- 1Department of Veterinary Surgery, Faculty of Veterinary Medicine, Tokyo University of Agriculture and Technology, Fuchu, Japan
- 2Department of Surgery, Anesthesiology, and Radiology, Faculty of Veterinary Medicine, Zagazig University, Zagazig, Egypt
- 3Department of Animal Medicine (Internal Medicine), Faculty of Veterinary Medicine, Suez Canal University, Ismailia, Egypt
Background: Myocardial infarction (MI) is one of the most common cardiac problems causing deaths in humans. Previously validated anesthetic agents used in MI model establishment are currently controversial with severe restrictions because of ethical concerns. The combination between medetomidine, midazolam, and butorphanol (MMB) is commonly used in different animal models. The possibility of MMB combination to establish the MI model in rats did not study yet which is difficult because of severe respiratory depression and delayed recovery post-surgery, resulting in significant deaths. Atipamezole is used to counter the cardiopulmonary suppressive effect of MMB.
Objectives: The aim of the present study is to establish MI model in rats using a novel anesthetic combination between MMB and Atipamezole.
Materials and methods: Twenty-five Sprague Dawley (SD) rats were included. Rats were prepared for induction of the Myocardial infarction (MI) model through thoracotomy. Anesthesia was initially induced with a mixture of MMB (0.3/5.0/5.0 mg/kg/SC), respectively. After endotracheal intubation, rats were maintained with isoflurane 1% which gradually reduced after chest closing. MI was induced through the left anterior descending (LAD) artery ligation technique. Atipamezole was administered after finishing all surgical procedures at a dose rate of 1.0 mg/kg/SC. Cardiac function parameters were evaluated using ECG (before and after atipamezole administration) and transthoracic echocardiography (before and 1 month after MI induction) to confirm the successful model. The induction time, operation time, and recovery time were calculated. The success rate of the MI model was also calculated.
Results: MI was successfully established with the mentioned anesthetic protocol through the LAD ligation technique and confirmed through changes in ECG and echocardiographic parameters after MI. ECG data was improved after atipamezole administration through a significant increase in heart rate (HR), PR Interval, QRS Interval, and QT correction (QTc) and a significant reduction in RR Interval. Atipamezole enables rats to recover voluntary respiratory movement (VRM), wakefulness, movement, and posture within a very short time after administration. Echocardiographic ally, MI rats showed a significant decrease in the left ventricular wall thickness, EF, FS, and increased left ventricular diastolic and systolic internal diameter. In addition, induction time (3.440 ± 1.044), operation time (29.40 ± 3.663), partial recovery time (10.84 ± 3.313), and complete recovery time (12.36 ± 4.847) were relatively short. Moreover, the success rate of the anesthetic protocol was 100%, and all rats were maintained for 1 month after surgery with a survival rate of 88%.
Conclusion: Our protocol produced a more easy anesthetic effect and time-saving procedures with a highly successful rate in MI rats. Subcutaneous injection of Atipamezole efficiently counters the cardiopulmonary side effect of MMB which is necessary for rapid recovery and subsequently enhancing the survival rate during the creation of the MI model in rats.
Introduction
Myocardial infarction (MI) is the main form of ischemic heart disease which occurs due to the blockage of one or more coronary vessels leading to myocardial ischemia and necrosis (1). Acute MI remains the most severe form of coronary artery disease in humans, accounting for almost 4 million deaths each year in Europe and Northern Asia (2). The development of a simple, effective, and time-saving MI animal model is crucial to enrich our knowledge about its pathophysiology and finding better therapeutic options. Various methods have been established in laboratory animal models (3), either by using chemicals that interrupt the coronary circulation (4), coronary artery ligation via open thoracic cage surgery (5), or through non-invasive catheter method (6).
Owing to an increasing concern for laboratory animal welfare and third-party certification of experimental facilities, advances in rodent anesthesia are currently a topic of interest. In MI models, achieving an appropriate anesthetic effect is not only essential from the welfare viewpoint but also constitutes a great challenge that controls the success rate of the model. Generally, achieving an appropriate anesthetic effect in rodent MI models requires sufficient anesthetic depth and fewer cardiorespiratory depression. It has reported that cardiorespiratory depression affecting recovery and survival rate post-MI, subsequently affecting experimental data and the statistical power (7). As a result, an anesthetic protocol that mediates appropriate anesthetic depth while minimizing cardiorespiratory impact is worth investigating, suitable for real-time control of anesthetic depth, and might be used for both short or long durations (8).
In veterinary studies, various anesthetic combinations have been previously proven to be effective to induce MI models in rodents such as ketamine and xylazine (9, 10), a mixture of ketamine, xylazine and acepromazine (11), sodium pentobarbital (12), chloral hydrate 10% (13, 14) and inhalational anesthesia as isoflurane (induction: 5%, maintenance: 2.5%) with buprenorphine was subcutaneously administered for analgesia (15, 16).
For instance, Isoflurane has the advantages of rapid induction of anesthesia, rapid recovery, and minimal influence on hepatic metabolism; however, isoflurane may produce some unfavorable respiratory depression (7), and is not sufficient to provide adequate analgesic effect when highly invasive surgical procedures are needed (17, 18). Although ketamine is widely used in MI model induction (19), ketamine is currently classified as a narcotic drug and many countries have strengthened restrictions on its purchase, storage, and associated record-keeping procedures (20). Moreover, pentobarbital sodium is inappropriate as a general anesthetic due to its minimal analgesic effect and narrow safety margin with the undesirable cardiodepressive effects of reducing heart rate, stroke index, and cardiac index in rodents (19, 20). Consequently, the ethical restrictions and limitations of some medicines which are crucial in MI model induction increase the challenges for successful MI model establishment.
The combination between medetomidine, midazolam, and butorphanol (MMB) anesthetic is recently used in experimental animal studies as a substitute for ketamine or pentobarbital sodium (21). The anesthetic duration of MMB is longer than those of ketamine or pentobarbital sodium and is often associated with depression of respiration and circulation, and reduction of general motor activity and neuronal activity (21). These side effects may limit the usability of MMB in MI model induction. More specifically, medetomidine causes significant cardio-respiratory depression (22). Atipamezole, a synthetic α2-adrenergic antagonist, can antagonize medetomidine-induced respiratory depression and results in rapid recovery from MMB anesthesia (21). Atipamezole is also effective for reducing the maintenance concentration of isoflurane which leads to the amelioration of cardio-respiratory depression induced by isoflurane (17).
To our knowledge combination between MMB and atipamezole has never been studied in MI models. The objective of the study is to provide an easy and successful protocol to induce the MI model using MMB, and atipamezole. Our result will be helpful for an easily induced model using ethically approved medications with a higher success rate for research purposes.
Materials and methods
Animals and ethical approval
The study was conducted on 25 male Sprague Dawley (SD) rats, 12 to 15 weeks of age, and weighing between 350 and 400 gm. All procedures followed the Guide for the Care and Use of Laboratory Animals and were approved by the Institutional Animal Care and Use Committee of the Tokyo University of Agriculture and Technology (Approval No R04-185). The rats had free access to food and water and were housed at 25°C with a 12 h light/dark cycle.
Anesthetic agents
The following anesthetics were used: Medetomidine hydrochloride (Domitor®, Orion Pharma Animal Health, Helsinki, Finland), Midazolam (Dormicum®, Astellas Pharma Inc., Tokyo, Japan), Butorphanol (Vetorphale, Meiji Seika Pharma Co., Ltd.), Isoflurane Inhalation Solution (Isoflurane, Pfizer Inc., New York, USA) and Atipamezole (ATI) (Antisedan, Orion Pharma Animal Health).
Anesthesia protocol
Firstly, a mixture was prepared by mixing medetomidine hydrochloride, midazolam, and butorphanol (MMB) at a dose rate of 0.3, 5.0, and 5.0 mg/kg BW (23, 24). The anesthetic mixture was freshly prepared and diluted with sterile saline as stock solution as described in Table 1. Rats were subcutaneously injected at a dose rate of 0.5 ml of mixture/100 gm BW. Following the loss of front paw reflex, hind paw reflex, tail reflex, corneal reflex, and body righting reflex, rats were rapidly transferred to endotracheal intubation and maintained with isoflurane 1.0 % using a rodent inhalant anesthesia apparatus.
Induction of MI model
Following the above-mentioned anesthetic protocol, the animals were intratracheally intubated using a 16-gauge intravenous catheter before being placed in a supine position on a temperature-controlled pad at a core temperature of 35.5°C. A small incision between the third and fourth intercostal spaces was made to perform a left-sided thoracotomy. A blunt-ended retractor was used to expand the incision away from the lung to avoid its collapse. To access the heart, the pericardial sac was cut open and the site of coronary artery ligation was determined. The site of ligation of the left anterior descending (LAD) coronary artery was determined 8 mm away from the origin, then a 6-0 prolene ligature was passed underneath the LAD and secured with three knots using a tapered atraumatic needle (9). Successful ligation was confirmed by visible blanching and cyanosis of the anterior wall of the left ventricle, as well as swelling of the left atrium (25). Ribs and muscles were closed using 3-0 vicryl absorbable sutures leaving a small gap to aspirate air left in the thoracic cavity. The air was aspirated through a tube (2 mm in diameter) without touching the lungs. Nonabsorbable suture materials, such as silk 3-0, were used to suture the skin. The surgical site was dressed daily to prevent infection and to monitor for suture site dehiscence.
Administration of atipamezole
After LAD ligation and closing of the chest, the maintenance with isoflurane was reduced to 0.5% till suturing the skin and finishing the surgical procedures. At this point, atipamezole was injected subcutaneously at a dose rate of 1.0 mg/kg (Table 1). Then, isoflurane was stopped, and rats were maintained with oxygen insufflation (2 l of oxygen per minute). After observing the initial movement of rats, the endotracheal tube was removed slowly, and rats were changed to a nose cone mask (additional oxygen insufflation) till complete recovery (Figure 1).
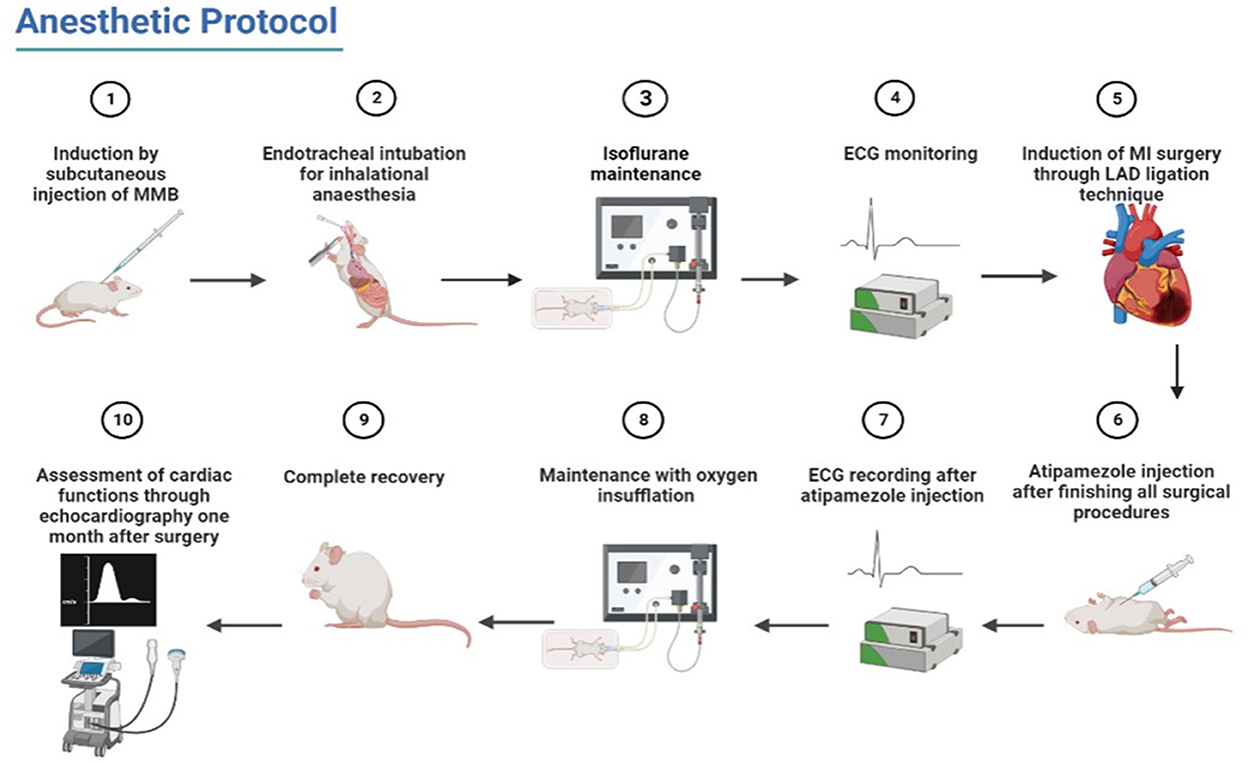
Figure 1. Schematic illustration of the used procedures of anesthetic protocol from induction to recovery and post-MI confirmation.
Post-operative care
Standard postoperative procedures were followed to control pain and infection. After recovery, all animals were treated with gentamicin (Nacalai Tesque Co., Ltd., Tokyo, Japan) which was injected intraperitoneally (2.4 × 104/kg/day) for 3 days (14) while carprofen (Rimadyl®, Zoetis Japan K.K., Tokyo, Japan) was used with a pre-surgical dose of 5.0 mg/kg/SC, followed by two post-surgery injections (26).
Measurement of time intervals during operation
Induction time was defined as the duration from injection of anesthetic mixture to the start of the loss of a body-righting reflex. Operation time was defined as the duration from the start of MI surgery till the end of all surgical procedures. Partial recovery time was defined as the duration from the end of the operation period (atipamezole injection) till trials of animals to remove the endotracheal tube (initial movement). Meanwhile, complete recovery time was defined as the duration from the removal of the endotracheal tube and the change to a nose cone mask to restore all vital reflexes. All these durations were recorded and expressed as mean ± SD.
ECG monitoring
The ECG signals were recorded with needle electrodes connected with PowerLab hardware (ML880 PowerLab 16/30, AD Instruments) and LabChart Pro software (LabChart v8, AD Instruments) using a previously published protocol (27). The setting of PowerLab for ECG measurements followed the instructions provided by the producer. The ECG recording started 10 min after the animal was anesthetized with (MMB; i.e., before atipamezole injection) and then was conducted for 10 min and repeated after atipamezole administration for another 10 min. For each record, the most stable continuous segment was chosen for ECG analysis.
The ECG was recorded in an anesthetized state before and after atipamezole administration ECG parameters included RR Interval (s), heart rate (HR), atrial complex (PR interval, P wave duration, and P wave amplitude), ventricular complexes (QRS complex, QT and QTc interval duration) were recorded and analyzed.
Confirmation of MI model
Cardiac functions were evaluated directly before and 1 month after MI. The echocardiographic machine (Hitachi-Aloka Medical Ltd., Tokyo, Japan, ProSound F75 ultrasonographic system) with a 12-MHz transducer and simultaneous ECG was used. The echocardiography was performed in accordance with the guidelines of the American Society of Echocardiography (ASE) (28, 29). All animals were anesthetized with MMB subcutaneously administered for easy and feasible examination and at the level of the papillary muscles, a two-dimensional right parasternal short-axis view of the LV was achieved using M-mode. LV was measured manually by the same observer using the ASE's leading-edge method (30), which has been validated for the rat MI model (31).
The LV internal diameter during diastole (LVIDd), LV internal diameter during systole (LVIDs), LV posterior wall diameter during diastole (LVPWd) systole (LVPWs) and (IVSd) and (IVSs) interventricular septal thickness in end-diastole and systole, respectively. Ejection fraction (EF%), and fractional shortening (FS%) were obtained from that view. From each rat, each echocardiographic parameter was measured five times and the data were averaged (32).
Statistical analysis
Data analysis was performed using GraphPad Prism8 version 7.01 (GraphPad Software, Inc, San Diego, California). The normality of the data was tested by the Shapiro–Wilk test. To compare the cardiac function parameters before and after MI model induction, the student T-test was used and a P < 0.05 was considered statistically significant.
Results
Operation intervals and success of the MI model
Initially, rats were subcutaneously administered MMB at a dose rate of 0.5 ml/100 gm BW. Anesthetic induction was generally quick and easy, and most rats were orotracheally intubated in the range of 2–5 min as Mean ± SD (3.440 ± 1.044) after MMB administration. Muscle relaxation and analgesia were sufficient to begin thoracotomy immediately after anesthetic administration and the recovery time was divided into two stages: partial as Mean ± SD (10.84 ± 3.313 min) and complete as Mean ± SD (12.36 ± 4.847 min). The total operation time was 29.40 ± 3.663 min as Mean ± SD (Table 2). The success rate of the anesthetic protocol was 100%. In addition, all animals used in the current study were maintained for 1 month after surgery with a survival rate of 88% (22/25), and three rats died within 24 h after MI induction.
Assessment of ECG
The ECG analysis before and after atipamezole injection in the operated rats is illustrated in Figures 2, 3. There were no significant differences in P duration, P amplitude, and QT interval. However, the RR interval was significantly decreased after atipamezole administration (P = 0.0001). In contrast, other ECG parameters such as HR, PR Interval, QRS Interval, and QTc were significantly increased (P = 0.0001, 0.002, 0.043, and 0.018, respectively) when compared with their values before atipamezole injection.
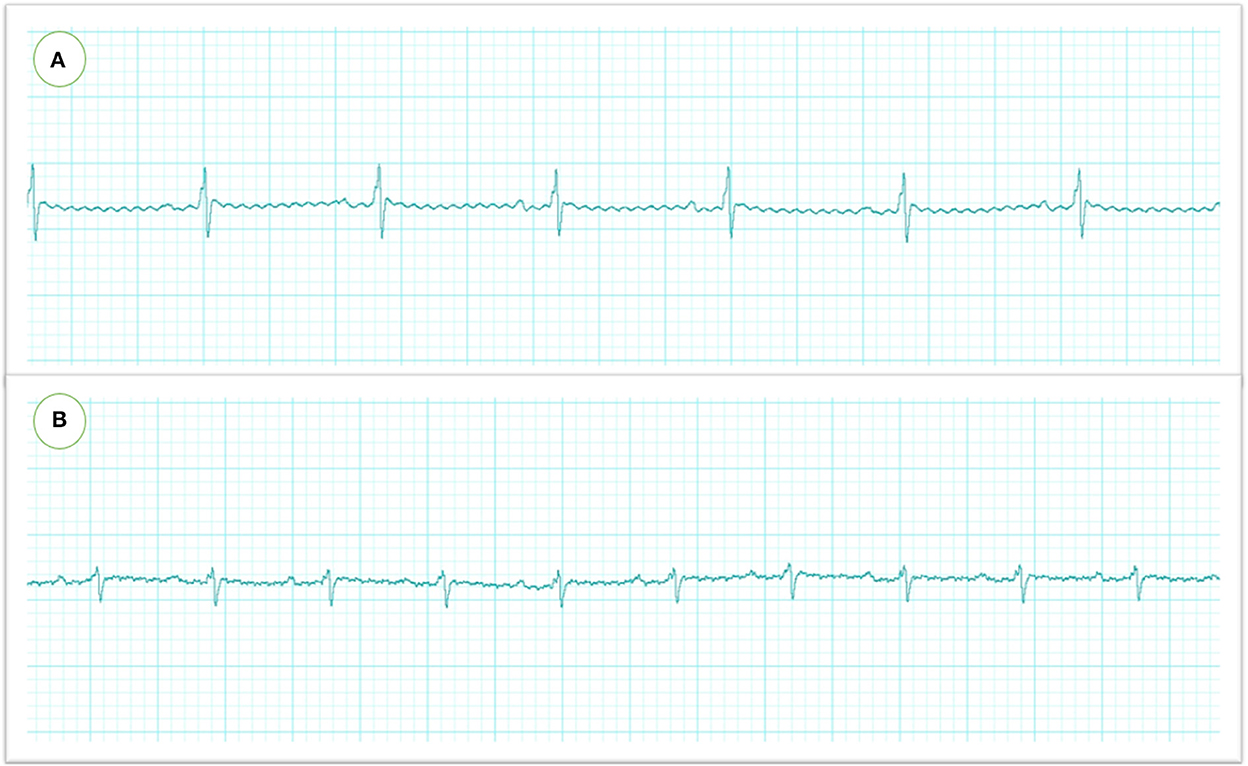
Figure 2. Electrocardiographic recordings in rats were measured by needle electrodes with Lab chart. The heart rate and RR intervals were significantly reduced after MMB injection (before atipamezole administration) (A) which were restored to the normal level after atipamezole administration (B).
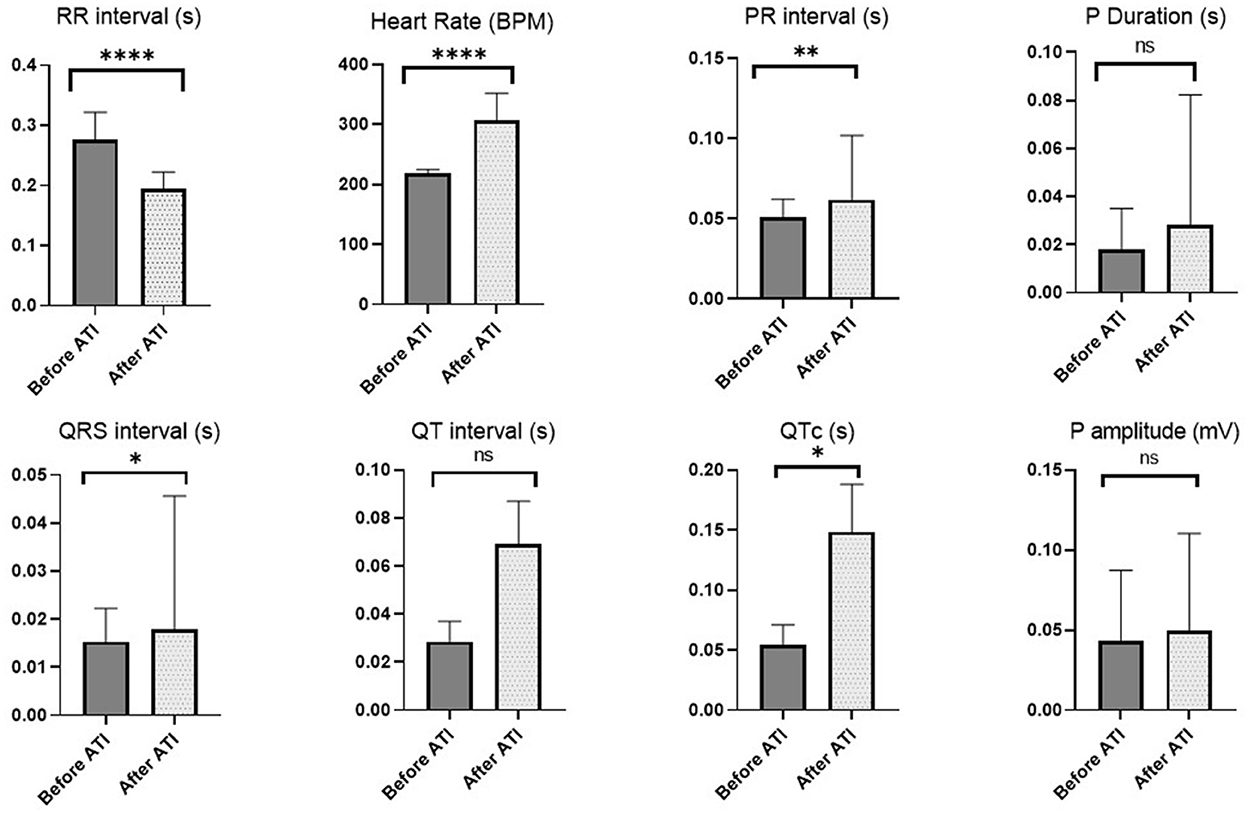
Figure 3. Changes in ECG parameters before atipamezole (i.e., directly after MMB) and after atipamezole administration. Asterisk used to indicate the significance, Ns P > 0.05 , *P ≤ 0.05, **P ≤ 0.01, ****P ≤ 0.0001.
Confirmation of MI model
The myocardial infarction model was successfully created with the aforementioned anesthetic protocol via LAD ligation approach, as evidenced by apparent blanching and cyanosis of the anterior wall of the left ventricle and swelling of the left atrium immediately following artery ligation. No clinical abnormalities were observed during the observation period. Three rats died within 24 h post-MI induction and showed rapid respiration and off food (Figure 4).
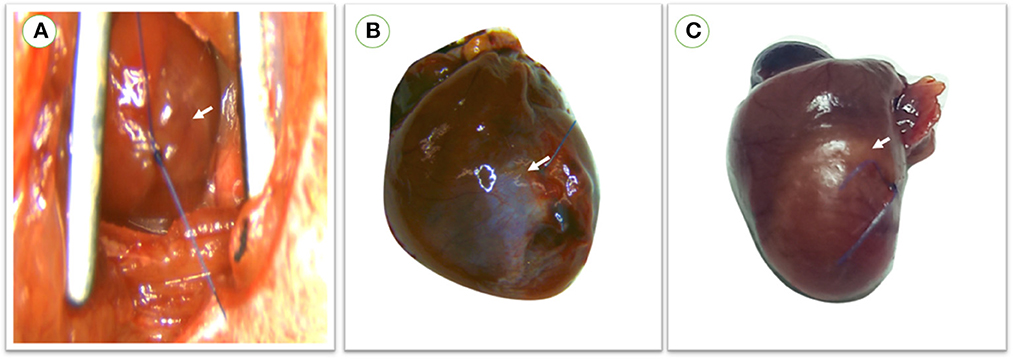
Figure 4. (A) During operation, myocardial infarction was confirmed in rats directly after LAD ligation through apparent blanching and cyanosis of the anterior wall of the left ventricle and swelling of the left atrium. (B) Color change of the left ventricular wall in survived rats which were maintained for 1 month with a small infarction size. (C) Large infarction size was observed in rats within 24 h following MI induction. red arrow; the site of infarction.
The echocardiographic parameters measured before and 1 month after MI induction are summarized in Figure 5. Changes in echocardiographic parameters can be seen after MI, as a significant decrease was observed in IVSd, IVSs, LVPWs, EF% and FS% (P = 0.047, 0.007, 0.007, 0.007, and 0.007, respectively). In addition, a significant increase was recorded in LVIDd and LVIDs after LAD ligation (P = 0.039; 0.007, respectively) (Figure 6).
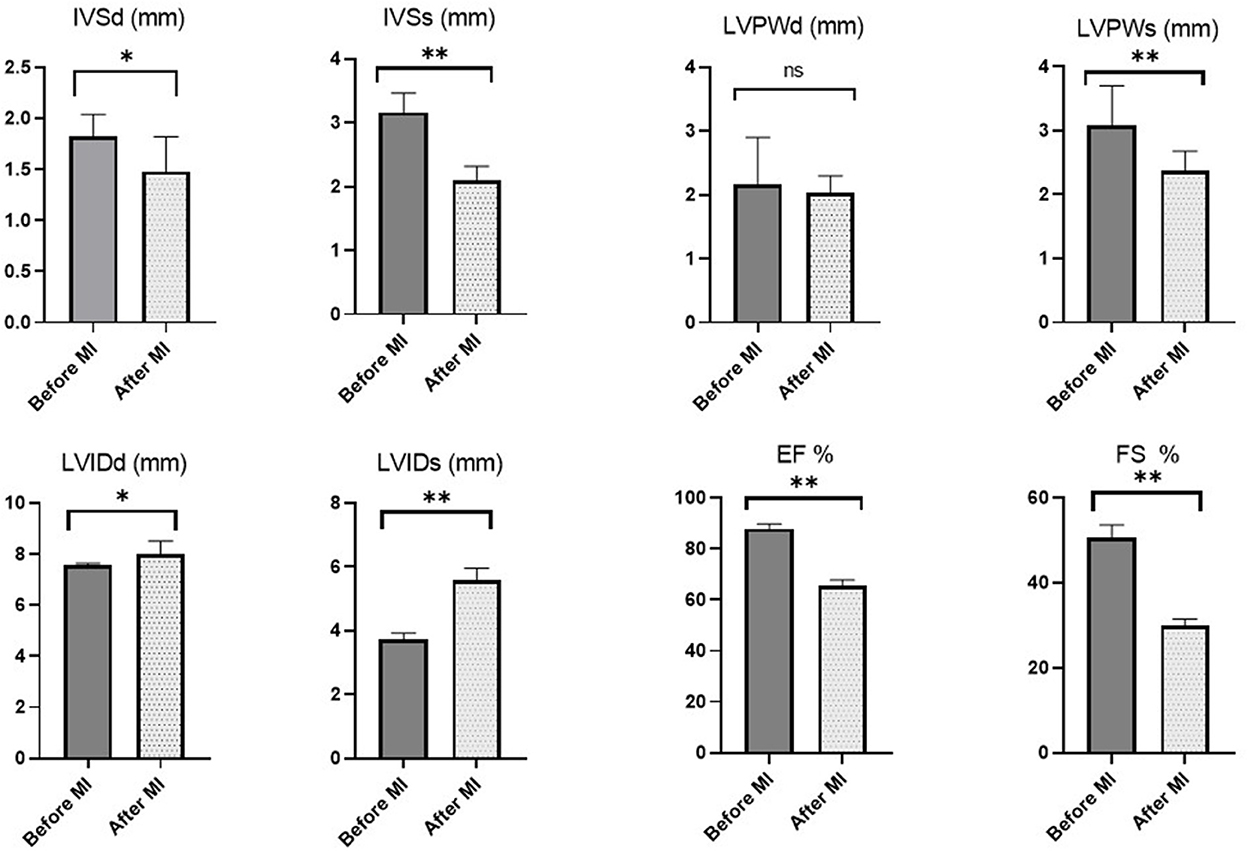
Figure 5. Echocardiographic measurements in rats before MI induction and 1 month later. Asterisk used to indicate the significance, Ns P > 0.05 , *p < 0.05, **p < 0.01.
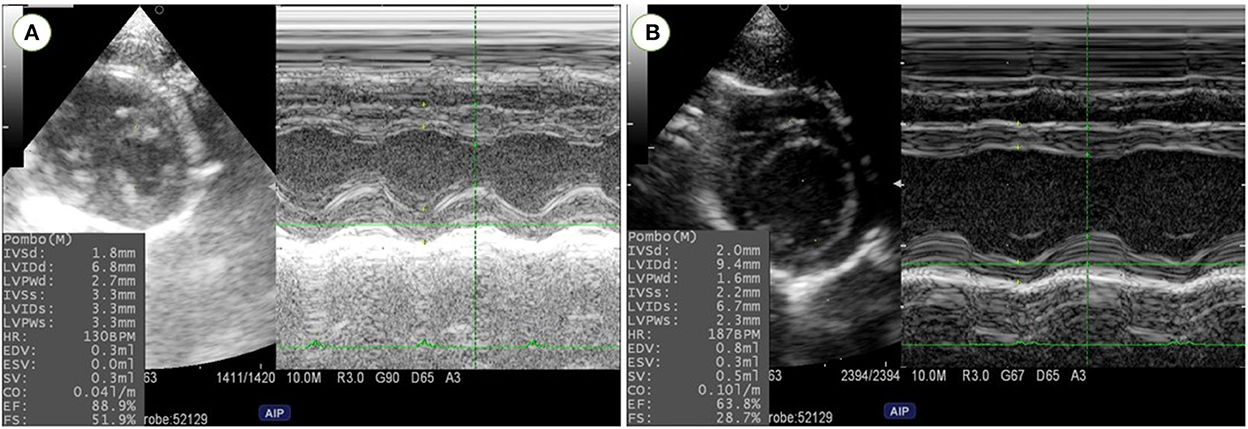
Figure 6. Left ventricular dimensions and function were evaluated using M-Mode echocardiography at right parasternal short axis in rats before MI induction (A) and one-month post-MI (B). Reduction in left ventricular wall thickness and cardiac function and increase in left ventricular diameters were confirmed in MI model rats.
Discussion
The rat model is the most commonly used to study the pathophysiology of cardiovascular diseases including ischemic heart diseases as well as other models (33, 34). Limitations regarding the anesthetic protocols because of animal welfare, ethical concerns, and public health circumstances limit the usability of well-known medications in MI models, making the establishment of MI models more difficult. In addition, scientific publications still cannot be relied upon to present a detailed description of analgesia and anesthesia protocols. Most recently, an assessment of anesthetic and analgesic regimens in publications involving non-human primates revealed the absence of critical details reporting (35). In the present study, we have developed a successful MI induction in all rats with a survival rate of 88% using the novel protocol of anesthesia (combination of MMB + Isoflurane and countered with atipamezole). The success rate of the anesthetic protocol was 100% which provides quick and easy induction of general anesthesia with facilitating orotracheally intubated, muscle relaxation and analgesia were sufficient to perform the surgery recording a very short recovery time with minimal side effects in comparison to other previous studies. Our protocol may be introduced as an alternative to ketamine, xylazine, and pentobarbital with sufficient anesthetic and time-saving effects in rats.
Regarding the anesthetic combination, the used MMB mixture has been created based on previous reports in rats and mice (23, 24, 36–39).
Midazolam is a benzodiazepine that is water soluble. In rodents, pigs, and primates, benzodiazepines can cause significant sedation; however, they are not analgesic and do not create a true general anesthetic state (40). Midazolam is used in conjunction with other drugs to induce anesthesia (41). Medetomidine is a more potent imidazole derivative than xylazine, with higher alpha2-adrenoceptor selectivity (41). Butorphanol, a synthetic opioid agonist-antagonist, is used in veterinary medicine as an analgesic agent (42). The combination of medetomidine, midazolam, and butorphanol has been reported as a reliable and safe anesthetic agent in the dog (43), sea lions (44), and red fox (45). In our study, we administered MMB in rats by subcutaneous injection as it is considered a more effective, and induced rapid, complete, and stable anesthetic effect than intraperitoneal injection (23). The intraperitoneal delivery route is the most commonly used for MMB administration in rats (37), but according to Sorrenti et al. (39), the induction time for a single dose of MMB combination administered subcutaneously in Sprague-Dawley rats was approximately 10 min; however, with intraperitoneal injection, this duration increased to 25 min and required one or two additional doses (39).
Isoflurane is known to have a relatively strong respiratory depression in various species (17, 46). Therefore, the main purpose of the current novel anesthetic protocol was to attenuate cardio-respiratory depression by reduction of isoflurane concentration and minimizing MMB side effects, producing more safe and time-saving protocol for induction of MI in rats.
Atipamezole is a highly selective 2-adrenergic antagonist that is known to counteract the anesthetic effect of MMB. We administrated atipamezole with the prescribed dosage as the recovery from the anesthetic effect and hypothermia was dosage-dependent, and even after a low dose of atipamezole, the same as medetomidine, the sedation continued, even after all reflexes had been restored. As a result, giving atipamezole at the same dose as medetomidine is insufficient to promote recovery from MMB anesthesia. In addition, atipamezole's ability to counteract MMB-induced anesthesia is partially attributable to the fact that MMB primarily exerts its anesthetic effect via the 2-adrenoceptor (47). Furthermore, it has been reported that atipamezole can also counteract the anesthetic effect of the combination of medetomidine, butorphanol, alfaxalone, and neurosteroid anesthetic, as well as can counter the anesthetic effect of MMB (48).
Generally, medetomidine is helpful in central analgesia while butorphanol is necessary for visceral analgesia (49, 50). Administration of atipamezole during early surgical procedures will abolish the analgesic effect of medetomidine and exposes the rat to pain. To avoid such situation in the current study, atipamezole was administrated after finishing all surgical procedures. In other words, during the operation, anesthesia was achieved through the effect of the used combination with isoflurane, and postoperative analgesia was achieved successfully through pain killer.
Rats were kept under oxygen insufflation (2 l of oxygen per minute) when isoflurane maintenance was stopped to prevent problems like hypoxia. This is in agreement with Mechelinck et al., who claimed that rats under ketamine-xylazine anesthesia are susceptible to hypoxia. This could result in an increase in delayed mortality from hypoxia-related lung failure. So they recommend using additional oxygen insufflation with the prescribed dose (51). Moreover, Ballard and Spadafora (52) stated that respiratory depression caused by ketamine-xylazine narcosis seems to be the key factor in lung damage. In principle, rats' lungs can be damaged by hypoxemia. This damage begins 8 h after the hypoxic incident with pulmonary edema, most likely due to sympathetic activation, increased vascular permeability, and hypoxic pulmonary vasoconstriction, and is followed by inflammation, pulmonary fibrosis, and vascular hypertrophy (52).
In our study, we reported that the induction time of our anesthetic protocol was < 5 min, these results were resemble that recorded with the ketamine/xylazine protocol (20), but with a short recovery time in total when compared to other protocols including ketamine/xylazine (20), MMB alone (23) and Pentobarbital (35). In other studies, The mean recovery time without atipamezole injection was 44.5 min and 50.0 min in males and females respectively (53).
All the operated rats in the present study were maintained for 1 month with a survival rate of 88%, and three rats died within 24 h post-surgery due to surgical errors, Lindsey et al. (54) stated that perioperative death within 24 h post-MI is usually due to surgical errors (or very large infarct sizes), and in the permanent occlusion MI model in mice, postoperative death may be due to rupture, acute heart failure, or arrhythmias (54).
The normal heart rate of rats has been reported 330–480 beats per minute (55). Kirihara et al. (56) stated that MMB had decreased heart rate and blood oxygen saturation in rats (56). The recorded heart rate in our study was in the range of 187 to 226 beats per minute, confirming the bradycardia expected with the use of an α2-agonist (57). After the administration of atipamezole, heart rate began to increase within 2 min and was fully restored after 4 min to reach 270–352 beats per minute. According to ECG data from our study, the main advantage of administering atipamezole is that it allows rats to recover voluntary respiratory movement, wakefulness, movement, and posture within a relatively short time after injection, this allows for faster extubating of operated rats, minimizing the post-anesthesia recovery period and, as a result, lowering the risk of side effects and residual effects of the anesthetics (58).
In operated rats, we found typical ischemic changes on transthoracic echocardiography, particularly significant increase in left ventricular diameters and a significant decrease in wall dimensions, EF%, and FS% following post-infarction LV remodeling in adult rats. Our findings are comparable to those of earlier studies on rats indicating a successful procedure (59, 60). Changes in cardiac function parameters with no clinical symptoms in the remaining rats suggest subclinical heart failure.
At 1-month post-infarction, morphological alterations such as an increase in LVESD and LVEDD, as well as functional changes such as a decrease in FS and EF, were clearly identified. Our data are similar to those of other reports (61–64). LAD-ligation significantly reduced regional contractility not just in the anterior, anteroseptal, and septal segments. Even adjacent regions like the lateral and posterior segments were affected. This can be explained by the distinctions between the geometries of the coronary arteries in rats and humans as there is no true circumflex artery and the LAD predominates in rats (65). As a result, the posterior and lateral regions of the left ventricle have a significant perfusion deficiency as a consequence of LAD ligation (64, 66). Differences in age, weight and echocardiographic transducers or procedures appear to provide slightly variable results in different laboratories. Based on our findings, we may conclude that we were successful in creating a novel anesthetic protocol for producing a MI model in adult rats and confirmed the efficacy of LAD ligation surgery under the used protocol.
Various animal models such as rats, rabbits, pigs and non-human primates, where sparse collateral coronary circulation is excisting, have been introduced to study MI; however, considerable mortalities due to delayed recovery is still controversial (67–69). Until now, our protocol did not utilized in any animal model. Therefore, the current protocol worth studying in other MI or ischemic dysfunction models.
Limitations
In our study, we did not compare our protocol to others such as ketamine and pentobarbital sodium. These drugs are no longer available in Japan and their import or use is not ethical due to being classified as narcotic drugs (20). In the current study, the effect of the used protocol on pulmonary function and blood pressure was not investigated. However, other studies have examined the detailed hemodynamic and respiratory impact of MMB alone or after antaonizing with atipamezole in rats and rabitts (23, 70) with no surgical approaches.
Conclusion
To our knowledge, this is the first study to use anesthetic combination of MMB and a light concentration of isoflurane with atipamezole in MI rats. Subcutaneous injection of atipamezole efficiently counters the cardiopulmonary side effect of MMB which is necessary for rapid recovery and subsequently enhancing the survival rate during the establishment of the MI model. Our approach produced a more easily anesthetic effect and time-saving procedures with a highly successful rate in MI rats which may be effective in the research field of cardiothoracic disorders using rat models. Our protocol worth studying in other animal models as well.
Data availability statement
The raw data supporting the conclusions of this article will be made available by the authors, without undue reservation.
Ethics statement
The animal study was reviewed and approved by the experimental procedures were approved by the local Ethical Committee of the Tokyo University of Agriculture and Technology, Japan (Approval No R04-185).
Author contributions
Experiment design: AF, AM, and RT. Induction of model: AF. Echocardiography and electrocardiography, data collection, and statistical analysis: AF and AM. Investigation: AF, AM, and KS. Writing and drafting: AF, TY, LH, and AM. Critical editing: AM. Supervision: RT. All authors reviewed and edited the final version.
Acknowledgments
The research was supported by a full scholarship provided by the Egypt-Japan Education Partnership (EJEP) from the Ministry of Higher Education, Egypt.
Conflict of interest
The authors declare that the research was conducted in the absence of any commercial or financial relationships that could be construed as a potential conflict of interest.
Publisher's note
All claims expressed in this article are solely those of the authors and do not necessarily represent those of their affiliated organizations, or those of the publisher, the editors and the reviewers. Any product that may be evaluated in this article, or claim that may be made by its manufacturer, is not guaranteed or endorsed by the publisher.
References
1. Eltzschig HK, Eckle T. Ischemia and reperfusion—from mechanism to translation. Nat Med. (2011) 17:1391–401. doi: 10.1038/nm.2507
2. Nichols M, Townsend N, Scarborough P, Rayner M. Cardiovascular disease in Europe 2014: epidemiological update. Eur Heart J. (2014) 35:2950–9. doi: 10.1093/eurheartj/ehu299
3. Katsanos K, Mitsos S, Koletsis E, Bravou V, Karnabatidis D, Kolonitsiou F, et al. Transauricular embolization of the rabbit coronary artery for experimental myocardial infarction: comparison of a minimally invasive closed-chest model with open-chest surgery. J Cardiothorac Surg. (2012) 7:1–9. doi: 10.1186/1749-8090-7-16
4. Zhang J, Knapton A, Lipshultz SE, Weaver JL, Herman EH. Isoproterenol-induced cardiotoxicity in sprague-dawley rats: correlation of reversible and irreversible myocardial injury with release of cardiac troponin T and roles of iNOS in myocardial injury. Toxicol Pathol. (2008) 36:277–8. doi: 10.1177/0192623307313010
5. Xu Z, Alloush J, Beck E, Weisleder N. A murine model of myocardial ischemia-reperfusion injury through ligation of the left anterior descending artery. JoVE. (2014) 3:e51329. doi: 10.3791/51329
6. Isorni M-A, Casanova A, Piquet J, Bellamy V, Pignon C, Puymirat E, Menasche P. Comparative analysis of methods to induce myocardial infarction in a closed-chest rabbit model. Biomed Res Int. (2015) 2015:3051. doi: 10.1155/2015/893051
7. Tsukamoto A, Uchida K, Maesato S, Sato R, Kanai E, Inomata T. Combining isoflurane anesthesia with midazolam and butorphanol in rats. Exp Anim. (2016) 65:223–30. doi: 10.1538/expanim.15-0113
8. Gargiulo S, Greco A, Gramanzini M, Esposito S, Affuso A, Brunetti A, et al. Mice anesthesia, analgesia, and care, Part I: anesthetic considerations in preclinical research. ILAR J. (2012) 53:E55–69. doi: 10.1093/ilar.53.1.55
9. Srikanth G, Prakash P, Tripathy N, Dikshit M, Nityanand S. Establishment of a rat model of myocardial infarction with a high survival rate: a suitable model for evaluation of efficacy of stem cell therapy. J Stem Cells Regen Med. (2009) 5:30. doi: 10.46582/jsrm.0501006
10. Azar AD, Tavakoli F, Moladoust H, Zare A, Sadeghpour A. Echocardiographic evaluation of cardiac function in ischemic rats: value of m-mode echocardiography. Res Cardiovasc Med. (2014) 3:22941. doi: 10.5812/cardiovascmed.22941
11. Samsamshariat SA, Samsamshariat ZA, Movahed MR. A novel method for safe and accurate left anterior descending coronary artery ligation for research in rats. Cardiovasc Revascul Med. (2005) 6:121–3. doi: 10.1016/j.carrev.2005.07.001
12. Dai Y, Chen Y, Wei G, Zha L, Li X. Ivabradine protects rats against myocardial infarction through reinforcing autophagy via inhibiting PI3K/AKT/mTOR/p70S6K pathway. Bioengineered. (2021) 12:1826–37. doi: 10.1080/21655979.2021.1925008
13. Jiang J, Gu X, Wang H, Ding S. Resveratrol improves cardiac function and left ventricular fibrosis after myocardial infarction in rats by inhibiting NLRP3 inflammasome activity and the TGF-β1/SMAD2 signaling pathway. PeerJ. (2021) 9:e11501. doi: 10.7717/peerj.11501
14. Fu Y, Wang S, Cui Q. Mechanism of Atorvastatin in Improving Cardiac Function in a Rat Model of Myocardial Infarction. Indian J Pharm Sci. (2020) 42:38–44. doi: 10.36468/pharmaceutical-sciences.spl.121
15. Sugiyama A, Ito R, Okada M, Yamawaki H. Long-term administration of recombinant canstatin prevents adverse cardiac remodeling after myocardial infarction. Sci Rep. (2020) 10:1–11. doi: 10.1038/s41598-020-69736-y
16. Gao G, Chen W, Yan M, Liu J, Luo H, Wang C, et al. Rapamycin regulates the balance between cardiomyocyte apoptosis and autophagy in chronic heart failure by inhibiting mTOR signaling. Int J Mol Med. (2020) 45:195–209. doi: 10.3892/ijmm.2019.4407
17. Tsukamoto A, Serizawa K, Sato R, Yamazaki J, Inomata T. Vital signs monitoring during injectable and inhalant anesthesia in mice. Exp Anim. (2015) 64:57–64. doi: 10.1538/expanim.14-0050
18. Eilers H. Anesthetic activation of nociceptors: adding insult to injury? Mol Interv. (2008) 8:226. doi: 10.1124/mi.8.5.6
19. Shekarforoush S, Fatahi Z, Safari F. The effects of pentobarbital, ketamine–pentobarbital and ketamine–xylazine anesthesia in a rat myocardial ischemic reperfusion injury model. Lab Anim. (2016) 50:179–84. doi: 10.1177/0023677215597136
20. Kawai S, Takagi Y, Kaneko S, Kurosawa T. Effect of three types of mixed anesthetic agents alternate to ketamine in mice. Exp Anim. (2011) 60:481–7. doi: 10.1538/expanim.60.481
21. Kamio K, Morita J, Nakanishi Y, Sasaki M, Wakamatsu M. Corneal lesions related to an anesthetic mixture of medetomidine, midazolam, and butorphanol treatment in rats. J Toxicol Sci. (2021) 46:561–8. doi: 10.2131/jts.46.561
22. Hedenqvist P, Roughan JV, Flecknell PA. Sufentanil and medetomidine anaesthesia in the rat and its reversal with atipamezole and butorphanol. Lab Anim. (2000) 34:244–51. doi: 10.1258/002367700780384762
23. Shibuta H, Yamana R, Kashimoto J, Kamio K, Suda A. Comparison of the anesthetic effect by the injection route of mixed anesthesia (medetomidine, midazolam and butorphanol) and the effect of this anesthetic agent on the respiratory function. J Vet Med Sci. (2020) 82:35–42. doi: 10.1292/jvms.19-0438
24. Fujiki M, Kuga K, Ozaki H, Kawasaki Y, Fudaba H. Blockade of motor cortical long-term potentiation induction by glutamatergic dysfunction causes abnormal neurobehavior in an experimental subarachnoid hemorrhage model. Front Neural Circuits. (2021) 15:670189. doi: 10.3389/fncir.2021.670189
25. Iborra-Egea O, Santiago-Vacas E, Yurista SR, Lupón J, Packer M, Heymans S, et al. Unraveling the molecular mechanism of action of empagliflozin in heart failure with reduced ejection fraction with or without diabetes. JACC Basic Transl Sci. (2019) 4:831–40. doi: 10.1016/j.jacbts.2019.07.010
26. Onohara D, Corporan DM, Kono T, Kumar S, Guyton RA, Padala M. Ventricular reshaping with a beating heart implant improves pump function in experimental heart failure. J Thorac Cardiovasc Surg. (2020) 163:e343–5. doi: 10.1016/j.jtcvs.2020.08.097
27. Doggett TM, Tur JJ, Alves NG, Yuan SY, Tipparaju SM, Breslin JW. Assessment of cardiovascular function and microvascular permeability in a conscious rat model of alcohol intoxication combined with hemorrhagic shock and resuscitation. Traum Isch Injury. (2018) 54:61–81. doi: 10.1007/978-1-4939-7526-6_6
28. Rychik J, Ayres N, Cuneo B, Gotteiner N, Hornberger L, Spevak PJ, et al. American Society of Echocardiography guidelines and standards for performance of the fetal echocardiogram. J Am Soc Echocardiography. (2004) 17:803–10. doi: 10.1016/j.echo.2004.04.011
29. Zacchigna S, Paldino A, Falcão-Pires I, Daskalopoulos EP, Dal Ferro M, Vodret S, et al. Towards standardization of echocardiography for the evaluation of left ventricular function in adult rodents: a position paper of the ESC working group on myocardial function. Cardiovasc Res. (2021) 117:43–59. doi: 10.1093/cvr/cvaa110
30. Lang RM, Bierig M, Devereux RB, Flachskampf FA, Foster E, Pellikka PA, et al. Recommendations for chamber quantification: a report from the American Society of echocardiography's guidelines and standards committee and the chamber quantification writing group, developed in conjunction with the European association of echocardiography, a branch of the European society of cardiology. J Am Soc Echocardiography. (2005) 18:1440–63. doi: 10.1016/j.echo.2005.10.005
31. Solomon SD, Greaves SC, Rayan M, Finn P, Pfeffer MA, Pfeffer JM. Temporal dissociation of left ventricular function and remodeling following experimental myocardial infarction in rats. J Card Fail. (1999) 5:213–23. doi: 10.1016/S1071-9164(99)90006-4
32. Yairo A, Mandour AS, Matsuura K, Yoshida T, Ma D, Kitpipatkun P, et al. Effect of loading changes on the intraventricular pressure measured by color M-mode echocardiography in rats. Diagnostics. (2021) 11:1403. doi: 10.3390/diagnostics11081403
33. Liu Chung Ming C, Sesperez K, Ben-Sefer E, Arpon D, McGrath K, McClements L, et al. Considerations to model heart disease in women with preeclampsia and cardiovascular disease. Cells. (2021) 10:899. doi: 10.3390/cells10040899
34. Ma D, Mandour AS, Elfadadny A, Hendawy H, Yoshida T, El-Husseiny HM, Nishifuji K, Takahashi K, Zhou Z, Zhao Y. Changes in cardiac function during the development of uremic cardiomyopathy and the effect of salvianolic acid B administration in a rat model. Front Vet Sci. (2022) 9:5759. doi: 10.3389/fvets.2022.905759
35. Herrmann K, Flecknell P. Retrospective review of anesthetic and analgesic regimens used in animal research proposals. ALTEX. (2019) 36:65–80. doi: 10.14573/altex.1804011
36. Hasegawa T, Takagi R, Tanaka Y, Ohta T, Shinohara M, Kageyama Y, et al. Differences in the Effects of pentobarbital anesthetic and combination of medetomidine hydrochloride, midazolam, and butorphanol tartrate anesthetic on electroretinogram in spontaneously diabetic torii fatty rats. Biomed Hub. (2022) 7:106–14. doi: 10.1159/000526189
37. Ochiai Y, Baba A, Hiramatsu M, Toyota N, Watanabe T, Yamashita K, et al. Blood biochemistry and hematological changes in rats after administration of a mixture of three anesthetic agents. J Vet Med Sci. (2018) 80:387–94. doi: 10.1292/jvms.17-0497
38. Doi M, Oka Y, Taniguchi M, Sato M. Transient expansion of the expression region of Hsd11b1, encoding 11β-hydroxysteroid dehydrogenase type 1, in the developing mouse neocortex. J Neurochem. (2021) 159:778–88. doi: 10.1111/jnc.15505
39. Sorrenti V, Cecchetto C, Maschietto M, Fortinguerra S, Buriani A, Vassanelli S. Understanding the effects of anesthesia on cortical electrophysiological recordings: a scoping review. Int J Mol Sci. (2021) 22:1286. doi: 10.3390/ijms22031286
40. Wolfe JW, Ehrenfeld JM. “Pharmacology of Intravenous Anesthetic Agents.,” Anesthesia Student Survival Guide. Springer (2022). p. 57–75 doi: 10.1007/978-3-030-98675-9_4
41. Meyer RE, Fish R. Pharmacology of injectable anesthetics, sedatives, and tranquilizers. (2008) doi: 10.1016/B978-012373898-1.50006-1
42. Heavner J, Cooper DM. Pharmacology of Analgesics, p 97–123. Anesthesia and analgesia in laboratory animals, 2nd ed. San Diego (CA): Academic Press[Google Scholar] (2008) doi: 10.1016/B978-012373898-1.50008-5
43. Itamoto K, Hikasa Y, Sakonjyu I, Itoh H, Kakuta T, Takase K. Anaesthetic and cardiopulmonary effects of balanced anaesthesia with medetomidine–midazolam and butorphanol in dogs. J Vet Med Series A. (2000) 47:411–20. doi: 10.1046/j.1439-0442.2000.00302.x
44. Spelman LH. Reversible anesthesia of captive California sea lions (Zalophus californianus) with medetomidine, midazolam, butorphanol, and isoflurane. J Zoo Wildlife Med. (2004) 35:65–9. doi: 10.1638/01-102
45. Bertelsen MF, Villadsen L, A. comparison of the efficacy and cardiorespiratory effects of four medetomidine-based anaesthetic protocols in the red fox (Vulpes vulpes). Vet Anaesth Analg. (2009) 36:328–33. doi: 10.1111/j.1467-2995.2009.00464.x
46. Mutoh T, Kojima K, Takao K, Nishimura R, Sasaki N. Comparison of Sevoflurane with Isoflurane for Rapid Mask Induction in Midazolam and Butorphanol-sedated Dogs. J Vet Med Series A. (2001) 48:223–30. doi: 10.1046/j.1439-0442.2001.00350.x
47. Salonen M, Reid K, Maze M. Synergistic interaction between alpha 2-adrenergic agonists and benzodiazepines in rats. Anesthesiology. (1992) 76:1004–11. doi: 10.1097/00000542-199206000-00022
48. Higuchi S, Yamada R, Hashimoto A, Miyoshi K, Yamashita K, Ohsugi T. Evaluation of a combination of alfaxalone with medetomidine and butorphanol for inducing surgical anesthesia in laboratory mice. Japanese J Vet Res. (2016) 64:131–9. doi: 10.14943/jjvr.64.2.131
49. Alves HC, Valentim AM, Olsson IAS, Antunes LM. Intraperitoneal anaesthesia with propofol, medetomidine, and fentanyl in mice. Lab Anim. (2009) 43:27–33. doi: 10.1258/la.2008.007036
50. Wenger S. Anesthesia and analgesia in rabbits and rodents. J Exot Pet Med. (2012) 21:7–16. doi: 10.1053/j.jepm.2011.11.010
51. Mechelinck M, Kupp C, Krüger JC, Habigt MA, Helmedag MJ, Tolba RH, et al. Oxygen inhalation improves postoperative survival in ketamine-xylazine anaesthetised rats: an observational study. PLoS ONE. (2019) 14:e0226430. doi: 10.1371/journal.pone.0226430
52. Ballard ST, Spadafora D. Fluid secretion by submucosal glands of the tracheobronchial airways. Respir Physiol Neurobiol. (2007) 159:271–7. doi: 10.1016/j.resp.2007.06.017
53. Nakamura T, Ichii O, Irie T, Hosotani M, Dantsuka A, Nakamura S, et al. Usefulness of an anesthetic mixture of medetomidine, midazolam, and butorphanol in cotton rats (Sigmodon hispidus). Japanese J Vet Res. (2016) 64:273–6. doi: 10.14943/jjvr.64.4.273
54. Lindsey ML, Bolli R, Canty Jr JM, Du X-J, Frangogiannis NG, Frantz S, et al. Guidelines for experimental models of myocardial ischemia and infarction. Am J Physiol Heart Circ Physiol. (2018) 314:H812–38. doi: 10.1152/ajpheart.00335.2017
55. Yardley CP, Hilton SM. The hypothalamic and brainstem areas from which the cardiovascular and behavioural components of the defence reaction are elicited in the rat. J Auton Nerv Syst. (1986) 15:227–44. doi: 10.1016/0165-1838(86)90066-4
56. Kirihara Y, Takechi M, Kurosaki K, Kobayashi Y, Saito Y, Takeuchi T. Effects of an anesthetic mixture of medetomidine, midazolam, and butorphanol in rats—strain difference and antagonism by atipamezole. Exp Anim. (2016) 65:27–36. doi: 10.1538/expanim.15-0036
57. Blaudszun G, Lysakowski C, Elia N, Tramer MR. Effect of perioperative systemic α2 agonists on postoperative morphine consumption and pain intensity: systematic review and meta-analysis of randomized controlled trials. J Am Soc Anesthesiol. (2012) 116:1312–22. doi: 10.1097/ALN.0b013e31825681cb
58. Barrasa JLM, Rodriguez NS, Rodríguez-Pérez JC, Hidalgo AC, Garcia AT, Camarillo JAI, et al. Electrocardiographic changes in rats undergoing thoracic surgery under combined parenteral anesthesia. Lab Anim (NY). (2008) 37:469–74. doi: 10.1038/laban1008-469
59. Scheer P, Sverakova V, Doubek J, Janeckova K, Uhrikova I, Svoboda P. Basic values of M-mode echocardiographic parameters of the left ventricle in outbreed Wistar rats. Vet Med. (2012) 57:42–52. doi: 10.17221/4971-VETMED
60. Wasmeier GH, Melnychenko I, Voigt J-U, Zimmermann WH, Eschenhagen T, Schineis N, et al. Reproducibility of transthoracic echocardiography in small animals using clinical equipment. Coron Artery Dis. (2007) 18:283–91. doi: 10.1097/MCA.0b013e3280d5a7e3
61. Slama M, Susic D, Varagic J, Ahn J, Frohlich ED. Echocardiographic measurement of cardiac output in rats. Am J Physiol Heart Circ Physiol. (2003) 284:H691–7. doi: 10.1152/ajpheart.00653.2002
62. Miranda A. Costa-e-Sousa RH, Werneck-de-Castro JPS, Mattos EC, Olivares EL, Ribeiro VP, Silva MG, Goldenberg R, Campos-de-Carvalho AC. Time course of echocardiographic and electrocardiographic parameters in myocardial infarct in rats. An Acad Bras Cienc. (2007) 79:639–48. doi: 10.1590/S0001-37652007000400006
63. Chen Y-F, Weltman NY Li X, Youmans S, Krause D, Gerdes AM. Improvement of left ventricular remodeling after myocardial infarction with eight weeks L-thyroxine treatment in rats. J Transl Med. (2013) 11:1–10. doi: 10.1186/1479-5876-11-40
64. Holinski S, Knebel F, Heinze G, Konertz W, Baumann G, Borges AC. Noninvasive monitoring of cardiac function in a chronic ischemic heart failure model in the rat: assessment with tissue Doppler and non-Doppler 2D strain echocardiography. Cardiovasc Ultrasound. (2011) 9:1–6. doi: 10.1186/1476-7120-9-15
65. Johns TNP, Olson BJ. Experimental myocardial infarction: I. A method of coronary occlusion in small animals. Ann Surg. (1954) 140:675. doi: 10.1097/00000658-195411000-00006
66. Thomas D, Ferrari VA, Janik M, Kim DH, Pickup S, Glickson JD, et al. Quantitative assessment of regional myocardial function in a rat model of myocardial infarction using tagged MRI. Mag Res Mat Physics Biol Med. (2004) 17:179–87. doi: 10.1007/s10334-004-0051-y
67. MIURA T, DOWNEY JM OOIWA H, OGAWA S, ADACHI T, NOTO T, SHIZUKUDA Y, et al. Progression of myocardial infarction in a collateral flow deficient species. Jpn Heart J. (1989) 30:695–708. doi: 10.1536/ihj.30.695
68. Pich S, Klein HH, Lindert S, Nebendahl K, Kreuzer H. Cell death in ischemic, re-perfused porcine hearts: a histochemical and functional study. Basic Res Cardiol. (1988) 83:550–9. doi: 10.1007/BF01906684
69. Hedström E, Engblom H, Frogner F, Åström-Olsson K, Öhlin H, Jovinge S, et al. Infarct evolution in man studied in patients with first-time coronary occlusion in comparison to different species-implications for assessment of myocardial salvage. J Cardiovasc Mag Res. (2009) 11:1–10. doi: 10.1186/1532-429X-11-38
Keywords: myocardial infarction, rat, MMB, atipamezole, anesthesia, ECG, echocardiography
Citation: Farag A, Mandour AS, Hamabe L, Yoshida T, Shimada K and Tanaka R (2022) Novel protocol to establish the myocardial infarction model in rats using a combination of medetomidine-midazolam-butorphanol (MMB) and atipamezole. Front. Vet. Sci. 9:1064836. doi: 10.3389/fvets.2022.1064836
Received: 08 October 2022; Accepted: 14 November 2022;
Published: 05 December 2022.
Edited by:
Muhammad Saqib, University of Agriculture, Faisalabad, PakistanReviewed by:
Muhammad Athar, Banfield Pet Hospital, United StatesJean-Claude Desfontis, Ecole Nationale Vétérinaire Agroalimentaire et de l'Alimentation, France
Copyright © 2022 Farag, Mandour, Hamabe, Yoshida, Shimada and Tanaka. This is an open-access article distributed under the terms of the Creative Commons Attribution License (CC BY). The use, distribution or reproduction in other forums is permitted, provided the original author(s) and the copyright owner(s) are credited and that the original publication in this journal is cited, in accordance with accepted academic practice. No use, distribution or reproduction is permitted which does not comply with these terms.
*Correspondence: Ahmed Farag, YWhtZWRmYXJhZzkzMzFAZ21haWwuY29t; Ahmed S. Mandour, ZHJfbWFuZG91ckB2ZXQuc3Vlei5lZHUuZWc=; Ryou Tanaka, cnlvQHZldC5uZS5qcA==