of pigs performing biting behavior. Studies showing that pigs with subclinical respiratory disease were more prone to bite the ears and tails support this (51). This might also explain why there is no association between lesions related to pneumonia and tail lesions at individual level (23, 47, 70) (Table 1). Indeed, Mycoplasma hyopneumoniae (M Hyo), the pathogen commonly responsible for pneumonia (75), is not spread to the lungs via the blood (76), so the pathogenesis of M Hyo—induced enzootic pneumonia is most probably unrelated to tail trauma. Nevertheless, Pandolfi et al. (58) and Valros et al. (26) found an association at animal level between severe tail lesions and pneumonia. In the study by Valros et al. (26), these cases of pneumonia were, however, almost certainly due to some other pathogen as M Hyo is eradicated in Finland.
There are studies showing batch level associations between pleurisy and tail lesions (58, 62, 68). Pleuritis is possibly linked to, for example, a high herd-level prevalence of pigs sero-positive for Actinobacillus pleuropneumoniae (APP) (75). Further, at the level of the individual animal there are strong associations between pleurisy, especially in severe forms [e.g., (70)] and lung abscesses and tail lesions, especially when tail lesions are severe (Table 1). Valros et al. (26) further showed an increase in the occurrence of pleurisy, specifically in pigs with tail lesions that had healed by the time of slaughter. A study including a pathological examination of 56 pigs [selected phenotypically as biters (16), victims of biting (16), controls in biting pen (10), and controls in non-biting pens (14)] from a farm with a tail-biting problem (31) showed that most animals in all these groups (78%) had signs of respiratory inflammations. The severity of these were, however, worse in victims of tail-biting than in the other pigs. Further, Sihvo et al. (69), showed that 9 out of 35 bitten pigs (all 9 had severely bitten tails) had chronic purulent or necrotizing interstitial or bronchopneumonia with (n = 7) or without (n = 2) abscesses. At least part of these lung lesions were caused by secondary, environmental bacteria, which indicates a possible spread via the venous system from the tail. Other types of lung lesions, such as pulmonary actinobacillosis, and mild lymphocytic interstitial pneumonia were evenly spread between control and bitten animals.
Carcass Lesions
There is a well-established association between tail lesions, trimming and condemnation of carcasses (partial or entire) particularly for abscessation at individual pig level (26, 30, 65) (Table 1). Indeed, the lack of an association between carcass condemnation for abscessation and severe tail lesions at individual level in the study by Harley et al. (64) is surprising given that abscessation is the primary reason for carcass condemnation in the Republic of Ireland (24). However, there was an association between mild tail lesions and partial carcass condemnation for abscessation. It is possible that the tail lesions were severe at some stage on-farm and thereby contributed to secondary infection and associated partial carcass condemnation but healed such that they scored mild at the time of slaughter. Infections of the joints (arthritis) and bones (osteomyelitis) were the second most common association between tail lesions and reasons for carcass condemnation/trimming (Table 1). The interrelationship between (poly)arthritis, vertebral osteomyelitis and tail lesions is well-documented [Meijer et al. (67) cited by (30, 63)]. Hence, it is unsurprising that most of these associations were at individual level (23, 25, 60, 66).
Common Risk Factors for Poor Health and Damaging Behavior
The associations described above could arise from a sharing of risk factors between DB and disease or poor health, even in the absence of any causal relationship. Poor health may take many forms, but the presence of inflammation and/or immune activation is a common feature represented by a centrally organized suite of non-specific “sickness behaviors” (77, 78). These include depression, inactivity, anorexia and sleepiness which evolved to conserve body resources for the high energetic costs of fighting infection (79). Risk factors for poor health span many areas and, in this review, we classify them by the physiological system afflicted e.g., respiratory, enteric and locomotory diseases. They may be infectious factors, such as exposure to pathogens involved in respiratory and digestive disorders, or non-infectious factors including animal predisposition and aspects of management such as climatic environment, biosecurity, housing and diet (75, 80–82). These management factors may increase the level of pathogen exposure, reduce robustness of animals when a challenge occurs, or predispose to physical injury or psychological disturbance. Risk factors for tail-biting are also diverse—decision support tools such as the Husbandry Advisory Tool (HAT) (15) and Schwanzbeiß-Interventions-Programm (SchwIP) (60, 83, 84) list more than 80 different risk factors based on scientific literature. The scientific evidence for risk factors comes from controlled trials [e.g., (85, 86)] or from epidemiological studies (6, 13–15, 57, 84, 87–89). Evidence also comes from commercial experience, e.g., (45, 46, 90, 91). It is beyond the scope of this exercise to review the vast body of scientific evidence on risk factors for DB and particularly disease. Hence, the papers listed are not comprehensive but were selected to illustrate commonality of risk factors for both. Examples of the correspondence of risk factors for DB, or the resulting tail lesions, with risk factors for a variety of health conditions are discussed in the following sections and synopsized in Table 2.
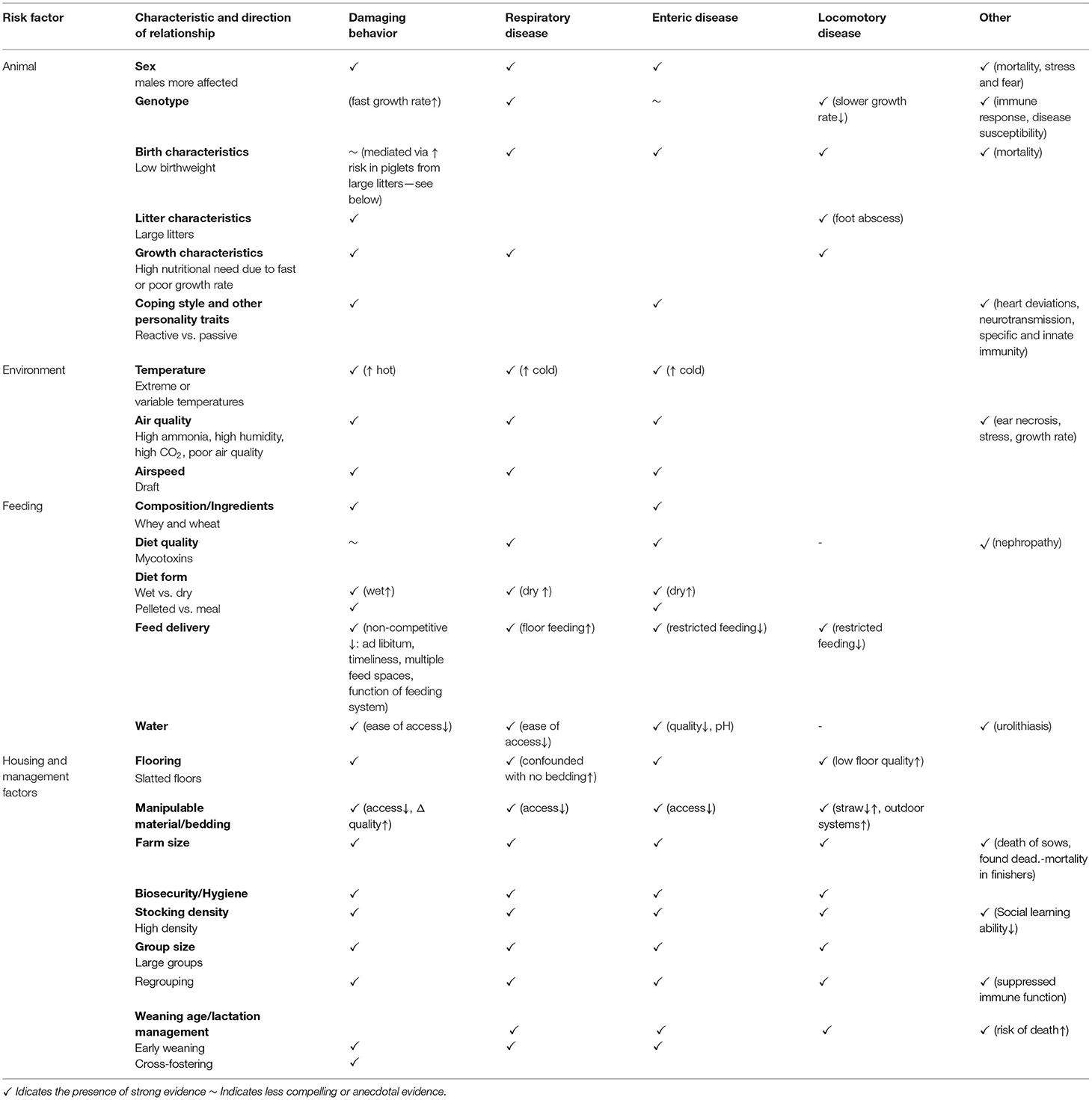
Table 2. Studies supporting animal, environment, feeding, housing and management related risk factors shared between health conditions and damaging behavior and the direction of the relationship for specific characteristics.
Animal Factors
Susceptibility to disease or poor health and to receipt of tail damage or expression of tail-biting behavior are influenced by factors inherent to the animal itself, as illustrated by the following examples.
Sex
Farm and abattoir based studies frequently report that male pigs are more likely to be the recipients of tail-biting (i.e., to have tail lesions) than female pigs irrespective of whether they are castrated (5, 23, 25) or entire (24, 64, 92–94). However, Sinisalo et al. (22) found no difference between the sexes. There is no evidence of a propensity of a particular sex to tail bite. Nevertheless, there was a faster increase in piglets with tail damage in all-female groups compared to piglets in all-male or mixed sex groups post-weaning (94). Furthermore, the duration of tail damage was higher in males in mixed sex groups. As mixed sex groups are the norm in practice, this could explain why more males show tail damage.
The evidence surrounding the propensity for a particular sex to succumb to disease is equally tentative. Male piglets are certainly at higher risk of dying than female piglets (95). They are also more likely to succumb to diseases such as post-weaning multisystemic wasting syndrome (96) and Lundeheim (97) reported that castrates had a higher burden of respiratory diseases than gilts. There is some evidence to suggest pathogenic mechanisms through which males may be more susceptible to disease. Castrated male piglets are more susceptible than females to physiological stress (98, 99) and are more fearful (98, 100, 101) which may increase susceptibility to disease (102). Furthermore, entire male pigs show higher cytokine levels in response to a lipopolysaccharide (LPS) challenge compared to females (103).
Genotype
There are a limited number of reports on breed differences in exhibition of DB, [e.g., (104, 105)] as well as in predisposition to be the recipient of tail-biting (5, 22, 33). Generally, it is difficult to separate effects of breed from effects of selection for production traits such as leanness on the propensity to bite or be bitten. Breuer (106) reported that predisposition to tail bite had a heritable component within Landrace pigs and that this predisposition has a genetic correlation with lean tissue growth rate and lack of subcutaneous fatness. Similarly, Ursinus et al. (10) found that gilts with high levels of tail and/or ear biting had a higher genetic potential for several production traits. On the other hand, there is clear evidence that genetic selection for high lean tissue growth rate is associated with changed immune response and increased disease susceptibility (81, 107, 108). Selection for high lean tissue growth rate is also unfavorably associated with leg weakness score linked to osteochondrosis in pigs (109). Finally, Hessing et al. (110) found a strongly significant “litter-effect” on gastric ulceration which might indicate a genetic predisposition.
Birth and Litter Characteristics
Tail-biting pigs are often smaller “runt” individuals (23, 111, 112). Munsterhjelm et al. (113) found that performers of “tail in mouth” behavior were smaller at birth. However, there is limited evidence that low birthweight (BW) is a risk factor for DB. Ursinus et al. (10) found no difference in BW between high-tail biters (which also performed more DB directed at other body parts), medium tail-biters and non-tail biters. Similarly, a recent, on-farm study (114) failed to link BW to pig-directed manipulation in the grower/finisher periods. There is some evidence of a predisposing effect of undernutrition during lactation, and therefore low weaning weight, on subsequent manipulatory behavior of weaned piglets whereby the later tail-biting behavior seems related more to growth rate immediately preceding onset of the problem [reviewed by (80)]. This review indicated that DB may be increased in piglets originating from large litters, which would tend to have lower average individual BW, although this is more likely explained by the fact that social competition is greater in large litters. Indeed, Ursinus et al. (10) found that gilts showing high levels of tail-biting originated from larger litters. When it comes to the risk of becoming a victim of biting, Hakansson and Houe (115) recently showed that the risk for tail damage was higher in piglets with a high weaning weight.
There is strong evidence that low BW piglets, more prevalent in large litters, are more susceptible to disease in both the short and longer term. Initially there are long-term dysfunctions in vital organs in low body weight piglets [e.g., gastrointestinal tract—(116)], but they are also less competitive at the udder and ingest less colostrum (117). Piglets with low immunoglobulin G serum concentration have a low survival rate (118), although this might also reflect nutritional and thermal challenges to neonates with low colostrum intake. Calderon Diaz et al. (92) reported that pigs with a low BW (<0.95 kg) were at higher mortality risk throughout the production cycle and were at higher risk of locomotor diseases. In line with this, pigs in large litters had a slightly increased risk of developing foot abscesses, compared with pigs in small litters (119). Also Feldpausch et al. (120) reported a highly increased mortality rate in piglets of low BW. Following on from this, pigs of low BW in larger litters are therefore likely to be at greater risk of death and to be lame prior to slaughter. Furthermore, low BW is a risk factor for respiratory disease (121) and the development of PMWS (96, 122) with the latter study suggesting a threshold <1.3 kg. Calderón Díaz et al. (92) presented a strong positive correlation between birth BW and weaning BW. They found that pigs with a low weaning weight were more likely to have an increased risk of lameness, pleurisy, pericarditis and heart condemnations at slaughter. This is in line with the predisposing effect of undernutrition during lactation on subsequent manipulatory behavior of weaned piglets [reviewed by Prunier et al. (80)], suggesting a common early life background to both health and behavior problems.
Growth Characteristics
Clearly, the occurrence of DB in a group negatively affects the growth of the victims. Several studies show lower average daily gain in victims of tail-biting compared to non-victims (22, 61). Similarly, Camerlink et al. (123) reported a poorer average daily gain in pigs that received a lot of oral manipulation (>2% of time), including tail and ear biting and other types of DB. On the other hand, Hakansson and Houe (115), as well as (114) found a greater risk of tail lesions in pigs with a high growth rate before and after weaning. In this case, however, the lesions were not severe, and the weight gain was recorded mainly before the lesions, which implies that high weight gain, or “nutritional need,” predisposes pigs to becoming victims of DB, instead of oral manipulation or tail damage reducing growth rate, as previously suggested. However, it is more likely that the two are not mutually exclusive; while faster growing pigs may be more likely to become victims—they may then have a reduced growth rate if severely bitten. The relationship between growth rate and risk of disease is clearer. Almost all of the common health problems/diseases of pigs are associated with poor growth [e.g., (97)]. A Brazilian study reported an association between lung lesions (pleuritis and embolic pneumonia) and locomotor problems in low body weight pigs at slaughter (59). Similarly, Kuchling et al. (124) reported that expected daily gains reduced significantly for pigs with at least one of the post-mortem findings arthritis, abscess, severe pneumonia, visceralis pleuritis and hepatitis.
The predisposing effect of undernutrition during lactation (80) corresponds to studies showing that pigs exhibiting tail-biting behavior were those that experienced a growth check (111, 112, 125). However, fast growth rates in older pigs may also play a role in the development of DB. At group level, Diana et al. (126) found that pigs with antibiotics in their diet (associated with higher growth rates), were more likely to have tail lesions and performed more ear biting than pigs without. In another study, the fastest growing pens of pigs on a commercial farm were more likely to have ear and tail lesions compared to compromised pigs (127). At individual level, Ursinus et al. (10) reported that gilts showing most biting behavior were also the largest and fastest growing animals. Similarly, Valros et al. (114) reported a high growth rate linked to a higher level of pig-directed manipulation. While pigs that are thriving and growing quickly might be more likely to engage in DB they are almost always less likely to experience health challenges (128). The only condition this does not hold true for is locomotor disorders where selection for fast growth rates is a risk factor for osteochondrosis (109). Furthermore, Quinn et al. (129) reported that gilts fed a diet formulated for slower growth rates (and resulting in reduced average daily gain and lower body weights) had improved locomotory ability and less severe humeral condyle joint lesions compared to gilts on diets designed for fast lean deposition.
Coping Style and Other Personality Traits
Prunier et al. (80) defines personality and coping style and elucidates how they may influence the propensity of pigs to perform DB. The effect of coping style appears to be mediated by the degree of environmental enrichment (130, 131), with pigs of a more reactive coping style showing greater frustration (132) more pessimism (133) and more DB with a lack of or loss of, rooting materials. Although, Ursinus et al. (42), found no such link between coping style and DB. Fearfulness is another personality trait relating to tail-biting. Ursinus et al. (134) reported that tail biters spent less time near a bucket introduced in a novel environment, and suggested that tail biters were more fearful. Zupan et al. (34) found the opposite, with tail biters being faster to touch a novel object, lying more and walking less in a novel environment. Linking tail biting to behavior in fear tests is complex as fear-related behaviors in such tests might reflect temporary states rather than stable personality traits. Moreover, tail biting is not consistent, except for those biters that display high levels of tail biting (10, 42). This could relate to the different types of biting identified, with possibly different underlying motivations and risk factors (33).
Pigs show genetic variation in coping style associated with neurotransmission (135). Tail biters had a lower store of serotonin in blood, and a higher blood platelet serotonin velocity. However, this only held for the period in which they were classified as tail biters (134). Valros et al. (136) reported increased serotonin metabolism in the pre-frontal cortex in tail biters and, only in tail biters, a link between central serotonin and tryptophan levels in the blood. However, it is unknown if these differences in serotonin metabolism are temporary states or stable differences between pigs prone to tail bite and those with a lower propensity to tail bite.
Little is known about the link between health and personality in pigs, except that reactive pigs are more susceptible to develop gastric lesions in barren housing than proactive pigs [(137), but see (138), who found no effect] or when housed with other reactive pigs only (139), whereas proactive pigs showed more heart deviations postmortem (139). Several studies point to a difference between proactive and reactive pigs in parameters related to innate and specific immunity [e.g., (101, 140–145)], although the consequences of these differences for disease susceptibility are unknown. According to Kanitz et al. (142), when exposed to stressful situations, the effects of coping style on humoral immunity differs depending on the specific function of the immunoglobulin classes, as proactive pigs may have an increase in IgA concentration but not in IgM. This makes coping style-related differences in disease susceptibility probable.
Summary—Animal Factors
There are numerous animal-based risk factors shared between tail-biting and disease but several of these are tentative particularly those relating to coping style and other personality traits (Table 2). Many studies show that male pigs are more likely to be bitten and there is evidence that they are more susceptible to stress (98, 99), disease [PMWS—(96)] and death (95). Furthermore, the limited evidence presented above suggests a propensity of females to bite tails. Hence, there might be sex-based differences in the likelihood of a pig to become a biter or a victim of DB, and to succumb to disease but there is surprisingly little research in either area. Birth weight is a major risk factor for disease (96, 119, 122). However, its effect on tail biting is confounded with litter size whereby piglets from larger litters, being smaller, may be more disposed to tail bite (80). This might suggest that better health and growth rate during lactation reduces the risk of a pig becoming a tail-biter in later life. However, genotypes with generally faster growth rates and lower fatness are more likely to tail bite (10, 106) and groups of pigs with high growth rates appear to be more susceptible to DB [e.g., (10, 127)]. These findings appear to contradict those suggesting that pigs experiencing a growth check are the tail-biting culprits. However, pigs in both cases may have a high “nutritional need/metabolic demand,” either because of their (history of) poor health, poor growth and undernutrition or, conversely, due to a high growth/production level demanding greater nutrients/energy [as per (80)]. This increases the pigs' motivation and competitiveness for access to feed, which in turn can lead to DB (33). Hence, it appears that rather than growth rate per se, a high “nutritional need/metabolic demand” is likely the common underlying factor linking health and DB. We elucidate this potential mechanism for a causal link below in section on Elucidating causal mechanisms.
Environmental Factors
Temperature
The effects of temperature on tail biting are contradictory. Smulders et al. (6) found that a higher temperature in the nursery pens was associated with a higher number of pens containing at least one pig with a tail or ear lesion. Similarly, Holling et al. (146) found that a temporary ventilation failure resulting in an increase in temperature was associated with tail-biting. This would suggest that tail biting is higher in the summer. Indeed, Sällvik and Walberg (147) reported that high summer temperatures inside the housing tended to increase tail-biting. However, several epidemiological studies report a higher likelihood of tail lesions in the winter (60, 72, 148). It is likely that fluctuations or extremes of temperature in either direction are risk factors for tail biting and other DB.
Heat stress caused by high temperatures is associated with numerous detrimental physiological changes in the pigs body (149). However, while it undoubtedly leads to poor welfare and poor growth and reproductive performance (150) high temperatures are not directly associated with specific diseases. In this case, there are more implications of cold temperatures. There is an increased incidence of diarrhea in weaned piglets maintained at chronic, moderate cold temperatures (18–20°C) [reports reviewed by (151)]. Armstrong and Cline (152) reported that artificial infection with enterotoxigenic Escherichia coli resulted in higher incidence of diarrhea in newly weaned piglets when exposed to severe cold stress (4–10°C). Temperature fluctuation is also a risk factor—Le Dividich (153) observed a greater incidence of post-weaning diarrhea in piglets kept in a continuous (hourly) fluctuating temperature, 23.5 ± 3°C compared with a constant environment, 23.5 ± 0.5°C. Burrough (154) also identified low temperature as predisposing the development of swine dysentery in endemically infected farms. Many reports of temperature as a risk factor for disease relate to respiratory diseases and Stark (155) cites numerous examples from cross-sectional and longitudinal studies. For example, Geers et al. (156) observed a negative relationship between coughing and air temperature, while the number of treatments for pneumonia was higher for low and highly variable temperatures (157). In a more recent study (75), a mean temperature in the finishing room below 23°C was a risk factor for pleuritis. Similarly, neonatal pigs maintained in a cold environment of 18°C and administered LPS experienced a period of hypothermia coupled with altered endocrine and proinflammatory cytokine responses (158). Additionally, growing pigs kept at high ambient temperature have greater production of heat shock proteins, which are protective during inflammation (159), than those kept at thermoneutrality (160).
Air Quality
Whilst linked to temperature, since ventilation rate affects both, several aspects of air quality are also important risk factors for DB and disease. Various authors describe ammonia as the primary noxious gas able to induce stress and consequent DB, including tail-biting (161, 162). Scollo et al. (88) found that high ammonia was the variable showing greatest importance in influencing prevalence of tail lesions with a threshold level of 28 ppm. Ammonia is also a risk factor for liquefaction necrosis (163) and low body weight (164) most likely due to less feeding behavior (165). These authors reported elevated monocyte, lymphocyte and neutrophil counts in weaner pigs exposed to a concentration of 35 ppm using gas exposure chambers, while pigs exposed to 50 ppm showed increased levels of the acute phase protein, haptoglobin. Exposure to ammonia also affects pig activity (164). Done et al. (166) found small pathological changes in the respiratory tract of pigs exposed to concentrations of ammonia representative of commercial conditions (0.6–37.0 ppm). Meanwhile, Stark (155) cited numerous studies implicating aspects of air quality including humidity, gases, bioaerosols and dust. For example, an air exchange rate of >60 m3 per h per pig, which would reduce all detrimental air contaminants, had a protective effect on pneumonia (63). Relative humidity (RH) correlated with the number of necessary treatments for pneumonia, but with a lag of 2 weeks (157). High humidity was also a risk factor for ear necrosis syndrome (167). A high mean CO2 concentration (>1,600 ppm) in the finishing room was a risk factor for pneumonia (82). This is consistent with previous findings, indicating that high CO2 concentrations may have detrimental effects on respiratory tract health (168–171). Furthermore, air quality (a composite score of airflow patterns, NH3, CO2, RH) is also a risk factor for post-weaning digestive disorders (172).
Air Speed/Draughts
Only, Sällvik and Walberg (147) demonstrated that cold air at high airspeed (i.e., draught) tended to increase tail-biting. Exposure to draughts also resulted in more redirected explorative behavior in weaned piglets, including ear biting, and agonistic behavior, especially head knocks with biting (173). In contrast there is good evidence that draughts are a risk factor for disease with increases in coughing, sneezing and diarrhea reported in weaned piglets (174). The setup of the ventilation system seems to be important for respiratory disease. A direct fresh air inlet from outside or from the corridor into the room vs. an indirect air inlet was a risk factor for pneumonia in weaned pigs (75) or for being seropositive to Actinobacillus pleuropneumoniae serovar 3 in finishing pigs (175). Fablet et al. (75) also found that the range of temperatures controlling ventilation rate, which affects how quickly fan speed increases and decreases and may create air draughts at the pig level, was associated with prevalence of pleuritis.
Summary—Environmental Factors
The evidence for temperature as a shared risk factor for DB and disease is conflicting. While higher temperatures are risk factors for DB (147), colder temperatures are more frequently cited in relation to disease (Table 2), particularly respiratory disease [e.g., (75)]. Nevertheless, these findings support the well-established requirement to maintain pigs in their thermoneutral zone to optimize welfare, health and performance as recently demonstrated by Chantziaras et al. (176). There is compelling evidence supporting the role of draughts and poor air quality, particularly high ammonia levels as risks for disease. However, it is only in the case of ammonia that this is shared with DB. This is in spite of strong anecdotal and farmer reported evidence of the detrimental effects of draughts (or “cold air pockets”) on tail biting (45).
Feeding Factors
Risk factors influencing health and DB relate either to the diet itself or to the method of provision. Additionally, both are related to changes in feeding behavior (177, 178). Feed is one of the most contested resources in pigs which is why feeder space is so important, particularly for low-ranking animals. Statements on the number of recommended trough sites vary between different studies. Spoolder et al. (179) recommended fewer than 20 finishing pigs per trough to reduce aggression. Moinard et al. (14) stated the risk of tail-biting increased (OR = 2.7) when five or more grower pigs share one feed space. In the Holmgren and Lundeheim (180) studies, one percent more tail-biting was observed at trough lengths of 30 cm or less per animal. In an experiment with docked piglets, the experimental group with 3.75 piglets per feeder showed no ear and tail lesions, while these injuries occurred in the groups with 5, 6.25, and 7.25, respectively (181). There are no studies demonstrating the effect of feeder space on health of pigs.
Composition and Ingredients
Certain dietary ingredients increase the risk of tail-biting. These include whey (87, 180) and wheat (87). Interestingly, a high level of wheat in the diet is also a risk factor for gastric ulcers (182, 183) and non-specific colitis (184, 185). Two old studies report on “whey disease syndrome,” characterized by sudden death associated with acute and extensive hemorrhage into the lumen of the small intestine (186, 187). A more recent study also describes deaths caused by “haemorrhagic bowel syndrome” in pigs fed whey (188). However, this is related to poor feed hygiene practices more than whey feeding per se.
The evidence around dietary crude fiber and tail-biting focuses on undocked pigs and is dominated by strong batch and age effects (189, 190). The pigs in these studies were young and therefore generally fed ad-lib and so unlike dry sows (and possibly older pigs—see below) do not experience extreme frustration of feeding/foraging motivation. This is supported by van der Peet-Schwering et al. (191), who found no effect of replacing part of the wheat in the diet with 12–14% of fiber rich feed ingredients on indicators of tail-biting in the nursery. However, they found a lower percentage of grower-finisher pigs with tail damage and with reduced tail length, and a lower number of animals removed to the hospital area on this dietary treatment. It is not surprising that minor increases in dietary fiber level (from 4.2 to 6.6% crude fiber) with minimal enrichment provision did not control tail-biting in undocked finisher pigs on slatted floors (192). Some studies indicate a direct link between ingested fiber and gastric ulcers, e.g., (193). This positive effect is possibly mediated by increased production of saliva (194).
Diet Quality
A widely studied aspect of feed quality is contamination with mycotoxins (195–197). There are anecdotal reports of contamination with mycotoxins being associated with tail-biting [e.g., (198)]. High levels of deoxynivalenol were found in feed and sow blood samples in herds where piglets were affected by tail necrosis (199). Similarly, both tail and ear necrosis was observed after a combination of ergotamine, ergocristine, and ergonovine (10 mg/kg of grain) was fed to older pigs (200). Such necrotic lesions may predispose pigs to perform DB but there is no published evidence of a direct link. In contrast, the immunosuppressive effects of mycotoxins and associated implications for disease in pigs is widely studied (201). Specifically, ingestion of mycotoxin-contaminated feed increases the susceptibility to infectious diseases, reactivates chronic infection and decreases vaccine efficacy (202–205). Aflatoxin B1 (AFB1) lowered the incubation period for swine dysentery and prolonged the clinical diarrhea compared to a control group (206). FB1-exposed piglets showed longer shedding of enterotoxigenic Escherichia coli (ETEC) following infection and a lower induction of antigen-specific immune response after oral immunization (207). Similarly, Pósa et al. (208) showed that FB1 aggravated the progression of respiratory disease.
Feed Delivery System
The feed delivery system is often confounded with the level, frequency and form of feeding, making it difficult to clearly determine the exact nature of risks. Housing systems with ad lib feeding and multiple feed spaces have less tail-biting compared to restricted and single space feeders (6, 14, 209–212). Similarly, other factors which result in increased feed competition such as dysfunction of the feeding system (15, 90) or deficiency in timeliness of feeding (88) are risk factors for tail-biting.
Stärk (155) identified an adverse influence of floor feeding on respiratory disease (213–215). As mentioned, beneficial effects of restricted feeding for leg health are mediated by slower growth rates [e.g., (129)]. Some studies indicate that strategically restricting feed to piglets in the first few days post-weaning reduces post-weaning diarrhea [e.g., (216)] though others contradict this [e.g., (217)]. Further, the likelihood of oesophago-gastric ulcers at slaughter was higher in pigs from herds with ad libitum feeding and herds with automatic feeding (218).
Diet Form
Liquid feeding may predispose to tail-biting (14, 17, 87, 219). However, Hunter et al. (209) found that meal or liquid feeding reduced the probability of long-tailed pigs being tail bitten compared to pelleted feed, and Smulders et al. (6) found a higher number of pens with pigs having tail and ear lesions when pigs were fed a dry diet in the growing unit. Differences are possibly linked to differences in feeding frequency, since both Temple et al. (17) and Kallio et al. (87) identified a higher number of smaller meals per day as a risk factor for tail-biting. In agreement, Hessel et al. (220) found that a higher frequency of daily liquid feeds fed to pigs restrictedly resulted in more competition at feeding than a lower feeding frequency.
Use of pelleted compound diets vs. meal was a risk factor for tail-biting in some studies (14, 87, 209). In this respect, it is interesting that pelleting of diets poses a risk for gastric ulcers (183, 221–223) and for non-specific colitis (184, 224, 225). Dry feeding, as opposed to liquid feeding, is a risk factor for Salmonella in pigs (222). It is also generates dust which might increase risk of respiratory diseases (226).
Provision of Water
In spite of the obvious importance of water for pig welfare, few studies demonstrate a role in tail-biting. Taylor et al. (15) highlighted a reduced risk of tail-biting when pigs had “good water access” (low ratio of pigs: drinkers, clean drinkers and good water pressure). Further, pig producers ranked “water available to all pigs” as the most important preventive factor for tail-biting (91) or ranked it very high (4th of 20 preventive measures) (46). Inadequate access to water is also a risk factor for respiratory disease (155). Madsen and Kristensen (227) revealed alterations in the circadian rhythm of the drinking behavior of young pigs that is an early indication of health impairment. Furthermore, gastric ulceration was associated with the source of water, with use of bore-hole water being a risk factor (218). This may relate to possible effects of pH, buffering capacity or microbiological quality. Urolithiasis (“stones” in the urinary system) in finishing pigs was partially associated with inadequate provision of drinking water (228).
Summary—Feeding Factors
The interrelations between all feeding related risk factors complicates extrapolation of commonality between DB and disease (Table 2). For example, feeding frequency is interrelated with feed form. Hence, while Taylor et al. (15) pointed out that an optimal number of meals/day can minimize tail-biting, the optimal number of meals would need to be determined for all feeding systems and diet forms. The inconsistency is reflected in the effects of liquid feeding, which is a risk factor for DB while dry feeding seems to be a risk factor for certain health problems. Dietary roughage (i.e., fiber from straw or silage) has numerous benefits in terms of facilitating foraging and exploratory behavior, as well as chewing which stimulates saliva production. It also has structural properties that are protective at gut level (193). Not surprisingly there are benefits both to pig behavior, e.g., (229), and health, e.g., (194). However, studies evaluating different levels of dietary fiber show less compelling benefits for tail biting or pig health possibly because growing pigs are generally fed to appetite. The detrimental effects of wheat and whey in the diet and diets in pelleted form are consistent for both DB and disease albeit not widely studied for the former. The same holds for the protective effects of non-competitive feeding systems and easy access to water of high quality. Mycotoxins are the major aspect of diet quality potentially posing risks to pig health and DB but while negative implications for immune function, if not disease itself, are numerous, the evidence for the latter is surprisingly scant.
Housing and Management Factors
Interrelationships between risk factors associated with housing and management are even more complex than feeding related factors. Such factors comprise confounded and interacting combinations of lactation and social group management, enrichment use, flooring and pen design in addition to all feeding and environmental factors. All of these pose risks for DB and disease.
Flooring
Slatted floors are a risk factor for tail-biting (14) with both the presence and the area of slatted flooring implicated (87, 230). This might relate to effects on thermal or physical comfort, air quality or the reduced likelihood of providing bedding. Slatted flooring is also a risk factor for gastric ulcers (48, 221). This could be confounded with the absence of straw bedding, although this is not always the case (231). Stärk (155) identified reports in which slatted floors and poor floor insulation (no bedding) had a negative impact on respiratory health. Sanchez Vasquez et al. (232) demonstrated that part slatted floors compared to solid floors with bedding were a risk factor for enzootic pneumonia-like lesions and pleurisy in the carcass. Aspects of flooring, are also associated with locomotory disorders in finisher pigs (233).
Manipulable Material and Use of Bedding
Straw bedding, as well as offering thermal, physical and nutritional benefits, is also a source of enrichment allowing pigs to express foraging and exploratory behaviors. Hence, the absence of this manipulable material is a consistent risk factor for tail-biting (14, 15, 48, 87, 112, 125, 209). Even moderate bedding decreases tail lesions in undocked finisher pigs (234). Smaller amounts of manipulable substrates such as chopped straw (235), light chopped straw and wood shavings (234) or fresh barley straw (236), mushroom compost (237), peat and sawdust (238), grass silage (194), whole-crop barley and pea silage (239), hessian fabric (10) or freshly cut wood (85) also reduces penmate-manipulations/tail and ear biting. Buijs and Muns (240) summarize the non-straw enrichment material benefits for tail-biting in more detail. It is also worth mentioning that a change in the quality of enrichment between the production stages is a risk factor for tail-biting (15).
There are some concerns for pig health associated with straw provided as bedding or enrichment due to the potential presence of mycotoxins (241). Pigs are susceptible to several types of mycotoxins which have a detrimental impact on immunocompetance (201, 242) if not pig health. Other authors show health challenges for pigs on deep bedding associated with poorer hygiene, including PMWS susceptibility and respiratory/enteric health though there were positive effects on leg health (48). Provision of straw or other fibrous material also reduces the risk of gastric ulceration (194, 221, 243–246) and other stomach and intestinal disorders (247). This could be due to the beneficial effect of fiber ingestion per se (193, 246). However, Gottardo et al. (231) found the provision of other forms of environmental enrichment (hanging chains, plastic objects) also decreased the risk of gastric ulceration on farms that did not provide bedding. There is also evidence of a protective effect of environmental enrichment against other disease challenges. For example, pigs reared under enriched conditions exhibited fewer days of diarrhea after weaning (248) while those raised with a combination of social and environmental enrichment factors had a faster viral clearance and developed fewer and less severe lung lesions after an artificial disease challenge (2).
Farm Size
Larger farm size is a consistent risk for disease and DB (14, 83, 87, 88, 249) with Moinard et al. (14) and Scollo et al. (88) also identifying more pigs per stockperson as a risk for tail-biting.
Stärk (155) cites numerous studies in which herd size was a risk for respiratory disease, with subsequent studies also highlighting a specific risk for pleurisy (250, 251). Ramis et al. (252) suggested that an increase in gastric ulceration in pigs from large farms might be due to increased infection pressure from other diseases, but there are implications of many other non-infectious factors associated with herd size. Goldberg et al. (253) see large herd size as a significant risk factor for mortality in sows, also supported by Bergman et al. (254). Further, Munsterhjelm et al. (177) found an association between farm size and “found dead” mortality in finishers.
Biosecurity/Hygiene
In epidemiological studies both Smulders et al. (6) and Pandolfi et al. (58) reported links between poor biosecurity practices and DB/tail lesions. For example, the former authors found that the absence of footbaths was a risk factor for tail and ear biting. Furthermore, tail-docked pigs subjected to low sanitary conditions showed increased ear-biting behavior and damage to ears in comparison to unchallenged animals, although this effect was diet dependent (43).
Good hygiene and health management can prevent, as well as interrupt, the spread of infection. Generally, poor biosecurity practices pose a greater risk of disease [e.g., (58, 255)]. Pen hygiene is particularly important for enteric disease. Madec et al. (172) showed that pen hygiene status on arrival posed a risk for post-weaning digestive disorders. Non-hygienic husbandry, inadequate quarantine and biosafety measures are also risk factors for PMWS (256–258). Pen hygiene predisposes development of swine dysentery in endemically infected farms (154). A Polish study reported that farms with an all-in/all-out hygiene policy (AIAO) had a significantly lower prevalence of Lawsonia intracellularis (259). In addition, the odds of having a Salmonella-positive sample was 3.9 times lower in farms practicing AIAO (222). The importance of biosecurity, including animal purchasing policy, AIAO management and hygiene in the occurrence of respiratory disease are well-known (253, 260, 261) [see also (155) for review]. Finally, pen hygiene influences prevalence of locomotory disease through increased risk of infectious agents, but also softening of the claw in wet conditions (262) and increased risk of slipping and injury on soiled floors (263).
Stocking Density
As stocking density is the combination of pen size and number of pigs per pen, either factor separately or in combination can influence its effect. Most studies, both epidemiological and experimental, report more tail-biting in pens with higher stocking densities (14, 88, 177, 219). For example, weaner pigs with lower stocking density (<38 kg/m2) had a lower prevalence of tail lesions than pigs in pens with a stocking density ≥38 kg/m2 (84).
High stocking density also increases the risk of many health problems, including non-specific colitis (224), swine dysentery (154) and respiratory disease (155), clinical leg weakness and claw disorders (264). Tuovinen et al. (265) estimated an odds ratio of partial carcass condemnation of 4.2 for a decrease of the total pen area per pig by 0.1 m2. Stocking density influences not just floor space, but also three-dimensional space. More than 3.5 m3 of air volume per pig was preventive for pleurisy (63).
Group Size and Composition
Group size, although sometimes confounded with stocking density, can influence disease transmission and the scope for DB. Holmgren and Lundeheim (180) found that an increase of one pig to the group increased the prevalence of tail-biting by +0.2% with long-tailed pigs, and suggested that this was a consequence of an increase in the number of potential victim pigs. Kallio et al. (87) also found more tail-biting when finishing group size was >9 pigs. Reducing the size of the group from 15 to 12 finisher pigs reduced tail-biting despite a tendency for more tail-directed behavior (266, 267).
Madec et al. (172) showed that larger group sizes (>23 pigs/pen) increased the risk for post-weaning digestive disorders, whilst the risk of PMWSwas greater with large pens (258) or more pigs per pen (122). Higher group size is also linked to risk of non-specific colitis (268) and to respiratory disorders (63, 168). Furthermore, pigs housed in large groups had poorer leg health compared with pigs housed in small groups (262, 269).
Regrouping
Friend et al. (270) found more tail lesions when more litters were regrouped. In agreement, Gruempel et al. (84) reported a lower prevalence of tail lesions in pens where there was less regrouping at weaning. Interestingly, Arey (271) reported an outbreak of tail-biting in pigs after regrouping in new pens with twice as much space as before. Regrouping unfamiliar pigs is a cause of stress and de Groot (272) suggested that this was responsible for suppressing the immune response to a viral vaccine. Regrouping is a risk factor for PMWS (256, 258) and for locomotory disorders (273). It is also a possible risk factor for gastric ulceration (110, 231) while more frequent movement of animals is a risk factor for respiratory disease (213, 214, 274).
Lactation Management and Weaning Age
Moinard et al. (14) found a higher incidence of tail-biting in farms where cross- fostering was practiced compared to farms with no cross-fostering. However, it was uncertain whether cross-fostering contributed directly to later likelihood of tail-biting occurrence or whether this association was related to a common causal factor (for example, herd size or litter size). Hakansson and Houe (115) reported cross-fostering was associated with a higher probability of tail damage post-weaning. In contrast, Calderón Diaz et al. (275) found no influence of early or late cross-fostering on the occurrence of tail lesions. However, pigs from fostered litters were more at risk for death and euthanasia, with severe tail lesions being one of the reasons for euthanasia. Furthermore, cross-fostering placed pigs at greater risk of subsequent pericarditis and heart condemnations (275). A high level of cross-fostering is also a risk factor for PMWS (258).
In pens of weaners with high stocking density, prevalence of tail lesions was lower if total piglet losses were <18 % (12%, n = 45) and higher, if suckling piglet losses were ≥18% (26%, n = 116) (84). This might suggest that better health during lactation reduces later tail-biting risk.
In general, piglets weaned later are more developed and perform less manipulatory behavior after weaning (276–279), which might reduce DB. However, available data suggest that age at weaning (one to 6 weeks) had no clear influence on tail and ear biting in growing pigs even though manipulatory behavior was at least transiently increased in the piglets weaned at the earlier ages (one to 2 weeks compared to 4 weeks, but also 4 weeks compared to 6 weeks) [reviewed by (80)].
Early weaning stress (16 and 18 days of age vs. 20 days of age) resulted in immediate and long-term deleterious effects on intestinal defense mechanisms (280). Early weaning age [e.g., <26.5 days) increases the risk of developing PMWS (172, 281). Piglet age at weaning was also a risk factor for respiratory disease (121, 282). Moreover, the risk of lameness for pigs weaned at a younger age was higher (275).
Weaning Stress
The weaning period is associated with a lot of stress for piglets including removal from the sow, dietary changes, regrouping of pigs from different barns or even farms as well as adapting to a new environment. Indirect evidence suggests that increased weaning stress is associated with more DB. Treatments designed to facilitate the weaning transition, such as perinatal flavor conditioning (283, 284) or playful foraging during the lactation period (285) not only improved growth and feed intake post-weaning, but also reduced DB (248, 284) or number of pigs with ear or tail damage (285).
At weaning, histological changes in the small intestine have a negative effect on the immune system and lead to intestinal dysfunction which favors post-weaning diarrhea (286) and is aggravated by reduced feed intake. Hence, post-weaning alterations in gut functioning can increase disease susceptibility and mortality rates [reviewed by (287)].
Summary—Housing and Management Factors
Animal, feeding and environmental risk factors have a direct effect on the animal. In contrast, some of the risk factors associated with housing and management influence the animal, and therefore DB and disease, indirectly. For example, effects of farm size and biosecurity are probably mediated by related issues such as staffing level, hygiene practices and farm layout (288). Large farms are also more likely to have large group sizes. These in turn are often associated with more regrouping of pigs, although poor matching of pen sizes or uneven growth can also be reasons for repeated regrouping (128, 289). In any case, the direct detrimental effects on tail-biting and health of frequent regrouping, large group size and high stocking densities are consistent (Table 2). The same holds for the protective effects of solid floors and provision of manipulable materials. The benefits of bedding for tail biting and disease likely relate to better thermal and physical comfort and the fact that straw also acts a source of roughage. Indeed, the fact that pigs on slatted flooring do not have bedding and therefore a source of roughage, is a major reason why slatted floors are a risk factor for tail-biting. Straw bedding has some detrimental impacts on pig health but these are likely mediated by management factors such as poor hygiene and use of poor quality straw. Clearly, any housing and management practices that minimize weaning stress can simultaneously improve pig health and welfare and thereby the risk of disease and tail biting. Higher age at weaning is protective for pig health but while there are indications it is also protective for DB, the evidence is limited. Finally, aspects of lactation management such as cross-fostering are increasingly associated with later tail-biting (14) and poor health. However, it is uncertain whether cross-fostering contributes directly to later likelihood of problems, or whether the association is related to a common causal factor (for example, herd size or litter size).
Evidence of Possible Causality in the Link Between Damaging Behavior and Disease
Previously, we outlined the risk factors shared between disease and DB and discussed how they can mediate tail biting and poor health. It is possible that apparent associations between DB and poor health are a result of independent responses to such risk factors, but there is also evidence of direct links between DB and poor health. In this section, we explore the rather limited evidence for a two-way relationship, suggestive of causality, between these two problems. Despite the lack of data, the presence of either of these problems on a farm facilitates the development of the other, as they are both stressors (6, 38, 290).
Disease—A Way to Spread Damaging Behavior
The best known example of disease stimulating DB is the link between exudative dermatitis or “greasy pig disease” and ear biting behavior, e.g., (50, 291, 292) cited by Schroder-Petersen and Simonsen (38). In general, these authors postulate that the associated lesions on the ears make them attractive to other pigs and encourage pigs to start biting the affected ears. Similarly, Clegg et al. (293) suggest that lesions on the body, and particularly on ears, is what leads to biting by other animals in the same pen. However, this hypothesis is untested.
Damaging Behavior—A Way to Spread Disease
Another hypothesis is that pig behavior causes trauma to the ears providing a point of entry for bacteria to set up infection and subsequently ear necrosis [(294, 295) cited by (167)]. Young piglets already perform seemingly non-injurious ear directed behavior in commercial systems [e.g., (296–298)]. Such behavior could be a precursor to DB and therefore causative in the development of ear necrosis. Indeed, most of the bacteria implicated in ear necrosis cannot proliferate in the absence of an initial trauma to the skin of the ear (299). While aggressive behaviors do not fit with the definition of DB employed in this review, the associated injuries to the face and ears arising from such behavior at weaning, also act as entry points for bacteria and thereby disease (294, 300). Similarly, Mirt (299) considers “playing with the tips of the ears,” and “thrusting at each other's flanks” as being enough to cause trauma to provide a point of entry of bacteria.
Damaging behavior can act as the vector of pathogens. Karlsson et al. (301) and Clegg et al. (293) suggest a possible infection route through biting illustrated by the relationship between the presence of Treponema spp. in porcine skin lesions and in gingiva. Thus, biters can infect their victims. Once tail lesions are infected, systemic spread of infection may occur mainly via the venous route targeting the lungs, and to a lesser extent via lymphatic spread (69) as suggested by Schroder-Petersen and Simonsen (38). Spread of pathogens from the bitten tail can also lead to the formation of abscesses, especially on the back area of carcasses (25) or to pyaemia (68) and to embolic pneumonia (59, 65).
Finally, tail-biting can be a transmission route for trichinosis by the ingestion of infected swine flesh (302, 303).
Elucidating Causal Mechanisms
This review indicates strongly that tail-biting and poor health are linked. A possible link between other forms of DB and poor health is hardly investigated, although there are indications that ear lesions arising from ear biting may lead to ear necrosis (299). However, ear biting differs from tail-biting in that pigs may damage each other's ears during aggressive interactions (304, 305) as well as through DB (37). Links between tail biting and disease are reflected in the positive correlations between tail lesions and (postmortem) signs of (particularly respiratory) disease at farm and batch level. In spite of issues of confounding and interrelationships, the numerous examples of commonality of risk factors between tail biting and disease provide the first line of evidence for possible causality. This means that efforts to address non-infectious risks for certain diseases will simultaneously reduce the risk of DB on the farm and vice versa. This will thereby contribute to more (cost) effective prevention strategies for both (306). Nevertheless, the sharing of risk factors also complicates elucidating causality experimentally. Proving causal relationships is only possible in controlled experimental studies but such studies usually only concentrate on one or a few risk factors simultaneously and therefore fail to address the multifactorial nature of the problem.
The evidence for causal relationships between lesions arising from tail-biting and disease, is limited. However, the findings of this review support two likely causal relationships. The first is that generalized poor health (e.g., enzootic pneumonia) on farms poses an increased risk of pigs performing DB. The second involves tail-biting causing poor health, both indirectly, through pathogens entering the body via the (tail) lesion followed by systemic spread of infection through the body, or directly, whereby biters infect victims. These causal mechanisms are supported by some actual proof of health problems increasing the risk of DB (51), and of DB increasing the risk of poor health [e.g., (69)].
As well as infection caused by disease itself, many of the non-infectious risk factors which are shared between poor health and tail-biting induce the production of cytokines by the innate immune system (53). Indeed this immune activation by non-infectious as well as infectious risk factors (307–309) could explain the seemingly cumulative effect of risk factors when it comes to outbreaks of tail-biting (8, 35). Studies in humans (78, 310–312) and rodents (313–315) indicate a causal role for pro-inflammatory cytokines in the deterioration in mood experienced during some types of illness, and their putative role in clinical depression for some patient subtypes is under investigation. These cytokine-mediated changes in mood could lower the threshold to show DB toward conspecifics. Experimental studies tested the effects of immune activation (by means of LPS injection) on behavior in gilts housed in triplets (52). In addition, there are correlations between cytokines and behaviors related to tail-biting in intact boars (51). LPS seemed to increase tail-biting related behavior in the gilts after the sickness symptoms abated, and there was a correlation between different cytokines and tail-biting related behavior in the intact boars. Nordgreen et al. (53) discussed these findings and the putative mechanisms linking cytokines to a change in behavior.
Vaccination stimulates the immune system in a similar way to a disease challenge. In laying hens, there is evidence that vaccination may stimulate feather pecking because of activation of the immune system (316). In pigs the limited evidence is to the contrary, i.e., that vaccination might reduce DB. For example, Almond and Bilkei (317) found that an oral vaccine against Lawsonia intracellularis resulted in less social stress and what they described as “cannibalism-related waste” compared to unvaccinated pigs. However, the paper did not investigate effects of the vaccine on DB. Similarly, other authors report that vaccination against PCV2 was effective in the reduction of ear necrosis syndrome, but we do not know if this was mediated by a reduction in DB (318, 319).
We describe numerous farm and abattoir based studies showing associations between tail and respiratory lesions reflecting infection with enzootic pneumonia. This disease is associated with generally poor health and therefore a generalized inflammatory response (68). Based on the putative mechanism outlined by Nordgreen et al. (53), the presence of such a respiratory disease likely increases the risk of pigs with a high disease burden to perform DB. This could conceivably lead to a circular relationship between poor health and DB, whereby all pigs on such a farm would have a generalized inflammation making (some of) them more likely to tail bite. In doing so, a strong inflammatory/acute phase response arising from tail damage would be induced in their already compromised (inflammatory wise) victims (29). In support of this, in some studies pigs act as both biters and victims (134). Clearly, this complicates ascertaining “what comes first,” tail-biting or illness.
Suboptimal nutrition could be an important link between generalized poor health on a farm, the associated activation of inflammatory processes and increased risk of DB. Several reviews point to suboptimal nutrition as a risk factor for DB [e.g., (33, 320)]. Dietary deficiencies enhance foraging-related exploratory behavior (321, 322) which, if directed at another pig might increase the risk of DB. Moreover, some dietary deficiencies may increase the attractiveness of blood [protein deficiency: (323); salt deficiency: (39, 40)], which could act as an incentive to sustain DB. Several mechanisms could play a role in the link between nutrition, poor health and DB. Firstly, there are negative effects of generalized poor health on the ingestion and processing of nutrients. At its simplest and as discussed earlier, poor health can in this way cause growth retardation, which is a potential cause of tail-biting (112, 125). Secondly, health problems also alter the requirements for specific nutrients, like protein or essential amino acids, which could potentially lead to dietary deficiencies, in particular if the diet contained just sufficient amounts of such nutrients for a healthy, unstressed state. The essential amino acid tryptophan is the precursor of serotonin, a neurotransmitter that is related with tail-biting (134) and its metabolism is associated with inflammatory processes. Part of the inflammatory response is to increase tryptophan catabolism (324) so that tryptophan is not available for pathogens (325). Hence, a bout of illness could spark a tryptophan deficiency, potentially leading to DB. Indeed, tryptophan supplementation decreases ear and tail-biting (326). Also low dietary protein levels increase the occurrence of DB like ear and tail-biting and other manipulation directed to pen mates (43). The same study also reported a diet-dependent effect of poor sanitary conditions on ear biting. Supplementing the diet with a 20% increase of amino acids important in the inflammatory response, i.e., tryptophan, threonine and methionine, reduced ear biting only in the pigs housed in poor sanitary conditions. In line with this, Pastorelli et al. (327) showed decreased growth and increased activity and trough-related exploration in response to a reduction in diet quality in pigs kept in unhygienic conditions. There was no such effect in pigs in good sanitary conditions, suggesting that immune-stimulated pigs may have greater susceptibility to specific nutrient deficiencies. Thus, there are clear examples of nutritional deficits influencing DB, suggesting that poor health could enhance DB via its effect on the metabolic status of pigs. Taken together this suggests that there may be scope for reducing DB when pigs are challenged via customized nutritional programs.
Linked to suboptimal nutrition, “gastrointestinal discomfort” is a risk factor for tail-biting (328). Indeed, Van den Berg et al. (329) reported a higher prevalence of gastric lesions in pigs from herds with clinical signs of tail-biting. However, causation was not proven and shared underlying risk factors such as diet form and ingredients, genotype and coping style cannot be ruled out. Perhaps pigs that suffer from gastric lesions and ulcers may increase their chewing behavior in an effort to increase saliva production and stomach pH and thereby reduce gastrointestinal discomfort, and this manifests in increased tail or ear biting (328). However, Munsterhjelm et al. (31) did not find a difference between tail biters and non-biters in gastric lesions. Furthermore, Palander et al. (330) hypothesized that tail biters might suffer from a poor ability to digest and absorb nutrients. Although there were differences in gut morphology between pens with and without tail-biting, blood minerals and amino acids, no signs of malabsorption was found in tail biters. Finally, a role of the “brain-gut-microbiota axis” in the occurrence of tail-biting is proposed (328) and there is early research suggesting a link between gut microbiota and DB (tail-biting) in pigs (331).
This review provides clear evidence of associations between tail lesions and pathological lesions indicative of secondary infection (abscessation, bone and joint infections) in the individual animal. Further, in individual victims of tail-biting, especially if the tail lesion is severe, there is an increased risk of secondary lesions in the lungs, or complications of lung inflammation. These findings support the theory of venous or cerebrospinal fluid spread of infection/pathogens from the tail lesion site beyond the coccygeal region (69). There is no supporting experiment evidence and indeed, it would pose considerable ethical challenges to do so (332). Logistically the low prevalence of abscessation in slaughter pigs means that, even in epidemiological abattoir-based studies, it is difficult to detect enough cases to generate support for the link. The absence of associations between lesions associated with secondary infection and tail lesions at batch level (62, 74) provides further evidence of a causal link. Should an association at batch level exist, it would suggest that the portal entry of the infectious agent was not necessarily the tail. Indeed Martinez et al. (66) proposed that an environment predisposing pigs to other injuries could have provided a port of entry for pathogens leading to abscess formation as a possible explanation for the lack of an association between tail lesions and abscessation in individual pigs in their study. In that study, only growth-retarded slaughter pigs were involved and there was a high prevalence of abscessation relative to pigs affected by tail lesions (only 0.25% of pigs affected). The authors also suggested that another explanation for the lack of association might be the fact that the pigs had tail lesions that had healed by the time of slaughter. Marques et al. (59) also noted this complication. In explaining the origin of some spinal abscesses in the absence of obvious tail damage, Huey (30) suggested that, since the development of spinal cord abscesses takes several weeks or even months, the original tail lesion could disappear before slaughter. Indeed Valros et al. (26) showed increased pathologies in slaughter pigs with fully healed tail lesions.
Antibiotics are used to treat pigs with tail lesions. Studies from Finland, where antibiotic use is low and tails are undocked, show that tail lesions are one of the most common reasons for treatment of individual pigs (333, 334). Therefore, associations between tail lesions and abscessation raise questions about the effectiveness of the antibiotics or treatment protocols. The Norwegian Medicines Agency recommends benzylpenicillin procaine to treat tail wounds (335). Penicillins reach sufficient concentrations in soft tissue and are therefore effective against anaerobic bacteria, which cause abscesses (336). Hence, if administered soon enough this active agent should kill the bacteria before abscesses form. However, delayed treatment means that secondary infections encapsulate locally within the body such that the antibiotic cannot reach them. Indeed, penetration of an antibiotic into an encapsulated purulent lesion is limited and highly dependent on the degree of abscess maturation (337). Spinal cord abscesses may take several weeks or even months to develop (30). Assuming some sort of intervention is made to protect a tail bitten pig from further damage [see (338)], cutaneous healing of tail injuries occurs over 3–7 days (339). Therefore, it is plausible that treatment of pigs with antibiotics stops once the original tail lesion heals. This might be too soon to prevent the development of abscesses. As far as we are aware, there are no studies showing the effectiveness of antibiotics in reducing the risk for secondary infections in relation to tail-biting. Neither are there studies defining how serious a tail lesion should be to benefit from treatment with antimicrobials, or which treatment protocols are most efficient. Given the risk of secondary infection to the welfare of the pig, to food safety and the threat of antimicrobial resistance, arising from mis/over use of medications there is clearly a need for research to inform guidance on the sustainable use of antimicrobials in the treatment of tail lesions.
Conclusions
Sharing of common risk factors and correlations between poor health and DB and sharing of risk factors is evidence of a clear link at individual and batch/farm level. Their circular relationship, with some evidence of two-way causal mechanisms, makes it difficult to understand the complex underlying mechanisms linking poor health to DB. Nevertheless, the undeniable links offer hope of simultaneous progress on two of the main challenges to the sustainability of pig production, namely, growing public concerns for pig welfare and the threat of antimicrobial resistance arising from mis/over use of antibiotics. The myriad of links between tail-biting, one of the major threats to pig welfare and poor health, the major cause of antimicrobial use, means that efforts to address non-infectious risks for certain diseases will simultaneously reduce the risk of DB and vice versa. At a practical level, the findings have two important implications for the renewed efforts to stop tail docking pigs in the EU. Firstly, the increased risk of tail-biting in long tailed pigs, at least in the interim, means there is an urgent need for protocols for antibiotic use in bitten pigs. Secondly, farmers need to focus on overcoming disease challenges, as well as on reducing common risk factors for disease and tail-biting if they are to raise undocked pigs successfully.
Author Contributions
All authors listed have made a substantial, direct, and intellectual contribution to the work and approved it for publication.
Conflict of Interest
The authors declare that the research was conducted in the absence of any commercial or financial relationships that could be construed as a potential conflict of interest.
The handling editor declared a past co-authorship with one of the authors, SE.
Publisher's Note
All claims expressed in this article are solely those of the authors and do not necessarily represent those of their affiliated organizations, or those of the publisher, the editors and the reviewers. Any product that may be evaluated in this article, or claim that may be made by its manufacturer, is not guaranteed or endorsed by the publisher.
Acknowledgments
This work formed part of a key deliverable of the GroupHouseNet (Cost Action 15134) supported by COST (European Cooperation in Science and Technology). The authors would like to thank Dr. Sabine Dippel for leading the early conceptualization of this manuscript and offer sincere thanks to Prof. Mari Heinonen for her helpful comments on the manuscript. We would also like to acknowledge Joana Pessoa and Martyna Lagoda for help with the formatting.
References
1. Nalon E, De Briyne N. Efforts to ban the routine tail docking of pigs and to give pigs enrichment materials via EU law: where do we stand a quarter of a century on? Animals. (2019) 9:132. doi: 10.3390/ani9040132
2. van Dixhoorn ID, Reimert I, Middelkoop J, Bolhuis JE, Wisselink HJ, Groot Koerkamp PW, et al. Enriched housing reduces disease susceptibility to co-infection with porcine reproductive and respiratory virus (PRRSV) and Actinobacillus pleuropneumoniae (A. pleuropneumoniae) in young pigs. PLoS ONE. (2016) 11:e0161832. doi: 10.1371/journal.pone.0161832
3. OIE Terrestrial Animal Health Code (2021) Section 7 - Animal Welfare. (2019). Available online at: https://www.oie.int/fileadmin/Home/eng/Health_standards/tahc/current/chapitre_aw_introduction.pdf (accessed January 4, 2022).
4. Brunberg E, Wallenbeck A, Keeling LJ. Tail biting in fattening pigs: associations between frequency of tail biting and other abnormal behaviours. Appl Anim Behav Sci. (2011) 133:18–25. doi: 10.1016/j.applanim.2011.04.019
5. Schrøder-Petersen DL, Simonsen H. Tail biting in pigs. Vet J. (2001) 162:196–210. doi: 10.1053/tvjl.2001.0605
6. Smulders D, Hautekiet V, Verbeke G, Geerst R. Tail and ear biting lesions in pigs: an epidemiological study. Anim Welfare. (2008) 17:61–69.
7. Weiler U, Isernhagen M, Stefanski V, Ritzmann M, Kress K, Hein C, et al. Penile injuries in wild and domestic pigs. Animals. (2016) 6:25. doi: 10.3390/ani6040025
8. Bracke MBM. RICHPIG: a semantic model to assess enrichment materials for pigs. Anim Welfare. (2008) 17:289–304.
9. Ladewig J, Kloeppel P, Kallweit E. A case of reversed cannibalism - the piglets damaging the sow. Ann Rech Vet. (1984) 15:275–7.
10. Ursinus WW, Wijnen HJ, Bartels AC, Dijvesteijn N, van Reenen CG, Bolhuis JE. Damaging biting behaviors in intensively kept rearing gilts: the effect of jute sacks and relations with production characteristics. J Anim Sci. (2014) 92:5193–202. doi: 10.2527/jas.2014-7918
11. Turner SP, Farnworth MJ, White IMS, Brotherstone S, Mendl M, Knap P, et al. The accumulation of skin lesions and their use as a predictor of individual aggressiveness in pigs. Appl Anim Behav Sci. (2006) 96:245–59. doi: 10.1016/j.applanim.2005.06.009
12. D'Eath RB, Turner SP. The Natural Behaviour of the Pig. The Welfare of Pigs. Dordrecht: Springer (2009). p. 13–45. doi: 10.1007/978-1-4020-8909-1_2
13. EFSA, Panel on Animal Health Welfare. Scientific Opinion concerning a Multifactorial approach on the use of animal and non-animal-based measures to assess the welfare of pigs. Efsa J. (2014) 12:3702. doi: 10.2903/j.efsa.2014.3702
14. Moinard C, Mendl M, Nicol CJ, Green LE. A case control study of on-farm risk factors for tail biting in pigs. Appl Anim Behav Sci. (2003) 81:333–55. doi: 10.1016/S0168-1591(02)00276-9
15. Taylor NR, Parker RM, Mendl M, Edwards SA, Main DC. Prevalence of risk factors for tail biting on commercial farms and intervention strategies. Vet J. (2012) 194:77–83. doi: 10.1016/j.tvjl.2012.03.004
16. van Staaveren N, Calderón Díaz JA, Garcia Manzanilla E, Hanlon A, Boyle LA. Prevalence of welfare outcomes in the weaner and finisher stages of the production cycle on 31 Irish pig farms. Irish Vet J. (2018) 71:9. doi: 10.1186/s13620-018-0121-5
17. Temple D, Courboulay V, Velarde A, Dalmau A, Manteca X. The welfare of growing pigs in five different production systems in France and Spain: assessment of health. Anim Welfare. (2012) 21:257–71. doi: 10.7120/09627286.21.2.257
18. Alban L, Petersen JV, Busch ME. A comparison between lesions found during meat inspection of finishing pigs raised under organic/free-range conditions and conventional, indoor conditions. Porcine Health Manag. (2015) 1:1–11. doi: 10.1186/2055-5660-1-4
19. Bracke MBM, De Lauwere CC, Wind SMM, Zonerland JJ. Attitudes of Dutch pig farmers towards tail biting and tail docking. J Agric Environ Ethics. (2013) 26:847–68. doi: 10.1007/s10806-012-9410-2
20. Walker PK, Bilkei G. Tail-biting in outdoor pig production. Vet J. (2006) 171:367–9. doi: 10.1016/j.tvjl.2004.10.011
21. van Staaveren N, Boyle LA, Manzanilla EG, O'Driscoll K, Shalloo L, Díaz JAC. Severe tail lesions in finisher pigs are associated with reduction in annual profit in farrow-to-finish pig farms. Vet Rec. (2021) 188:e13. doi: 10.1002/vetr.13
22. Sinisalo A, Niemi JK, Heinonen M, Valros A. Tail biting and production performance in fattening pigs. Livest Sci. (2012) 143:220–5. doi: 10.1016/j.livsci.2011.09.019
23. Wallgren P, Lindahl E. The influence of tail biting on performance of fattening pigs. Acta Vet Scand. (1996) 37:453–460. doi: 10.1186/BF03548085
24. Harley S, More SJ, O'Connell NE, Hanlon A, Teixeira D, Boyle L. Evaluating the prevalence of tail biting and carcase condemnations in slaughter pigs in the Republic and Northern Ireland, and the potential of abattoir meat inspection as a welfare surveillance tool. Vet Rec. (2012) 171:621. doi: 10.1136/vr.100986
25. Valros A, Ahlstrom S, Rintala H, Hakkinen T, Saloniemi H. The prevalence of tail damage in slaughter pigs in Finland and associations to carcass condemnations. Acta Agr Scand a-An. (2004) 54:213–9. doi: 10.1080/09064700510009234
26. Valros A, Välimäki E, Nordgren H, Vugts J, Fàbrega E, Heinonen M. Intact tails as a welfare indicator in finishing pigs? Scoring of tail lesions and defining intact tails in undocked pigs at the abattoir. Front Vet Sci. (2020) 7:405. doi: 10.3389/fvets.2020.00405
27. Di Giminiani P, Nasirahmadi A, Malcolm EM, Leach MC, Edwards SA. Docking piglet tails: how much does it hurt and for how long? Physiol Behav. (2017) 182:69–76. doi: 10.1016/j.physbeh.2017.09.028
28. Munsterhjelm C, Brunberg E, Heinonen M, Keeling L, Valros A. Stress measures in tail biters and bitten pigs in a matched case-control study. Anim Welfare. (2013) 22:331–8. doi: 10.7120/09627286.22.3.331
29. Heinonen M, Orro T, Kokkonen T, Munsterhjelm C, Peltoniemi O, Valros A. Tail biting induces a strong acute phase response and tail-end inflammation in finishing pigs. Vet. J. (2010) 184:303–7. doi: 10.1016/j.tvjl.2009.02.021
30. Huey RJ. Incidence, location and interrelationships between the sites of abscesses recorded in pigs at a bacon factory in Northern Ireland. Vet Rec. (1996) 138:511–4. doi: 10.1136/vr.138.21.511
31. Munsterhjelm C, Simola O, Keeling L, Valros A, Heinonen M. Health parameters in tail biters and bitten pigs in a case–control study. Animal. (2013) 7:814–21. doi: 10.1017/S1751731112002194
32. Heinonen ML, Välimäki E, Laakkonen A-M, Toppari I, Vugts J, Fàbrega E, et al. Evaluation of tail lesions of finishing pigs at the slaughterhouse: associations with herd-level observations. Front Vet Sci. (2021) 8:462. doi: 10.3389/fvets.2021.650590
33. Taylor NR, Main DCJ, Mendl M, Edwards SA. Tail-biting a new perspective. Vet J. (2010) 186:137–47. doi: 10.1016/j.tvjl.2009.08.028
34. Zupan M, Janczak AM, Framstad T, Zanella AJ. The effect of biting tails and having tails bitten in pigs. Physiol Behav. (2012) 106:638–44. doi: 10.1016/j.physbeh.2012.04.025
35. Valros A. Tail Biting, Advances in Pig Welfare. Woodhead Publishing (2018). p. 137–66. doi: 10.1016/B978-0-08-101012-9.00004-6
36. Park J. Investigation of Exudative Epidermitis and Ear Necrosis in Pigs. Guelph, ON: University of Guelph (2011).
37. Diana A, Carpentier L, Piette D, Boyle LA, Berckmans D, Norton T. An ethogram of biter and bitten pigs during an ear biting event: first step in the development of a Precision Livestock Farming tool. Appl Anim Behav Sci. (2019) 215:26–36. doi: 10.1016/j.applanim.2019.03.011
38. Schroder-Petersen DL, Simonsen HB, Lawson LG. Tail-in-mouth behaviour among weaner pigs in relation to age, gender and group composition regarding gender. Acta Agr Scand a-An. (2003) 53:29–34. doi: 10.1080/09064700310002017
39. Fraser D. Attraction to blood as a factor in tail-biting by pigs. Appl Anim Behav Sci. (1987) 17:61–8. doi: 10.1016/0168-1591(87)90008-6
40. Fraser D. Mineral-deficient diets and the pig's attraction to blood: implications for tail-biting. Can J Anim Sci. (1987) 67:909–18. doi: 10.4141/cjas87-096
41. Camerlink I, Ursinus WW, Bijma P, Kemp B, Bolhuis JE. Indirect genetic effects for growth rate in domestic pigs alter aggressive and manipulative biting behaviour. Behav Genet. (2015) 45:117–26. doi: 10.1007/s10519-014-9671-9
42. Ursinus WW, Van Reenen CG, Kemp B, Bolhuis JE. Tail biting behaviour and tail damage in pigs and the relationship with general behaviour: predicting the inevitable? Appl Anim Behav Sci. (2014) 156:22–36. doi: 10.1016/j.applanim.2014.04.001
43. van der Meer Y, Gerrits WJJ, Jansman AJM, Kemp B, Bolhuis JE. A link between damaging behaviour in pigs, sanitary conditions, and dietary protein and amino acid supply. PLoS ONE. (2017) 12:e0174688. doi: 10.1371/journal.pone.0174688
44. Goossens X, Sobry L, Odberg F, Tuyttens F, Maes D, De Smet S, et al. A population-based on-farm evaluation protocol for comparing the welfare of pigs between farms. Anim Welfare. (2008) 17:35–41.
45. Haigh A, O'Driscoll K. Irish pig farmer's perceptions and experiences of tail and ear biting. Porcine Health Manag. (2019) 5:30. doi: 10.1186/s40813-019-0135-8
46. Valros A, Munsterhjelm C, Hnninen L, Kauppinen T, Heinonen M. Managing undocked pigs - on-farm prevention of tail biting and attitudes towards tail biting and docking. Porcine Health Manag. (2016) 2:2. doi: 10.1186/s40813-016-0020-7
47. Teixeira DL, Harley S, Hanlon A, O'Connell NE, More SJ, Manzanilla EG, et al. Study on the association between tail lesion score, cold carcass weight, and viscera condemnations in slaughter pigs. Front Vet Sci. (2016) 3:24. doi: 10.3389/fvets.2016.00024
48. Scott K, Chennells D, Campbell FM, Hunt B, Armstrong D, Taylor L, et al. The welfare of finishing pigs in two contrasting housing systems: fully-slatted versus straw-bedded accommodation. Livest Sci. (2006) 103:104–15. doi: 10.1016/j.livsci.2006.01.008
49. Nordgreen J, Munsterhjelm C, Aae F, Popova A, Boysen P, Ranheim B, et al. The effect of lipopolysaccharide (LPS) on inflammatory markers in blood and brain and on behavior in individually-housed pigs. Physiol Behav. (2018) 195:98–111. doi: 10.1016/j.physbeh.2018.07.013
50. Fritchen R, Hogg A. G75-246 Preventing Tail Biting in Swine (Anti-Comfort Syndrome) (Revised January 1983). Historical Materials from University of Nebraska-Lincoln Extension (1975). p. 1680.
51. Munsterhjelm C, Nordgreen J, Aae F, Heinonen M, Olstad K, Aasmundstad T, et al. To be blamed or pitied? The effect of illness on social behavior, cytokine levels and feed intake in undocked boars. Physiol Behav. (2017) 179:298–307. doi: 10.1016/j.physbeh.2017.06.024
52. Munsterhjelm C, Nordgreen J, Aae F, Heinonen M, Valros A, Janczak AM. Sick and grumpy: changes in social behaviour after a controlled immune stimulation in group-housed gilts. Physiol Behav. (2019) 198:76–83. doi: 10.1016/j.physbeh.2018.09.018
53. Nordgreen J, Edwards SA, Boyle LA, Bolhuis JE, Veit C, Sayyari A, et al. A proposed role for pro-inflammatory cytokines in damaging behavior in pigs. Front Vet Sci. (2020) 7:646. doi: 10.3389/fvets.2020.00646
54. Malika M, Chiers K, Boyen F, Croubels S, Maes D. Porcine ear necrosis. Vet J. (2021) 271:105655. doi: 10.1016/j.tvjl.2021.105655
55. Pessoa J, McAloon C, Rodrigues da Costa M, et al. Adding value to food chain information: using data on pig welfare and antimicrobial use on-farm to predict meat inspection outcomes. Porc Health Manag. (2021) 7:55. doi: 10.1186/s40813-021-00234-x
56. Elst WET, Vaessen MA, Vos HJMP, Binnendijk GP, Huirne REM, Backus GBC. Proefverslag-Varkensproefbedrijf. Zuid-en-west-Nederland. No Pl. (1998) 200:1–54.
57. Scollo A, Contiero B, Gottardo F. Frequency of tail lesions and risk factors for tail biting in heavy pig production from weaning to 170 kg live weight. Vet J. (2016) 207:92–98. doi: 10.1016/j.tvjl.2015.10.056
58. Pandolfi F, Edwards SA, Maes D, Kyriazakis I. Connecting different data sources to assess the interconnections between biosecurity, health, welfare, and performance in commercial pig farms in great Britain. Front Vet Sci. (2018) 5:41. doi: 10.3389/fvets.2018.00041
59. Marques BMF, Bernardi ML, Coelho CF, Almeida M, Morales OE, Mores TJ, et al. Influence of tail biting on weight gain, lesions and condemnations at slaughter of finishing pigs. Pesqui. Vet Bras. (2012) 32:967–74. doi: 10.1590/S0100-736X2012001000003
60. Vom Brocke A, Karnholz C, Madey-Rindermann D, Gauly M, Leeb C, Winckler C, et al. Tail lesions in fattening pigs: relationships with postmortem meat inspection and influence of a tail biting management tool. Animal. (2019) 13:835–44. doi: 10.1017/S1751731118002070
61. Niemi JK, Sinisalo A, Valros A, Heinonen M. The timing and treatment of tail biting in fattening pigs. In: Hulgren J, Persson P, Nadeau E, Fogelberg, editors. 24th NJF Congress Food, Feed, Fuel andFun-Nordic Light on Future Land Use and Rural Development, NJF Report. Sweden: NJK (2011). p. 50–5.
62. Elbers A, Tielen M, Snijders J, Cromwijk W, Hunneman W. Epidemiological studies on lesions in finishing pigs in the Netherlands. I. Prevalence, seasonality and interrelationship. Prev Vet Med. (1992) 14:217–31. doi: 10.1016/0167-5877(92)90018-B
63. Flesjå KI, Ulvesæter HO. Pathological lesions in swine at slaughter. III. Inter-relationship between pathological lesions, and between pathological lesions and carcass quality and carcass weight. Acta Vet. Scand. (1980) 74:1–22.
64. Harley S, Boyle L, O'Connell N, More S, Teixeira D, Hanlon A. Docking the value of pigmeat? Prevalence and financial implications of welfare lesions in Irish slaughter pigs. Anim Welfare. (2014) 23:275–85. doi: 10.7120/09627286.23.3.275
65. Kritas SK, Morrison RB. Relationships between tail biting in pigs and disease lesions and condemnations at slaughter. Vet Rec. (2007) 160:149–52. doi: 10.1136/vr.160.5.149
66. Martínez J, Jaro PJ, Aduriz G, Gómez EA, Peris B, Corpa JM. Carcass condemnation causes of growth retarded pigs at slaughter. Vet J. (2007) 174:160–4. doi: 10.1016/j.tvjl.2006.05.005
67. Meijer P, Rijnvis HJ, Van Logtestijn JG. [Inflammation of the tail in swine. Slaughter-house findings during 1972, 1973, and 1974 (author's transl)]. Tijdschr Diergeneeskd. (1976) 101:1073–8.
68. Sanchez-Vazquez MJ, Nielen M, Edwards SA, Gunn GJ, Lewis FI. Identifying associations between pig pathologies using a multi-dimensional machine learning methodology. BMC Vet Res. (2012) 8:151. doi: 10.1186/1746-6148-8-151
69. Sihvo HK, Simola O, Munsterhjelm C, Syrjä P. Systemic spread of infection in tail-bitten pigs. J Comp Pathol. (2012) 1:73–8.
70. van Staaveren N, Vale AP, Manzanilla EG, Teixeira DL, Leonard FC, Hanlon A, et al. Relationship between tail lesions and lung health in slaughter pigs. Prev Vet Med. (2016) 127:21–6. doi: 10.1016/j.prevetmed.2016.03.004
71. Kritas SK, Morrison RB. An observational study on tail-biting in commercial grower-finisher barns. J Swine Health Prod. (2004) 12:17–22
72. Pandolfi F, Stoddart K, Wainwright N, Kyriazakis I, Edwards SA. The ‘Real Welfare’ scheme: benchmarking welfare outcomes for commercially farmed pigs. Animal. (2017) 11:1816–24. doi: 10.1017/S1751731117000246
73. Hälli O, Laurila T, Riihimäki A, Heinonen M. Post mortem inspection criteria for pleuritis in swine differs between slaughterhouses. In: 4th European Symposium of Porcine Health Management, P059. (2012). p. 141.
74. Harley S, More S, Boyle L, Connell NO, Hanlon A. Good animal welfare makes economic sense: potential of pig abattoir meat inspection as a welfare surveillance tool. Irish Vet J. (2012) 65:11. doi: 10.1186/2046-0481-65-11
75. Fablet C, Marois C, Dorenlor V, Eono F, Eveno E, Jolly J, et al. Bacterial pathogens associated with lung lesions in slaughter pigs from 125 herds. Res. Vet Sci. (2012) 93:627–30. doi: 10.1016/j.rvsc.2011.11.002
76. Maes D, Sibila M, Kuhnert P, Segalés J, Haesebrouck F, Pieters M. Update on Mycoplasma hyopneumoniae infections in pigs: knowledge gaps for improved disease control. Transbound Emerg Dis. (2018) 65(Suppl. 1):110–24. doi: 10.1111/tbed.12677
77. Dantzer R. Cytokine-induced sickness behavior: where do we stand? Brain Behav Immun. (2001) 15:7–24. doi: 10.1006/brbi.2000.0613
78. Dantzer R, O'connor JC, Freund GG, Johnson RW, Kelley KW. From inflammation to sickness and depression: when the immune system subjugates the brain. Nat Rev Neurosci. (2008) 9:46–56. doi: 10.1038/nrn2297
79. Johnson RW. The concept of sickness behavior: a brief chronological account of four key discoveries. Vet Immunol Immunopathol. (2002) 87:443–50. doi: 10.1016/S0165-2427(02)00069-7
80. Prunier A, Averos X, Dimitrov I, Edwards S, Hillmann E, Holinger M, et al. Early life predisposing factors for biting in pigs. Animal. (2020) 14:570–87. doi: 10.1017/S1751731119001940
81. Prunier A, Heinonen M, Quesnel H. High physiological demands in intensively raised pigs: impact on health and welfare. Animal. (2010) 4:886–98. doi: 10.1017/S175173111000008X
82. Fablet C, Dorenlor V, Eono F, Eveno E, Jolly JP, Portier F, et al. Noninfectious factors associated with pneumonia and pleuritis in slaughtered pigs from 143 farrow-to-finish pig farms. Prev Vet Med. (2012) 104:271–80. doi: 10.1016/j.prevetmed.2011.11.012
83. Grümpel A, Krieter J, Dippel S. Reducing estimated tail biting risk in German weaner pigs using a management tool. Vet J. (2019) 254:105406. doi: 10.1016/j.tvjl.2019.105406
84. Grümpel A, Krieter J, Veit C, Dippel S. Factors influencing the risk for tail lesions in weaner pigs (Sus scrofa). Livest Sci. (2018) 216:219–26. doi: 10.1016/j.livsci.2018.09.001
85. Telkänranta H, Bracke MBM, Valros A. Fresh wood, plastic pipe or metal chain-which objects reduce tail and ear biting on commercial pig farms? In: 48th Congress of the International Society for Applied Ethology. ISAE (2014). p. 282–2.
86. Larsen MLV, Andersen H, Pedersen L. Which is the most preventive measure against tail damage in finisher pigs: tail docking, straw provision or lowered stocking density? Animal. (2018)12:1260–7. doi: 10.1017/S175173111700249X
87. Kallio P, Janczak AM, Valros A, Edwards S, Heinonen M. Case control study on environmental, nutritional and management-based risk factors for tail-biting in long-tailed pigs. Anim Welfare. (2018) 7:21–34. doi: 10.7120/09627286.27.1.021
88. Scollo A, Gottardo F, Contiero B, Edwards SA. A cross-sectional study for predicting tail biting risk in pig farms using classification and regression tree analysis. Prev Vet Med. (2017) 146:114–20. doi: 10.1016/j.prevetmed.2017.08.001
89. Smulders D, Hautekiet V, Verbeke G, Geers R. Tail and ear biting lesions in pigs: an epidemiological study. Anim Welfare. (2008) 17:61–9.
90. Paul E, Moinard C, Green L, Mendl M. Farmers' attitudes to methods for controlling tail biting in pigs. Vet Rec. (2007) 160:803. doi: 10.1136/vr.160.23.803
91. Valros A, Barber C. Producer perceptions of the prevention of tail biting on UK farms: association to bedding use and tail removal proportion. Animals. (2019) 9:628. doi: 10.3390/ani9090628
92. Calderón Díaz JA, Boyle LA, Diana A, Leonard FC, Moriarty JP, McElroy MC, et al. Early life indicators predict mortality, illness, reduced welfare and carcass characteristics in finisher pigs. Prev Vet Med. (2017) 146:94–102. doi: 10.1016/j.prevetmed.2017.07.018
93. Zonderland J, Kemp B, Bracke M, Den Hartog L, Spoolder H. Individual piglets' contribution to the development of tail biting. Animal. (2011) 5:601–7. doi: 10.1017/S1751731110002132
94. Zonderland JJ, Bracke MB, den Hartog LA, Kemp B, Spoolder HA. Gender effects on tail damage development in single-or mixed-sex groups of weaned piglets. Livest Sci. (2010) 129:151–8. doi: 10.1016/j.livsci.2010.01.018
95. Baxter EM, Jarvis S, Palarea-Albaladejo J, Edwards SA. The weaker sex? The propensity for male-biased piglet mortality. PLoS ONE. (2012) 7:e30318. doi: 10.1371/journal.pone.0030318
96. Corrégé I, Pirouelle H, Gaudré D, Le Tiran M-H. La Maladie de l'Amaigrissement du Porcelet (MAP). J Recherche Porcine. (2001) 33:283–90.
97. Lundeheim N. Health disorders and growth performance at a Swedish pig progeny testing station. Acta Agric Scand. (1988) 38:77–88. doi: 10.1080/00015128809436590
98. Zupan M, Framstad T, Zanella AJ. Behaviour, heart rate, and heart rate variability in pigs exposed to novelty. Rev Bras Zootecnia. (2016) 45:121–9. doi: 10.1590/S1806-92902016000300006
99. Zupan M, Zanella AJ. Peripheral regulation of stress and fear responses in pigs from tail-biting pens. Rev Bras Zootecnia. (2017) 46:33–38. doi: 10.1590/s1806-92902017000100006
100. Reimert I, Bolhuis JE, Kemp B, Rodenburg TB. Indicators of positive and negative emotions and emotional contagion in pigs. Physiol Behav. (2013) 109:42–50. doi: 10.1016/j.physbeh.2012.11.002
101. Reimert I, Bolhuis JE, Kemp B, Rodenburg TB. Social support in pigs with different coping styles. Physiol Behav. (2014) 129:221–9. doi: 10.1016/j.physbeh.2014.02.059
102. Moberg GP, Mench JA. The Biology of Animal Stress: Basic Principles and Implications for Animal Welfare. Wallingford: CABI (2000). doi: 10.1079/9780851993591.0000
103. Llamas Moya S, Boyle L, Lynch P, Arkins S. Pro-inflammatory cytokine and acute phase protein responses to low-dose lipopolysaccharide (LPS) challenge in pigs. Anim Sci. (2006) 82:527–34. doi: 10.1079/ASC200665
104. Bench CJ, Gonyou HW. Temperature preference in piglets weaned at 12–14 days of age. Can J Anim Sci. (2007) 87:299–302. doi: 10.4141/CJAS06040
105. Breuer K, Sutcliffe M, Mercer J, Rance K, Beattie V, Sneddon I, et al. The effect of breed on the development of adverse social behaviours in pigs. Appl Anim Behav Sci. (2003) 84:59–74. doi: 10.1016/S0168-1591(03)00147-3
106. Breuer K, Sutcliffe M, Mercer J, Rance K, O'Connell N, Sneddon I, et al. Heritability of clinical tail-biting and its relation to performance traits. Livestock Prod Sci. (2005) 93:87–94. doi: 10.1016/j.livprodsci.2004.11.009
107. Clapperton M, Glass E, Bishop S. Pig peripheral blood mononuclear leucocyte subsets are heritable and genetically correlated with performance. Animal. (2008) 2:1575–84. doi: 10.1017/S1751731108002929
108. Rauw W, Kanis E, Noordhuizen-Stassen E, Grommers F. Undesirable side effects of selection for high production efficiency in farm animals: a review. Livestock Prod Sci. (1998) 56:15–33. doi: 10.1016/S0301-6226(98)00147-X
109. Stern S, Lundeheim N, Johansson K, Andersson K. Osteochondrosis and leg weakness in pigs selected for lean tissue growth rate. Livestock Prod Sci. (1995) 44:45–52. doi: 10.1016/0301-6226(95)00056-Q
110. Hessing M, Geudeke M, Scheepens C, Tielen M, Schouten W, Wiepkema P. Mucosal lesions in the pars esophagus in swine: prevalence and the effect of stress. Tijdschrift Voor Diergeneeskunde. (1992) 117:445–50.
111. Zonderland JJ, Schepers F, Bracke M, Den Hartog L, Kemp B, Spoolder H. Characteristics of biter and victim piglets apparent before a tail-biting outbreak. Animal. (2011) 5:767–75. doi: 10.1017/S1751731110002326
112. Van de Weerd H, Docking C, Day J, Edwards S. The development of harmful social behaviour in pigs with intact tails and different enrichment backgrounds in two housing systems. Anim Sci. (2005) 80:289298. doi: 10.1079/ASC40450289
113. Munsterhjelm C, Heinonen M, Valros A. Can tail-in-mouth behaviour in weaned piglets be predicted by behaviour and performance? Appl Anim Behav Sci. (2016) 184:16–24. doi: 10.1016/j.applanim.2016.08.013
114. Valros A, Salib V, Hällib O, Saarib S, Heinonen M. Does weight matter? Exploring links between birth weight, growth and pig-directed manipulative behaviour in growing-finishing pigs. Appl Anim Behav Sci. (2021) 245. doi: 10.1016/j.applanim.2021.105506
115. Hakansson F, Houe H. Risk factors associated with tail damage in conventional non-docked pigs throughout the lactation and rearing period. Prev Vet Med. (2020) 184:105160. doi: 10.1016/j.prevetmed.2020.105160
116. Li N, Huang S, Jiang L, Wang W, Li T, Zuo B, et al. Differences in the gut microbiota establishment and metabolome characteristics between low-and normal-birth-weight piglets during early-life. Front Microbiol. (2018) 9:1798. doi: 10.3389/fmicb.2018.01798
117. Hales J, Moustsen VA, Nielsen MB, Hansen CF. Individual physical characteristics of neonatal piglets affect preweaning survival of piglets born in a noncrated system. J Anim Sci. (2013) 91:4991–5003. doi: 10.2527/jas.2012-5740
118. Cabrera RA, Lin X, Campbell JM, Moeser AJ, Odle J. Influence of birth order, birth weight, colostrum and serum immunoglobulin G on neonatal piglet survival. J Anim Sci Biotechnol. (2012) 3:1–10. doi: 10.1186/2049-1891-3-42
119. Gardner I, Hird D. Risk factors for development of foot abscess in neonatal pigs. J Am Vet Med Assoc. (1994) 204:1062–7.
120. Feldpausch JA, Jourquin J, Bergstrom JR, Bargen JL, Bokenkroger CD, Davis DL, et al. Birth weight threshold for identifying piglets at risk for preweaning mortality. Transl Anim Sci. (2019) 3:633–40. doi: 10.1093/tas/txz076
121. Gardner I, Hird D. Host determinants of pneumonia in slaughter weight swine. Am J Vet Res. (1990) 51:1306–11.
122. Baumgartner M, Brugnera E, Sydler T, Bürgi E, Hässig M, Sidler X. Risk factors causing postweaning multisystemic wasting syndrome (PMWS) onset in Swiss pig farms. Schweiz Arch Tierheilkd. (2012) 154:429–36. doi: 10.1024/0036-7281/a000379
123. Camerlink I, Bijma P, Kemp B, Bolhuis JE. Relationship between growth rate and oral manipulation, social nosing, and aggression in finishing pigs. Appl Anim Behav Sci. (2012) 142:11–7. doi: 10.1016/j.applanim.2012.09.004
124. Kuchling S, Schleicher C, Raith J, Koefer J. Analysis of the impact of animal health parameters on the average daily net gain in pigs for fattening. Berliner und Munchener Tierarztliche Wochenschrift. (2015) 128:193–9.
125. Beattie VE, Breuer K, O'Connell NE, Sneddon IA, Mercer JT, Rance KA, et al. Factors identifying pigs predisposed to tail biting. Anim Sci. (2005) 80:307–12. doi: 10.1079/ASC40040307
126. Diana A, Manzanilla EG, Calderón Díaz JA, Leonard FC, Boyle LA. Do weaner pigs need in-feed antibiotics to ensure good health and welfare? PLoS ONE. (2017) 12:e0185622. doi: 10.1371/journal.pone.0185622
127. Diana A, Boyle LA, Manzanilla EG, Leonard FC, Díaz JAC. Ear, tail and skin lesions vary according to different production flows in a farrow-to-finish pig farm. Porcine Health Manag. (2019) 5:1–9. doi: 10.1186/s40813-019-0126-9
128. Calderón Díaz JA, Diana A, Boyle LA, Leonard FC, McElroy M, McGettrick S, et al. Delaying pigs from the normal production flow is associated with health problems and poorer performance. Porcine Health Manag. (2017) 3:1–6. doi: 10.1186/s40813-017-0061-6
129. Quinn AJ, Green LE, Lawlor PG, Boyle LA. The effect of feeding a diet formulated for developing gilts between 70kg and ~140kg on lameness indicators and carcass traits. Livest Sci. (2015) 174:87–95. doi: 10.1016/j.livsci.2014.12.016
130. Bolhuis JE, Schouten WG, Schrama JW, Wiegant VM. Behavioural development of pigs with different coping characteristics in barren and substrate-enriched housing conditions. Appl Anim Behav Sci. (2005) 93:213–28. doi: 10.1016/j.applanim.2005.01.006
131. Luo L, Reimert I, Middelkoop A, Kemp B, Bolhuis JE. Effects of early and current environmental enrichment on behavior and growth in pigs. Front Vet Sci. (2020) 7:268. doi: 10.3389/fvets.2020.00268
132. Jansen J, Bolhuis JE, Schouten WG, Spruijt BM, Wiegant VM. Spatial learning in pigs: effects of environmental enrichment and individual characteristics on behaviour and performance. Anim Cogn. (2009) 12:303–15. doi: 10.1007/s10071-008-0191-y
133. Asher L, Friel M, Griffin K, Collins LM. Mood and personality interact to determine cognitive biases in pigs. Biol Lett. (2016) 12:20160402. doi: 10.1098/rsbl.2016.0402
134. Ursinus WW, Van Reenen CG, Reimert I, Bolhuis JE. Tail biting in pigs: blood serotonin and fearfulness as pieces of the puzzle? PLoS ONE. (2014) 9:e107040. doi: 10.1371/journal.pone.0107040
135. Chu Q, Liang T, Fu L, Li H, Zhou B. Behavioural genetic differences between Chinese and European pigs. J Genet. (2017) 96:707–15. doi: 10.1007/s12041-017-0826-3
136. Valros A, Palander P, Heinonen M, Munsterhjelm C, Brunberg E, Keeling L, et al. Evidence for a link between tail biting and central monoamine metabolism in pigs (Sus scrofa domestica). Physiol Behav. (2015) 143:151–7. doi: 10.1016/j.physbeh.2015.02.049
137. Bolhuis JE, Schouten WG, Schrama JW, Wiegant VM. Effects of rearing and housing environment on behaviour and performance of pigs with different coping characteristics. Appl Anim Behav Sci. (2006) 101:68–85. doi: 10.1016/j.applanim.2006.01.001
138. Hessing MJ, Hagelsø AM, Schouten WG, Wiepkema PR, Van Beek JA. Individual behavioral and physiological strategies in pigs. Physiol Behav. (1994) 55:39–46. doi: 10.1016/0031-9384(94)90007-8
139. Hessing M, Schouten W, Wiepkema P, Tielen M. Implications of individual behavioural characteristics on performance in pigs. Livestock Prod Sci. (1994) 40:187–96. doi: 10.1016/0301-6226(94)90048-5
140. Hessing M, Coenen G, Vaiman M, Renard C. Individual differences in cell-mediated and humoral immunity in pigs. Vet Immunol Immunopathol. (1995) 45:97–113. doi: 10.1016/0165-2427(94)05338-S
141. Luo L, Jansen CA, Bolhuis JE, Arts JA, Kemp B, Parmentier HK. Early and later life environmental enrichment affect specific antibody responses and blood leukocyte subpopulations in pigs. Physiol Behav. (2020) 217:112799. doi: 10.1016/j.physbeh.2020.112799
142. Kanitz E, Tuchscherer M, Otten W, Tuchscherer A, Zebunke M, Puppe B. Coping style of pigs is associated with different behavioral, neurobiological and immune responses to stressful challenges. Front Behav Neurosci. (2019) 13:173. doi: 10.3389/fnbeh.2019.00173
143. Oster M, Scheel M, Muráni E, Ponsuksili S, Zebunke M, Puppe B, et al. The Fight-Or-Flight response is associated with PBMC expression profiles related to immune defence and recovery in swine. PLoS ONE. (2015) 10:e0120153. doi: 10.1371/journal.pone.0120153
144. Schrama JW, Schouten JM, Swinkels JWGM, Gentry JL, de Vries Reilingh G, Parmentier HK. Effect of hemoglobin status on humoral immune response of weanling pigs differing in coping styles. J Anim Sci. (1997) 75:2588–96. doi: 10.2527/1997.75102588x
145. Bolhuis JE, Parmentier H, Schouten W, Schrama J, Wiegant V. Effects of housing and individual coping characteristics on immune responses of pigs. Physiol Behav. (2003) 79:289–96. doi: 10.1016/S0031-9384(03)00090-8
146. Holling C, grosse Beilage E, Vidondo B, Nathues C. Provision of straw by a foraging tower–effect on tail biting in weaners and fattening pigs. Porcine Health Manag. (2017) 3:1–14. doi: 10.1186/s40813-017-0052-7
147. Sällvik K, Walberg K. The effects of air velocity and temperature on the behaviour and growth of pigs. J Agricult Eng Res. (1984) 30:305–12. doi: 10.1016/S0021-8634(84)80031-1
148. Kongsted H, Sørensen JT. Lesions found at routine meat inspection on finishing pigs are associated with production system. Vet J. (2017) 223:21–6. doi: 10.1016/j.tvjl.2017.04.016
149. Aberle ED, Merkel RA, Forrest JC, Alliston CW. Physiological responses of stress susceptible and stress resistant pigs to heat stress. J AnimSci. (1974) 13:954–9. doi: 10.2527/jas1974.385954x
150. Ross JW, Hale BJ, Gabler NK, Rhoads RP, Keating AF, Baumgard LH. Physiological consequences of heat stress in pigs. Anim Prod Sci. (2015) 55:1381–90. doi: 10.1071/AN15267
151. Le Dividich J, Herpin P. Effects of climatic conditions on the performance, metabolism and health status of weaned piglets: a review. Livestock Prod Sci. (1994) 38:79–90. doi: 10.1016/0301-6226(94)90052-3
152. Armstrong WD, Cline TR. Effects of various nutrient levels and enviromental temperatures on the incidence of colibacillary diarrhea in pigs: intestinal fistulation and titration studies. J Anim Sci. (1977) 45:1042–50. doi: 10.2527/jas1977.4551042x
153. Le Dividich J. Effects of environmental temperature on the growth rates of early-weaned piglets. Livestock Prod Sci. (1981) 8:75–86. doi: 10.1016/0301-6226(81)90032-4
154. Burrough E. Swine dysentery: etiopathogenesis and diagnosis of a reemerging disease. Vet Pathol. (2017) 54:22–31. doi: 10.1177/0300985816653795
155. Stark K. Epidemiological investigation of the influence of environmental risk factors on respiratory diseases in swine—a literature review. Vet J. (2000) 159:37–56. doi: 10.1053/tvjl.1999.0421
156. Geers R, Dellaert B, Goedseels V, Hoogerbrugge A, Vranken E, Maes F, et al. An assessment of optimal air temperatures in pig houses by the quantification of behavioural and health-related problems. Anim Prod. (1989) 48:571–8. doi: 10.1017/S0003356100004098
157. Done SH. Environmental factors affecting the severity of pneumonia in pigs. Vet Rec. (1991) 128:582–6. doi: 10.1136/vr.128.25.582
158. Carroll J, Burdick N, Chase C Jr, Coleman S, Spiers D. Influence of environmental temperature on the physiological, endocrine, and immune responses in livestock exposed to a provocative immune challenge. Domestic Anim Endocrinol. (2012) 43:146–53. doi: 10.1016/j.domaniend.2011.12.008
159. Heidemann SM, Glibetic M. Heat stress protects against lung injury in the neutropenic, endotoxemic rat. Inflammation. (2005) 29:47–53. doi: 10.1007/s10753-006-8969-4
160. Pearce S, Gabler N, Ross J, Escobar J, Patience J, Rhoads R, et al. The effects of heat stress and plane of nutrition on metabolism in growing pigs. J Anim Sci. (2013) 91:2108–18. doi: 10.2527/jas.2012-5738
161. Smith JH, Wathes CM, Baldwin BA. The preference of pigs for fresh air over ammoniated air. Appl Anim Behav Sci. (1996) 49:417–24. doi: 10.1016/0168-1591(96)01048-9
162. Wathes CM, Jones JB, Kristensen HH, Jones EKM, Webster AJF. Aversiveness of Atmospheric ammonia to pigs and domestic fowl. Trans ASAE. (2002) 85:1–15.
163. Groot Koerkamp P, Metz J, Uenk G, Phillips V, Holden M, Sneath R, et al. Concentrations and emissions of ammonia in livestock buildings in Northern Europe. J Agric Eng Res. (1998) 70:79–95. doi: 10.1006/jaer.1998.0275
164. Drummond JG, Curtis SE, Simon J, Norton HW. Effects of aerial ammonia on growth and health of young pigs. J Anim Sci. (1980) 50:1085–91. doi: 10.2527/jas1980.5061085x
165. Von Borell E, Eslinger K, Schnitz A, Zhao Y, Mitloehner F. Acute and prolonged effects of ammonia on hematological variables, stress responses, performance, and behavior of nursery pigs. J Swine Health Prod. (2007) 15:137–45.
166. Done S, Chennells D, Gresham A, Williamson S, Hunt B, Taylor L, et al. Clinical and pathological responses of weaned pigs to atmospheric ammonia and dust. Vet Rec. (2005) 157:71–80. doi: 10.1136/vr.157.3.71
167. Park J, Friendship RM, Poljak Z, DeLay J, Slavic D, Dewey CE. An investigation of ear necrosis in pigs. Can Vet J. (2013) 54:491–5.
168. Pointon A, Heap P, McCloud P. Enzootic pneumonia of pigs in South Australia—factors relating to incidence of disease. Austral Vet J. (1985) 62:98–101. doi: 10.1111/j.1751-0813.1985.tb14149.x
169. Madec F, Josse J. The risk factors of respiratory diseases on fatteners in intensive breeding-finishing [pig] units. In: 8. International Pig Veterinary Society Congress. Gent, Belgium (1985). p. 27–31.
170. Donham KJ. Association of environmental air contaminants with disease and productivity in swine. Am J Vet Res. (1991) 52:1723–30.
171. Köfer J, Awad-Masalmeh M, Thiemann G. The effect of husbandry, management and stall climate on lung changes in swine. Deutsche Tierarztliche Wochenschrift. (1993) 100:319–22.
172. Madec F, Bridoux N, Bounaix S, Jestin A. Measurement of digestive disorders in the piglet at weaning and related risk factors. Prev Vet Med. (1998) 35:53–72. doi: 10.1016/S0167-5877(97)00057-3
173. Scheepens CJM, Hessing MJC, Laarakker E, Schouten WGP, Tielen MJM. Influences of intermittent daily draught on the behaviour of weaned pigs. Appl Anim Behav Sci. (1991) 31:69–82. doi: 10.1016/0168-1591(91)90154-P
174. Scheepens CJM, Tielen MJM, Hessing MJC. Influence of daily intermittent draught on the health status of weaned pigs. Livestock Prod Sci. (1991) 29:241–54. doi: 10.1016/0301-6226(91)90069-3
175. Maes D, Chiers K, Haesebrouck F, Laevens H, Verdonck M, de Kruif A. Herd factors associated with the seroprevalences of Actinobacillus pleuropneumoniae serovars 2, 3 and 9 in slaughter pigs from farrow-to-finish pig herds. Vet Res. (2001) 32:409–19. doi: 10.1051/vetres:2001133
176. Chantziaras I, De Meyer D, Vrielinck L, Van Limbergen T, Pineiro C, Dewulf J, et al. Environment-, health-, performance-and welfare-related parameters in pig barns with natural and mechanical ventilation. Prev Vet Med. (2020) 183:105150. doi: 10.1016/j.prevetmed.2020.105150
177. Munsterhjelm C, Heinonen M, Valros A. Application of the Welfare Quality® animal welfare assessment system in Finnish pig production, part II: associations between animal-based and environmental measures of welfare. Anim Welfare. (2015) 24:161–72. doi: 10.7120/09627286.24.2.161
178. Wallenbeck A, Keeling L. Using data from electronic feeders on visit frequency and feed consumption to indicate tail biting outbreaks in commercial pig production. J Anim Sci. (2013) 91:2879–84. doi: 10.2527/jas.2012-5848
179. Spoolder H, Edwards S, Corning S. Effects of group size and feeder space allowance on welfare in finishing pigs. Anim Sci. (1999) 69:481–9. doi: 10.1017/S135772980005133X
180. Holmgren N, Lundeheim N. Risk factors for tail biting. In: 18th IPVS Congress, International Pig Veterinary Society. Hamburg: IPVS (2004). p. 786.
181. Laskoski F, Faccin JE, Vier CM, Gonçalves MA, Orlando UA, Kummer R, et al. Effects of pigs per feeder hole and group size on feed intake onset, growth performance, and ear and tail lesions in nursery pigs with consistent space allowance. J Swine Health Prod. (2019) 27:12–8.
182. Smith WJ, Edwards SA. Ulceration of the pars oesophagea—the role of a factor in wheat. Pig J. (1996) 36:194–200.
183. Nielsen EK, Ingvartsen KL. Effect of cereal type, disintegration method and pelleting on stomach content, weight and ulcers and performance in growing pigs. Livestock Prod Sci. (2000) 66:271–82. doi: 10.1016/S0301-6226(00)00165-2
184. Smith WJ, Nelson EP. Grower scour/non-specific colitis. Vet Rec. (1987) 121:334. doi: 10.1136/vr.121.14.334-b
185. Thomson J. Feed-associated colitis of growing pigs and its interaction with enteric infections. Acta Sci Vet. (2009) 37:s1–9.
186. Hanrahan T. Whey solids in the diet of growing-finishing pigs: 2. concentrated whey as a feed for pigs. Irish J Agricult Res. (1971) 10:9–16.
187. Jones J. An intestinal haemorrhage syndrome in pigs. Br Vet J. (1967) 123:286–94. doi: 10.1016/S0007-1935(17)39905-0
188. Grahofer A, Gurtner C, Nathues H. Haemorrhagic bowel syndrome in fattening pigs. Porcine Health Manag. (2017) 3:1–6. doi: 10.1186/s40813-017-0074-1
189. Honeck A, Ahlhorn J, Burfeind O, Gertz M, Grosse Beilage E, Hasler M, et al. Influence on tail-biting in weaning pigs of crude fibre content and different crude fibre components in pigs' rations. J Agricult Sci. (2020) 158:233–40. doi: 10.1017/S0021859620000404
190. Naya A, Gertz M, Hasler M, Beilage EG, Krieter J. Does a higher content of fibre in the piglet diet have an influence on tail biting in growing pigs? Livest Sci. (2019) 223:133–7. doi: 10.1016/j.livsci.2019.03.010
191. van der Peet-Schwering C, Dirx N, Kluivers-Poodt M, Binnendijk G, Bolhuis L, van der Peet G. Effect van Voersamenstelling op Bijtgedrag bij Varkens. Wageningen: Wageningen Livestock Research (2017). doi: 10.18174/402570
192. Chou J-Y, O'Driscoll K, Sandercock DA, D'Eath RB. Can increased dietary fibre level and a single enrichment device reduce the risk of tail biting in undocked growing-finishing pigs in fully slatted systems? PLoS ONE. (2020) 15:e0241619. doi: 10.1371/journal.pone.0241619
193. Dirkzwager A, Elbers A, Van der Aar P, Vos J. Effect of particle size and addition of sunflower hulls to diets on the occurrence of oesophagogastric lesions and performance in growing-finishing pigs. Livestock Prod Sci. (1998) 56:53–60. doi: 10.1016/S0301-6226(98)00143-2
194. Holinger M, Früh B, Stoll P, Kreuzer M, Hillmann E. Grass silage for growing-finishing pigs in addition to straw bedding: effects on behaviour and gastric health. Livest Sci. (2018) 218:50–7. doi: 10.1016/j.livsci.2018.10.012
195. Forsyth D, Yoshizawa T, Morooka N, Tuite J. Emetic and refusal activity of deoxynivalenol to swine. Appl Environ Microbiol. (1977) 34:547–52. doi: 10.1128/aem.34.5.547-552.1977
196. Bauer J, Heinritzi K, Gareis M, Gedek B. Changes in the genital tract of female swine after feeding with practice-relevant amounts of zearalenone. Tierarztliche Praxis. (1987) 15:33–6.
197. Morgavi D, Riley R. An historical overview of field disease outbreaks known or suspected to be caused by consumption of feeds contaminated with Fusarium toxins. Anim Feed Sci Technol. (2007) 137:201–12. doi: 10.1016/j.anifeedsci.2007.06.002
199. Van Limbergen T, Devreese M, Croubels S, Broekaert N, Michiels A, De Saeger S, et al. Role of mycotoxins in herds with and without problems with tail necrosis in neonatal pigs. Vet Rec. (2017) 181:539. doi: 10.1136/vr.104385
200. López TA, Campero CM, Chayer R, de Hoyos M. Ergotism and photosensitization in swine produced by the combined ingestion of Claviceps purpurea sclerotia and Ammi majus seeds. J Vet Diagn Invest. (1997) 9:68–71. doi: 10.1177/104063879700900112
201. Pierron A, Alassane-Kpembi I, Oswald ssane-Kpembi IP. Impact of mycotoxin on immune response and consequences for pig health. Anim Nutr. (2016) 2:63–8. doi: 10.1016/j.aninu.2016.03.001
202. Stoev SD, Gundasheva D, Zarkov I, Mircheva T, Zapryanova D, Denev S, et al. Experimental mycotoxic nephropathy in pigs provoked by a mouldy diet containing ochratoxin A and fumonisin B1. Exp Toxicol Pathol. (2012) 64:733–41. doi: 10.1016/j.etp.2011.01.008
203. Antonissen G, Martel A, Pasmans F, Ducatelle R, Verbrugghe E, Vandenbroucke V, et al. The impact of Fusarium mycotoxins on human and animal host susceptibility to infectious diseases. Toxins. (2014) 6:430–52. doi: 10.3390/toxins6020430
204. Savard C, Provost C, Alvarez F, Pinilla V, Music N, Jacques M, et al. Effect of deoxynivalenol (DON) mycotoxin on in vivo and in vitro porcine circovirus type 2 infections. Vet Microbiol. (2015) 176:257–67. doi: 10.1016/j.vetmic.2015.02.004
205. Pierron A, Alassane-Kpembi I, Oswald IP. Impact of two mycotoxins deoxynivalenol and fumonisin on pig intestinal health. Porcine Health Manag. (2016) 2:21. doi: 10.1186/s40813-016-0041-2
206. Joens LA, Pier AC, Cutlip RC. Effects of aflatoxin consumption on the clinical course of swine dysentery. Am J Vet Res. (1981) 42:1170–2.
207. Devriendt B, Gallois M, Verdonck F, Wache Y, Bimczok D, Oswald I, et al. The food contaminant fumonisin B1 reduces the maturation of porcine CD11R1+ intestinal dendritic cells, leading to reduced antigen-specific immune responses and a prolonged intestinal ETEC infection. Vet. Res. (2009) 40:40. doi: 10.1051/vetres/2009023
208. Pósa R, Magyar T, Stoev S, Glávits R, Donkó T, Repa I, et al. Use of computed tomography and histopathologic review for lung lesions produced by the interaction between Mycoplasma hyopneumoniae and fumonisin mycotoxins in pigs. Vet Pathol. (2013) 50:971–9. doi: 10.1177/0300985813480510
209. Hunter EJ, Jones TA, Guise HJ, Penny RH, Hoste S. The relationship between tail biting in pigs, docking procedure and other management practices. Vet J. (2001) 161:72–9. doi: 10.1053/tvjl.2000.0520
210. Hansen LL, Hagelsø AM, Madsen A. Behavioural results and performance of bacon pigs fed “ad libitum” from one or several self-feeders. Appl Anim Ethol. (1982) 8:307–333. doi: 10.1016/0304-3762(82)90065-7
211. Botermans JA, Svendsen J. Effect of feeding environment on performance, injuries and behaviour in growing-finishing pigs: group-based studies. Acta Agricult Scand A Anim Sci. (2000) 50:237–49. doi: 10.1080/090647000750069430
212. Georgsson L, Svendsen J. One or two feeders for groups of 16 growing–finishing pigs: effects on health and production. Acta Agricult Scand A Anim Sci. (2001) 51:257–64. doi: 10.1080/09064700152717245
213. Hurnik D, Dohoo IR, Bate LA. Types of farm management as risk factors for swine respiratory disease. Prev Vet Med. (1994) 20:147–57. doi: 10.1016/0167-5877(94)90113-9
214. Hurnik D, Dohoo IR, Donald A, Robinson NP. Factor analysis of swine farm management practices on Prince Edward Island. Prev Vet Med. (1994) 20:135. doi: 10.1016/0167-5877(94)90112-0
215. Stärk K, Pfeiffer D, Morris R. Risk factors for respiratory diseases in New Zealand pig herds. N Zeal Vet J. (1998) 46:3–10. doi: 10.1080/00480169.1998.36043
216. Rantzer D, Svendsen J, Weström B. Effects of a strategic feed restriction on pig performance and health during the post-weaning period. Acta Agricult Scand A Anim Sci. (1996) 46:219–26. doi: 10.1080/09064709609415874
217. Laine TM, Lyytikäinen T, Yliaho M, Anttila M. Risk factors for post-weaning diarrhoea on piglet producing farms in Finland. Acta Vet Scand. (2008) 50:21. doi: 10.1186/1751-0147-50-21
218. Robertson ID, Accioly JM, Moore KM, Driesen SJ, Pethick DW, Hampson DJ. Risk factors for gastric ulcers in Australian pigs at slaughter. Prev Vet Med. (2002) 53:293–303. doi: 10.1016/S0167-5877(01)00286-0
219. Bracke MBM, Hulsegge B, Keeling L, Blokhuis HJ. Decision support system with semantic model to assess the risk of tail biting in pigs: 1. Modelling. Appl Anim Behav Sci. (2004) 87:31–44. doi: 10.1016/j.applanim.2003.12.005
220. Hessel EF, Wülbers-Mindermann M, Berg C, Van den Weghe HF, Algers B. Influence of increased feeding frequency on behavior and integument lesions in growing-finishing restricted-fed pigs. J Anim Sci. (2006) 84:1526–34. doi: 10.2527/2006.8461526x
221. Amory JR, Mackenzie AM, Pearce GP. Factors in the housing environment of finisher pigs associated with the development of gastric ulcers. Vet Rec. (2006) 158:260–4. doi: 10.1136/vr.158.8.260
222. Farzan A, Friendship RM, Dewey CE, Warriner K, Poppe C, Klotins K. Prevalence of Salmonella spp. on Canadian pig farms using liquid or dry-feeding. Prev Vet Med. (2006) 73:241–54. doi: 10.1016/j.prevetmed.2005.09.003
223. Wondra K, Hancock J, Behnke K, Stark C. Effects of mill type and particle size uniformity on growth performance, nutrient digestibility, and stomach morphology in finishing pigs. J Anim Sci. (1995) 73:2564–73. doi: 10.2527/1995.7392564x
224. Kavanagh NT. Non-specific colitis: observations of methods of control and prevention at the farm level. In: 12th International Pig Veterinary Society Congress. The Hague: IPVS (1992). p. 522.
225. Thomson J, Smith W, Murray B. Investigations into field cases of porcine colitis with particular reference to infection with Serpulina pilosicoli. Vet Rec. (1998) 142:235–9. doi: 10.1136/vr.142.10.235
226. Jericho KW, Harries N. Dusty feed and acute respiratory disease in pigs. Can Vet J. (1975) 16:360–6.
227. Madsen TN, Kristensen AR. A model for monitoring the condition of young pigs by their drinking behaviour. Comput Electron Agricult. (2005) 48:138–54. doi: 10.1016/j.compag.2005.02.014
228. Maes D, Vrielinck J, Millet S, Janssens G, Deprez P. Urolithiasis in finishing pigs. Vet J. (2004) 168:317–22. doi: 10.1016/j.tvjl.2003.09.006
229. Studnitz M, Jensen MB, Pedersen LJ. Why do pigs root and in what will they root?: a review on the exploratory behaviour of pigs in relation to environmental enrichment. Appl Anim Behav Sci. (2007) 107:183–97. doi: 10.1016/j.applanim.2006.11.013
230. Ruiterkamp WA. The Behaviour of Fattening Pigs in Relation to Housing. Utrecht: University of Utrecht (1985).
231. Gottardo F, Scollo A, Contiero B, Bottacini M, Mazzoni C, Edwards SA. Prevalence and risk factors for gastric ulceration in pigs slaughtered at 170 kg. Animal. (2017) 11:2010–18. doi: 10.1017/S1751731117000799
232. Sanchez-Vazquez MJ, Smith R, Gunn GJ, Lewis F, Strachan WD, Edwards SA. The identification of risk factors for the presence of enzootic pneumonia-like lesions and pleurisy in slaughtered finishing pigs utilizing existing British pig industry data. Pig J. (2010) 63:25–33.
233. KilBride A, Gillman C, Ossent P, Green L. Impact of flooring on the health and welfare of pigs. Practice. (2009) 31:390–5. doi: 10.1136/inpract.31.8.390
234. Munsterhjelm C, Peltoniemi OAT, Heinonen M, Hälli O, Karhapää M, Valros A. Experience of moderate bedding affects behaviour of growing pigs. Appl Anim Behav Sci. (2009) 118:42–53. doi: 10.1016/j.applanim.2009.01.007
235. Zonderland JJ, Wolthuis-Fillerup M, Van Reenen CG, Bracke MB, Kemp B, Den Hartog LA, et al. Prevention and treatment of tail biting in weaned piglets. Appl Anim Behav Sci. (2008) 110:269–81. doi: 10.1016/j.applanim.2007.04.005
236. Guy J, Rowlinson P, Chadwick J, Ellis M. Behaviour of two genotypes of growing–finishing pig in three different housing systems. Appl Anim Behav Sci. (2002) 75:193–206. doi: 10.1016/S0168-1591(01)00197-6
237. Beattie V, Walker N, Sneddon I. An investigation of the effect of environmental enrichment and space allowance on the behaviour and production of growing pigs. Appl Anim Behav Sci. (1996) 48:151–8. doi: 10.1016/0168-1591(96)01031-3
238. Beattie V, Walker N, Sneddon I. Preference testing of substrates by growing pigs. Anim Welfare. (1998) 7:27–34.
239. Olsen AW. Behaviour of growing pigs kept in pens with outdoor runs: I. Effect of access to roughage and shelter on oral activities. Livestock Prod Sci. (2001) 69:255–64. doi: 10.1016/S0301-6226(01)00172-5
240. Buijs S, Muns R. A review of the effects of non-straw enrichment on tail biting in pigs. Animals. (2019) 9:824. doi: 10.3390/ani9100824
241. Nordkvist E, Häggblom P. Fusarium mycotoxin contamination of cereals and bedding straw at Swedish pig farms. Anim Feed Sci Technol. (2014) 198:231–7. doi: 10.1016/j.anifeedsci.2014.10.002
242. Gerez JR, Pinton P, Callu P, Grosjean F, Oswald IP, Bracarense APF. Deoxynivalenol alone or in combination with nivalenol and zearalenone induce systemic histological changes in pigs. Exp Toxicol Pathol. (2015) 67:89–98. doi: 10.1016/j.etp.2014.10.001
243. Bolhuis JE, van den Brand H, Staals S, Gerrits WJJ. Effects of pregelatinized vs. native potato starch on intestinal weight and stomach lesions of pigs housed in barren pens or on straw bedding. Livest Sci. (2007) 109:108–10. doi: 10.1016/j.livsci.2007.01.100
244. Di Martino G, Capello K, Scollo A, Gottardo F, Stefani AL, Rampin F, et al. Continuous straw provision reduces prevalence of oesophago-gastric ulcer in pigs slaughtered at 170 kg (heavy pigs). Res Vet Sci. (2013) 95:1271–3. doi: 10.1016/j.rvsc.2013.08.012
245. Herskin MS, Jensen HE, Jespersen A, Forkman B, Jensen MB, Canibe N, et al. Impact of the amount of straw provided to pigs kept in intensive production conditions on the occurrence and severity of gastric ulceration at slaughter. Res Vet Sci. (2016) 104:200–6. doi: 10.1016/j.rvsc.2015.12.017
246. Holinger M, Früh B, Stoll P, Graage R, Wirth S, Bruckmaier R, et al. Chronic intermittent stress exposure and access to grass silage interact differently in their effect on behaviour, gastric health and stress physiology of entire or castrated male growing-finishing pigs. Physiol Behav. (2018) 195:58–68. doi: 10.1016/j.physbeh.2018.07.019
247. Christensen G, Vraa-Andersen L, Mousing J. Causes of mortality among sows in Danish pig herds. Vet Rec. (1995) 137:395–9. doi: 10.1136/vr.137.16.395
248. Oostindjer M, Bolhuis JE, Mendl M, Held S, Gerrits W, van den Brand H, et al. Effects of environmental enrichment and loose housing of lactating sows on piglet performance before and after weaning. J Anim Sci. (2010) 88:3554–62. doi: 10.2527/jas.2010-2940
249. Chambers C, Powell L, Wilson E, Green LE. A postal survey of tail biting in pigs in south west England. Vet Rec. (1995) 136:147–8. doi: 10.1136/vr.136.6.147
250. Enøe C, Mousing J, Schirmer AL, Willeberg P. Infectious and rearing-system related risk factors for chronic pleuritis in slaughter pigs. Prev Vet Med. (2002) 54:337–49. doi: 10.1016/S0167-5877(02)00029-6
251. Hälli O, Haimi-Hakala M, Oliviero C, Heinonen M. Herd-level risk factors for chronic pleurisy in finishing pigs: a case-control study. Porcine Health Manag. (2020) 6:21. doi: 10.1186/s40813-020-00156-0
252. Ramis G, Gómez S, Pallarés F, Munoz Luna A. Comparison of the severity of esophagogastric, lung and limb lesions at slaughter in pigs reared under standard and enriched conditions. Anim Welfare. (2005) 14:27–34.
253. Goldberg TL, Weigel RM, Hahn EC, Scherba G. Associations between genetics, farm characteristics and clinical disease in field outbreaks of porcine reproductive and respiratory syndrome virus. Prev Vet Med. (2000) 43:293–302. doi: 10.1016/S0167-5877(99)00104-X
254. Bergman P, Munsterhjelm C, Virtala A-M, Peltoniemi O, Valros A, Heinonen M. Structural characterization of piglet producing farms and their sow removal patterns in Finland. Porcine Health Manag. (2019) 5:12. doi: 10.1186/s40813-019-0119-8
255. Postma M, Backhans A, Collineau L, Loesken S, Sjölund M, Belloc C, et al. The biosecurity status and its associations with production and management characteristics in farrow-to-finish pig herds. Animal. (2016) 10:478–89. doi: 10.1017/S1751731115002487
256. Madec F, Eveno E, Morvan P, Hamon L, Blanchard P, Cariolet R, et al. Post-weaning multisystemic wasting syndrome (PMWS) in pigs in France: clinical observations from follow-up studies on affected farms. Livestock Prod Sci. (2000) 63:223–33. doi: 10.1016/S0301-6226(99)00138-4
257. Madec F, Rose N, Grasland B, Cariolet R, Jestin A. Post-weaning multisystemic wasting syndrome and other PCV2-related problems in pigs: a 12-year experience. Transbound Emerg Dis. (2008) 55:273–83. doi: 10.1111/j.1865-1682.2008.01035.x
258. Rose N, Larour G, Le Diguerher G, Eveno E, Jolly JP, Blanchard P, et al. Risk factors for porcine post-weaning multisystemic wasting syndrome (PMWS) in 149 French farrow-to-finish herds. Prev Vet Med. (2003) 61:209–25. doi: 10.1016/j.prevetmed.2003.07.003
259. Dors A, Pomorska-Mól M, Czyzewska E, Wasyl D, Pejsak Z. Prevalence and risk factors for Lawsonia intracellularis, Brachyspira hyodysenteriae and Salmonella spp. in finishing pigs in Polish farrow-to-finish swine herds. Pol J Vet Sci. (2015) 18:825–31. doi: 10.1515/pjvs-2015-0107
260. Bäckström L, Bremer H. The relationship between disease incidences of fatteners registered at slaughter and environmental factors in herds. Nord Vet Med. (1978) 30:526–33.
261. Scheidt AB, Cline TR, Clark LK. The effect of all-in-all-out growing-finishing on the health of pigs. J Swine Health Prod. (1995) 3:202–5.
262. Cador C, Pol F, Hamoniaux M, Dorenlor V, Eveno E, Guyomarc'h C, et al. Risk factors associated with leg disorders of gestating sows in different group-housing systems: a cross-sectional study in 108 farrow-to-finish farms in France. Prev Vet Med. (2014) 116:102–10. doi: 10.1016/j.prevetmed.2014.05.004
263. McKee CI, Dumelow J. A review of the factors involved in developing effective non-slip floors for pigs. J Agricult Eng Res. (1995) 60:35–42. doi: 10.1006/jaer.1995.1094
264. Jørgensen B. Influence of floor type and stocking density on leg weakness, osteochondrosis and claw disorders in slaughter pigs. Anim Sci. (2003) 77:439–49. doi: 10.1017/S1357729800054382
265. Tuovinen VK, Gröhn Y, Saloniemi HS. Effect of non-requested consultation in poor-doing feeder pig finishing herds on daily gain and partial carcass condemnation—an intervention trial. Prev Vet Med. (1994) 20:1–10. doi: 10.1016/0167-5877(94)90103-1
266. Brandt P, Hakansson F, Jensen T, Nielsen MBF, Lahrmann HP, Hansen CF, et al. Effect of pen design on tail biting and tail-directed behaviour of finishing pigs with intact tails. Animal. (2020) 14:1034–42. doi: 10.1017/S1751731119002805
267. Sell A, Vidondo B, Wechsler B, Burla J, Nathues H. Risk factors for tail lesions in undocked fattening pigs reared on Swiss farms. Schweiz Arch Tierheilkd. (2020) 162:683–95. doi: 10.17236/sat00278
268. Chase-Topping ME, Gunn G, Strachan WD, Edwards SA, Smith WJ, Hillman K, et al. Epidemiology of porcine non-specific colitis on Scottish farms. Vet J. (2007) 173:353–60. doi: 10.1016/j.tvjl.2005.12.002
269. Street BR, Gonyou HW. Effects of housing finishing pigs in two group sizes and at two floor space allocations on production, health, behavior, physiological variables. J Anim Sci. (2008) 86:982–91. doi: 10.2527/jas.2007-0449
270. Friend TH, Knabe DA, Tanksley TD Jr. Behavior and performance of pigs grouped by three different methods at weaning. J Anim Sci. (1983) 57:1406–11. doi: 10.2527/jas1983.5761406x
272. de Groot J, Ruis MA, Scholten JW, Koolhaas JM, Boersma WJ. Long-term effects of social stress on antiviral immunity in pigs. Physiol Behav. (2001) 73:145–58. doi: 10.1016/S0031-9384(01)00472-3
273. Jensen TB, Toft N. Causes of and predisposing risk factors for leg disorders in growing-finishing pigs. CAB Rev Perspect Agricult Vet Sci Nutr Nat Resour. (2009) 4:1–8. doi: 10.1079/PAVSNNR20094010
274. Tielen MJ, Truijen WT, Groes CAvd, Verstegen MA, de Bruin JJ, Conbey RA. [Conditions of management and the construction of piggeries on pig-fattening farms as factors in the incidence of diseases of the lung and liver in slaughtered pigs (author's transl)]. Tijdschr Diergeneeskd. (1978) 103:1155–65.
275. Calderón Díaz JA, García Manzanilla E, Diana A, Boyle LA. Cross-fostering implications for pig mortality, welfare and performance. Front Vet Sci. (2018) 5:123. doi: 10.3389/fvets.2018.00123
276. Weary DM, Pajor EA, Bonenfant M, Ross SK, Fraser D, Kramer DL. Alternative housing for sows and litters: 2. Effects of a communal piglet area on pre- and post-weaning behaviour and performance. Appl Anim Behav Sci. (1999) 65:123–35. doi: 10.1016/S0168-1591(99)00053-2
277. Worobec EK, Duncan IJH, Widowski TM. The effects of weaning at 7, 14 and 28 days on piglet behaviour. Appl Anim Behav Sci. (1999) 62:173–182. doi: 10.1016/S0168-1591(98)00225-1
278. Jarvis S, Moinard C, Robson SK, Sumner BEH, Douglas AJ, Seckl JR, et al. Effects of weaning age on the behavioural and neuroendocrine development of piglets. Appl Anim Behav Sci. (2008) 110:166–81. doi: 10.1016/j.applanim.2007.03.018
279. van der Meulen J, Koopmans SJ, Dekker RA, Hoogendoorn A. Increasing weaning age of piglets from 4 to 7 weeks reduces stress, increases post-weaning feed intake but does not improve intestinal functionality. Animal. (2010) 4:1653–61. doi: 10.1017/S1751731110001011
280. McLamb BL, Gibson AJ, Overman EL, Stahl C, Moeser AJ. Early weaning stress in pigs impairs innate mucosal immune responses to enterotoxigenic e. coli challenge and exacerbates intestinal injury and clinical disease. PLoS ONE. (2013) 8:e59838. doi: 10.1371/journal.pone.0059838
281. López-Soria S, Segalés J, Rose N, Viñas MJ, Blanchard P, Madec F, et al. An exploratory study on risk factors for postweaning multisystemic wasting syndrome (PMWS) in Spain. Prev Vet Med. (2005) 69:97–107. doi: 10.1016/j.prevetmed.2004.11.015
282. Vraa-Andersen L. Analysis of risk factors related to infection with Actinobacillus pleuropneumonia and Mycoplasma hyopneumoniae in swine. In: 6th Int. Symp. Vet. Epi. Econ (Ottawa, ON) (1991). p. 419–21.
283. Oostindjer M, Bolhuis JE, van den Brand H, Roura E, Kemp B. Prenatal flavor exposure affects growth, health and behavior of newly weaned piglets. Physiol Behav. (2010) 99:579–86. doi: 10.1016/j.physbeh.2010.01.031
284. Oostindjer M, Bolhuis JE, Simon K, van den Brand H, Kemp B. perinatal flavour learning and adaptation to being weaned: all the pig needs is smell. PLoS ONE. (2011) 6:e25318. doi: 10.1371/journal.pone.0025318
285. Middelkoop A, Costermans N, Kemp B, Bolhuis JE. Feed intake of the sow and playful creep feeding of piglets influence piglet behaviour and performance before and after weaning. Sci Rep. (2019) 9:16140. doi: 10.1038/s41598-019-52530-w
286. Rhouma M, Fairbrother JM, Beaudry F, Letellier A. Post weaning diarrhea in pigs: risk factors and non-colistin-based control strategies. Acta Vet Scand. (2017) 59:31. doi: 10.1186/s13028-017-0299-7
287. Moeser AJ, Pohl CS, Rajput M. Weaning stress and gastrointestinal barrier development: implications for lifelong gut health in pigs. Anim Nutr. (2017) 3:313–21. doi: 10.1016/j.aninu.2017.06.003
288. Robbins JA, von Keyserlingk MA, Fraser D, Weary DM. INVITED REVIEW: Farm size and animal welfare. J Anim Sci. (2016) 94:5439–55. doi: 10.2527/jas.2016-0805
289. da Costa MR, Manzanilla EG, Diana A, van Staaveren N, Torres-Pitarch A, Boyle LA, et al. Identifying challenges to manage body weight variation in pig farms implementing all-in-all-out management practices and their possible implications for animal health: a case study. Porcine Health Manag. (2021) 7:1–10. doi: 10.1186/s40813-021-00190-6
290. Kakanis M, Sossidou E, Kritas S, Tzika E. Update on Tail biting in pigs: an undesirable damaging behaviour. J Hellenic Vet Med Soc. (2021) 72:2629–46. doi: 10.12681/jhvms.26745
291. Wegener HC, Andresen LO, Bille-Hansen V. Staphylococcus hyicus virulence in relation to exudative epidermitis in pigs. Can J Vet Res. (1993) 57:119.
293. Clegg SR, Sullivan LE, Bell J, Blowey RW, Carter SD, Evans NJ. Detection and isolation of digital dermatitis treponemes from skin and tail lesions in pigs. Res Vet Sci. (2016) 104:64–70. doi: 10.1016/j.rvsc.2015.12.003
294. Richardson J, Morter R, Rebar A, Olander H. Lesions of porcine necrotic ear syndrome. Vet Pathol. (1984) 21:152–7. doi: 10.1177/030098588402100203
296. Dybkjær L. The identification of behavioural indicators of ‘stress’ in early weaned piglets. Appl Anim Behav Sci. (1992) 35:135–47. doi: 10.1016/0168-1591(92)90004-U
297. Schmitt O, O'Driscoll K, Boyle LA, Baxter EM. Artificial rearing affects piglets pre-weaning behaviour, welfare and growth performance. Appl Anim Behav Sci. (2019) 210:16–25. doi: 10.1016/j.applanim.2018.10.018
298. Schmitt O, Poidevin A, O'Driscoll K. Does diversity matter? Behavioural differences between piglets given diverse or similar forms of enrichment pre-weaning. Animals. (2020) 10:1837. doi: 10.3390/ani10101837
299. Mirt D. Lesions of so-called flank biting and necrotic ear syndrome in pigs. Vet Rec. (1999) 144:92–6. doi: 10.1136/vr.144.4.92
300. Busch ME, Dedeurwaerdere A, Wachmann H. The development and the consequences of ear necrosis in one herd. In: 20th International Pig Veterinary Society Congress. Durban: IPVS (2008). p. 278.
301. Karlsson F, Svartström O, Belák K, Fellström C, Pringle M. Occurrence of Treponema spp. in porcine skin ulcers and gingiva. Vet Microbiol. (2013) 165:402–9. doi: 10.1016/j.vetmic.2013.03.031
302. Visnjakow JI, Georgiev M. Swine caudophagy, a new epizootiological link of trichinellosis in the industrial swine farms. Acta Parasitol Polon. (1972) 597–604.
303. Cowen P, Pacer RA, Van Peteghem PN, Fetrow JF. Management factors affecting trichinosis seropositivity among 91 North Carolina swine farms. Prev Vet Med. (1990) 9:165–72. doi: 10.1016/0167-5877(90)90063-N
304. McGlone JJ. A quantitative ethogram of aggressive and submissive behaviors in recently regrouped pigs. J Anim Sci. (1985) 61:559–65. doi: 10.2527/jas1985.613556x
305. Oczak M, Viazzi S, Ismayilova G, Sonoda LT, Roulston N, Fels M, et al. Classification of aggressive behaviour in pigs by activity index and multilayer feed forward neural network. Biosyst Eng. (2014) 119:89–97. doi: 10.1016/j.biosystemseng.2014.01.005
306. Cowie CE, Marreos N, Gortázar C, Jaroso R, White PC, Balseiro A. Shared risk factors for multiple livestock diseases: a case study of bovine tuberculosis and brucellosis. Res Vet Sci. (2014) 97:491–7. doi: 10.1016/j.rvsc.2014.09.002
307. Le Floch N, Jondreville C, Matte JJ, Seve B. Importance of sanitary environment for growth performance and plasma nutrient homeostasis during the post-weaning period in piglets. Arch Anim Nutr. (2006) 60:23–34. doi: 10.1080/17450390500467810
308. Patterson R, Nevel A, Diaz AV, Martineau HM, Demmers T, Browne C, et al. Exposure to environmental stressors result in increased viral load and further reduction of production parameters in pigs experimentally infected with PCV2b. Vet. Microbiol. (2015) 177:261–9. doi: 10.1016/j.vetmic.2015.03.010
309. Chatelet A, Gondret F, Merlot E, Gilbert H, Friggens NC, Le Floc'h N. Impact of hygiene of housing conditions on performance and health of two pig genetic lines divergent for residual feed intake. Animal. (2018) 12:350–8. doi: 10.1017/S1751731117001379
310. Smith RS. The macrophage theory of depression. Med Hypoth. (1991) 35:298–306. doi: 10.1016/0306-9877(91)90272-Z
311. Maes M. Depression is an inflammatory disease, but cell-mediated immune activation is the key component of depression. Progress Neuro Psychopharmacol Biol Psychiatry. (2011) 35:664–75. doi: 10.1016/j.pnpbp.2010.06.014
312. Capuron L, Ravaud A, Dantzer R. Early depressive symptoms in cancer patients receiving interleukin 2 and/or interferon alfa-2b therapy. J Clin Oncol. (2000) 18:2143–51. doi: 10.1200/JCO.2000.18.10.2143
313. O'Connor JC, Lawson MA, Andre C, Moreau M, Lestage J, Castanon N, et al. Lipopolysaccharide-induced depressive-like behavior is mediated by indoleamine 2, 3-dioxygenase activation in mice. Mol Psychiatry. (2009) 14:511–22. doi: 10.1038/sj.mp.4002148
314. Remus JL, Dantzer R. Inflammation models of depression in rodents: relevance to psychotropic drug discovery. Int J Neuropsychopharmacol. (2016) 19:pyw028. doi: 10.1093/ijnp/pyw028
315. Alzarea S, Rahman S. Alpha-7 nicotinic receptor allosteric modulator PNU120596 prevents lipopolysaccharide-induced anxiety, cognitive deficit and depression-like behaviors in mice. Behav Brain Res. (2019) 366:19–28. doi: 10.1016/j.bbr.2019.03.019
316. Parmentier HK, Rodenburg TB, De Vries Reilingh G, Beerda B, Kemp B. Does enhancement of specific immune responses predispose laying hens for feather pecking? Poul Sci. (2009) 88:536–42. doi: 10.3382/ps.2008-00424
317. Almond PK, Bilkei G. Effects of oral vaccination against Lawsonia intracellularis on growing-finishing pig's performance in a pig production unit with endemic porcine proliferative enteropathy (PPE). Deutsche tierarztliche Wochenschrift. (2006) 113:232–5.
318. Pejsak Z, Markowska-Daniel I, Pomorska-Mól M, Porowski M, Kołacz R. Ear necrosis reduction in pigs after vaccination against PCV2. Res Vet Sci. (2011) 91:125–8. doi: 10.1016/j.rvsc.2010.08.015
319. Papatsiros V. Ear necrosis syndrome in weaning pigs associated with PCV2 infection: a case report. In: Veterinary Research Forum, Vol. 3. Urmia: Faculty of Veterinary Medicine, Urmia University (2012). p. 217.
320. Lawrence AB, Terlouw EC, Kyriazakis I. The behavioural effects of undernutrition in confined farm animals. Nutr Soc. (1993) 52:219–29. doi: 10.1079/PNS19930054
321. Jensen MB, Kyriazakis I, Lawrence AB. The activity and straw directed behaviour of pigs offered foods with different crude protein content. Appl Anim Behav Sci. (1993) 37:211–21. doi: 10.1016/0168-1591(93)90112-3
322. Meunier-Salaün M, Edwards S, Robert S. Effect of dietary fibre on the behaviour and health of the restricted fed sow. Anim Feed Sci Technol. (2001) 90:53–69. doi: 10.1016/S0377-8401(01)00196-1
323. Fraser D, Bernon D, Ball R. Enhanced attraction to blood by pigs with inadequate dietary protein supplementation. Can J Anim Sci. (1991) 71:611–9. doi: 10.4141/cjas91-076
324. Melchior D, Sève B, Le Floc'h N. Chronic lung inflammation affects plasma amino acid concentrations in pigs. J Anim Sci. (2004) 82:1091–9. doi: 10.2527/2004.8241091x
325. Takikawa O. Biochemical and medical aspects of the indoleamine 2,3-dioxygenase-initiated l-tryptophan metabolism. Biochem Biophys Res Commun. (2005) 338:12–9. doi: 10.1016/j.bbrc.2005.09.032
326. Martínez-Trejo G, Ortega-Cerrilla M, Rodarte-Covarrubias L, Herrera-Haro J, Figueroa-Velasco J, Galindo-Maldonado F, et al. Aggressiveness and productive performance of piglets supplemented with tryptophan. J Anim Vet Adv. (2009) 8:608–11.
327. Pastorelli H, Le Floc'H N, Merlot E, Meunier-Salaün M-C, van Milgen J, Montagne L. Feed restriction applied after weaning has different effects on pig performance and health depending on the sanitary conditions. J Anim Sci. (2012) 90:4866–75. doi: 10.2527/jas.2012-5309
328. Brunberg EI, Rodenburg TB, Rydhmer L, Kjaer JB, Jensen P, Keeling LJ. Omnivores going astray: a review and new synthesis of abnormal behavior in pigs and laying hens. Front Vet Sci. (2016) 3:57. doi: 10.3389/fvets.2016.00057
329. Van den Berg A, Brülisauer F, Regula G. Prevalence of gastric lesions in the pars proventricularis in finishing pigs at slaughter in Switzerland. Schweizer Archiv fur Tierheilkunde. (2005) 147:297–303. doi: 10.1024/0036-7281.147.7.297
330. Palander P, Heinonen M, Simpura I, Edwards S, Valros A. Jejunal morphology and blood metabolites in tail biting, victim and control pigs. Animal. (2013) 7:1523–31. doi: 10.1017/S1751731113000669
331. Rabhi N, Thibodeau A, Côté J-C, Devillers N, Laplante B, Fravalo P, et al. Association between tail-biting and intestinal microbiota composition in pigs. Front Vet Sci. (2020) 7:563762. doi: 10.3389/fvets.2020.563762
332. Franco NH, Correia-Neves M, Olsson IAS. How “Humane” is your endpoint?—refining the science-driven approach for termination of animal studies of chronic infection. PLoS Pathog. (2012) 8:e1002399. doi: 10.1371/journal.ppat.1002399
333. Heinonen M, Hämeenoja P, Saloniemi H, Tuovinen V. Diagnoses and treatments in health-classified fattening herds rearing pigs all in – all out. Acta Vet Scand. (2001) 42:365. doi: 10.1186/1751-0147-42-365
334. Stygar AH, Chantziaras I, Toppari I, Maes D, Niemi JK. High biosecurity and welfare standards in fattening pig farms are associated with reduced antimicrobial use. Animal. (2020) 14:2178–86. doi: 10.1017/S1751731120000828
336. Warnke PH, Becker ST, Springer IN, Haerle F, Ullmann U, Russo PA, Wiltfang et al. Penicillin compared with other advanced broad spectrum antibiotics regarding antibacterial activity against oral pathogens isolated from odontogenic abscesses. J Craniomaxillofac Surg. (2008) 36:462–7. doi: 10.1016/j.jcms.2008.07.001
337. Wagner C, Sauermann R, Joukhadar C. Principles of antibiotic penetration into abscess fluid. Pharmacology. (2006) 78:1–10. doi: 10.1159/000094668
338. Chou J-Y, O'Driscoll K, D'Eath RB, Sandercock DA, Camerlink I. Multi-step tail biting outbreak intervention protocols for pigs housed on slatted floors. Animals. (2019) 9:582. doi: 10.3390/ani9080582
Keywords: welfare, health, lesion, risk, behavior, pig, tail biting
Citation: Boyle LA, Edwards SA, Bolhuis JE, Pol F, Šemrov MZ, Schütze S, Nordgreen J, Bozakova N, Sossidou EN and Valros A (2022) The Evidence for a Causal Link Between Disease and Damaging Behavior in Pigs. Front. Vet. Sci. 8:771682. doi: 10.3389/fvets.2021.771682
Received: 06 September 2021; Accepted: 06 December 2021;
Published: 27 January 2022.
Edited by:
Marian Stamp Dawkins, University of Oxford, United KingdomReviewed by:
Suzanne D. E. Held, University of Bristol, United KingdomIrene Camerlink, Polish Academy of Sciences (PAS), Poland
Copyright © 2022 Boyle, Edwards, Bolhuis, Pol, Šemrov, Schütze, Nordgreen, Bozakova, Sossidou and Valros. This is an open-access article distributed under the terms of the Creative Commons Attribution License (CC BY). The use, distribution or reproduction in other forums is permitted, provided the original author(s) and the copyright owner(s) are credited and that the original publication in this journal is cited, in accordance with accepted academic practice. No use, distribution or reproduction is permitted which does not comply with these terms.
*Correspondence: Laura A. Boyle, bGF1cmEuYm95bGVAdGVhZ2FzYy5pZQ==