- 1Department of Animal Production, Faculty of Agriculture, Damietta University, Damietta, Egypt
- 2Biological Sciences Department, College of Science & Arts, King Abdulaziz University, Rabigh, Saudi Arabia
- 3Department of Biology, College of Science, University of Jeddah, Jeddah, Saudi Arabia
- 4Department of Biological Sciences, Zoology, King Abdulaziz University, Jeddah, Saudi Arabia
- 5Department of Poultry Production, Faculty of Agriculture, Damietta University, Damietta, Egypt
- 6Department of Clinical Pathology, Faculty of Veterinary Medicine, Zagazig University, Zagazig, Egypt
- 7Department of Animal Production, Faculty of Agriculture, Al-Azhar University, Cairo, Egypt
- 8Department of Animal Production, Faculty of Agriculture, Zagazig University, Zagazig, Egypt
- 9Department of Medical Laboratory Sciences, Faculty of Applied Medical Sciences, King Abdulaziz University, Jeddah, Saudi Arabia
- 10Center of Excellence in Genomic Medicine Research, King Abdulaziz University, Jeddah, Saudi Arabia
- 11Smart-Health Initiative and Red Sea Research Center, Division of Biological and Environmental Sciences and Engineering, King Abdullah University of Science and Technology, Thuwal, Saudi Arabia
A 14-week feeding study was conducted to assess the effects of feed supplementation with prebiotics β-glucan (BG group) and/or probiotics Bacillus coagulans (BC group) on O. niloticus growth performance, body analysis, intestinal structure, immunological response, and antioxidant status. The fish were equally divided into six groups, as follows: the fish group fed an un-supplemented diet served as a control group; the other fish groups were fed supplemented diets with 0.1 g β-glucan kg−1; 1 g Bacillus coagulans kg−1; 2 g B. coagulans kg−1; 0.1 g β-glucan combined with 1 g B. coagulans kg−1; 0.1 g β-glucan combined with 2 g B. coagulans kg−1. The findings revealed that supplementing B. coagulans and the β-glucan mixture improved growth performance and feed efficiency parameters (RGR and SGR) more than the other groups. The fish flesh analysis revealed increased crude protein and dry matter content and lower lipid and ash levels in the BG and BC supplemented groups than in the Control group. On the other hand, β-glucan and B. coagulans supplementation significantly boosted antioxidant activity and immunological responses in serum as determined by CAT, MDA, lysozyme, and phagocytic activity. Dietary β-glucan and B. coagulans supplementation remarkedly enhanced anterior intestine villus histomorphometry characteristics. Furthermore, B. coagulans, alone or in combination with β-glucan, could reduce HSP70 and IL-1β gene expression while increasing IL-8 and GH gene expression. According to the findings, B. coagulans and/or BG increased growth performance by increasing gut health and morphology. Furthermore, β-glucan and B. coagulans supplementation enhanced Tilapia's body composition, immunological responses, and antioxidant status.
Introduction
Over the previous several decades, a broad range of chemicals like antiseptics, antibiotics, and antiparasitic agents have been applied in the production of sustainable aquaculture industry (1). Worldwide, antibiotics of various types are widely used for treating and controlling bacterial infections. However, this raises the risk of resistant pathogenic bacteria, antibiotic accumulation in fish flesh, and food safety risks for human health (2, 3). Nowadays, the theory of free-antibiotic aquaculture has been generally approved in the aquatic industry and worldwide. Furthermore, alternative antibiotic chemicals like prebiotics and probiotics have been widely accepted because they can improve general health status, alleviate stress threats and reduce infectious diseases in several aquatic fish species (4–6). Although, the prebiotic and probiotic enriched fish feed has been extensively investigated in various fish species, little is known about the influence of synergistic combinations of pro- and prebiotics on the productivity and immunity of farmed fish.
Amongst the most widespread prebiotics are β-glucans, polysaccharides found in the cell walls structure of bacteria, fungi, and plants (7). The β-glucans applied in aquatic feeds are derived mostly from Saccharomyces cerevisiae and have a linear structure of β-1,3 connected D-glucopyranosyl units with β-1,6 bending glucose side-chains (8). Recently, β-glucan has been extensively used as an effective immunostimulant due to its ability to stimulate the immune system by boosting the binding activity of specific receptors with macro and micro-phages, neutrophils, and natural killer (NK) cells. However, the exact mode of action is still unknown (9). In addition, β-glucan could have several other biological activities such as antibacterial, antioxidant and antitoxic (10). It is well known that β-glucan supplementation might improve immunological responses of several species of aquatic creatures such as Oncorhynchus mykiss (11), Trachinotus ovatus (12), Cyprinus carpio (13), Lutjanus peru (14) and Litopenaeus vannamei (15). Likewise, previous reports investigated that the inclusion β-glucan within fish feed could stimulate the performance of some fish species such as Cyprinus carpio (16), Pseudosciaena crocea (17), Oncorhynchus mykiss (18) and Apostichopus japonicas (19). In contrast, some studies have found no significant influence of β-glucan dietary administration on the performance of Oreochromis niloticus (20, 21) and Dicentrarchus labrax (22). While, Dawood et al., (23) demonstrated that β-glucan dietary administration stimulated the lysozyme and phagocytic activities in Nile tilapia However, increasing the efficacy of employing β-glucan by combining various probiotic strains has not been explored.
On the other hand, probiotic strains, even live or dead bacteria might promote intestinal microbiota homeostasis by influencing the interspecific interaction within the entire microbial population (24). According to the data from previous research, dietary probiotic administration might promote general host health status via influencing organ development, nutrition metabolism, and the immune response (4, 25, 26). Many substances in the bacterial cell walls may stimulate the host's immune system and boost innate and adaptive immunity against infectious pathogens (27). Nowadays, many probiotics products are applied in aquaculture that contain bacterial strains such as Lactobacillus sp., Bifidobacterium sp., Bacillus spp., Pseudomonas sp., and others (5, 28, 29). Among Bacillus spp., Bacillus coagulans is the most extensively employed of these strains (30). It has a beneficial influence on the immunological activity and growth of several aquatic animals such as common carp (31), grass carp (32), and white shrimp (33). Furthermore, several research have shown that probiotic dietary supplementation can stimulate growth and immune response in Oreochromis niloticus (34, 35). Specifically, previous reports have implied that dietary supplementation with B. coagulans can promote growth, immune response and disease resistance in white shrimp (36) and common carp (37).
Tilapia, a cichlid fish species, is a main economic freshwater fish extensively cultivated in Egypt. Recently, disease outbreaks have become increasingly prevalent in farmed fish due to the expansion of intensive aquaculture and worsening of water physiochemical features. Many studies have investigated the biological influences of β-glucan or B. coagulans on some fish species, but little is known about their combined effects in Tilapia. Therefore, the main objective of the current trial was to evaluate the synergistic benefits of β-glucan combined with B. coagulans inclusion in tilapia diets that enhanced β-glucan utilization, which affects growth performance intestinal histomorphometry, immune activity, antioxidant status and regulation of some related gene expression of Tilapia.
Materials and methods
Tested additives
In this experiment, two available commercial products were employed. B. coagulans DSM 32016 (Technospore® Biochem co., Germany) commercial products containing 2.5 × 109 CFU/g have been used as a safe feed additive. Also, β-glucan powder extract (Batch No: 2809115, Pharma Health Co. Egypt) was applied as a prebiotic compound.
Experimental design and rearing conditions
Three hundred healthy O. niloticus fingerlings (6.95 ± 0.15 g) were purchased from a private hatchery in Fowa, Kafrelsheikh governate, Egypt, and transported into the wet Laboratory of the Faculty of Agriculture, Damietta University, Egypt, using plastic bags containing sufficient purified oxygen. For 2 weeks, the transported fish were kept in a 2,000 L fiberglass tank to acclimate to Laboratory conditions. The fish were then randomly assigned to six equal groups within each of the five replicates in a glass aquarium with ten fish. Fish were fed to apparent satiation three times daily (8:00, 13:00, and 16: 00) for 14-weeks. Throughout the feeding trial period, the fish rearing conditions and the water physiochemical features (mean ± SD) were preserved at the optimum conditions for tilapia; aquarium dimensions: 40 × 60 × 70 cm, photoperiod 12 h L:12 h D, water temperature (26.5 ± 1°C), pH (7.2 ± 0.5), dissolved oxygen (7.3 ± 0.5 mg/L) and total ammonia as nitrogen (<0.3 mg/L). The tested level of β-glucan in this experiment was a 0.1 g β-glucan kg−1 diet as endorsed by the manufacturer's instruction and previous reports (20, 38). The B. coagulans product levels examined in the present trial were decided to be within the sufficient average (1 or 2 g kg−1 diet) based on the Xu et al. (31) report. The feeding study was extended to 14 weeks. While the fish were divided into six equal groups, as follows:
CNT, the fish group fed un-supplemented diet (Control group);
BG, the fish group fed basal diet supplemented with 0.1 g β-glucan kg−1;
BC1, the fish group fed basal diet supplemented with 1 g B. coagulans kg−1;
BC2, the fish group fed basal diet supplemented with 2 g B. coagulans kg−1;
BG+BC1, the fish group fed basal diet supplemented by 0.1 g β-glucan combined with 1 g B. coagulans kg−1;
BG+BC2, fish group fed basal diet supplemented with 0.1 g β-glucan combined with 2 g B. coagulans kg−1.
Feed ingredients and diet preparation
The formulated diets ingredients, which are commonly applied in tilapia rations, were Fishmeal (CP, 62%), Soybean meal (CP, 44%), Wheat bran (CP, 16%), Yellow corn (CP, 4%) and Corn gluten meal (CP, 60%). The basal ration (Table 1) was prepared to meet the essential nutrient requirements of tilapia fish as ascribed by NRC (39) guidelines. Each prepared diet was produced by thoroughly combining all of the ingredients. After that, 200 ml of water per kilogram diet were blended, and the obtained combination (ingredients, feed additive, and water) was homogenized to produce a suitable mixture for each diet. Each diet was pelleted via a laboratory pellet machine with a 1 mm diameter die. Then, the produced wet pellets were left to completely dry at room temperature. The dried pellets were kept in dark plastic bags and then preserved in the refrigerator at −4°C until use. The viable count and activity of the probiotic bacteria in the prepared diets were examined after 24 h of storage following Kumaree et al. (40) procedure. Proximate analysis of the examined diets was shown in Table 1, and the chemical composition of formulated diet samples was estimated owing to AOAC (41) procedures.
Growth parameters and efficiency of feed
Survived fish were fed and weighed biweekly to estimate actual consumed diets and growth. For assessing the performance and efficiency of the consumed diet of fish, different parameters such as Cumulative body weight gain (CBWG), Average daily gain (ADG), Specific growth rate (SGR), Relative Growth Rate (RGR), Feed Conversion Rate (FCR) and Protein Efficiency Ratio (PER) were taken into consideration and were determined applying the following formula.
Average daily gain (ADG) = ,
Specific growth rate (SGR) ,
Relative growth rate (RGR)= .
Feed conversion ratio (FCR) ,
Protein efficiency ratio (PER)= ,
Where W1 and W2 are the initial and final biomass, respectively. TFI, PI, and T are the total consumed feed, total protein intake and the total number of feeding trial days (14-weeks), respectively.
Fish body chemical analysis
Six fish from each treatment were transferred into plastic bags and stored at −20°C to determine the proximate analysis of the total fish body. The crude protein, lipid, and ash content of the fish body were determined using the Association of Official Analytical Chemists standard techniques (41). The moisture content was determined by drying the samples until obtaining a consistent weight using a drying oven (GCA, model 18 EM, Precision Scientific group, Chicago, IL, USA) at 85°C for 24 h.
Blood sampling protocol
Fish were anesthetized with 100 μg ml−1 MS222 (Tricaine methane-sulfonate, Sigma- Aldrich Co. LLC.) before blood collection. Blood samples were collected from two fish from each aquarium (ten fish per treatment) at random (42). Blood samples were taken from the caudal vein using 2.5 ml sterile syringes and split into equal parts. The first part was stored in a heparinized tube for hematological measurements. In contrast, the second portion was allowed to clot for 30 mins at ambient temperature before being stored in a refrigerator at 4°C for 3 h. Afterward, the clotted samples were centrifuged at 3,000 rpm for 10 mins at 4°C to extract serum, which was kept at −20°C until further biochemical, antioxidant, and immunological investigation. All samples have always been analyzed within 30 days after being kept frozen.
Blood hematological assessments
Following Stoskopf (43) procedure, the erythrocytes and leukocytes counts were estimated using a hemocytometer and Natt-Herrik solution. While, the hemoglobin level was determined using the cyanmethaemoglobin procedure as endorsed by Balasubramaniam and Malathi (44). Moreover, the microhematocrit method was applied for estimation of the PCV% and calculation MCV, MCH, and MCHC (45). To determine the differential leukocytic count, blood smear slides were prepared, air dried, fixed with methanol for 3–5 min, stained with Giemsa stain for 8–10 min, washed with distilled water, and after left to dry under room temperature following Blaxhall and Daisley (46) technique.
Blood biochemical analysis
Blood protein content (TP: total protein; ALB: albumin) was estimated via colorimetrical technique, whereas globulin (GB) level was determined by subtracting albumin value from total protein concentration. Moreover, liver function enzymes (ALT: alanine transaminase; AST: aspartate transaminase) were estimated using commercial kits (Assay Kit, 384 well, Colorimetric/Fluorometric, ABACM241035) following Wilkinson et al., (47) procedure. In addition, triglycerides, cholesterol and glucose concentrations were measured using commercial kits (Bio-Diagnostic Co. Egypt) by applying colorimetric methods following Fossati and Prencipe (48), Richmond (49) and Caraway (50) procedures, respectively.
Serum antioxidant and immunity parameters
The activity of serum oxidative remarks, including catalase (CAT), superoxide dis-mutase (SOD) and malonaldehyde (MDA) was estimated using the specific-commercial kits (Bio-diagnostic Co., Egypt). While, Cortisol level was assessed using Fluorescence Immunoassay rapid quantitative procedure applying a specific-commercial kit and FIA meter (Finecare, FIA meter plus, Guangzhou, Wondfo, Biotech. Co., China). The determination process was operated owing to product guidelines.
Immunoglobulin M (IgM) level was estimated via applying ELISA technique using a commercial kit (Fish Immunoglobulin M, ELISA Kit, Cat.No:MBS042385, My-BioSource, Co., Southern California, San Diego, USA) as ascribed by Wuthiekanun et al. (51). The lysozyme activity was evaluated using the Micrococcus lysodeikticus (Sigma, USA) technique at 450 nm using microplate ELISA reader as described by Demers and Bayne (52) procedure. The Cai et al. (53) method was applied to assess leukocyte phagocytic function activity. Furthermore, the used bacterial strain (Aeromonas hydrophila, 1 × 106) was obtained from the Department of Fish Diseases and Management, Sakha Aquaculture Research Unit, Central Lab. For Aquaculture Research, A.R.C., Egypt. The applied bacterial strain was identified using morphological and biochemical features, as described by Joseph and Carnahan (54).
Intestinal histological description, histomorphometric, and digestive enzymes analysis
The intestinal samples were eviscerated, weighed to 1 g, and blended with an appropriate quantity of phosphate buffer saline (PBS) solution (at a weight ratio of 1 g sample per 4 ml PBS) according to Kiernan (55) method and then transferred into a 10 mL Eppendorf tube. After that emulsified completely with an electric homogenizer in ice water bath for 15 s. Samples were centrifuged at 12,000 rpm at 4°C for 20 min. Lastly, the liquid supernatant was isolated for digestive enzymes estimation. The activity of the digestive enzymes, including Lipase (REF:281 001 Spectrum, Egyptian Co. Biotechnology, Egypt) and Amylase (CAT. NO. AY 10 50 Bio-diagnostic Co. Egypt) were estimated using commercial kits. The analysis procedure was directed according to the manufacture's instruction.
Anterior intestines of three fish per aquarium were gathered, removed, retained in Bouin's fixative solution for 24 h and then reserved in 70% absolute ethanol. Prepared samples for light microscopy were dehydrated in a graded concentration of ethanol, then cleared in xylene and fixed in paraffin wax. Hereafter, approximately 0.5 cm length segments of the anterior intestine were sliced transversely into 5 μm sections by microtome (Manually operated Rotary Microtome CUT 4055, D-69190 Walldorf, Germany) and stained with haematoxylin and eosin (HE) pigments. The prepared slides were inspected under a light microscope (Nikon, Tokyo, Japan) to determine the morphological parameters. The length, width of intestinal villi and crypt depth, inter-villi space and goblet cell count were estimated using image analysis software (NIH, Bethesda, MD) (30 measurements per fish, three fish per aquarium). The villi surface area was computed following Sakamoto et al. (56) formula.
RNA extraction, and quantitative real-time PCR
Total RNA was isolated and extracted from the liver samples using Trizol reagents (iNtRON Biotechnology) according to the manufacturer's instructions. To prevent RNA contamination, 2 μl of RNase was blended with 20 μl of DNA dissolved in Tris-buffer solution (pH = 8.0) and incubated for 3–4 h at 37°C. Then, RNA concentration was determined by Nanodrop (Quawell, USA). Real-time PCRs were achieved for selected genes, including interleukin-8 (IL-6), interleukin-1β (IL-1β), heat shock protein (HSP70), and growth hormone (GH). The β-actin and GAPDH genes were the main housekeeping genes to normalize cDNA loading. The primers employed in the present study are illustrated in Table 2. Real-time PCR amplifications were performed following the Pereira-Gomez et al. (57) procedure using SensiFast SYBR Lo-Rox kit (Bioline) in 20 μl reaction mixtures containing 2 μl of cDNA, the gene-specific primers (0.5 μM each); and SYBR 10 μl. The conditions for the thermal cycling were initial denaturation at 95°C for 10 min, followed by 40 cycles at 95°C for 15 s and 60°C for 1 min. The estimated genes were performed in triplicate. The fold change was estimated using the 2−ΔΔCT formula (58).
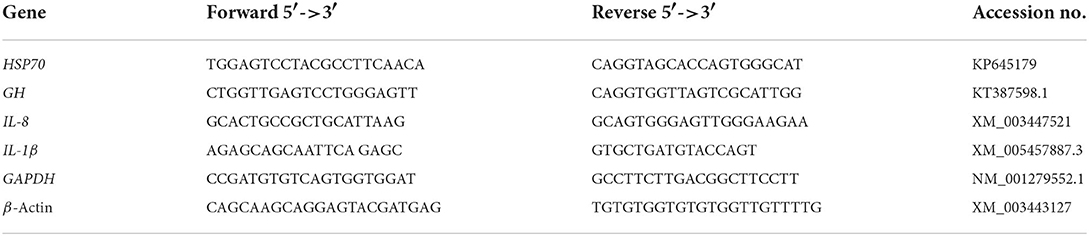
Table 2. The sequences of applicable primers used for real-time q-PCR investigation of gene expression.
Ethical approval statements
This in-vivo study was carried out in faithful agreement with the ethical guidelines of the Ethical principles of the Experimental Animal Welfare Ethics Committee of Zagazig University. The protocol was approved by the Committee on Research Ethics of the department of Animal Production, Zagazig University. Whereas, all efforts were applied to minimize the suffering and painful for experimental fish.
Statistical procedure
The normality and homogeneity of data were examined using Shapiro-Wilk test. Furthermore, all computed and estimated data were subjected to a one-way ANOVA statistical analysis method and differences between the means were verified by Tukey range test. The tested level of significance was set at P < 0.05. The results are presented as mean ± pooled SE values and all statistical examinations were achieved using SPSS 22.0 (SPSS Inc., 2013, USA).
Results
Growth performance and feed utilization
At the end of a 14-week feeding trial, compared to the control group administered un-supplemented diets, the fish groups fed BG plus BC2 had markedly higher final biomass, cumulative body gain, and average daily growth (P > 0.001), as well as a higher protein efficiency ratio (Table 3). Furthermore, dietary supplementation with β-glucan in combination with any dose of B. coagulans could significantly improve SGR and RGR compared to the control group (P < 0.001). Even though there was no significant variation in FCR values due to the effects of feed additives, the lowest FCR values were recorded in the BC2 groups alone or in combination with BG.
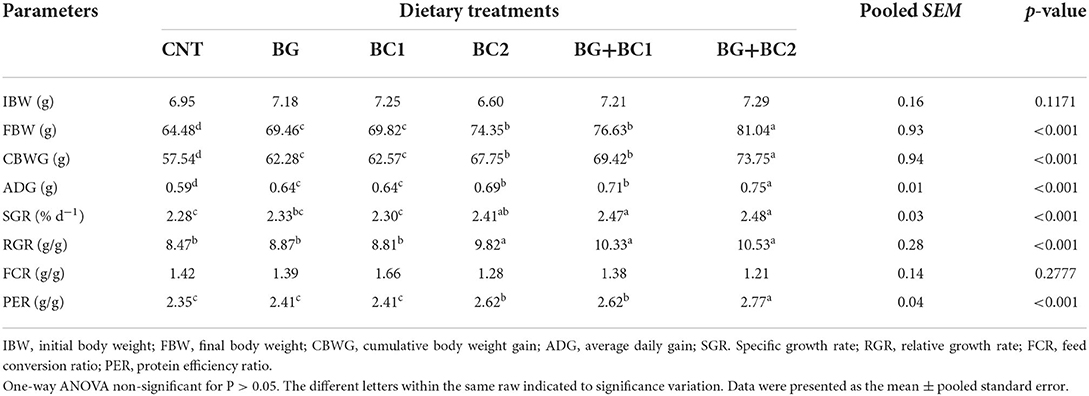
Table 3. Effects of dietary supplementation with β-glucan and/or Bacillus coagulans on performance, and efficiency of feed of tilapia fish.
Fish body chemical analysis
Dietary supplementation significantly altered the moisture content, dry matter, crude protein, crude lipid, and crude ash of the total body of fish among the treated groups (P > 0.001) (Table 4). When dietary supplements BG were paired with a high amount of BC, the moisture content, crude fat, and crude ash were significantly reduced compared to the control group. Conversely, fish fed high doses of B. coagulans alone or in combination with BG had considerably higher crude protein and dry matter levels in their bodies.
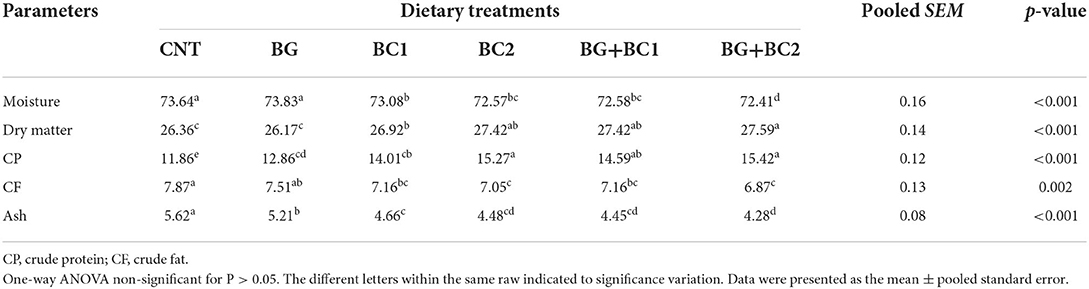
Table 4. Effects of dietary supplementation with β-glucan and/or Bacillus coagulans on whole body composition (% wet weight basis) of tilapia fish.
Blood hematological differentiations
Red blood cell, hemoglobin, and PCV concentrations were significantly (P < 0.05 or 0.001) improved in all fish groups fed any dose of BC alone or in combination with BG compared to other treatments (Table 5). Moreover, the highest RBC, Hb, and PCV levels were found in the tilapia group fed BG with a low dose of BC. In the same context, all fish groups fed BC1 alone or in combination with BG significantly (P ? 0.05 or 0.001) increased white blood cell counts, a lymphocyte level, and lymphocyte percentage compared to other treatments. While, dietary supplements did not affect MCV, MCH, MCHC, monocytes, eosinophil, or basophil levels.
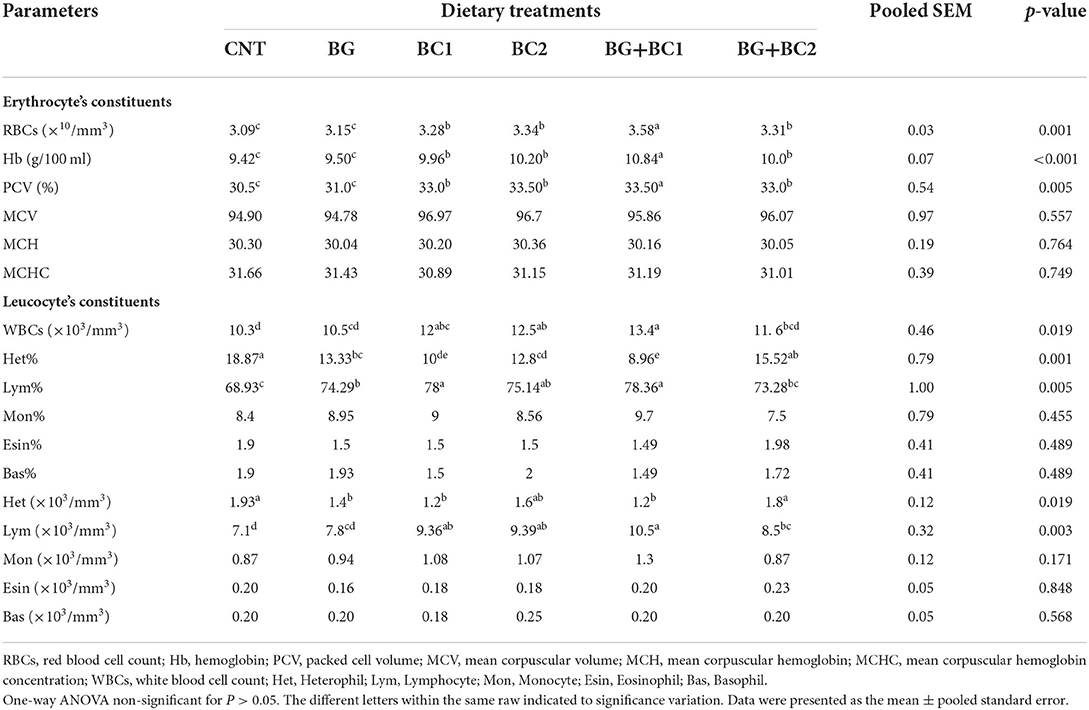
Table 5. Effects of dietary supplementation with β-glucan and/or Bacillus coagulans on blood hematological measurements of tilapia fish.
Blood biochemical profile, antioxidant and immunity parameters
Results of serum biochemical profiles, oxidative remarks activities and immune response were exhibited in Table 6. The serum total protein, albumin, and globulin levels in the BG plus BC1 group were significantly higher than in the other treated groups (P > 0.05 or 0.001). At the same time, the control and all supplemented groups had no significant (P > 0.05) difference in AST, ALT, total glyceride, cholesterol, and glucose levels.
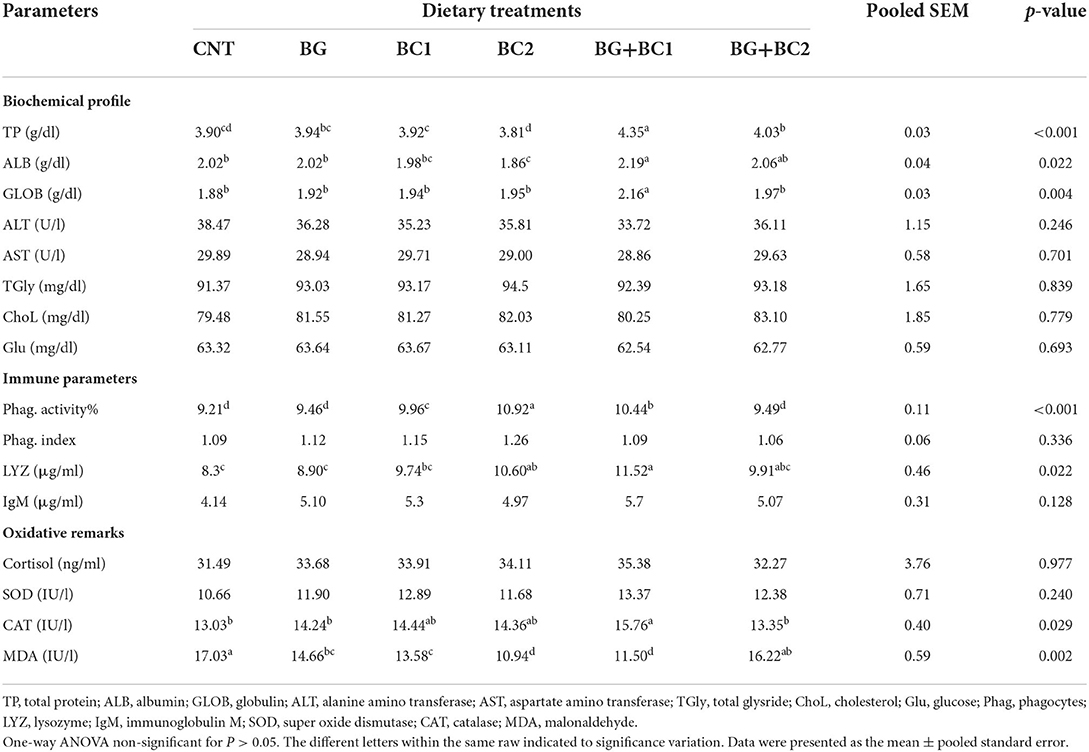
Table 6. Effects of dietary supplementation with β-glucan and/or Bacillus coagulans on blood biochemical profile, oxidative remarks and immune activity of tilapia fish.
According to the immunity data presented in Table 6, the serum phagocytic activity was significantly (P > 0.001) stimulated in the BC2 group, followed by the BG+BC1 fish group, in which the effects of BC supplementation alone or in combination with BG significantly (P > 0.05) activated lysozyme concentrations compared to the other two groups. Whereas no significant variances in phagocytic index or IgM concentrations were detected between the treatment and control groups (P > 0.05).
According to the oxidative remarks findings in Table 6, the BC2 and BG+BC1 groups had the lowest MDA content. In contrast, the CAT level in the BG+BC1 group was significantly (P > 0.05) higher than in the other groups. While, cortisol and SOD activity levels had no significant effects on any of the groups studied.
Digestive enzymes and intestinal histomorphometry indices
The digestive enzymes and morphometric indices of the intestine were influenced by supplementation with β-glucan and/or B. coagulans (Table 7). There are no significant (P > 0.05) variations in amylase and lipase activity across all treated and non-treated groups.
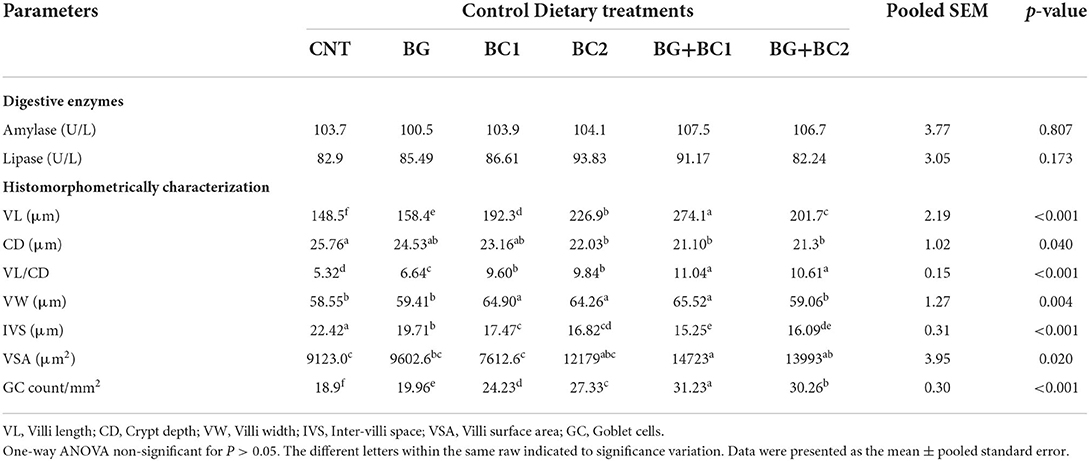
Table 7. Effects of dietary supplementation with β-glucan and/or Bacillus coagulans on intestinal digestive enzymes and histomorphometry features of tilapia fish.
Owing to the histomorphometrically features of small intestine, the villus length increased significantly (P > 0.001) when BG was combined with BC1. However, the crypt depth and inter villi space decreased significantly (P > 0.05) in all fish supplemented groups. The fish-fed supplemented diets with BG and any quantity of BC had the lowest crypt depth and inter villi spacing values. In contrast, the villus length per crypt depth ratio improved significantly (P > 0.001) in fish groups fed BG supplemented diets in combination with any amount of BC compared to the control group. Conversely, villus width increased significantly (P > 0.01) in fish groups receiving BC supplemented diets alone or in combination with BG compared to the control group. Compared to other groups, the villus surface area and goblet cell count significantly (P > 0.01 or 0.001) improved in fish fed BG+BC1, BG+BC2, and BG2, respectively.
Anterior intestinal morphometric structures
Light microscopy has been applied to estimate the morphological features of the anterior section of the small intestine (Figure 1). The histological examination of the intestinal villi revealed a higher length and normal structure, which confirms that the tilapia intestine is out of inflammation. The fish group that received BG + BC1 supplemented diets had the maximum villus length compared to other groups.
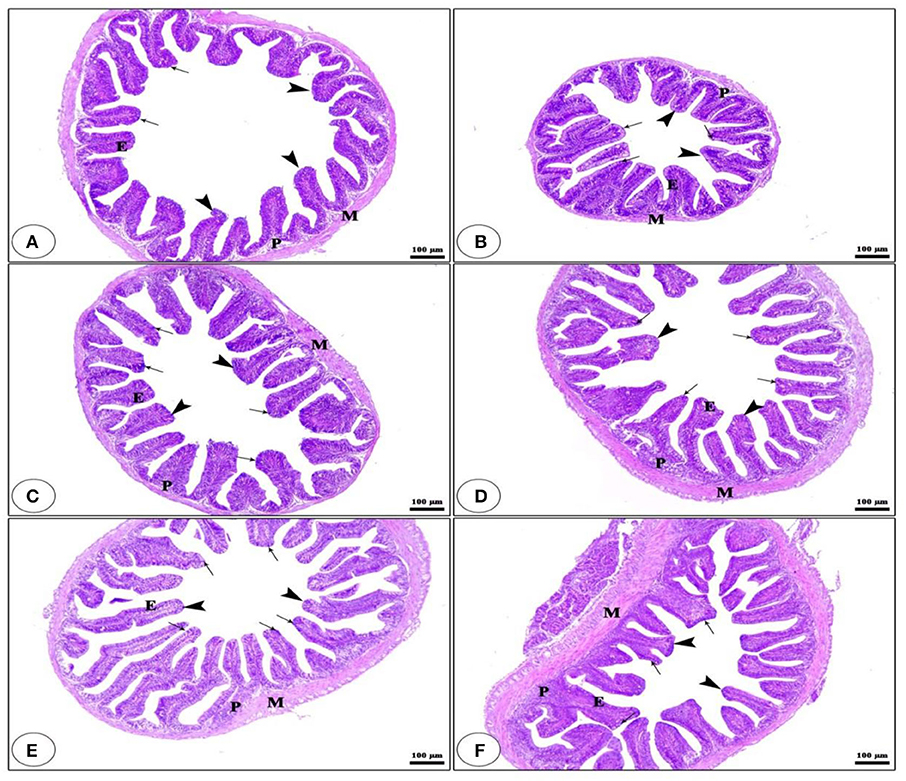
Figure 1. Histological description for transversal section photomicrograph of O. niloticus anterior part of intestine. While, CNT (A), BG (B), BC1 (C), BC2 (D), BG + BC1 (E), and BG + BC2 (F). The intestinal villi (arrow heads) with intact simple columnar epithelium (E) rested on lamina propria of loose connective tissue (p) and lamina muscularis (M) with numerous goblet cells (arrows) present in the lamina epithelia. The examined sections stained with H&E (×100 μm).
m-RNA gene expression
The mRNA level of the HSP7 gene was significantly lowered in all supplemented diets, with the maximum significance decrease observed under the impact of BG+BC1 or BC2 dietary supplementation compared to the control group (Figure 2). In contrast, feeding BG or/and BC elevated GH, IL-1β, and IL-8 transcription compared to the control group. However, high levels of GH transcription were identified in fish fed low levels of BC, whereas IL-1β levels increased under the impacts of BC1 alone or in combination with BG compared to the control group and other treated groups. Finally, IL-8 transcription was activated at a higher level in fish groups that received BG in combination with any amount of BC compared to other experimental groups.
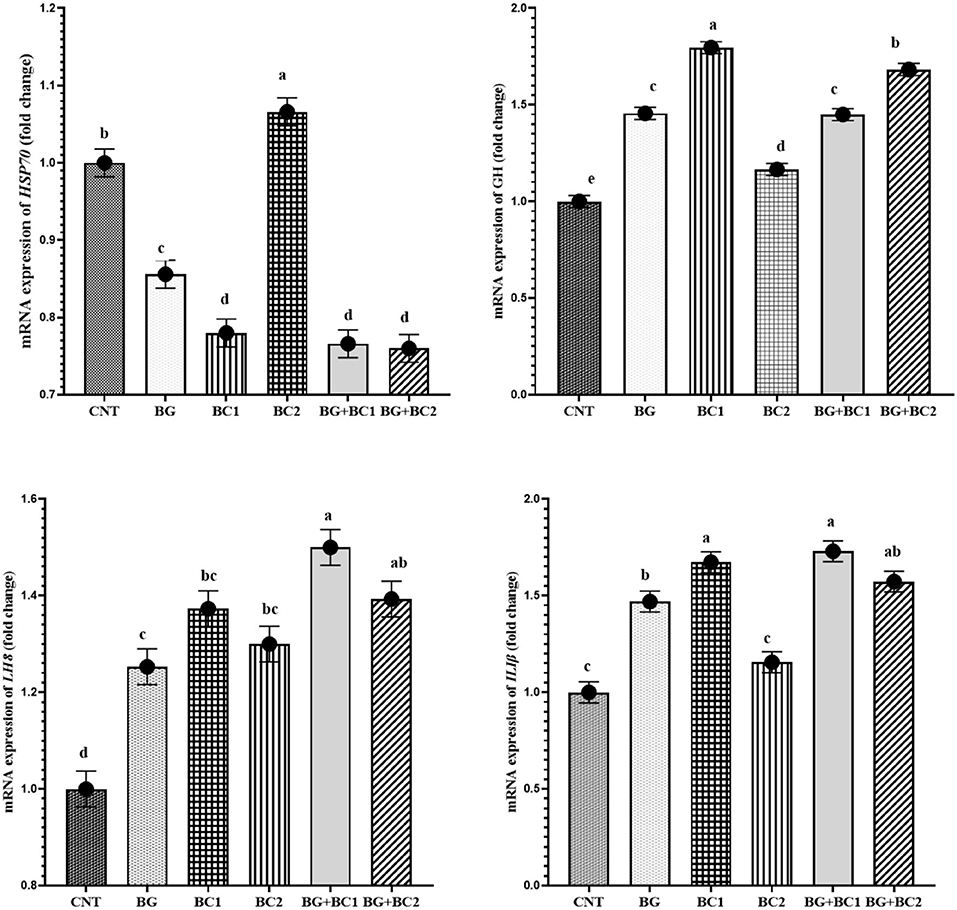
Figure 2. mRNA expression levels of heat shock protein-70 (HSP70), growth hormone (GH), interleukin-1 beta (IL-Iβ) and interleukin-8 (IL-8) genes in the liver tissue of Nile tilapia, Oreochromis niloticus (mean ± MSE) fed on diets supplemented with graded levels of β-glucan and/or Bacillus coagulans for 14 weeks. Columns with different superscribt letters are significantly different (P < 0.05).
Discussion
Although numerous research has been conducted on applying β-glucan or probiotics in tilapia diets (59), the current trial is the first to describe the relevance of employing both β-glucan and B. coagulans on tilapia growth and performance. The feeding trial findings showed that incorporating BG and B. coagulans in O. niloticus diets significantly influenced growth performance indices. The BG/BC mixture contains β-glucan and B. coagulans, associated with increased growth and feed efficiency in fish. While, the current experiment merge between BG and BC in promoting O. niloticus growth is owing to BC's function in boosting the variety of beneficial bacteria in the gastrointestinal tract of fish (60). The development of beneficial bacterial strains in the digestive tract facilitates nutrient digestion and absorption through epithelial cells (61).
Furthermore, dominating the beneficial microorganisms in the intestinal microbiota reduces the influence of pathogenic bacteria on intestinal immunity (62). Thus, the general body's immune system is connected to the intestine's immunity and health (63). The same effects are generated by prebiotics, specifically β-glucan, which encourage the development and activity of the desirable healthy intestine microbial population while suppressing pathogen colonization and preventing inflammation (64). Based on this hypothesis, both BG and BC synergistically impacted the performance of O. niloticus.
The possible significance of BG/BC may also be linked to their function in enhancing feed intake by promoting feed palatability, which subsequently leads to enhanced feed efficiency (20, 32). Feeding O. niloticus with Bacillus sp. improved growth performance and feed efficiency (65), whereas feeding with BG also affected growth rates, as previously documented by Pilarski et al. (20).
The improved feed efficiency may be explained by referring to how BG/BC affects gastrointestinal morphometry indices (66). The findings showed that feeding fish BG and BC positively impacted the length, surface area and width of the intestinal villi and the number of goblet cells. These findings support the synergistic role of both BG and BC in increasing the absorption capacity of intestinal barriers, allowing enough quantities of nutrients to be digested for biological and metabolic activities in fish tissues (1, 67). Furthermore, the increased number of goblet cells is linked to their function in defending the intestinal membranes from dangerous germs by producing glycoprotein and antibacterial substances (68). Likewise, Ghalwash et al. (69) study demonstrated that Bacillus spp. dietary incorporation into tilapia diets improved all intestinal histomorphometric parameters.
Feed additives are often claimed to be the main cause of enhanced or reduced hematological and biochemical variables in fish compared to normal values (70). The findings showed that fish fed BG and BC, alone or in combined form had normal biochemical levels that were regarded within the typical range for healthy fish. In parallel, fish fed BG/BC1 had increased total protein, albumin, and globulin levels compared to other treated groups. Moreover, it has been observed that a low level of Bacillus spp. in combined with beta-glucan increases the total protein contents in Nile tilapia (71, 72). Thus, supplementing fish feed with probiotics or prebiotics promotes intestinal immunological responses against pathogens, including humoral and cell-mediated responses, results in an increment in immunoglobulin levels in the blood and an increase in total protein (73).
Phagocytes are white blood cells continuously generated from the bone marrow and have been identified as responsible for removing dead cells and invading bacteria (74). Furthermore, white blood cells are part of the cellular-immune system, which protects the fish body from infectious diseases (75). Moreover, lysozyme, a small cationic protein, destroys or kills bacteria by lysing their cell wall peptidoglycan, breaking bacterial membranes, and activating autolytic enzymes in the bacterial cell wall (76). Our research findings concluded that the impact of BC alone or combined with BG supplemented diets boost cellular-immune activity. Major probiotic mechanisms of action involve epithelial barrier improvements, enhanced adherence to the intestinal mucosa, and associated inhibition of pathogen adhesion, competitive exclusion of pathogenic bacteria, generation of anti-microorganism molecules, and innate immune system regulation (77). Specifically, probiotic-based diets could stimulate cellular immune response features through the activation of macrophages, natural killer (NK) cells, antigen-specific cytotoxic T-lymphocytes, and the release of various cytokines in a strain-specific and dose-dependent manner (78).
Probiotics and prebiotics are well known for their protective role against oxidative stress in fish (79, 80). Under stressful situations, reactive oxygen species (ROS) may be produced in large quantities, damaging the cellular membrane by causing lipid peroxidation (81). Biologically, the cell triggered many internal anti-oxidative reactions to deal with the harmful effects of ROS on the cellular membrane by boosting the release of anti-oxidative enzymes such as Catalase (CAT), and alleviating the MDA activation (82). The present investigation shows higher CAT activity and lower MDA levels in fish fed BG and BC, consistent with previous reports (1, 67, 83). Kim et al. (84) reported higher CAT activities after being pre-treated with β-glucan for 15 days in grass carp. The increased CAT activities and lower MDA levels might be attributed to distinct activation of up-regulating antioxidant-related enzyme gene expression via the antioxidant properties of β-glucan (84). Furthermore, fish administered BC had higher CAT, which the beneficial bacterial population may explain in boosting the overall immune response (85).
When studying the influence of functional feed additives on aquatic organisms, the transcription of some growth, immunological, and stress-associated genes is often used to understand the mechanism of action on a genetic basis (86). Under stress, fish cells secrete high heat shock protein 70 (HSP70), which increases protein integrity and reduces apoptosis (87). The current results exhibited HSP70 downregulated in tilapia fish received diets supplemented with BG/BC combination, which is connected with the possible function of BG and BC in sustaining fish health. Furthermore, the lower mRNA level of the HSP70 gene indicates that the tilapia is not subjected to stressful rearing conditions (88). Moreover, it is well known that growth hormone (GH) regulates various key physiological activities in fish, such as mineral homeostasis, growth, and metabolism (89). The findings of our study showed that the impacts of BC alone or in combination with BG resulted in increased GH expression levels in tilapia fish. These findings were consistent with other previous reports (20, 90). Probiotics significantly altered the expression of growth-related genes, demonstrating a desirable influence of these probiotics in overall fish metabolic activities (4, 91). Interleukin genes were estimated to maintain growth, differentiation, and activation during inflammatory and immunological responses (92). The gene findings showed that fish fed BG/BC had higher levels of interleukin-1 beta (IL-Iβ) and interleukin-8 (IL-8). The activation of the IL-8 and IL-Iβ genes in response to BG and BC supplemented diets verified the synergistic protective potential function of these mixtures in attracting and activating neutrophils in inflammatory regions and promoting the immune system response function and overall health status of fish (17, 93).
Conclusion
Finally, the current findings revealed that dietary supplementation with B. coagulans alone or combined with beta glucan might improve tilapia growth performance. Furthermore, supplementation of feed additives (β-glucan and B. coagulans) might boost fish health by promoting immune responses and antioxidant capacity and altering some associated blood biochemical and hematological parameters in tilapia.
Data availability statement
The all data presented in the study are available under reasonable request from the corresponding author.
Ethics statement
The animal study was reviewed and approved by the Ethical Principles of the Experimental Animal Welfare Ethics Committee of Zagazig University (No. ZU-IACUC/2/F/110/2022).
Author contributions
AF, IE-R, AN-A, AE-R, and AE designed the experiment and drafted the manuscript. AE-R, MA-E, and MN conducted the trial, sample collection, analysis, data collection, and wrote the manuscript. KM, HS, MS, MJ, and MA assisted in sample collection, induced breeding, data analysis, and revised the final drafted manuscript. All named authors have significantly contributed toward the final version of this research study and approval for publication.
Conflict of interest
The authors declare that the research was conducted in the absence of any commercial or financial relationships that could be construed as a potential conflict of interest.
Publisher's note
All claims expressed in this article are solely those of the authors and do not necessarily represent those of their affiliated organizations, or those of the publisher, the editors and the reviewers. Any product that may be evaluated in this article, or claim that may be made by its manufacturer, is not guaranteed or endorsed by the publisher.
References
1. Cao H, Yu R, Zhang Y, Hu B, Jian S, Wen C, et al. Effects of dietary supplementation with β-glucan and Bacillus subtilis on growth, fillet quality, immune capacity, and antioxidant status of Pengze crucian carp (Carassius auratus var. Pengze). Aquaculture. (2019) 508:106–12. doi: 10.1016/j.aquaculture.2019.04.064
2. Limbu SM. Antibiotics use in African aquaculture: their potential risks on fish and human health. in Current Microbiological Research in Africa. Cham: Springer (2020). p. 203–21. doi: 10.1007/978-3-030-35296-7_8
3. Limbu SM, Chen LQ, Zhang ML, Du ZY. A global analysis on the systemic effects of antibiotics in cultured fish and their potential human health risk: a review. Rev Aquacult. (2021) 13:1015–59. doi: 10.1111/raq.12511
4. Naiel ME, Shehata AM, El-kholy AI, El-Naggar K, Farag MR, Alagawany M. The mitigating role of probiotics against the adverse effects of suboptimal temperature in farmed fish: a review. Aquaculture. (2022) 2022:737877. doi: 10.1016/j.aquaculture.2021.737877
5. Naiel MA, Farag MR, Gewida AG, Elnakeeb MA, Amer MS, Alagawany M. Using lactic acid bacteria as an immunostimulants in cultured shrimp with special reference to Lactobacillus spp. Aquac Int. (2021) 29:219–31. doi: 10.1007/s10499-020-00620-2
6. Yilmaz S, Yilmaz E, Dawood MA, Ringø E, Ahmadifar E, Abdel-Latif HM. Probiotics, prebiotics, and synbiotics used to control vibriosis in fish: a review. Aquaculture. (2022) 547:737514. doi: 10.1016/j.aquaculture.2021.737514
7. Meena D, Das P, Kumar S, Mandal S, Prusty A, Singh S, et al. Beta-glucan: an ideal immunostimulant in aquaculture (a review). Fish Physiol Biochem. (2013) 39:431–57. doi: 10.1007/s10695-012-9710-5
8. Han B, Baruah K, Cox E, Vanrompay D, Bossier P. Structure-functional activity relationship of β-glucans from the perspective of immunomodulation: a mini-review. Front Immunol. (2020) 2020:658. doi: 10.3389/fimmu.2020.00658
9. Mueller A, Raptis J, Rice PJ, Kalbfleisch JH, Stout RD, Ensley HE, et al. The influence of glucan polymer structure and solution conformation on binding to (1 → 3)-β-D-glucan receptors in a human monocyte-like cell line. Glycobiology. (2000) 10:339–46. doi: 10.1093/glycob/10.4.339
10. Zeng L, Wang Y-H, Ai C-X, Zheng J-L, Wu C-W, Cai R. Effects of β-glucan on ROS production and energy metabolism in yellow croaker (Pseudosciaena crocea) under acute hypoxic stress. Fish Physiol Biochem. (2016) 42:1395–405. doi: 10.1007/s10695-016-0227-1
11. Ghaedi G, Keyvanshokooh S, Azarm HM, Akhlaghi M. Effects of dietary β-glucan on maternal immunity and fry quality of rainbow trout (Oncorhynchus mykiss). Aquaculture. (2015) 441:78–83. doi: 10.1016/j.aquaculture.2015.02.023
12. Do Huu H, Sang HM, Thuy NTT. Dietary β-glucan improved growth performance, Vibrio counts, haematological parameters and stress resistance of pompano fish, Trachinotus ovatus Linnaeus, 1758. Fish Shellfish Immunol. (2016) 54:402–10. doi: 10.1016/j.fsi.2016.03.161
13. Pionnier N, Falco A, Miest J, Frost P, Irnazarow I, Shrive A, et al. Dietary β-glucan stimulate complement and C-reactive protein acute phase responses in common carp (Cyprinus carpio) during an Aeromonas salmonicida infection. Fish Shellfish Immunol. (2013) 34:819–31. doi: 10.1016/j.fsi.2012.12.017
14. Reyes-Becerril M, Guardiola FA, Sanchez V, Maldonado M, Angulo C. Sterigmatomyces halophilus β-glucan improves the immune response and bacterial resistance in Pacific red snapper (Lutjanus peru) peripheral blood leucocytes: in vitro study. Fish Shellfish Immunol. (2018) 78:392–403. doi: 10.1016/j.fsi.2018.04.043
15. Bai N, Gu M, Zhang W, Xu W, Mai K. Effects of β-glucan derivatives on the immunity of white shrimp Litopenaeus vannamei and its resistance against white spot syndrome virus infection. Aquaculture. (2014) 426:66–73. doi: 10.1016/j.aquaculture.2014.01.019
16. Lin S, Pan Y, Luo L, Luo L. Effects of dietary β-1, 3-glucan, chitosan or raffinose on the growth, innate immunity and resistance of koi (Cyprinus carpio koi). Fish Shellfish Immunol. (2011) 31:788–94. doi: 10.1016/j.fsi.2011.07.013
17. Ai Q, Mai K, Zhang L, Tan B, Zhang W, Xu W, et al. Effects of dietary β-1, 3 glucan on innate immune response of large yellow croaker, Pseudosciaena crocea. Fish Shellfish Immunol. (2007) 22:394–402. doi: 10.1016/j.fsi.2006.06.011
18. Ji L, Sun G, Li J, Wang Y, Du Y, Li X, et al. Effect of dietary β-glucan on growth, survival and regulation of immune processes in rainbow trout (Oncorhynchus mykiss) infected by Aeromonas salmonicida. Fish Shellfish Immunol. (2017) 64:56–67. doi: 10.1016/j.fsi.2017.03.015
19. Zhao Y, Ma H, Zhang W, Ai Q, Mai K, Xu W, et al. Effects of dietary β-glucan on the growth, immune responses and resistance of sea cucumber, Apostichopus japonicus against Vibrio splendidus infection. Aquaculture. (2011) 315:269–74. doi: 10.1016/j.aquaculture.2011.02.032
20. Pilarski F, de Oliveira CAF, de Souza FPBD, Zanuzzo FS. Different β-glucans improve the growth performance and bacterial resistance in Nile tilapia. Fish Shellfish Immunol. (2017) 70:25–9. doi: 10.1016/j.fsi.2017.06.059
21. Lu D-L, Limbu SM, Lv H-B, Ma Q, Chen L-Q, Zhang M-L, et al. The comparisons in protective mechanisms and efficiencies among dietary α-lipoic acid, β-glucan and l-carnitine on Nile tilapia infected by Aeromonas hydrophila. Fish Shellfish Immunol. (2019) 86:785–93. doi: 10.1016/j.fsi.2018.12.023
22. Bagni M, Romano N, Finoia M, Abelli L, Scapigliati G, Tiscar PG, et al. Short-and long-term effects of a dietary yeast β-glucan (Macrogard) and alginic acid (Ergosan) preparation on immune response in sea bass (Dicentrarchus labrax). Fish Shellfish Immunol. (2005) 18:311–25. doi: 10.1016/j.fsi.2004.08.003
23. Dawood MA, Abdel-Razik NI, Gewaily MS, Sewilam H, Paray BA, Soliman AA, et al. β-Glucan improved the immunity, hepato-renal, and histopathology disorders induced by chlorpyrifos in Nile tilapia. Aquaculture Reports. (2020) 18:100549. doi: 10.1016/j.aqrep.2020.100549
24. Yang G, Peng M, Tian X, Dong S. Molecular ecological network analysis reveals the effects of probiotics and florfenicol on intestinal microbiota homeostasis: an example of sea cucumber. Sci Rep. (2017) 7:1–12. doi: 10.1038/s41598-017-05312-1
25. Sánchez B, Delgado S, Blanco-Míguez A, Lourenço A, Gueimonde M, Margolles A. Probiotics, gut microbiota, and their influence on host health and disease. Mol Nutr Food Res. (2017) 61:1600240. doi: 10.1002/mnfr.201600240
26. Thomas LV, Suzuki K, Zhao J. Probiotics: a proactive approach to health. A symposium report. Br J Nutr. (2015) 114:S1–S15. doi: 10.1017/S0007114515004043
27. Nayak SK. Probiotics and immunity: a fish perspective. Fish Shellfish Immunol. (2010) 29:2–14. doi: 10.1016/j.fsi.2010.02.017
28. Pérez-Sánchez T, Ruiz-Zarzuela I, de Blas I, Balcázar JL. Probiotics in aquaculture: a current assessment. Rev Aquacult. (2014) 6:133–46. doi: 10.1111/raq.12033
29. Abdel-Latif HM, Yilmaz E, Dawood MA, Ringø E, Ahmadifar E, Yilmaz S. Shrimp vibriosis and possible control measures using probiotics, postbiotics, prebiotics, and synbiotics: a review. Aquaculture. (2022) 2022:737951. doi: 10.1016/j.aquaculture.2022.737951
30. Jenson I. BACILLUS | Introduction. In: Batt CA, Tortorello ML, editors. Encyclopedia of Food Microbiology (Second Edition). Oxford: Academic Press (2014). p. 111–7.
31. Xu Y, Wang Y, Lin J. Use of Bacillus coagulans as a dietary probiotic for the common Carp, Cyprinus carpio. J World Aquac Soc. (2014) 45:403–11. doi: 10.1111/jwas.12139
32. Wang Y. Use of probiotics Bacillus coagulans, Rhodopseudomonas palustris and Lactobacillus acidophilus as growth promoters in grass carp (Ctenopharyngodon idella) fingerlings. Aquac Nutr. (2011) 17:e372–e8. doi: 10.1111/j.1365-2095.2010.00771.x
33. Wang Y, Fu L, Lin J. Probiotic (Bacillus coagulans) cells in the diet benefit the white shrimp Litopenaeus vannamei. J Shellfish Res. (2012) 31:855–60. doi: 10.2983/035.031.0333
34. Mehisan M, El-moghazy G, El-Sayed AE-K, Iraqi M, Soltan M. Role of probiotics in improving growth performance, immunity and controlling Aeromonas hydrophila in the Nile tilapia Oreochromis niloticus. Egypt J Aquat Biol Fish. (2015) 19:55–70. doi: 10.21608/ejabf.2015.2271
35. Zhou X, Tian Z, Wang Y, Li W. Effect of treatment with probiotics as water additives on tilapia (Oreochromis niloticus) growth performance and immune response. Fish Physiol Biochem. (2010) 36:501–9. doi: 10.1007/s10695-009-9320-z
36. Amoah K, Huang Q-C, Tan B-P, Zhang S, Chi S-Y, Yang Q-H, et al. Dietary supplementation of probiotic Bacillus coagulans ATCC 7050, improves the growth performance, intestinal morphology, microflora, immune response, and disease confrontation of Pacific white shrimp, Litopenaeus vannamei. Fish Shellfish Immunol. (2019) 87:796–808. doi: 10.1016/j.fsi.2019.02.029
37. Chang X, Chen Y, Feng J, Huang M, Zhang J. Amelioration of Cd-induced bioaccumulation, oxidative stress and immune damage by probiotic Bacillus coagulans in common carp (Cyprinus carpio L). Aquaculture Reports. (2021) 20:100678. doi: 10.1016/j.aqrep.2021.100678
38. Amphan S, Unajak S, Printrakoon C, Areechon N. Feeding-regimen of β-glucan to enhance innate immunity and disease resistance of Nile tilapia, Oreochromis niloticus Linn, against Aeromonas hydrophila and Flavobacterium columnare. Fish Shellfish Immunol. (2019) 87:120–8. doi: 10.1016/j.fsi.2018.12.062
40. Kumaree KK, Akbar A, Anal AK. Bioencapsulation and application of Lactobacillus plantarum isolated from catfish gut as an antimicrobial agent and additive in fish feed pellets. Ann Microbiol. (2015) 65:1439–45. doi: 10.1007/s13213-014-0982-0
41. AOAC. Official Analytical Chemists. Coffee and Tea Official Methods of Analysis, 17th ed. Gaithersburg, Maryland: AOAC (2000).
42. Naiel MA, Abdelghany MF, Khames DK, El-hameed A, Samah A, Mansour EM, et al. Administration of some probiotic strains in the rearing water enhances the water quality, performance, body chemical analysis, antioxidant and immune responses of Nile tilapia, Oreochromis niloticus. Appl Water Sci. (2022) 12:1–13. doi: 10.1007/s13201-022-01733-0
43. Stoskopf M. Fish Medicine. Philadelphia, London, Toronto, Montreal, Sydney, Tokyo: WB Saunders company Harcourt Brace Jovanovich Inc. (1993).
44. Balasubramaniam P, Malathi A. Comparative study of hemoglobin estimated by Drabkin's and Sahli's methods. J Postgrad Med. (1992) 38:8.
45. Dacie J, Lewis S. Miscellaneous Tests. Practical Haematology, 7th Edition. London: Churchill Livingstone (1991). p. 227–57.
46. Blaxhall P, Daisley K. Routine haematological methods for use with fish blood. J Fish Biol. (1973) 5:771–81. doi: 10.1111/j.1095-8649.1973.tb04510.x
47. Wilkinson J, Baron D, Moss D, Walker P. Standardization of clinical enzyme assays: a reference method for aspartate and alanine transaminases. J Clin Pathol. (1972) 25:940. doi: 10.1136/jcp.25.11.940
48. Fossati P, Prencipe L. Serum triglycerides determined colorimetrically with an enzyme that produces hydrogen peroxide. Clin Chem. (1982) 28:2077–80. doi: 10.1093/clinchem/28.10.2077
49. Richmond W. Preparation and properties of a cholesterol oxidase from Nocardia sp. and its application to the enzymatic assay of total cholesterol in serum. Clin Chem. (1973) 19:1350–6. doi: 10.1093/clinchem/19.12.1350
50. Caraway WT. A stable starch substrate for the determination of amylase in serum and other body fluids. Am J Clin Pathol. (1959) 32:97–9. doi: 10.1093/ajcp/32.1_ts.97
51. Wuthiekanun V, Amornchai P, Chierakul W, Cheng AC, White NJ, Peacock SJ, et al. Evaluation of immunoglobulin M (IgM) and IgG rapid cassette test kits for diagnosis of melioidosis in an area of endemicity. J Clin Microbiol. (2004) 42:3435–7. doi: 10.1128/JCM.42.8.3435-3437.2004
52. Demers NE, Bayne CJ. The immediate effects of stress on hormones and plasma lysozyme in rainbow trout. Develop Comp Immunol. (1997) 21:363–73. doi: 10.1016/S0145-305X(97)00009-8
53. Cai W-q, Li S-f, Ma J-y. Diseases resistance of Nile tilapia (Oreochromis niloticus), blue tilapia (Oreochromis aureus) and their hybrid (female Nile tilapia × male blue tilapia) to Aeromonas sobria. Aquaculture. (2004) 229:79–87. doi: 10.1016/S0044-8486(03)00357-0
54. Joseph SW, Carnahan A. The isolation, identification, and systematics of the motile Aeromonas species. Annu Rev Fish Dis. (1994) 4:315–43. doi: 10.1016/0959-8030(94)90033-7
55. Kiernan JA. Histological and Histochemical Methods: Theory and Practice. 3rd edition. Oxford: Oxford University Press (2003).
56. Sakamoto K, Hirose H, Onizuka A, Hayashi M, Futamura N, Kawamura Y, et al. Quantitative study of changes in intestinal morphology and mucus gel on total parenteral nutrition in rats. J Surg Res. (2000) 94:99–106. doi: 10.1006/jsre.2000.5937
57. Pereira-Gomez M, Fajardo A, Echeverria N, Lopez-Tort F, Perbolianachis P, Costabile A, et al. Evaluation of SYBR Green real time PCR for detecting SARS-CoV-2 from clinical samples. J Virol Methods. (2021) 289:114035. doi: 10.1016/j.jviromet.2020.114035
58. Livak KJ, Schmittgen TD. Analysis of relative gene expression data using real-time quantitative PCR and the 2−ΔΔCT method. methods. (2001) 25:402–8. doi: 10.1006/meth.2001.1262
59. Al-Deriny SH, Dawood MA, Abou Zaid AA, Wael F, Paray BA, Van Doan H, et al. The synergistic effects of Spirulina platensis and Bacillus amyloliquefaciens on the growth performance, intestinal histomorphology, and immune response of Nile tilapia (Oreochromis niloticus). Aquaculture Reports. (2020) 17:100390. doi: 10.1016/j.aqrep.2020.100390
60. Soltani M, Ghosh K, Hoseinifar SH, Kumar V, Lymbery AJ, Roy S, et al. Genus Bacillus, promising probiotics in aquaculture: aquatic animal origin, bio-active components, bioremediation and efficacy in fish and shellfish. Rev Fish Sci Aquacult. (2019) 27:331–79. doi: 10.1080/23308249.2019.1597010
61. Kavitha M, Raja M, Perumal P. Evaluation of probiotic potential of Bacillus spp. isolated from the digestive tract of freshwater fish Labeo calbasu (Hamilton, 1822). Aquacult Rep. (2018) 11:59-69. doi: 10.1016/j.aqrep.2018.07.001
62. Burr G, Gatlin D III, Ricke S. Microbial ecology of the gastrointestinal tract of fish and the potential application of prebiotics and probiotics in finfish aquaculture. J World Aquac Soc. (2005) 36:425–36. doi: 10.1111/j.1749-7345.2005.tb00390.x
63. Dawood MA. Nutritional immunity of fish intestines: important insights for sustainable aquaculture. Rev Aquacult. (2021) 13:642–63. doi: 10.1111/raq.12492
64. Yan Q, Li J, Yu Y, Wang J, He Z, Van Nostrand JD, et al. Environmental filtering decreases with fish development for the assembly of gut microbiota. Environ Microbiol. (2016) 18:4739–54. doi: 10.1111/1462-2920.13365
65. Ridha MT, Azad IS. Preliminary evaluation of growth performance and immune response of Nile tilapia Oreochromis niloticus supplemented with two putative probiotic bacteria. Aquaculture Res. (2012) 43:843–52. doi: 10.1111/j.1365-2109.2011.02899.x
66. Tengjaroenkul B, Smith BJ, Caceci T, Smith SA. Distribution of intestinal enzyme activities along the intestinal tract of cultured Nile tilapia, Oreochromis niloticus L. Aquaculture. (2000) 182:317–27. doi: 10.1016/S0044-8486(99)00270-7
67. Boonanuntanasarn S, Wongsasak U, Pitaksong T, Chaijamrus S. Effects of dietary supplementation with β-glucan and synbiotics on growth, haemolymph chemistry, and intestinal microbiota and morphology in the Pacific white shrimp. Aquacult Nutr. (2016) 22:837–45. doi: 10.1111/anu.12302
68. Lauriano E, Pergolizzi S, Aragona M, Montalbano G, Guerrera M, Crupi R, et al. Intestinal immunity of dogfish Scyliorhinus canicula spiral valve: a histochemical, immunohistochemical and confocal study. Fish Shellfish Immunol. (2019) 87:490–8. doi: 10.1016/j.fsi.2019.01.049
69. Ghalwash HR, Salah AS, El-Nokrashy AM, Abozeid AM, Zaki VH, Mohamed RA. Dietary supplementation with Bacillus species improves growth, intestinal histomorphology, innate immunity, antioxidative status and expression of growth and appetite-regulating genes of Nile tilapia fingerlings. Aquac Res. (2022) 53:1378–94. doi: 10.1111/are.15671
70. Femi-Oloye OP, Owoloye A, Olatunji-Ojo AM, Abiodun AC, Adewumi B, Ibitoye BO, et al. Effects of commonly used food additives on haematological parameters of Wistar rats. Heliyon. (2020) 6:e05221. doi: 10.1016/j.heliyon.2020.e05221
71. Azevedo RV. Pereira SL, Cardoso LD, Andrade DRd, Vidal Júnior MV. Dietary mannan oligosaccharide and Bacillus subtilis in diets for Nile tilapia (Oreochromis niloticus). Anim Sci. (2016) 38:347–53. doi: 10.4025/actascianimsci.v38i4.31360
72. de Souza FP, de Lima ECS, Pandolfi VCF, Leite NG, Furlan-Murari PJ, Leal CNS, et al. Effect of β-glucan in water on growth performance, blood status and intestinal microbiota in tilapia under hypoxia. Aquacult Rep. (2020) 17:100369. doi: 10.1016/j.aqrep.2020.100369
73. Hoseinifar SH, Sun Y-Z, Wang A, Zhou Z. Probiotics as means of diseases control in aquaculture, a review of current knowledge and future perspectives. Front Microbiol. (2018) 2018:2429. doi: 10.3389/fmicb.2018.02429
74. Secombes C, Fletcher T. The role of phagocytes in the protective mechanisms of fish. Annu Rev Fish Dis. (1992) 2:53–71. doi: 10.1016/0959-8030(92)90056-4
75. Haghighi M, Rohani MS. The effects of powdered ginger (Zingiber officinale) on the haematological and immunological parameters of rainbow trout Oncorhynchus mykiss. J Med Plant Herbal Therapy Res. (2013) 1:8–12. doi: 10.33500/jmphtr.2013.1.002
76. Whang I, Lee Y, Lee S, Oh M-J, Jung S-J, Choi CY, et al. Characterization and expression analysis of a goose-type lysozyme from the rock bream Oplegnathus fasciatus, and antimicrobial activity of its recombinant protein. Fish Shellfish Immunol. (2011) 30:532–42. doi: 10.1016/j.fsi.2010.11.025
77. Bermudez-Brito M, Plaza-Díaz J, Muñoz-Quezada S, Gómez-Llorente C, Gil A. Probiotic mechanisms of action. Ann Nutr Metab. (2012) 61:160–74. doi: 10.1159/000342079
78. Ashraf R, Shah NP. Immune system stimulation by probiotic microorganisms. Crit Rev Food Sci Nutr. (2014) 54:938–56. doi: 10.1080/10408398.2011.619671
79. El-Shall NA, Awad AM, El-Hack MEA, Naiel MA, Othman SI, Allam AA, et al. The simultaneous administration of a probiotic or prebiotic with live Salmonella vaccine improves growth performance and reduces fecal shedding of the bacterium in Salmonella-challenged broilers. Animals. (2019) 10:70. doi: 10.3390/ani10010070
80. Merrifield DL, Dimitroglou A, Foey A, Davies SJ, Baker RT, Bøgwald J, et al. The current status and future focus of probiotic and prebiotic applications for salmonids. Aquaculture. (2010) 302:1–18. doi: 10.1016/j.aquaculture.2010.02.007
81. Slaninova A, Smutna M, Modra H, Svobodova Z. Reviews: Oxidative stress in fish induced by pesticides. Neuroendocrinol Lett. (2009) 30:2. Available online at: https://pubmed.ncbi.nlm.nih.gov/20027135/
82. Bandyopadhyay U, Das D, Banerjee RK. Reactive oxygen species: oxidative damage and pathogenesis. Curr Sci. (1999) 1999:658–66.
83. Wongsasak U, Chaijamrus S, Kumkhong S, Boonanuntanasarn S. Effects of dietary supplementation with β-glucan and synbiotics on immune gene expression and immune parameters under ammonia stress in Pacific white shrimp. Aquaculture. (2015) 436:179–87. doi: 10.1016/j.aquaculture.2014.10.028
84. Kim Y-s, Ke F, Zhang Q-Y. Effect of β-glucan on activity of antioxidant enzymes and Mx gene expression in virus infected grass carp. Fish Shellfish Immunol. (2009) 27:336–40. doi: 10.1016/j.fsi.2009.06.006
85. Adorian TJ, Jamali H, Farsani HG, Darvishi P, Hasanpour S, Bagheri T, et al. Effects of probiotic bacteria Bacillus on growth performance, digestive enzyme activity, and hematological parameters of Asian sea bass, Lates calcarifer (Bloch). Probiotics Antimicrob Proteins. (2019) 11:248–55. doi: 10.1007/s12602-018-9393-z
86. Dawood MA, Koshio S, Esteban MÁ. Beneficial roles of feed additives as immunostimulants in aquaculture: a review. Rev Aquacult. (2018) 10:950–74. doi: 10.1111/raq.12209
87. Jun Q, Hong Y, Hui W, Didlyn KM, Jie H, Pao X. Physiological responses and HSP70 mRNA expression in GIFT tilapia juveniles, Oreochromis niloticus under short-term crowding. Aquac Res. (2015) 46:335–45. doi: 10.1111/are.12189
88. Shi GC, Dong XH, Chen G, Tan BP, Yang QH, Chi SY, et al. Physiological responses and HSP 70 m RNA expression of GIFT strain of Nile tilapia (Oreochromis niloticus) under cold stress. Aquac Res. (2015) 46:658–68. doi: 10.1111/are.12212
89. Douros JD, Baltzegar DA, Mankiewicz J, Taylor J, Yamaguchi Y, Lerner DT, et al. Control of leptin by metabolic state and its regulatory interactions with pituitary growth hormone and hepatic growth hormone receptors and insulin like growth factors in the tilapia (Oreochromis mossambicus). Gen Comp Endocrinol. (2017) 240:227–37. doi: 10.1016/j.ygcen.2016.07.017
90. Dawood MA, Metwally AE-S, El-Sharawy ME, Atta AM, Elbialy ZI, Abdel-Latif HM, et al. The role of β-glucan in the growth, intestinal morphometry, and immune-related gene and heat shock protein expressions of Nile tilapia (Oreochromis niloticus) under different stocking densities. Aquaculture. (2020) 523:735205. doi: 10.1016/j.aquaculture.2020.735205
91. Ibrahem MD. Evolution of probiotics in aquatic world: potential effects, the current status in Egypt and recent prospectives. J Adv Res. (2015) 6:765–91. doi: 10.1016/j.jare.2013.12.004
92. McGeachy MJ, Chen Y, Tato CM, Laurence A, Joyce-Shaikh B, Blumenschein WM, et al. The interleukin 23 receptor is essential for the terminal differentiation of interleukin 17-producing effector T helper cells in vivo. Nat Immunol. (2009) 10:314–24. doi: 10.1038/ni.1698
Keywords: Bacillus coagulans, growth, tilapia, immunity, prebiotic
Citation: Fath El-Bab AF, Majrashi KA, Sheikh HM, Shafi ME, El-Ratel IT, Neamat-Allah ANF, El-Raghi AA, Elazem AYA, Abd-Elghany MF, Abdelnour SA, Abduh MS, Jaremko M and Naiel MAE (2022) Dietary supplementation of Nile tilapia (Oreochromis niloticus) with β-glucan and/or Bacillus coagulans: Synergistic impacts on performance, immune responses, redox status and expression of some related genes. Front. Vet. Sci. 9:1011715. doi: 10.3389/fvets.2022.1011715
Received: 04 August 2022; Accepted: 29 August 2022;
Published: 23 September 2022.
Edited by:
Ndakalimwe Naftal Gabriel, University of Namibia, NamibiaReviewed by:
Mahmoud Tanekhy, Alexandria University, EgyptSevdan Yilmaz, Çanakkale Onsekiz Mart University, Turkey
Miao Wang, Pearl River Fisheries Research Institute (CAFS), China
Hala Ayoub, Agricultural Research Center, Egypt
Copyright © 2022 Fath El-Bab, Majrashi, Sheikh, Shafi, El-Ratel, Neamat-Allah, El-Raghi, Elazem, Abd-Elghany, Abdelnour, Abduh, Jaremko and Naiel. This is an open-access article distributed under the terms of the Creative Commons Attribution License (CC BY). The use, distribution or reproduction in other forums is permitted, provided the original author(s) and the copyright owner(s) are credited and that the original publication in this journal is cited, in accordance with accepted academic practice. No use, distribution or reproduction is permitted which does not comply with these terms.
*Correspondence: Mohammed A. E. Naiel, bW9oYW1tZWRuYWllbC4xOTg0QGdtYWlsLmNvbQ==
†ORCID: Mohammed A. E. Naiel orcid.org/0000-0002-8172-5366