Spotted Fever Group Rickettsia Infecting Ticks (Acari: Ixodidae), Yak (Bos grunniens), and Tibetan Sheep (Ovis aries) in the Qinghai–Tibetan Plateau Area, China
- 1State Key Laboratory of Plateau Ecology and Agriculture, Qinghai University, Xining, China
- 2College of Agriculture and Animal Husbandry, Qinghai University, Xining, China
- 3National Research Center for Wildlife-Born Diseases, University of Chinese Academy of Sciences, Beijing, China
- 4National Institute of Parasitic Diseases Chinese Center for Disease Control and Prevention, Shanghai, China
- 5Qinghai Provincial Center for Animal Disease Control and Prevention, Xining, China
- 6Animal Disease Prevention and Control Center of Yushu, Yushu, China
The Qinghai–Tibet Plateau Area (QTPA) has a complex natural ecosystem, causing a greatly increased risk of spreading various tick-borne diseases including rickettsial infections, which are regarded as one of the oldest known vector-borne zoonoses. However, the information of one of its pathogen, spotted fever group Rickettsia (SFG Rickettsia), is limited in tick vectors and animals in this area. Therefore, this study focused on the investigation of SFG Rickettsia in tick vectors, yaks (Bos grunniens), and Tibetan sheep (Ovis aries) in the QTPA. A total of 1,000 samples were collected from nine sampling sites, including 425 of yaks, 309 of Tibetan sheep, 266 of ticks. By morphological examination, PCR, and sequencing, we confirmed the species of all collected ticks. All tick samples, all yak and Tibetan sheep blood samples were detected based on SFG Rickettsia ompA and sca4 gene. The results showed that all tick samples were identified to be Haemaphysalis qinghaiensis, and the positive rates of SFG Rickettsia were 5.9% (25/425), 0.3% (1/309), and 54.1% (144/266) in yaks, Tibetan sheep, and ticks, respectively. All positive samples were sequenced, and BLASTn analysis of the ompA gene sequences of SFG Rickettsia showed that all positive samples from animals and ticks had 99.04–100% identity with yak and horse isolates from Qinghai Province, China. BLASTn analysis of the sca4 gene sequences of SFG Rickettsia showed that all positive samples had 97.60–98.72% identity with tick isolates from Ukraine. In addition, the phylogenetic analysis showed that all the SFG Rickettsia ompA and sca4 sequences obtained from this study belong to the same clade as Rickettsia raoultii isolated from livestock and ticks from China and other countries. Molecularly, this study detected and characterized SFG Rickettsia both in the tick vectors and animals, suggesting that the relationship between SFG Rickettsia, tick species and animal hosts should be explored to understand their interrelationships, which provide a theoretical basis for preventing control of this pathogen.
Introduction
Rickettsia spp. (Rickettsiales: Rickettsiaceae) are obligate intracellular bacteria that causes rickettsioses, which is one of the oldest known vector-borne zoonoses diseases (1). The genus Rickettsia consists of four members: spotted fever group (SFG) rickettsiae, typhus group rickettsiae, the Rickettsia bellii group, and the Rickettsia canadensis group (2). At present, 26 SFG Rickettsia species had been reported, 16 of which were associated with human diseases (3, 4). In addition to the typical triad of fever, rash, and headache, the classic symptoms of new cases of Rickettsia varied by type, and in some cases, the typical symptoms might not appear or be ignored (4). Therefore, the clinical diagnosis of rickettsioses is a huge challenge for both physicians and veterinarians (3, 4).
SFG Rickettsia includes more than 20 different species, including pathogenic species that cause human and animal incidence, and nonpathogenic species (5). In Asia, a variety of SFG Rickettsia have been reported, and these rickettsiae are mostly distributed in border areas of various countries. In the Russian Far East, Kazakhstan, northern China, and Mongolia, R. sibirica and R. raoultii are widely distributed (4). In China, 11 SFG Rickettsia species have been detected in tick vectors and animals, some of which have been characterized as human pathogens (6). In previous reports, SFG Rickettsia was detected in a variety of ticks, including Haemaphysalis qinghaiensis in Yunnan Province and Harbin Province in China (5, 7, 8). However, there are few reports of the determination of SFG Rickettsia in H. qinghaiensis in the Qinghai–Tibetan Plateau Area (QTPA) (7, 9).
The QTPA is well known for the largest and highest plateau in the world, which is located in northwestern China and with a unique natural ecosystem (7). Yaks and Tibetan sheep are indigenous animals on the QTPA, which are the important sources of milk, meat, fur, skins, and dung in the area, contributing to the local economic development and the lives of herdsmen (10). Although SFG rickettsioses in livestock have been reported in this area, the information on these pathogens are limited (6, 11). H. qinghaiensis is a common and endemic three-host tick on the QTPA, which is easy to parasitize on Tibetan sheep, yaks, sheep, goats, and other domestic animals to transmit a variety of diseases (9). Therefore, the existence of H. qinghaiensis is a potential threat to the animal husbandry in the QTPA (12).
This study aimed to determine the existence and molecular characteristics of SFG Rickettsia from yaks, Tibetan sheep, and tick vectors in the QTPA, so as to further understand the prevalence of SFG Rickettsia and clarify the interaction between ticks and animals.
Materials and Methods
Sample Collection and DNA Extraction
In the present study, all of 1,000 samples including yak and Tibetan sheep blood samples, and tick samples were collected from nine cites of Yushu Tibetan Autonomous Prefecture (Yushu) and Guoluo Tibetan Autonomous Prefecture (Guoluo) in Qinghai Province of QTPA (Figure 1). The 734 blood samples of yaks and Tibetan sheep were collected in tubes containing an EDTA anticoagulant, and genomic DNA was extracted using the MagPure Blood DNA KF Kit (Magen, China) according to the manual of the manufacturer. The 266 tick samples were collected, some of which were engorged ticks collected on the surface of yaks and Tibetan sheep, and the rest of ticks were collected by dragging the grasslands with a flannel cloth. DNA was extracted by using the MagPure Mollusc DNA KF Kit (Magen, China) and stored at −80°C until further use.
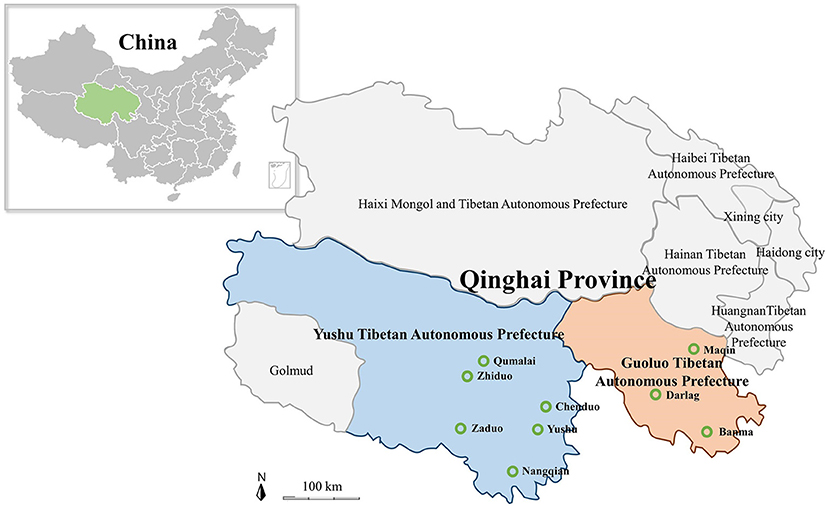
Figure 1. Map of the Qinghai–Tibetan Plateau Area and Qinghai Province showing the sampling sites. The green hollow circle indicates the location of the sample collection in this study.
Identification of Ticks and Detection of Pathogens
Ticks were identified by the mitochondrial 16S rRNA gene (forward primer 5′-CTG CTC AAT GAT TTT TTA AAT TGC TGT GG-3′, reverse primer 5′-CCG GTC TGA ACT CAG ATC AAG T-3′) and morphological characteristics (13, 14). Outer membrane protein A (ompA) gene and surface cell antigen 4 (sca4) gene were used to investigate the molecular characterization of the SFG Rickettsia by using genus-specific primers (forward primer 5′-GCT TTA TTC ACC ACC TCA AC-3′ and reverse primer 5′-TRA TCA CCA CCG TAA GTA AAT-3′; D767f: 5′-CGA TGG TAG CAT TAA AAG CT-3′ and D1390r: 5′-CTT GCT TTT CAG CAA TAT CAC-3′) (15, 16). PCR reaction volume of 10 μl containing 3 μl of DNA template, 0.5 μl each of forward and reverse primer (100 μM), 0.1 μl of Taq polymerase (0.5 U; New England BioLab, USA), 0.2 μl of deoxyribonucleotide triphosphate (200 μM; New England BioLab, USA), 1 μl of 10× ThermoPol Reaction Buffer (New England BioLab, USA), and double-distilled water to a volume of 10 μl. Positive yak DNA samples of Rickettsia spp. ompA gene from our previous study were used as positive controls, and double-distilled water was used as a negative control (11).
Sequencing and Phylogenetic Analyses
All PCR positive tick and pathogen samples were purified using EasyPure® Quick Gel Extraction Kit (TransGen, China) and cloned into E. coli DH5α using the PMDTM 19-T Vector Cloning Kit (TaKaRa, Japan). At least two positive clones from each positive sample were selected for sequencing in Sangon Biotech (Shanghai) Co., Ltd. Nucleotide sequence identities were determined by performing GenBank BLASTn analysis (https://blast.ncbi.nlm.nih.gov/Blast.cgi). Phylogenetic trees based on the obtained sequences were constructed using the neighbor-joining method, and genetic distance matrix among various Rickettsia clades in a phylogenetic tree were calculated using the maximum composite likelihood model in MEGA7.0 (17).
Statistical Analysis
The Chi-square test was used to evaluate the difference in prevalence between different parameters. Exposure variables included prefecture (Guoluo and Yushu), altitude (3,000–4,000 and 4,000–5,000 m), and animals (yak and Tibetan sheep). When the p-values were lower than 0.05, the result was considered to be of statistically significant difference (https://www.mathsisfun.com/data/chi-square-calculator.html).
Results
Identification of Ticks
By morphological examination, all the tick samples were identified as Haemaphysalis (Figure 2) (14); then the mitochondrial 16S rRNA gene target was used as an identification molecular marker, and 30 tick specimens were randomly selected for sequencing. By BLASTn analysis and phylogenetic analysis, the evidence demonstrated that there is an extensive homology between the Haemaphysalis species 16S rRNA gene sequence and the published sequence of H. qinghaiensis (MF629844) from Gansu Province, China, and grouped with the H. qinghaiensis clades (Figure 3 and Table 1).
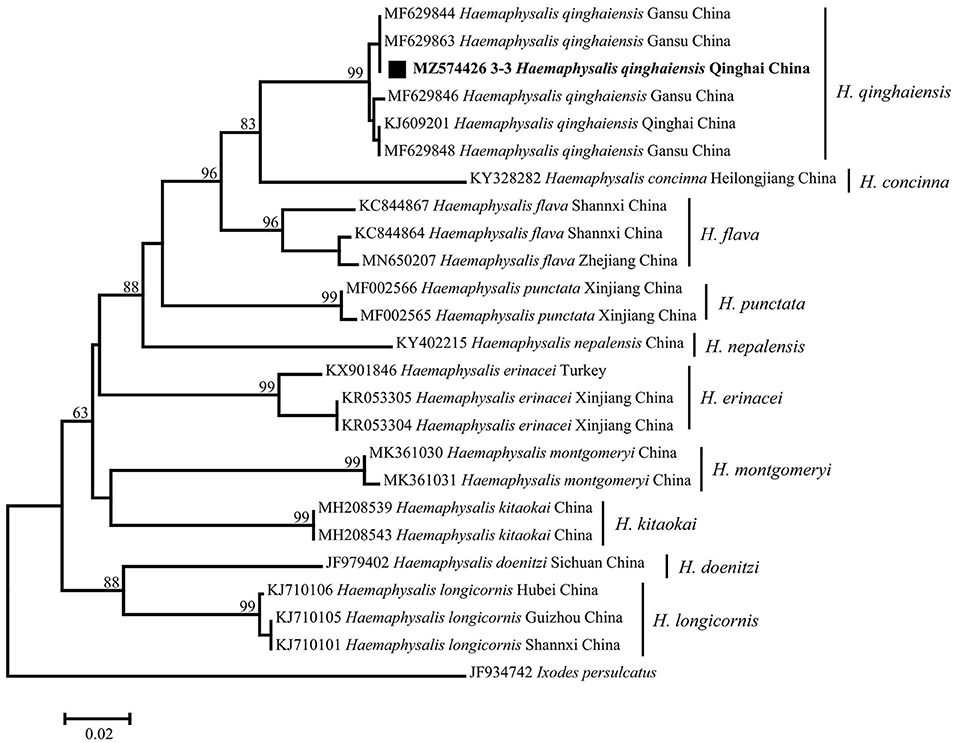
Figure 3. Phylogenetic tree based on the mitochondrial 16S rRNA gene (460 bp) of Haemaphysalis spp. obtained in this study. Bootstraps analysis was performed with 500 replicates. The solid square indicates sequence from this study. One sequence from this study is bolded. Ixodes persulcatus (JF934742) was used as outgroup.
Infection Rates of Spotted Fever Group Rickettsia
In the current study, the overall infection rate of SFG Rickettsia was 17% (170/1,000), including the infection rate of 51.35% (76/148) in animal-derived engorged ticks, 57.6% (68/118) in free ticks, 5.9% (25/425) in yaks, and 0.3% (1/309) in Tibetan sheep (Table 2).
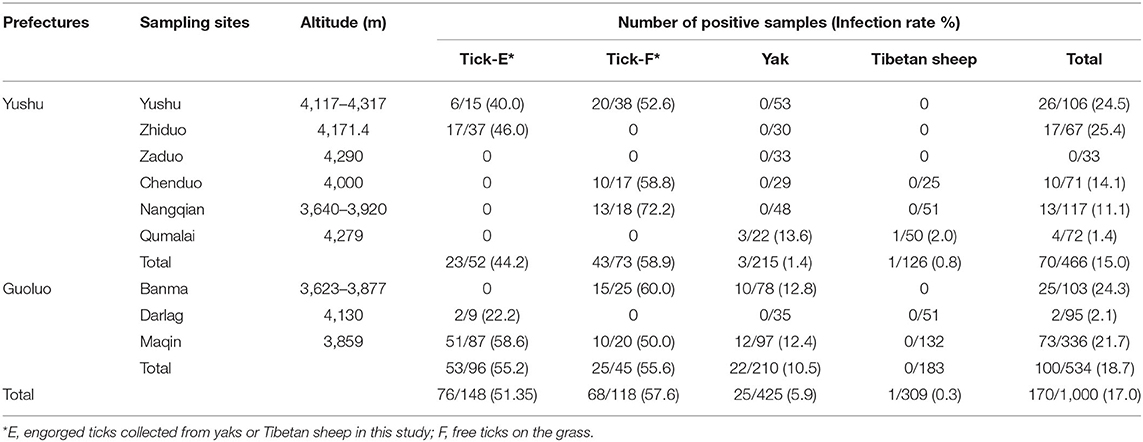
Table 2. The positive samples of spotted fever group (SFG) Rickettsia in ticks and animals in this study.
Statistical analysis showed that for yaks, the infection rate of SFG Rickettsia in Guoluo was significantly higher than that of Yushu (p = 0.0002), and the infection rate at altitudes of 3,000–4,000 m was significantly higher than that at altitudes of 4,000–5,000 m (p = 0.0005) (Table 3). Moreover, altitude also was a significant impact factor on the total infection rate, such as the infection rate of SFG Rickettsia at an altitude of 3,000–4,000 m was remarkably higher than that of 4,000–5,000 m (p = 0.0183) (Table 3). However, there was no significant difference in the infection rate of SFG Rickettsia from engorged H. qinghaiensis divided from yaks and Tibetan sheep, and also no significant difference in the infection rate of SFG Rickettsia of free H. qinghaiensis and engorged those in this study (p > 0.05) (Table 4).
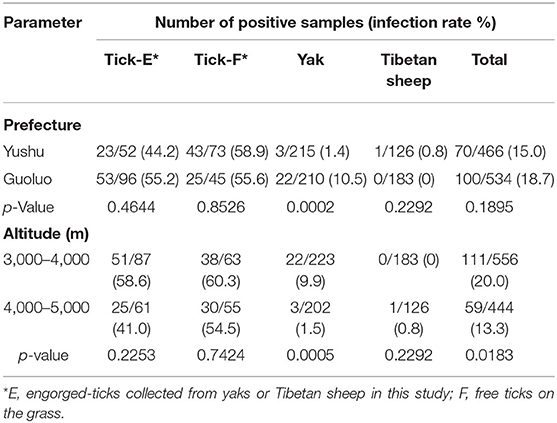
Table 3. The patterns of infection rates of spotted fever group (SFG) Rickettsia in ticks, yaks, and Tibetan sheep grouped by prefecture and altitude of the sampling sites.
Sequencing and Phylogenetic Analysis of Spotted Fever Group Rickettsia ompA Gene
In this study, a total of 19 SFG Rickettsia ompA gene sequences (MZ130267–MZ130285) were obtained, and the identity between each sequence was 94.2−100%, and the identity was 99.04–100% between MK307883, MN394797, MN394798, MN394801, and MN394800. The identity was 95.7–98.6% between the sequences of ticks and those of animals. Phylogenetic analysis of SFG Rickettsia ompA gene revealed that 19 sequences were in the same clade as R. raoultii isolated from horse, camel, Haemaphysalis erinacei, Ixodes persulclcatus, and three Dermacentor species from China, Austria, and Turkey (Figure 4). Genetic distance among various Rickettsia clades was 1–21% and R. raoultii with 8–13% genetic distance with other rickettsiae (Table 5).
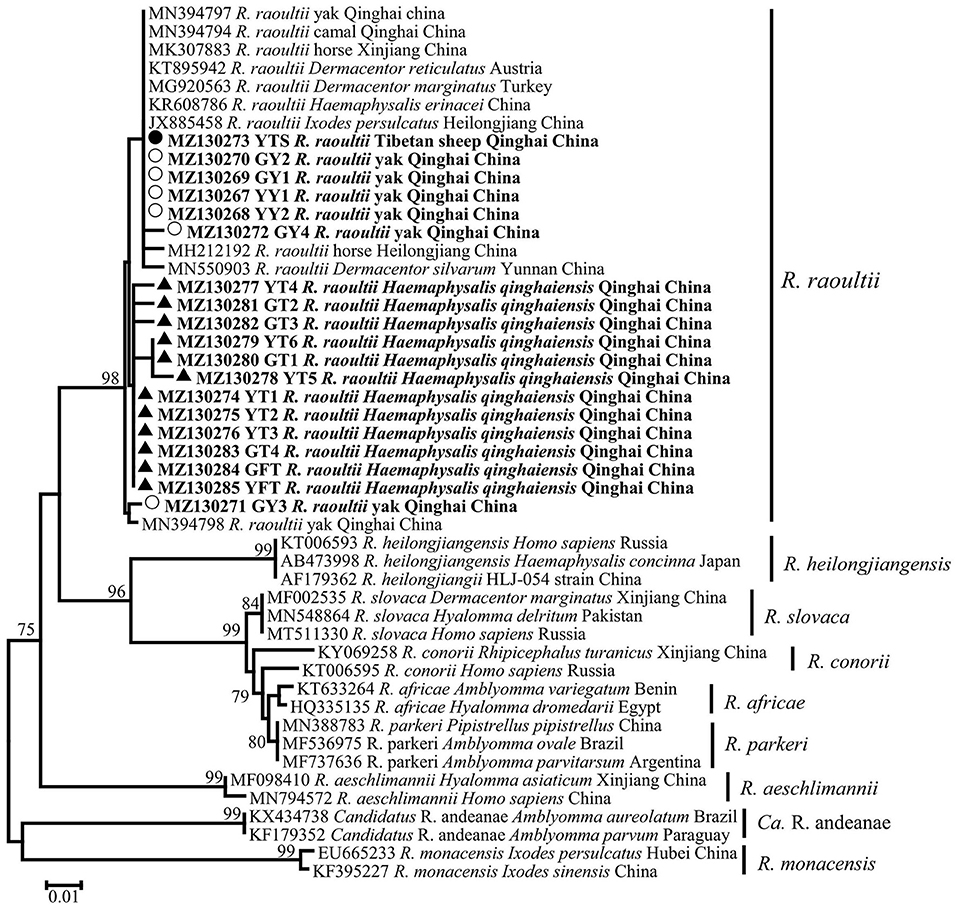
Figure 4. Phylogenetic tree based on ompA particle sequences (209/212 bp) of spotted fever group (SFG) Rickettisa obtained in this study. Bootstraps analysis was performed with 500 replicates. The solid circle indicates sequences from Tibetan sheep, the empty circle indicates sequences from yaks, and the solid triangle indicate sequences from Haemaphysalis qinghaiensis. All sequences from this study are bolded.
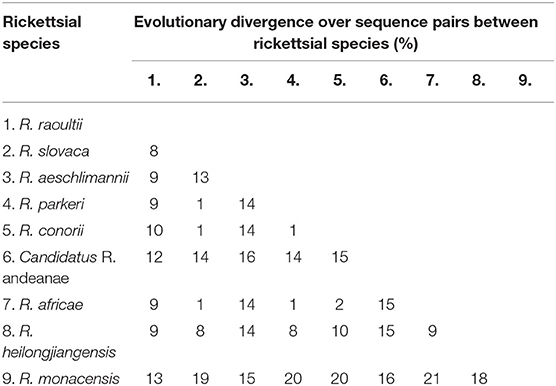
Table 5. Genetic distance matrix among various Rickettsia species in a phylogenetic tree based on the ompA gene in this study.
Sequencing and Phylogenetic Analysis of Spotted Fever Group Rickettsia sca4 Gene
All of five SFG Rickettsia sca4 gene sequences (OL621221–OL621225) were obtained, and the identity between each sequences was 98.6−99.8%, and the identity was 97.60−98.72% in KP768191. Phylogenetic analysis of SFG Rickettsia sca4 gene revealed that five sequences were in the same clade as R. raoultii isolated from Dermacentor reticulatus from Ukraine (Figure 5). Genetic distance among various Rickettsia clades was 1−42%, R. raoultii with 2−25% genetic distance with other rickettsiae (Table 6).
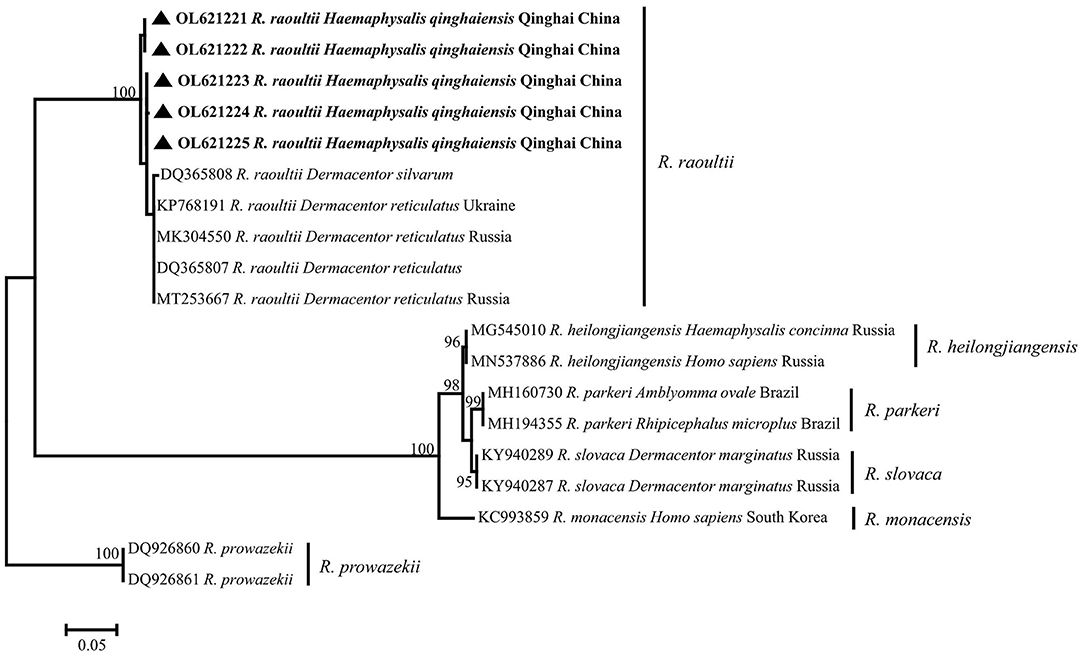
Figure 5. Phylogenetic tree based on sca4 particle sequences (624 bp) of spotted fever group (SFG) Rickettsia obtained in this study. Bootstraps analysis was performed with 500 replicates. The solid triangle indicates sequences from Haemaphysalis qinghaiensis. All sequences from this study are bolded.
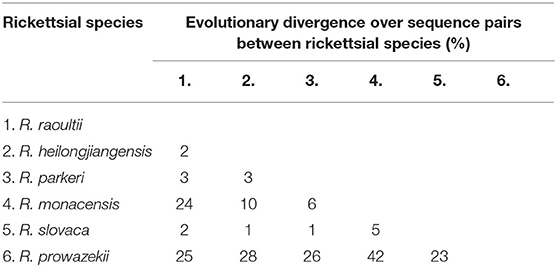
Table 6. Genetic distance matrix among various Rickettsia species in a phylogenetic tree based on sca4 gene in this study.
Discussion
Haemaphysalis qinghaiensis was first discovered in Huangyuan County, Qinghai Province in 1980, and was subsequently discovered in Gansu, Sichuan, Ningxia, Yunnan, Tibet provinces of China (14, 18). There was no record abroad. In nature, a complete life history of H. qinghaiensis is generally 3 years (14). It was the dominant tick species in Qinghai Province of the QTPA, China, and it is mainly parasitic on various domestic animals, and as the vector of Theileria sp. and Babesia sp. (12, 14). Therefore, H. qinghaiensis and its transmitted diseases were the main economic burden of animal husbandry in Qinghai Province in the QTPA (12).
In the past 30 years, the scope and importance of pathogenic rickettsiae associated with ticks had increased dramatically (19). Most of the pathogenic Rickettsia species discovered had been found in ticks, and ticks were considered to be the most important vector and reservoir host of Rickettsia in almost every region of the word (4, 20, 21). Later, they were discovered to be related to disease symptoms in humans, such as headache, arthralgias, multiple eschars, and meningoencephalitis (22, 23). Although ticks were parasitic on humans appearing to be opportunistic, the risk of Rickettsia infection was greatly increased among herders and livestock living on the Tibetan Plateau, where ticks are widely distributed.
R. raoultii is a bacterium that was first isolated in 1999 from D. nuttalli and Rhipicephalus pumilio and was described as a novel species in 2008 (24, 25). In China, the first reported case of R. raoultii in 2012 was from D. silvarum in Xinjiang Province (26). In a previous report of Wen et al., R. raoultii was detected in at least 13 species of ticks belonging to six genera, including Dermacentor, Ixodes, Rhipicephalus, Haemaphysalis, Amblyomma, and Hyalomma (27). Moreover, R. raoultii also was detected in animals, such as yaks, Bactrian camel (Camelus bactrianus), and red fox (Vulpes vulpes) (11, 28). Importantly, R. raoultii was considered to be a human pathogen that had been shown to cause Tick-borne lymphadenopathy (TIBOLA) and Dermacentor-borne necrosis erythema and lymphadenopathy (DEBONEL) (29), and it should be emphasized that the cases had also been reported in China (30, 31).
ompA is considered to be a good candidate gene for phylogenetic analysis of most SFG representatives, with a higher degree of interspecies variation than 16S rRNA and citrate synthase-encoding gene (gltA), and ompA is present in almost all SFG members (32). sca4 also has been used to rapidly and reliably differentiate members of the genus Rickettsia (16). Thus, ompA and sca4 gene fragments were used to specifically detect rickettsial infection in ticks in many studies, such as Ishikura et al. (33), Orkun et al. (34), Taylor et al. (35), Jiang et al. (36), Kooshki et al. (37) and Nadim et al. (38). Therefore, in the current study, ompA and sca4 were selected as the target genes to detect SFG Rickettsia in yak, Tibetan sheep blood samples, and tick samples. This study results showed that SFG Rickettsia infection was detected in both tick and animal blood samples. By sequencing and BLASTn analysis, the evidence demonstrated that there were 99.04–100% sequence identities between obtained SFG Rickettsia ompA sequences (MZ130267–MZ130285) and R. raoultii (MK307883, MN394797, MN394798, MN394801, and MN394800) isolated from China. Meanwhile, BLASTn analysis of sca4 sequences showed 97.60–98.72% similarity with R. raoultii (KP768191) isolated from D. reticulatus from Ukraine. Furthermore, phylogenetic analysis showed that the current study obtained DNA sequences that formed an independent clade with R. raoultii from China, Austria, Turkey, Ukraine, and Russia, with a high bootstrap value. The genetic distance matrix based on Rickettsia ompA and sca4 genes showed that the genetic distances between R. raoultii and other rickettsiae were 8–13% and 2–25%, respectively (Tables 5, 6). In previous studies, this pathogen was also reported in various provinces such as Jilin (39), Heilongjiang (30), Gansu (40), Shaanxi (40), Xinjiang (26), and Yunnan (5), which suggests that the geographic distribution of R. raoultii covered most of China.
Molecular biology detection revealed that bacteria of genus Rickettsia were distributed all over the world, which was related to the geographical distribution of their vectors (4). Ticks, as the most important vectors of rickettsiae, were distributed throughout the world; the risk of rickettsioses in a given region might depend on the presence and biotopes of specific species of ticks (41). Among Asian countries, R. sibirica was endemic in most of the areas of Russia, China, Mongolia, and Kazakhstan, and was mainly transmitted by the Dermacentor tick (3). In addition, it was reported in Russia and China that R. heilongjiangensis isolated from H. concina and D. silvarum, and the cases caused by this Rickettsia species, had also been reported in Japan (1, 42). R. japonica was also found in Japan and was transmitted by a variety of Haemaphysalis ticks, other Ixodes and Dermacentor ticks (1). Rickettsia strains closely related to R. japonica were detected in ticks in South Korea and northern Thailand (43, 44). In Turkey, researchers detected different SFG Rickettsia in different ticks; R. aeschlimanii was detected in H. marginatum, R. raoultii and R. slovaca were detected in D. marginatus, and R. hoogstraalii in H. parva (45). In India, R. conorii subsp. indica was widespread, and the cases were also reported in Laos and Sri Lanka (1). Ticks are the most important external parasites of livestock and wildlife in Iran. A total of 46 species had been reported (46), in which Argas persicus was the important ectoparasite of poultry in Iran. In previous studies, R. Hoogstraalii (37) and SFG Rickettsia (47) had been detected in Argas persicus. In addition, the epidemiological investigation on SFG Rickettsia of D. marginatus Sulzer, H. sulcata, H. inermis, and Hyalomma asiaticum in Iran was reported (38, 47).
In the current study, the infection rates of SFG Rickettsia in free H. qinghaiensis, engorged H. qinghaiensis, yaks, and Tibetan sheep were 57.6%, 51.35%, 5.9%, and 0.3%, respectively. Studies had reported the detection of R. raoultii in yaks, camels, and several ticks in Qinghai Province (7, 11). Combined with the experimental results of this study, it was suggested that H. qinghaiensis might be the host of this pathogen, but because the infection rate of this pathogen between animals and ticks was remarkably different, therefore, whether the pathogen could be transmitted by H. qinghaiensis needed further study. Meanwhile, a phylogenetic tree showed that the ompA sequences of SFG Rickettsia isolated from H. qinghaiensis in Qinghai belonged to the same clade as the reported one, while the ompA gene sequences of SFG Rickettsia isolated from animals (except MZ130271) were in same clade as those of other tick species (including Dermacentor and Ixodes). In addition, Han et al. also detected this pathogen in several Dermacentor ticks in Qinghai Province (7). According to a previous report (48) that R. raoultii was closely associated with Dermacentor spp., we suspected that this pathogen isolated from animals in this study was not mainly transmitted by H. qinghaiensis. The high infection rate of this pathogen in H. qinghaiensis suggested that H. qinghaiensis might be the reservoir of R. raoultii.
In the current study, the survey results showed that the infection rate of SFG Rickettsia in yaks was affected by region and altitude (p = 0.0002 and p = 0.0005), and the total infection rates were affected by altitude (p = 0.018). However, the current infection rates of SFG Rickettsia showed no significant difference between yaks and Tibetan sheep, which implied that the infection of SFG Rickettsia might not be related to the host species in detected areas. So further research was needed to confirm this suspicion.
In conclusion, this study revealed the existence of SFG Rickettsia in tick vectors, yaks, and Tibetan sheep in QTPA, China. The phylogenetic analysis showed that all sequences obtained in the current study were closely related to the R. raoultii isolated from different tick species and domestic animals. Although the case of human infection by R. raoultii was not reported in the sampling regions, high infection rates should be paid more attention. Importantly, the relationship between SFG Rickettsia, tick species, and animal hosts should be further explored to understand their interrelationships.
Data Availability Statement
The datasets presented in this study can be found in online repositories. The names of the repository/repositories and accession number(s) can be found in the article/supplementary material.
Ethics Statement
The study was conducted in compliance with the ethical policies of the journal and the rules of the Ethics Committee of Qinghai University.
Author Contributions
YL, Y-CH, J-XL, Y-LS, H-XH, J-SC, Y-HG, YW, PM, Y-PW, R-SL, W-KC, Z-HC, and JL designed the experiments and sampling. Y-CH, PM, Y-PW, R-SL, W-KC, and Z-HC performed the experiments. Y-CH and J-XL analyzed the data. Y-CH wrote the original manuscript. T-SQ, J-FY, Q-XZ, J-YC, Q-BZ, G-WH, MK, and YL reviewed the manuscript. All authors have read and agreed to the published version of the manuscript.
Funding
This research was funded by the Regular Assistance Project of International Department of the Ministry of Science and Technology of China (Grant No. KY201904013), the Special Project for Scientific and Technological International Cooperation of the Science and Technology Department, Qinghai Province (2021-HZ-801), and the Veterinary Bureau Scientific Research Foundation of Qinghai Province (NMSY-2021-05).
Conflict of Interest
The authors declare that the research was conducted in the absence of any commercial or financial relationships that could be construed as a potential conflict of interest.
Publisher's Note
All claims expressed in this article are solely those of the authors and do not necessarily represent those of their affiliated organizations, or those of the publisher, the editors and the reviewers. Any product that may be evaluated in this article, or claim that may be made by its manufacturer, is not guaranteed or endorsed by the publisher.
References
1. Parola P, Paddock CD, Raoult D. Tick-borne rickettsioses around the world: emerging diseases challenging old concepts. Clin Microbiol Rev. (2005) 18:719–56. doi: 10.1128/CMR.18.4.719-756.1
2. Merhej V, Raoult D. Rickettsial evolution in the light of comparative genomics. Biol Rev Camb Philos Soc. (2011) 86:379–405. doi: 10.1111/j.1469-185X.2010.00151.x
3. Parola P, Paddock CD, Socolovschi C, Labruna MB, Mediannikov O, Kernif T, et al. Update on tick-borne rickettsioses around the world: a geographic approach. Clin Microbiol Rev. (2013) 26:657–702. doi: 10.1128/CMR.00032-13
4. Piotrowski M, Rymaszewska A. Expansion of Tick-Borne Rickettsioses in the World. Microorganisms. (2020) 8:1906. doi: 10.3390/microorganisms8121906
5. Liu H, Liang X, Wang H, Sun X, Bai X, Hu B, et al. Molecular evidence of the spotted fever group Rickettsiae in ticks from Yunnan Province, Southwest China. Exp Appl Acarol. (2020) 80:339–48. doi: 10.1007/s10493-020-00467-5
6. Jian Y, Li J, Adjou Moumouni PF, Zhang X, Tumwebaze MA, Wang G, et al. Human spotted fever group rickettsia infecting yaks (Bos grunniens) in the Qinghai-Tibetan plateau area. Pathogens. (2020) 9:249. doi: 10.3390/pathogens9040249
7. Han R, Yang J, Niu Q, Liu Z, Chen Z, Kan W, et al. Molecular prevalence of spotted fever group rickettsiae in ticks from Qinghai Province, northwestern China. Infect Genet Evol. (2018) 57:1–7. doi: 10.1016/j.meegid.2017.10.025
8. Shao JW, Zhang XL Li WJ, Huang HL, Yan J. Distribution and molecular characterization of rickettsiae in ticks in Harbin area of Northeastern China. PLoS Negl Trop Dis. (2020) 14:e0008342. doi: 10.1371/journal.pntd.0008342
9. Yang J, Tian Z, Liu Z, Niu Q, Han R, Li Y, et al. Novel spotted fever group rickettsiae in Haemaphysalis qinghaiensis ticks from Gansu, Northwest China. Parasit Vectors. (2016) 9:146. doi: 10.1186/s13071-016-1423-7
10. Li K, Shahzad M, Zhang H, Jiang X, Mehmood K, Zhao X, et al. Socio-economic burden of parasitic infections in yaks from 1984 to 2017 on Qinghai Tibetan Plateau of China-A review. Acta Trop. (2018) 183:103–9. doi: 10.1016/j.actatropica.2018.04.011
11. Li J, Jian Y, Jia L, Galon EM, Benedicto B, Wang G, et al. Molecular characterization of tick-borne bacteria and protozoans in yaks (Bos grunniens), Tibetan sheep (Ovis aries) and Bactrian camels (Camelus bactrianus) in the Qinghai-Tibetan Plateau Area, China. Ticks Tick Borne Dis. (2020) 11:101466. doi: 10.1016/j.ttbdis.2020.101466
12. Gao J, Luo J, Fan R, Fingerle V, Guan G, Liu Z, et al. Cloning and characterization of a cDNA clone encoding calreticulin from Haemaphysalis qinghaiensis (Acari: Ixodidae). Parasitol Res. (2008) 102:737–46. doi: 10.1007/s00436-007-0826-y
13. Black WC. 4th, Piesman J. Phylogeny of hard- and soft-tick taxa (Acari: Ixodida) based on mitochondrial 16S rDNA sequences. Proc Natl Acad Sci U S A. (1994) 91:10034–8. doi: 10.1073/pnas.91.21.10034
14. Deng GF, Jiang ZL. Economic insect fauna of China, Fasc 39 Acari: Ixodiate. Beijing: Science Press. (1991).
15. Kidd L, Maggi R, Diniz PP, Hegarty B, Tucker M, Breitschwerdt E. Evaluation of conventional and real-time PCR assays for detection and differentiation of Spotted Fever Group Rickettsia in dog blood. Vet Microbiol. (2008) 129:294–303. doi: 10.1016/j.vetmic.2007.11.035
16. Sekeyova Z, Roux V, Raoult D. Phylogeny of Rickettsia spp. inferred by comparing sequences of 'gene D', which encodes an intracytoplasmic protein. Int J Syst Evol Microbiol. (2001) 51:1353–60. doi: 10.1099/00207713-51-4-1353
17. Kumar S, Stecher G. Tamura K. MEGA7: molecular evolutionary genetics analysis version 70 for bigger datasets. Mol Biol Evol. (2016) 33:1870–4. doi: 10.1093/molbev/msw054
18. Chen Z, Yang X, Bu F, Yang X, Yang X, Liu J. Ticks (acari: ixodoidea: argasidae, ixodidae) of China. Exp Appl Acarol. (2010) 51:393–404. doi: 10.1007/s10493-010-9335-2
19. Delord M, Socolovschi C, Parola P. Rickettsioses and Q fever in travelers (2004–2013). Travel Med Infect Dis. (2014) 12:443–58. doi: 10.1016/j.tmaid.2014.08.006
20. Socolovschi C, Huynh TP, Davoust B, Gomez J, Raoult D, Parola P. Transovarial and trans-stadial transmission of Rickettsiae africae in Amblyomma variegatum ticks. Clin Microbiol Infect. (2009) 15:317–8. doi: 10.1111/j.1469-0691.2008.02278.x
21. Socolovschi C, Matsumoto K, Brouqui P, Raoult D, Parola P. Experimental infection of Rhipicephalus sanguineus with Rickettsia conorii conorii. Clin Microbiol Infect. (2009) 15:324–5. doi: 10.1111/j.1469-0691.2008.02259.x
22. Raoult D, Roux V. Rickettsioses as paradigms of new or emerging infectious diseases. Clin Microbiol Rev. (1997) 10:694–719. doi: 10.1128/CMR.10.4.694
23. Paddock CD, Sumner JW, Comer JA, Zaki SR, Goldsmith CS, Goddard J, et al. Rickettsia parkeri: a newly recognized cause of spotted fever rickettsiosis in the United States. Clin Infect Dis. (2004) 38:805–11. doi: 10.1086/381894
24. Rydkina E, Roux V, Rudakov N, Gafarova M, Tarasevich I, Raoult D. New Rickettsiae in ticks collected in territories of the Former Soviet Union. Emerg Infect Dis. (1999) 5:811–4. doi: 10.3201/eid0506.990612
25. Mediannikov O, Matsumoto K, Samoylenko I, Drancourt M, Roux V, Rydkina E, et al. Rickettsia raoultii sp. nov, a spotted fever group rickettsia associated with Dermacentor ticks in Europe and Russia. Int J Syst Evol Microbiol. (2008) 58:1635–9. doi: 10.1099/ijs.0.64952-0
26. Tian ZC, Liu GY, Shen H, Xie JR, Luo J, Tian MY. First report on the occurrence of Rickettsia slovaca and Rickettsia raoultii in Dermacentor silvarum in China. Parasit Vectors. (2012) 5:19. doi: 10.1186/1756-3305-5-19
27. Wen J, Jiao D, Wang JH, Yao DH, Liu ZX, Zhao G, et al. Rickettsia raoultii, the predominant Rickettsia found in Dermacentor silvarum ticks in China-Russia border areas. Exp Appl Acarol. (2014) 63:579–85. doi: 10.1007/s10493-014-9792-0
28. Liu G, Zhao S, Tan W, Hornok S, Yuan W, Mi L, et al. Rickettsiae in red fox (Vulpes vulpes), marbled polecat (Vormela peregusna) and their ticks in northwestern China. Parasit Vectors. (2021) 14:204. doi: 10.1186/s13071-021-04718-1
29. Parola P, Rovery C, Rolain JM, Brouqui P, Davoust B, Raoult D. Rickettsia slovaca and R. raoultii in tick-borne Rickettsioses. Emerg Infect Dis. (2009) 15:1105–8. doi: 10.3201/eid1507.081449
30. Jia N, Zheng YC, Ma L, Huo QB Ni XB, Jiang BG, et al. Human infections with Rickettsia raoultii, China. Emerg Infect Dis. (2014) 20:866–8. doi: 10.3201/eid2005.130995
31. Li H, Zhang PH, Huang Y, Du J, Cui N, Yang ZD, et al. Isolation and identification of Rickettsia raoultii in human cases: a surveillance study in 3 medical centers in China. Clin Infect Dis. (2018) 66:1109–15. doi: 10.1093/cid/cix917
32. Fournier PE, Roux V, Raoult D. Phylogenetic analysis of spotted fever group rickettsiae by study of the outer surface protein rOmpA. Int J Syst Bacteriol. (1998) 48:839–49. doi: 10.1099/00207713-48-3-839
33. Ishikura M, Ando S, Shinagawa Y, Matsuura K, Hasegawa S, Nakayama T, et al. Phylogenetic analysis of spotted fever group rickettsiae based on gltA, 17-kDa, and rOmpA genes amplified by nested PCR from ticks in Japan. Microbiol Immunol. (2003) 47:823–32. doi: 10.1111/j.1348-0421.2003.tb03448.x
34. Orkun Ö, Karaer Z, Çakmak A, Nalbantoglu S. Spotted fever group rickettsiae in ticks in Turkey. Ticks Tick Borne Dis. (2014) 5:213–8. doi: 10.1016/j.ttbdis.2012.11.018
35. Taylor AJ, Vongphayloth K, Vongsouvath M, Grandadam M, Brey PT, Newton PN, et al. Large-Scale Survey for Tickborne Bacteria, Khammouan Province, Laos. Emerg Infect Dis. (2016) 22:1635–9. doi: 10.3201/eid2209.151969
36. Jiang J, Choi YJ, Kim J, Kim HC, Klein TA, Chong ST, et al. Distribution of Rickettsia spp. in Ticks from Northwestern and Southwestern Provinces, Republic of Korea Korean. J Parasitol. (2019) 57:161–6. doi: 10.3347/kjp.2019.57.2.161
37. Kooshki H, Goudarzi G, Faghihi F, Telmadarraiy Z, Edalat H, Hosseini-Chegeni A. The first record of Rickettsia hoogstraalii (Rickettsiales: Rickettsiaceae) from Argas persicus (Acari: Argasidae) in Iran. Syst Appl Acarol. (2020) 25:1611–7. doi: 10.11158/saa.25.9.7
38. Nadim A, Khanjani M, Hosseini-Chegeni A, Telmadarraiy Z. Identity and microbial agents related to Dermacentor marginatus Sulzer (Acari: Ixodidae) with a new record of Rickettsia slovaca (Rickettsiales: Rickettsiaceae) in Iran. Syst Appl Acarol. (2021) 26:367–78. doi: 10.11158/saa.26.2.4
39. Cao WC, Zhan L, De Vlas SJ, Wen BH, Yang H, Richardus JH, et al. Molecular detection of spotted fever group Rickettsia in Dermacentor silvarum from a forest area of northeastern China. J Med Entomol. (2008) 45:741–4. doi: 10.1603/0022-2585(2008)45[741:mdosfg]2.0.co;2
40. Guo WP, Wang YH, Lu Q, Xu G, Luo Y, Ni X, et al. Molecular detection of spotted fever group rickettsiae in hard ticks, northern China. Transbound Emerg Dis. (2019) 66:1587–96. doi: 10.1111/tbed.13184
41. Merhej V, Angelakis E, Socolovschi C, Raoult D. Genotyping, evolution and epidemiological findings of Rickettsia species. Infect Genet Evol. (2014) 25:122–37. doi: 10.1016/j.meegid.2014.03.014
42. Ando S, Kurosawa M, Sakata A, Fujita H, Sakai K, Sekine M, et al. Human Rickettsia heilongjiangensis infection, Japan. Emerg Infect Dis. (2010) 16:1306–8. doi: 10.3201/eid1608.100049
43. Chung MH, Lee SH, Kim MJ, Lee JH, Kim ES, Lee JS, et al. Japanese spotted fever, South Korea. Emerg Infect Dis. (2006) 12:1122–4. doi: 10.3201/eid1207.051372
44. Takada N, Fujita H, Kawabata H, Ando S, Sakata A, Takano A, et al. Spotted fever group Rickettsia sp. closely related to Rickettsia japonica, Thailand. Emerg Infect Dis. (2009) 15:610–1. doi: 10.3201/eid1504.071271
45. Keskin A, Bursali A, Keskin A, Tekin S. Molecular detection of spotted fever group rickettsiae in ticks removed from humans in Turkey. Ticks Tick Borne Dis. (2016) 7:951–3. doi: 10.1016/j.ttbdis.2016.04.015
46. Hosseini-Chegeni A, Tavakoli M, Telmadarraiy Z. The updated list of ticks (Acari: Ixodidae & Argasidae) occurring in Iran with a key to the identification of species. Syst Appl Acarol. (2019) 24:2133–66. doi: 10.11158/saa.24.11.8
47. Hosseini-Chegeni A, Tavakoli M, Telmadarraiy Z, Faghihi F. Molecular detection of spotted fever group Rickettsia (Rickettsiales: Rickettsiaceae) in ticks of Iran. Arch Razi Inst. (2020) 75:317–25. doi: 10.22092/ari.2019.125746.1317
Keywords: Spotted Fever Group Rickettsia, Haemaphysalis qinghaiensis, yak, Tibetan sheep, Qinghai-Tibetan Plateau Area
Citation: He Y-C, Li J-X, Sun Y-L, Kang M, He H-X, Guo Y-H, Ma P, Wei Y-P, Li R-S, Chen W-K, Chen Z-H, Li J, Qi T-S, Yang J-F, Zhang Q-X, Wang Y, Cai J-S, Zhao Q-B, Hu G-W, Chen J-Y and Li Y (2022) Spotted Fever Group Rickettsia Infecting Ticks (Acari: Ixodidae), Yak (Bos grunniens), and Tibetan Sheep (Ovis aries) in the Qinghai–Tibetan Plateau Area, China. Front. Vet. Sci. 8:779387. doi: 10.3389/fvets.2021.779387
Received: 18 September 2021; Accepted: 13 December 2021;
Published: 08 February 2022.
Edited by:
Fabrizio Bertelloni, University of Pisa, ItalyReviewed by:
Asadollah Hosseini-Chegeni, Lorestan University, IranArunee Ahantarig, Mahidol University, Thailand
Copyright © 2022 He, Li, Sun, Kang, He, Guo, Ma, Wei, Li, Chen, Chen, Li, Qi, Yang, Zhang, Wang, Cai, Zhao, Hu, Chen and Li. This is an open-access article distributed under the terms of the Creative Commons Attribution License (CC BY). The use, distribution or reproduction in other forums is permitted, provided the original author(s) and the copyright owner(s) are credited and that the original publication in this journal is cited, in accordance with accepted academic practice. No use, distribution or reproduction is permitted which does not comply with these terms.
*Correspondence: Ying Li, yingli@126.com